Features and driving factors of microbial metabolic limitation in mountain ecosystems in arid areas: A case study on the Helan Mountains, Northwest China
- 1Breeding Base for State Key Laboratory of Land Degradation and Ecological Restoration in Northwest China, Ningxia University, Yinchuan, China
- 2Ministry of Education Key Laboratory for Restoration and Reconstruction of Degraded Ecosystem in Northwest China, Ningxia University, Yinchuan, China
- 3School of Ecology and Environment, Ningxia University, Yinchuan, China
- 4Research Institute of Forestry, Chinese Academy of Forestry, Beijing, China
- 5School of Agriculture, Ningxia University, Yinchuan, China
- 6Northwest Institute of Eco-Environment and Resources, Chinese Academy of Sciences, Lanzhou, China
Insights into what limits the growth of soil microorganisms in mountain ecosystems increase our understanding of microbial functions and processes. Although the distribution pattern of soil microorganisms in mountain ecosystems has been widely studied, their role in biogeochemical cycles along elevation gradients of mountain ecosystems in arid regions is poorly understood. In this study we analyzed the soil physicochemical properties, soil microbial community structure, extracellular enzymatic activities, ecoenzymatic stoichiometry, microbial metabolism, and their relationships along the 1,300–2,500 m elevational gradient of the Helan Mountains, northwest China. The results showed that the total microbial biomass and its components did not significantly vary with elevation. The GP:GN (gram-positive: gram-negative bacteria) ratios at low elevations were higher than those at the mid and high elevations, indicating enrichment of oligotrophic bacteria at low elevations. The five extracellular enzymes significantly differed with elevation gradient, while the levels of carbon (C)- and nitrogen (N)- acquiring enzymes first increased and then decreased with increasing elevations. Ecoenzymatic stoichiometry indicated that significant limitation of microbial growth by carbon (C) and phosphorus (P) levels occurred at high and medium elevations. Soil physicochemical characteristics, microbial community composition, and ecoenzymatic activities accounted for 43.94 and 22.21% of the microbial C and P restriction, respectively. Our study suggests that mountain ecosystems with high organic C storage possess abundant microbial populations limited by relative C and P. The study also provides important insights linking microbial metabolisms to the environmental gradients in arid mountain ecosystems.
Introduction
Mountain ecosystems, especially in arid and semiarid areas, are vulnerable to global climate change (Zeng et al., 2020; Wu et al., 2021). Unlike fertilized agroecosystems (Carbonetto et al., 2014), mountain ecosystems endure cumulations of organic substances characterized by continuous depletion of soil nutrients, particularly nitrogen (N) and phosphorus (P) (Cui et al., 2018). Since most microbial growth is limited by carbon (C), N, or P levels (Moorhead et al., 2016), low nutrient conditions lead to nutrient competition between microorganisms and plants (Ouyang et al., 2016), which may alter the functions of natural ecosystems and accelerate vegetation degeneration (Huang et al., 2016). Although previous studies have shown that microorganisms respond to elevation-induced temperature change, directly affected by their soil nutrient uptake (Cui et al., 2019a; Nottingham et al., 2019), the mechanisms by which elevation gradients affect microbial nutrient limitation and metabolism are still unclear. Thus, understanding how microbial processes respond to elevation-induced temperature change is important for predicting the impact of climate change on microbial nutrient acquisition. In this sense, it is pivotal to explore the nutrient limitations of microbial communities and elucidate the mechanisms of nutrient (C, N, and P) cycling in elevations of mountain ecosystems.
Microbes enzymatically break down organic substances (Cui et al., 2019a) for growth and respiration (Manzoni, 2017). Since different microbes have different energy and growth requirements, they are susceptible to soil nutrient status (Lamb et al., 2011; Li et al., 2019). Soil extracellular enzymes are essential for organic matter decomposition (Peng and Wang, 2016), and their activities (EEA) are critical in microbial metabolism (Duan et al., 2018). Hence, β-glucosidase (βG), cellobiosidase (CBH), β-N-acetylglucosaminidase (NAG), L-leucine aminopeptidase (LAP), and alkaline phosphatase (AP) activities are widely used to assess the acquisition and allocation of C, N, and P by microorganisms (Deng et al., 2019). When nutrient availability changes, microbes typically acquire energy and nutrients from the soil by altering the allocation of these enzyme activities. Assessing the responses of soil extracellular enzymes to elevation change may improve future predictions of resource availability and climate change in mountain ecosystems (Nottingham et al., 2016). Previous studies showed that vegetation and environmental factors change drastically with elevation and that EEA and their ratios vary along elevation gradients (Zuo et al., 2018; Zhang et al., 2022). CBH, BG, and NAG activities decreased, while LAP increased with the increasing mean annual temperature (Feyissa et al., 2022). These studies suggest that the metabolic activities of soil microbes may be strictly regulated by temperature and nutrient availability; however, few studies have explored the EEA pattern along elevational gradients in arid climate zone. Therefore, investigating the altitudinal pattern of EEA would help identify the key drivers of the dynamics of microbial metabolic activities.
Extracellular enzyme stoichiometry (EES) represents the biogeochemical equilibrium between the metabolism and nutrition demands of microbial assemblages (Hill et al., 2012). EES determines the associations between microbial nutrition requirements and nutrient supply (Sinsabaugh and Shah, 2012), making it an index of microbial nutrition acquisition capacity (Cui et al., 2021). EES has also been employed in evaluating the factors limiting soil microbial metabolism, as reflected by C, N, and P levels (Chen et al., 2018; Jiang et al., 2019; Li et al., 2020). For example, previous studies showed that soil microbes experienced N limitation in Qinling Mountains, which significantly increased with elevation (Zhang et al., 2022). Furthermore, Yu et al. (2022) observed that C and P significantly limited microbial metabolism with increasing elevation in the alpine grassland ecosystem, eastern Tibetan Plateau, under simulated warming. Moreover, EES and soil microbial metabolism are largely governed by temperature, precipitation, soil physiochemical parameters, and soil microbial properties (Burns et al., 2013; Zhang et al., 2019b). Zuo et al. (2018) found that soil nutrient alters EES indirectly through microbial biomass at low elevation, while soil moisture influences soil EES via pH at the mid-elevation. Ren et al. (2021) found that the elevation-dependent differences in the microbial community and enzyme activity between the rhizosphere and bulk soil were affected mainly by climatic factors and soil properties. However, our current understanding of microbial metabolism patterns and drivers along elevation gradients in mountain ecosystems is limited due to few studies and inconsistent results. Therefore, it is necessary to explore the microbial resource limitations and metabolic processes to reveal their underlying mechanisms along elevation gradients.
The Helan Mountains, located in northwest China, lie at the boundary between desert and temperate grassland regions. The soil characteristics of the Helan Mountains change greatly along the elevation gradient because of the diverse soil evolution and regional climates. Depending on elevation, these mountains have five vegetation types: desert grassland, sparse mountainous shrubs, mountainous warm temperate coniferous forests, mountainous mixed forests, and mountainous cold temperate coniferous forests (Wu et al., 2021). The vegetation diversity and chemical structure of organic carbon over certain elevations in the Helan Mountains have been studied (Zhu et al., 2009; Wu et al., 2021), yet little is known about the soil ecoenzymatic stoichiometry along its elevation gradient. Therefore, we determined the nutrient (N and P) constraints facing microbial communities in the five vegetation types along an elevation gradient on the eastern slope of the Helan Mountains. Microbial metabolism limitations in the Helan Mountains were investigated via ecoenzymatic stoichiometry analysis. Since temperature decreases with increasing altitude (Baldos et al., 2015), we hypothesized that (1) microbial community structure and enzyme activities in the soil differ under different elevation gradients; and (2) microbial metabolic limitation would increase with altitude, especially microbial C metabolism which is more significantly limited at high elevations than at lower ones. The main objectives of this study were (1) to identify the patterns of microbial metabolism constraints along elevation gradients on the Helan Mountains, and (2) to reveal the driving factors of microbial metabolism constraints on the Helan Mountains. Our findings highlighted the impact of elevation on microbial metabolic limitation and provided insights for soil nutrient management in mountain ecosystems in arid regions.
Materials and methods
Study site and soil sampling
The study sites were located in the Helan Mountains (38°27′–39°30′N, 105°41′–106°41′E). Mean annual temperature and precipitation were −0.8°C and 420 mm, respectively (60–80% of the rainfall occurred between June and August) (Wu et al., 2021). The vegetation varied from desert steppe to boreal coniferous forests with increasing elevation (Zeng et al., 2020; Wu et al., 2021).
In July 2020, we established six representative standard plots for each vegetation type along an elevation gradient of 1,300–2,500 m on the eastern slope of the Helan Mountains, which covered desert steppe (1,380 m), Prunus mongolica shrubs (1,650 m), Pinus tabuliformis (2,139 m), Pinus tabuliformis and Picea crassifolia (2,249 m), and Picea crassifolia (2,438 m). Plots of the same vegetation type were more than 20 m apart from the forest edge. Six plots were randomly established at each elevation gradient, measuring 1 m × 1 m in steppe desert, 10 m × 10 m in shrubs, and 20 m × 20 m in forests. The plot sizes were determined by considering the minimum community area of each vegetation type (Zhang, 2005; Zhang and Dong, 2010). Tree species, shrubs, and herbs were measured in each plot, and the species were identified (Zhang and Dong, 2010). After removing the litter layer, topsoil samples (0–20 cm) were collected from each plot. Five soil cores were randomly collected from each plot using soil anger (5 cm diameter) and mixed to obtain six composite samples. Soil samples were screened through a 2-mm mesh sieve to remove roots, stones, and other debris. Each composite sample was divided into three parts and immediately transported to the laboratory. One subsample was stored at 4°C for the analysis of extracellular enzyme activity, another one was stored at −80°C to determine phospholipid fatty acid (PLFA), and the remaining one was air-dried for the analysis of soil physicochemical properties.
Soil physicochemical properties analysis
Soil water content (SWC) was measured by mass loss after drying at 105°C for 24 h. The soil bulk density (BD) (g cm–3) was measured using a soil bulk sampler with a stainless steel cutting ring at points adjacent to the soil sampling quadrats (Deng et al., 2019). Soil pH was determined via water: soil ratios of 2.5:1 (PHSJ-4A, Leici, China), and the soil texture was determined using a laser particle size analyzer (Master-sizer 2000, Malvern, UK). SOC was determined by dichromate oxidation via titration with ferrous ammonium sulfate. The dry combustion method was used for Total N analysis using an elemental analyzer (Elementar, Vario Max CN, Germany), while Total P was measured spectrophotometrically with a continuous flow auto-analyzer (Bran Luebbe, AA3, Germany) after digestion with H2SO4–HClO4. The soil physicochemical properties are shown in Table 1.
