Editorial: Spatial constraints on multiple dimensions of biodiversity
- 1Department of Life, Health and Environmental Sciences, University of L’Aquila, L’Aquila, Italy
- 2Department of Biological Sciences, Virginia Tech, Blacksburg, VA, United States
- 3Department of Geography & Environmental Systems, University of Maryland, Baltimore, MD, United States
Editorial on the Research Topic
Spatial constraints on multiple dimensions of biodiversity
Earth’s biological diversity is investigated, in ecological research, relying on a broad spectrum of measures, including species richness at local-to-landscape scales (i.e., α and γ diversity), compositional dissimilarity between biotic communities (i.e., β diversity), and amount and complexity of interspecific links within food-webs (Pereira et al., 2013; Gaüzère et al., 2022). All these complementary measures change in response to spatially structured environmental gradients, anthropogenic disturbances, and large-scale climatic and geologic shifts. However, the relative contribution of these factors to spatio-temporal variation in biodiversity largely varies based on the specific ecosystems analyzed and the spatial scales over which observations are taken (Keil et al., 2012; Galiana et al., 2021). Disentangling the drivers behind observed biodiversity patterns at the spatial scale of interest has become more feasible with recent theoretical and practical advances in ecological analyses. The latter include, among others: virtual simulations of species’ niches, dispersal processes and biotic interactions (Zurell et al., 2010; O’Sullivan et al., 2021); Distance-decay and Generalized Dissimilarity Models targeting β diversity (Brown and Swan, 2010; Gómez-Rodríguez and Baselga, 2018; Mokany et al., 2022); and Habitat Suitability and Landscape Connectivity Models highlighting the environmental factors which shape the species’ realized niches and possibilities for dispersal (Thuiller et al., 2009; Huang et al., 2020; Cerasoli et al., 2021).
In this context, we launched the Research Topic entitled “Spatial Constraints on Multiple Dimensions of Biodiversity” to provide space for researchers to showcase novel evidence about how different biodiversity features vary along spatial gradients associated with environmental, anthropogenic, and biotic factors. The five papers published herein span virtual landscapes, riverine and groundwater ecosystems, and terrestrial habitats (Figure 1).
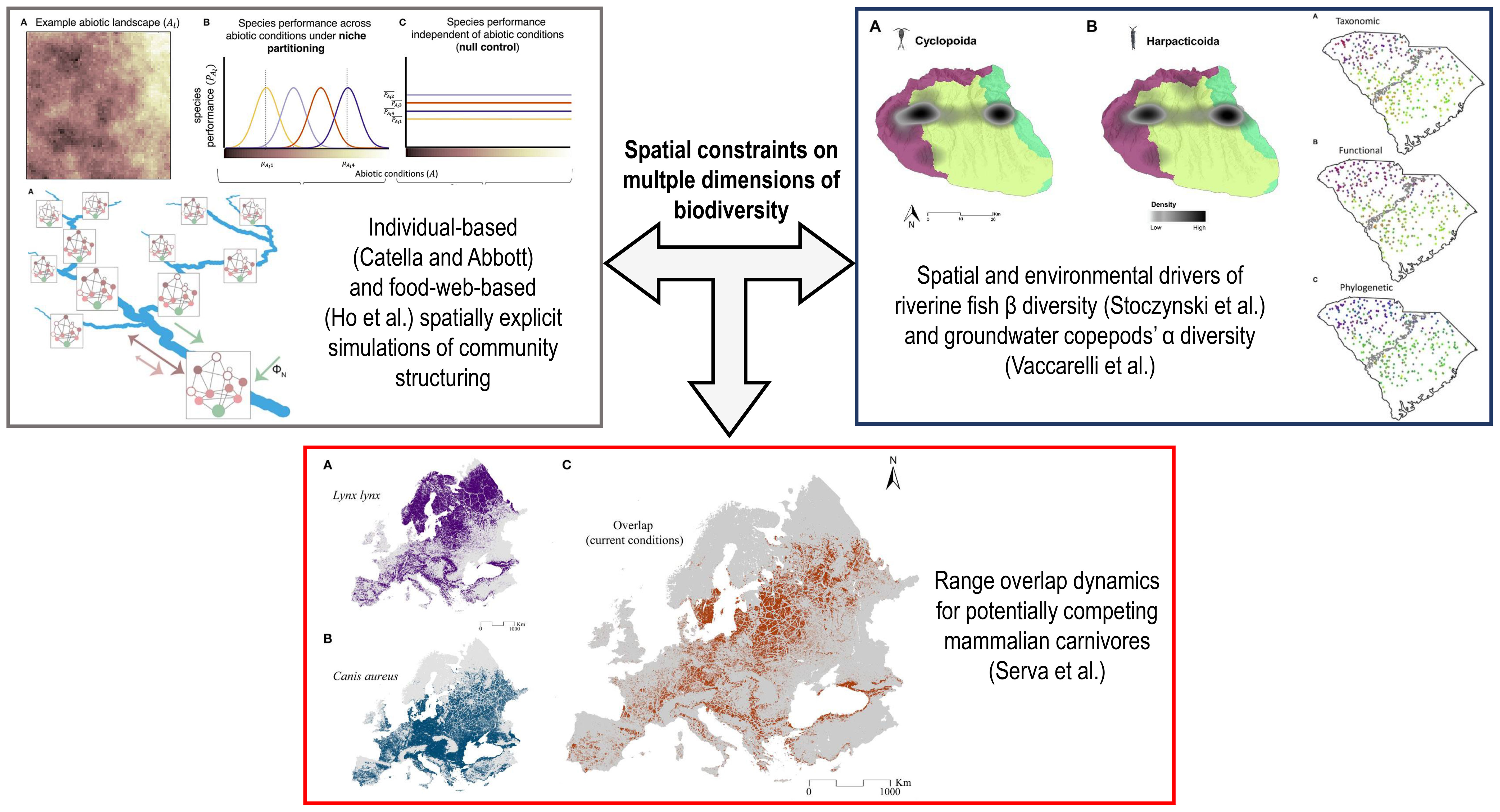
Figure 1 Schematic representation of how the five papers published within the Research Topic “Spatial Constraints on Multiple Dimensions of Biodiversity” explored the spatial and environmental drivers shaping biodiversity within simulated and real landscapes. Plots and maps within each colored rectangle are extracted from the papers cited therein.
