Factors influencing tree biomass and carbon stock in the Western Himalayas, India
- Soil Ecosystem and Restoration Ecology Lab, Department of Botany, Panjab University, Chandigarh, India
The assessment of tree biomass and its carbon (C) stock at the local and regional level is considered a crucial criterion for understanding the impact of changing environments on the global carbon cycle. In this context, we selected three sites in the western Himalayas, covering parts of Himachal Pradesh and north-eastern Haryana. Each study site experiences distinct climatic conditions, vegetation types, and elevations. We seek to elucidate the determinants of tree biomass and carbon stock across different forest types in the Western Himalayas. We found that temperate forests contributed the most biomass and carbon stock, with Cedrus deodara attaining the highest values of 782.6 ± 107.9 Mg/ha and 360 ± 49.7 Mg C/ha. In contrast, Quercus leucotrichophora mixed temperate had the lowest 286.6 ± 57.2 and 128.9 ± 25.7 Mg/C ha, respectively. Only a few species, such as Abies pindrow, Cedrus deodara, Quercus floribunda, and Quercus semecarpifolia, accounted for significant biomass and carbon stock. The lower elevation subtropical forests had the highest species richness (8–12 species) and stem density (558.3 ± 62.9 to 866.6 ± 57.7 trees/ha). Furthermore, tree diameter, total basal cover, and height emerged as the strongest predictors of biomass and C stock. The remaining variables showed no significant associations, including species diversity, climatic attributes and elevation. Thus, our study extended the assertion that vegetation composition and structural attributes, apart from climatic and topographic factors, are equally important in determining biomass and C stock in forest ecosystems. Our study indicated that the temperate forests in the western Himalayas possess significant carbon storage and climate change mitigation potential.
1 Introduction
Anthropogenic activities have raised the earth’s temperature by 1°C beyond pre-industrial levels, and this is expected to climb to 1.5°C by 2,052 if current emission rates continue (IPCC, 2018). The 2°C of global warming is anticipated to negatively influence livelihood, food security, health, and biodiversity (Smith et al., 2018). Therefore, countries have set targets to keep global temperatures below 2°C within the framework of the Paris Agreement. Furthermore, the pact requires countries to reach carbon (C) neutrality (zero C emissions) by the second half of this century (UNFCCC, 2015). As a result, mitigation techniques focus primarily on CO2 removal from the atmosphere and its secure storage. In this context, forests provide a viable solution because they cover around 31% of the earth’s surface area (FAO, 2022) and sequester 15–20% of annual human C emissions (Le Quéré et al., 2018; Case et al., 2021). Furthermore, around 80% of Earth’s total plant biomass is confined to forests (Kindermann et al., 2008) and they hold a more significant amount of carbon in their biomass and soil than stored in the atmosphere (Pan et al., 2013). Contrastingly, tropical forests have the highest C storage capacity (471 Pg C), while boreal and temperate forests have 272 Pg C and 119 Pg C, respectively (Pan et al., 2011). Most carbon is stored in aboveground biomass (AGB) components in tropical forests, whereas C is limited to belowground, primarily soils, in boreal and temperate forests (Malhi et al., 1999). Given their high C sequestration potential, the assessment of biomass and C stock inventories at local or regional scale is crucial for understanding the contribution of forests to the global carbon cycle, particularly in the context of changing climatic conditions (Gibbs et al., 2007; Huynh et al., 2023). Furthermore, this information is valuable in attaining the objectives of global obligations such as the “Reduction of Emissions from Deforestation and Forest Degradation (REDD)” initiative, which aims to offset forest loss and earn carbon credits (Lung and Espira, 2015; Sahoo et al., 2021).
The carbon pool in forests varies widely on a regional and global scale. Various factors, including vegetation composition, structural attributes, topography, climatic conditions, disturbances, and stand age, are attributed to this variation (Pregitzer and Euskirchen, 2004; Islam et al., 2017; Kothandaraman et al., 2020; Gogoi et al., 2022). Tree C storage, especially aboveground, is a function of various structural attributes, such as stem density, mean tree diameter (Poorter et al., 2015; Islam et al., 2017), height (Moles et al., 2009), and basal area (Mensah et al., 2016, 2020). The role of DBH in predicting C stock is more pronounced in temperate forests, as a significant fraction of the AGB (40%) is comprised of large diameter (>60 cm) trees (Lutz et al., 2018). Apart from this, species diversity influences C stock in many ways as positive (Mensah et al., 2016; Lie et al., 2018; Kaushal and Baishya, 2021), negative (Jerzy and Anna, 2007), and no relationships (Khanalizadeh et al., 2023; Pinto et al., 2023) have been observed globally.
In addition to these, environmental variables also play a crucial role in forest C stock. For example, topographic elements (elevation, slope and aspect) create microclimatic conditions and regulate soil moisture, light availability and vegetation patterns, ultimately determining biomass. Among topographic elements, elevation is the most studied factor (Singh, 2018; Cheng et al., 2023) due to its role in vegetation patterns and productivity through temperature and precipitation effects (Xu et al., 2017; Sanaei et al., 2018). Studies have shown that climatic variables (temperature and precipitation) influence forest biomass through direct and indirect effects on species diversity (Stegen et al., 2011; Mensah et al., 2023).
The Indian Himalayan region (IHR), a biodiversity hotspot, is home to varied species of flora and fauna and provides numerous ecosystem services (Negi et al., 2019; Ahirwal et al., 2021) such as carbon sequestration, water regulation and livelihood to a million of people. Considering its vast natural wealth and unique environmental conditions, IHR is reported to sequester 65 million tonnes of carbon annually (Tolangay and Moktan, 2020) and possess more significant climate change mitigation potential. In this context, the Central and Western Himalaya has been investigated extensively for biomass and carbon stock estimation (Sharma et al., 2010, 2016, 2018; Gairola et al., 2011; Dar and Sundarapandian, 2015; Dar et al., 2017; Kaushal and Baishya, 2021; Dar and Parthasarathy, 2022; Haq et al., 2022; Tiwari et al., 2023). Meanwhile, in terms of factors influencing C storage, Himachal Pradesh and the bordering Siwalik ranges in the Western Himalayas are less explored. Despite the limited number of studies (Nagar, 2012; Banday et al., 2017; Chisanga et al., 2018; Singh and Verma, 2018; Bhardwaj et al., 2021; Kumari et al., 2022), the region still lacks a thorough understanding of the variables impacting biomass and C stock development over a wide spatial scale. Therefore, the current research aims to understand the carbon stock dynamics in different forest ecosystems, each with contrasting climatic conditions and elevations. Furthermore, due to the inherent vulnerability of the Himalayan region and its significant degree of disturbance, we have chosen three designated protected areas (Wildlife Sanctuaries) as the focal sites for our investigation. Because protected sites bear high species richness and offer multiple ecosystem services including C sequestration (Collins and Mitchard, 2017). A recent study showed that approximately 26% of terrestrial woody C is present in AGC stock of protected areas (Duncanson et al., 2023). The current investigation addresses the following questions: (1) What is the tree biomass and carbon stock status across the studied region? (2) What are the determinants of tree biomass and carbon stock? (3) How do vegetation types and forest attributes influence biomass and C stock?
2 Materials and methods
2.1 Study sites
The study sites are located in the lesser Himalayan region of Himachal Pradesh (H.P.) (32.1024° N, 77.5619° E) and the Siwalik region of Haryana (29.0588° N, 76.0856° E) states, which are situated in the north-western part of India (Table 1; Figure 1). The chosen study sites are part of three Wildlife Sanctuaries (WLS), specifically Khol Hi-Raitan, commonly referred to as Morni Hills (hereafter KHR) (300–800 m), Chail WLS (900–2,100 m), and Churdhar WLS (1,900–3,600 m) (Figure 1). The former falls within the jurisdiction of the Haryana State Forest Department, while the latter two are under the control of the H.P. Wildlife Department. These study sites displayed considerable heterogeneity in climatic conditions, topographic features, and elevation levels (Figure 2). The KHR WLS experiences a subtropical monsoonal climate characterized by seasonal patterns of hot summers, wet monsoons, and cold winters. The annual temperature ranges from 3°C during the winter season to 44°C during the summer season. The annual precipitation exhibited an average of 1,200 mm, with most rainfall from July to September. The Chail WLS represents a transition zone between subtropical and temperate environments. It has a subtropical climate at lower elevations and a temperate one at higher reaches. The annual precipitation averaged 1,700 mm during the monsoon season (July to September). The maximum temperature can reach 35°C in summer, whereas the minimum temperature can drop below 0°C in winter. The Churdhar WLS spans across a wide elevation range of 1,900–3,600 m with temperate humid climates. It experiences cool, pleasant summer weather, where the temperature hardly exceeds 23°C, whereas it drops below freezing during winter. The study area receives annual precipitation averaging 1,600 mm, typically in rainfall and snow. The higher reaches of Churdhar WLS remain snow-covered from early December to March.