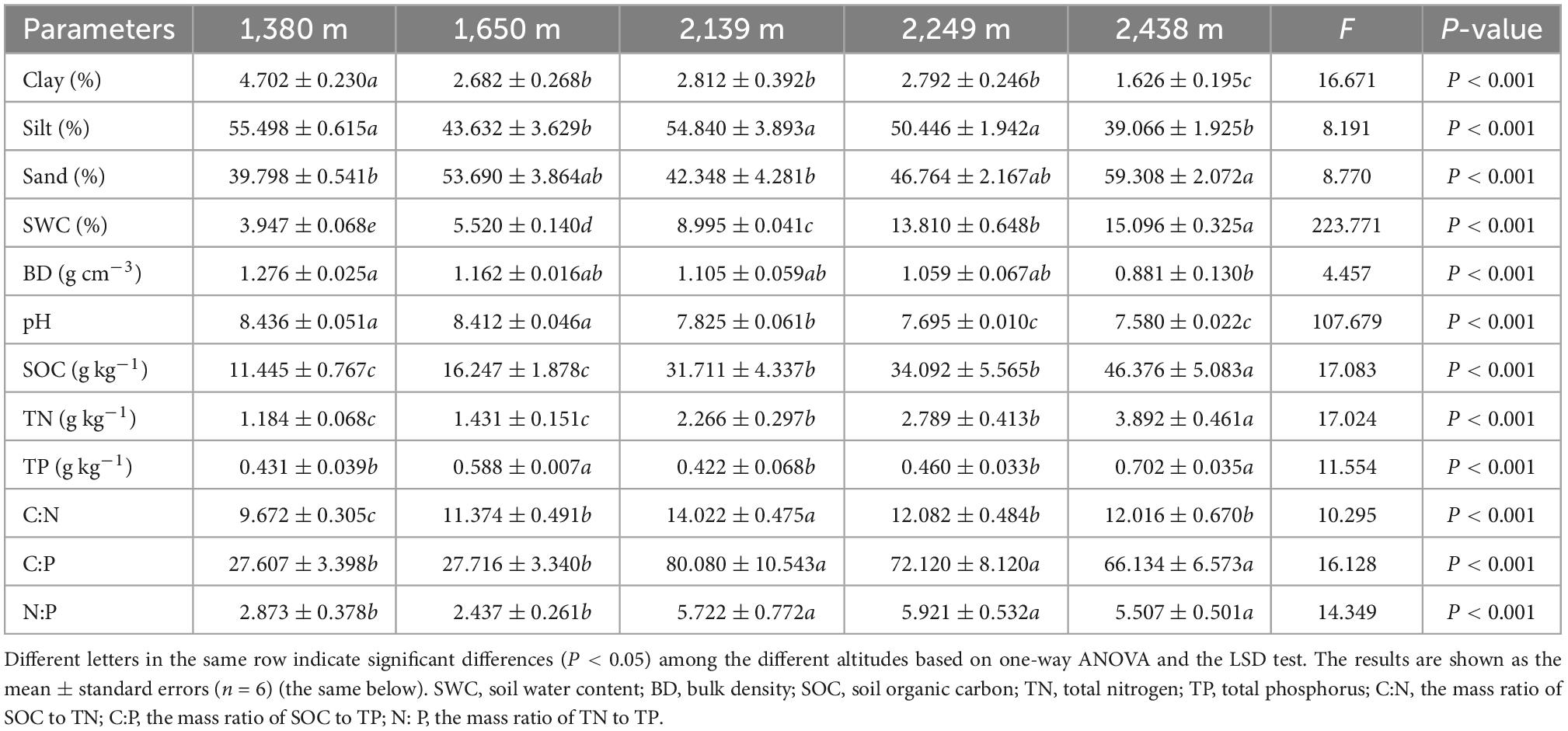
Table 1. Selected soil physicochemical properties along an elevational gradient in the Helan Mountains.
Soil microbial community properties analysis
Phospholipid fatty acids (PLFAs) were used to infer the soil microbial community structure using the modified Bligh-Dyer method (Frostegård et al., 2011). Briefly, 8 g of fresh freeze-dried soil was taken from each sample and mixed with extraction solution (chloroform, methanol, and phosphate buffer, 1:2:0.8 v/v/v). The mixture was shaken for 30 s, subjected to ultrasonic treatment for 10 min (100 Hz, 37°C), and incubated in a water bath for 30 min (37°C). The supernatant was transferred to a conical flask, and 6 mL of CHCl3 and potassium phosphate buffer was added, followed by overnight incubation. Thereafter, phospholipids in the sample were transformed into free methyl esters by alkaline methanolysis and analyzed using a gas chromatography system (GC; Hewlett-Packard 5890) with a flame ionization detector (19091B-102, Agilent, USA). The internal standard (19:0 non-adecanoic methyl ester) was used to convert the peak area into nanomoles per gram of dry soil (nmol g–1 DM). The abundance of each fatty acid was expressed as nmol g–1 dry soil and classified according to standard nomenclature (Frostegård and Bååth, 1996).
The fatty acids used to represent bacteria were 12:0, 13:0, 14:0, 15:0, 16:0, 22:0, and 24:0. The fatty acids selected to represent gram-positive (GP) bacteria were i13:0, a13:0, i14:0, a14:0, i15:0, a15:0, i17:1ω9c, and i15:1ω6c, while those representing gram-negative (GN) bacteria were 14:1ω5c, 16:1ω7cDMA, 17:1ω8c, 17:0cyclo ω7c, 19:0cyclo ω7c, 10:0 2OH, and 16:02OH. Moreover, the fatty acids 18:1ω9c, 21:0, 23:0, and 18:2w6c represented fungi, whereas 10Me17:0, 10Me 18:1ω7c, 10Me 19:1ω7c, 10Me 17:1ω7c, and 10Me 18:0 represented actinomycetes. Protozoa were represented by the fatty acids 20:4ω6c, 20:3ω6c, 20:5ω3c, and 19:3ω6c. The 16:1ω5c fatty acids represented the arbuscular mycorrhizal fungi (AMF) (Frostegård et al., 2011; Brockett et al., 2012; Liu et al., 2012).
Soil potential enzymatic activities analysis
The C- and N- acquisition enzymes of β-1,4-glucosidase (BG), β-D-cellobiosidase (CBH), β-1,4-N-acetylglucosaminidase (NAG), and L-leucine aminopeptidase (LAP) were measured using a 96-well microplate fluorometer. Briefly, for ecoenzyme extraction, 1.5 g of fresh soil was homogenized in a blender with 150 mL of sodium acetate buffer (50 mM, pH 5.0) for 1 min. Subsequently, 200 μL of the soil homogenates and 50 μL of 200 μM enzyme-substrate were added into the 96-well plates and incubated in the dark at 25°C for 2.5 h. The plates were shaken each hour during incubation to ensure the homogenization of the reaction mixtures. After incubation, 10 μL of 1 mol L–1 NaOH was added to terminate the enzyme activity. The fluorescence was measured at an excitation wavelength of 365 nm and an emission wavelength of 450 nm in the 96 well microplates to calculate enzyme activity (SynergyH1M, Biotek, USA) (Saiya-Cork et al., 2002; Sinsabaugh et al., 2008, 2009; Steinweg et al., 2012; Moorhead et al., 2016). Soil enzymatic activity was then expressed as nmol h–1 g–1 dry soil after correcting for quenching and negative controls. The alkaline phosphatase (AP) activity was measured using the method by Guan et al. (1991). The potential activities of the five enzymes are related to microbial metabolism and nutrient limitation (Table 2).

Table 2. Soil enzymes and corresponding abbreviations, substrates, and enzyme commission (EC) numbers.
Calculation of soil microbial diversity indices and resource limitation
The Shannon-Wiener (H), Brillouin diversity (B), Pielou uniformity (J), Simpson dominance (D), and Mcintosh diversity (M) indices were used to infer the microbial diversity (Magurran, 1988; Ge, 2002; Li et al., 2014).
(1) Shannon-Wiener diversity index (H)
Pi = Ni/N, where Ni represents the number of treatments, i denotes the characteristic fatty acids, and N represents the total number of characteristic fatty acids in the experiment.
(2) Brillouin diversity index (B)
where ni represents the number of individuals with the first PLFA biomarker, n2 denotes the number of individuals with the second PLFA biomarker, ni designates the number of individuals with the i-th PLFA biomarker, and N is the total number of individuals with PLFA biomarkers in all treatments.
(3) Pielou uniformity index (J)
where S represents the total PLFAs in the microbial community.
(4) Simpson dominance Index (D)
where Pi represents the proportion of i characteristic PLFAs in the total number of characteristic fatty acids in the experiment.
(5) Mcintosh diversity index (M)
where N is the total number of characteristic PLFAs.
The geometric means of soil enzyme activities (GME) for each vegetation type were calculated according to a previous study (Ma et al., 2020). The nutrient limitation was quantified by calculating the vector length and angle; using the following equations:
where H, B, J, D, and M are the five soil microbial community diversity indices. Vector length was used to describe C limitation, where x represents the relative amount of C vs. P enzymes, and y represents the relative amounts of C vs. N enzymes (Moorhead et al., 2016). Meanwhile, vector angle represented N/P limitation, while values >45° and <45° represented P and N limitation, respectively. The P limitation increases, while the N limitation decreases with the increasing vector angle (Cui et al., 2021).
Statistical analysis
Prior to statistical analyses, all dependent variables were tested for normal distribution. One-way analysis of variance (ANOVAs) with LSD (least significant difference) was used to determine the significance of differences in plant diversity index, soil physicochemical properties, PLFA biomarkers, enzyme activities, and microbial metabolic limitation at different elevations (P < 0.05). Statistical analyses were performed using SPSS 25.0 (SPSS Inc., Chicago. IL, USA). The plant diversity index of the five different vegetation types was calculated using a method by Zhang and Dong (2010). Soil C, N, and P stoichiometric ratios were calculated based on the mass of C, N, and P (Pang et al., 2018), and NMDS (non-metric multidimensional scaling) analysis of the enzyme activities was conducted at different elevations. Pearson correlation analysis was performed to identify the relationships between microorganism communities, enzyme activities, and soil physicochemical properties. The statistical analyses were performed in R (3.6.2 version), using “corrplot” packages. Moreover, linear regressions and Pearson’s tests were used to explore the relationships between soil enzymatic activity and enzymatic stoichiometry, random forest analysis was used to identify the critical factors that affected soil microbial C and N/P limitations. Graphs were generated using Origin 2018 (Origin Lab., Hampton, MA, USA).
Results
Microbial biomass and community composition
The total soil microbial biomass and its components, bacteria, fungi, actinomycetes, GP, GN, and AMF biomass did not differ with elevation (P > 0.05; Figure 1). The bacterial, fungal, and protozoa biomass first increased and then decreased with increasing elevation, whereas total microbial, actinomycetes, GP, GN, and AMF biomass decreased at low elevations (1,380–1,650 m), followed by an increase at 2,249 m, and then decrease at 2,438 m (Figure 1). Furthermore, the diversity indices of the soil microbial communities did not differ with elevation, although the GMM was higher at 2,249 m than at other elevations (Table 3). The characteristic community structure of F:B did not differ significantly with elevation (P > 0.05; Figure 2A). Conversely, the GP:GN was highest and lowest at 1,650 and 2,249 m, respectively (P < 0.05; Figure 2B), and the distribution of PLFA biomarkers differed with elevation (Figure 3). Generally, the PLFA biomarkers were more abundant at 2,249 m than at other elevations, and the dominant biomarkers were bacterial, GP, and GN PLFAs (Figure 4).
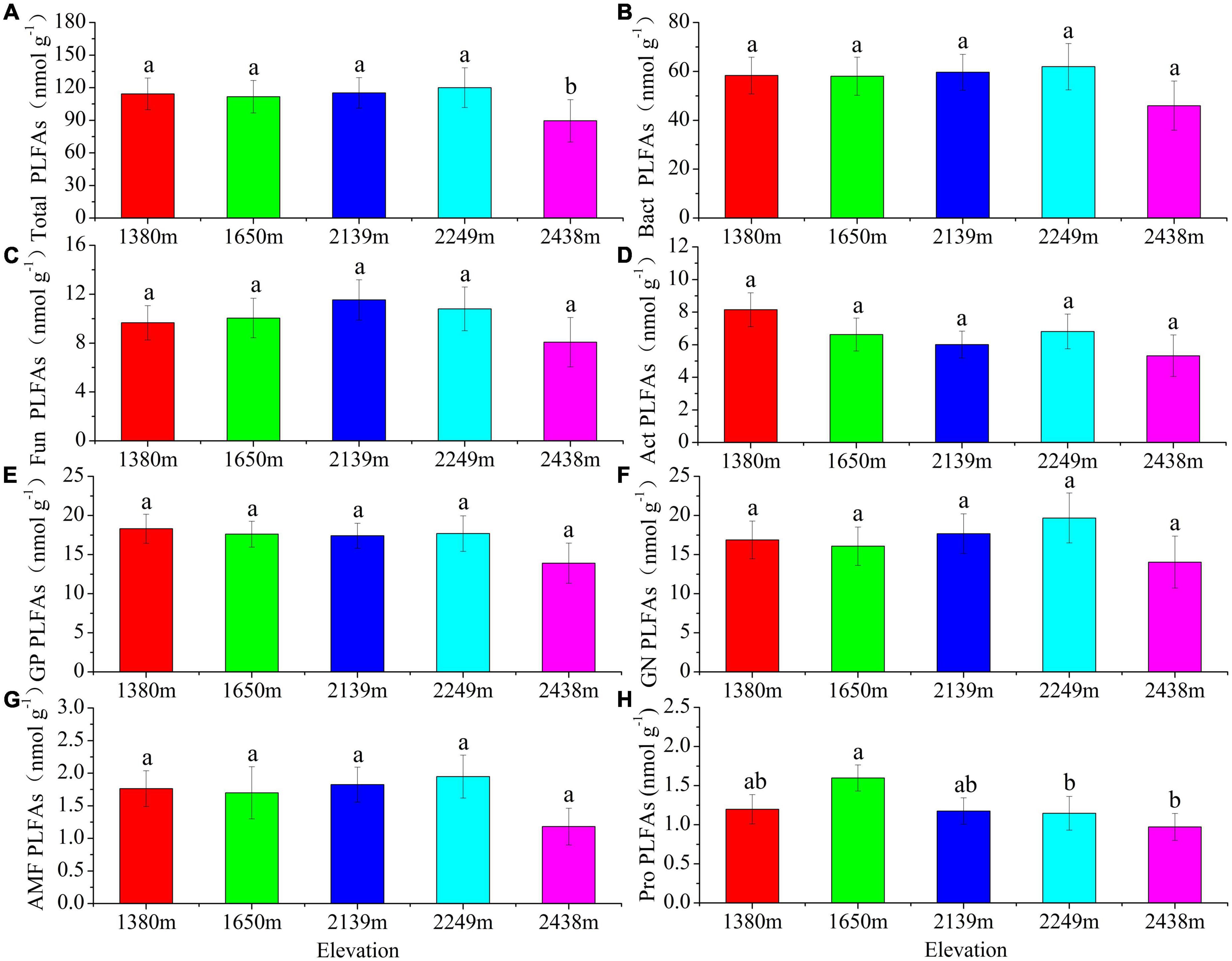
Figure 1. Concentrations of soil microbial phospholipid fatty acids (PLFAs) and biomarker ratios at different elevations. Different lowercase letters indicate statistically significant differences among the different altitude gradients (P < 0.05) based on one-way ANOVA and LSD test (n = 6). Bact, bacteria; Fun, fungi; Act, actinomycetes; GP, gram-positive bacteria; GN, gram-negative bacteria; AMF, arbuscular mycorrhizal fungi; Pro, protozoa.