Catella and Abbott used a spatially explicit, individual-based model to simulate virtual landscapes with varying degrees of environmental heterogeneity and spatial autocorrelation, over which virtual plant communities structured themselves according to two distinct pathways: (i) environmental factors influencing species’ persistence probability during the germination phase, thus shaping initial densities; and (ii) the same factors affecting the strength of interspecific interactions after germination occurs, thus influencing species’ persistence later on during their life cycle. The authors showed that higher landscape heterogeneity increased species richness and interspecific competitive balance when the environmental gradients affected germination probability. At the same time, the same did not occur when such gradients only affected biotic interactions. However, species richness also decreased within this second pathway in simulations where overall landscape heterogeneity was intentionally lowered. This suggests that different heterogeneity-diversity relationships can emerge when species respond to environmental pressures during distinct life stages.
Ho et al. simulated a virtual river network composed of 236 reaches, classified as high-elevation headwaters, mid-positioned reaches, lowland headwaters, or downstream reaches. Within each node (i.e., reach), local food webs were simulated based on a generalized Lotka-Volterra model where the consumer-resource relationships depended on body size and trophic connectance. In contrast, nutrient availability depended on hydrographic parameters and node position within the network. These local food webs were integrated into different realizations of a meta-food-web by simulating downward nutrient fluxes and bidirectional species’ dispersal. Within this spatial food-web (SFW) model, predicted species richness and food-web metrics were compared among the four classes of river reaches. Further, local food web metrics were regressed against the distance of the reaches from the river outlet and against drainage area. Finally, outputs from the SFW model were compared to those from three different null models. Modeled species richness was high, driven by high nutrient availability, in lowland headwaters and downstream reaches, where food webs also showed high modularity and link density and low interspecific overlap in trophic niches. Additionally, changes in how nutrient availability and species dispersal were simulated between the SFW and the null models led to different emerging meta-food-web properties, suggesting that riverine metacommunities are shaped by the synergistic effects of hydrographic structure, trophic interactions, nutrient fluxes, and organism displacement.
