Dynamics of Soil CO2 Efflux and Vertical CO2 Production in a European Beech and a Scots Pine Forest
- 1Working Group “Ecosystem Modelling”, Research Platform “Data Analysis & Simulation”, Leibniz Centre for Agricultural Landscape Research (ZALF), Müncheberg, Germany
- 2Working Group “Microbial Biogeochemistry”, Research Area 1 “Landscape Functioning”, Leibniz Centre for Agricultural Landscape Research (ZALF), Müncheberg, Germany
- 3Department Soil and Environment, Forest Research Institute of Baden-Württemberg (FVA), Freiburg im Breisgau, Germany
- 4Soil Ecology, University of Freiburg, Freiburg im Breisgau, Germany
- 5Working Group “Hydropedology”, Research Area 1 “Landscape Functioning”, Leibniz Centre for Agricultural Landscape Research (ZALF), Müncheberg, Germany
The conversion of coniferous forest to deciduous forest is accompanied by changes in the vertical distribution of fine roots and soil organic carbon (SOC) content. It is unclear how these changes affect soil CO2 efflux and vertical soil CO2 production, considering changing climate. Here, we present the results of a 6-year study on CO2 efflux, covering relatively warm-dry and cool-wet years. A combination of the flux-gradient method and closed chamber measurements was used to study the CO2 efflux and the vertical distribution of soil CO2 production in a beech (Fagus sylvatica L.) and a pine (Pinus sylvestris L.) forest in northeast Germany. We observed, on average, similar CO2 efflux with 517 (±126) and 559 (±78) g C m–2 a–1 for the beech site and the pine site, respectively. CO2 efflux at the beech site exceeded that at the pine site during the wet year 2017, whereas in dry years, the opposite was the case. Water availability as indicated by precipitation was the primary determining long-term factor of CO2 efflux, whereas seasonal variation was mainly affected by soil temperature, and—in the case of beech—additionally by soil water content. CO2 efflux decreased more dramatically (-43%) at the beech site than at the pine site (-22%) during the warm-dry year 2018 compared to the cool-wet year 2017. We assumed that drought reduces heterotrophic respiration (Rh) at both sites, but additionally decreases autotrophic respiration (Ra) at the beech stand. Soil CO2 production at the beech site ranged over a greater soil depth than at the pine site, attributed to different fine root distribution. The organic layer and the A horizon contributed 47 and 68% of total CO2 efflux at the beech site and the pine site, respectively. The seasonal patterns of different CO2 efflux between both sites were assumed to relate to different phases of tree physiological activity of deciduous compared to evergreen tree species.
Introduction
Forest ecosystems store more than 80% of all terrestrial aboveground carbon (C) and more than 70% of all soil organic carbon (SOC) (Six et al., 2002). In other words, significant amounts of carbon are fixed in forest soils, representing an essential source or sink of greenhouse gases such as carbon dioxide (CO2) and methane (CH4), depending on their long-term C balance. German forest ecosystems store approximately 2.5 billion tonnes of carbon (=224 Mg C ha–1), more than half of which is assumed to be located in the soil compartment (Wellbrock et al., 2017). In a system in a steady state over the long term, the amount of organic carbon stored in the soil is determined by carbon inputs from aboveground and belowground plant-derived detritus on the one hand, and carbon losses via decomposition processes and heterotrophic respiration (Rh) on the other. In addition, autotrophic (root-derived) soil respiration (Ra) contributes to soil CO2 production, depending on the physiological activity of trees. In turn, these fluxes are determined by the soil’s physicochemical properties and by climate variables, especially temperature and precipitation.
For centuries, Germany’s natural deciduous forests have been converted into fast-growing coniferous forests (Wulf et al., 2017). The country’s forests are currently dominated by coniferous tree species, with Scots pine (Pinus sylvestris L.) predominating in the northeast German lowlands (BMEL, 2015; MLUL, 2015). In recent decades, forest management has undertaken significant efforts to convert the homogeneous, even-aged coniferous forests into more close-to-nature deciduous forests. The reasons for this conversion are to (1) increase the stability of forests against detrimental atmospheric deposition, climate change and other hazards; (2) enhance biodiversity; (3) increase ground water recharge and carbon sequestration; and (4) improve the chemical status of soils (Zerbe, 2002; Spiecker, 2003; Schröder et al., 2007; Müller, 2009; Achilles et al., 2021). The previous conversion of natural deciduous forest into coniferous forest and the reconversion that began a few decades ago, were accompanied by a number of ecological consequences (Leuschner et al., 2022). Coniferous and deciduous forests differ in their SOC stock and their vertical SOC distribution. Germany’s National Forest Soil Inventory (NFSI) has shown that pine forests have significantly higher C stocks in the soil organic layer than beech and other deciduous forests but lower stocks in the mineral soil (Grüneberg et al., 2019). Similar results regarding carbon stocks were found in the Austrian Forest Soil Survey but without differences in their vertical gradients (Jandl et al., 2021). Reasons for this included different lignin contents and C/N ratios; moreover, litter from leaves and needles differ in decomposability, resulting in different SOC stocks in the organic layer and mineral soil, as well as in different humus forms and chemistry (Fischer et al., 2002; Leuschner et al., 2013; Vesterdal et al., 2013; Diers et al., 2021). Moreover, fine root biomass was found to be 6.5 times higher and fine root productivity 1.9 times greater in beech stands than in pine stands (Förster et al., 2021). As a result, forest management that seeks to convert forest may be accompanied by considerable changes in the soil carbon budget, including soil CO2 production.
The increasing frequency, intensity and duration of drought and heat stress across much of the northern Hemisphere are affecting the growth, development and functions of forest ecosystems, from immediate reductions in the metabolic activity of plants and soil organisms to shifts in species distribution during prolonged or recurrent drought (von Rein et al., 2016; Schimel, 2018). Notably, information is lacking concerning mature forest ecosystems, where these processes are particularly difficult to quantify. Monitoring changes in soil carbon stocks and fluxes is a current challenge in the context of soil carbon sequestration and the development of mitigation strategies (Bispo et al., 2017), in part because of reporting requirements on the carbon balance of forests concerning the Kyoto Protocol reporting obligations (Krug, 2018) and the associated methodological uncertainties. To provide a more mechanistic understanding of changes in C fluxes, it is essential to apply long-term continuous measurement approaches with a high temporal resolution to establish a process-related baseline of natural exchange, production and transport of CO2. It is particularly necessary to assess the influence of short-term effects from precipitation events or sudden temperature changes on the soil CO2 budget. For this reason, it is essential to better understand the processes of soil carbon dynamics and, ultimately, the rates of carbon release.
CO2 efflux from soils is, after photosynthesis, the second-largest carbon flux in terrestrial ecosystems and plays a vital role within the global carbon budget (Bond-Lamberty and Thomson, 2010). Since aboveground plant parts are readily accessible, carbon-related processes of those compartments can be measured quite reliably providing data for the calibration of models. In contrast, belowground processes generally remain incompletely understood, and simulations are subject to significant uncertainties; this often leaves open the question of whether the ecosystem can even be considered a carbon store and active carbon sink, or a source of carbon (Peltoniemi et al., 2007; Mol Dijkstra et al., 2009). For this reason, measurements are required to calibrate the belowground processes of terrestrial ecosystem models on regional to global scales in more detail.
Soil CO2 production is strongly influenced by substrate availability; consequently, it is linked to photosynthesis, processes transporting carbohydrates to roots and mycorrhizae, and the production of dead organic matter such as aboveground and belowground litterfall (Ryan and Law, 2005). Due to the temperature-sensitivite nature of soil CO2 production, it has been subject to various studies on the consequences of climate change on the carbon budget of terrestrial ecosystems. Processes involved in biomass production, such as photosynthesis and plant respiration on the one hand and the decomposition of soil organic matter on the other, might react differently to changing climate. This would affect the carbon balance of ecosystems, with consequences for the C sequestration potential (Davidson and Janssens, 2006). To unravel the reactions of soil CO2 production to changing climate, it is necessary to distinguish between these processes. Soil temperature and soil moisture are the main factors that influence soil CO2 efflux, which is usually interpreted as instantaneous soil CO2 production, which is not always true (Maier et al., 2011). Nevertheless, the effect of these factors on the distribution of CO2 production in a soil profile has been little studied since it requires partitioning vertical CO2 production, e.g., by applying the flux-gradient method (FGM) (De Jong and Schappert, 1972; Tang et al., 2003; Maier and Schack-Kirchner, 2014; Osterholt et al., 2022).
Here we present 6-year measurements of soil CO2 efflux and vertical partitioning of CO2 production at two intensive forests monitoring sites located on sandy soils in the northeast German lowlands: a European beech (Fagus sylvatica L.) and a Scots pine (Pinus sylvestris L.) forest stand. The sites are part of the International Co-operative Programme on Assessment and Monitoring of Air Pollution Effects on Forests (ICP Forests), launched in 1985 under the UNECE Convention on Long-Range Transboundary Air Pollution (ICP-Forests, 2021). The intensive monitoring of ICP Forests (Level II) initially aimed to clarify the cause-and-effect relationships of air pollution on the vitality of forests but it was expanded in the 1990s to include issues related to climate change and the importance of forests as carbon sinks (Lorenz and Fischer, 2013). Our study relies on a measurement system that allows continuous recording of CO2 concentrations at different soil depths. CO2 efflux and the vertical distribution of CO2 production in the soil were calculated using the FGM, based on measured CO2 concentrations at the soil surface and different soil depths down to 1 m. An essential aspect of this study was the optimisation of several parameters of the FGM through inverse modelling. In the process, we endeavoured to avoid known problems in applying the FGM, such as inappropriate soil gas diffusion coefficients or negative CO2 production rates when applying the FGM with defined model parameters (Wordell-Dietrich et al., 2020). The idea of including inverse modelling was to obtain more meaningful comparisons of the dynamics and vertical distribution of soil CO2 production at a beech and a pine forest site under various meteorological conditions.
The main objective was to study the short-term effects and long-term trends of soil CO2 production and efflux in a beech forest and a pine forest and to determine how soil moisture and temperature regimes affected that CO2 production. Special emphasis was placed on the comparison of the vertical distribution of soil CO2 production between both sites, which required the application of the FGM.
We hypothesised that (1) temperature and precipitation effects are the most sensitive factors for soil CO2 production and its vertical distribution at the (1a) inter-annual and (1b) seasonal time scale. We also hypothesised, that (2) tree species characteristics and soil properties such as seasonal patterns of leaf area index (LAI), vertical distribution of SOC and fine roots all influence (2a) seasonal variation as also (2b) vertical and (2c) horizontal distribution of soil CO2 production, as well as (2d) the sensitivity of CO2 efflux to drought.
Materials and Methods
Investigated Forest Sites
We investigated two mature forest stands, a European beech (Fagus sylvatica L.) and a Scots pine (Pinus sylvestris L.) forest, both representing intensive monitoring plots in the ICP Forests programme (ICP-Forests, 2021), located in Brandenburg, northeast Germany. The beech stand (Beerenbusch, ICP Forests Level II plot number 04-1207, long. 12.99 dec°, lat. 53.15 dec°) consists of a pure, 126-year-old (in 2014) beech forest, with 216 stems ha–1, a basal area of 29 m2 ha–1, and 95% canopy coverage. The herb layer of ground vegetation covers only 1%, and the moss layer 5% (mainly Hypnum cupressiforme Hedw. S. Str.). The plant community is classified as a Majanthemo-Fagetum. The pine stand (Kienhorst, 04-1203, long. 13.64 dec°, lat. 52.98 dec°) consists of a 110-year-old (in 2014) pine forest (338 stems ha–1, basal area 38 m2 ha–1, 75% canopy coverage) with minor contributions of rowan (Sorbus aucuparia L.), silver birch (Betula pendula ROTH.), and black cherry (Prunus serotina EHRH.) trees, each covering 1%. The herb layer of ground vegetation covers 80%, consisting mainly of blueberry (Vaccinium myrtillus L.) and hairgrass [Deschampsia flexuosa (L.) Trin.]. The moss layer consists mainly of neat feathermoss [Scleropodium purum (HEDW.) LIMPR., 75% coverage] and red-stemmed feathermoss [Pleurozium schreberi (Brid.) Mitt., 20%]. The plant community is classified as Vaccinio-Pinetum.
The humid climate is similar for both sites with mean annual temperatures (MAT) of 8.6°C (beech) and 9.2°C (pine) and mean annual precipitation (MAP) of 594 and 576 mm a–1, respectively, according to nearby German Meteorological Service (DWD) weather stations for 1981–2010.
Both sites are located on sandy soils within a Late Pleistocene landscape. The soil of the beech site is classified as Brunic Arenosol (Dystric) according to WRB (2015) within a Weichselian outwash plain (sandur) in the foreland of Late Pleistocene terminal moraines. The soil of the pine site is classified as Haplic Podzol. The beech forest soil is characterised by higher silt and clay contents compared to the pine forest. The humus form of the beech forest soil is mull-like moder (thickness of Of/Oh = 3.8 cm), while raw humus (thickness of Of/Oh = 6.3 cm) was prevalent at the pine site. Accordingly, the soil of the beech stand is characterised by a lower C/N ratio, a higher pHKCl value, and higher base saturation in the organic layer. The soils are decalcified down to depths of at least 1.7 m. Below this depth, carbonaceous sediments raise the pH to >7.0. A detailed analysis of the soil profiles according to Germany’s second National Forest Soil Inventory (NFSI II) (König et al., 2005; Wellbrock et al., 2016; Höhle et al., 2018) is presented in Table 1. The distribution of fine roots was studied in 1995 at the pine site (Riek and Strohbach, 2001) and in June 2002 at the beech site (Lachmann, 2002). To this end, soil profiles of 4 m width and 2 m depth were established near at least two trees at a distance of 0.5–1 m at a typical site of the stand, and the number of fine and coarse root tips was counted in a grid of 0.1 × 0.1 m.
LAI, measured in August of each year, varied in the beech forest between 5.2 in 2016 and 6.3 in 2018, but fell sharply to 3.1 in 2019 due to intense thinning conducted in February 2019. Nevertheless, this had hardly any effect on soil temperature, as a tree to the south was still shading the experimental zone. LAI of the evergreen pine forest is much lower, ranging between annual maximum values of 2.0–2.3 in August and 1.7–2.0 in February.