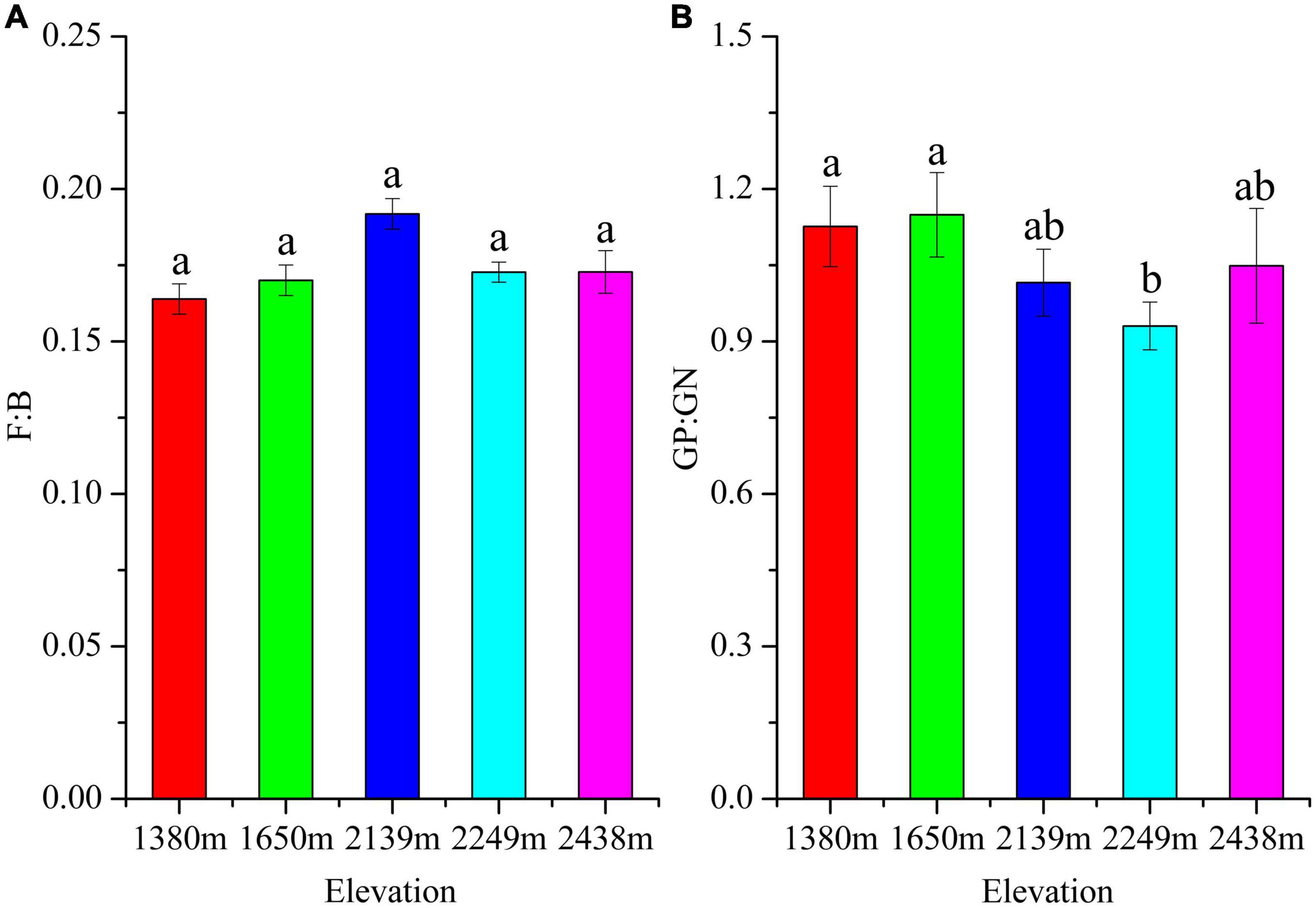
Figure 2. Structural ratios of the microbial phospholipid fatty acid (PLFA) biomarkers in soils at different elevations. The small letters show significant differences (P < 0.05) among different altitudes. GP:GN, represent the ratio of gram-positive bacteria to that of gram-negative bacteria, while F:B, denotes the ratio between fungi and bacteria.
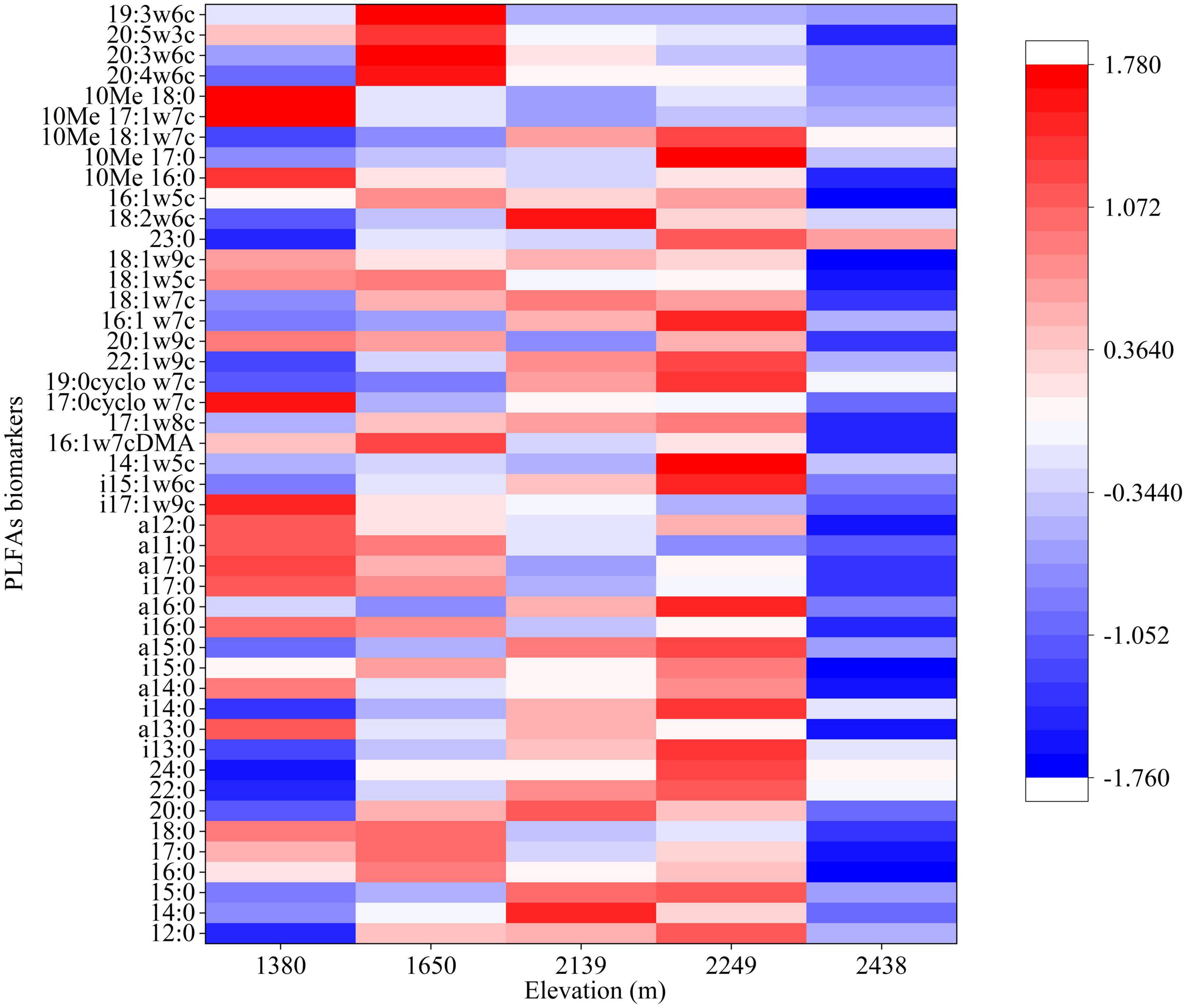
Figure 3. Mean abundance of the microbial phospholipid fatty acid (PLFA) biomarkers at different elevations.
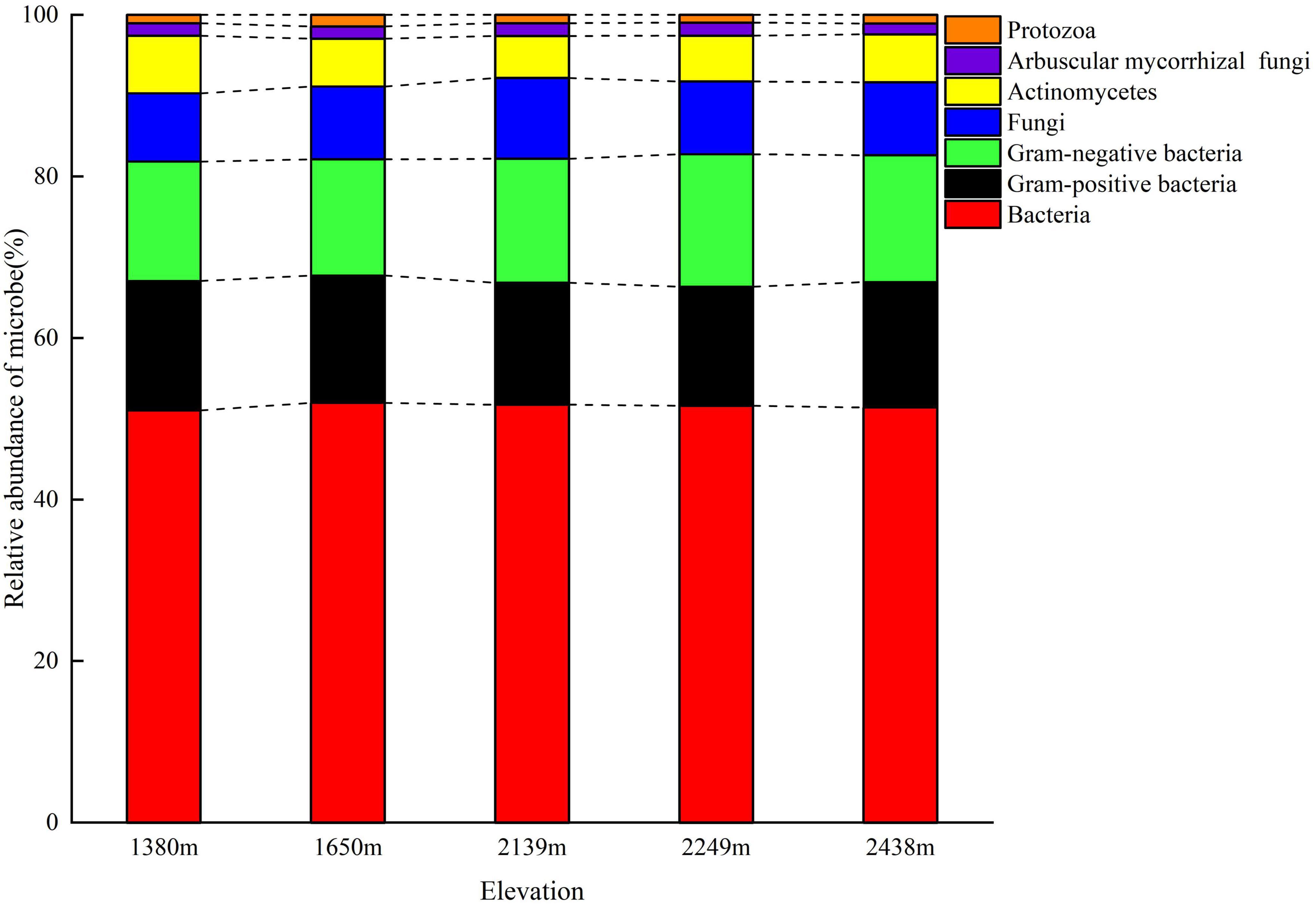
Figure 4. Relative abundance of the microbial phospholipid fatty acid (PLFA) biomarkers in the soil at different elevations.