These findings were complemented by those from Stoczynski et al., who analyzed fish abundance data across 350 sites spanning the entire South Carolina state, four distinct watersheds, and two ecoregions (i.e., upstate versus lowlands). The two ecoregions were defined by a geomorphic break corresponding to the maximum inland extent of sea waters during the Cretaceous period. The authors computed taxonomic, phylogenetic, and functional β diversity between sites according to the three metacommunity delineations (i.e., whole state, ecoregions, or single watersheds), then assessing how much variation was explained within each setting by natural environmental (e.g., dissolved oxygen), anthropogenic (e.g., dam density) and purely spatial (i.e., vectors from Principal Coordinate Neighbor Matrices) variables. Taxonomic diversity was generally higher than phylogenetic and functional diversities, and the use of biologically relevant anthropogenic variables increased explained variation. Further, the relative weight of the three classes of variables varied according to the chosen metacommunity delineation, indicating that methodological choices influence our capability of explaining β diversity patterns in riverine networks.
In a different aquatic system, groundwater of 52 karst caves from northern Italy, Vaccarelli et al. investigated the α diversity of copepods (Crustacea Copepoda), its climatic and geologic correlates, and its spatial patterns. They found that overall species richness, richness of single orders (i.e., Cyclopoida versus Harpacticoida) and that of obligate versus non-obligate groundwater dwellers, was explained by a mix of factors, particularly the drainage basin the single caves belong to, their lithology, and surface temperature variability. The authors argued that drainage basin and lithology represent historical factors shaping underground habitat heterogeneity and possible dispersal barriers, while considerable above-ground thermal variations may favor the colonization of non-obligate groundwater dwellers from surface waters due to their generally wider thermal niche compared to obligate groundwater species. Groundwater biodiversity is thus sensitive to spatial features of karst systems, their hydrogeology, and surface climate, highlighting the need for further research to appropriately preserve it.
Finally, Serva et al. investigated the potential range overlap in Europe for an iconic predator, the Eurasian lynx, and its potential kleptoparasite, the golden jackal, which is in rapid expansion. The authors took advantage of climatic, topographic, anthropogenic (e.g., built-up areas), and habitat-related (e.g., forest cover) variables to model habitat suitability across Europe for these two carnivores under current conditions and various future scenarios delineated by different Shared Socio-Economic Pathways (SSPs). Further, the authors estimated the possible increase in range overlap between these species and the Eurasian wolf, one of their strongest competitors. Outputs of their modelling exercise provided useful information about which European regions could host broader coexistence in the future among these three predators and thus possible novel biotic interactions.
In conclusion, the papers published in this Research Topic expand current understanding about the spatial – and associated environmental – constraints that ecological systems face at various scales, paving the way to future studies aiming at effectively deploying the gained ecological knowledge to protect biodiversity.
Author contributions
FC: Conceptualization, Visualization, Writing – original draft, Writing – review & editing. BB: Conceptualization, Writing – review & editing. CS: Conceptualization, Writing – review & editing.
Conflict of interest
The authors declare that the research was conducted in the absence of any commercial or financial relationships that could be construed as a potential conflict of interest.
The author(s) declared that they were an editorial board member of Frontiers, at the time of submission. This had no impact on the peer review process and the final decision.
Publisher’s note
All claims expressed in this article are solely those of the authors and do not necessarily represent those of their affiliated organizations, or those of the publisher, the editors and the reviewers. Any product that may be evaluated in this article, or claim that may be made by its manufacturer, is not guaranteed or endorsed by the publisher.
