Understory plant communities show resistance to drought, hurricanes, and experimental warming in a wet tropical forest
- 1Gund Institute for Environment, The University of Vermont, Burlington, VT, United States
- 2Rubenstein School of Environment and Natural Resources, The University of Vermont, Burlington, VT, United States
- 3International Institute of Tropical Forestry, USDA Forest Service, Río Piedras, Puerto Rico
- 4College of Forest Resources and Environmental Science, Michigan Technological University, Houghton, MI, United States
- 5Southwest Biological Science Center, US Geological Survey, Moab, UT, United States
- 6Department of Plant Biology, Ecology, and Evolution, Oklahoma State University, Stillwater, OK, United States
Global climate change has led to rising temperatures and to more frequent and intense climatic events, such as storms and droughts. Changes in climate and disturbance regimes can have non-additive effects on plant communities and result in complicated legacies we have yet to understand. This is especially true for tropical forests, which play a significant role in regulating global climate. We used understory vegetation data from the Tropical Responses to Altered Climate Experiment (TRACE) in Puerto Rico to evaluate how plant communities responded to climate warming and disturbance. The TRACE understory vegetation was exposed to a severe drought (2015), 2 years of experimental warming (4°C above ambient in half of the plots, 2016–2017 and 2018–2019), and two major hurricanes (Irma and María, September 2017). Woody seedlings and saplings were censused yearly from 2015 to 2019, with an additional census in 2015 after the drought ended. We evaluated disturbance-driven changes in species richness, diversity, and composition across ontogeny. We then used Bayesian predictive trait modeling to assess how species responded to disturbance and how this might influence the functional structure of the plant community. Our results show decreased seedling richness after hurricane disturbance, as well as increased sapling richness and diversity after warming. We found a shift in species composition through time for both seedlings and saplings, yet the individual effects of each disturbance were not significant. At both ontogenetic stages, we observed about twice as many species responding to experimental warming as those responding to drought and hurricanes. Predicted changes in functional structure point to disturbance-driven functional shifts toward a mixture of fast-growing and drought-tolerant species. Our findings demonstrate that the tropical forest understory community is more resistant to climatic stressors than expected, especially at the sapling stage. However, early signs of changes in species composition suggest that, in a warming climate with frequent droughts and hurricanes, plant communities might shift over time toward fast-growing or drought-tolerant species.
Introduction
The function and composition of tropical forests are shaped by legacy effects of prior disturbance and recovery events (Johnstone et al., 2016; Zimmerman et al., 2020). The ecological consequences of these events can vary substantially depending on the timing, severity, and duration of the disturbance, the interactions among disturbances, and the biotic and abiotic conditions of the forest in question (Smith, 2011; Johnstone et al., 2016). Currently, tropical forests are projected to experience more frequent and intense disturbance events atop significant changes in temperature and precipitation due to anthropogenic climate change (Emanuel, 2013; Knutson et al., 2013; Holland and Bruyère, 2014; Stott, 2016; Ummenhofer and Meehl, 2017). These complex and interactive dynamics, paired with the large uncertainties that persist in tropical climate models (Grainger, 2010; Cavaleri et al., 2015; Gallup et al., 2021), complicate our understanding of how tropical forests respond to climate change. Reducing this uncertainty is of high importance because tropical forests harbor a significant portion of Earth’s biodiversity and play a disproportionately large role in regulating future climate (Malhi, 2012; Mitchard, 2018). In this study, we contribute to reducing this knowledge gap by evaluating how a decadal drought, hurricane disturbance, and experimental warming affect the species composition and functional structure of understory plant communities in a tropical forest.
Alterations in disturbance regimes have already been observed in Puerto Rico and other tropical Caribbean islands, which have recently experienced increased exposure to extreme climatic events such as hurricanes and droughts (Henareh Khalyani et al., 2016; Mote et al., 2017; Feng et al., 2020). Yet, due to a long disturbance history, plant communities in these forests often develop adaptation strategies that increase their resistance (i.e., ability to withstand a stressor) and resilience (i.e., speed and amount of recovery after a stressor) to a wide range of stressors (Ostertag et al., 2005; Zimmerman et al., 2020). The question remains, however, whether these plant communities will continue to exhibit high resistance and resilience to increased and repeated disturbances within the context of a changing climate. Changes in disturbance regimes (i.e., frequency, severity, size, or timing) could result in rapid reorganization of plants into new communities by generating large gaps that favor the establishment of pioneer and early successional species (Canham et al., 2010). Moreover, directional changes in climate (e.g., warming temperatures) can erode resistance and resilience of plant communities in ways that may not be observed until a major disturbance occurs, which could further alter plant community composition and result in novel ecosystem states (Johnstone et al., 2016).
There is a wealth of research on how tropical forest communities respond to physical disturbance and climate variability (Brokaw and Walker, 1991; Zimmerman et al., 1994, 2021; Canham et al., 2010; Uriarte et al., 2012, 2016). Most studies, however, tend to focus on only one disturbance event – such as plant community recovery following hurricane disturbance in Puerto Rico (see summaries by Brokaw and Walker, 1991; Zimmerman et al., 2021). This body of research has provided valuable insights regarding which tree species are at highest risk (Zimmerman et al., 1994; Canham et al., 2010) and how the forest regenerates following a hurricane event (Uriarte et al., 2012). Drought effects on plant communities have also been documented for many ecosystems, including tropical moist forests (Phillips et al., 2010; Corlett, 2016). These studies have shown that drought is generally associated with increased tree mortality (Erfanian et al., 2017), decreased species richness, and subsequent changes in forest composition (Costa et al., 2020).
Conversely, much less research has been conducted on the effects of warming on tropical forest plant communities, even though evidence suggests tropical trees are at certain times already operating above their optimum temperature for photosynthesis and may be nearing a high temperature threshold (Clark et al., 2003; Doughty and Goulden, 2008; Mau et al., 2018; Wood et al., 2019; Carter et al., 2021; Miller et al., 2021). Increased temperatures have been associated with increased flower production in Puerto Rico, but whether this results in increased seed production is yet to be determined (Pau et al., 2013; Uriarte et al., 2016). The relative paucity of data on tropical forest warming effects is in part due to the considerable difficulties involved in experimentally manipulating these forests (Zhou et al., 2013; Cavaleri et al., 2015). Nevertheless, long-term data on understory plant communities have highlighted important changes in seedling dynamics associated with both temperature and precipitation (see meta-analyses by Way and Oren, 2010; Comita et al., 2014; Bachelot et al., 2015; Uriarte et al., 2018). Further, the effects of multiple disturbances (e.g., hurricanes and warming) can be synergistic or antagonistic and thus difficult to predict from the study of single disturbances (e.g., Norby and Luo, 2004). To better understand how plant communities will respond to increased exposure to climate warming and disturbance, there is great value in assessing the effects of climatic events within the context of experimental manipulations that mimic changes in climate, such as the increasing temperatures predicted with climate change.
The Tropical Responses to Altered Climate Experiment (TRACE), a field warming experiment located in northeastern Puerto Rico, offers an ideal site to explore these questions. Since its inception in 2015, TRACE has been exposed to naturally occurring stressors in addition to experimental warming, offering an unprecedented opportunity to assess how varying disturbances affect seedling and sapling dynamics (Figure 1). In 2015, the Caribbean and Central American tropics were enduring the peak of the most severe drought event experienced since 1950 (Herrera and Ault, 2017). Eastern Puerto Rico in particular suffered rainfall deficits of up to 45%, causing water shortages and rationing for more than two million residents (Mote et al., 2017). Drought conditions were even harsher within the Luquillo Mountains, the most biodiverse region in eastern Puerto Rico and where our study took place, with rainfall deficits continuing until late October 2015 (Mote et al., 2017; Gutiérrez-Fonseca et al., 2020). TRACE began warming understory plants and soil in a tropical forest by 4°C higher than ambient in September 2016 (Kimball et al., 2018). During the very active 2017 hurricane season, eastern Puerto Rico was subsequently struck by two major hurricanes, Irma (September 7th, Category 5) and María (September 20th, Category 4). As the strongest hurricane affecting Puerto Rico since 1928, María brought record-breaking rainfall and sustained winds up to 250 km/hr (Keellings and Hernández Ayala, 2019) that had severe effects on tropical forests across the island (Uriarte et al., 2019; Hall et al., 2020). After 1 year of monitoring forest recovery after hurricane disturbance in the absence of experimental warming, TRACE resumed warming once again in September 2018.
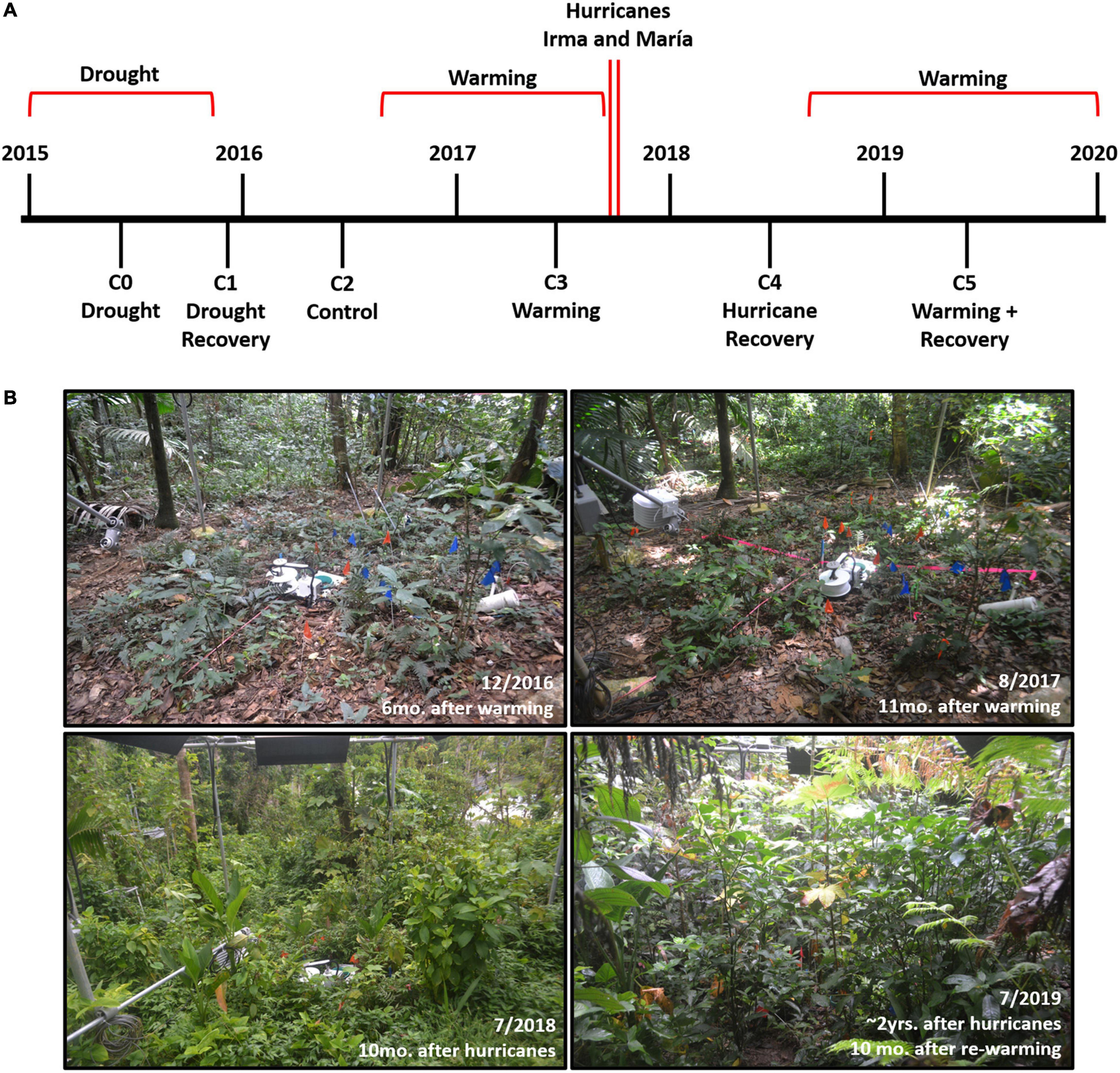
Figure 1. (A) Timeline of disturbance events and seedling and sapling censuses (C0 = June 2015, C1 = November 2015, C2 = June 2016, C3 = June 2017, C4 = May 2018, C5 = April 2019). (B) Changes in the understory plant community observed in one of the TRACE warming plots (Plot 2) from December 2016 to July 2019.