Soil ecoenzymatic activities and stoichiometry
The five extracellular enzymes significantly differed with elevation (P < 0.05; Figures 5, 6). The (BG + CBH) were highest (150.98 n mol g SOM–1 h–1) at the 2,249 m (Figure 5A), whereas the (NAG + LAP) values were highest and lowest (80.82 and 50.62 n mol g SOM–1 h–1) at the 2,139 and 1,380 m, respectively (Figure 5B). Similarly, the AP was highest and lowest (95.73 and 62.95 n mol g SOM–1 h–1) at the 2,139 and 1,380 m, respectively (Figure 5C), and the activities of BG + CBH and NAG + LAP first increased and then decreased with increasing elevation (Figures 5A, B).
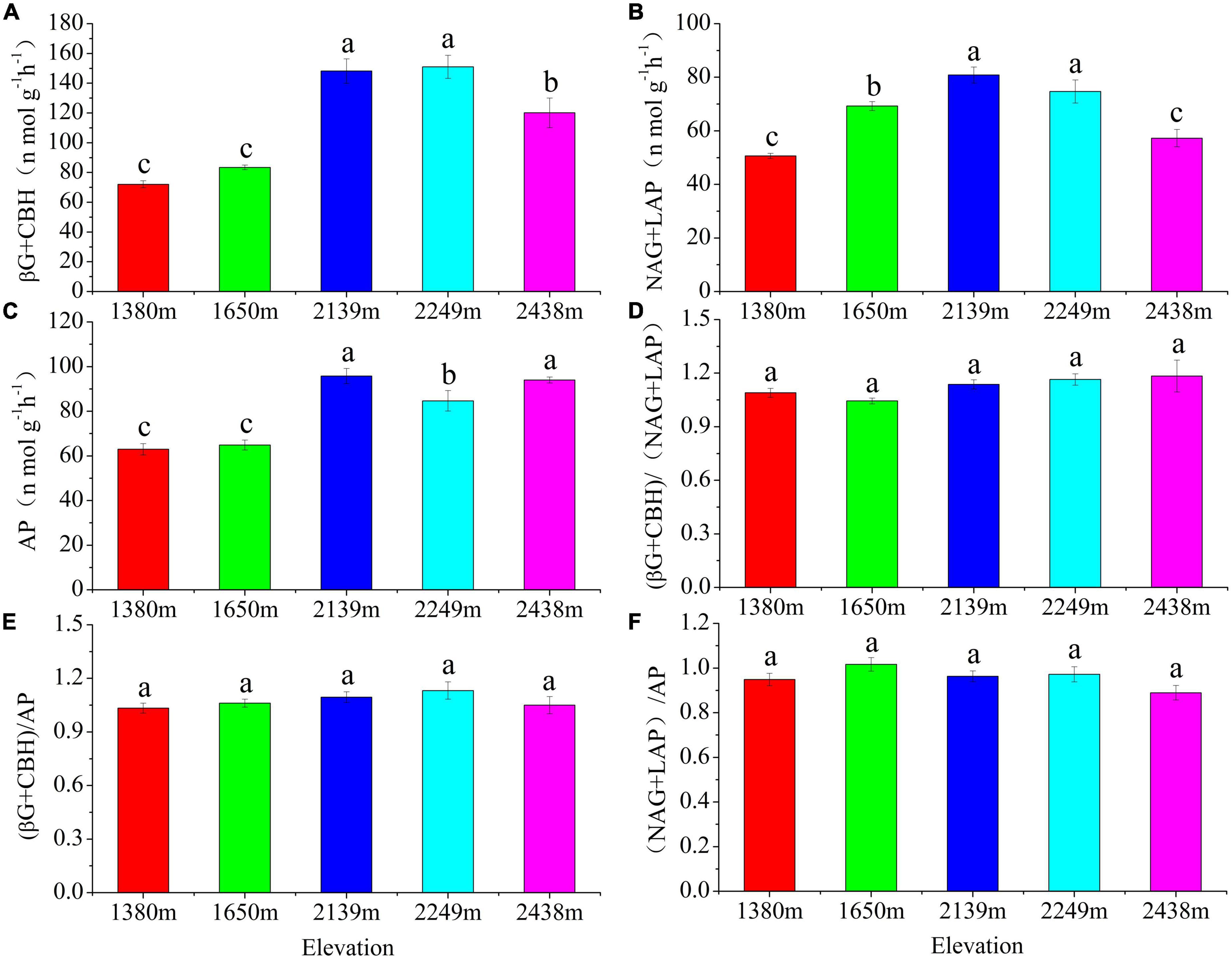
Figure 5. Variations in soil enzymatic activity and enzymatic stoichiometry at different elevations. (A) C acquiring enzymes: (BG + CBH); (B) N-acquiring enzymes: (NAG + LAP); (C) P-acquiring enzymes: AP; (D) (BG + CBH)/(NAG + LAP); (E) (BG + CBH)/AP; (F) (NAG + LAP)/AP.
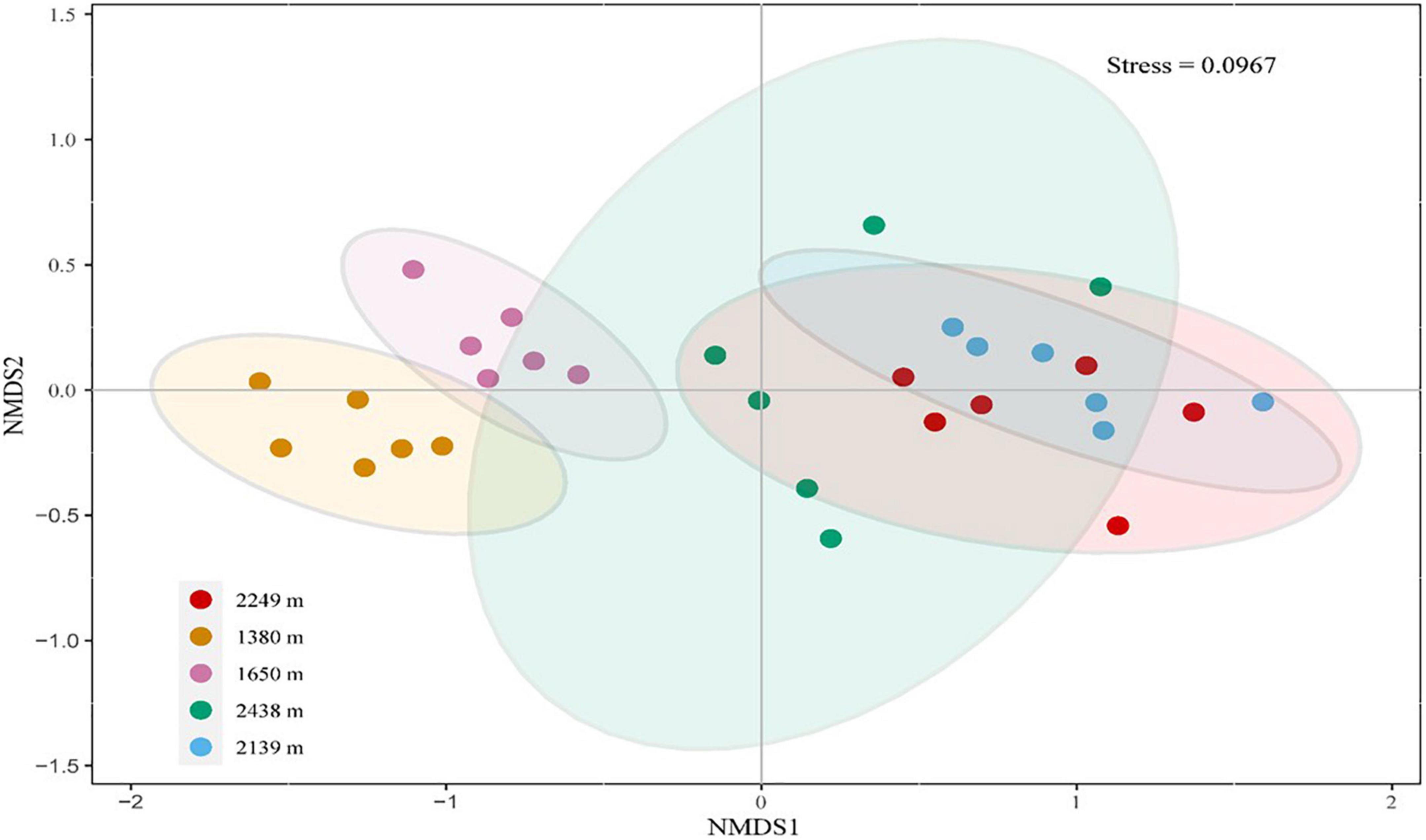
Figure 6. Non-metric multidimensional scaling (NMDS) analysis the composition of the enzyme activity at different elevations. Bray-Curtis similarity was used for the resemblance distance measure. The NMDS plots show the clustering of enzyme activities at different altitude gradients.
The ratios of BG + CBH: NAG + LAP, BG + CBH: AP, and NAG + LAP: AP did not differ significantly among the different elevations (P > 0.05; Figures 5D–F). However, the ratio between (BG + CBH): (NAG + LAP) increased with increasing elevation, peaking (1.18) at 2,438 m. The (BG + CBH): AP and (NAG + LAP): AP ratios first increased and then decreased with increasing elevation, with the highest levels (1.13 and 1.012) being observed at 2,249 and 1,650 m, respectively (Figures 5E, F).
Characteristics limitation of microbial resources
The limitation of microbial resources differed with elevation (Figure 7). All data points, except those obtained at 1,650 m, were above the line (1:1) and had vector angles > 45°, indicating P rather than N limitation (Figures 7A, C).