Experimental Set-Up
In each investigated forest stand, we excavated three small soil pits at three radial distances (1.0, 2.5, 3.5 m) from a stem. Next, we installed soil gas probes horizontally into the walls of each soil pit at different depths (0, 10, 20, 30 and 100 cm mineral soil depth), and one sensor was placed directly at the surface of the humus layer. Soil water content (SWC) sensors in the form of TDR probes (TRIME-pico64, IMKO GmbH, Ettlingen, Germany) were installed at 10, 20, 30 and 100 cm soil depth. The probes monitored the volumetric soil water content and the soil temperature at 30-min intervals. Once all probes had been installed, the soil pit was carefully refilled, re-establishing the original soil layer sequence. The soil gas probes consisted of a stainless-steel tube with a hydrophobic, gas permeable polypropylene membrane (ACCUREL PP V8/2HF, Membrana GmbH, Wuppertal, Germany). The diffusion-based gas probes allow gas circulation in closed loops to achieve CO2 concentrations in equilibrium with the surrounding soil air. CO2 concentrations were analysed in 30-min intervals with a CO2 monitoring system (CO2MS16, METER Group, Munich, Germany), containing small NDIR sensors (MSH-P-CO2, Dynament Ltd., South Normanton, United Kingdom); measurement range: 0–1,000 ppmv (soil surface), 0–5,000 ppmv (0 cm soil depth), or 0–10,000 ppmv (deeper soil) with an accuracy of ±2% full-scale deflection, circulation pumps, and a data logger (DT85M; dataTaker, Thermo Fisher Scientific, Scoresby, Australia). Meteorological data (air temperature, air humidity, solar radiation, precipitation, air pressure, wind speed) were measured at an open land meteorological station close to and within the forest stands. A more detailed description of the experimental set-up can be found in Jochheim et al. (2018).
The total porosity and soil gas diffusion coefficients were measured on undisturbed soil core samples in the laboratory. At each site, five soil rings per soil depth (volume 200 cm3, height 5 cm) were taken from the humus layer (O horizon), from the A horizon (0–8 cm and 0–10 cm for the beech site and the pine site, respectively), and in two soil layers of the B horizon (8/10–20 cm, and 20–30 cm depth). Vacuum pycnometry (Hartge and Horn, 1999) was employed to measure total pore space [TPS (m3 m–3)]. From the difference between TPS and soil water content [SWC (m3 m–3)], we calculated the air-filled pore space [AFPS (m3 m–3)]:
Soil gas diffusion coefficients were measured at four different levels of SWC using a one-chamber method similar to that described by Jassal et al. (2005), but using neon (Ne) as a tracer gas, as described in detail by Maier et al. (2010). The relative soil gas diffusivity () relates the effective soil gas diffusion coefficient [Ds (m2 s–1)] to the free air CO2 diffusion coefficient under standard conditions [D0 (m2 s–1)]. Thus, DS can be calculated by multiplying Dr by D0 of a given temperature and barometric pressure, while Dr can be calculated by empirical functions from Eq. (2) that are fitted for each horizon, with parameters a and b in Table 2 and Supplementary Figure 1.
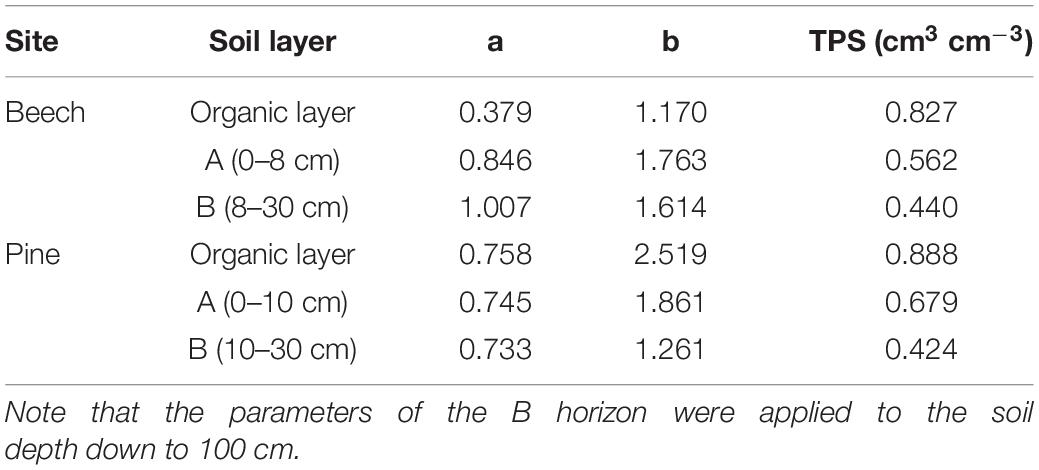
Table 2. Measured values of total pore space (TPS) and fitted parameters (a, b) to calculate the relative diffusion coefficient (Dr) as a function of AFPS for the two sites and the soil horizons according to Eq. (2).
The CO2 efflux from the soil surface was occasionally measured (67 days for the beech site, 64 days for the pine site) from April 2018 to December 2019, applying the closed chamber method. At each location, six collars were inserted 1–3 cm deep into the upper mineral soil, arranged in two gradients at increasing distances of 1.25, 2.50, and 3.75 m from the central tree, directly before the first measurements. We did not remove the ground vegetation, but pruned the newly grown parts of the blueberry shrubs. For the measurements of CO2 efflux, an opaque chamber with a basal area (A) of 0.167 m2 and a volume (V) of 0.0387 m3 was placed on these collars. The effective chamber volume was 0.033–0.037 m3, depending on the part of the collar (1–3 cm) inserted in the upper mineral soil.
The CO2 concentration (c) development in the chamber was measured using a Vaisala CarboCap GMP343 (0–1,000 ppmv), installed in the centre below the chamber lid, over at least 10 min. A fan inside the chamber was not used. The linear line segment with the maximum slope over at least 2 min of increasing CO2 concentration (dC/dt) [μmol mol–1 s–1] was taken to calculate the CO2 efflux Fc [μmol m–2 s–1] from soil using Eq. (3),
where MV is the molar volume of gas under standard conditions (T0 = 273.15 K, p0 = 101,325 Pa) (MV 0.022414 m3 mol–1), p and p0 represent the air pressure (Pa) and Tand T0 represent the temperature (K) within the chamber during measurement and under standard conditions, respectively.
Pre-processing of Data
Gaps in the time series of soil CO2 concentration, soil temperature, and SWC were filled using the machine learning approach known as Nearest Neighbor Analysis (KNN) and by Linear Interpolation (IBM-SPSS version 27) using the sequence to generate substitute values of (1) parallel sensors of the same soil depth, (2) other sensors of the adjacent soil depths, and (3) air temperature values within the forest stand or open field. Most of the data gaps related to CO2 concentrations measured in 2014 and 2015, when only one CO2 monitoring system was available, and we had to switch it between both forest sites every 2–3 weeks.
The soil water content of the organic layers (O) of the two sites, which could not be directly measured, were obtained from results of numerical simulations of the soil water balance using the HYDRUS-1D program (Šimůnek et al., 2009). In the HYDRUS program, the Richards equation for variably saturated soil water movement is numerically solved using a finite element scheme. The model was calibrated and simulations were run based on soil hydraulic parameters, meteorological data, tree canopy interception, LAI and phenology information (see the Supplementary Material for a more detailed description).
To apply the FGM to soil CO2, SWC and the temperature between measured soil depths, we discretised the soil profile into 2–5 cm steps by linear interpolation.
Flux-Gradient Modelling of Soil Gas Fluxes
To calculate the CO2 fluxes and the vertical partitioning of CO2 production in the soil profiles at both forest sites, we employed the FGM (Freijer and Leffelaar, 1996). We assumed that CO2 is produced in soils but never consumed, and that any amount of CO2 produced is removed via a measurable efflux from the soil. Thus, by setting constant production rates for homogeneous layers within the soil profile, it was possible to obtain a 1D vertical concentration profile in the first step. In the second step, we varied the production rates by inverse modelling to match the observed soil gas concentrations in the soil and the efflux from the surface.
The vertical soil profiles were subdivided into four homogenous layers with constant conditions, (1) the organic layer, (2) the A horizon (0–8 cm for beech, 0–10 cm for pine), (3) 8–30 cm for beech or 10–30 cm for pine, and (4) 30–100 cm for both beech and pine. In each layer, the density of the air (ρ), the effective soil gas diffusion coefficient (Ds) and the production rate (P) are assumed to be constant, so the detailed formulae listed in the Supplementary Material apply to the CO2 flux and concentration within each layer.
To account for uncertainties and spatial heterogeneities in transport parameters, we tested several sets of parameter values for the total pore space of the humus layer, the A and B horizons (85–110% of the mean value), and the thickness of the humus layer within the observed range of measurements (4–8 cm). This enabled us to identify a parameterisation that (a) best predicted the efflux at the soil-atmosphere interface, measured independently by chambers, and (b) best fitted the concentration profiles. This was achieved by a parameter sweep, in which all chamber measurements and concurrent soil gas profiles were run in the inverse model according to the parameter set. The results were evaluated according to quality control measures laid down in objectives (a) and (b). Detailed information about this evaluation can be found in the Supplementary Material. The best parameter set of effective total pore space and humus layer thickness was used for further analysis.
Statistical Tests
To analyse the impacts of environmental factors on CO2 efflux, we applied Times Series Analysis using the decompose() function. The decomposed time series were analysed using the Generalised Linear Model (GLM) using the glm() function of the stats package in R (R-Core-Team, 2020). To reduce data complexity, we aggregated all soil depth-related data (CO2 concentration, CO2 production, SWC, soil temperature, and other soil physical parameters) into two distinct soil layers: upper (O + A horizons) and lower (B horizon). Time series of CO2 efflux and the parameters were then decomposed to separate the trend (Tr), seasonal (S), and random (R) component of the time series based on an additive model according to Eq. (4) using a frequency of 365.25 ⋅ 48 data entries per year.
Correlation analysis was applied to identify key parameters to be used in the GLMs. GLMs were applied to analyse the effect of these predictor variables on CO2 efflux for both the trend and seasonal time scales separately.
Differences between the CO2 concentrations, efflux and production rates of the different groups were verified using non-parametric tests (NPTESTS of IBM SPSS Statistics 27). We employed the related-samples Wilcoxon Signed Rank Test to compare the differences between the two forest sites. Differences between years and soil layers were tested by Friedman’s Two-Way Analysis of Variance by Ranks for related samples using mean values of the three replicate profiles at 30-min intervals.
Results
Meteorological Conditions During the Monitoring Period
Soil gas concentrations and relevant environmental parameters were monitored from 2014 to 2019 (Figures 1, 2). The weather conditions during this period were highly variable. 2016 was dry, 2017 was exceptionally wet, 2018 was exceptionally dry and warm, and 2019 was exceptionally warm (Figure 3). Mean annual temperatures were on average 0.3°C higher at the pine site than at the beech site. No differences were found in the average precipitation between both sites. The mean temperatures at the soil surface and at 0 cm mineral soil depth were about 1.6–1.8°C higher at the pine site than at the beech site in spring and summer, but similar in autumn and winter. Temperature extremes were more pronounced at the pine site compared to at the beech site, especially with regard to maximum values. The soil moisture of the organic layer down to the 30 cm depth of the mineral soil was higher at the beech site than at the pine site. At 100 cm soil depth, the opposite was the case. The relative soil gas diffusion coefficients (Dr) of the humus layer and the A horizon were, on average, considerably higher for the pine site compared to the beech site. These relations changed from soil depths of about 10 cm and deeper, meaning that in summer, Dr was higher at the beech site than at the pine site.
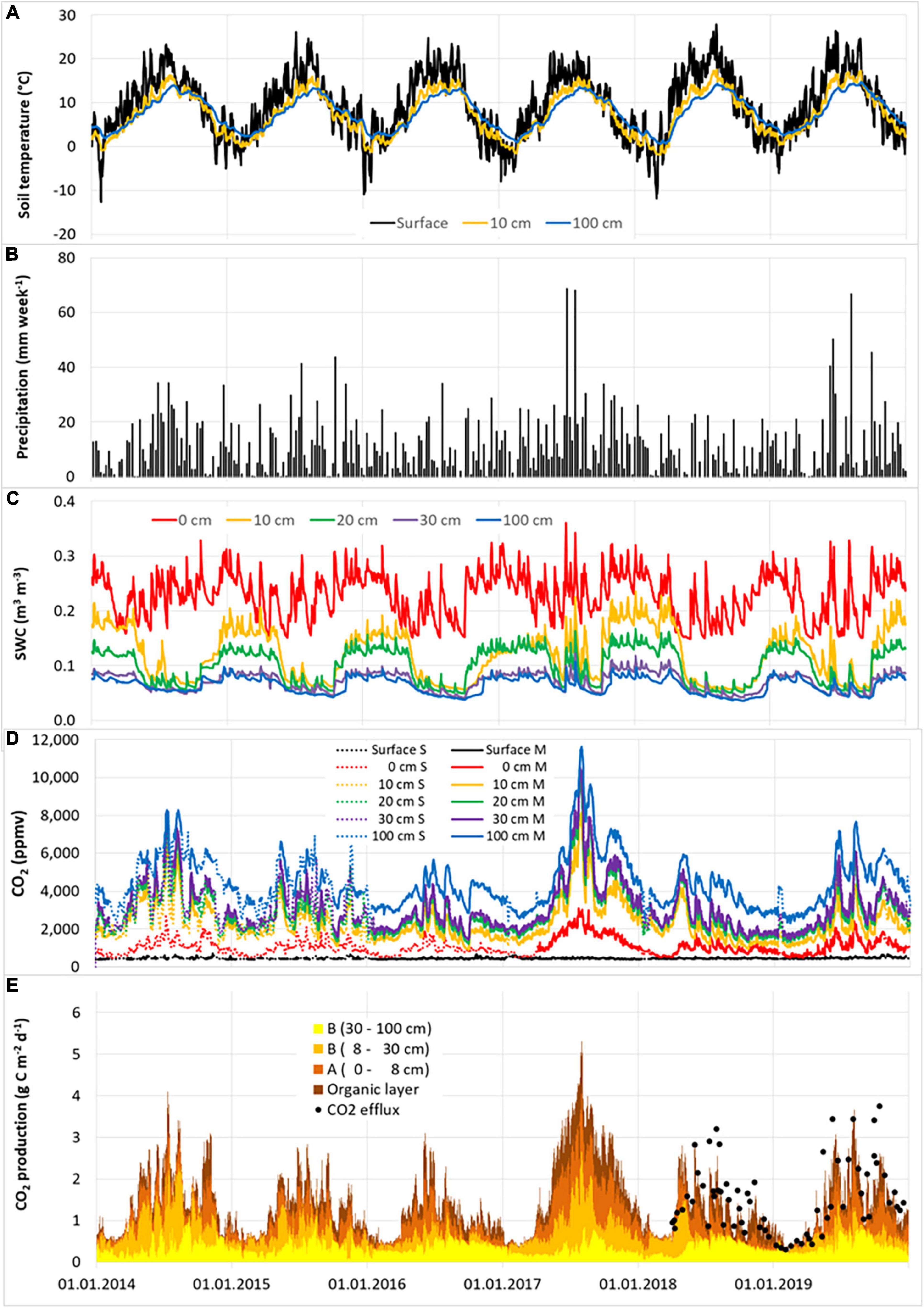
Figure 1. Weekly precipitation total (B) and daily averages of soil temperature (A), soil water content (SWC) (C), measured (solid lines) and gap-filled (dotted lines) soil CO2 concentration (D), and soil CO2 production rate, including CO2 efflux from chamber measurements (E) at different soil depths at the beech site for the years 2014–2019. Total of CO2 production over all the soil layers corresponds to the CO2 efflux from the soil surface. Note that the measurement refers to the respective time of the day, while the calculated values represent daily averages. For this reason, part of the deviations between measured and calculated efflux rates is due to the deviating time scale.