Taken together, the TRACE site experienced a severe drought, two major hurricanes, and for half of the study area (i.e., the heated plots), significantly warmer understory temperatures. Previous research at our study site revealed hurricane-driven changes in herbaceous community composition irrespective of prior warming and drought (Kennard et al., 2020). However, drought and sustained warming induced positive density-dependent controls on seedling mortality, which could accelerate the loss of species diversity (Bachelot et al., 2020). Understanding how climate warming and disturbance affect seedling and sapling communities is particularly important, both because they contribute significantly to carbon cycling and biodiversity in tropical forests, and because they represent the potential forest community of the future (Clark, 2007). As such, we take advantage of this unique combination of events to explore the following questions: (1) how do drought, hurricanes, and warming affect seedling and sapling richness, diversity, and species composition? (2) which species demonstrate a stronger response to drought, hurricanes, and warming across ontogeny? and (3) do these observed changes alter the functional composition of the forest?
We predicted that drought would lead to a decrease in richness and diversity as well as a significant shift in plant community composition, with a functional shift toward drought-resistant strategies with high water storage capacity and thus higher leaf succulence (von Willert et al., 1992; Uriarte et al., 2018). We predicted that hurricanes would also lead to a decrease in richness and diversity as well as a significant shift in plant community composition, with a functional shift toward fast-growing species (Zimmerman et al., 1994). Finally, we predicted that experimental warming would be associated with a marked loss in richness and diversity (Bachelot et al., 2020) as well as a significant shift in plant community composition, with a functional shift toward fast-growing strategies (Way and Oren, 2010). Plant sensitivity to biotic and abiotic factors shift throughout ontogeny, in part due to changes in functional traits (Lasky et al., 2015). As such, we also predicted there would be differences in seedling and sapling responses to stressors, with higher effects on seedling composition and abundance relative to saplings, since the dynamics of seedlings are strongly altered by abiotic and biotic conditions. While changes in plant community assembly are often observed over long timeframes (Lasky et al., 2014), given the number and severity of stressors observed in a relatively short period (5 years), results from this study provide key insights into the initial vulnerability of a tropical forest to disturbance under a changing climate.
Materials and methods
Study site
This study was conducted in the Luquillo Experimental Forest (LEF; 18°18′N, 65°50′W) in northeastern Puerto Rico. Categorized as a subtropical wet forest according to the Holdridge Life Zone System (Holdridge, 1967), this site holds the TRACE experimental plots, which were established in 2015 in a secondary forest that has regenerated naturally after agricultural development during the first half of the 20th century (Kimball et al., 2018). Located at 100 m elevation, the site contains clay-rich and acidic soils classified as Ultisols (Scatena, 1989), with >60% of root biomass concentrated in the top 10 cm soil layer (Silver and Vogt, 1993; Yaffar and Norby, 2020). In the study area, the dominant canopy trees are Syzygium jambos, Ocotea leucoxylon, and Casearia arborea, and the site is also dominated by the palm Prestoea montana.
Mean annual temperature of the site is 24°C with relatively low seasonal variation, and mean annual rainfall is 3,500 mm (García-Martinó et al., 1996). During the 2015 drought, total precipitation measured at a neighboring site (El Verde Field Station, ∼15 km away) was 48% less than the mean in the previous decade (O’Connell et al., 2018). On September 06, 2017, Hurricane Irma brought >300 mm of rain to the northeast of the island, and just 2 weeks later on September 20, 2017 Hurricane María brought ∼1,500 mm of rain in only 48 h (Hall et al., 2020).
Experimental plots
The understory plant community was evaluated inside each of the six 12 m2 hexagonal TRACE plots (i.e., three heated and three control), which range in slope from 15 to 26° and are separated by at least 10 m. All plots contain naturally occurring understory vegetation but were installed in areas with no adult trees to allow space for experimental equipment. To evaluate the effects of warming on understory plants and soil, three of the plots were warmed with infrared (IR) heaters +4°C above ambient temperatures of the three control plots. Temperatures at the heated plots were constantly controlled to maintain a 4 ± 0.1°C increase in hourly average temperatures compared to control plots, as sensed by IR thermometers (Kimball et al., 2018). The design of the warming system was adapted from Kimball et al. (2008), with six IR heaters (Model Raymax 1010, Watlow Electric Manufacturing Co., St. Louis, MO, United States) per warmed plot installed on crossbars at approximately 3.6 m from the ground and aimed downward to maintain uniform and constant temperatures for long periods of time. Control plots contained the same infrastructure (i.e., concrete footings, posts, and crossbars) but with sheet metal plates the same size as the heaters to control for potential effects of shading and equipment installation. More details on the layout of the experimental site, infrastructure of the plots, and success of the warming method can be found in Kimball et al. (2018). Experimental warming began in September 2016, was paused in September 2017 due to the passage of Hurricanes Irma and María, and was resumed in September 2018 after 1 year of monitoring hurricane disturbance effects.
Seedling surveys
All woody seedlings (≥10 cm and <20 cm height) and saplings (≥20 cm height) inside the TRACE experimental plots were initially tagged and identified to species in June 2015 (Census 0; C0), during the peak of the drought. Previously tagged seedlings were reassessed for drought damage in November 2015 (C1), after the rains resumed. The following censuses were conducted in June 2016 (C2; control year, 10 months after the drought ended), June 2017 (C3; 8 months after the warming started), May 2018 (C4; 7 months after the hurricanes, in the absence of warming), and April 2019 (C5; 7 months after warming resumed and 14 months after the hurricanes). Seedlings that surpassed 20 cm height in a census year were reclassified as saplings. Additional details about the seedling surveys can be found in Figure 1A and Bachelot et al. (2020). Raw data from these seedling and sapling surveys is available on the ESS-DIVE data archive at https://data.ess-dive.lbl.gov/datasets/doi:10.15485/1873618.
Functional traits
Functional trait data for the woody tree and shrub species found in our plots were obtained from the Luquillo Forest Dynamics Plot (LFDP) database, which were measured with standard procedures (Cornelissen et al., 2003; Swenson et al., 2012) within the Luquillo Experimental Forest (∼15 km away from our study site; Swenson et al., 2019). We selected functional traits that capture life history differentiation among tree species (Kraft et al., 2010; Wright et al., 2010), which included: wood density (WD; g/cm3), specific leaf area (SLA; cm–2/g1), and leaf succulence (LS; g H20/cm2 leaf area), as well as leaf phosphorous (P; percentage P of oven-dry mass), leaf nitrogen (N; percentage N of oven-dry mass), and leaf carbon (C; percentage C of oven-dry mass) concentrations. Wood density was measured on 10 samples per species using an increment borer in trees of 10–20 cm diameter at 1 m height, or using branch material for smaller trees (Swenson et al., 2012). Leaf traits were measured on 25 samples per species using sun-exposed leaves or on leaves from the crown of large trees (Swenson et al., 2012).
For each treatment (i.e., disturbance type), we calculated the community-weighted means (CWM) of each functional trait by averaging the trait value across all species present in each plot weighted by the number of stems in that same plot. Measuring the CWM trait value is one of the most common approaches for quantifying functional composition at the community level and analyzing trait-environment relationships (Funk et al., 2017). These values were calculated separately for seedlings and saplings, to evaluate changes in CWMs observed along ontogeny.
Statistical analyses
To assess how plant community responses to climate warming and disturbance differed across ontogeny, we conducted all the analyses described below separately for seedlings and saplings. First, we investigated how climatic events influenced broad biodiversity patterns by running linear mixed models of species richness (i.e., number of species) and diversity (i.e., Shannon’s Diversity Index) as a function of treatment (i.e., control, drought, hurricanes, and warming). Since each of the six plots was censused six times from 2015 to 2019, we used plot identity as a random effect to account for repeated measurements. All linear mixed models were run in R (R Core Team, 2020) with the lmerTest package (Kuznetsova et al., 2017), using the Satterthwaite method for calculating degrees of freedom and corresponding p-values from t-tests.
We then investigated how each event was associated with changes in species composition. As heterogeneity in dispersions across groups can alter the results of composition analyses (Anderson and Walsh, 2013), we tested for homogeneity of dispersions in species composition and found no significant differences in dispersions across censuses (seedling: F5,30 = 0.54 and P = 0.77, sapling: F5,30 = 0.11 and P = 0.97) or across treatments (seedling: F3,32 = 0.62 and P = 0.57, sapling: F3,32 = 0.08 and P = 0.94). To detect significant changes in species composition at the beginning and the end of the study period, we used permutational multivariate analysis of variance (PERMANOVA) using data from the first (C0) and last (C5) censuses. Then, to evaluate how variation in plant community composition was explained by time (six census dates) and treatment (i.e., control, drought, hurricanes, and warming), we used distance-based redundancy analysis (RDA; Rao, 1964). We also used PERMANOVAs to investigate changes in species composition following each individual treatment. This enabled us to assess specifically how species composition changed following drought (by comparing composition in all plots in C0 and C2), warming (by comparing composition in all plots in C2 and C3), hurricanes (by comparing composition in all plots in C2 and C4), and warming before and after hurricanes (by comparing composition in the heated plots in C3 and C5). All analyses were run in R (R Core Team, 2020) with the vegan package (Oksanen et al., 2020), using 999 permutations and based on standardized Euclidean dissimilarity matrices of the raw abundances (count of plant individuals) aggregated per plot in a given census (Clarke and Warwick, 2001). We visualized all the results using non-metric multidimensional scaling (NMDS; Kruskal, 1964).
To evaluate how individual species responded to treatments and to predict the consequences for the functional composition of the forest, we used generalized joint attribute modeling (GJAM; Clark et al., 2017) in order to implement a Bayesian predictive trait model (Clark, 2016). This approach models species weights as composition data, using multivariate normal distribution, before using them to predict traits. Specifically, we modeled species weights (wi, a vector of relative species abundances in plot i) of all species found inside the plots as a function of treatment and plot as random effect (contained in the predictor vector xi) using a multivariate normal distribution:
where β is the matrix of coefficients and ∑ the species covariance matrix. Once this first step is achieved, a variable change allows the fitted model to be transformed to traits from which trait predictions can be made. Specific details about the model implementation can be found in Clark et al. (2017). This method allows evaluating changes in species abundances not only in response to the variables of interest (with treatment and plot as random effect to account for repeated measurements) but also to the other species. Since species influence each other, using a multivariate normal distribution is more appropriate than using a linear regression on individual species. Furthermore, this method has been shown to perform better than directly modeling traits (Clark, 2016). To fit the model, we used three chains with 2,000 iterations and 500 burn-ins. Model fit was assessed with predictive checks of both species counts and CWMs of functional traits. All analyses were conducted in R (R Core Team, 2020), using the GJAM package for predictive trait modeling (Clark et al., 2017).