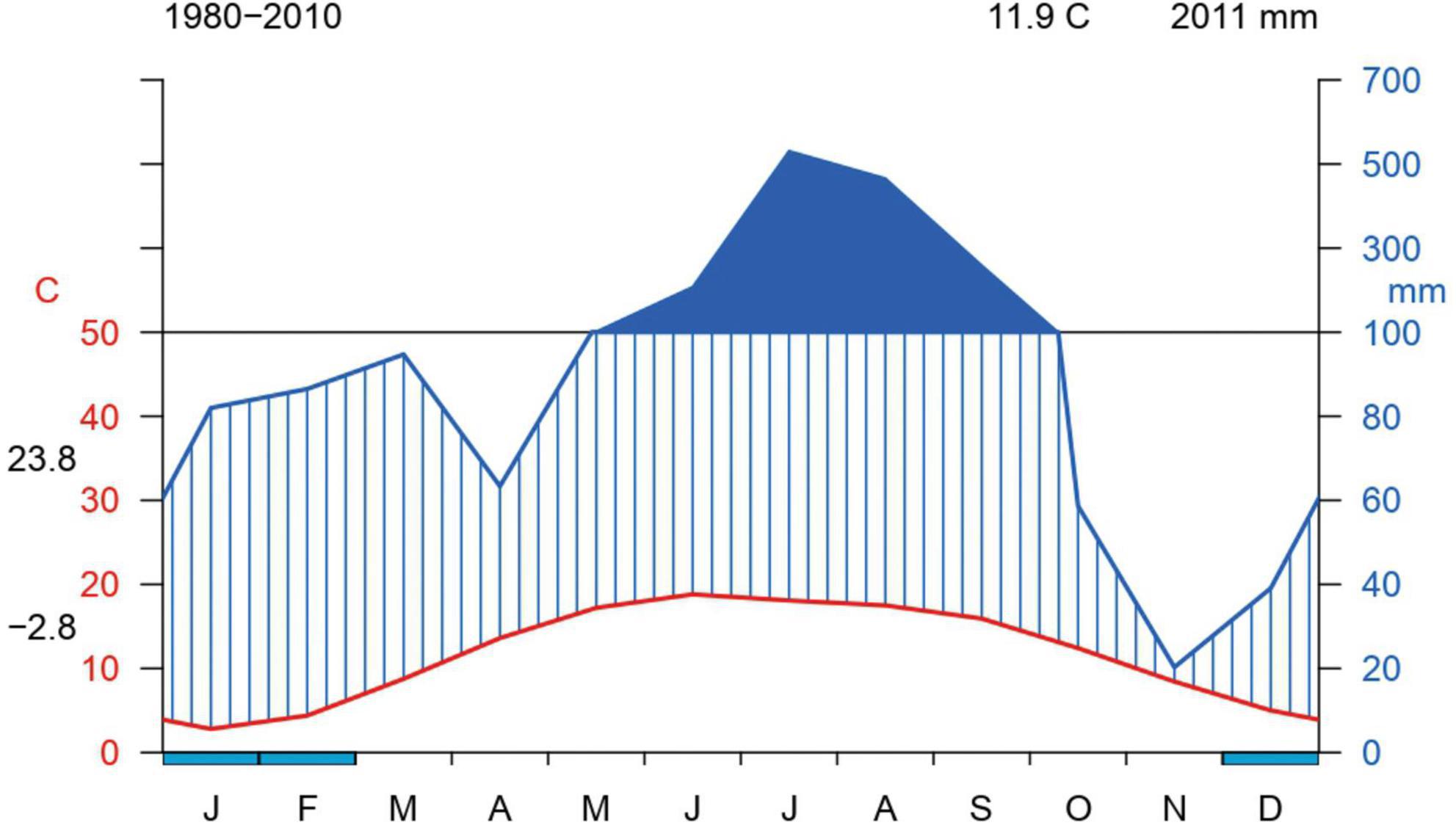
Figure 2. Walter and Lieth climate diagram for the sampling plots illustrating the variation in average monthly temperature (red) and precipitation (blue) from 1980 to 2010.
2.2 Sampling design and data collection
A preliminary survey was undertaken in 2020-21 across the proposed sampling sites to evaluate various factors, including vegetation types, elevation, and topography. The vegetation at the three study sites was categorized into eight forest types (FT) based on visual observation, following the classification of Indian forests by Champion and Seth (1968) (Table 1; Supplementary Figure 3). Tree inventory was conducted using square plots of 31.6 m × 31.6 m (equivalent to 0.1 hectares) (FSI, 2002). A total of 3 plots (0.3 ha) were established at each forest type. However, in a few forests at KHR, where the topography exhibited undulating or steep slopes, a plot measuring 10 m × 10 m was chosen as the optimal size. Plot selection was based on considering the threat of disturbance, as forests near human settlements or the periphery of WLS were avoided. To investigate the impact of elevation on stand structural metrics, a minimum distance of 100 meters was maintained between consecutive plots, if feasible. Geographical coordinates and elevation data were collected via a portable Global Positioning System (GPS) device (Garmin eTrex 10 model). The identification of trees that fell within each sampling plot was done with established sources such as the Flora of Himachal Pradesh (Chowdhery and Wadhwa, 1984), the Floristic reconnaissance of Churdhar Wildlife Sanctuary (Choudhary and Lee, 2012; Subramani et al., 2014), and the Flora of Haryana (Kumar, 2001). In each sampling plot, all trees with a girth of more than 10 cm were measured for circumference over bark at a height of 1.37 m. The measured values were then converted to diameter by dividing the circumference by 3.14. The tree height was measured using a BLUME-LEISS altimeter. The mean diameter of trees at breast height (DBH) was used to calculate tree basal area. The tree basal cover within each plot was summed up to get the total basal cover (TBC), expressed as basal cover/hectare. The stem density or tree density (SD) represents the number of tree individual per area is expressed as individuals/hectare. Species richness was determined using Margalef’s richness index (SR) = S-1/ln N (Margalef, 1958), where S = total number of species, ln = natural log and N = total number of individuals. For the determination of species diversity, the Shannon-Weiner (H’) index was used: H’ = −∑ ni/N ln ni/N (Shannon and Weaver, 1949), where ni represents the number of individuals of the ith species, and N is the number of individuals of all species in the population. The climate data including mean annual temperature (MAT) and total annual precipitation (MAP) for the study sites was accessed at 30-arc-sec (∼1 km) resolution from the CHELSA1 database version 2.1 (Karger et al., 2017). The values of these variables were extracted for a particular sampling plot using the R package terra version 1.7.39 (Hijmans, 2023).
2.3 Estimation of tree biomass and carbon stock
A non-destructive approach based on allometric equations was used to estimate aboveground tree biomass. Firstly, the volume of individual tree species was computed using species-specific volumetric equations developed by the Forest Survey of India (FSI, 1996; Supplementary Table 1), and the volume of all the tree species in a plot was summed up to get the growing stock volume density (GSVD m3 ha). To get aboveground biomass (AGB), the GSVD was multiplied with the appropriate biomass expansion factor (BEF) available for hardwood and coniferous species (Pine and Spruce-fir), as given in Sharma et al. (2010, 2016) (Supplementary Table 1). For the estimation of belowground biomass (BGB), the following equation given by Cairns et al. (1997) was used: BGB = exp [−1.059 + 0.884 × ln (AGB) + 0.284]. Both AGB and BGB were summed to get total biomass (TB). For the calculation of carbon stock, the following formula was used: Carbon stock (Mg C/ha) = Biomass (Mg/ha) × C (%). When evergreen coniferous species comprise more than 50% of forest types, a carbon factor of 46% was employed. Conversely, a carbon factor of 45% was used where broadleaved species were predominant (Negi et al., 2003).
2.4 Data analysis
Before subjecting the statistical analysis, the data were checked for normality assumptions using the Shapiro-Wilk test. Differences between forest attributes (stem density, diameter at breast height, tree height, total basal cover and biomass) among forest types were tested through one-way analysis of variance (One-way ANOVA) and Tukey’s HSD post-hoc test. Pearson’s correlation test was performed to check if there is a significant association between carbon density and its candidate variables. The “corrplot” package in R was used for correlogram preparation (Wei and Simko, 2021). Linear regression analyses were used to study the effect of explanatory variables on C stock. In addition, we also performed the principal component analysis (PCA), as it can reduce a large number of variables to a few main variables without compromising the data originality (Jolliffe and Cadima, 2016) and effectively remove multicollinearity among variables. The PCA was performed using the package “factoextra” in R (Kassambara and Mundt, 2020). All the statistical tests were performed using packages “stats,” and “multcompView” in R programming language 4.3.0 (Graves et al., 2023; R Core Team, 2023).
3 Results
3.1 Stand composition and structural attributes
A total of 29 tree species were recorded across the studied forest types. The species richness (no. of species) varied from 8 to 12 species in subtropical forests and 3–6 species in temperate forests. The ALD and LSDD forests attained the highest stem density (SD) values (558.3 ± 62.9 and 866.6 ± 57.7), respectively (Figure 3A; Supplementary Table 2). Across the temperate forests, the SD value ranged from (303 ± 20.8 to 573.3 ± 55), with the lowest in CDP and the highest in QSAM. The SD values differed considerably between forest types (F = 18.261, p < 0.001). In terms of DBH, the ALD-dominated stand had the lowest value (23.3 ± 1.6), while the CDP stand had the greatest (46.8 ± 11.6) (Figure 3B). A similar trend was observed for height, with values lowest in ALD (12.1 ± 1.7) and highest in CDP (29.3 ± 1.1) (Figure 3C; Supplementary Table 2). The variation in mean DBH and mean height data across different forest types was statistically significant (F = 4.741, p = 0.005; F = 11.538, p < 0.001). Similarly, the TBC differed significantly among forest types (F = 3.541, p = 0.01), with the highest value (74.4 ± 25.9) in QSAM, while the lowest (24.9 ± 4.01) in ALD (Figure 3D; Supplementary Table 2).