References
Brown B. L., Swan C. M. (2010). Dendritic network structure constrains metacommunity properties in riverine ecosystems. J. Anim. Ecol. 79, 571–580. doi: 10.1111/j.1365-2656.2010.01668.x
Cerasoli F., Besnard A., Marchand M.-A., D’Alessandro P., Iannella M., Biondi M. (2021). Determinants of habitat suitability models transferability across geographically disjunct populations: Insights from Vipera ursinii ursinii. Ecol. Evol. 11, 3991–4011. doi: 10.1002/ece3.7294
Galiana N., Barros C., Braga J., Ficetola G. F., Maiorano L., Thuiller W., et al. (2021). The spatial scaling of food web structure across European biogeographical regions. Ecography 44, 653–664. doi: 10.1111/ecog.05229
Gaüzère P., O’Connor L., Botella C., Poggiato G., Münkemüller T., Pollock L. J., et al. (2022). The diversity of biotic interactions complements functional and phylogenetic facets of biodiversity. Curr. Biol. 32, 2093–2100. doi: 10.1111/j.1365-2745.2011.01908.x
Gómez-Rodríguez C., Baselga A. (2018). Variation among European beetle taxa in patterns of distance decay of similarity suggests a major role of dispersal processes. Ecography 41, 1825–1834. doi: 10.1111/ecog.03693
Huang J. L., Andrello M., Martensen A. C., Saura S., Liu D. F., He J. H., et al. (2020). Importance of spatio–temporal connectivity to maintain species experiencing range shifts. Ecography 43, 591–603. doi: 10.1111/ecog.04716
Keil P., Schweiger O., Kühn I., Kunin W. E., Kuussaari M., Settele J., et al. (2012). Patterns of beta diversity in Europe: the role of climate, land cover and distance across scales. J. Biogeography 39, 1473–1486. doi: 10.1111/j.1365-2699.2012.02701.x
Mokany K., Ware C., Woolley S. N., Ferrier S., Fitzpatrick M. C. (2022). A working guide to harnessing generalized dissimilarity modelling for biodiversity analysis and conservation assessment. Global Ecol. Biogeography 31, 802–821. doi: 10.1111/geb.13510
O’Sullivan J. D., Terry J. C. D., Rossberg A. G. (2021). Intrinsic ecological dynamics drive biodiversity turnover in model metacommunities. Nat. Commun. 12, 3627. doi: 10.1038/s41467-021-23769-7
Pereira H. M., Ferrier S., Walters M., Geller G. N., Jongman R. H., Scholes G. R. J., et al. (2013). Essential biodiversity variables. Science 339, 277–278. doi: 10.1126/science.1229931
Thuiller W., Lafourcade B., Engler R., Araújo M. B. (2009). BIOMOD–a platform for ensemble forecasting of species distributions. Ecography 32, 369–373. doi: 10.1111/j.1600-0587.2008.05742.x
Keywords: biodiversity, ecological simulations, community ecology, beta diversity (β), spatial ecology, riverine networks, groundwater, mammalian carnivores
Citation: Cerasoli F, Brown BL and Swan C (2024) Editorial: Spatial constraints on multiple dimensions of biodiversity. Front. Ecol. Evol. 12:1371970. doi: 10.3389/fevo.2024.1371970
Received: 17 January 2024; Accepted: 01 February 2024;
Published: 12 February 2024.
Edited and Reviewed by:
Peter Convey, British Antarctic Survey (BAS), United KingdomCopyright © 2024 Cerasoli, Brown and Swan. This is an open-access article distributed under the terms of the Creative Commons Attribution License (CC BY). The use, distribution or reproduction in other forums is permitted, provided the original author(s) and the copyright owner(s) are credited and that the original publication in this journal is cited, in accordance with accepted academic practice. No use, distribution or reproduction is permitted which does not comply with these terms.
*Correspondence: Francesco Cerasoli, francesco.cerasoli@univaq.it