Results
Across the 5 years of data collection, we tagged 5,197 individuals from 50 different tree species. Specifically, we tagged 3,498 individuals from 44 species at the seedling stage, and 1,699 individuals from 47 species at the sapling stage. Seven species were common at the seedling stage with more than 100 tagged individuals and making up more than 80% of the entire seedling cohort: Rourea surinamensis (31% of the seedlings), Guarea guidonia (18%), Psychotria brachiata (11%), Inga vera (7%), Ocotea leucoxylon (6%), Nectandra turbacensis (6%), and Piper glabrescens (5%). Three species were common at the sapling stage with more than 100 tagged individuals and making up 47% of the entire sapling cohort: Psychotria brachiata (26% of the saplings), Piper glabrescens (13%), and Rourea surinamensis (8%). We further describe observed changes in biodiversity, species composition, and functional composition below.
Changes in biodiversity and species composition
To assess changes in broad biodiversity patterns, we evaluated variations in species richness and diversity throughout the study period using linear mixed models. As expected, responses to drought, hurricanes, and experimental warming depended on plant ontogenetic stage (Figure 2). Hurricane disturbance was marginally associated with a decrease in seedling richness (t27 = −2.01, P = 0.055), while seedling diversity showed no significant response to any disturbance type. In contrast, sapling richness (t28 = 3.40, P = 0.002) and diversity (t28 = 3.40, P < 0.001) were positively associated with experimental warming, and sapling diversity was marginally associated with drought (t27 = 1.87, P = 0.072). Full results of linear mixed models can be found in Supplementary Table 1.
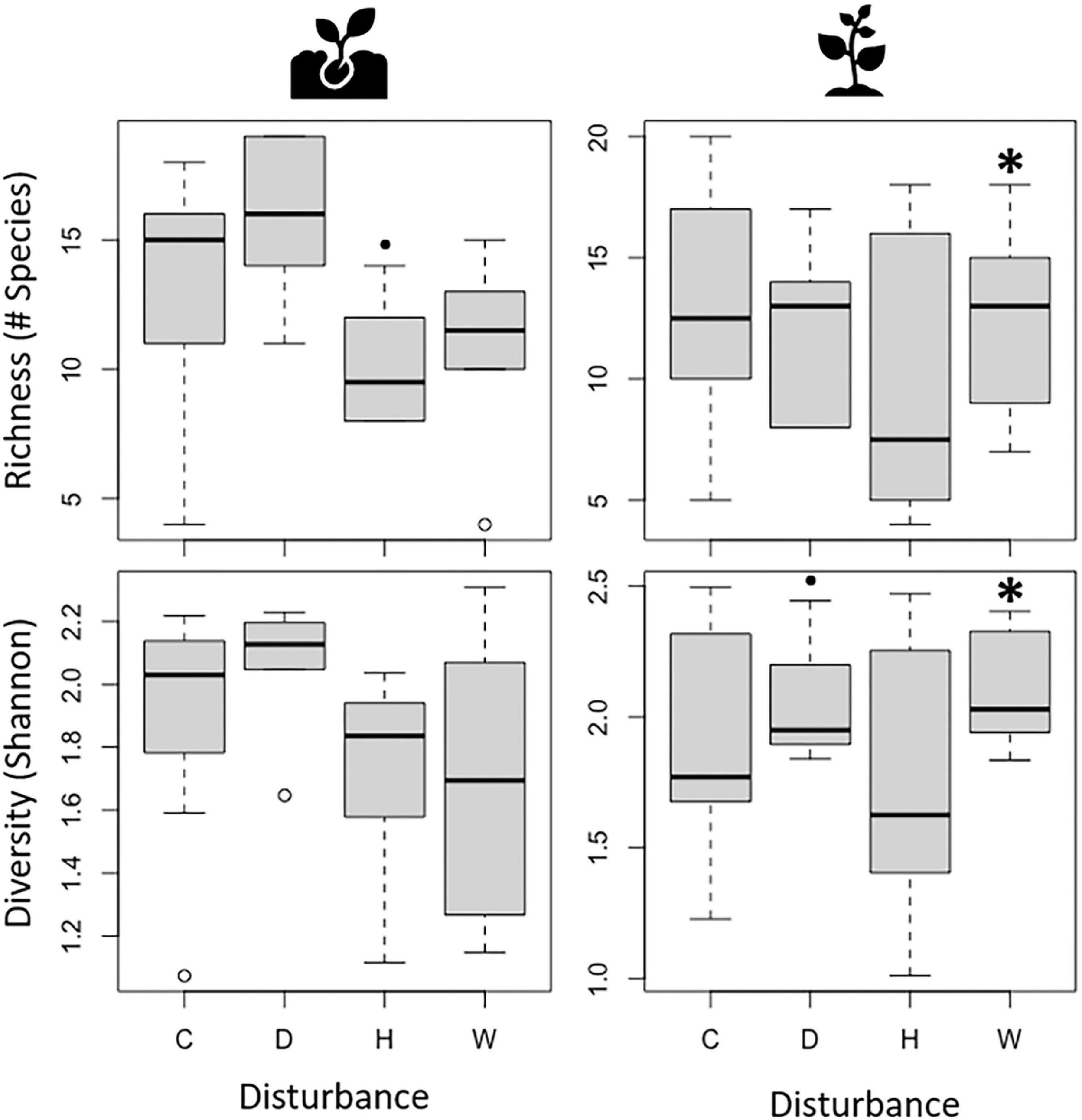
Figure 2. Richness (number of species) and Diversity (Shannon’s diversity index) of seedlings (Left) and saplings (Right) during control (C), drought (D), hurricanes (H), and warming (W). Black asterisks above bars denote significant differences from the control at α = 0.05, while black filled circles denote significant differences at α = 0.1 resulting from Linear Mixed Models.
As expected, seedling and sapling species composition significantly changed between the first and last census (Table 1, History model). However, the effects of disturbance type and time on species composition were not as pronounced (Figure 3). Redundancy analyses showed that 27% of the variation in seedling composition was explained by disturbance and time (RDA, pseudo-F6,29 = 1.82, P = 0.027, Table 1). Specifically, disturbance type, although marginally significant, explained 11% of the variation in seedling composition (pseudo-F3,29 = 1.51, P = 0.103) and time captured 16% of the variation in seedling composition (pseudo-F3,29 = 2.13, P = 0.023). Meanwhile, sapling composition did not show a strong response to disturbance and time (RDA, pseudo-F6,29 = 1.82, P = 0.188, Table 1). When evaluating the individual effects of each disturbance type on species composition, we detected no significant effects of drought, hurricanes, or warming on either seedling or sapling composition (Table 1).
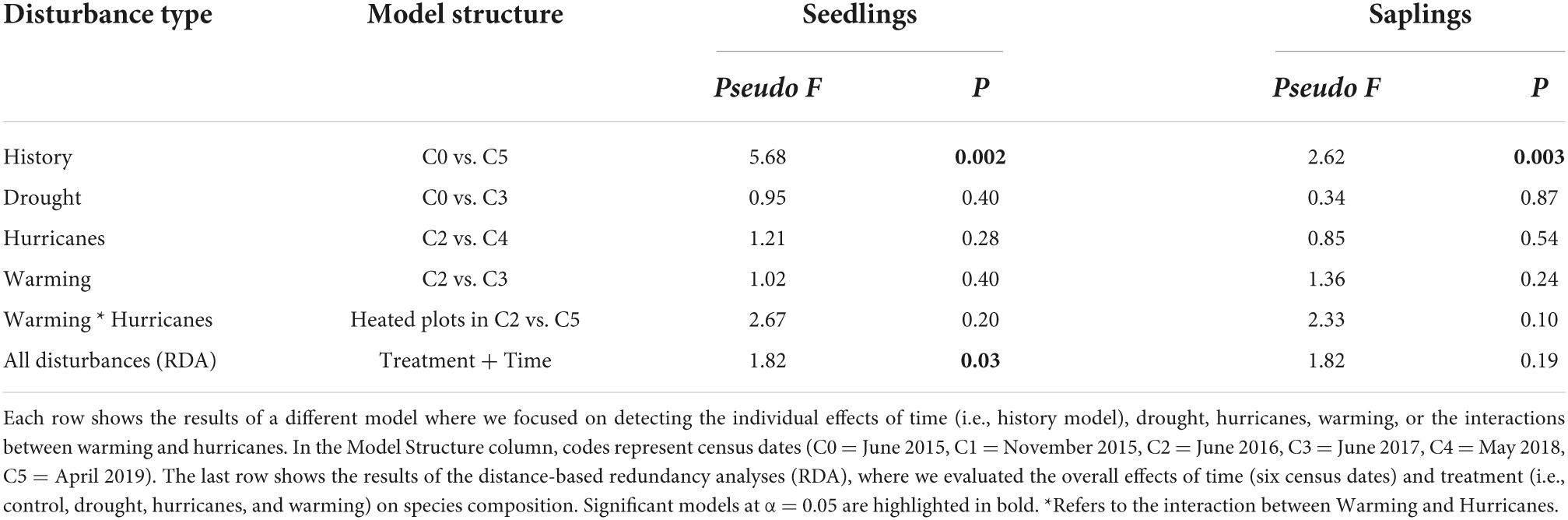
Table 1. Results of permutational multivariate analysis of variance (PERMANOVA) between each disturbance type and its respective control conditions for seedling and sapling species composition.
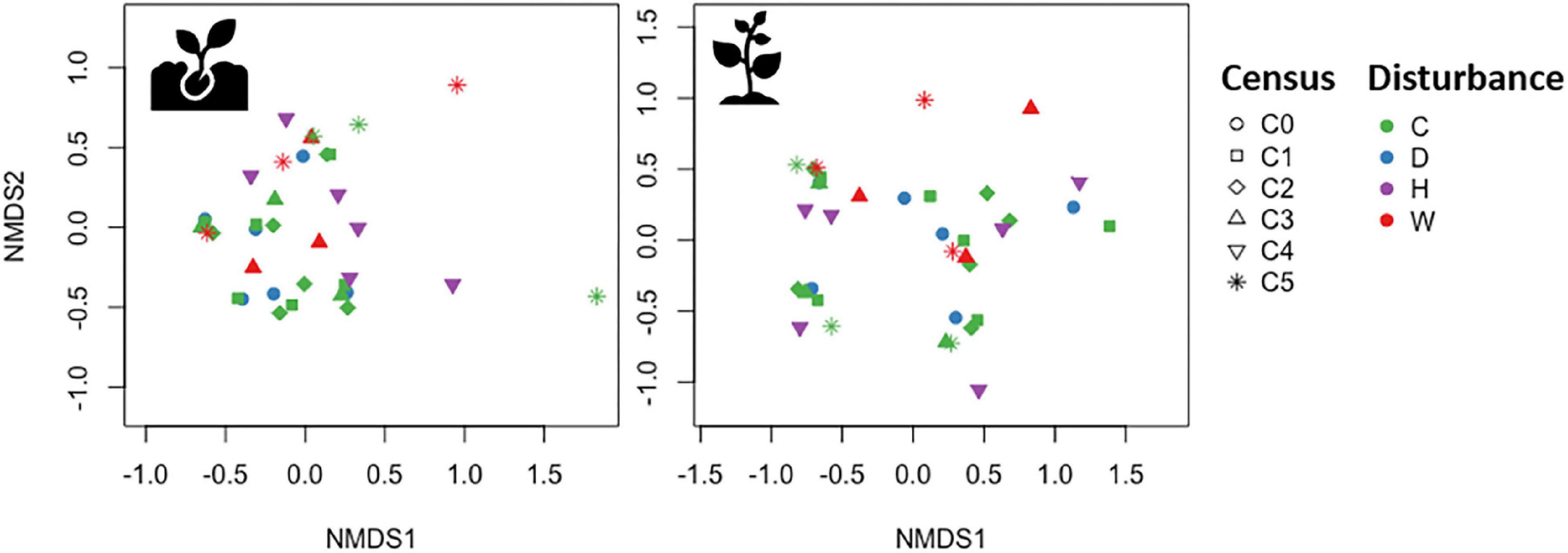
Figure 3. Non-metric multidimensional scaling of seedling (Left) and sapling (Right) species composition. Each symbol represents the species composition in an experimental plot, during a specific census date (symbols; C0 = June 2015, C1 = November 2015, C2 = June 2016, C3 = June 2017, C4 = May 2018, C5 = April 2019) and disturbance type (colors; C = control, D = drought, H = hurricanes, W = warming).