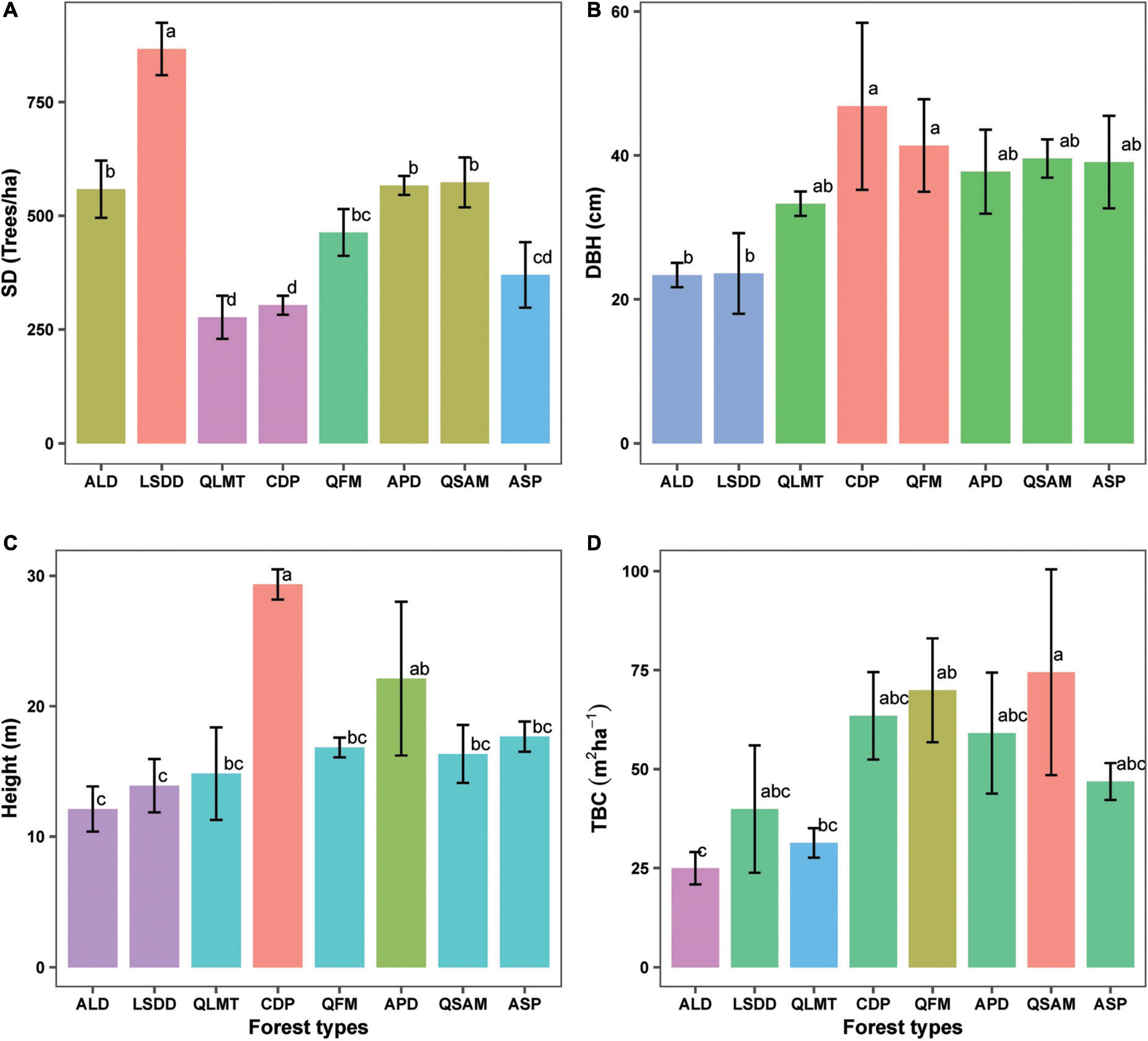
Figure 3. Variations in stand structural attributes (A) stem density, (B) diameter at breast height, (C) tree height, and (D) total basal cover across different forest types. Error bars represent the standard deviation of the data. Forest types are listed in ascending order of elevation. Different letters on bar tips indicate significant differences between mean values by Tukey’s HSD post-hoc test, (p < 0.05).
3.2 Mapping of biomass and carbon stock
The biomass (AGB, BGB, TB) and carbon stocks were highest in Cedrus deodara forest (CDP) with values ranging from (641.7 ± 53.3, 140.9 ± 15.5, 782.6 ± 107.9 Mg/ha and 360 ± 49.7 Mg C/ha), respectively (Supplementary Table 2; Figures 4A–D). Apart from this, temperate forests such as APD (322.1 ± 154.1), QFM (309.2 ± 84.5), and QSAM (284.9 ± 84 Mg C/ha) contributed substantially to C stock formation (Supplementary Table 2; Figure 4D). Among the studied forest types, the QLMT contributed the least to C stock formation (128.9 ± 25.7 Mg C/ha) (Figure 4D). The C stock in subtropical forests, ALD and LSDD, varied between (207.03 ± 19.5 and 250.9 ± 41.4 Mg C/ha), respectively (Supplementary Table 2; Figure 4D).
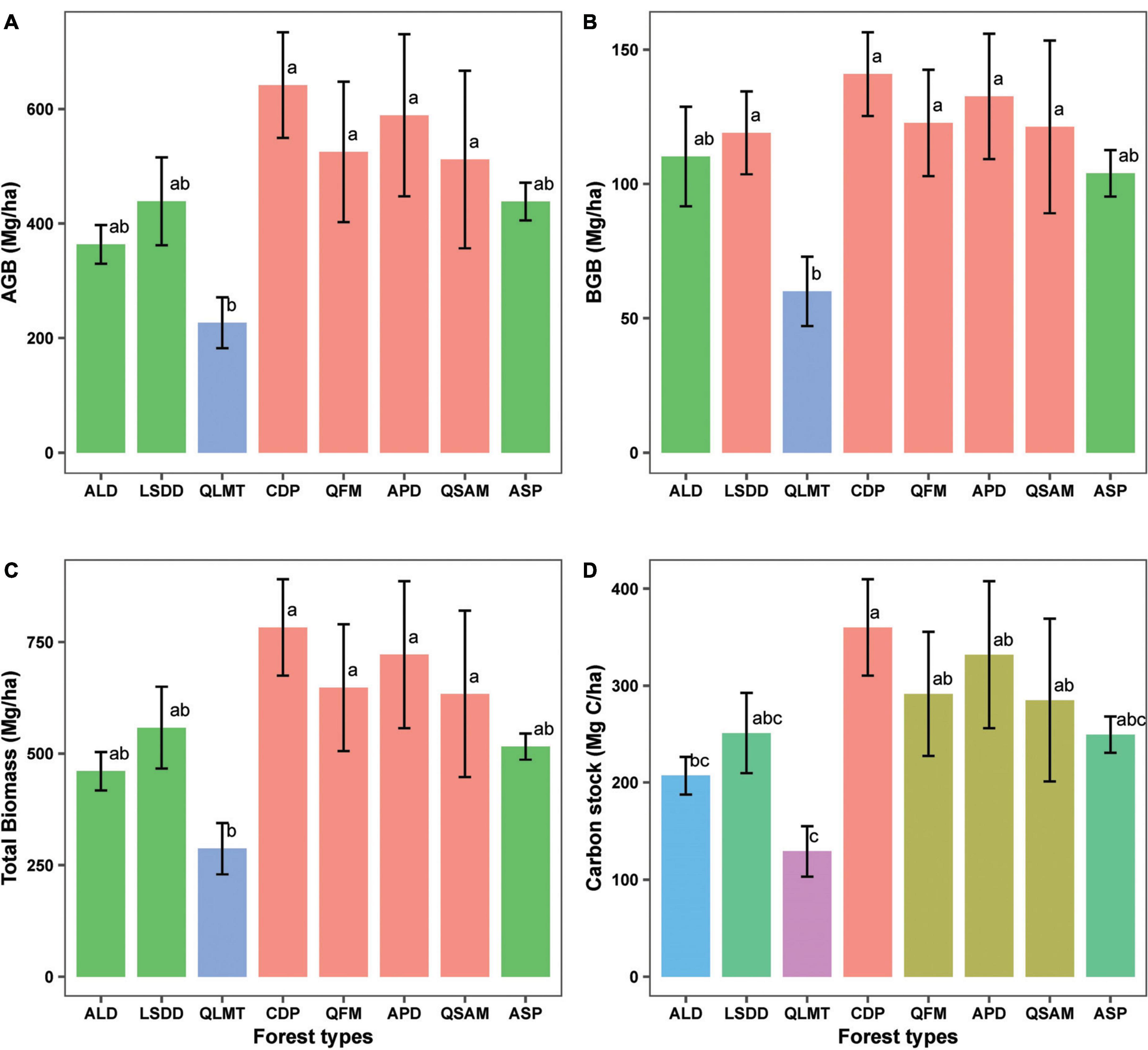
Figure 4. Variations in biomass and C stock (A) aboveground biomass, (B) belowground biomass, (C) total biomass, and (D) carbon stock among different forest types. Error bars represent the standard deviation of data. Forest types are listed in ascending order of elevation. Different letters on bar tips indicate significant differences between mean values by Tukey’s HSD post-hoc test, (p < 0.05).
3.3 Species contribution in C stock formation
In terms of species-wise contribution across all forests, Abies pindrow contributed the maximum to C stock formation (375.1 Mg C/ha), followed by Cedrus deodara (353.5 Mg C/ha), Quercus semecarpifolia (282 Mg C/ha) and Q. floribunda (250 Mg C/ha) (Supplementary Figure 1). In the case of subtropical species, Anogeissus latifolia (143.8 Mg C/ha) contributed the highest in C stock followed by Mallotus philippensis (110.9 Mg C/ha), and Lannea coromandelica (57.8 Mg C/ha) (Supplementary Figure 1).
3.4 Relationships between forest attributes and C stock
Pearson’s correlation matrix showed that C stock is linked with different forest attributes. Among the structural variables, only the TBC (r = 0.80, p < 0.001), DBH (r = 0.65, p < 0.001), and height (r = 0.62, p < 0.01) showed significant association with C stock (Figure 5). In contrast, the other variables directly or indirectly influenced C stock but were not significantly associated. For instance, stem density (r = 0.10, p = 0.64) showed a positive association, whereas diversity attributes (species richness index and Shannon index) were negatively correlated (r = −0.36, p = 0.09; r = −0.35, p = 0.09) (Figure 5). Among climatic variables, we found that MAP was positively correlated (r = 0.27, p = 0.19), whereas MAT (r = −0.29, p = 0.17) had a negative association. Similarly, elevation (r = 0.31, p = 0.14) showed a positive association with C stock, but its effect was not significant.