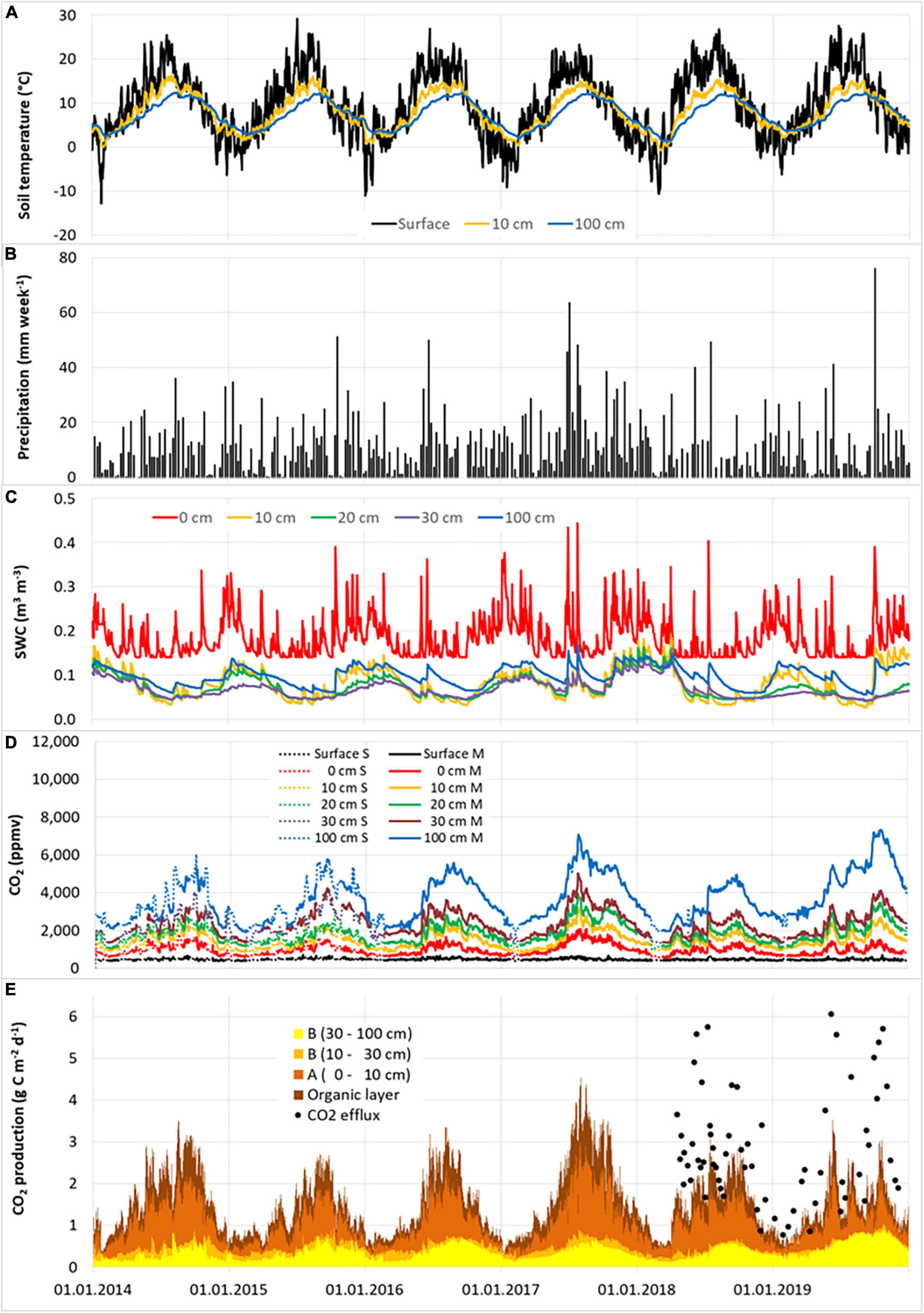
Figure 2. Weekly precipitation total (B) and daily averages of soil temperature (A), soil water content (SWC) (C), measured (solid lines) and gap-filled (dotted lines) soil CO2 concentration (D), and soil CO2 production rate, including CO2 efflux from chamber measurements (E) at different soil depths at the pine site for the years 2014–2019. Total of CO2 production over all the soil layers corresponds to the CO2 efflux from the soil surface. Note that the measurement refers to the respective time of the day, while the calculated values represent daily averages. For this reason, part of the deviations between measured and calculated efflux rates is due to the deviating time scale.
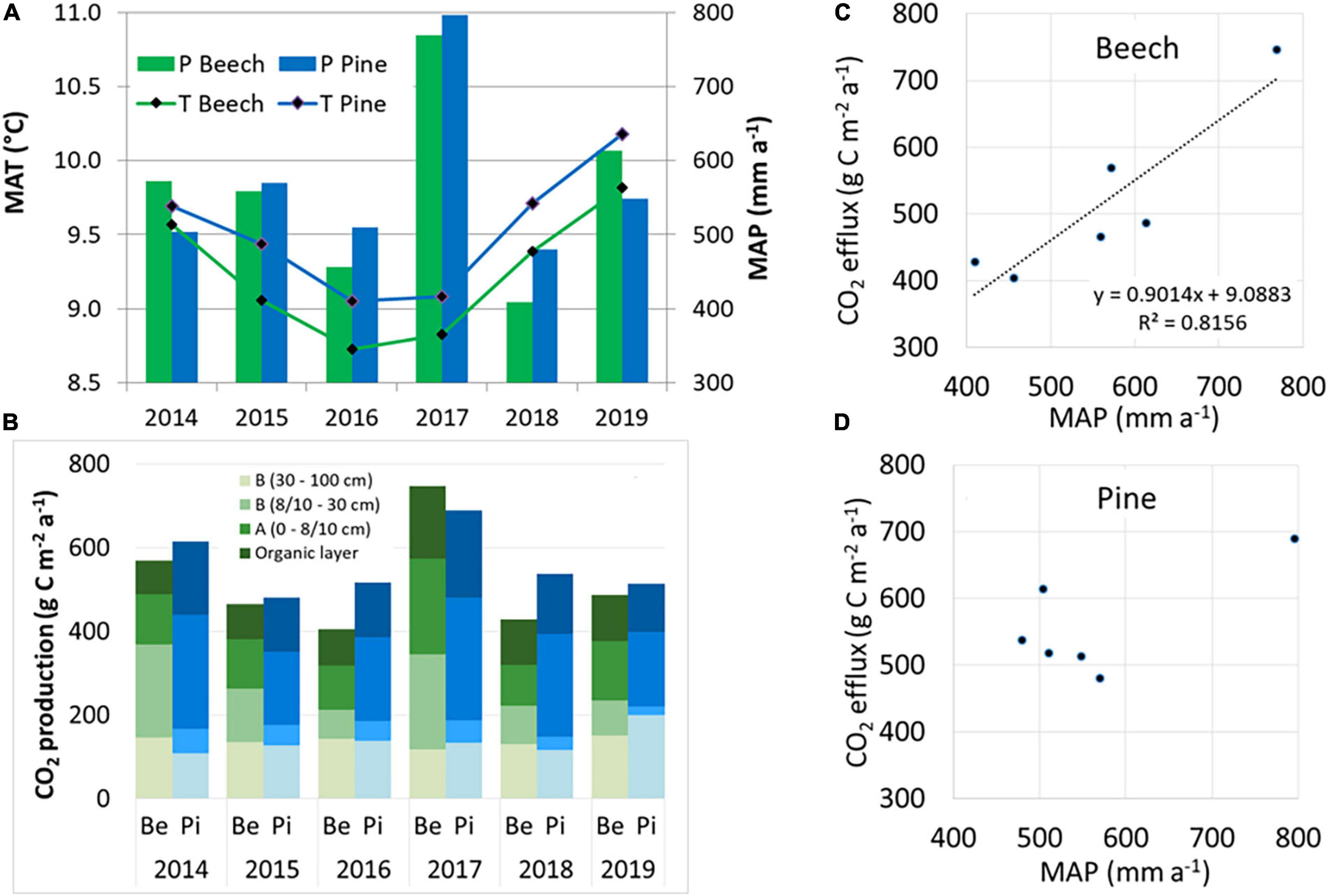
Figure 3. Mean annual air temperature (T) and annual precipitation (P) (A) and mean annual CO2 production in four soil layers with the sum as the CO2 efflux (B) of the beech (Be) site and the pine (Pi) site over the years 2014–2019. The relationship between annual CO2 efflux rates and annual precipitation is indicated for the beech site (C) and pine site (D).
Inter-Annual Effects of Meteorological Conditions on CO2 Production and Efflux
Annual CO2 efflux rates from soils averaged 517 (±126) g C m–2 a–1 and 559 (±78) g C m–2 a–1 for the beech site and pine site, respectively (Figure 3), with a significant difference when comparing daily averages but not for annual averages (Table 3). The CO2 efflux at the pine site exceeded that at the beech site every year, except for the wet year of 2017, when it was the other way round.
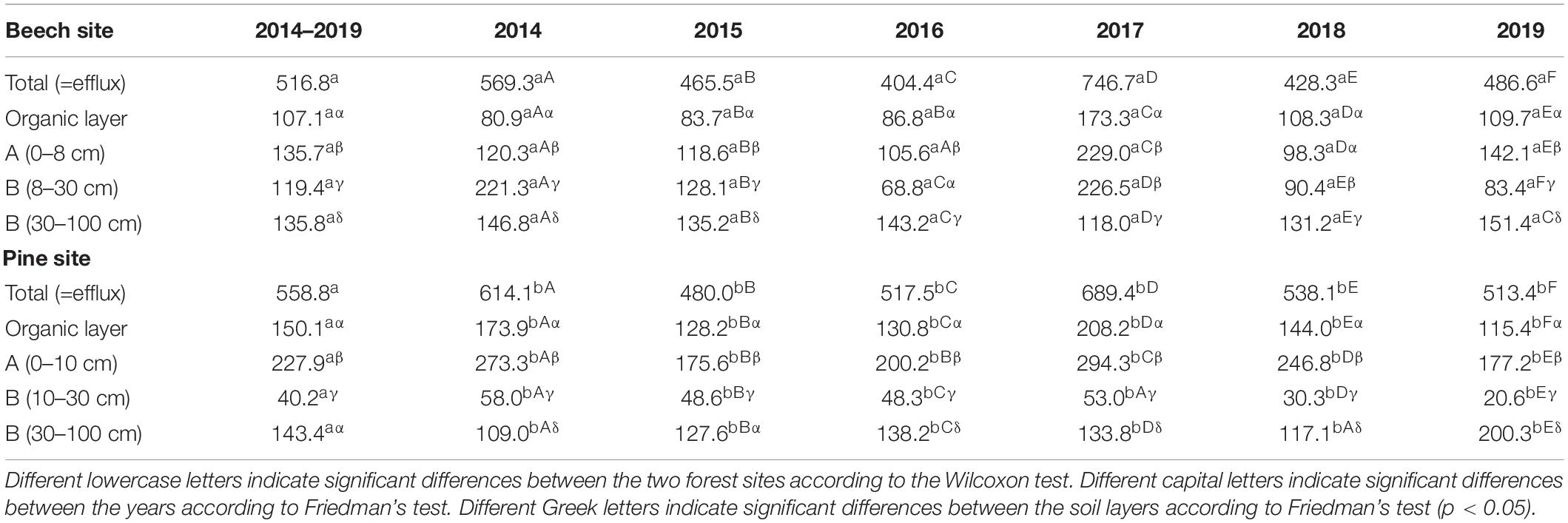
Table 3. Annual layer-related and total CO2 production rates (g C m–2 a–1) at the beech site and the pine site over the entire observation period.
The annual CO2 efflux was lower during dry years, especially at the beech site (Figure 3 and Table 3). Annual CO2 efflux showed a strong positive correlation with MAP at the beech stand, while there was no clear correlation at the pine stand. No clear correlation between the annual CO2 efflux and MAT could be identified for either site. The reduction in annual CO2 efflux due to the warm and dry year 2018 was 43% for the beech forest and 22% for the pine forest compared to the cool and wet year of 2017 (Figure 3 and Table 3). During the summer months (6–8) of 2018, SWC at 0–30 cm soil depth fell by 25–50% at the beech site, and by 17–36% at the pine site compared to during the summer months of 2017. CO2 efflux fell from 3.57 to 1.56 g C m–2 d–1 in the summer months of 2018 compared to the summer months of 2017 for beech (-56%) and from 3.02 to 1.94 g C m–2 d–1 for pine (-36%), respectively. The reduction of CO2 efflux was caused mainly by reduced CO2 production in the organic layer and the A horizon at both sites. At the beech site, moreover, there was considerably less CO2 production at 8–30 cm (-92%). Only minor changes could be observed in the deepest soil layer (30–100 cm).