Changes in functional composition
Several species showed significant changes in abundance associated with disturbance at both the seedling and the sapling ontogenetic stages (Figure 4 and Supplementary Table 2). In general, drought and warming were more often associated with higher abundance in both seedlings and saplings, while the effects of hurricanes were more varied (Figure 4). Across the three disturbance types, warming was significantly associated with about twice as many species as drought and hurricanes. Warming resulted in high abundance of six species in the seedling stage and seven species in the sapling stage, and with low abundance of two species in the seedling stage and three species in the sapling stage. In contrast, drought was significantly associated with high abundance of four species and low abundance of one species in the seedling stage and with high abundance of two species and low abundance of one species at the sapling stage. Finally, hurricanes were associated with high abundance of two species in the seedling stage and three species in the sapling stage, and with low abundance of three species in the seedling stage and one species in the sapling stage. These species covered a wide range of life forms and successional status (Table 2).
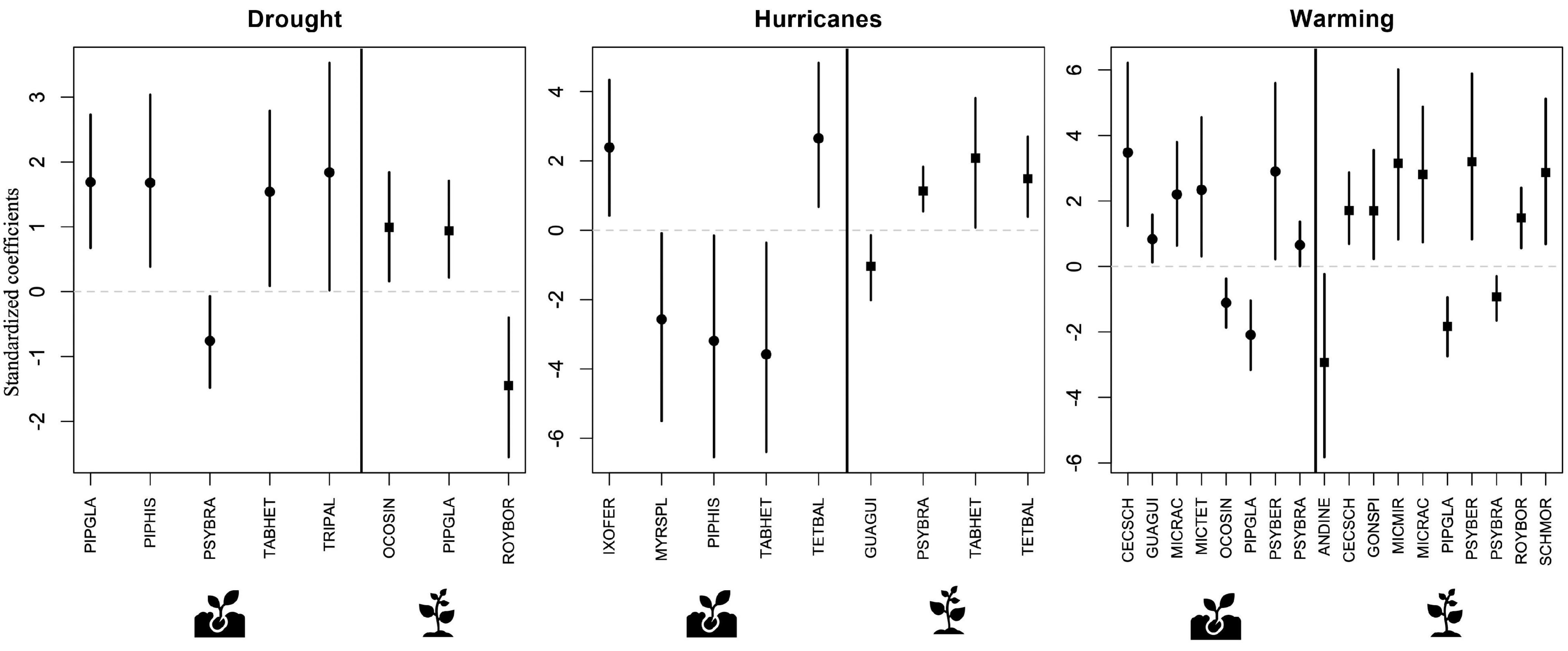
Figure 4. Results of predictive trait modeling, showing species with abundances that were significantly associated with each disturbance type at the seedling (circles) and sapling (squares) ontogenetic stages. In each plot, the vertical solid line separates seedlings from saplings. Lines expand to the 95% credible intervals. Full species name, life form, and successional status can be found on Table 2.
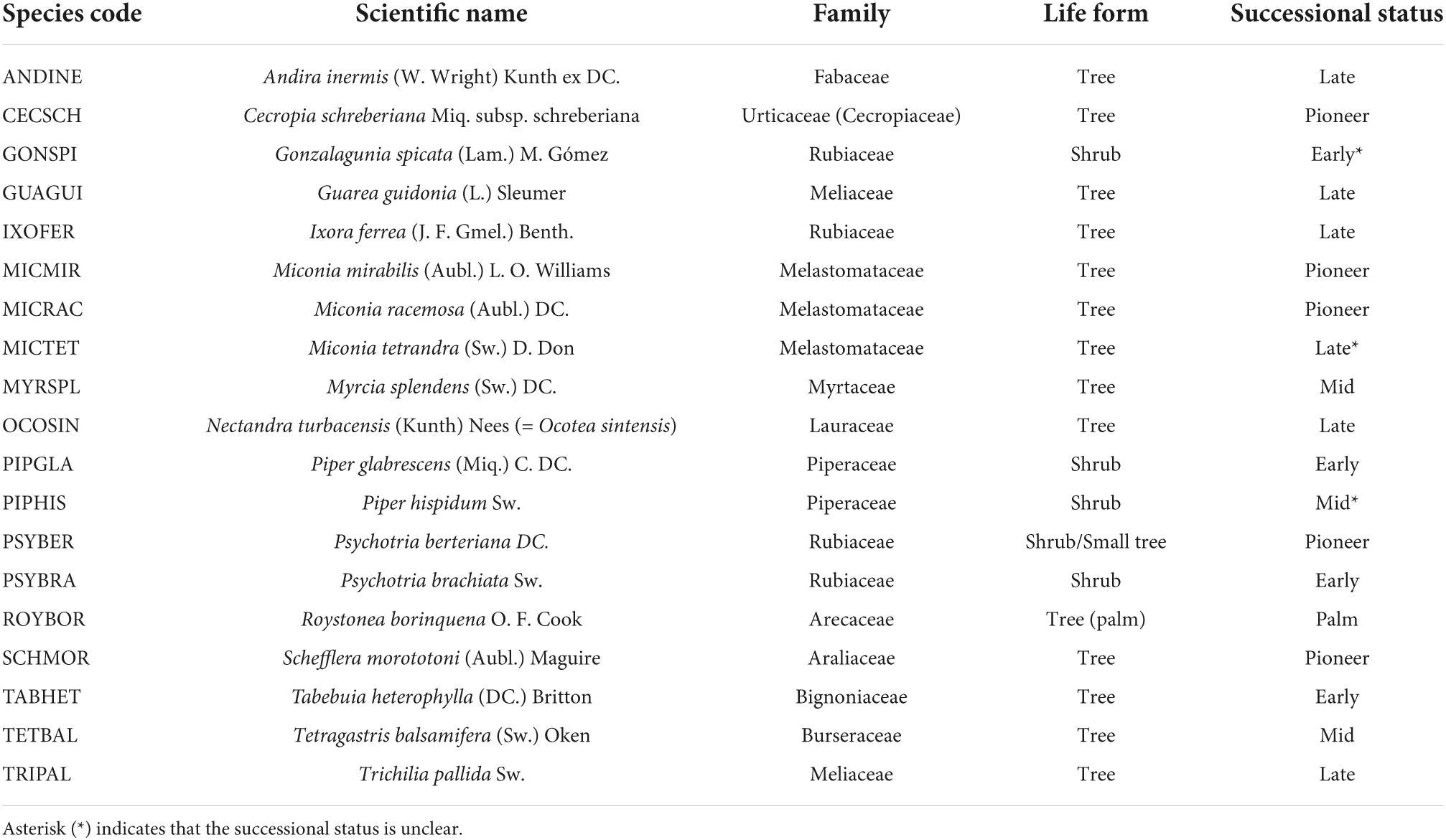
Table 2. Species codes with the full scientific name, family, life form, and successional status of plant species that were significantly associated with drought, hurricanes, or warming (i.e., as shown in Figure 4).
Some of these species demonstrated a significant response to more than one disturbance type. For example, seedlings of one of the most common species in the study area, the early successional shrub Psychotria brachiata (PSYBRA), showed significantly lower abundance during drought but higher abundance under warming conditions. Meanwhile, seedlings of the early successional tree Tabebuia heterophylla (TABHET) showed increased abundance during drought but lower abundance after hurricane disturbance. Observed responses of plant species to the different disturbances also showed variation across ontogenetic stages. In particular, the previously mentioned P. brachiata shrub had higher abundance at the seedling stage but lower abundance at the sapling stage under warming conditions. Lastly, a few other species showed a consistent response to the disturbances across ontogenetic stages, such as the early successional shrub Piper glabrescens (PIPGLA), which showed higher abundance during drought and lower abundance during warming at both the seedling and sapling stages (Figure 4).
We found no disturbance effect on any of the six sapling CWM functional traits for predicted changes in the functional structure of the forest (Figure 5 and Supplementary Table 3). Specifically, drought had no effect on the functional structure of either seedling or sapling communities in our study site. In contrast, hurricane and experimental warming were significantly associated with changes in CWM functional traits of seedling communities (Figure 5). Both the hurricanes and the experimental warming led to an increase in CWM leaf P concentrations and leaf succulence, and a decrease in CWM leaf C concentrations in seedlings. The seedling CWMs for wood density, specific leaf area and leaf N concentrations showed no association to any of the disturbance types included in our study.