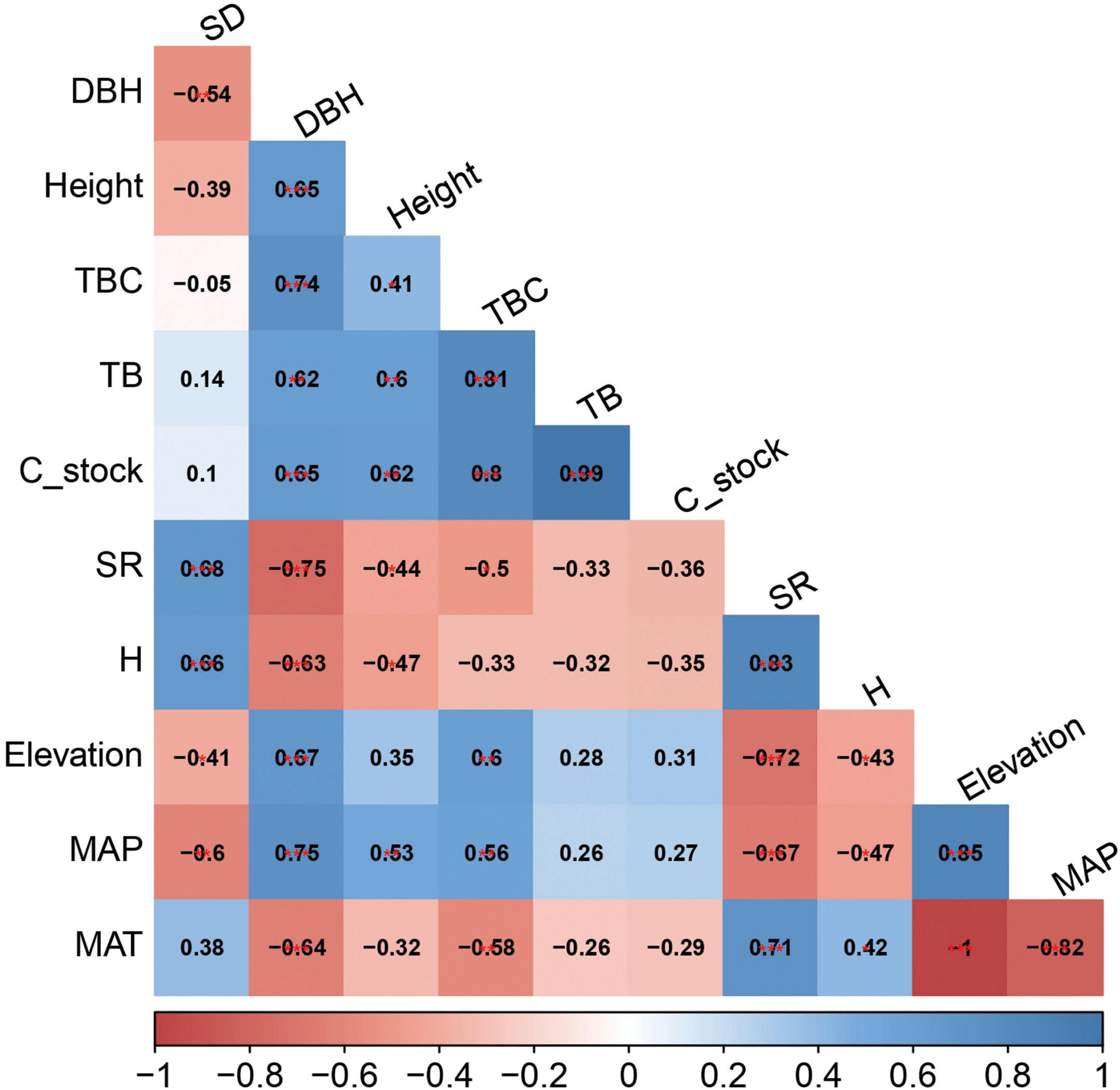
Figure 5. Pearson correlogram matrix between variables and carbon stock. SD, stem density; DBH, diameter at breast height; TBC, total basal cover; TB, total biomass; SR, species richness index; H, Shannon diversity index; MAP, total annual precipitation; MAT, mean annual temperature.
The candidate variables were found to exhibit a correlation with each other. For example, stem density showed a negative correlation with DBH (r = −0.54, p < 0.01), whereas it was positively associated with species richness (r = 0.68, p < 0.001) and Shannon diversity (r = 0.66, p < 0.001). Elevation displayed a significant influence on climatic variables and diversity attributes, such as MAP (r = 0.85, p < 0.001) and MAT (r = −1, p < 0.001), SR (r = −0.72, p < 0.001) and H‘ (r = −0.43, p < 0.05) (Figure 5).
3.5 Factors influencing biomass and C stock
The bivariate analyses revealed that structural attributes except for stem density (Supplementary Figure 2A) explained the most significant variation among all the variables tested against C stock. The total basal cover (TBC) emerged as the strongest predictor of C stock (64%), followed by DBH (42%) and height (39%) (Figure 6). The rest of the tested variables showed no significant variation in C stock, with a contribution of SR and H′ varied from 13% and 12%, respectively (Supplementary Figures 2B, C). Climatic variables, including MAP and MAT, displayed only 7.5% and 8.5% of the variation in C stock (Supplementary Figures 2D, E). Among the topographic factors, elevation explains 9% of the variance (Supplementary Figure 2F). Furthermore, the principal component analysis (PCA) biplot explains the factors affecting biomass and C stock. It demonstrates that factors such as TBC, DBH, height, and MAP are grouped with biomass and C stock, whereas MAT, SD, species richness (SR), and Shannon diversity (H′) are oppositely placed. The principal component axes (PC1 and PC2) explained 77.5% of the variance (Figure 7).
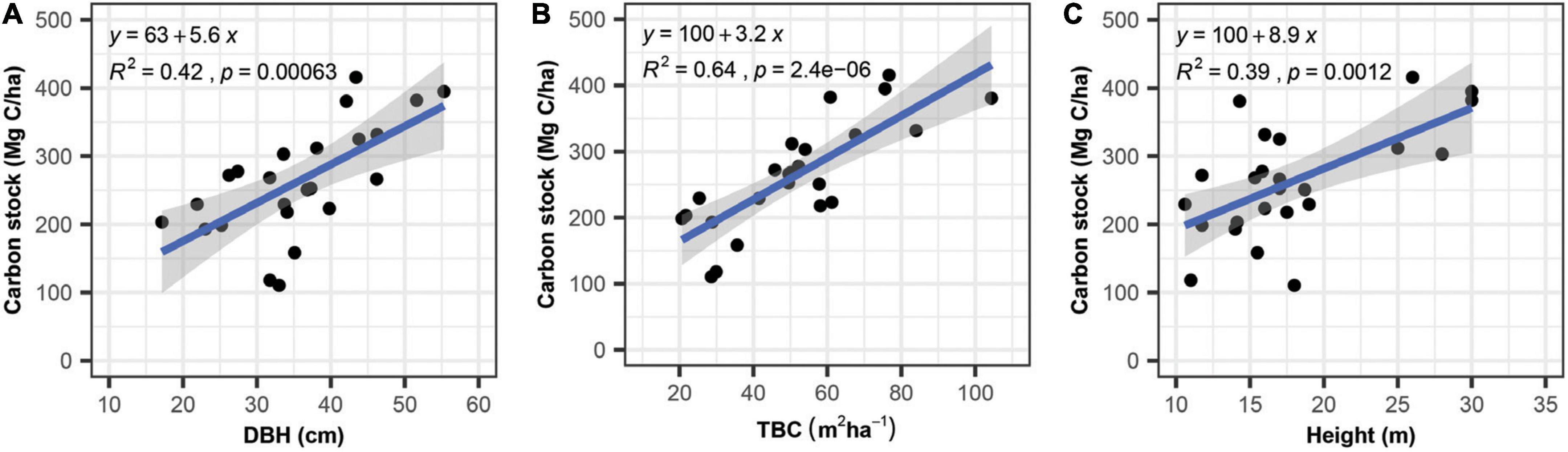
Figure 6. Bivariate relationships of carbon stock with diameter at breast height (A), total basal cover (B) and tree height (C).