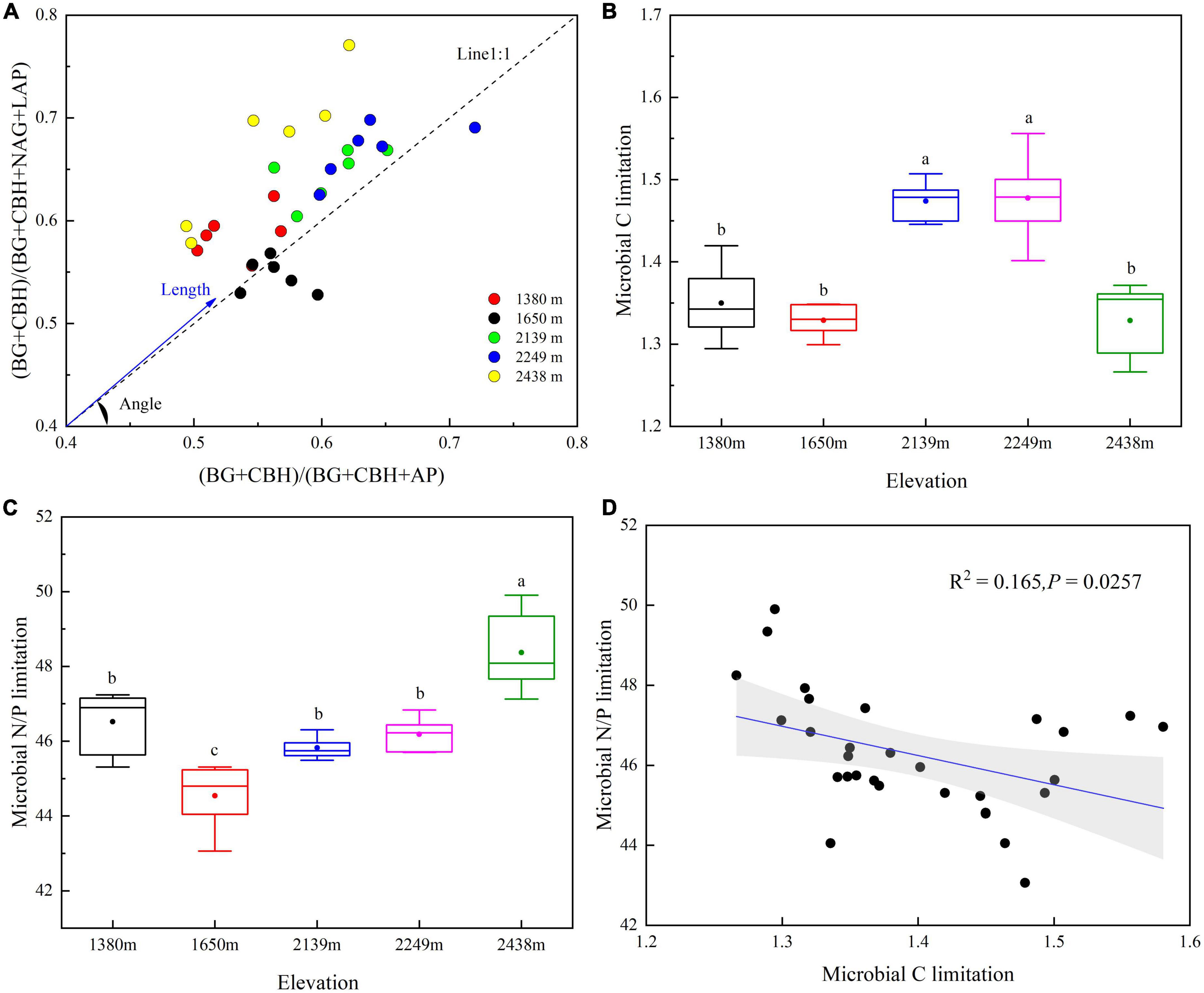
Figure 7. Extracellular enzyme stoichiometry of the relative proportions of C to N acquisition versus C to P acquisition (A), variation in soil microbial C limitation (B), soil microbial N/P limitation (C), and the linear regression relationship between soil microbial C limitation and microbial N/P limitation (D) at different elevations. BG, β-1,4-glucosidase; CBH, β-D-cellobiosidase; NAG, β-1,4-N-acetylglucosaminidase; LAP, L-leucine aminopeptidase; AP, alkaline phosphatase. Soil microbial C limitation is represented by vector length, while soil microbial N/P limitation is represented by vector angles whereby angles < 45° represent N limitation, and angles > 45° represent P limitation.
The maximum vector length at 2,249 m indicated the highest limitation of C (Figure 7B), while the maximum vector angle at 2,438 m indicated the highest limitation of P (Figure 7C). Furthermore, microbial C limitation negatively correlated with microbial N/P limitation (P < 0.05; Figure 7D).
Relationships among soil nutrients, microbial activity, and ecoenzymatic stoichiometry
The C-, N-, and P-acquiring enzymes (BG, CBH, NAG, LAP, and AP) and their stoichiometry were significantly related to soil physicochemical properties. There were significant correlations between BG and SWC, pH, and soil nutrient stoichiometry (C/N, C/P, N/P) (r = 0.483, −0.592, 0.532, 0.528, and 0.471, respectively, P < 0.05; Table 4). The CBH and AP positively correlated with SWC, SOC, TN, and soil nutrient stoichiometry (C/N, C/P, N/P) (P < 0.05; Table 4), whereas NAG positively correlated with soil nutrient stoichiometry (C/N, C/P, N/P) (r = 0.367, 0.565, and 0.557, respectively, P < 0.05; Table 4). Additionally, LAP positively correlated with C/N (r = 0.475, P < 0.05; Table 4), while the ratio between (BG + CBH) and (NAG + LAP) positively correlated with SWC, SOC, TN, C/P, and N/P (r = 0.697, 0.453, 0.418, 0.419, and 0.409, respectively, P < 0.05; Table 4).
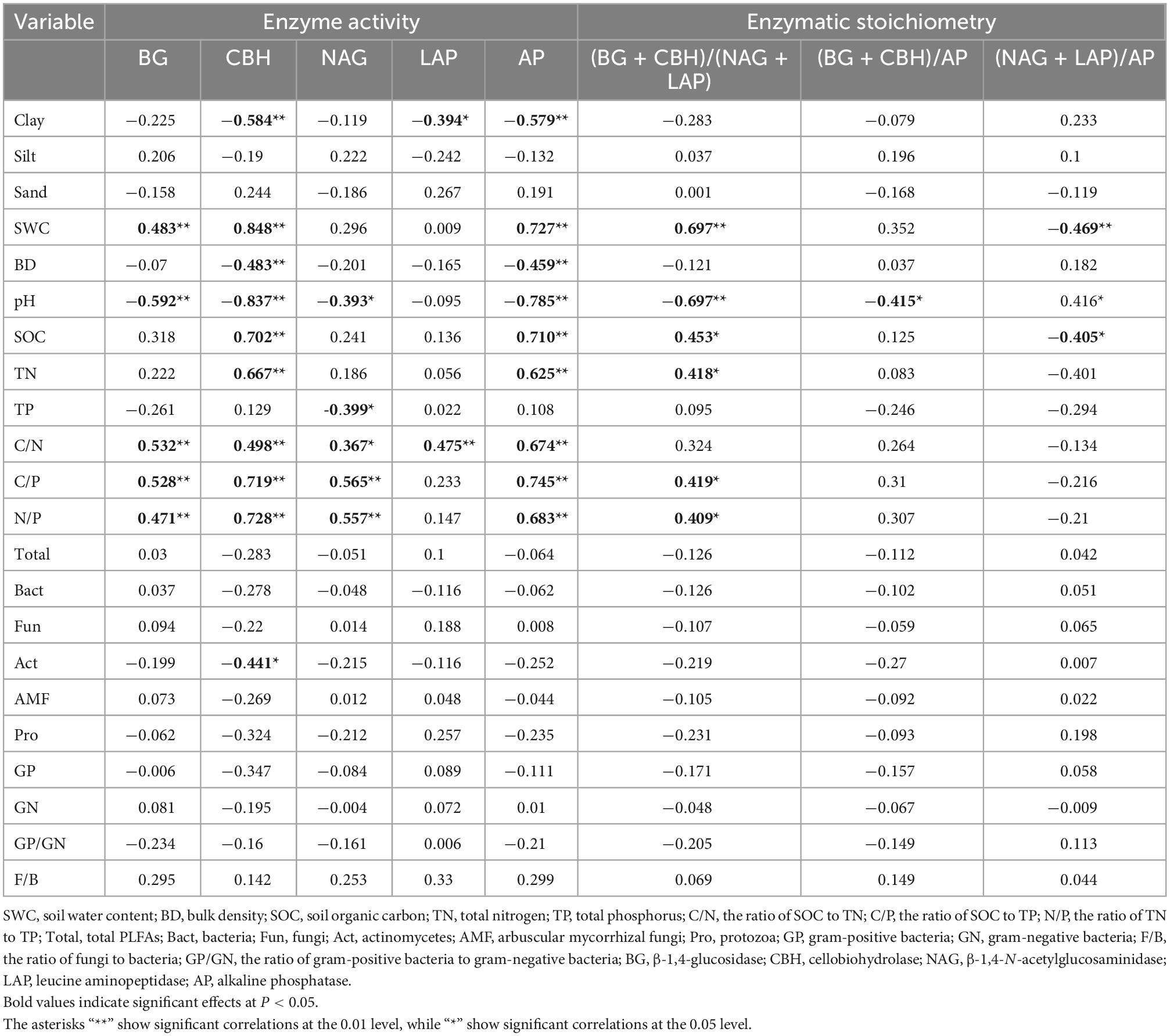
Table 4. Pearson correlation analysis showing the relationships between soil properties, soil enzyme activities, and enzymatic stoichiometry.
Soil physicochemical properties, the composition of the microbial community, and ecoenzymatic activities accounted for 43.94 and 22.21% of the microbial C and N/P limitation, respectively (Figure 8). The most significant effects of the selected properties on soil microbial C limitation followed the order BG > NAG > CBH > pH > silt > TP, whereas those on microbial N/P limitation followed the order NAG > pH > LAP > AP > TP > AMF (Figure 8).
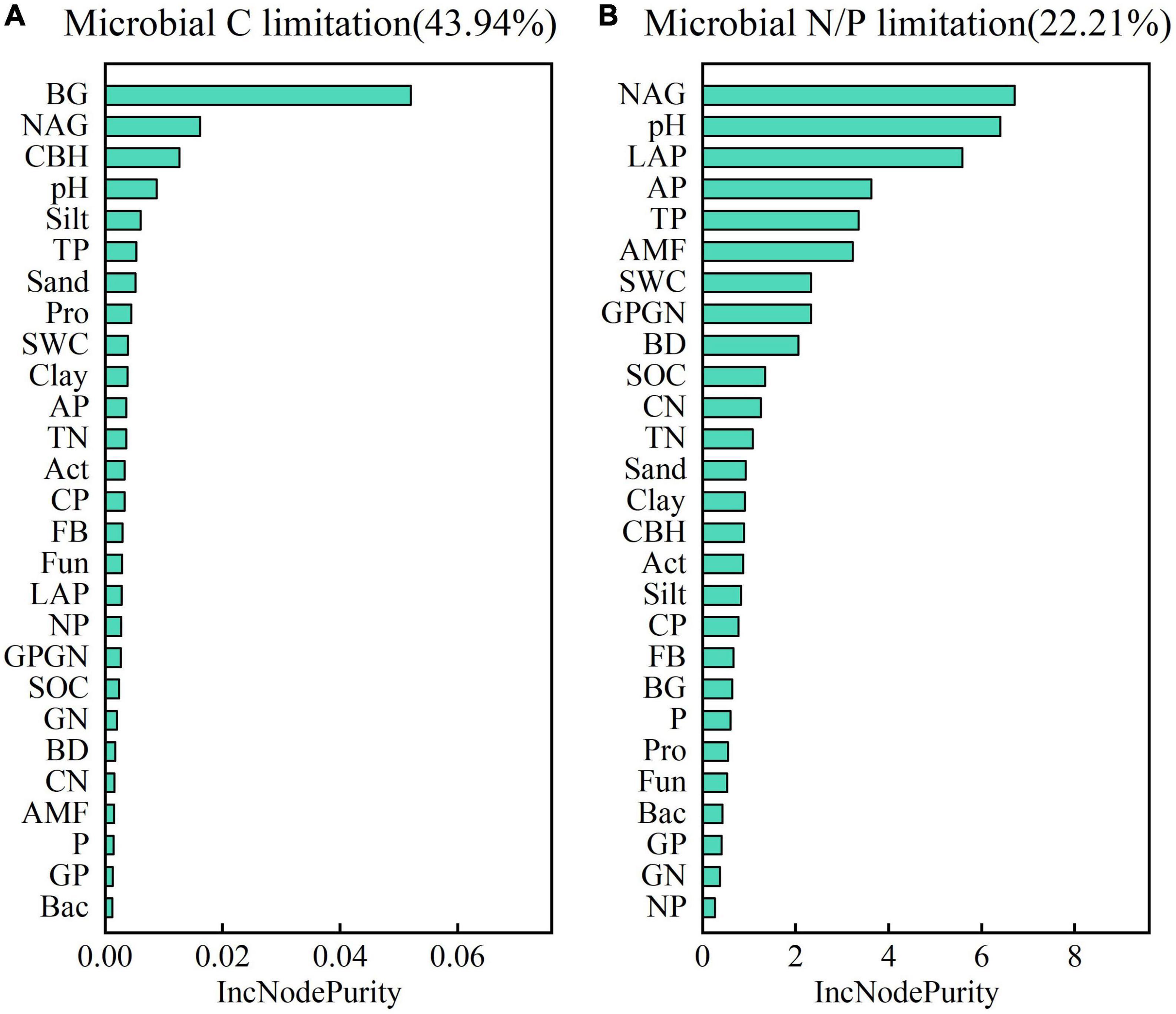
Figure 8. Effects of soil physico-chemical properties and microbial properties on soil microbial C limitation (A) and soil microbial N/P limitation (B). SWC, soil water content; BD, bulk density; SOC, soil organic carbon; TN, total nitrogen; TP, total phosphorus; CN, the ratio of SOC to TN; CP, the ratio of SOC to TP; NP, the ratio of TN to TP; Total, total PLFAs; Bact, bacteria; Fun, fungi; Act, actinomycetes; AMF, arbuscular mycorrhizal fungi; Pro, protozoa; GP, gram-positive bacteria; GN, gram-negative bacteria; FB, the ratio of fungi to bacteria; GPGN, the ratio of gram-positive bacteria to gram-negative bacteria; BG, β-1,4-glucosidase; CBH, cellobiohydrolase; NAG, β-1,4-N-acetylglucosaminidase; LAP, leucine aminopeptidase; AP, alkaline phosphatase.