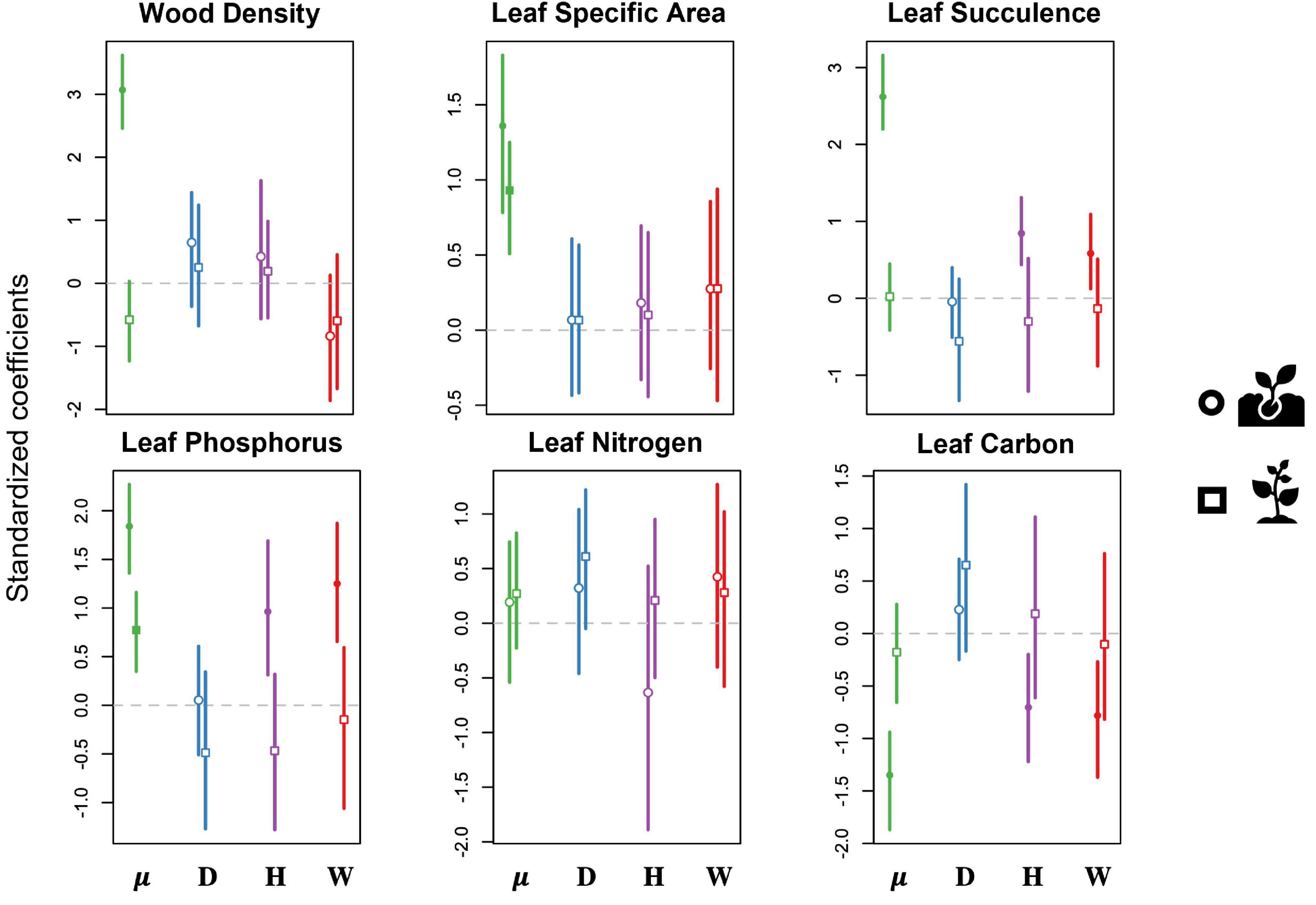
Figure 5. Results of predictive trait modeling, showing the predicted effects of each disturbance type on the community-weighted means of functional traits for both seedlings (circles) and saplings (squares). Lines expand to the 95% credible intervals. Open symbols show non-significant effects (credible intervals overlap with 0), whereas filled symbols indicate significant effects. Data are shown for the intercept (μ, green) and for drought (D, blue), hurricanes (H, purple), and warming (W, red). Traits include wood density (WD; g/cm3), specific leaf area (SLA; cm–2/g1), leaf succulence (LS; g H20/cm2 leaf area), leaf phosphorous (P; percentage P of oven-dry mass), leaf nitrogen (N; percentage N of oven-dry mass), and leaf carbon (C; percentage C of oven-dry mass).
Discussion
In this study, we document the effects of multiple climatic stressors in a tropical forest understory plant community. Within the time frame of this study (2015–2019), seedlings and saplings in the TRACE plots experienced stronger than average natural hazards and significantly warmer temperatures. As such, we anticipated severe losses in biodiversity as well as a shift in species and functional composition of the understory plant community. In stark contrast to our expectations, we observed only a slight reduction in seedling richness after hurricane disturbance, while drought and experimental warming increased sapling diversity. There was also no evidence of pronounced shifts in species composition in response to the various disturbance types, as our analyses detected only minor effects of disturbance and time on seedling composition. Several species showed significant changes in abundance in response to the various stressors, leading to predicted changes in functional composition of these forest sites for the seedling community. Yet the overall lack of strong changes in the understory plant community, despite increased exposure to climatic extremes, highlights the high capacity of these forests to resist environmental stressors and their potential to continue supporting critical ecosystem functions and services in the face of a changing climate.
Minimal effects of disturbance on seedling and sapling communities
Despite the observed shift in species composition between 2015 and 2019, we found few significant changes in seedling and sapling communities specifically associated with drought, hurricanes, and experimental warming. This result suggests that the woody understory might be more resistant to climate warming and disturbance than expected. As mentioned in previous studies, plant communities in this forest may have already developed pre-existing tolerance to a wide range of stressors over a long history of repeated disturbance events, increasing their resistance and resilience through time (Ostertag et al., 2005; Zimmerman et al., 2020, 2021). Alternatively, the lack of marked shifts in seedling and sapling communities associated with these disturbances might suggest lagged responses that take longer to emerge (Xie et al., 2020).
Tropical seedlings have been shown to be sensitive to water availability (Bunker and Carson, 2005; Slot and Poorter, 2007; Álvarez-Yépiz et al., 2018; Uriarte et al., 2018). However, the effects of drought on seedlings have mostly been assessed with experimental manipulations, which may simulate more extreme conditions than those observed in nature and thus exacerbate seedling responses to water stress. In addition, controlled experiments might obscure the balancing effects of other natural occurring mechanisms, such as indirect effects on soil pathogens and mycorrhizal associations that influence seedling survival (Liang et al., 2015; Bachelot et al., 2020). Observational studies provide more realistic data for understanding these cross-trophic dynamics, but extreme climatic events are unpredictable and often with long return intervals (Scatena and Larsen, 1991; Boose et al., 2004). Further, the results may be confounded by other biotic or abiotic components of the ecosystem (Soong et al., 2020).
Our results show a slight increase in sapling diversity in response to drought. In the TRACE site, Bachelot et al. (2020) found higher seedling growth and survival during the 2015 drought when compared to other censuses, which could in turn influence sapling diversity. However, given that we have no data prior to the drought, we were unable to assess the drought response with respect to the baseline. Thus, the survey in the drought year recorded late-stage effects of the drought and might represent an assessment of seedlings and saplings that already possessed high fitness and drought tolerance (Zimmerman et al., 2021).
Tropical forests in Puerto Rico have shown a high degree of resistance and resilience to hurricanes (Beard et al., 2005; Zimmerman et al., 2021). Yet Hurricane María’s combined extreme precipitation and strong winds caused significantly higher stem breakage and tree mortality than Hurricanes Hugo (1989) and Georges (1998) in the Luquillo Experimental Forest (Uriarte et al., 2019). Despite the unprecedented strength of this storm, we only found a mild reduction in seedling richness following the hurricanes, and no effects on diversity or species composition. Reductions in seedling richness might have been caused by the significant increase in herbaceous cover observed after the hurricanes within the TRACE plots (Kennard et al., 2020), potentially leading to an increase in intra and interspecific competition for resources. In the initial years following a hurricane, tropical forest understory herbs, shrubs, and newly recruited tree saplings tend to increase in abundance due to higher light availability (Zimmerman et al., 2021). However, as canopy cover increases through successional time, we can expect a reduction in herbaceous cover, leaving space for shade-tolerant woody species to grow and recover (Scatena et al., 1996; Chinea, 1999; Walker and Sharpe, 2010).
Species richness and diversity of saplings, but not seedlings, increased in response to warmer temperatures. This finding contrasts with our expectation that temperature would negatively affect tropical plant diversity (Stork et al., 2009; Wright et al., 2009). Warmer temperatures might be selecting for pioneer species that thrive in hotter conditions, leaving the forest in a transition phase comprised of a mix of species where species that are not doing well have yet to die out (Connell, 1978; Corlett, 2011). The TRACE site is also a secondary forest with a long history of climatic and anthropogenic disturbance (Kimball et al., 2018). Secondary forests have been shown to retain high levels of late successional species, possibly in response to environmental filtering that has selected for species that do well under a range of conditions (e.g., Chazdon et al., 2009). Species composition in our study did not change in response to warming, which supports the idea that, while there is a shift in diversity of the saplings, the community is relatively resistant to change. Yet recent evidence of a decrease in root production and slower root recovery after hurricane disturbance in the TRACE heated plots points to complex interactive effects of warming and disturbance that could affect biodiversity patterns in the long-term (Yaffar et al., 2021). As such, whether this resistance continues under persistent and longer-term warming is yet to be determined.
Responses to disturbance were variable across ontogeny
Seedling and sapling communities differed in their responses to the various climatic stressors assessed in our study. This was expected, as ontogeny alters many ecological processes that influence plant demographics (Lasky et al., 2015) and responses to environmental change (Comita et al., 2009; Fortunel et al., 2020). In the case of drought, we only found a marginal increase in sapling richness and a significant response from a few species at the seedling and sapling stage. The abundance of P. glabrescens, an early successional shrub, was significantly higher during the drought at both the seedling and sapling stages. This result is in line with the theory that shrubs in Puerto Rico might have adapted to a wide range of soil moisture conditions (Zimmerman et al., 2021). However, the abundance of another early successional shrub species, P. brachiata, was reduced at the seedling stage during the drought. This range of results emphasizes the need to understand the functional characteristics of each plant species.
Hurricane disturbance had varied effects on seedling and sapling communities. Only seedling richness was negatively affected by hurricane disturbance, which could suggest that seedlings are more sensitive than saplings due to their lower belowground biomass or higher desiccation potential (Uriarte et al., 2005). Previous studies have highlighted life history differentiation amongst tropical woody species that result in varied susceptibility to hurricanes (Zimmerman et al., 1994; Walker et al., 2003; Canham et al., 2010). These studies support our results of both positive and negative effects of hurricane disturbance on different species. For example, the early-mid successional shrubs P. brachiata and Piper hispidum showed contrasting responses to hurricanes as well as drought, which points to niche differentiation (Connell, 1978; Uriarte et al., 2004). Heterogeneity in the severity and timing of disturbance might enable these two competing shrubs to coexist in the understory.
To date, TRACE is the only field-scale in situ warming experiment of understory plants in a tropical forest ecosystem (Kimball et al., 2018). As such, knowledge of how increased temperatures might affect seedling and sapling dynamics in tropical forests is still in its infancy, which is critical to predict future global carbon balance (Clark, 2004; Reed et al., 2012; Zuidema et al., 2013). Previous studies have shown high thermal tolerance during juvenile growth (Cheesman and Winter, 2013; Slot and Winter, 2018). Similarly, we found stronger effects of warming on sapling rather than seedling biodiversity, with species showing both positive and negative responses to warming. In particular, P. brachiata responded positively to warming at the seedling stage but negatively at the sapling stage, while P. glabrescens responded negatively to warming at both the seedling and sapling stages. After 1 year of warming prior to the hurricanes, P. brachiata demonstrated greater photosynthetic thermal acclimation capacity than P. glabrescens (Carter et al., 2020). The differing capacity of these understory shrubs to physiologically acclimate to warming could explain why the abundance of P. brachiata saplings increased with warmer temperatures, while the abundance of P. glabrescens did not. Finally, this variability in species responses to climate warming and disturbance can act as a mechanism to maintain species biodiversity through non-linearity (Chesson, 2000; Bachelot and Lee, 2020).
Evidence of functional shift in woody understory community following disturbance
Overall, our finding of species-specific effects of disturbance suggests that the functional composition of the forest might eventually change. In other words, disturbance-driven changes in species abundance were predicted to significantly influence the functional composition of seedling and sapling communities. We predicted that drought would lead to higher leaf succulence (i.e., higher water storage capacity), while warming and hurricanes would lead to the prevalence of pioneer and early successional species with fast-growing strategies. Contrary to our expectations, however, we found no change in leaf succulence, or any other functional trait, associated with drought. This result could reflect the lack of pre-drought data on the woody composition, such that the community already reflects traits that are best adapted to drought.