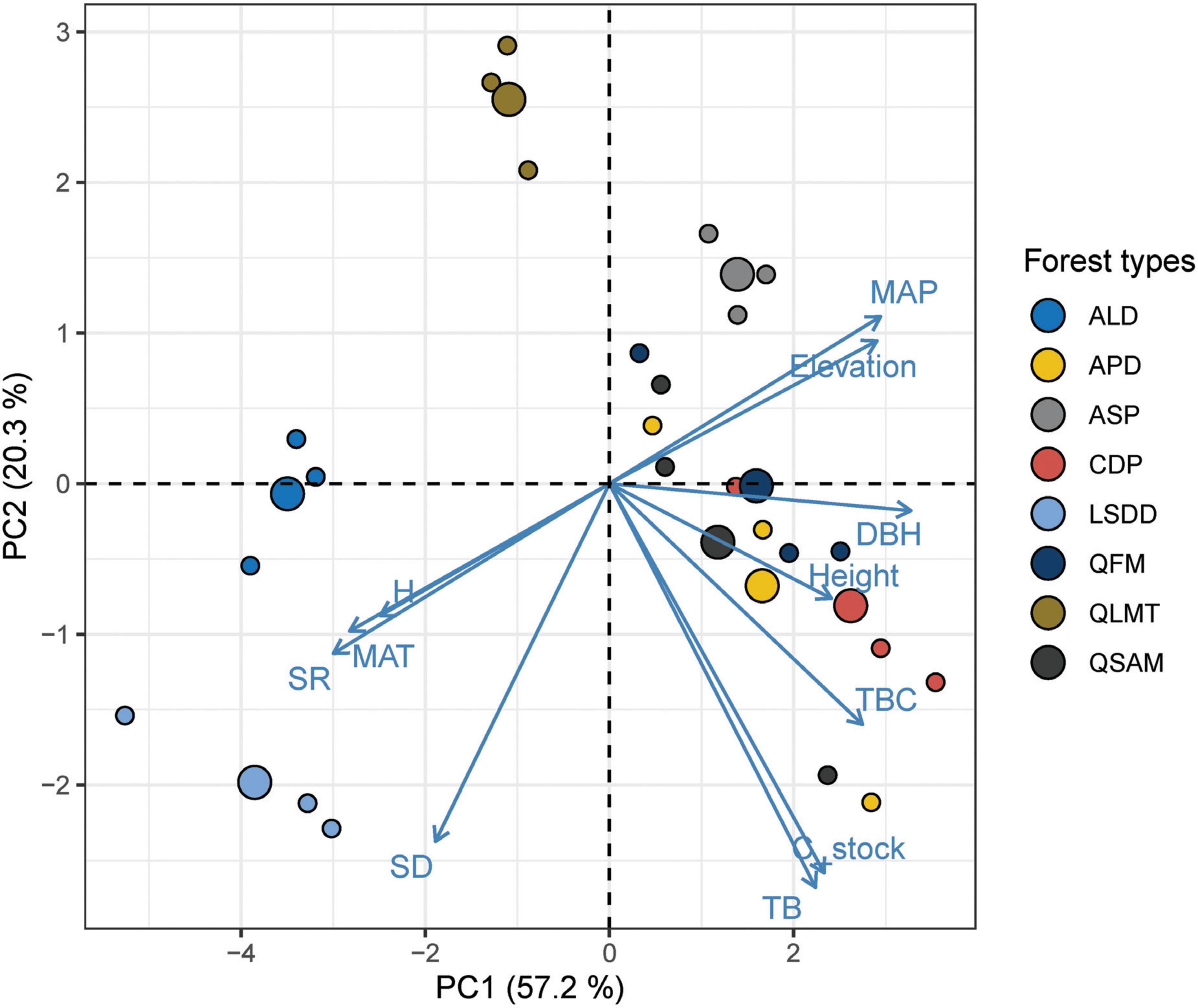
Figure 7. A principal component analysis between structural variables and forest types depicting a correlation biplot matrix diagram. The points represent forest types and the arrow indicates variables. SD, stem density; SR, species richness; H, Shannon index; MAT, mean annual temperature; DBH, diameter at breast height; MAP, total annual precipitation; TB, total biomass; TBC, total basal cover.
4 Discussion
The current study unravels the factors affecting biomass and C stock across the different forest types in the Western Himalayas. The biomass and C stock values ranged from 286.6 Mg/ha to 782.6 Mg/ha and 128.9 Mg C/ha to 360 Mg/C ha, respectively (Supplementary Table 2). The current observations are comparatively higher but within the range of previous studies in similar environments. Carbon stocks in the Indian Himalayas have been reported to vary between 59.20–245.31 Mg C/ha (Sharma et al., 2010), 107.8–234.1 Mg C/ha (Gairola et al., 2011), 85.22–234.32 Mg C/ha (Sharma et al., 2018), 22.7–236.8 Mg C/ha (Haq et al., 2022), 133.04–273.28 Mg C/ha (Dar and Parthasarathy, 2022) and 207.32–270.98 Mg C/ha (Tiwari et al., 2023). However, our results are comparatively on the lower side, contrary to the study of Kaushal and Baishya (2021), wherein they reported that the total biomass density and C stock varied (566.17–1280.79 and 258.22–577.77 Mg C/ha) in different forests. The reported range of biomass C stock at a global scale varied from 506–627 Mg C/ha in the USA (Smithwick et al., 2002), 58.9–386.5 Mg C/ha in NE China (Wei et al., 2013), and 12.96–856.50 Mg C/ha in Panama (Ruiz-Jaen and Potvin, 2011) across temperate and tropical forests, respectively. A recent study by Di Matteo et al. (2023) showed that the tree biomass (living + root) ranged from 546.7 to 695.1 Mg/ha in temperate old-growth forests of Italy. The variation in results is attributed to stand age, edaphic conditions, vegetation type, disturbance, and topography.
Trees provide a win -win strategy to mitigate global climate change as they regulate light availability, litter quantity and quality and ultimately govern C dynamics (Shirima et al., 2015). Carbon storage in forest ecosystems, especially in temperate forests where only one or few species are dominant, is mainly contained in large diameter, and old-age species. Furthermore, our results revealed that a more significant fraction of biomass and C stock is contributed by temperate species including Cedrus deodara, Abies pindrow, Quercus floribunda and Quercus semecarpifolia (Supplementary Figure 1), highlighting the fact that large trees contribute disproportionally to stand biomass compared to small trees (Poorter et al., 2015). In our study, the strong positive influence of DBH and TBC on C stock is in conformity with previous studies in tropical (Gebeyehu et al., 2019; Saimun et al., 2021) and temperate environments (Yuan et al., 2018; Bisht et al., 2022).
Another crucial structural attribute, stem density, showed no relationship with C stock. This probably due to the fact that a greater stem density may induce a crowding effect, due to which plant species compete for resource acquisition, ultimately causing reduced tree growth (Sullivan et al., 2017; Bhandari et al., 2021; Ulak et al., 2022). In terms of species diversity (species richness and Shannon diversity), we found no significant effect on C stock. The negligible role of species diversity on biomass C stock reflected the influential role of a few dominant species (Larsary et al., 2021). Generally, two hypotheses, niche complementarity and selection effect hypotheses (Tilman et al., 2001; Cardinale et al., 2009) explain how species diversity promotes biomass production. In diverse communities (tropical regions), plant species prefer niche partitioning for maximum utilization of resources and to facilitate each other, unlike communities where only a few dominant species are present. In our case, the lack of significant association of species diversity with biomass is probably due to the selection effect where dominant large tree species outline the other species. Previous studies (Paquette and Messier, 2011; Arasa-Gisbert et al., 2018) have observed that competitive exclusion, rather than species diversity, is the relevant explanation for high biomass and C stock in temperate forests growing under favorable conditions. Furthermore, the diversity-productivity hypothesis is reported to be scale-dependent. Generally, the positive influence of diversity on biomass/carbon is restricted to smaller sampling plots (0.1 ha), whereas negative or neutral relationships at a larger scale (0.25–1 ha) (Chisholm et al., 2013; Poorter et al., 2015; Fotis et al., 2018).
In our study, we expected elevation to be one of the critical elements in biomass and C stock formation. However, its effect was insignificant (r = 0.31; p = 0.13). Several reasons could be attributed to this: (1) our sampling plots are distantly related and span over different environmental conditions, so maybe that role of elevation is overcome by other factors, like forest types and species attributes; (2) we have analyzed our data across sites not within a single site, this might have caused sizeable environmental heterogeneity where the influence of elevation is negated. Previous studies in mountainous areas (Sharma et al., 2018; Kaushal and Baishya, 2021; Maza et al., 2022) have also observed similar trends. Although elevation didn’t play a role in biomass and C stock development, its significant effect can be followed on other forest attributes. For example, tree species diversity decreases with a rise in elevation, a trend previously observed in other studies in the Western and central Himalayas as well (Sharma et al., 2018; Kaushal and Baishya, 2021; Wani et al., 2022; Tiwari et al., 2023). This could be due to the harsh climatic conditions at higher elevations, which retard tree growth and development (Wieser et al., 2014; Wani et al., 2023).
In bivariate analysis, none of the climatic variables (precipitation and temperature) showed significant association with C stock. Precipitation is a crucial environmental factor that governs moisture availability for plant growth and development and indirectly drives biomass production (McCarthy and Enquist, 2007; Lie et al., 2018). The positive but insignificant role of precipitation on C stock could be explained by the fact that the selected sites receive abundant rainfall as a whole. Therefore, moisture may not be the limiting factor. On the other hand, temperature has a predominant role in biomass and carbon stock formation, whereas in montane and temperate forests, studies have shown that it doesn’t influence much (Selmants et al., 2014; Yue et al., 2018). Given that most of our sampling plots fall under a temperate environment, experiencing more or less the same temperature probably leads to homogeneity in temperature and ultimately negates its effect.
Overall, our finding revealed that structural variables (DBH, TBC, and tree height) override the role of abiotic (MAT, MAP, elevation) variables in biomass and C stock formation. Our results are in line with previous studies in the Himalayas (Sharma et al., 2010; Kaushal and Baishya, 2021; Dar and Parthasarathy, 2022) and (Poorter et al., 2017; Balima et al., 2021; Maza et al., 2022) elsewhere in the world. The PCA biplot also showed that biomass and C stock were positively associated with structural variables such as DBH, TBC and height. In contrast, stem density, diversity attributes and MAT were negatively correlated (Figure 7). Furthermore, the PCA biplot revealed that certain variables like elevation and MAP were positively associated with biomass and C stock despite insignificant effects in bivariate analysis.
Management activities at the community or regional levels are attributed to enhanced carbon storage (Adekunle et al., 2014; Solomon et al., 2017). In our study, we can say that, besides forest attributes, the management regime may be one of the contributing factors in biomass and C stock. Because each forest type is legally protected, there is less likelihood of external disturbance. As a result, it can be asserted that protected areas provide a conducive environment for the growth of plants and biodiversity conservation. Numerous studies have supported the significance of protected areas in shaping and maintaining the structure and functioning of ecosystems. For instance, a study by Keith et al. (2014) in the montane ash forests of southern Australia observed that the biomass carbon stock of logged forests was 55% lower than that of old-growth forests. The study of Dimobe et al. (2019) in W National Park in Burkina Faso, Western Africa, reported higher species richness (89 sp.) and carbon density (94.73 Mg/ha) compared to non-protected sites.