Quantifying the impact of environmental conditions on the CO2 efflux using GLM based on the trend and seasonal components from a time series decomposition (Supplementary Figure 4) showed that precipitation alone explained 75% of the variation for the beech site and 59% for the pine site on the annual time scale (trend component). Adding more parameters was either not significant or produced only marginally increased R2. Models with the strongest predictive power (highest R2) are shown in Table 4. For the beech, the trend component of the CO2 production rates in the upper and lower soil layers explained the trend of the CO2 efflux. In contrast, for the pine stand, only the trend of the upper soil production (O + A horizon) was a significant predictor of the trend of CO2 efflux.
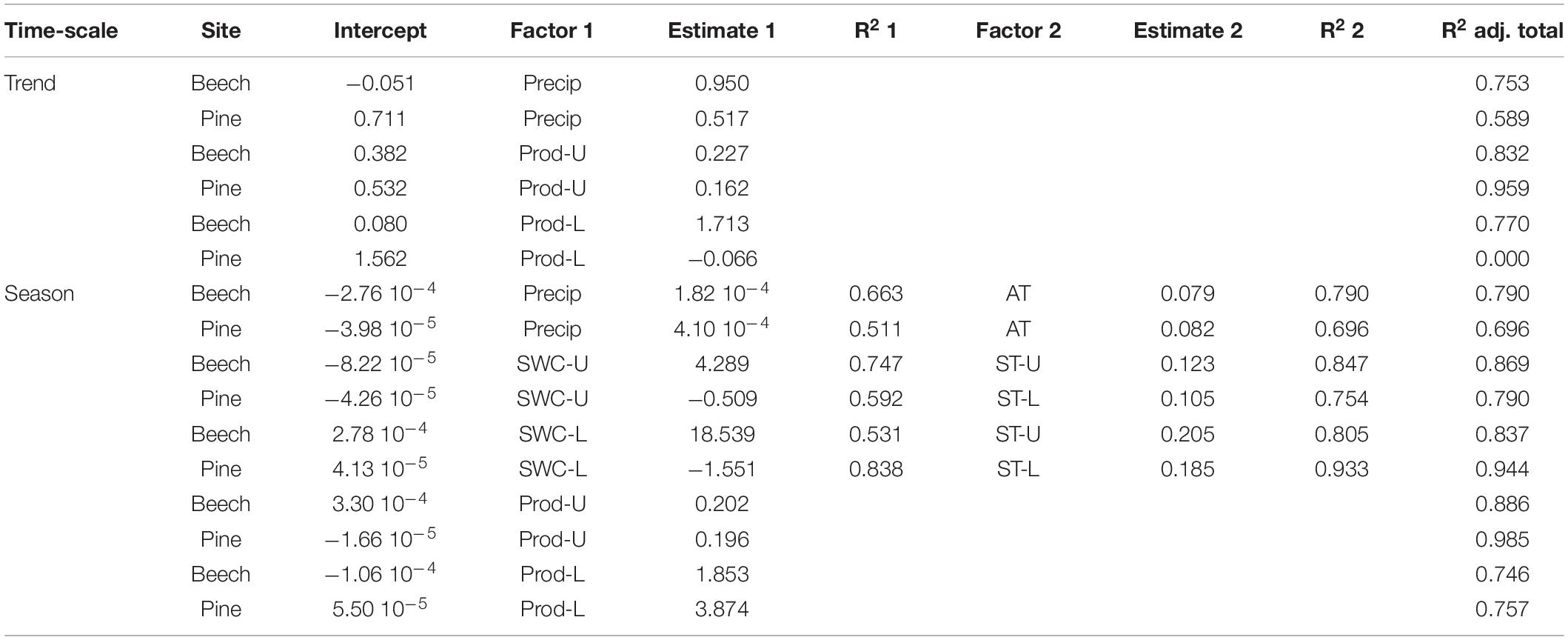
Table 4. Generalised linear model (GLM) output for the trend and the seasonal component from a time series decomposition of CO2 efflux (g C m–2 d–1) using precipitation [Precip (mm d–1)], SWC (m3 m–3), air temperature (AT) (°C), soil temperature (ST) (°C), and CO2 production (Prod) (g C m–3 d–1) in the upper (–U) or lower (–L) soil layers as predictors.
Seasonal Dynamics of CO2 Concentration, Efflux, and Production Rates
Temperature at the soil surface followed the expected seasonality with maxima in summer and minima in winter and increasingly smoothed and shifted in time as soil depth increased (Figures 1, 2). The dynamics of SWC followed precipitation events (at least for the upper soil layers) as well as the pattern of transpiration (Figures 1, 2). Heavy rain events led to an immediate and sharp increase in SWC, followed by gradual decreases during dry periods in summer. In 2018, several unusual periods of more than 14 consecutive virtually rainless days were observed. The temporal dynamics of the relative soil gas diffusion coefficient (Dr) were a nearly reciprocal reflection of SWC, with high values during drought periods in summer and decreasing values after rain events (data not shown).
Soil CO2 concentrations exhibited distinct seasonal dynamics at all soil depths for both forest sites with minimum values in winter and peaks in summer (Figures 1, 2). At both sites, soil CO2 concentrations showed significant vertical gradients (Supplementary Figure 2). CO2 concentrations were higher at the beech site than at the pine site at all soil depths except for the soil surface. The vertical gradient of soil CO2 concentration at a depth between 0 and 10 cm was more pronounced at the beech site than at the pine site (Supplementary Figure 2). The seasonal dynamics roughly follow the soil temperature at shallow depths, except for in the years 2014–2016 and 2018, where a shift in the CO2 peak concentration from summer to autumn was observed at the pine site. Soil CO2 concentrations correlated more closely with soil temperature during periods of high SWC and with SWC during periods of high soil temperature. Heavy precipitation events were followed by increases in CO2 concentration, which decreased again as the soil dried out (Supplementary Figures 1,2).
Daily CO2 efflux rates from soil averaged 1.41 (±0.90) and 1.53 (±0.85) g C m–2 d–1 for the beech site and pine site, respectively. These rates exhibited high temporal variation with maxima during summer and minima in winter (Figures 1, 2). As was the case with the soil CO2 concentration, the annual maximum values of soil CO2 efflux at the pine site had shifted from summer to autumn in most years. Daily averages ranged from 0.26 g C m–2 d–1 for both sites to 5.36 and 4.55 g C m–2 d–1 for the beech site and the pine site, respectively. For both sites, the maximum efflux was detected in summer 2017, during the highest precipitation and SWC. The maximum efflux in other years ranged from 2.8 to 4.1 g C m–2 d–1 for the beech site, and from 2.7 to 3.5 g C m–2 d–1 for the pine site.
Daily mean CO2 production per soil layer ranged from nearly zero during winter to 2.3 g C m–2 d–1 in O and A in summer. The overall mean values ranged between 0.12 g C m–2 d–1 at a depth of 10–30 cm to 0.62 g C m–2 d–1 in the A horizon of the pine site (Figures 1, 2). Peak values in the O and A horizons reached 1–2 g C m–2 d–1 in summer after rain events. The greatest temporal variation of CO2 production appeared in the upper soil layers; this variation roughly followed the seasonal trend of soil temperature throughout the year, and was limited by low soil moisture during summer. The only exception was the soil layer at a depth of 10–30 cm at the pine site, where no clear seasonal pattern was observed. The most distinct temporal changes in the CO2 production within the soil profiles were detected in the upper three layers down to a depth of 30 cm for the beech stand and the upper two layers down to a depth of 10 cm in the pine stand (Figures 1–3). CO2 production rates in the subsoil (below 30 cm) were relatively constant at both sites. The GLM results of the seasonal component of the time series decomposition showed that the seasonal CO2 efflux was explained by the soil CO2 production of both the upper and lower soil layers (Table 4 and Supplementary Figure 4). Seasonal CO2 efflux dynamics were mainly driven by soil temperature changes in the upper and lower soil layers for both sites. Soil water content had a positive effect at the beech site but a slightly negative impact at the pine site.
Differences in CO2 Production and Efflux Between Beech and Pine
Vertical Distribution of CO2 Production
At the pine stand, CO2 production was concentrated in the O and A horizons and decreased in deeper soil depths (Figures 3, 4). In contrast, CO2 production was considerably lower in the O and A horizons at the beech site, but this was counterbalanced by the layer at 8–30 cm soil depth. Below 30 cm, the mean CO2 production rates were very similar at both sites.
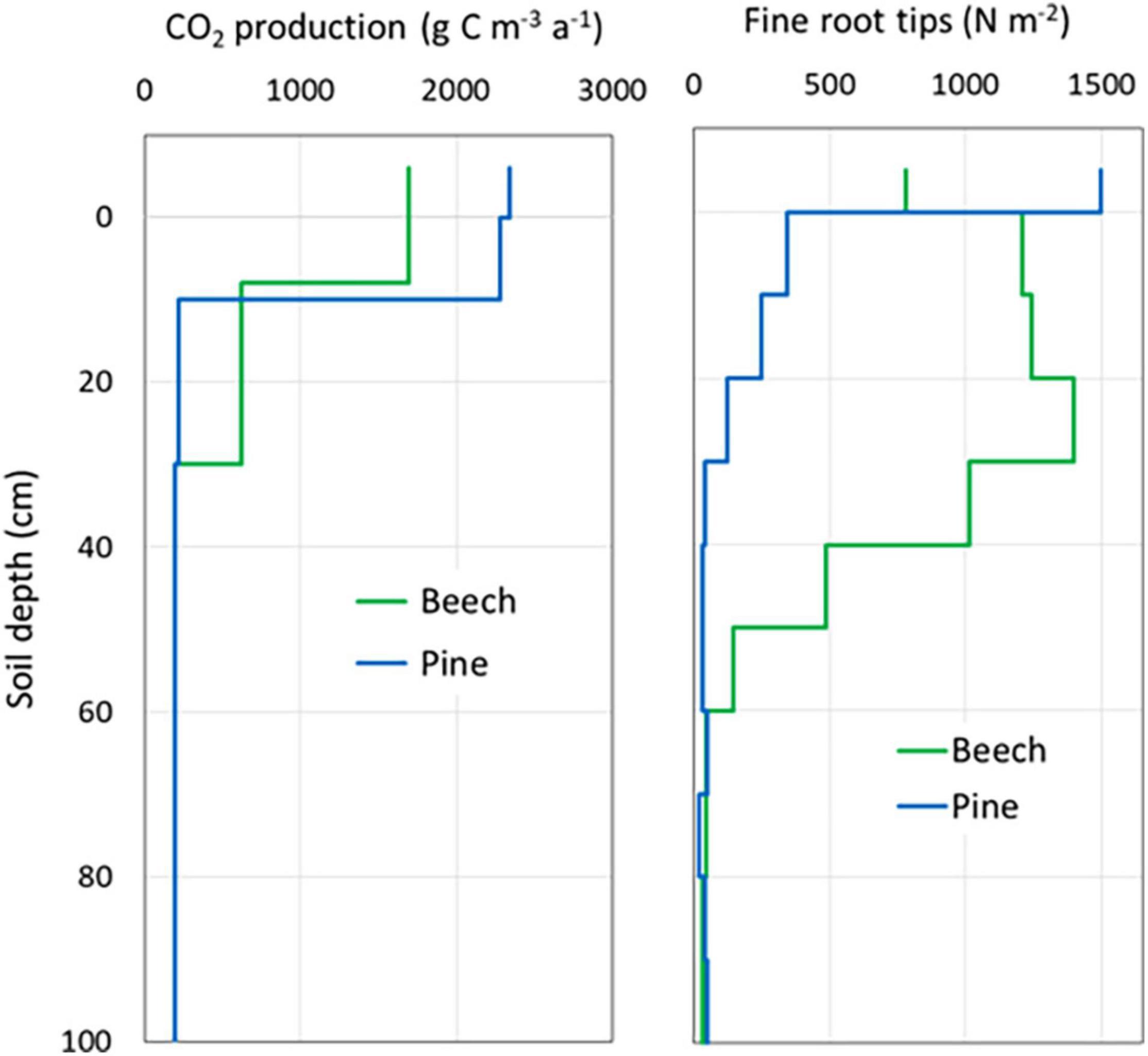
Figure 4. Vertical distribution of volume-related CO2 production rates as mean values over the entire study period (left) and the number of fine root tips (right) in soils of the beech stand and the pine stand.
The organic layer contributed an average of 21 and 27% to total CO2 production at the beech site and the pine site, respectively (Table 3). In the pine forest, two-thirds of the soil CO2 was produced in the O and A horizons. At the beech site, the same amount of CO2 production was distributed over a greater soil depth up to 25 cm (Figure 4). About three-quarters of the CO2 was produced in the upper three soil layers down to 30 cm soil depth at both forest sites.
The vertical distribution of fine roots was also different at the two sites (Figure 4). The highest root density in the pine forest was found in the organic layer and the A horizon, and then decreased significantly in mineral soil below 10 cm. In contrast, the beech stand had lower root density in the organic layer, becoming denser in the mineral soil with a maximum at 20–30 cm. In contrast to the pine stand, the main root zone of the beech stand reached a depth of 60 cm. In deeper soil layers between 1.1 and 1.6 m, fine root density increased slightly at both sites.
CO2 Efflux and Production During Physiological Phases
Our analysis of averaged annual cycles showed that during the late winter and early spring months of February and March (weeks 5–13), CO2 efflux from the soil of the beech site was significantly lower than from the pine site on average (0.57 ± 0.16 vs. 0.75 ± 0.20 g C m–2 d–1) (Figure 5). From the end of April to May (weeks 17–21), the beech soil emitted on average more CO2 than the pine soil (1.70 ± 0.57 vs. 1.34 ± 0.39 g C m–2 d–1). Between mid-August and October (weeks 33–43), CO2 efflux at the beech site fell temporarily from a similar level to that at the pine site to values of up to 30% below it (1.69 ± 0.83 vs. 2.31 ± 0.68 g C m–2 d–1). However, reduced efflux from the beech site during summer compared to the pine site was only observed in 2014, 2016, and 2018. No significant differences between both sites were observed at any other time periods.