Previous studies have shown that tropical forest seedlings and saplings experience drastically different environments following a hurricane, including increased water input more similar in chemistry to rainfall when compared with water that has passed through a closed canopy forest (Heartsill-Scalley et al., 2007). However, despite the increased water input, forests recovering from hurricane disturbance tend to have drier litter layers with slower decomposition rates due to increased temperature and solar radiation (González et al., 2014), which could in turn increase water stress and select for seedling communities with higher leaf succulence, as we observed. Similar to prior studies that have followed recruitment after hurricane disturbance (Zimmerman et al., 1994), we also found a shift toward fast-growing strategy traits such as low leaf carbon and high leaf phosphorus concentrations at the seedling stage. Overall, our findings support our hypothesis that hurricanes would shift the understory plant community toward fast-growing or drought-tolerant species, at least at the seedling ontogenetic stage. Specifically, our results show two distinct shifts through time (Reich, 2014) – water stress immediately after the hurricanes selects for drought-resistant species, while subsequent changes in the environment favor the recruitment of fast-growing species (Zimmerman et al., 1994).
Surprisingly, many more species were significantly influenced by warming when compared with drought and hurricanes, and most responded with an increase in abundance. As expected, most of these favored species were pioneer trees and shrubs such as Cecropia schreberiana, Miconia racemosa, and Psychotria berteriana. As a result, we found a shift toward low leaf carbon and high leaf phosphorus, which is consistent with a community shifting toward fast-growing strategies (Strauss-Deberiedetti and Bazzaz, 1996; Bonal et al., 2007). Similar to the functional shifts observed after the hurricane, warming also favored drought-resistant species with high leaf succulence, which tend to be slow-growing species (Reich, 2014). The selection of contrasting strategies might be the result of the short length of our study – warming favors fast-growing strategies in the short-term, but drought-tolerant species with conservative strategies might be favored under prolonged warming stress. While we did observe these shifts for the seedlings, there was no significant change in CWM of functional traits for the saplings in response to warming. Together, this might suggest that significant environmental filtering is occurring at the seedling stage (Lebrija-Trejos et al., 2010), while prevailing shrubs already possess traits that are responsive to changing environmental conditions.
Conclusion
Overall, we found that both the seedling and sapling communities exhibited strong resistance to a wide range of climatic stressors in this forest. However, it is possible that multiple disturbance legacies and interactions are confounding our results, making it difficult to decouple the effects of each of these events on the understory plant community. When we examine responses at the individual and functional level, we see that both warming and drought generally had positive effects on species richness and diversity, with drought having a stronger influence on the seedling and warming on the sapling ontogenetic stages. Despite repeated hurricane disturbance in this forest, the hurricanes had an overall negative effect on richness and diversity, with more species doing poorly under these conditions. Taken together, these findings suggest that past disturbances may have already selected for a range of species that are better adapted to shocks and stressors, with different species being “released” depending on the environmental change. Longer-term monitoring of seedling and sapling dynamics in this forest is needed to understand the long-term implications of repeated disturbances in a warmer world. However, disturbance-driven changes in species dominance and functional structure suggest that longer-term and repeated disturbance will ultimately shift the community composition of this forest, particularly if precipitation declines, temperature continues rising, and hurricane disturbance becomes more frequent and severe in the tropics.
Data availability statement
The datasets presented in this study can be found in online repositories. The names of the repository/repositories and accession number(s) can be found below: ESS-DIVE Data Archives https://data.ess-dive.lbl.gov/datasets/doi:10.15485/1873618.
Author contributions
TEW, MAC, and SCR contributed to the conception and design of the warming experiment, while BB developed and oversaw the seedling study. TEW and AMA-R managed project operations on site. BB, AMA-R, and JT-D conducted and supervised field work and data curation. BB performed the statistical analysis. AMA-R wrote the first draft, while BB and TEW wrote sections of the manuscript. All authors contributed to manuscript revision, read, and approved the submitted version.
Funding
Funding for this research was provided by United States Department of Energy award numbers DE-SC-0012000, DE-SC-0011806, DE-SC-0008168, 89243018S-SC-000014, DE-SC0022095, and DE-SC-0018942. Additional funding was provided by the National Science Foundation awards DEB 1754713, DEB 2120085, and the Long Term Research in Environmental Biology (LTREB) award DEB-1754435.
Acknowledgments
We are very grateful to the many TRACE interns and volunteers that participated in the seedling surveys, and to Kelsey Carter for support with sample processing. We also thank the Luquillo Long-term Ecological Research (LUQ-LTER) program for providing invaluable field assistance as well as access to relevant field protocols and plant identification keys. The USDA Forest Service’s International Institute of Tropical Forestry (IITF) and University of Puerto Rico-Río Piedras provided additional support. All research at IITF is done in collaboration with the University of Puerto Rico. SCR was also supported by the United States Geological Survey Ecosystems Mission Area. Any use of trade, firm, or product names is for descriptive purposes only and does not imply endorsement by the United States Government.
Conflict of interest
The authors declare that the research was conducted in the absence of any commercial or financial relationships that could be construed as a potential conflict of interest.
Publisher’s note
All claims expressed in this article are solely those of the authors and do not necessarily represent those of their affiliated organizations, or those of the publisher, the editors and the reviewers. Any product that may be evaluated in this article, or claim that may be made by its manufacturer, is not guaranteed or endorsed by the publisher.
Supplementary material
The Supplementary Material for this article can be found online at: https://www.frontiersin.org/articles/10.3389/ffgc.2022.733967/full#supplementary-material
References
Álvarez-Yépiz, J. C., Martínez-Yrízar, A., and Fredericksen, T. S. (2018). Special issue: resilience of tropical dry forests to extreme disturbance events. For. Ecol. Manag. 426, 1–6. doi: 10.1016/j.foreco.2018.05.067
Anderson, M. J., and Walsh, D. C. (2013). PERMANOVA, ANOSIM, and the Mantel test in the face of heterogeneous dispersions: what null hypothesis are you testing? Ecol. Monogr. 83, 557–574. doi: 10.1890/12-2010.1
Bachelot, B., Alonso-Rodríguez, A. M., Aldrich-Wolfe, L., Cavaleri, M. A., Reed, S. C., and Wood, T. E. (2020). Altered climate leads to positive density-dependent feedbacks in a tropical wet forest. Glob. Change Biol. 26, 3417–3428. doi: 10.1111/gcb.15087
Bachelot, B., and Lee, C. T. (2020). Disturbances can promote and hinder coexistence of competitors in ongoing partner choice mutualisms. Am. Nat. 195, 445–462. doi: 10.1086/707258
Bachelot, B., Kobe, R. K., and Vriesendorp, C. (2015). Negative density-dependent mortality varies over time in a wet tropical forest, advantaging rare species, common species, or no species. Oecologia 179, 853–861. doi: 10.1007/s00442-015-3402-7
Beard, K. H., Vogt, K. A., Vogt, D. J., Scatena, F. N., Covich, A. P., Sigurdardottir, R., et al. (2005). Structural and functional responses of a subtropical forest to 10 years of hurricanes and droughts. Ecol. Monogr. 75, 345–361. doi: 10.1890/04-1114
Bonal, D., Born, C., Brechet, C., Coste, S., Marcon, E., Roggy, J. C., et al. (2007). The successional status of tropical rainforest tree species is associated with differences in leaf carbon isotope discrimination and functional traits. Ann. For. Sci. 64, 169–176. doi: 10.1051/forest:2006101
Boose, E. R., Serrano, M. I., and Foster, D. R. (2004). Landscape and regional impacts of hurricanes in Puerto Rico. Ecol. Monogr. 74, 335–352. doi: 10.1890/02-4057
Brokaw, N. V. L., and Walker, L. R. (1991). Summary of the effects of Caribbean hurricanes on vegetation. Biotropica 23, 442–447. doi: 10.2307/2388264
Bunker, D. E., and Carson, W. P. (2005). Drought stress and tropical forest woody seedlings: effect on community structure and composition. J. Ecol. 93, 794–806. doi: 10.1111/j.1365-2745.2005.01019.x
Canham, C. D., Thompson, J., Zimmerman, J. K., and Uriarte, M. (2010). Variation in susceptibility to hurricane damage as a function of storm intensity in Puerto Rican tree species. Biotropica 42, 87–94. doi: 10.1111/j.1744-7429.2009.00545.x
Carter, K. R., Wood, T. E., Reed, S. C., Butts, K. M., and Cavaleri, M. A. (2021). Experimental warming across a tropical forest canopy height gradient reveals minimal photosynthetic and respiratory acclimation. Plant Cell Environ. 44, 2879–2897. doi: 10.1111/pce.14134
Carter, K. R., Wood, T. E., Reed, S. C., Schwartz, E. C., Reinsel, M. B., Yang, X., et al. (2020). Photosynthetic and respiratory acclimation of understory shrubs in response to in situ experimental warming of a wet tropical forest. Front. For. Glob. Change 3:765–785. doi: 10.3389/ffgc.2020.576320
Cavaleri, M. A., Reed, S. C., Smith, W. K., and Wood, T. E. (2015). Urgent need for warming experiments in tropical forests. Glob. Change Biol. 21, 2111–2121. doi: 10.1111/gcb.12860
Chazdon, R. L., Peres, C. A., Dent, D., Sheil, D., Lugo, A. E., Lamb, D., et al. (2009). The potential for species conservation in tropical secondary forests. Conserv. Biol. 23, 1406–1417. doi: 10.1111/j.1523-1739.2009.01338.x
Cheesman, A. W., and Winter, K. (2013). Elevated night-time temperatures increase growth in seedlings of two tropical pioneer tree species. New Phytol. 197, 1185–1192. doi: 10.1111/nph.12098
Chesson, P. (2000). Mechanisms of maintenance of species diversity. Annu. Rev. Ecol. Syst. 31, 343–366. doi: 10.1146/annurev.ecolsys.31.1.343
Chinea, J. D. (1999). Changes in the herbaceous and vine communities at the Bisley Experimental Watersheds, Puerto Rico, following Hurricane Hugo. Can. J. For. Res. 29, 1433–1437. doi: 10.1139/x99-108
Clark, D. A. (2004). Sources or sinks? The responses of tropical forests to current and future climate and atmospheric composition. Philos. Trans. R. Soc. Lond. B. Biol. Sci. 359, 477–491. doi: 10.1098/rstb.2003.1426
Clark, D. A. (2007). Detecting tropical forests’ responses to global climatic and atmospheric change: current challenges and a way forward. Biotropica 39, 4–19. doi: 10.1111/j.1744-7429.2006.00227.x
Clark, D. A., Piper, S. C., Keeling, C. D., and Clark, D. B. (2003). Tropical rain forest tree growth and atmospheric carbon dynamics linked to interannual temperature variation during 1984-2000. PNAS 100, 5852–5857. doi: 10.1073/pnas.0935903100
Clark, J. S. (2016). Why species tell more about traits than traits about species: predictive analysis. Ecology 97, 1979–1993. doi: 10.1002/ecy.1453
Clark, J. S., Nemergut, D., Seyednasrollah, B., Turner, P. J., and Zhang, S. (2017). Generalized joint attribute modeling for biodiversity analysis: median-zero, multivariate, multifarious data. Ecol. Monogr. 87, 34–56. doi: 10.1002/ecm.1241
Clarke, K. R., and Warwick, R. M. (2001). Change in Marine Communities: An Approach to Statistical Analysis and Interpretation. 2nd Edition. Plymouth: Plymouth Marine Laboratory.