Discussion
Variations in the composition of microbial communities and enzyme activities
Microorganisms play a pivotal role in maintaining ecological stability. The composition and diversity of the soil microbial community reflect both the biological activity and mechanisms of ecological stress in soil (Looby and Martin, 2020; Zhou et al., 2021). In this study, we used PLFAs to quantitatively assess the structure and diversity of soil microbial communities along an elevation gradient on the eastern slope of the Helan Mountains. Bacteria were the most abundant, followed by fungi and actinomycetes, and the least were protozoans at all elevations (Figures 1, 4). Total microbial biomass, including bacteria, fungi, GP, and AMF, first increased and then decreased with increasing elevation. The microbial abundance was the highest at mid-high elevations, mainly because of the thick litter layer (Supplementary Tables 1, 2), which provides a substrate for microbial growth and increases the synergy between soil and microorganisms. Moreover, the suitable temperature and water conditions at mid-high elevation also facilitate microbial growth and development (Liu et al., 2013, 2018; Lange et al., 2015). GMMs were the highest at 2,249 m, possibly because (1) at 2,249 m, the plant community is highly diverse, impacting the structure and diversity of the soil microbial community (Supplementary Table 2), and (2) the rich litter layer at this elevation provides abundant nutrients for microbial growth.
The F:B reflects the characteristics of the bacterial and fungal biomass in a microbial community, with higher F:B values indicating higher soil C mineralization rates and consequently a more stable soil ecosystem (Vries et al., 2006; Camenzind et al., 2020). Our results indicate that the fungal and bacteria abundances were the highest at an elevation of 2,249 m, consistent with the findings of previous studies (Liu et al., 2013; Ma et al., 2022). The GP:GN ratio reflects the diverse characteristics of bacterial communities, facilitating the understanding of soil fertility (Hammesfahr et al., 2008). High GP:GN values indicate the microbial community dominance by gram-positive bacteria, suggesting a eutrophic to oligotrophic transformation (Saetre and Baath, 2000; Wang and Wang, 2008; Nicolas et al., 2019). In our study, the GP: GN was higher at low (1,380–1,650 m) than at medium elevations (2,139–2,438 m) (Figure 2B), indicating enrichment of oligotrophic bacteria at low elevations.
Soil microorganisms can regulate the production of extracellular enzyme activities when either the substrate or other resources become limited or saturated (Stark et al., 2014; Dong et al., 2021). Our results show that (BG + CBH) and (NAG + LAP) first increased and then decreased with increasing elevation, with the highest level at medium elevations (2,139–2,249 m) (Figures 5A, B). In combination with the NMDS analysis, we found that the activities of the five selected enzymes significantly differed along the elevation gradient (Figure 6). This may be due to vegetation type, high plant species richness, well-developed root system, high litter quality, and high diversity of plant root exudates found at 1,700–2,300 m in the Helan Mountains (Liu et al., 2013; Wu et al., 2021). Previous research also reported that vegetation coverage and nutrient availability affect EEA (Qiu et al., 2021), which might also explain the high EEA levels at medium elevations. In general, these results support our first hypothesis that a difference exists between the structure of the soil microbial community and enzyme activities along the elevation gradient of the Helan Mountains.
Ecoenzymatic stoichiometry and microbial metabolism
The ecological stoichiometry theory (EST) is applied when determining biogeochemical relationships between microbial metabolic demand and nutrient limitation in soil ecosystems (Di and Fornara, 2017). Both the soil ecoenzymatic C:P and C:N ratios and vector length were higher at medium and high elevations (2,139–2,248 m) than at low elevations (1,380–1,650 m), indicating a relative C requirement of soil microbial communities at mid and high elevations. One possible explanation is that as secondary succession progresses, plant communities succession change from being dominated by annual herbs to perennial species (Mooshammer et al., 2014; Zhong et al., 2020), thus altering the organic matter input and limiting the available carbon for microorganisms. Another possible explanation is that high elevations are characterized by low temperature, high radiation, and strong winds (Mccain, 2007; Zhou et al., 2016), which may lead to insufficient nutrient circulation and inhibit microbial metabolic activities.
It has been proposed that the vector length and angle could denote relative C vs. nutrient limitation (N and P) and relative P vs. N limitation for soil microbes (Moorhead et al., 2016). In our study, the (NAG + LAP): AP activity ratios were the highest at 1,650 m, and their respective vector angles were <45, indicating N rather than P limitation. Moreover, numerous long-term vegetation restoration projects have shown that P is also a limiting element in various ecosystems (Rode et al., 2017; Zhang et al., 2019a). The present study showed that vector angles were significantly higher at medium and high elevations (2,139–2,248 m) compared to low elevations (1,380–1,650 m), indicating a more severe P limitation at medium and high elevations (Figure 6). There are two possible reasons for this; first, plant litter and organic matter inputs increase with vegetation succession, resulting in nutrient accumulation. However, because of the low temperatures at high elevations, nutrient circulation is suppressed, impeding the metabolic activity and nutrient acquisition of microbes (Jansson and Taş, 2014; McIntosh et al., 2016; Zhou et al., 2016). Second, low temperature and precipitation can suppress soil weathering, leading to a slow release of P from the parent material, which may further decrease the P levels in soils (Jansson and Taş, 2014; Zhou et al., 2016). The ecoenzymatic stoichiometry indicated that microbial growth limitation by C and P in the Helan Mountains is more significant at higher and medium elevations, consistent with our first hypothesis. Furthermore, the (NAG + LAP): AP ratio was close to 1:1, indicating possible homeostatic microbial N/P cycles in the Helan Mountains (Sinsabaugh et al., 2008).
Factors affecting enzyme activities and stoichiometry
Soil nutrients can regulate EEA and EES by influencing the available substrates and nutrient stoichiometry (Dong et al., 2019). In arid areas, water is the main limiting factor for plant growth, precipitation increases with elevation in the Helan Mountains, changing the vegetation from desert steppe to mountain sparse and pure forests. These vegetation changes are accompanied by increased plant biomass (Ji, 2015), which also increases the organic matter input. Moreover, the low temperature of high elevations limits microbial growth and activity (Figures 1–3), thereby reducing SOM decomposition and leading to more accumulation of SOC and TN at high altitudes. Our results on the SOC and TN accumulations along the elevation gradient in arid areas were consistent with the previous studies (Bi et al., 2018; Kong et al., 2020). Moreover, previous studies indicated that SWC significantly affects EEA and EES, especially in water-limited regions (Zuo et al., 2018; Cui et al., 2019b). High SWC generally stimulates the diffusion rate of microbial substrates and enzymes, promoting microbial activity and functioning (Steinweg et al., 2012). In our study, BG, CBH, AP, and (BG + CBH): (NAG + LAP) positively correlated with SWC, similar to the results reported in Larix principis-rupprechtii plantations (Qiu et al., 2021). We also observed a negative correlation between pH and enzyme activities and their stoichiometry, consistent with previous findings (Zhang et al., 2021), thus confirming the assumption that soil pH strongly controls enzymatic activities (Cenini et al., 2016).
Soil C-, N-, and P-acquiring extracellular enzymes and their stoichiometry varied with elevation in the Helan Mountains. Large amounts of soil nutrients provide sufficient substrate for microbial and enzyme activities (Cenini et al., 2016; Qiu et al., 2021), and variations in vegetation type, soil nutrient availability, and microbial-mediated factors change the EEA and EES (Luo et al., 2020; Zhao et al., 2021). SOC, TN, TP, BG, CBH, NAG, LAP, AP, and (BG + CBH): (NAG + LAP) exhibited positive correlations among themselves (Table 4); however, negative correlations existed between soil nutrients and EEA and EES in different ecosystems (Zheng et al., 2020). Our results also agreed with the previous findings (Bowles et al., 2014; Wang et al., 2020; Qin et al., 2021). We found that soil nutrient stoichiometry (C/N, C/P, and N/P) influenced EEA and EES, contrary to the previous findings (Wu et al., 2020). Furthermore, soil nutrients greatly affected EEA and EES, microbial biomass, and soil particle composition, possibly because the production of soil enzymes is directly regulated by soil nutrients and microorganisms (Yang et al., 2020). In summary, nutrient availability strongly influences microbial community composition, enzymatic activity, and stoichiometry.
Conclusion
We investigated the soil physicochemical properties, microbial community composition, and enzyme activities and determined their correlations along an elevation gradient in the Helan Mountains. Total microbial biomass and its microbial components, including bacteria, fungi, actinomycetes, and AMF, did not vary with elevation, whereas the PLFA biomarkers showed significant differences along the elevation gradient, with the highest levels occurring at 2,249 m. The selected five extracellular enzymes were significantly affected by elevation, and the microbial C and P limitations were more severe at high elevations. Moreover, soil physicochemical properties, microbial community composition, and ecoenzymatic activities accounted for 43.94 and 22.21% of the microbial C and P limitations, respectively. This study provides insights into the pattern and driving factors of microbial metabolic limitation along elevation gradients in the Helan Mountains. Metagenomics studies should be conducted on the structure and function of soil microbial communities at different elevations in the future to obtain a deeper understanding of microbial participation in biogeochemical cycles and their underlying mechanisms.
Data availability statement
The original contributions presented in this study are included in the article/Supplementary material, further inquiries can be directed to the corresponding author.
Author contributions
DP and BL: methodology, visualization, formal analysis, data curation, and writing – original draft. MW and JM: data curation, visualization, and software. XL and WZ: validation and resources. YH and LC: conceptualization, funding acquisition, supervision, and writing – review and editing. All authors contributed to the article and approved the submitted version.
Funding
This project was funded by the National Natural Science Foundation of China (32201631), the Ningxia Hui Autonomous Region Key Research and Development Project (2021BEG02005), the open Project Program of Key Laboratory of Desertification Control and Soil and Water Conservation of Ningxia (KF202203), and the Local projects guided by the central government (2022FRD05001).
Acknowledgments
We appreciate the editor and the reviewers for giving us constructive suggestions.
Conflict of interest
The authors declare that the research was conducted in the absence of any commercial or financial relationships that could be construed as a potential conflict of interest.
Publisher’s note
All claims expressed in this article are solely those of the authors and do not necessarily represent those of their affiliated organizations, or those of the publisher, the editors and the reviewers. Any product that may be evaluated in this article, or claim that may be made by its manufacturer, is not guaranteed or endorsed by the publisher.