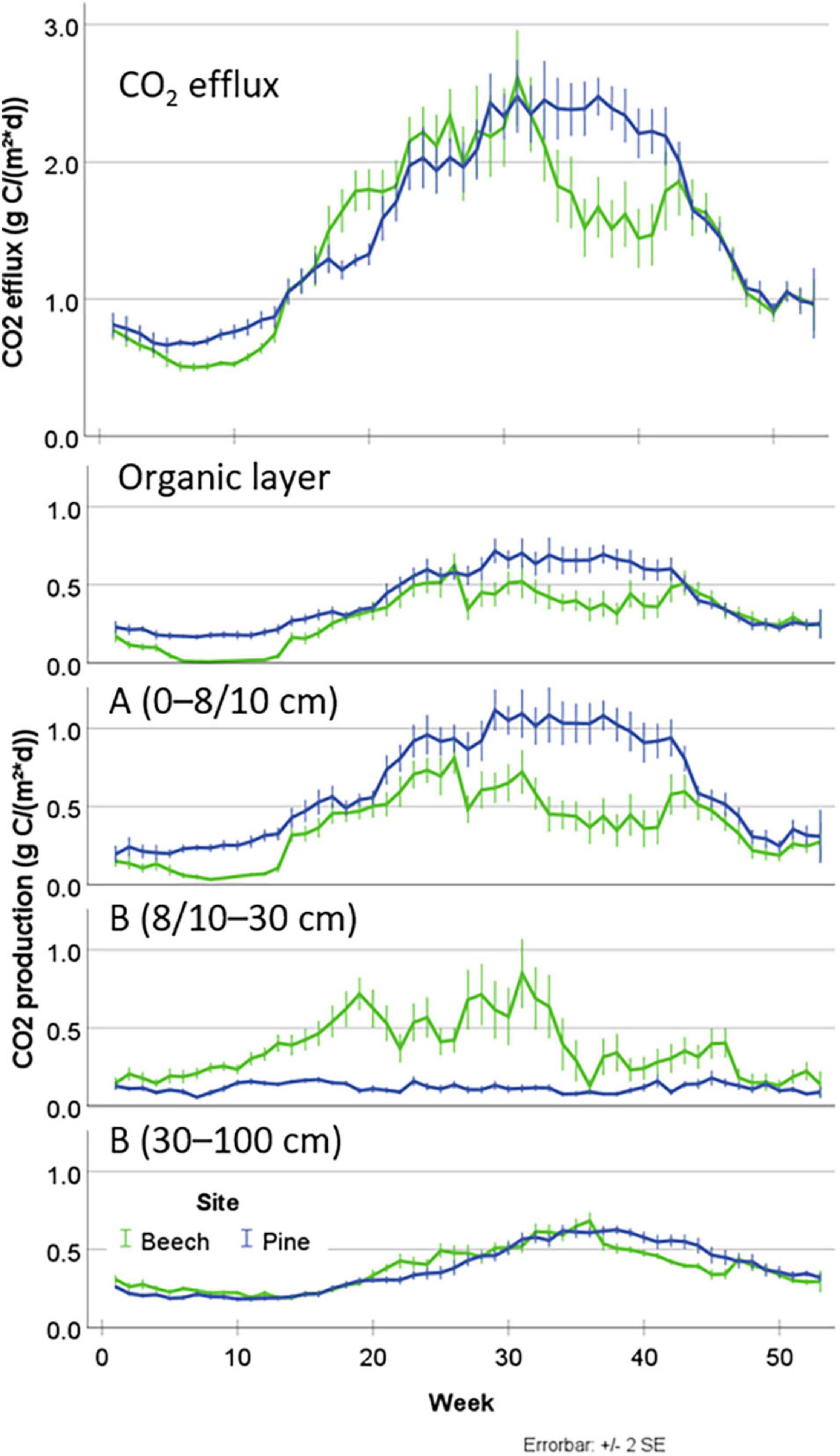
Figure 5. Seasonal dynamics of CO2 efflux (upper graph) and layer-related CO2 production (lower graphs) with mean values and error bars ±2 standard error units (SE) from the beech site and the pine site as weekly means over the years 2014–2019.
The reduced CO2 efflux at the beech sites during weeks 5–13 and 33–43 was primarily caused by reduced CO2 production in the O and A horizons. The lower CO2 production of the beech site was counterbalanced by higher CO2 production in the upper part of the B horizon (8–30 cm soil depth) during weeks 12–34. In the lowest soil layer (30–100 cm), only weak differences regarding CO2 production between both sites were detected.
Stem Distance Effect on CO2 Efflux and Production
We observed the highest CO2 efflux rates close to the stem for both forest sites by chamber measurements within a stem distance gradient across nearly 4 m. These data were used to calibrate the inverse model for estimating the soil CO2 production and efflux. Consequently, we found significant differences regarding the effect of stem distance on the CO2 efflux calculated by the inverse model (Figure 6). The CO2 efflux was on average 32% higher close to the stem at the beech site and 35% higher at the pine site (Table 5). The difference increased to 45% in summer and autumn at the beech site and decreased to zero in winter. In contrast, the CO2 efflux across the stem distance gradient at the pine site remained relatively constant throughout the year.
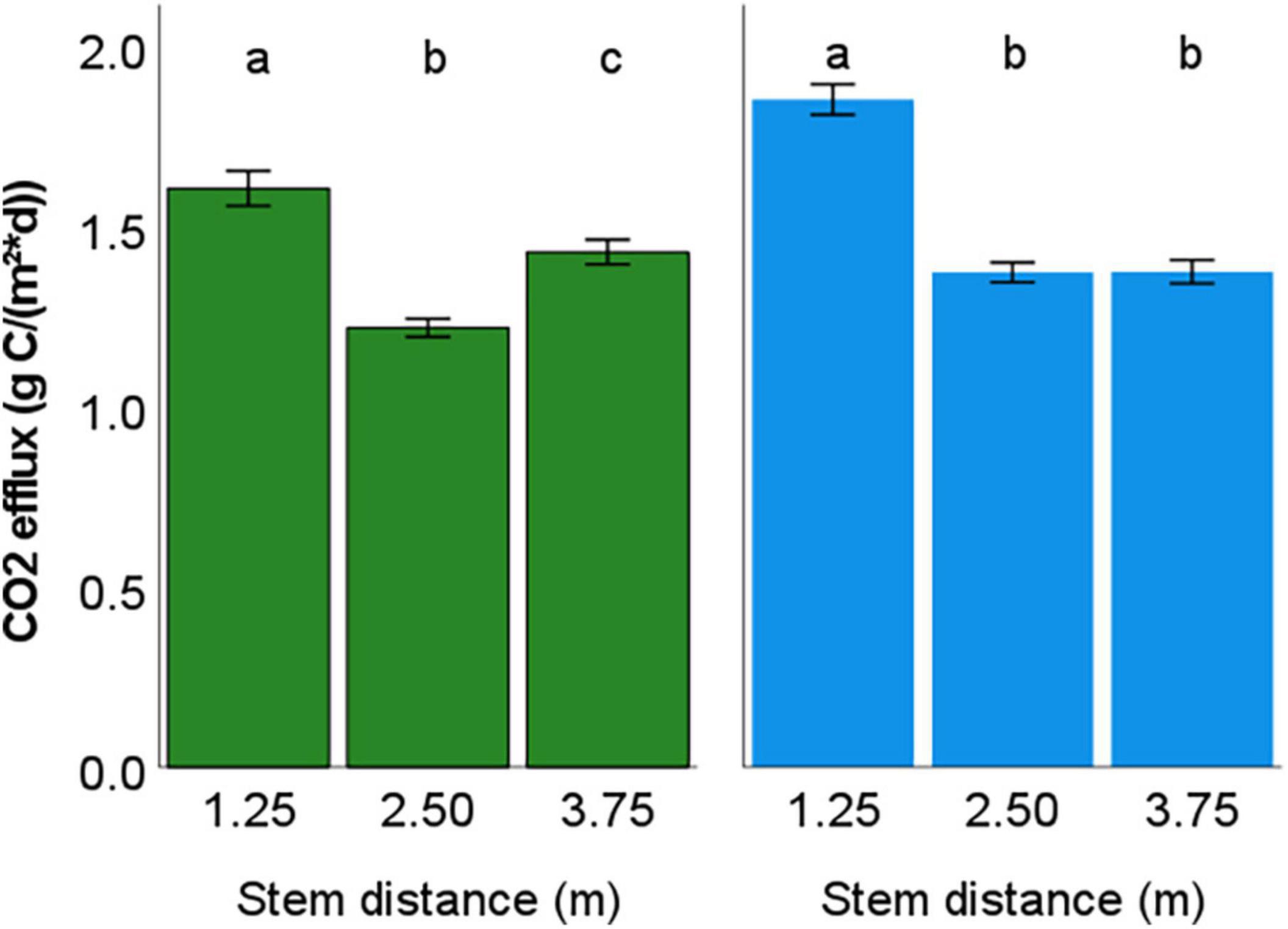
Figure 6. Mean annual CO2 efflux of the beech site (left) and the pine site (right) at different stem distances including error bars ±2 standard error units (SE). Different letters indicate significant differences in CO2 efflux between the stem distances according to Friedman’s test (p < 0.05).
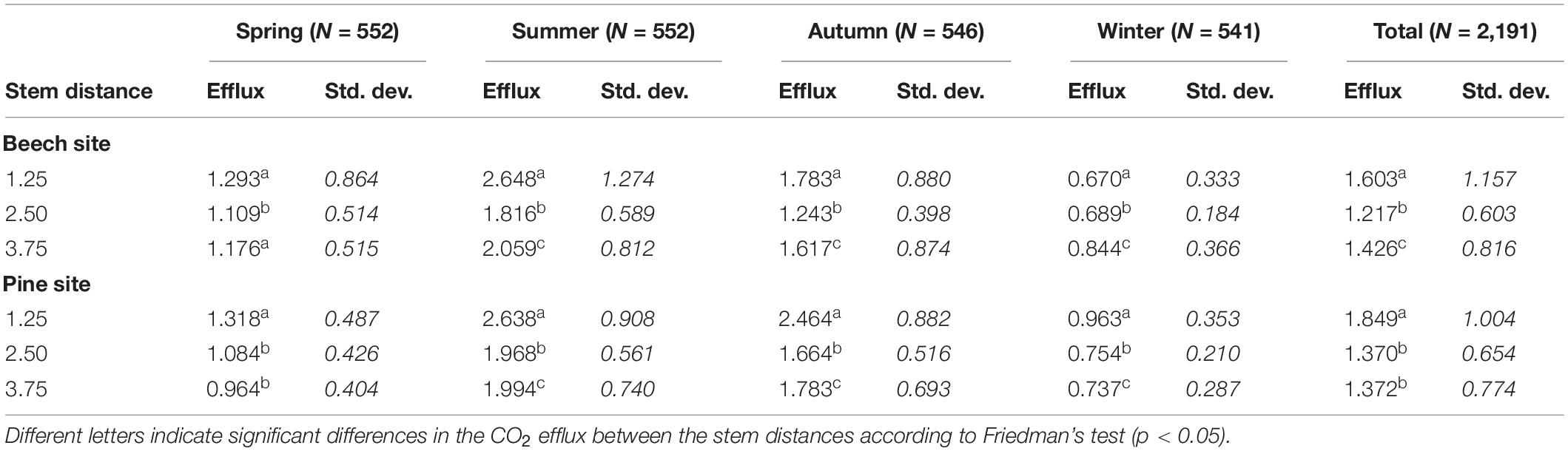
Table 5. Mean CO2 efflux (g C m–2 d–1) and standard deviation (in italics) during four seasons (spring = months 3–5; summer = 6–8; autumn = 9–11; winter = 12–2).
Discussion
We studied the CO2 efflux and the vertical distribution of CO2 production of a European beech forest and a Scots pine forest ecosystem over 6 years. Different meteorological conditions over the investigation period allowed us to quantify the effects of meteorological impacts on soil CO2 production. We found water supply, as indicated by precipitation, to be the primary long-term determinant of CO2 efflux. Seasonal variation of CO2 efflux was mainly attributed to changes in the soil temperature and—in the case of beech—also SWC. On average, we detected similar CO2 efflux rates for both forest sites. During the year with high precipitation, the CO2 production of the beech site exceeded that of the pine site, whereas in dry years, the opposite was the case. We found that soil CO2 production was spread through a greater soil depth at the beech site than at the pine site. We were also able to identify different phases with different patterns of soil CO2 production between the two tree species. At both sites, CO2 efflux rates were higher closer to the stem than more distant from it.
Environmental Impacts on the Temporal Dynamics of Soil CO2 Production and Efflux
Soil temperature and moisture have been known to be the main impact factors of soil CO2 production for a long time and have successfully been described by different statistical models with an exponential reliance on soil temperature and a limitation by SWC (Borken et al., 1999, 2006; Savage and Davidson, 2001; Reichstein et al., 2003; Webster et al., 2009; Luo et al., 2012). A higher coefficient of determination of our results between precipitation and CO2 efflux on the long-term scale indicates a stronger influence of water availability on CO2 efflux for the beech stand compared to the pine stand (Figure 3 and Table 4). At the seasonal scale, soil temperature was the main predictor for both sites (Figures 1, 2, and Table 4). Soil moisture at the seasonal scale, however, was only a predictor for CO2 efflux at the beech site.
While soil CO2 production under drought stress fell mainly in the O and A horizons at the pine site, decline of soil CO2 production at the beech stand was most pronounced at 8–30 cm soil depth—in spite of the reductions in the O and A horizons; this is also the range with the highest fine root density. This could be interpreted as a strong influence of drought on Ra for beech. Primary production and subsequently Ra may have fallen due to beech reaching its climatic distribution limit at the study site (Kölling, 2007; Kasper et al., 2022). The higher LAI of the beech canopy in summer leads to increased evapotranspiration under comparable weather conditions and causes more drought, as indicated by the greater decrease in soil moisture during summer drought observed at the beech site compared to the pine site. We conclude that drought reduces Rh at both sites, but additionally decreases Ra in the beech stand. These results contradict the results of an experimentally induced drought in a mixed deciduous forest, which reduced CO2 efflux by 10–30%, probably mainly affecting Rh in the O horizon (Borken et al., 2006). Similarly, a literature review concluded that in mesic and xeric ecosystems, drought reduces Rh more significantly than it does Ra (Wang et al., 2014). To analyse whether Rh or Ra would be more affected by drought, it is necessary to apply methods to distinguish both processes, such as isotope techniques or other approaches (Hanson et al., 2000; Comeau et al., 2018).