Comita, L. S., Queenborough, S. A., Murphy, S. J., Eck, J. L., Xu, K., Krishnadas, M., et al. (2014). Testing predictions of the Janzen–Connell hypothesis: a meta-analysis of experimental evidence for distance-and density-dependent seed and seedling survival. J. Ecol. 102, 845–856. doi: 10.1111/1365-2745.12232
Comita, L. S., Uriarte, M., Thompson, J., Jonckheere, I., Canham, C. D., and Zimmerman, J. K. (2009). Abiotic and biotic drivers of seedling survival in a hurricane-impacted tropical forest. J. Ecol. 97, 1346–1359. doi: 10.1111/j.1365-2745.2009.01551.x
Connell, J. H. (1978). Diversity in tropical rain forests and coral reefs. Science 199, 1302–1310. doi: 10.1126/science.199.4335.1302
Corlett, R. T. (2011). Impacts of warming on tropical lowland rainforests. Trends Ecol. Evol. 26, 606–613. doi: 10.1016/j.tree.2011.06.015
Corlett, R. T. (2016). The impacts of droughts in tropical forests. Trends Plant Sci. 21, 584–593. doi: 10.1016/j.tplants.2016.02.003
Cornelissen, J. H. C., Lavorel, S., Garnier, E., Díaz, S., Buchmann, N., Gurvich, D. E., et al. (2003). A handbook of protocols for standardised and easy measurement of plant functional traits worldwide. Aust. J. Bot. 51, 335–380. doi: 10.1071/BT02124
Costa, F. R. C., Zuanon, J., Baccaro, F. B., de Almeida, J. S., Menger, J. D. S., Souza, J. L. P., et al. (2020). Effects of climate change on central Amazonian forests: a two decades synthesis of monitoring tropical biodiversity. Oecol. Aust. 24, 317–335. doi: 10.4257/oeco.2020.2402.07
Doughty, C. E., and Goulden, M. L. (2008). Are tropical forests near a high temperature threshold? J. Geophys. Res 113:G00B07. doi: 10.1029/2007JG000632
Emanuel, K. A. (2013). Downscaling CMIP5 climate models shows increased tropical cyclone activity over the 21st century. PNAS 110, 12219–12224. doi: 10.1073/pnas.1301293110
Erfanian, A., Wang, G., and Fomenko, L. (2017). Unprecedented drought over tropical South America in 2016: significantly under-predicted by tropical SST. Sci. Rep. 7:5811. doi: 10.1038/s41598-017-05373-2
Feng, Y., Negrón-Juárez, R. I., and Chambers, J. Q. (2020). Remote sensing and statistical analysis of the effects of hurricane María on the forests of Puerto Rico. Remote Sens. Environ. 247:111940. doi: 10.1016/j.rse.2020.111940
Fortunel, C., Stahl, C., Heuret, P., Nicolini, E., and Baraloto, C. (2020). Disentangling the effects of environment and ontogeny on tree functional dimensions for congeneric species in tropical forests. New Phytol. 226, 385–395. doi: 10.1111/nph.16393
Funk, J. L., Larson, J. E., Ames, G. M., Butterfield, B. J., Cavender-Bares, J., Firn, J., et al. (2017). Revisiting the Holy Grail: using plant functional traits to understand ecological processes. Biol. Rev. 92, 1156–1173. doi: 10.1111/brv.12275
Gallup, S. M., Baker, I. T., Gallup, J. L., Restrepo-Coupe, N., Haynes, K. D., Geyer, N. M., et al. (2021). Accurate simulation of both sensitivity and variability for Amazonian Photosynthesis: is it too much to ask? J. Adv. Model. Earth Syst 13:e2021MS002555. doi: 10.1029/2021MS002555
García-Martinó, A. R., Warner, G. S., Scatena, F. N., and Civco, D. L. (1996). Rainfall, runoff and elevation relationships in the Luquillo Mountains of Puerto Rico. Caribb. J. Sci. 32, 413–424.
González, G., Lodge, D. J., Richardson, B. A., and Richardson, M. J. (2014). A canopy trimming experiment in Puerto Rico: the response of litter decomposition and nutrient release to canopy opening and debris deposition in a subtropical wet forest. For. Ecol. Manag. 332, 32–46. doi: 10.1016/j.foreco.2014.06.024
Grainger, A. (2010). Uncertainty in the construction of global knowledge of tropical forests. Prog. Phys. Geogr. 34, 811–844. doi: 10.1177/0309133310387326
Gutiérrez-Fonseca, P. E., Ramírez, A., Pringle, C. M., Torres, P. J., McDowell, W. H., Covich, A., et al. (2020). When the rainforest dries: drought effects on a montane tropical stream ecosystem in Puerto Rico. Freshw. Sci. 39, 197–212. doi: 10.1086/708808
Hall, J., Muscarella, R., Quebbeman, A., Arellano, G., Thompson, J., Zimmerman, J. K., et al. (2020). Hurricane-induced rainfall is a stronger predictor of tropical forest damage in Puerto Rico than maximum wind speeds. Sci. Rep. 10:4318. doi: 10.1038/s41598-020-61164-2
Heartsill-Scalley, T., Scatena, F. N., Estrada, C., McDowell, W. H., and Lugo, A. E. (2007). Disturbance and long-term patterns of rainfall and throughfall nutrient fluxes in a subtropical wet forest in Puerto Rico. J. Hydrol. 333, 472–485. doi: 10.1016/j.jhydrol.2006.09.019
Henareh Khalyani, A., Gould, W. A., Harmsen, E., Terando, A., Quinones, M., and Collazo, J. A. (2016). Climate change implications for tropical islands: interpolating and interpreting statistically downscaled GCM projections for management and planning. JAMC 55, 265–282. doi: 10.1175/JAMC-D-15-0182.1
Herrera, D., and Ault, T. (2017). Insights from a new high-resolution drought atlas for the Caribbean spanning 1950–2016. J. Clim. 30, 7801–7825. doi: 10.1175/JCLI-D-16-0838.1
Holland, G., and Bruyère, C. L. (2014). Recent intense hurricane response to global climate change. Clim. Dyn. 42, 617–627. doi: 10.1007/s00382-013-1713-0
Johnstone, J. F., Allen, C. D., Franklin, J. F., Frelich, L. E., Harvey, B. J., Higuera, P. E., et al. (2016). Changing disturbance regimes, ecological memory, and forest resilience. Front. Ecol. Environ. 14:369–378. doi: 10.1002/fee.1311
Keellings, D., and Hernández Ayala, J. J. (2019). Extreme rainfall associated with Hurricane Maria over Puerto Rico and its connections to climate variability and change. Geophys. Res. Lett. 46, 2964–2973. doi: 10.1029/2019GL082077
Kennard, D. K., Matlaga, D., Sharpe, J., King, C., Alonso-Rodríguez, A. M., Reed, S. C., et al. (2020). Tropical understory herbaceous community responds more strongly to hurricane disturbance than to experimental warming. Ecol. Evol. 10, 8906–8915. doi: 10.1002/ece3.6589
Kimball, B. A., Alonso-Rodríguez, A. M., Cavaleri, M. A., Reed, S. C., González, G., and Wood, T. E. (2018). Infrared heater system for warming tropical forest understory plants and soils. Ecol. Evol. 8, 1932–1944. doi: 10.1002/ece3.3780
Kimball, B. A., Conley, M. M., Wang, S., Lin, X., Luo, C., Morgan, J., et al. (2008). Infrared heater arrays for warming ecosystem field plots. Glob. Change Biol. 14, 309–320. doi: 10.1111/j.1365-2486.2007.01486.x
Knutson, T. R., Sirutis, J. J., Vecchi, G. A., Garner, S., Zhao, M., Kim, H. S., et al. (2013). Dynamical downscaling projections of twenty-first-century Atlantic hurricane activity: cMIP3 and CMIP5 model-based scenarios. J. Clim. 26, 6591–6617. doi: 10.1175/JCLI-D-12-00539.1
Kraft, N. J. B., Metz, M. R., Condit, R. S., and Chave, J. (2010). The relationship between wood density and mortality in a global tropical forest data set. New Phytol. 188, 1124–1136. doi: 10.1111/j.1469-8137.2010.03444.x
Kruskal, J. B. (1964). Nonmetric multidimensional scaling: a numerical method. Psychometrika 29, 115–129. doi: 10.1007/BF02289694
Kuznetsova, A., Brockhoff, P. B., and Christensen, R. H. B. (2017). lmerTest Package: tests in linear mixed effects models. J. Stat. Softw. 82, 1–26. doi: 10.18637/jss.v082.i13
Lasky, J. R., Bachelot, B., Muscarella, R., Schwartz, N., Forero-Montaña, J., Nytch, C. J., et al. (2015). Ontogenetic shifts in trait-mediated mechanisms of plant community assembly. Ecology 96, 2157–2169. doi: 10.1890/14-1809.1
Lasky, J. R., Uriarte, M., Boukili, V. K., and Chazdon, R. L. (2014). Trait-mediated assembly processes predict successional changes in community diversity of tropical forests. PNAS 111, 5616–5621. doi: 10.1073/pnas.1319342111
Lebrija-Trejos, E., Pérez-García, E. A., Meave, J. A., Bongers, F., and Poorter, L. (2010). Functional traits and environmental filtering drive community assembly in a species-rich tropical system. Ecology 91, 386–398. doi: 10.1890/08-1449.1
Liang, M., Liu, X., Etienne, R. S., Huang, F., Wang, Y., and Yu, S. (2015). Arbuscular mycorrhizal fungi counteract the Janzen-Connell effect of soil pathogens. Ecology 96, 562–574. doi: 10.1890/14-0871.1
Malhi, Y. (2012). The productivity, metabolism and carbon cycle of tropical forest vegetation. J. Ecol. 100, 65–75. doi: 10.1111/j.1365-2745.2011.01916.x
Mau, A. C., Reed, S. C., Wood, T. E., and Cavaleri, M. A. (2018). Temperate and tropical forest canopies are already functioning beyond their thermal thresholds for photosynthesis. Forests 9:47. doi: 10.3390/f9010047
Miller, B. D., Carter, K. C., Reed, S. C., Wood, T. E., and Cavaleri, M. A. (2021). Only sun-lit leaves of the uppermost canopy exceed both air temperature and photosynthetic thermal optima in a wet tropical forest. Agric. For. Meteorol. 301–302, 108347. doi: 10.1016/j.agrformet.2021.108347
Mitchard, E. T. A. (2018). The tropical forest carbon cycle and climate change. Nature 559, 527–534. doi: 10.1038/s41586-018-0300-2
Mote, T. L., Ramseyer, C. A., and Miller, P. W. (2017). The Saharan air layer as an early rainfall season suppressant in the eastern Caribbean: the 2015 Puerto Rico drought. J. Geophys. Res. Atmos. 122, 10966–10982. doi: 10.1002/2017JD026911
Norby, R. J., and Luo, Y. (2004). Evaluating ecosystem responses to rising atmospheric CO2 and global warming in a multi-factor world. New Phytol. 162, 281–293. doi: 10.1111/j.1469-8137.2004.01047.x
O’Connell, C. S., Ruan, L., and Silver, W. L. (2018). Drought drives rapid shifts in tropical rainforest soil biogeochemistry and greenhouse gas emissions. Nat. Commun. 9:1348. doi: 10.1038/s41467-018-03352-3
Oksanen, J., Blanchet, F. G., Friendly, M., Kindt, R., Legendre, P., McGlinn, D., et al. (2020). Vegan: Community Ecology Package. R Package Version 2.5-7. Available Online at: https://CRAN.R-project.org/package=vegan. (accessed February 8, 2021).