In another study, Måren and Sharma (2021) in the temperate forests of Nepal revealed that protected forests exhibited a more significant carbon stock (163.71 Mg C/ha) in comparison to unprotected forests (114 Mg C/ha). A separate investigation was carried out by Poudel et al. (2020), who conducted a study in the reserved oak forests of Nepal, whereby they determined that the carbon stock within this ecosystem exhibited a range of 52.8–194 Mg C/ha. Recently, a study conducted by Chaudhury et al. (2022) in North-east India found higher stand composition, biomass and C stock under protected forests compared to reserve forests and village forests, highlighting the fact that enhanced management practices in disturbed forests can lead to more significant CO2 sequestration and climate change mitigation.
Nevertheless, it is worth noting that anthropogenic disruption cannot be disregarded, even considering the legal status of the study sites. For example, illegal deforestation, fire, and agriculture expansion within and near protected sites may lead to forest loss (Collins and Mitchard, 2017). Apart from this, unregulated tourism is also a significant concern for protected sites. For example, in the present study, we observed that Chail and Churdhar WLS, are major tourist hotspots in north-western India. Hence, they incur a heavy tourist influx during the summer, posing a substantial burden on fragile ecosystems. Indeed, the investigated ecosystems have an appreciable amount of biomass C stock, but its long-term persistence requires integrated efforts of authorities and the local population.
5 Conclusion
The present study’s findings demonstrate that the selected sites in the Western Himalayas serve as a substantial repository of tree biomass and carbon stock. Temperate forests account for greater biomass and C stock than subtropical forests. We found that structural attributes govern the C stock in selected forest types mainly mean tree DBH, total basal cover (TBC), and tree height. However, the role of species diversity, elevation and climatic attributes in determining C stock was insignificant. Furthermore, in agreement with previous studies in the Himalayas, we found that only a few dominant species with large diameters account for the majority of the C stock of these forests. Hence, the cutting and felling of these species must be regulated for long-term ecosystem sustainability. Our findings highlight the role of protected sites in achieving carbon neutrality and the effective implementation of sustainable development goals (SDGs) strategies. At the same time, despite the legal status of these study sites, there is an urgent need to regulate permissible human activities such as tourism. Furthermore, the current findings may be useful to policymakers and stakeholders in developing management plans and climate change mitigation strategies for the Western Himalayas. Further studies are recommended to understand the detailed mechanism of factors involved in biomass and C storage in the Western Himalayas.
Data availability statement
The original contributions presented in the study are included in the article/Supplementary material, further inquiries can be directed to the corresponding author.
Author contributions
PK: Conceptualization, Data curation, Formal analysis, Funding acquisition, Investigation, Methodology, Project administration, Resources, Software, Validation, Visualization, Writing—original draft, Writing—review and editing. AK: Formal analysis, Funding acquisition, Investigation, Methodology, Validation, Visualization, Writing—original draft, Writing—review and editing. MP: Data curation, Formal analysis, Funding acquisition, Methodology, Validation, Visualization, Writing—review and editing. SH: Formal analysis, Funding acquisition, Investigation, Methodology, Validation, Visualization, Writing—review and editing. ANS: Conceptualization, Data curation, Formal analysis, Investigation, Methodology, Project administration, Resources, Supervision, Validation, Visualization, Writing—review and editing.
Funding
The author(s) declare financial support was received for the research, authorship, and/or publication of this article. The University Grants Commission (UGC), Government of India, New Delhi, was acknowledged for financial support in the form of Senior Research Fellowships to PK [443/(CSIR-UGC NET DEC. 2017)], AK [507/(OBC) (CSIR-UGC NET DEC. 2016)], and MP [492/(CSIR-UGC NET JUNE 2017)].
Acknowledgments
We are thankful to the Chairperson, Dept. of Botany, Panjab University, Chandigarh, for providing necessary research facilities. We would like to thank the Principal Chief Conservator of Forests (PCCF) of Haryana and H.P. for granting permission to visit forest areas. We acknowledge the support provided by Mr. N Ravi Sankar, Divisional Forest Officer, Wildlife (Shimla), and forest guards, Mr. Govind Verma and Mr. Rajinder Singh, for their invaluable support throughout the survey at Churdhar Wildlife Sanctuary. We would also like to thank Mr. Alok Sharma, Pravesh Bhardwaj, and Kamal Rana for their assistance during the field visits and reviewers for their critical appraisal of the manuscript, which helped improve the final draft.
Conflict of interest
The authors declare that the research was conducted in the absence of any commercial or financial relationships that could be construed as a potential conflict of interest.
Publisher’s note
All claims expressed in this article are solely those of the authors and do not necessarily represent those of their affiliated organizations, or those of the publisher, the editors and the reviewers. Any product that may be evaluated in this article, or claim that may be made by its manufacturer, is not guaranteed or endorsed by the publisher.
Supplementary material
The Supplementary Material for this article can be found online at: https://www.frontiersin.org/articles/10.3389/ffgc.2023.1328694/full#supplementary-material
Footnotes
References
Adekunle, V. A., Nair, N. K., Srivastava, A. K., and Singh, N. K. (2014). Volume yield, tree species diversity and carbon hoard in protected areas of two developing countries. Forest Sci. Technol. 10, 89–103.
Ahirwal, J., Nath, A., Brahma, B., Deb, S., Sahoo, U. K., and Nath, A. J. (2021). Patterns and driving factors of biomass carbon and soil organic carbon stock in the Indian Himalayan region. Sci. Total Environ. 770:145292. doi: 10.1016/j.scitotenv.2021.145292
Arasa-Gisbert, R., Vayreda, J., Román-Cuesta, R. M., Villela, S. A., Mayorga, R., and Retana, J. (2018). Forest diversity plays a key role in determining the stand carbon stocks of Mexican forests. For. Ecol. Manag. 415, 160–171. doi: 10.1016/j.foreco.2018.02.023
Balima, L. H., Kouamé, F. N. G., Bayen, P., Ganamé, M., Nacoulma, B. M. I., Thiombiano, A., et al. (2021). Influence of climate and forest attributes on aboveground carbon storage in Burkina Faso, West Africa. Environ. Chall. 4:100123. doi: 10.1016/j.envc.2021.100123
Banday, M., Bhardwaj, D. R., Pala, N. A., and Rajput, B. S. (2017). Quantitative analysis of woody vegetation in subtropical forests of Himachal Pradesh, India. Indian For. 143, 617–629.
Bhandari, S. K., Veneklaas, E. J., McCaw, L., Mazanec, R., Whitford, K., and Renton, M. (2021). Effect of thinning and fertilizer on growth and allometry of Eucalyptus marginata. For. Ecol. Manag. 479:118594. doi: 10.1016/j.foreco.2020.118594
Bhardwaj, D. R., Tahiry, H., Sharma, P., Pala, N. A., Kumar, D., and Kumar, A. (2021). Influence of aspect and elevational gradient on vegetation pattern, tree characteristics and ecosystem carbon density in North-western Himalayas. Land 10:1109. doi: 10.3390/land10111109
Bisht, S., Bargali, S. S., Bargali, K., Rawat, G. S., Rawat, Y. S., and Fartyal, A. (2022). Influence of anthropogenic activities on forest carbon stocks—a case study from Gori Valley, Western Himalaya. Sustainability 14:16918. doi: 10.3390/su142416918
Cairns, M. A., Brown, S., Helmer, E. H., and Baumgardner, G. A. (1997). Root biomass allocation in the world’s upland forests. Oecologia 111, 1–11. doi: 10.1007/s004420050201
Cardinale, B. J., Bennett, D. M., Nelson, C. E., and Gross, K. (2009). Does productivity drive diversity or vice versa? A test of the multivariate productivity–diversity hypothesis in streams. Ecology 90, 1227–1241. doi: 10.1890/08-1038.1
Case, M. J., Johnson, B. G., Bartowitz, K. J., and Hudiburg, T. W. (2021). Forests of the future: Climate change impacts and implications for carbon storage in the Pacific Northwest, USA. For. Ecol. Manag. 482:118886. doi: 10.1016/j.foreco.2020.118886
Champion, H. G., and Seth, S. K. (1968). A revised survey of the forest types of India. Delhi: Government of India.
Chaudhury, G., Darji, C. B., Basumatari, M., Dutta, G., Devi, A., and Bharadwaj, N. (2022). Stand structure, biomass and carbon stock along disturbance gradients in differently managed tropical forests of Assam, northeast India. Trees For. People 9:100296. doi: 10.1016/j.tfp.2022.100296
Cheng, Z., Aakala, T., and Larjavaara, M. (2023). Elevation, aspect, and slope influence woody vegetation structure and composition but not species richness in a human-influenced landscape in north-western Yunnan, China. Front. For. Glob. Change 6:1187724. doi: 10.3389/ffgc.2023.1187724
Chisanga, K., Bhardwaj, D. R., Pala, N. A., and Thakur, C. L. (2018). Biomass production and carbon stock inventory of high-altitude dry temperate land use systems in North Western Himalaya. Ecol Process 7:22. doi: 10.1186/s13717-018-0134-8
Chisholm, R. A., Muller-Landau, H. C., Rahman, K. A., Daniel, P., Bebber, D. P., Bin, Y., et al. (2013). Scale-dependent relationships between tree species richness and ecosystem function in forests. J. Ecol. 101, 1214–1224. doi: 10.1111/1365-2745.12132
Choudhary, R. K., and Lee, J. (2012). A floristic reconnaissance of Churdhar wildlife sanctuary of Himachal Pradesh, India. Manthan 13, 2–12.
Chowdhery, H. J., and Wadhwa, B. M. (1984). Flora of Himachal Pradesh. Calcutta: Botanical Survey of India.