The climate change scenarios projected for the investigated region include increasing temperatures, but only low changes regarding MAP (Hübener et al., 2017). Instead, they envision a shift in precipitation distribution over the years with more dramatic extremes such as the frequency and intensity of dry periods. This means we can expect increasing CO2 efflux due to rising temperatures, but also reduced soil CO2 production due to increasing drought. However, since drought and heat waves may additionally decrease primary production (Davidson and Janssens, 2006; Wang et al., 2014; von Buttlar et al., 2018), the impact on the carbon sequestration of soils remains unclear. Increased SOC stocks as a result of decreased decomposition processes could only be expected if the input of aboveground and belowground litter into the SOC pool is not decreased to the same extent. Such a relationship could be one reason for the increase in the SOC pool observed in northern German dystrophic forest soils between 2002 and 2012 (Grüneberg et al., 2014). Assessments on the carbon balance of both forest sites would also require considering data on primary production, which was beyond the aim of this study.
Tree Species Effects
Tree Species Effect on Soil CO2 Production
The range of annual CO2 efflux rates of 517 (±126) g C m–2 a–1 for the beech site and 559 (±78) g C m–2 a–1 for the pine site (Figure 3 and Table 3) is consistent with other studies from forests under comparable site conditions (Borken et al., 2002; Elberling and Ladegaard-Pedersen, 2005; Subke et al., 2006; Brumme et al., 2009; Phillips et al., 2010; Jurasinski et al., 2012; Goffin et al., 2014; Oertel et al., 2015; Wordell-Dietrich et al., 2020). Although the CO2 efflux over the entire period does not differ between the two forest sites, significant differences can be observed for each year—with higher CO2 efflux at the beech site only in the wet year of 2017 and lower CO2 efflux in all other years. This finding can be attributed to the beech site having higher sensitivity to drought stress with respect to soil CO2 production compared to the pine site.
The CO2 efflux rate of the beech stand, which is on average (but not significantly) lower compared to that of the pine stand, was somewhat surprising to us. We had expected the better soil conditions to lead to higher productivity and soil CO2 production. Simulations on the carbon budget of these two forest ecosystems using the physiologically based Biome-BGC model, calibrated with measured data, showed slightly higher net primary productivity (NPP) for the beech site (692 g C m–2 a–1) than for the pine site (630 g C m–2 a–1) (Jochheim, unpublished). Similarly, aboveground net primary productivity (ANPP) of beech forests in the northern German lowlands was found to exceed that of pine stands by 36.4% (Leuschner et al., 2022), while fine root productivity of beech stands was 1.9 times higher than in pine stands (Förster et al., 2021). Another estimate based on litterfall and root turnover showed that annual total litterfall from the investigated beech stand averaged to 327 g C m–2 a–1 from 2001 to 2019. Adding the fine root biomass down to 50 cm soil depth of 168 g C m–2 (Jochheim, unpublished) and assuming a turnover rate of 1 a–1 (Brunner et al., 2013; Hertel et al., 2013) results in an annual carbon input of 495 g C–2 a–1 into the SOC pool of the beech forest. This SOC fuels heterotrophic respiration and corresponds approximately to the amount of the CO2 efflux (517 g C m–2 a–1) calculated in our study, which, however, includes both Ra and Rh. The difference between both fluxes would allow a contribution of only 22 g C m–2 a–1—or 4%—for root respiration, which seems too low. A total litterfall rate of 231 g C m–2 a–1 was determined for the pine stand for 2002–2018. Combined with a fine root biomass of 166 g C m–2 a–1 over 0–70 cm soil depth (Hornschuch et al., 2007) and assuming a turnover rate of 1 a–1 (Janssens et al., 2002), this amount added up to an annual litter production of 397 g C m–2 a–1. The difference to the simulated CO2 efflux of 559 g C m–2 a–1 would thus result in a contribution of 162 g C m–2 a–1—or 29%—for root respiration, which is more realistic than the results for the beech forest. In relation to these estimates, the CO2 efflux calculated by the FGM in this study appeared to be too low for the beech stand. However, the use of radiocarbon (14C) methods suggests lower turnover rates of fine roots, which would considerably reduce the estimated annual input by roots to the SOC pool (Gaudinski et al., 2001, 2010; Ahrens et al., 2014), although a recent study based on annual growth rings of roots concluded that the radiocarbon method without considering a carbon storage pool underestimates the turnover rates of fine roots (Solly et al., 2018). Additionally, a precondition for all these considerations is that SOC stocks are in a steady state, which is probably not the case.
Tree Species Effect on the Seasonal Dynamics of Soil CO2 Production and Efflux
The higher temporal variation of CO2 efflux at the seasonal scale in the beech forest compared to the pine forest (Figure 5) seems plausible, since the beech as a deciduous tree species varies its physiological activity more than the evergreen pine trees, with root respiration evident throughout the year. The higher CO2 efflux in the pine stand from February to March indicates the earlier physiological activity of the evergreen species during spring compared to the beech stand. In contrast, the beech trees catch up later with their physiological activity during leaf unfolding, which could be associated with rising root growth and accompanying growth in root-derived soil CO2 production (Pregitzer et al., 2000; Montagnoli et al., 2012; Wang et al., 2019). The more substantial decline in soil CO2 production from mid-August to October at the beech site compared to the pine site was probably caused by drought. Conversely, in the wet year of 2017, the beech site experienced higher CO2 efflux than the pine site. Thus, the beech site’s decomposing soil microbiota and/or root respiration were probably more inhibited by drought than those at the pine site. Granier et al. (2007) reported corroborating findings that under drought conditions, total ecosystem respiration decreased more dramatically in deciduous forests than in coniferous forests.
Tree Species Effect on Vertical Distribution of Soil CO2 Production
Differences in the vertical gradients of CO2 production between the investigated forest sites were primarily caused by the quality and quantity of decomposable organic substrates (Conant et al., 2011), rooting intensity and microbial activity. The vertical distribution of fine roots differs considerably between the two sites. The number of fine root tips in the organic layer is almost twice as high at the pine site as it is at the beech site. In contrast, the fine root density in the mineral soil down to a depth of 60 cm is significantly higher at the beech site than at the pine site. These findings correspond to the results of Förster et al. (2021), who found 6.5 times higher fine root biomass in beech stands than in pine stands of Pleistocene lowlands of northern Germany. Consequently, the different vertical distributions of CO2 production between the two sites corresponded to the vertical distribution of fine roots (Figure 4). In contrast, a higher root biomass would be expected due to the higher basal area of the pine site compared to the beech site.
Furthermore, the beech forest soil, with its higher pH and base saturation, lower C/N ratio, and better humus quality (mull-like moder vs. raw humus), might contribute to the differences in the vertical distributions of soil CO2 production vis-à-vis the pine site. The limited conditions for SOC decomposition at the pine site leads to higher carbon stocks in the organic layer and the upper mineral soil compared to at the beech site (Table 1), whereas the beech forest soil has a greater potential to promote soil microbiological activity.
Thus, the differences in the vertical distribution of soil CO2 production, 68% of which was concentrated in the O and A horizon at the pine site, in contrast to only 47% at the beech site, could be explained by the differences in root distribution as well as by the different chemical conditions of the soil. Several litter manipulation experiments have reported contributions of 9–37% by the organic layer to total CO2 efflux (Bowden et al., 1993; Nadelhoffer et al., 2004; Borken and Beese, 2005; Kim et al., 2005; Sulzman et al., 2005). In that sense, our results on the vertical distribution of CO2 production fit well into the range of other investigations.
Horizontal Variability of Soil CO2 Production and Efflux
Stand structure parameters such as stem density, LAI, and fine root biomass influence CO2 efflux at the spatial scale. These are parameters that might, in turn, affect SOC content and soil chemical properties such as the C/N ratio of the organic layer, as well as soil temperature and SWC and thus the soil CO2 production (Søe and Buchmann, 2005; Conant et al., 2011; Jurasinski et al., 2012; Ngao et al., 2012; D’Andrea et al., 2020).
In our study, the highest CO2 efflux rates were observed near the trunks of trees, rather than locations more distant from the trunk; this was true for both sites (Figure 6). Regarding CO2 efflux, the stem distance gradient at the beech site was more pronounced in summer and autumn than during the rest of the year. In contrast, the gradient was similar in the pine stand all year round (Table 5). We suspect that fine root distribution was the most important influencing factor, and therefore the autotrophic fraction of soil CO2 production would increase nearer to the trunk. Obviously, root respiration under the beech as a deciduous tree species showed higher seasonal dynamics, while root respiration of the evergreen pine was reduced in winter to a similar extent as Rh. However, Ra and Rh could not be separated in our approach. A possible approach to partitioning Rh and Ra in forests would be to apply a model based on root allometry as a function of stem distance and tree diameter at breast height (Zhao et al., 2021). Fine roots in the investigated beech stand exhibited a maximum density at a 2.5 m stem distance at 0–30 cm mineral soil depth, but also close to the stem at a 30–60 cm soil depth (Jochheim et al., 2022). Previous researchers have found similar horizontal distribution patterns of fine roots for beech forests and pine forests (Friedrich, 1992; Hölscher et al., 2002; Hornschuch et al., 2007; Hornschuch, 2009; Jurasinski et al., 2012). We therefore assume that the steeper stem distance gradient of CO2 efflux in the beech stand in summer compared to winter indicates more intense seasonal variation of root respiration than in the pine stand.
Methodological Uncertainties
For the discussion of the advantages and disadvantages of the CO2 monitoring system we applied, we refer to our previous methodological paper (Jochheim et al., 2018).
Compared to the chamber measurements conducted between April 2018 and December 2019, the estimated CO2 efflux rates from the FGM resulted in a slight underestimation for the beech site but a significant underestimate for the pine site (Figures 1, 2). The largest discrepancies occurred during wet periods in summer, where only the lowest values of the chamber measurements often agreed with the FGM estimates. In contrast, the figures corresponded more closely during autumn and winter, as well as during summer drought. We suspect that dense ground vegetation significantly contributes to the CO2 captured under the opaque chambers at the pine site. From the differences between simulated efflux and the chamber measurements (Figures 1, 2), we can derive fractions of up to 50% originating from the ground vegetation during summer. Unfortunately, the 2 years with chamber measurements 2018 (low precipitation) and 2019 (highest air temperature) paralleled to periods of weather extremes (Figure 3). Thus, the weather conditions of the other cooler and wetter years were under-represented and this may be partly responsible for biased calibration of the inverse model.
Several approaches have been developed to calculate soil-atmosphere fluxes using the FGM and subsequently deriving vertical CO2 production profiles (Maier and Schack-Kirchner, 2014). De Jong and Schappert (1972) and several other authors (e.g., Tang et al., 2003, 2005; Hirano, 2005; Maier et al., 2010) have calculated fluxes based on discrete gradients. Here, we followed a different approach, and derived the flux and production profiles through inverse modelling. The soil CO2 concentration profiles were (forward) calculated based on theoretical profiles of the production and diffusivity of soil CO2; the production profile was then optimised to fit the measured soil CO2 concentration profile and the CO2 efflux from chamber measurements. We performed parameter-sweeps for the total pore space of all soil layers and the thickness of the humus layer to identify the most suitable set of parameter values within the observed variability of the soil physical measurements. The selected set of effective soil physical parameters corresponded closely to the concentration profile and the efflux estimates, and line up well with root density profiles at both sites (Figure 4). For a more detailed discussion on the assumptions and limitations of modelling soil gas fluxes, please refer to the Supplementary Material. The soil moisture of the organic layer could not be monitored but was simulated using HYDRUS-1D (see Supplementary Material). Since the potential hydrophobicity of the humus layer (Doerr et al., 2000) was not included in the model, there may be additional uncertainty concerning estimates of the humus moisture content.
Our approach did not allow direct differentiation between Rh and Ra. However, combining our method with an analysis of the isotopic signatures of CO2 would allow those options. In addition, data on the vertical distribution and turnover of roots at the specific measuring points for CO2 concentrations where the FGM was applied, would improve interpretability and open up further options for partitioning Rs into Rh and Ra.
Conclusion
The climate change scenarios projected for Central Europe with increasing temperatures and more frequent and intense droughts will noticeably impact the intensity of soil CO2 production in forest ecosystems and will affect primary production. Our results confirm the hypothesis that soil temperature and moisture are the main determining factors of soil CO2 production, although soil moisture affected the beech site considerably more than the pine site. For both the pine forest and the beech forest, rising temperatures generally lead to an increase in the CO2 production of soils; this could contribute to a decrease in SOC stocks. However, lower precipitation also seems to limit soil CO2 production, particularly at the beech site. Based on our results, we conclude that drought reduced Rh at both sites, but also decreased Ra in the beech stand. The consequences of drought for the SOC stock depend on whether drought-induced reduction mainly affects Ra or Rh. The similar CO2 efflux observed at both the beech site and the pine site contrasted with the observed higher NPP of the beech stand. This might indicate rising SOC stocks in the beech stand in contrast to stocks in the pine stand. Further detailed studies on soil CO2 production, including vertical partitioning and analysis of autotrophic and heterotrophic fractions, e.g., using isotope techniques, are necessary to better estimate the relationship between primary production and soil CO2 production under future climate scenarios. Differences in seasonal and spatial patterns of the soil CO2 production between the two forest sites could be explained by specific physiological activities of the tree species during needle/leaf development and by their different rooting depths. The different properties of the tree species involved in forest conversion, e.g., deciduous vs. coniferious trees, their vertical root distribution, and their effects on soil CO2 production must be taken into account for forest management when addressing climate change mitigation strategies.
Data Availability Statement
The raw data supporting the conclusions of this article will be made available by the authors, without undue reservation.
Author Contributions
HJ and SW conceptualised the investigation, acquired funding, and conducted the installation of measurement devices. HJ conducted data curation, evaluated and visualised the data, and wrote the original draft. VG and MM developed the inverse model, carried out the simulations regarding the inverse model and the multivariate analyses, and wrote the corresponding text parts. SP conducted the measurement of soil gas diffusion coefficients. CH carried out simulations with HYDRUS-1D. HG advised simulations with HYDRUS-1D and wrote the corresponding text parts. All authors reviewed and edited the text.
Funding
The investigation was financially supported by institutional funding of the Leibniz Centre for Agricultural Landscape Research (ZALF). Investments were funded by the European Regional Development Fund (ERDF) of the EC (CCI code 2007 DE 16 1 PO 002).
Conflict of Interest
The authors declare that the research was conducted in the absence of any commercial or financial relationships that could be construed as a potential conflict of interest.