Ostertag, R., Silver, W. L., and Lugo, A. E. (2005). Factors affecting mortality and resistance to damage following hurricanes in a rehabilitated subtropical moist forest. Biotropica 37, 16–24. doi: 10.1111/j.1744-7429.2005.04052.x
Pau, S., Wolkovich, E. M., Cook, B. I., Nytch, C. J., Regetz, J., Zimmerman, J. K., et al. (2013). Clouds and temperature drive dynamic changes in tropical flower production. Nat. Clim. Change 3, 838–842. doi: 10.1038/nclimate1934
Phillips, O. L., van der Heijden, G., Lewis, S. L., López-González, G., Aragão, L. E. O. C., Lloyd, J., et al. (2010). Drought–mortality relationships for tropical forests. New Phytol. 187, 631–646. doi: 10.1111/j.1469-8137.2010.03359.x
R Core Team (2020). R: A Language And Environment For Statistical Computing. Vienna: R Foundation for Statistical Computing.
Rao, C. R. (1964). The use and interpretation of principal component analysis in applied research. Sankhya Indian J. Stat. 26, 329–358.
Reed, S. C., Wood, T. E., and Cavaleri, M. A. (2012). Tropical forests in a warming world. New Phytol. 193, 27–29. doi: 10.1111/j.1469-8137.2011.03985.x
Reich, P. B. (2014). The world-wide ‘fast–slow’ plant economics spectrum: a traits manifesto. J. Ecol. 102, 275–301. doi: 10.1111/1365-2745.12211
Scatena, F. N. (1989). An introduction to the Physiography and History of the Bisley Experimental Watersheds in the Luquillo Mountains of Puerto rico. Gen. Tech. Rep. So-72. New Orleans, LA: U.S. Dept. of Agriculture, Forest Service, Southern Forest Experiment Station, 22. doi: 10.2737/SO-GTR-72
Scatena, F. N., and Larsen, M. C. (1991). Physical aspects of hurricane Hugo in Puerto Rico. Biotropica 23, 317–323. doi: 10.2307/2388247
Scatena, F. N., Moya, S., Estrada, C., and Chinea, J. D. (1996). The first five years in the reorganization of aboveground biomass and nutrient use following Hurricane Hugo in the Bisley Experimental Watersheds, Luquillo Experimental Forest, Puerto Rico. Biotropica 28, 424–440. doi: 10.2307/2389086
Silver, W. L., and Vogt, K. A. (1993). Fine root dynamics following single and multiple disturbances in a subtropical wet forest ecosystem. J. Ecol. 81, 729–738. doi: 10.2307/2261670
Slot, M., and Poorter, L. (2007). Diversity of tropical tree seedling responses to drought. Biotropica 39, 683–690. doi: 10.1111/j.1744-7429.2007.00328.x
Slot, M., and Winter, K. (2018). High tolerance of tropical sapling growth and gas exchange to moderate warming. Funct. Ecol. 32, 599–611. doi: 10.1111/1365-2435.13001
Smith, M. D. (2011). An ecological perspective on extreme climatic events: a synthetic definition and framework to guide future research. J. Ecol. 99, 656–663. doi: 10.1111/j.1365-2745.2011.01798.x
Soong, J. L., Janssens, I. A., Grau, O., Margalef, O., Stahl, C., Van Langenhove, L., et al. (2020). Soil properties explain tree growth and mortality, but not biomass, across phosphorous-depleted tropical forests. Sci. Rep. 10:2302. doi: 10.1038/s41598-020-58913-8
Stork, N. E., Coddington, J. A., Colwell, R. K., Chazdon, R. L., Dick, C. W., Peres, C. A., et al. (2009). Vulnerability and resilience of tropical forest species to land-use change. Conserv. Biol. 23, 1438–1447. doi: 10.1111/j.1523-1739.2009.01335.x
Stott, P. (2016). How climate change affects extreme weather events. Science 352, 1517–1518. doi: 10.1126/science.aaf7271
Strauss-Deberiedetti, S., and Bazzaz, F. A. (1996). “Photosynthetic characteristics of tropical trees along successional gradients,” in Tropical Forest Plant Ecophysiology, eds S. S. Mulkey, R. L. Chazdon, and A. P. Smith (Boston, MA: Springer), doi: 10.1007/978-1-4613-1163-8_6
Swenson, N. G., Anglada-Cordero, P., and Barone, J. A. (2019). Data From: Deterministic Tropical Tree Community Turnover: Evidence From Patterns Of Functional Beta Diversity Along An Elevational Gradient. Washington, DC: Dryad. doi: 10.5061/dryad.rh6h5d0
Swenson, N. G., Stegen, J. C., Davies, S. J., Erickson, D. L., Forero-Montaña, J., Hurlbert, A. H., et al. (2012). Temporal turnover in the composition of tropical tree communities: functional determinism and phylogenetic stochasticity. Ecology 93, 490–499. doi: 10.1890/11-1180.1
Ummenhofer, C. C., and Meehl, G. A. (2017). Extreme weather and climate events with ecological relevance: a review. Philos. Trans. R. Soc. Lond. B. Biol. Sci. 372:20160135. doi: 10.1098/rstb.2016.0135
Uriarte, M., Canham, C. D., Thompson, J., and Zimmerman, J. K. (2004). A neighborhood analysis of tree growth and survival in a hurricane-driven tropical forest. Ecol. Monogr. 74, 591–614. doi: 10.1890/03-4031
Uriarte, M., Canham, C. D., Thompson, J., Zimmerman, J. K., and Brokaw, N. (2005). Seedling recruitment in a hurricane-driven tropical forest: light limitation, density-dependence and the spatial distribution of parent trees. J. Ecol. 93, 291–304. doi: 10.1111/j.0022-0477.2005.00984.x
Uriarte, M., Clark, J. S., Zimmerman, J. K., Comita, L. S., Forero-Montaña, J., and Thompson, J. (2012). Multidimensional trade-offs in species responses to disturbance: implications for diversity in a subtropical forest. Ecology 93, 191–205. doi: 10.1890/10-2422.1
Uriarte, M., Muscarella, R., and Zimmerman, J. K. (2018). Environmental heterogeneity and biotic interactions mediate climate impacts on tropical forest regeneration. Glob. Change Biol. 24:e692–e704. doi: 10.1111/gcb.14000
Uriarte, M., Schwartz, N., Powers, J. S., Marín-Spiotta, E., Liao, W., and Werden, L. K. (2016). Impacts of climate variability on tree demography in second growth tropical forests: the importance of regional context for predicting successional trajectories. Biotropica 48, 780–797. doi: 10.1111/btp.12380
Uriarte, M., Thompson, J., and Zimmerman, J. K. (2019). Hurricane María tripled stem breaks and doubled tree mortality relative to other major storms. Nat. Commun. 10:1362. doi: 10.1038/s41467-019-09319-2
von Willert, D. J., Eller, B. M., Werger, M. J., Brinckmann, E., and Ihlenfeldt, H. D. (1992). Life Strategies Of Succulents In Deserts: With Special Reference To The Namib Desert. Cambridge: Cambridge University Press.
Walker, L. R., and Sharpe, J. M. (2010). “Ferns, disturbance and succession,” in Fern Ecology, eds K. Mehltreter, L. R. Walker, and J. M. Sharpe (Cambridge: Cambridge University Press), 177–219. doi: 10.1017/CBO9780511844898.007
Walker, L. R., Lodge, D. J., Guzmán-Grajales, S. M., and Fetcher, N. (2003). Species-specific seedling responses to hurricane disturbance in a Puerto Rican rain forest. Biotropica 35, 472–485. doi: 10.1111/j.1744-7429.2003.tb00604.x
Way, D. A., and Oren, R. (2010). Differential responses to changes in growth temperature between trees from different functional groups and biomes: a review and synthesis of data. Tree Physiol. 30, 669–688. doi: 10.1093/treephys/tpq015
Wood, T. E., Cavaleri, M. A., Giardina, C. P., Khan, S., Mohan, J. E., Nottingham, A. T., et al. (2019). “Soil warming effects on tropical forests with highly weathered soils,” in Ecosystem Consequences of Soil Warming, ed. J. E. Mohan (Cambridge: Academic Press), 385–439. doi: 10.1016/B978-0-12-813493-1.00015-6
Wright, S. J., Kitajima, K., Kraft, N. J. B., Reich, P. B., Wright, I. J., Bunker, D. E., et al. (2010). Functional traits and the growth–mortality trade-off in tropical trees. Ecology 91, 3664–3674. doi: 10.1890/09-2335.1
Wright, S. J., Muller-Landau, H. C., and Schipper, J. (2009). The future of tropical species on a warmer planet. Conserv. Biol 23, 1418–1426. doi: 10.1111/j.1523-1739.2009.01337.x
Xie, X., Li, A., Tan, J., Lei, G., Jin, H., and Zhang, Z. (2020). Uncertainty analysis of multiple global GPP datasets in characterizing the lagged effect of drought on photosynthesis. Ecol. Indic. 113:106224. doi: 10.1016/j.ecolind.2020.106224
Yaffar, D., and Norby, R. J. (2020). A historical and comparative review of 50 years of root data collection in Puerto Rico. Biotropica 52, 563–576. doi: 10.1111/btp.12771
Yaffar, D., Wood, T. E., Reed, S. C., Branoff, B. L., Cavaleri, M. A., and Norby, R. J. (2021). Experimental warming and its legacy effects on root dynamics following two hurricane disturbances in a wet tropical forest. Glob. Change Biol. 27, 6423–6435. doi: 10.1111/gcb.15870
Zhou, X., Fu, Y., Zhou, L., Li, B., and Luo, Y. (2013). An imperative need for global change research in tropical forests. Tree Physiol. 33, 903–912. doi: 10.1093/treephys/tpt064
Zimmerman, J. K., Everham, E. M. III, Waide, R. B., Lodge, D. J., Taylor, C. M., and Brokaw, N. V. L. (1994). Responses of tree species to hurricane winds in subtropical wet forest in Puerto Rico: implications for tropical tree life histories. J. Ecol. 82, 911–922. doi: 10.2307/2261454
Zimmerman, J. K., Willig, M. R., and Hernández-Delgado, E. A. (2020). Resistance, resilience, and vulnerability of social-ecological systems to hurricanes in Puerto Rico. Ecosphere 11:e03159. doi: 10.1002/ecs2.3159
Zimmerman, J. K., Wood, T. E., González, G., Ramirez, A., Silver, W. L., Uriarte, M., et al. (2021). Disturbance and resilience in the Luquillo Experimental Forest. Biol. Conserv. 253:108891. doi: 10.1016/j.biocon.2020.108891
Keywords: tropical forest, global warming, climate change, drought, hurricane, plant functional traits, ontogeny, TRACE
Citation: Alonso-Rodríguez AM, Wood TE, Torres-Díaz J, Cavaleri MA, Reed SC and Bachelot B (2022) Understory plant communities show resistance to drought, hurricanes, and experimental warming in a wet tropical forest. Front. For. Glob. Change 5:733967. doi: 10.3389/ffgc.2022.733967
Received: 30 June 2021; Accepted: 28 June 2022;
Published: 27 July 2022.
Edited by:
Tomas Ferreira Domingues, University of São Paulo, BrazilReviewed by:
Florian Hofhansl, International Institute for Applied Systems Analysis (IIASA), AustriaLuciana F. Alves, University of California, Los Angeles, United States
Copyright © 2022 Alonso-Rodríguez, Wood, Torres-Díaz, Cavaleri, Reed and Bachelot. This is an open-access article distributed under the terms of the Creative Commons Attribution License (CC BY). The use, distribution or reproduction in other forums is permitted, provided the original author(s) and the copyright owner(s) are credited and that the original publication in this journal is cited, in accordance with accepted academic practice. No use, distribution or reproduction is permitted which does not comply with these terms.
*Correspondence: Aura M. Alonso-Rodríguez, aalonsor@uvm.edu
†ORCID: Aura M. Alonso-Rodríguez, 0000-0002-6500-9503; Tana E. Wood, 0000-0001-6322-6224; Molly A. Cavaleri, 0000-0003-0984-611X; Sasha C. Reed, 0000-0002-8597-8619; Benedicte Bachelot, 0000-0003-3348-9757