Supplementary material
The Supplementary Material for this article can be found online at: https://www.frontiersin.org/articles/10.3389/ffgc.2022.1024656/full#supplementary-material
References
Baldos, A. P., Corre, M. D., and Veldkamp, E. (2015). Response of N cycling to nutrient inputs in forest soils across a 1000–3000 m elevation gradient in the Ecuadorian Andes. Ecology 96, 749–761. doi: 10.1890/14-0295.1
Bi, X., Li, B., Nan, B., Fan, Y., Fu, Q., and Zhang, X. S. (2018). Characteristics of soil organic carbon and total nitrogen under various grassland types along a transect in a mountain-basin system in Xinjiang, China. J. Arid Land 10, 612–627. doi: 10.1007/s40333-018-0006-1
Bowles, T. M., Acosta-Martinez, V., Calderon, F., and Jackson, L. E. (2014). Soil enzyme activities, microbial communities, and carbon and nitrogen availability in organic agroecosystems across an intensively-managed agricultural landscape. Soil Biol. Biochem. 68, 252–262. doi: 10.1016/j.soilbio.2013.10.004
Brockett, B. F. T., Prescott, C. E., and Grayston, S. J. (2012). Soil moisture is the major factor influencing microbial community structure and enzyme activities across seven biogeoclimatic zones in western Canada. Soil Biol. Biochem. 44, 9–20. doi: 10.1016/j.soilbio.2011.09.003
Burns, R. G., DeForest, J. L., Marxsen, J., Sinsabaugh, R. L., Stromberger, M. E., Wallenstein, M. D., et al. (2013). Soil enzymes in a changing environment: Current knowledge and future directions. Soil Biol. Biochem. 58, 216–234. doi: 10.1016/j.soilbio.2012.11.009
Camenzind, T., Grenz, K. P., Lehmann, J., and Rillig, M. C. (2020). Soil fungal mycelia have unexpectedly flexible stoichiometric C:N and C:P ratios. Ecol. Lett. 24, 208–218. doi: 10.1111/ele.13632
Carbonetto, B., Rascovan, N., Álvarez, R., Mentaberry, A., and Vázquez, M. P. (2014). Structure, composition and metagenomic profile of soil microbiomes associated to agricultural land use and tillage systems in Argentine Pampas. PLoS One 9:e99949. doi: 10.1371/journal.pone.0099949
Cenini, V. L., Fornara, D. A., McMullan, G., Ternan, N., Carolan, R., Crawley, M. J., et al. (2016). Linkages between extracellular enzyme activities and the carbon and nitrogen content of grassland soils. Soil Biol. Biochem. 96, 198–206. doi: 10.1016/j.soilbio.2016.02.015
Chen, H., Li, D. J., Xiao, K. C., and Wang, K. L. (2018). Soil microbial processes and resource limitation in karst and non-karst forests. Funct. Ecol. 32, 1400–1409. doi: 10.1111/1365-2435.13069
Cui, Y. X., Bing, H. J., Fang, L. C., Jiang, M., Shen, G. T., Yu, J. L., et al. (2019a). Extracellular enzyme stoichiometry reveals the carbon and phosphorus limitations of microbial metabolisms in the rhizosphere and bulk soils in alpine ecosystems. Plant Soil 458, 7–20. doi: 10.1007/s11104-019-04159-x
Cui, Y. X., Fang, L. C., Guo, X. B., Han, F., Ju, W. L., Ye, L. P., et al. (2019b). Natural grassland as the optimal pattern of vegetation restoration in arid and semiarid regions: Evidence from nutrient limitation of soil microbes. Sci. Total Environ. 648, 388–397. doi: 10.1016/j.scitotenv.2018.08.173
Cui, Y. X., Fang, L. C., Guo, X. B., Wang, X., Zhang, Y. J., Li, P. F., et al. (2018). Ecoenzymatic stoichiometry and microbial nutrient limitation in rhizosphere soil in the arid area of the northern Loess Plateau, China. Soil Biol. Biochem. 116, 11–21. doi: 10.1016/j.soilbio.2017.09.025
Cui, Y. X., Moorhead, D. L., Guo, X. B., Peng, S. S., Wang, Y. Q., Zhang, X. C., et al. (2021). Stoichiometric models of microbial metabolic limitation in soil systems. Glob. Change Boil. 30, 2297–2311. doi: 10.1111/geb.13378
Deng, L., Peng, C. H., Huang, C. B., Wang, K. B., Liu, Q. Y., Liu, Y. L., et al. (2019). Drivers of soil microbial metabolic limitation changes along a vegetation restoration gradient on the Loess Plateau, China. Geoderma 353, 188–200. doi: 10.1016/j.geoderma.2019.06.037
Di, P. F., and Fornara, D. A. (2017). Plant and soil nutrient stoichiometry along primary ecological successions: Is there any link? PLoS One 12:e0182596. doi: 10.1371/journal.pone.0182569
Dong, C. C., Wang, W., Liu, H. Y., Xu, X. T., and Zeng, H. (2019). Temperate grassland shifted from nitrogen to phosphorus limitation induced by degradation and nitrogen deposition: Evidence from soil extracellular enzyme stoichiometry. Ecol. Ind. 101, 453–464. doi: 10.1016/j.ecolind.2019.01.046
Dong, H. Y., Ge, J. F., Sun, K., Wang, B. Z., Xue, J. M., Wakelin, S. A., et al. (2021). Change in root-associated fungal communities affects soil enzymatic activities during Pinus massoniana forest development in subtropical China. For. Ecol. Manag. 482:118817. doi: 10.1016/j.foreco.2020.118817
Duan, C. J., Fang, L. C., Yang, C. L., Chen, W. B., Cui, Y. X., and Li, S. Q. (2018). Reveal the response of enzyme activities to heavy metals through in situ zymography. Ecotoxicol. Environ. Saf. 156, 106–115. doi: 10.1016/j.ecoenv.2018.03.015
Feyissa, A., Gurmesa, G. A., Yang, F., Long, C. Y., Zhang, Q., and Cheng, X. L. (2022). Soil enzyme activity and stoichiometry in secondary grasslands along a climatic gradient of subtropical China. Sci. Total Environ. 825:154019. doi: 10.1016/j.scitotenv.2022.154019
Frostegård, A., and Bååth, E. (1996). The use of phospholipid fatty acid analysis to estimate bacterial and fungal biomass in soil. Biol. Fertil. Soils 22, 59–65. doi: 10.1007/BF00384433
Frostegård, Å., Tunlid, A., and Bååth, E. (2011). Use and misuse of PLFA measurements in soils. Soil Biol. Biochem. 43, 1621–1625. doi: 10.1016/j.soilbio.2010.11.021
Guan, S. Y., Zhang, D. S., and Zhang, Z. M. (1991). Methods of soil enzyme activities analysis. (Beijing: Chinese Agricultural Press), 263–271.
Hammesfahr, U., Heuer, H., Manzke, B., Smalla, K., and Thiele-Bruhn, S. (2008). Impact of the antibiotic sulfadiazine and pig manure on the microbial community structure in agricultural soils. Soil Biol. Biochem. 40, 1583–1591. doi: 10.1016/j.soilbio.2008.01.010
Hill, B. H., Elonen, C. M., Seifert, L. R., May, A. A., and Tarquinio, E. (2012). Microbial enzyme stoichiometry and nutrient limitation in US streams and rivers. Ecol. Ind. 18:540551. doi: 10.1016/j.ecolind.2012.01.007
Huang, J. Y., Yu, H. L., Lin, H., Zhang, Y., Searle, E. B., and Yuan, Z. Y. (2016). Phosphorus amendment mitigates nitrogen addition-induced phosphorus limitation in two plant species in a desert steppe, China. Plant Soil 399, 221–232. doi: 10.1007/s11104-015-2649-4
Jansson, J. K., and Taş, N. (2014). The microbial ecology of permafrost. Nat. Rev. Microbiol. 12, 414–425. doi: 10.1038/nrmicro3262
Ji, B. (2015). Study on biomass and carbon storage of main forest communities in Helan Mountain in Ningxia. Yinchuan: Ningxia University.
Jiang, Y. L., Lei, Y. B., Qin, W., Korpelainen, H., and Li, C. Y. (2019). Revealing microbial processes and nutrient limitation in soil through ecoenzymatic stoichiometry and glomalinrelated soil proteins in a retreating glacier forefield. Geoderma 338, 313–324. doi: 10.1016/j.geoderma.2018.12.023
Kong, J. Q., He, Z. B., Chen, L. F., Yang, R., Du, J., Lin, P. F., et al. (2020). Elevational gradients and distributions of aggregate associated organic carbon and nitrogen and stability in alpine forest ecosystems. Soil Sci. Soc. Am. J. 84, 1971–1982. doi: 10.1002/saj2.20121
Lamb, E. G., Han, S., Lanoil, B. D., Henry, G. H. R., Brummell, M. E., Banerjee, S., et al. (2011). A High Arctic soil ecosystem resists long-term environmental manipulations. Glob. Change Biol. 17, 3187–3194. doi: 10.1111/j.1365-2486.2011.02431.x
Lange, M., Eisenhauer, N., Sierra, C. A., Bessler, H., Engels, C., Griffiths, R. I., et al. (2015). Plant diversity increases soil microbial activity and soil carbon storage. Nat. Commun. 6:6707. doi: 10.1038/ncomms7707
Li, Q. W., Liu, Y., Gu, Y. F., Guo, L., Huang, Y. Y., Zhang, J., et al. (2020). Ecoenzymatic stoichiometry and microbial nutrient limitations in rhizosphere soil along the Hailuogou Glacier forefield chronosequence. Sci. Total Environ. 704:135413. doi: 10.1016/j.scitotenv.2019.135413
Li, X., Jiao, Y., and Yang, M. D. (2014). Microbial diversity of different saline-alkaline soil analysing by PLFA in the Hetao area of inner Mongolia. Ecol. Sci. 33, 488–494.
Li, Y. B., Bezemer, T. M., Yang, J. J., Lü, X. T., Li, X. Y., Liang, W. J., et al. (2019). Changes in litter quality induced by N deposition alter soil microbial communities. Soil Biol. Biochem. 130, 33–42. doi: 10.1016/j.soilbio.2018.11.025
Liu, B. R., Zhang, X. Z., Hu, T. H., and Li, W. J. (2013). Soil microbial diversity under typical vegetation zones along an elevation gradient in Helan Mountains. Acta Ecol. Sin. 33, 7211–7220.
Liu, D., Huang, Y. M., Sun, H. Y., and An, S. S. (2018). The restoration age of Robinia pseudoacacia plantation impacts soil microbial biomass and microbial community structure in the loess plateau. Catena 165, 192–200. doi: 10.1016/j.catena.2018.02.001
Liu, L., Gundersen, P., Zhang, T., and Mo, J. M. (2012). Effects of phosphorus addition on soil microbial biomass and community composition in three forest types in tropical China. Soil Biol. Biochem. 44, 31–38. doi: 10.1016/j.soilbio.2011.08.017
Looby, C. I., and Martin, P. H. (2020). Diversity and function of soil microbes on montane gradients: The state of knowledge in a changing world. FEMS Microbiol. Ecol. 96:fiaa122. doi: 10.1093/femsec/fiaa122
Luo, D., Cheng, R. M., Liu, S., Shi, Z. M., and Feng, Q. H. (2020). Responses of soil microbial community composition and enzyme activities to land-use change in the eastern Tibetan Plateau, China. Forests 11:483. doi: 10.3390/f11050483
Ma, J. P., Pang, D. B., Chen, L., Wan, H. Y., Chen, G. L., and Li, X. B. (2022). Characteristics of soil microbial community structure under vegetation at different altitudes in Henan Mountains. Acta Ecol. Sin. 42, 667–676.
Ma, W. W., Li, G., Wu, J. H., Xu, G. R., and Wu, J. Q. (2020). Response of soil labile organic carbon fractions and carbon-cycle enzyme activities to vegetation degradation in a wet meadow on the Qinghai–Tibet Plateau. Geoderma 377:114565. doi: 10.1016/j.geoderma.2020.114565
Magurran, A. E. (1988). Ecological diversity and its measurement. (Princeton, NJ: Princeton University Press), 141–162.