Publisher’s Note
All claims expressed in this article are solely those of the authors and do not necessarily represent those of their affiliated organizations, or those of the publisher, the editors and the reviewers. Any product that may be evaluated in this article, or claim that may be made by its manufacturer, is not guaranteed or endorsed by the publisher.
Acknowledgments
We would like to thank Alexander Russ and Winfried Riek (Eberswalde Forestry Competence Centre, LFE) for providing us with Supplementary Data from the Level II plot with pine. We thank Dieter Sowa and Marten Schmidt for the excellent operational and technical maintenance of the measurement devices used during the study period. Special thanks go to Lisa Koch and Annina Hittmeyer for the careful data processing. We are grateful for the helpful comments and suggestions from two reviewers.
Supplementary Material
The Supplementary Material for this article can be found online at: https://www.frontiersin.org/articles/10.3389/ffgc.2022.826298/full#supplementary-material
References
Achilles, F., Tischer, A., Bernhardt-Römermann, M., Heinze, M., Reinhardt, F., Makeschin, F., et al. (2021). European beech leads to more bioactive humus forms but stronger mineral soil acidification as Norway spruce and Scots pine – results of a repeated site assessment after 63 and 82 years of forest conversion in Central Germany. For. Ecol. Manage. 483:118769. doi: 10.1016/j.foreco.2020.118769
Ahrens, B., Hansson, K., Solly, E. F., and Schrumpf, M. (2014). Reconcilable differences: a joint calibration of fine-root turnover times with radiocarbon and minirhizotrons. New Phytol. 204, 932–942. doi: 10.1111/nph.12979
Bispo, A., Andersen, L., Angers, D. A., Bernoux, M., Brossard, M., Cécillon, L., et al. (2017). Accounting for carbon stocks in soils and measuring GHGs emission fluxes from soils: do we have the necessary standards? Front. Environ. Sci. 5:41. doi: 10.3389/fenvs.2017.00041
BMEL (2015). The Forests in Germany – Selected Results of the Third National Forest Inventory. Bonn: Federal Ministry of Food and Agriculture (BMEL).
Bond-Lamberty, B., and Thomson, A. (2010). A global database of soil respiration data. Biogeosciences 7, 1915–1926. doi: 10.5194/bg-7-1915-2010
Borken, W., and Beese, F. (2005). Soil respiration in pure and mixed stands of European beech and Norway spruce following removal of organic horizons. Can. J. For. Res. 35, 2756–2764. doi: 10.1139/x05-192
Borken, W., Savage, K., Davidson, E. A., and Trumbore, S. E. (2006). Effects of experimental drought on soil respiration and radiocarbon efflux from a temperate forest soil. Glob. Change Biol. 12, 177–193. doi: 10.1111/j.1365-2486.2005.001058.x
Borken, W., Xu, Y. J., Brumme, R., and Lamersdorf, N. (1999). A climate change scenario for carbon dioxide and dissolved organic carbon fluxes from a temperate forest soil: drought and rewetting fffects. Soil Sci. Soc. Am. J. 63, 1848–1855. doi: 10.2136/sssaj1999.6361848x
Borken, W., Xu, Y. J., Davidson, E. A., and Beese, A. (2002). Site and temporal variation of soil respiration in European beech, Norway spruce, and Scots pine forests. Glob. Change Biol. 8, 1205–1216. doi: 10.1046/j.1365-2486.2002.00547.x
Bowden, R. D., Nadelhoffer, K. J., Boone, R. D., Melillo, J. M., and Garrison, J. B. (1993). Contributions of aboveground litter, belowground litter, and root respiration to total soil respiration in a temperate mixed hardwood forest. Can. J. For. Res. 23, 1402–1407. doi: 10.1139/x93-177
Brumme, R., Borken, W., and Prenzel, J. (2009). “Soil respiration” in Functioning and Management of European Beech Ecosystems, eds R. Brumme and P. K. Khanna (Berlin: Springer), 337–351.
Brunner, I., Bakker, M. R., Bjork, R. G., Hirano, Y., Lukac, M., Aranda, X., et al. (2013). Fine-root turnover rates of European forests revisited: an analysis of data from sequential coring and ingrowth cores. Plant Soil 362, 357–372. doi: 10.1007/s11104-012-1313-5
Comeau, L. P., Lai, D. Y. F., Cui, J. J., and Farmer, J. (2018). Separation of soil respiration: a site-specific comparison of partition methods. Soil 4, 141–152. doi: 10.5194/soil-4-141-2018
Conant, R. T., Ryan, M. G., Agren, G. I, Birge, H. E., Davidson, E. A., Eliasson, P. E., et al. (2011). Temperature and soil organic matter decomposition rates – synthesis of current knowledge and a way forward. Glob. Change Biol. 17, 3392–3404. doi: 10.1111/j.1365-2486.2011.02496.x
D’Andrea, E., Guidolotti, G., Scartazza, A., De Angelis, P., and Matteucci, G. (2020). Small-scale forest structure influences spatial variability of belowground carbon fluxes in a mature Mediterranean beech forest. Forests 11, 255. doi: 10.3390/f11030255
Davidson, E. A., and Janssens, I. A. (2006). Temperature sensitivity of soil carbon decomposition and feedbacks to climate change. Nature 440, 165–173. doi: 10.1038/nature04514
De Jong, E., and Schappert, H. J. V. (1972). Calculation of soil respiration and activity from CO2 profiles in the soil. Soil Sci. 113, 328–333. doi: 10.1097/00010694-197205000-00006
Diers, M., Weigel, R., Culmsee, H., and Leuschner, C. (2021). Soil carbon and nutrient stocks under Scots pine plantations in comparison to European beech forests: a paired-plot study across forests with different management history and precipitation regimes. For. Ecosyst. 8:47. doi: 10.1186/s40663-021-00330-y
Doerr, S. H., Shakesby, R. A., and Walsh, R. P. D. (2000). Soil water repellency: its causes, characteristics and hydro-geomorphological significance. Earth Sci. Rev. 51, 33–65. doi: 10.1016/S0012-8252(00)00011-8
Elberling, B., and Ladegaard-Pedersen, P. (2005). Subsurface CO2 dynamics in temperate beech and spruce forest stands. Biogeochemistry 75, 479–506. doi: 10.1007/s10533-005-3690-9
Fischer, H., Bens, O., and Huttl, R. F. (2002). Changes in humus form, humus stock and soil organic matter distribution caused by forest transformation in the north-eastern lowlands of germany. Forstwissenschaftliches Centralblatt 121, 322–334. doi: 10.1046/j.1439-0337.2002.02037.x
Förster, A., Hertel, D., Werner, R., and Leuschner, C. (2021). Belowground consequences of converting broadleaf to conifer forest: comparing the fine root systems of European beech and Scots pine. For. Ecol. Manage. 496, 119457. doi: 10.1016/j.foreco.2021.1194571
Freijer, J. I., and Leffelaar, P. A. (1996). Adapted Fick’s law applied to soil respiration. Water Resour. Res. 32, 791–800. doi: 10.1029/95WR03820
Friedrich, J. (1992). Räumliche Variation bodenchemischer und physikalischer Merkmalsgrößen sowie der Wurzelverteilung in Buchen- und Fichtenwaldökosystemen. Ber. Forschzent. Waldökosyst. Reihe A Bd 83:146.
Gaudinski, J. B., Torn, M. S., Riley, W. J., Dawson, T. E., Joslin, J. D., and Majdi, H. (2010). Measuring and modeling the spectrum of fine-root turnover times in three forests using isotopes, minirhizotrons, and the Radix model. Glob. Biochem. Cycles 24:GB3029. doi: 10.1029/2009GB003649
Gaudinski, J. B., Trumbore, S. E., Davidson, E. A., Cook, A. C., Markewitz, D., and Richter, D. D. (2001). The age of fine-root carbon in three forests of the eastern United States measured by radiocarbon. Oecologia 129, 420–429. doi: 10.1007/s004420100746
Goffin, S., Aubinet, M., Maier, M., Plain, C., Schack-Kirchner, H., and Longdoz, B. (2014). Characterization of the soil CO2 production and its carbon isotope composition in forest soil layers using the flux-gradient approach. Agric. For. Meteorol. 188, 45–57. doi: 10.1016/j.agrformet.2013.11.005
Granier, A., Reichstein, M., Bréda, N., Janssens, I. A., Falge, E., Ciais, P., et al. (2007). Evidence for soil water control on carbon and water dynamics in European forests during the extremely dry year: 2003. Agric. For. Meteorol. 143, 123–145. doi: 10.1016/j.agrformet.2006.12.004
Grüneberg, E., Schöning, I., Riek, W., Ziche, D., and Evers, J. (2019). “Carbon stocks and carbon stock changes in german forest soils” in Status and Dynamics of Forests in Germany, eds N. Wellbrock and A. Bolte (New York, NY: Springer International Publishing), 167–198. doi: 10.1007/978-3-030-15734-0_6
Grüneberg, E., Ziche, D., and Wellbrock, N. (2014). Organic carbon stocks and sequestration rates of forest soils in Germany. Glob. Change Biol. 20, 2644–2662. doi: 10.1111/gcb.12558
Hanson, P. J., Edwards, N. T., Garten, C. T., and Andrews, J. A. (2000). Separating root and soil microbial contributions to soil respiration: a review of methods and observations. Biogeochemistry 48, 115–146.
Hartge, K. H., and Horn, R. (1999). Die Physikalische Untersuchung von Böden. Stuttgart: Ferdinand Enke Verlag.
Hertel, D., Strecker, T., Müller-Haubold, H., and Leuschner, C. (2013). Fine root biomass and dynamics in beech forests across a precipitation gradient – is optimal resource partitioning theory applicable to water-limited mature trees? J. Ecol. 101, 1183–1200. doi: 10.1111/1365-2745.12124
Hirano, T. (2005). Seasonal and diurnal variations in topsoil and subsoil respiration under snowpack in a temperate deciduous forest. Glob. Biogeochem. Cycles 19:GB2011. doi: 10.1029/2004gb002259
Höhle, J., Bielefeldt, J., Dühnelt, P., König, N., Ziche, D., Eickenscheidt, N., et al. (2018). “Bodenzustandserhebung im Wald - Dokumentation und Harmonisierung der Methoden,” in Proceedings of the Thünen Working Paper, (Braunschweig: Thünen-Institut für Waldökosysteme).
Hölscher, D., Hertel, D., Leuschner, C., and Hottkowitz, M. (2002). Tree species diversity and soil patchiness in a temperate broad-leaved forest with limited rooting space. Flora Morphol. Distrib. Funct. Ecol. Plants 197, 118–125. doi: 10.1078/0367-2530-00021
Hornschuch, F. (2009). Ausbreitungsstrategien der Feinwurzelsysteme von Wald-Kiefer (Pinus sylvestris L.) und Rot-Buche (Fagus sylvatica L.) in Rein- und Umbaubeständen – Modifikation durch Konkurrenz und kleinräumige Bodenheterogenität. Stuttgart: Ulmer.
Hornschuch, F., Krakau, U., and Riek, W. (2007). “Das Wurzelsystem der Kiefer - artspezifische Strategie und ökologische Anpassungen,” in Eberswalder Forstliche Schriftenreihe -Die Kiefer Im Nordostdeutschen Tiefland – Ökologie Und Bewirtschaftung, Vol. XXXII, eds R. Kätzel, K. Möller, S. Löffler, J. Engel, and K. Liero (Eberswalde: Landesforstanstalt Eberswalde, MLUV), 108–116.
Hübener, H., Bülow, K., Fooken, C., Früh, B., Hoffmann, P., Höpp, S., et al. (2017). ReKliEs-De Ergebnisbericht. doi: 10.2312/WDCC/ReKliEsDe_Ergebnisbericht
ICP-Forests (2021). ICP-Forests [Online]. Eberswalde: Thünen Institute of Forest Ecosystems. Available online at: http://icp-forests.net/ [Accessed 01.11.2021].
Jandl, R., Ledermann, T., Kindermann, G., and Weiss, P. (2021). Soil organic carbon stocks in mixed-deciduous and coniferous forests in austria. Front. For. Glob. Change 4:688851. doi: 10.3389/ffgc.2021.688851
Janssens, I. A., Sampson, D. A., Curiel-Yuste, J., Carrara, A., and Ceulemans, R. (2002). The carbon cost of fine root turnover in a Scots pine forest. For. Ecol. Manage. 168, 231–240. doi: 10.1016/S0378-1127(01)00755-1
Jassal, R., Black, A., Novak, M., Morgenstern, K., Nesic, Z., and Gaumont-Guay, D. (2005). Relationship between soil CO2 concentrations and forest-floor CO2 effluxes. Agric. For. Meteorol. 130, 176–192. doi: 10.1016/j.agrformet.2005.03.005
Jochheim, H., Lüttschwager, D., and Riek, W. (2022). Stem distance as an explanatory variable for the spatial distribution and chemical conditions of stand precipitation and soil solution under beech (Fagus sylvatica L.) trees. J. Hydrol. 608: 127629. doi: 10.1016/j.jhydrol.2022.127629
Jochheim, H., Wirth, S., and Von Unold, G. (2018). A multi-layer, closed-loop system for continuous measurement of soil CO2 concentration. J. Plant Nutr. Soil Sci. 181, 61–68. doi: 10.1002/jpln.201700259
Jurasinski, G., Jordan, A., and Glatzel, S. (2012). Mapping soil CO2 efflux in an old-growth forest using regression kriging with estimated fine root biomass as ancillary data. For. Ecol. Manage. 263, 101–113. doi: 10.1016/j.foreco.2011.09.025
Kasper, J., Leuschner, C., Walentowski, H., Petritan, A. M., and Weigel, R. (2022). Winners and losers of climate warming: declining growth in Fagus and Tilia vs. stable growth in three Quercus species in the natural beech–oak forest ecotone (western Romania). For. Ecol. Manage. 506:119892. doi: 10.1016/j.foreco.2021.119892
Kim, H., Hirano, T., Koike, T., and Urano, S. (2005). Contribution of litter CO2 production to total soil respiration in two different deciduous forests. Phyton Ann. Rei Bot. 45, 385–388.
König, N., Blum, U., Symossek, F., Bartonek, G., Bussian, B., Ellinghaus, R., et al. (2005). Handbuch Forstliche Analytik – Eine Loseblatt-Sammlung der Analysemethoden im Forstbereich. Göttingen: Gutachterausschuss Forstliche Analytik.