Collins, M. B., and Mitchard, E. T. (2017). A small subset of protected areas are a highly significant source of carbon emissions. Sci. Rep. 7:41902. doi: 10.1038/srep41902
Dar, A. A., and Parthasarathy, N. (2022). Patterns and drivers of tree carbon stocks in Kashmir Himalayan forests: implications for climate change mitigation. Ecol. Process. 11, 1–13. doi: 10.1186/s13717-022-00402-z
Dar, J. A., Rather, M. Y., Subashree, K., Sundarapandian, S., and Khan, M. L. (2017). Distribution patterns of tree, understorey, and detritus biomass in coniferous and broad-leaved forests of Western Himalaya, India. J. Sustain. For. 36, 787–805. doi: 10.1080/10549811.2017.1363055
Dar, J. A., and Sundarapandian, S. (2015). Variation of biomass and carbon pools with forest type in temperate forests of Kashmir Himalaya, India. Environ. Monit. Assess. 187:55. doi: 10.1007/s10661-015-4299-7
Di Matteo, G., Luzzi, G., Basile, A., Sposato, A., Bertini, G., Neri, U., et al. (2023). Carbon concentrations and carbon storage capacity of three old-growth forests in the Sila National Park, Southern Italy. J. For. Res. 34, 233–242. doi: 10.1007/s11676-022-01549-3
Dimobe, K., Kuyah, S., Dabré, Z., Ouédraogo, A., and Thiombiano, A. (2019). Diversity-carbon stock relationship across vegetation types in W National Park in Burkina Faso. Forest Ecol. Manag. 438, 243–254. doi: 10.1016/j.foreco.2019.02.027
Duncanson, L., Liang, M., Leitold, V., Armston, J., Krishna Moorthy, S. M., Dubayah, R., et al. (2023). The effectiveness of global protected areas for climate change mitigation. Nat. Commun. 14:2908. doi: 10.1038/s41467-023-38073-9
FAO (2022). The State of the World’s Forests 2022. Forest pathways for green recovery and building inclusive, resilient and sustainable economies. Rome: Food and Agriculture Organization, doi: 10.4060/cb9360en
Fotis, A. T., Murphy, S. J., Ricart, R. D., Krishnadas, M., Whitacre, J., Wenzel, J. W., et al. (2018). Aboveground biomass is driven by mass-ratio effects and stand structural attributes in a temperate deciduous forest. J. Ecol. 106, 561–570. doi: 10.1111/1365-2745.12847
FSI (1996). Volume Equation for the Forests of India, Nepal, and Bhutan. Dehradun: Forest Survey of India.
Gairola, S., Sharma, C. M., Ghildiyal, S. K., and Suyal, S. (2011). Live tree biomass and carbon variation along an altitudinal gradient in moist temperate valley slopes of the Garhwal Himalaya (India). Curr. Sci. 100, 1862–1870.
Gebeyehu, G., Soromessa, T., Bekele, T., and Teketay, D. (2019). Carbon stocks and factors affecting their storage in dry Afromontane forests of Awi Zone, north-western Ethiopia. J. Ecol. Environ. 43, 1–18. doi: 10.1186/s41610-019-0105-8
Gibbs, H. K., Brown, S., Niles, J. O., and Foley, J. A. (2007). Monitoring and estimating tropical forest carbon stocks: making REDD a reality. Environ. Res. Lett. 2:45023. doi: 10.1088/1748-9326/2/4/045023
Gogoi, A., Ahirwal, J., and Sahoo, U. K. (2022). Evaluation of ecosystem carbon storage in major forest types of Eastern Himalaya: Implications for carbon sink management. J. Env. Manage. 302:113972. doi: 10.1016/j.jenvman.2021.113972
Graves, S., Piepho, H., and Luciano, S. (2023). multcompView: Visualizations of Paired Comparisons. R package version 0.1-9. Vienna: R Core Team.
Haq, S. M., Rashid, I., Calixto, E. S., Ali, A., Kumar, M., Srivastava, G., et al. (2022). Unravelling patterns of forest carbon stock along a wide elevational gradient in the Himalaya: Implications for climate change mitigation. For. Ecol. Manag. 521:120442. doi: 10.1016/j.foreco.2022.120442
Huynh, T., Lee, D. J., Lewis, T., and Applegate, G. (2023). Effects of site characteristics and stand management on biomass and carbon sequestration in spotted gum (Corymbia citriodora subsp. variegata) plantations. For. Ecol. Manag. 529:120725. doi: 10.1016/j.foreco.2022.120725
IPCC (2018). Global warming of 1.5°C. Available online at: https://www.ipcc.ch/sr15/ (accessed August 11, 2023).
Islam, M., Deb, G. P., and Rahman, M. (2017). Forest fragmentation reduced carbon storage in a moist tropical forest in Bangladesh: Implications for policy development. Land Use Policy 65, 15–25. doi: 10.1016/j.landusepol.2017.03.025
Jerzy, S., and Anna, G. (2007). Aboveground standing biomass and tree species diversity in natural stands of Central Europe. J. Veg. Sci 18, 563–570. doi: 10.1111/j.1654-1103.2007.tb02569.x
Jolliffe, I. T., and Cadima, J. (2016). Principal component analysis: a review and recent developments. Phil. Trans. R. Soc. A 374:20150202. doi: 10.1098/rsta.2015.0202
Karger, D. N., Conrad, O., Böhner, J., Kawohl, T., Kreft, H., Soria-Auza, R. W., et al. (2017). Climatologies at high resolution for the earth’s land surface areas. Sci. Data 4:170122. doi: 10.1038/sdata.2017.122
Kassambara, A., and Mundt, F. (2020). factoextra: Extract and Visualize the Results of Multivariate Data Analyses_. R package version 1.0.7. Vienna: R Core Team.
Kaushal, S., and Baishya, R. (2021). Stand structure and species diversity regulate biomass carbon stock under major Central Himalayan Forest types of India. Ecol. Process. 10, 1–18. doi: 10.1186/s13717-021-00283-8
Keith, H., Lindenmayer, D., MacKey, B., Blair, D., Carter, L., McBurney, L., et al. (2014). Managing temperate forests for carbon storage: Impacts of logging versus forest protection on carbon stocks. Ecosphere 5, 1–34. doi: 10.1890/ES14-00051.1
Khanalizadeh, A., Eshaghi Rad, J., Amiri, G. Z., Zare, H., Schall, P., and Lexer, M. J. (2023). The relationship between plant diversity and aboveground biomass in managed and unmanaged temperate forests. Eur. J. Forest Res. 142, 1167–1175. doi: 10.1007/s10342-023-01583-8
Kindermann, G., McCallum, I., Fritz, S., and Obersteiner, M. (2008). A global forest growing stock, biomass and carbon map based on FAO statistics. Silva Fenn. 42, 387–396. doi: 10.14214/sf.244
Kothandaraman, S., Dar, J. A., Sundarapandian, S., Dayanandan, S., and Khan, M. L. (2020). Ecosystem-level carbon storage and its links to diversity, structural and environmental drivers in tropical forests of Western Ghats, India. Sci. Rep. 10:13444. doi: 10.1038/s41598-020-70313-6
Kumari, B., Tiwari, A., and Anjum, J. (2022). Carbon sequestration potential in natural forests of Himachal Pradesh, India. Curr. Sci. 122:846.
Larsary, M. K., Pourbabaei, H., Sanaei, A., Salehi, A., Yousefpour, R., and Ali, A. (2021). Tree-size dimension inequality shapes aboveground carbon stock across temperate forest strata along environmental gradients. For. Ecol. Manag. 496:119482. doi: 10.1016/j.foreco.2021.119482
Le Quéré, C., Andrew, R. M., Friedlingstein, P., Sitch, S., Hauck, J., Pongratz, J., et al. (2018). Global Carbon Budget 2018. Earth Syst. Sci. Data 10, 2141–2194. doi: 10.5194/essd-10-2141-2018
Lie, Z., Xue, L., and Jacobs, D. F. (2018). Allocation of forest biomass across broad precipitation gradients in China’s forests. Sci. Rep. 8:10536.
Lung, M., and Espira, A. (2015). The influence of stand variables and human use on biomass and carbon stocks of a transitional African forest: Implications for forest carbon projects. For. Ecol. Manag. 351, 36–46. doi: 10.1016/j.foreco.2015.04.032
Lutz, J. A., Furniss, T. J., Johnson, D. J., Davies, S. J., Allen, D., Alonso, A., et al. (2018). Global importance of large-diameter trees. Glob. Ecol. Biogeogr. 27, 849–864. doi: 10.1111/geb.12747
Malhi, Y., Baldocchi, D. D., and Jarvis, P. G. (1999). The carbon balance of tropical, temperate and boreal forests. Plant Cell Environ. 22, 715–740. doi: 10.1046/j.1365-3040.1999.00453.x
Måren, I. E., and Sharma, L. N. (2021). Seeing the wood for the trees: Carbon storage and conservation in temperate forests of the Himalayas. For Ecol. Manag. 487:119010. doi: 10.1016/j.foreco.2021.119010
Margalef, D. R. (1958). Information theory in ecology, General systems. Real Acad. Cienc. Barcel. 32, 373–449.
Maza, B., Rodes-Blanco, M., and Rojas, E. (2022). Aboveground biomass along an elevation gradient in an evergreen Andean–Amazonian forest in Ecuador. Front. For. Glob. Chang. 5:738585. doi: 10.3389/ffgc.2022.738585
McCarthy, M. C., and Enquist, B. J. (2007). Consistency between an allometric approach and optimal partitioning theory in global patterns of plant biomass allocation. Funct. Ecol. 21, 713–720. doi: 10.111/j.1365-2435.2007.01276.x
Mensah, S., Noulekoun, F., and Ago, E. E. (2020). Aboveground tree carbon stocks in West African semi-arid ecosystems: Dominance patterns, size class allocation and structural drivers. Glob. Ecol. Conserv. 24, e01331. doi: 10.1016/j.gecco.2020.e01331
Mensah, S., Noulèkoun, F., Dimobe, K., Seifert, T., and Glèlè Kakaï, R. (2023). Climate and soil effects on tree species diversity and aboveground carbon patterns in semi-arid tree savannas. Sci. Rep. 13:11509. doi: 10.1038/s41598-023-38225-3
Mensah, S., Veldtman, R., Assogbadjo, A. E., GlèlèKakaï, R., and Seifert, T. (2016). Tree species diversity promotes aboveground carbon storage through functional diversity and functional dominance. Ecol. Evol. 6, 7546–7557. doi: 10.1002/ece3.2525
Moles, A. T., Warton, D. I., Warman, L., Swenson, N. G., Laffan, S. W., Zanne, A. E., et al. (2009). Global patterns in plant height. J. Ecol. doi: 10.1111/j.1365-2745.2009.01526.x [Epub ahead of print].