Manzoni, S. (2017). Flexible carbon-use efficiency across litter types and during decomposition partly compensates nutrient imbalances—Results from analytical stoichiometric models. Front. Microbiol. 8:661. doi: 10.3389/fmicb.2017.00661
Mccain, C. M. (2007). Could temperature and water availability drive elevational species richness patterns? A global case study for bats. Glob. Ecol. Biogeogr. 16, 1–13. doi: 10.1111/j.1466-8238.2006.00263.x
McIntosh, A. C., Macdonald, S. E., and Quideau, S. A. (2016). Understory plant community composition is associated with fine-scale above-and below-ground resource heterogeneity in mature lodgepole pine (Pinus contorta) Forests. PLoS One 11:e0151436. doi: 10.1371/journal.pone.0151436
Moorhead, D. L., Sinsabaugh, R. L., Hill, B. H., and Weintraub, M. N. (2016). Vector analysis of ecoenzyme activities reveal constraints on coupled C, N and P dynamics. Soil Biol. Biochem. 93, 1–7. doi: 10.1016/j.soilbio.2015.10.019
Mooshammer, M., Wanek, W., Zechmeister-Boltenstern, S., and Bichter, A. (2014). Stoichiometric imbalances between terrestrial decomposer communities and their resources: Mechanisms and implications of microbial adaptations to their resources. Front. Microbiol. 5:22. doi: 10.3389/fmicb.2014.00022
Nicolas, F., Paul, K., Mark, F., Marie-Charlotte, N., Michael, J. G., and David, A. W. (2019). The ratio of gram-positive to gram-negative bacterial PLFA markers as an indicator of carbon availability in organic soils. Soil Biol. Biochem. 128, 111–114. doi: 10.1016/j.soilbio.2018.10.010
Nottingham, A. T., Bååth, E., Reischke, S., Salinas, N., and Meir, P. (2019). Adaptation of soil microbial growth to temperature: Using a tropical elevation gradient to predict future changes. Glob. Change Biol. 25, 827–838. doi: 10.1111/gcb.14502
Nottingham, A. T., Turner, B. L., Whitaker, J., Ostle, N., Bardgett, R. D., McNamara, N. P., et al. (2016). Temperature sensitivity of soil enzymes along an elevation gradient in the Peruvian Andes. Biogeochemistry 127, 217–230. doi: 10.1007/s10533-015-0176-2
Ouyang, S. N., Tian, Y. Q., Liu, Q. Y., Zhang, L., Wang, R. X., and Xu, X. L. (2016). Nitrogen competition between three dominant plant species and microbes in a temperate grassland. Plant Soil 408, 121–132. doi: 10.1007/s11104-016-2904-3
Pang, D. B., Wang, G. Z., Li, G. J., Sun, Y. L., Liu, Y. G., and Zhou, J. X. (2018). Ecological stoichiometric characteristics of two typical plantations in the karst ecosystem of southwestern China. Forests 9:56. doi: 10.3390/f9020056
Peng, X. Q., and Wang, W. (2016). Stoichiometry of soil extracellular enzyme activity along a climatic transect in temperate grasslands of northern China. Soil Biol. Biochem. 98, 74–84. doi: 10.1016/j.soilbio.2016.04.008
Qin, L., Freeman, C., Jia, X. Y., Zhang, Z. S., Liu, B., Zhang, S. Q., et al. (2021). Microbial enzyme activity and stoichiometry signal the effects of agricultural intervention on nutrient cycling in peatlands. Ecol. Ind. 122:107242. doi: 10.1016/j.ecolind.2020.107242
Qiu, X. C., Peng, D. L., Tian, H. X., Wang, H. B., Liu, X., Cao, L., et al. (2021). Soil ecoenzymatic stoichiometry and microbial resource limitation driven by thinning practices and season types in Larix principis-rupprechtii plantations in North China. For. Ecol. Manag. 482:118880. doi: 10.1016/j.foreco.2020.118880
Ren, C. J., Zhou, Z. H., Guo, Y. X., Yang, G. H., Zhao, F. Z., Wei, G. H., et al. (2021). Contrasting patterns of microbial community and enzyme activity between rhizosphere and bulk soil along an elevation gradient. Catena 196:104921. doi: 10.1016/j.catena.2020.104921
Rode, M., Lemoine, N. P., and Smith, M. D. (2017). Prospective evidence for independent nitrogen and phosphorus limitation of grasshopper (Chorthippus curtipennis) growth in a tallgrass prairie. PLoS One 12:e0177754. doi: 10.1371/journal.pone.0177754
Saetre, P., and Baath, E. (2000). Spatial variation and patterns of soil microbial community structure in a mixed spruce-birch stand. Soil Biol. Biochem. 32, 909–917. doi: 10.1016/S0038-0717(99)00215-1
Saiya-Cork, K. R., Sinsabaugh, R. L., and Zak, D. R. (2002). The effects of long term nitrogen deposition on extracellular enzyme activity in an Acer saccharum forest soil. Soil Biol. Biochem. 34, 1309–1315. doi: 10.1016/S0038-0717(02)00074-3
Sinsabaugh, R. L., Hill, B. H., and Follstad Shah, J. J. (2009). Ecoenzymatic stoichiometry of microbial organic nutrient acquisition in soil and sediment. Nature 462, 795–798. doi: 10.1038/nature08632
Sinsabaugh, R. L., Lauberaw, C. C. L., Weintraub, M. N., Ahmed, B., Allison, S. D., Crenshaw, C., et al. (2008). Stoichiometry of soil enzyme activity at global scale. Ecol. Lett. 11, 1252–1264. doi: 10.1111/j.1461-0248.2008.01245.x
Sinsabaugh, R. L., and Shah, J. J. F. (2012). Ecoenzymatic stoichiometry and ecological theory. Annu. Rev. Ecol. Evol. Syst. 43, 313–343. doi: 10.1146/annurev-ecolsys-071112-124414
Stark, S., Männistö, M. K., and Eskelinen, A. (2014). Nutrient availability and pH jointly constrain microbial extracellular enzyme activities in nutrient-poor tundra soils. Plant Soil 383, 373–385. doi: 10.1007/s11104-014-2181-y
Steinweg, J. M., Dukes, J. S., and Wallenstein, M. D. (2012). Modeling the effects of temperature and moisture on soil enzyme activity: Linking laboratory assays to continuous field data. Soil Biol. Biochem. 55, 85–92. doi: 10.1016/j.soilbio.2012.06.015
Vries, F. T. D., Hoffland, E., Eekeren, N. V., Brussaard, L., and Bloem, J. (2006). Fungal/bacterial ratios in grasslands with contrasting nitrogen management. Soil Biol. Biochem. 38, 2092–2103. doi: 10.1016/j.soilbio.2006.01.008
Wang, J., Wang, X., Liu, G., Wang, G., Wu, Y., and Zhang, C. (2020). Fencing as an effective approach for restoration of alpine meadows: Evidence from nutrient limitation of soil microbes. Geoderma 363:114148. doi: 10.1016/j.geoderma.2019.114148
Wang, Q. K., and Wang, S. L. (2008). Soil microbial properties and nutrients in pure and mixed Chinese fir plantations. J. For. Res. 19, 131–135. doi: 10.1007/s11676-008-0022-7
Wu, M. Y., Pang, D. B., Chen, L., Li, X. B., Liu, L. Z., Liu, B., et al. (2021). Chemical composition of soil organic carbon and aggregate stability along an elevation gradient in Helan Mountains, northwest China. Ecol. Ind. 131:108228. doi: 10.1016/j.ecolind.2021.108228
Wu, Y., Chen, W. J., Li, Q., Guo, Z. Q., Li, Y. Z., Zhao, Z. W., et al. (2020). Ecoenzymatic stoichiometry and nutrient limitation under a natural secondary succession of vegetation on the Loess Plateau, China. Land Degrad. Dev. 32, 399–409. doi: 10.1002/ldr.3723
Yang, Y., Liang, C., Wang, Y. Q., Cheng, H., An, S. S., and Chang, S. X. (2020). Soil extracellular enzyme stoichiometry reflects the shift from P- to N-limitation of microorganisms with grassland restoration. Soil Biol. Biochem. 149:107928. doi: 10.1016/j.soilbio.2020.107928
Yu, J. L., Bing, H. J., Chang, R. Y., Cui, Y. X., Shen, G. T., Wang, X. X., et al. (2022). Microbial metabolic limitation response to experimental warming along an altitudinal gradient in alpine grasslands, eastern Tibetan Plateau. Catena 214:106243. doi: 10.1016/j.catena.2022.106243
Zeng, X. M., Wei, C. F., Liu, X. H., and Zhang, L. N. (2020). Qinghai spruce (Picea crassifolia) and Chinese pine (Pinus tabuliformis) show high vulnerability and similar resilience to early growing-season drought in the Helan Mountains, China. Ecol. Ind. 110:105871. doi: 10.1016/j.ecolind.2019.105871
Zhang, J. T. (2005). Succession analysis of plant communities in abandoned croplands in the eastern Loess Plateau of China. J. Arid Environ. 63, 458–474. doi: 10.1016/j.jaridenv.2005.03.027
Zhang, J. T., and Dong, Y. R. (2010). Factors affecting species diversity of plant communities and the restoration process in the loess area of China. Ecol. Eng. 36, 345–350. doi: 10.1016/j.ecoleng.2009.04.001
Zhang, Q., Zhang, D. D., Wu, J. J., Li, J. S., Feng, J., and Cheng, X. L. (2021). Soil nitrogen-hydrolyzing enzyme activity and stoichiometry following a subtropical land use change. Land Degrad. Dev. 32, 4277–4287. doi: 10.1002/ldr.4034
Zhang, S. H., Pan, Y., Zhou, Z. H., Deng, J., Zhao, F. Z., Guo, Y. X., et al. (2022). Resource limitation and modeled microbial metabolism along an elevation gradient. Catena 209:105807. doi: 10.1016/j.catena.2021.105807
Zhang, W., Xu, Y. D., Gao, D. X., Wang, X., Liu, W. C., Deng, J., et al. (2019b). Ecoenzymatic stoichiometry and nutrient dynamics along a revegetation chronosequence in the soils of abandoned land and Robinia pseudoacacia plantation on the Loess Plateau, China. Soil Biol. Biochem. 134, 1–14. doi: 10.1016/j.soilbio.2019.03.017
Zhang, W., Liu, W. C., Xu, M. P., Deng, J., Han, X. H., Yang, G. H., et al. (2019a). Response of forest growth to C:N:P stoichiometry in plants and soils during Robinia pseudoacacia afforestation on the Loess Plateau, China. Geoderma 337, 280–289. doi: 10.1016/j.geoderma.2018.09.042
Zhao, Y. Z., Liang, C. F., Shao, S., Chen, J. H., Qin, H., and Xu, Q. F. (2021). Linkages of litter and soil C:N:P stoichiometry with soil microbial resource limitation and community structure in a subtropical broadleaf forest invaded by Moso bamboo. Plant Soil 465, 473–490. doi: 10.1007/s11104-021-05028-2
Zheng, L., Chen, H., Wang, Y. Q., Mao, Q. G., Zheng, M. H., Su, Y. R., et al. (2020). Responses of soil microbial resource limitation to multiple fertilization strategies. Soil Tillage Res. 196:104474. doi: 10.1016/j.still.2019.104474
Zhong, Z. K., Li, W. J., Lu, X. Q., Gu, Y. Q., Wu, S. J., Shen, Z. Y., et al. (2020). Adaptive pathways of soil microorganisms to stoichiometric imbalances regulate microbial respiration following afforestation in the Loess Plateau, China. Soil Biol. Biochem. 151:108048. doi: 10.1016/j.soilbio.2020.108048
Zhou, J. Z., Deng, Y., Shen, L. N., Wen, C. Q., Yan, Q. Y., Ning, D. L., et al. (2016). Temperature mediates continental-scale diversity of microbes in forest soils. Nat. Commun. 7:12083. doi: 10.1038/ncomms12083
Zhou, Y. J., Jia, X., Han, L., Liu, Z., Kang, S. Z., and Zhao, Y. H. (2021). Fungal community diversity in soils along an elevation gradient in a Quercus aliena var. acuteserrata forest in Qinling Mountains, China. Appl. Soil Ecol. 167:104104. doi: 10.1016/j.apsoil.2021.104104
Zhu, Y., Jiang, Y., Liu, Q. R., Kang, M. Y., Spehn, E. M., and Körner, C. (2009). Elevational trends of biodiversity and plant traits do not converge—a test in the Helan Range, NW China. Plant Ecol. 205, 273–283. doi: 10.1007/s11258-009-9616-1
Keywords: soil microbial community, enzymatic stoichiometry, microbial nutrient limitation, elevation gradient, Helan Mountains
Citation: Pang D, Liu B, Wu M, Li X, Hu Y, Ma J, Zhao W and Chen L (2022) Features and driving factors of microbial metabolic limitation in mountain ecosystems in arid areas: A case study on the Helan Mountains, Northwest China. Front. For. Glob. Change 5:1024656. doi: 10.3389/ffgc.2022.1024656
Received: 22 August 2022; Accepted: 12 December 2022;
Published: 23 December 2022.
Edited by:
Mehrdad Zarafshar, Agricultural Research, Education and Extension Organization (AREEO), IranReviewed by:
Yang Yang, Northwest A&F University, ChinaMaryam Teimouri, Research Institute of Forests and Rangelands, Iran
Copyright © 2022 Pang, Liu, Wu, Li, Hu, Ma, Zhao and Chen. This is an open-access article distributed under the terms of the Creative Commons Attribution License (CC BY). The use, distribution or reproduction in other forums is permitted, provided the original author(s) and the copyright owner(s) are credited and that the original publication in this journal is cited, in accordance with accepted academic practice. No use, distribution or reproduction is permitted which does not comply with these terms.
*Correspondence: Lin Chen, ✉ chenlin198388@163.com
†These authors have contributed equally to this work