Krug, J. H. A. (2018). Accounting of GHG emissions and removals from forest management: a long road from Kyoto to Paris. Carbon Balance Manage. 13:1. doi: 10.1186/s13021-017-0089-6
Lachmann, M. (2002). Intensität und räumliche Verteilung der Durchwurzelung im Buchenbestand in Abhängigkeit von den Bodenbedingungen auf einer Dauerbeobachtungsfläche. Bachelorarbeit Fachhochschule Eberswalde
Leuschner, C., Förster, A., Diers, M., and Culmsee, H. (2022). Are northern German Scots pine plantations climate smart? The impact of large-scale conifer planting on climate, soil and the water cycle. For. Ecol. Manage. 507:120013. doi: 10.1016/j.foreco.2022.120013
Leuschner, C., Wulf, M., Bäuchler, P., and Hertel, D. (2013). Soil C and nutrient stores under scots pine afforestations compared to ancient beech forests in the german pleistocene: the role of tree species and forest history. For. Ecol. Manage. 310, 405–415. doi: 10.1016/j.foreco.2013.08.043
Lorenz, M., and Fischer, R. (2013). “Chapter 2 – pan-european forest monitoring: an overview” in Developments in Environmental Science, eds M. Ferretti and R. Fischer (Amsterdam: Elsevier), 19–32. doi: 10.1016/b978-0-08-098222-9.00002-9
Luo, G. J., Brüggemann, N., Wolf, B., Gasche, R., Grote, R., and Butterbach-Bahl, K. (2012). Decadal variability of soil CO2, NO, N2O, and CH4 fluxes at the Höglwald Forest, Germany. Biogeosciences 9, 1741–1763. doi: 10.5194/bg-9-1741-2012
Maier, M., and Schack-Kirchner, H. (2014). Using the gradient method to determine soil gas flux: a review. Agric. For. Meteorol. 192-193, 78–95. doi: 10.1016/j.agrformet.2014.03.006
Maier, M., Schack-Kirchner, H., Hildebrand, E. E., and Holst, J. (2010). Pore-space CO2 dynamics in a deep, well-aerated soil. Eur. J. Soil Sci. 61, 877–887. doi: 10.1111/j.1365-2389.2010.01287.x
Maier, M., Schack-Kirchner, H., Hildebrand, E. E., and Schindler, D. (2011). Soil CO2 efflux vs. soil respiration: implications for flux models. Agric. For. Meteorol. 151, 1723–1730. doi: 10.1016/j.agrformet.2011.07.006
MLUL (2015). Wälder Brandenburgs. Potsdam: Ministerium für Ländliche Entwicklung, Umwelt und Landwirtschaft des Landes Brandenburg.
Mol Dijkstra, J. P., Reinds, G. J., Kros, H., Berg, B., and De Vries, W. (2009). Modelling soil carbon sequestration of intensively monitored forest plots in Europe by three different approaches. For. Ecol. Manage. 258, 1780–1793. doi: 10.1016/j.foreco.2008.09.011
Montagnoli, A., Terzaghi, M., Di Iorio, A., Scippa, G. S., and Chiatante, D. (2012). Fine-root seasonal pattern, production and turnover rate of European beech (Fagus sylvatica L.) stands in Italy Prealps: possible implications of coppice conversion to high forest. Plant Biosyst. 146, 1012–1022. doi: 10.1080/11263504.2012.741626
Müller, J. (2009). Forestry and water budget of the lowlands in northeast Germany – consequences for the choice of tree species and for forest management. J. Water Land Dev. 13a, 133–148. doi: 10.2478/v10025-010-0024-7
Nadelhoffer, K. J., Boone, R. D., Bowden, R. D., Canary, J. D., Kaye, J., Micks, P., et al. (2004). “The DIRT experiment: litter and root influences on forest soil organic matter stocks and function” in Forest in Time: The Environmental Consequences of 1000 Years Of Change In New England, eds D. Foster and J. Aber (London: Yale University Press), 300–3154.
Ngao, J., Epron, D., Delpierre, N., Breda, N., Granier, A., and Longdoz, B. (2012). Spatial variability of soil CO2 efflux linked to soil parameters and ecosystem characteristics in a temperate beech forest. Agric. For. Meteorol. 154, 136–146. doi: 10.1016/j.agrformet.2011.11.003
Oertel, C., Matschullat, J., Andreae, H., Drauschke, T., Schröder, C., and Winter, C. (2015). Soil respiration at forest sites in Saxony (Central Europe). Environ. Earth Sci. 74, 2405–2412. doi: 10.1007/s12665-015-4241-x
Osterholt, L., Kolbe, S., and Maier, M. (2022). A differential CO2 profile probe approach for field measurements of soil gas transport and soil respiration. J. Plant Nutr. Soil Sci. 185, 282–296. doi: 10.1002/jpln.202100155
Peltoniemi, M., Thürig, E., Ogle, S., Palosuo, T., Schrumpf, M., Wutzler, T., et al. (2007). Models in country scale carbon accounting of forest soils. Silva Fennica 41, 575–602. doi: 10.14214/sf.290
Phillips, S. C., Varner, R. K., Frolking, S., Munger, J. W., Bubier, J. L., Wofsy, S. C., et al. (2010). Interannual, seasonal, and diel variation in soil respiration relative to ecosystem respiration at a wetland to upland slope at Harvard Forest. J. Geophys. Res. 115:G02019. doi: 10.1029/2008JG000858
Pregitzer, K. S., King, J. A., Burton, A. J., and Brown, S. E. (2000). Responses of tree fine roots to temperature. New Phytol. 147, 105–115. doi: 10.1046/j.1469-8137.2000.00689.x
R-Core-Team (2020). A Language And Environment For Statistical Computing. Vienna: Foundation for Statistical Computing.
Reichstein, M., Rey, A., Freibauer, A., Tenhunen, J., Valentini, R., Banza, J., et al. (2003). Modeling temporal and large-scale spatial variability of soil respiration from soil water availability, temperature and vegetation productivity indices. Glob. Biogeochem. Cycles 17:1104. doi: 10.1029/2003gb002035
Riek, W., and Strohbach, B. (2001). “Untersuchungen zum Wasserhaushalt auf Level II-Standorten in Brandenburg” in Forstliche Umweltkontrolle - Ergebnisse aus zehnjährigen Untersuchungen zur Wirkung von Luftverunreinigungen in Brandenburgs Wäldern, ed. Landesforstanstalt. Eberswalde, 116–131.
Ryan, M. G., and Law, B. E. (2005). Interpreting, measuring, and modeling soil respiration. Biogeochemistry 73, 3–27. doi: 10.1007/s10533-004-5167-7
Savage, K. E., and Davidson, E. A. (2001). Interannual variation of soil respiration in two New England forests. Glob. Biogeochem. Cycles 15, 337–350. doi: 10.1029/1999GB001248
Schimel, J. P. (2018). Life in dry soils: effects of drought on soil microbial communities and processes. Annu. Rev. Ecol. Evol. Syst. 49, 409–432. doi: 10.1146/annurev-ecolsys-110617-062614
Schröder, J., Röhle, H., Gerold, D., and Münder, K. (2007). Modeling individual-tree growth in stands under forest conversion in East Germany. Eur. J. For. Res. 126, 459–472. doi: 10.1007/s10342-006-0167-x
Šimůnek, J., Šejna, M., Saito, H., Sakai, M., and Genuchten, M. T. V. (2009). The HYDRUS-1D Software Package For Simulating The One-Dimensional Movement Of Water, Heat, And Multiple Solutes In Variably-Saturated Media – Version 4.08. Riverside: Department of Environmental Sciences, University of California.
Six, J., Callewaert, P., Lenders, S., De Gryze, S., Morris, S. J., Gregorich, E. G., et al. (2002). Measuring and understanding carbon storage in afforested soils by physical fractionation. Soil Sci. Soc. Am. J. 66, 1981–1987. doi: 10.2136/sssaj2002.1981
Søe, A. R. B., and Buchmann, N. (2005). Spatial and temporal variations in soil respiration in relation to stand structure and soil parameters in an unmanaged beech forest. Tree Physiol. 25, 1427–1436. doi: 10.1093/treephys/25.11.1427
Solly, E. F., Brunner, I., Helmisaari, H. S., Herzog, C., Leppalammi-Kujansuu, J., Schoning, I., et al. (2018). Unravelling the age of fine roots of temperate and boreal forests. Nat. Commun. 9:3006. doi: 10.1038/s41467-018-05460-6
Spiecker, H. (2003). Silvicultural management in maintaining biodiversity and resistance of forests in Europe—temperate zone. J. Environ. Manage. 67, 55–65. doi: 10.1016/S0301-4797(02)00188-3
Subke, J. A., Inglima, I., and Cotrufo, M. F. (2006). Trends and methodological impacts in soil CO2 efflux partitioning: a metaanalytical review. Glob. Change Biol. 12, 921–943. doi: 10.1111/j.1365-2486.2006.01117.x
Sulzman, E. W., Brant, J. B., Bowden, R. D., and Lajtha, K. (2005). Contribution of aboveground litter, belowground litter, and rhizosphere respiration to total soil CO2 efflux in an old growth coniferous forest. Biogeochemistry 73, 231–256. doi: 10.1007/s10533-004-7314-6
Tang, J., Baldocchi, D. D., and Xu, L. (2005). Tree photosynthesis modulates soil respiration on a diurnal time scale. Glob. Change Biol. 11, 1298–1304. doi: 10.1111/j.1365-2486.2005.00978.x
Tang, J., Baldocchi, D. D., Qi, Y., and Xu, L. (2003). Assessing soil CO2 efflux using continuous measurements of CO2 profiles in soils with small solid-state sensors. Agric. For. Meteorol. 118, 207–220. doi: 10.1016/S0168-1923(03)00112-6
Vesterdal, L., Clarke, N., Sigurdsson, B. D., and Gundersen, P. (2013). Do tree species influence soil carbon stocks in temperate and boreal forests? For. Ecol. Manage. 309, 4–18. doi: 10.1016/j.foreco.2013.01.017
von Buttlar, J., Zscheischler, J., Rammig, A., Sippel, S., Reichstein, M., Knohl, A., et al. (2018). Impacts of droughts and extreme-temperature events on gross primary production and ecosystem respiration: a systematic assessment across ecosystems and climate zones. Biogeosciences 15, 1293–1318. doi: 10.5194/bg-15-1293-2018
von Rein, I., Gessler, A., Premke, K., Keitel, C., Ulrich, A., and Kayler, Z. E. (2016). Forest understory plant and soil microbial response to an experimentally induced drought and heat-pulse event: the importance of maintaining the continuum. Glob. Change Biol. 22, 2861–2874. doi: 10.1111/gcb.13270
Wang, C. G., Brunner, I., Zong, S. W., and Li, M. H. (2019). The dynamics of living and dead fine roots of forest biomes across the northern hemisphere. Forests 10:953. doi: 10.3390/f10110953
Wang, Y. F., Hao, Y. B., Cui, X. Y., Zhao, H. T., Xu, C. Y., Zhou, X. Q., et al. (2014). Responses of soil respiration and its components to drought stress. J. Soils Sediments 14, 99–109. doi: 10.1007/s11368-013-0799-7
Webster, K. L., Creed, I. F., Skowronski, M. D., and Kaheil, Y. H. (2009). Comparison of the performance of statistical models that predict soil respiration from forests. Soil Sci. Soc. Am. J. 73, 1157–1167. doi: 10.2136/sssaj2008.0310
Wellbrock, N., Bolte, A., and Flessa, H. (2016). Dynamik Und Räumliche Muster Forstlicher Standorte in Deutschland – Ergebnisse der Bodenzustandserhebung im Wald. Braunschweig: Johann Heinrich von Thünen-Institut.
Wellbrock, N., Grüneberg, E., Riedel, T., and Polley, H. (2017). Carbon stocks in tree biomass and soils of German forests. Cent. Eur. For. J. 63, 105–112. doi: 10.1515/forj-2017-0013
Wordell-Dietrich, P., Wotte, A., Rethemeyer, J., Bachmann, J., Helfrich, M., Kirfel, K., et al. (2020). Vertical partitioning of CO2 production in a forest soil. Biogeosciences 17, 6341–6356. doi: 10.5194/bg-17-6341-2020
WRB (2015). World Reference Base for Soil Resources 2014, Update 2015 - International Soil Classification System for Naming Soils and Creating Legends for Soil Maps. World Soil Resources Reports 106. Rome: FAO.
Wulf, M., Jahn, U., Meier, K., and Radtke, M. (2017). Tree species composition of a landscape in north-eastern Germany in 1780, 1890 and 2010. Forestry 90, 174–186. doi: 10.1093/forestry/cpw061
Zerbe, S. (2002). Restoration of natural broad-leaved woodland in Central Europe on sites with coniferous forest plantations. For. Ecol. Manage. 167, 27–42. doi: 10.1016/S0378-1127(01)00686-7
Keywords: soil respiration, CO2 efflux, vertical partitioning, beech forest, pine forest, flux-gradient method, chamber measurement, drought
Citation: Jochheim H, Wirth S, Gartiser V, Paulus S, Haas C, Gerke HH and Maier M (2022) Dynamics of Soil CO2 Efflux and Vertical CO2 Production in a European Beech and a Scots Pine Forest. Front. For. Glob. Change 5:826298. doi: 10.3389/ffgc.2022.826298
Received: 30 November 2021; Accepted: 21 April 2022;
Published: 11 May 2022.
Edited by:
Arthur Gessler, Swiss Federal Institute for Forest, Snow and Landscape Research (WSL), SwitzerlandReviewed by:
Werner Borken, University of Bayreuth, GermanyZachary E. Kayler, University of Idaho, United States
Copyright © 2022 Jochheim, Wirth, Gartiser, Paulus, Haas, Gerke and Maier. This is an open-access article distributed under the terms of the Creative Commons Attribution License (CC BY). The use, distribution or reproduction in other forums is permitted, provided the original author(s) and the copyright owner(s) are credited and that the original publication in this journal is cited, in accordance with accepted academic practice. No use, distribution or reproduction is permitted which does not comply with these terms.
*Correspondence: Hubert Jochheim, hubert.jochheim@zalf.de
†Present addresses: Sinikka Paulus Max Planck Institute for Biogeochemistry, Jena, Germany; Christoph Haas Institute of Plant Nutrition and Soil Science, Kiel University, Kiel, Germany