Nagar, D. K. (2012). Biomass and carbon density in relation to altitude in moist temperate western Himalayan ecosystem. Master’s thesis. Solan: Dr YS Parmar University of Horticulture and Forestry.
Negi, J. D. S., Manhas, R. K., and Chauhan, P. S. (2003). Carbon allocation in different components of some tree species of India: a new approach for carbon estimation. Curr. Sci. 85, 1528–1531.
Negi, V. S., Pathak, R., Rawal, R. S., Bhatt, I. D., and Sharma, S. (2019). Long-term ecological monitoring on forest ecosystems in Indian Himalayan Region: criteria and indicator approach. Ecol. Indic. 102, 374–381. doi: 10.1016/j.ecolind.2019.02.035
Pan, Y., Birdsey, R., Fang, J., Houghton, R., Kauppi, P., Kurz, W., et al. (2011). A large and persistent carbon sink in the world’s forests. Science 333, 988–993. doi: 10.1126/science.1201609
Pan, Y., Birdsey, R. A., Phillips, O. L., and Jackson, R. B. (2013). The structure, distribution, and biomass of the world’s forests. Annu. Rev. Ecol. Evol. Syst. 44, 593–622. doi: 10.1146/annurev-ecolsys-110512-135914
Paquette, A., and Messier, C. (2011). The effect of biodiversity on tree productivity: From temperate to boreal forests. Glob. Ecol. Biogeogr. doi: 10.1111/j.1466-8238.2010.00592.x [Epub ahead of print].
Pinto, E., Cuesta, F., Bernardi, A., Llerena-Zambrano, M., Pérez, A. J., van der Sande, M. T., et al. (2023). Determinants of aboveground carbon stocks and productivity in secondary forests along a 3000-m elevation gradient in the Ecuadorian Andes. Plant Ecol. Divers. doi: 10.1080/17550874.2023.2274844 [Epub ahead of print].
Poorter, L., van der Sande, M. T., Arets, E. J. M. M., Ascarrunz, N., Enquist, B., Finegan, B., et al. (2017). Biodiversity and climate determine the functioning of Neotropical forests. Global Ecol. Biogeogr. 26, 1423–1434. doi: 10.1111/geb.12668
Poorter, L., vander Sande, M. J., Thompson, J., Aretes, E. J. M. M., Alarcon, A., Alvarez Sanchez, J., et al. (2015). Diversity enhances carbon storage in tropical forests. Global Ecol. Biogeogr. 24, 1314–1328. doi: 10.1111/geb.12364
Poudel, A., Sasaki, N., and Abe, I. (2020). Assessment of carbon stocks in oak forests along the altitudinal gradient: A case study in the Panchase Conservation Area in Nepal. Glob. Ecol. Conserv. 23, e01171. doi: 10.1016/j.gecco.2020.e01171
Pregitzer, K. S., and Euskirchen, E. S. (2004). Carbon cycling and storage in world forests, biome patterns related to forest age. Glob. Chang. Biol. 10, 2052–2077. doi: 10.1111/j.1365-2486.2004.00866.x
R Core Team (2023). R: A language and environment for statistical computing (Version 4.3.0). Vienna: R Foundation for Statistical Computing.
Ruiz-Jaen, M. C., and Potvin, C. (2011). Can we predict carbon stocks in tropical ecosystems from tree diversity? Comparing species and functional diversity in a plantation and a natural forest. New Phytol. 189, 978–987. doi: 10.1111/j.1469-8137.2010.03501.x
Sahoo, U. K., Tripathi, O. P., Nath, A. J., Deb, S., Das, D. J., Gupta, A., et al. (2021). Quantifying tree diversity, carbon stocks, and sequestration potential for diverse land uses in Northeast India. Front. Environ. Sci. 9:724950. doi: 10.3389/fenvs.2021.724950
Saimun, M. S. R., Karim, M. R., Sultana, F., and Arfin-Khan, M. A. (2021). Multiple drivers of tree and soil carbon stock in the tropical forest ecosystems of Bangladesh. Trees For. People 5:100108. doi: 10.1016/j.tfp.2021.100108
Sanaei, A., Ali, A., and Chahouki, M. A. Z. (2018). The positive relationships between plant coverage, species richness, and aboveground biomass are ubiquitous across plant growth forms in semi-steppe rangelands. J. Environ. Manage. 205, 308–318. doi: 10.1016/j.jenvman.2017.09.079
Selmants, P. C., Litton, C. M., Giardina, C. P., and Asner, G. P. (2014). Ecosystem carbon storage does not vary with mean annual temperature in Hawaiian tropical montane wet forests. Glob. Change Biol 20, 2927–2937. doi: 10.1111/gcb.12636
Shannon, C. E., and Weaver, W. (1949). The mathematical theory of communication. Urbana, IL: University of Illinois Press.
Sharma, C. M., Baduni, N. P., and Gairola, S. (2010). Tree diversity and carbon stocks of some major forest types of Garhwal Himalaya, India. For. Ecol. Manag. 260, 2170–2179. doi: 10.1016/j.foreco.2010.09.014
Sharma, C. M., Mishra, A. K., Krishan, R., Tiwari, O. P., and Rana, Y. S. (2016). Variation in vegetation composition, biomass production, and carbon storage in ridge top forests of high mountains of Garhwal Himalaya. J. Sustain. For. 35, 119–132. doi: 10.1080/10549811.2015.1118387
Sharma, C. M., Tiwari, O. P., Rana, Y. S., Krishan, R., and Mishra, A. K. (2018). Elevational behavior on dominance–diversity, regeneration, biomass, and carbon storage in ridge forests of Garhwal Himalaya, India. For. Ecol. Manag. 424, 105–120. doi: 10.1016/j.foreco.2018.04.038
Shirima, D. D., Totland, O., Munishi, P. K., and Moe, S. R. (2015). Relationships between tree species richness, evenness and aboveground carbon storage in montane forests and miombo woodlands of Tanzania. Basic Appl. Ecol. 16, 239–249. doi: 10.1016/j.baae.2014.11.008
Singh, S. (2018). Understanding the role of slope aspect in shaping the vegetation attributes and soil properties in Montane ecosystems. Trop. Ecol. 59, 417–430.
Singh, S., and Verma, A. K. (2018). Biomass and carbon stocks in different forest types of Western Himalaya. Trop. Ecol. 59, 647–658.
Smith, D. M., Scaife, A. A., Hawkins, E., Bilbao, R., Boer, G. J., Caian, M., et al. (2018). Predicted chance that global warming will temporarily exceed 1.5 C. Geophys. Res. Lett. 45, 11–895. doi: 10.1029/2018GL079362
Smithwick, E. A., Harmon, M. E., Remillard, S. M., Acker, S. A., and Franklin, J. F. (2002). Potential upper bounds of carbon stores in forests of the Pacific Northwest. Ecol. Appl 12, 1303–1317.
Solomon, N., Birhane, E., Tadesse, T., Treydte, A. C., and Meles, K. (2017). Carbon stocks and sequestration potential of dry forests under community management in Tigray, Ethiopia. Ecol Process 6:20. doi: 10.1186/s13717-017-0088-2
Stegen, J. C., Swenson, N. G., Enquist, B. J., White, E. P., Phillips, O. L., Jørgensen, P. M., et al. (2011). Variation in aboveground forest biomass across broad climatic gradients. Glob. Ecol. Biogeogr. 20, 744–754. doi: 10.1111/j.1466-8238.2010.00645.x
Subramani, S. P., Kapoor, K. S., and Goraya, G. S. (2014). Additions to the floral wealth of Sirmaur district, Himachal Pradesh from Churdhar wildlife sanctuary. J. Threat. Taxa 6, 6427–6452. doi: 10.11609/jott.o2845.6427-52
Sullivan, M. J., Talbot, J., Lewis, S. L., Phillips, O. L., Qie, L., Begne, S. K., et al. (2017). Diversity and carbon storage across the tropical forest biome. Sci. Rep. 7:39102. doi: 10.1038/srep39102
Tilman, D., Reich, P. B., Knops, J., Wedin, D., Mielke, T., and Lehman, C. (2001). Diversity and productivity in a long-term grassland experiment. Science 294, 843–845.
Tiwari, A., Khanduri, V. P., Rawat, D., Singh, B., Riyal, M. K., Mishra, G., et al. (2023). Vegetation composition, soil properties, and carbon stock of montane forests along a disturbance in the Garhwal Himalaya, India. Front. For. Glob. Change 6:1193792. doi: 10.3389/ffgc.2023.1193792
Tolangay, D., and Moktan, S. (2020). Trend of studies on carbon sequestration dynamics in the Himalaya hotspot region: A review. J. Appl. Nat. Sci. 12, 647–660.
Ulak, S., Ghimire, K., Gautam, R., Bhandari, S. K., Poudel, K. P., Timilsina, Y. P., et al. (2022). Predicting the upper stem diameters and volume of a tropical dominant tree species. J For Res 33, 1725–1737. doi: 10.1007/s11676-022-01458-5
UNFCCC (2015). Adoption of the Paris Agreement. Available online at: https://unfccc.int/process-and-meetings/the-paris-agreement (accessed September 10, 2023).
Wani, Z. A., Khan, S., Bhat, J. A., Malik, A. H., Alyas, T., Pant, S., et al. (2022). Pattern of β-diversity and plant species richness along vertical gradient in Northwest Himalaya, India. Biology 11:1064. doi: 10.3390/biology11071064
Wani, Z. A., Negi, V. S., Bhat, J. A., Satish, K. V., Kumar, A., Khan, S., et al. (2023). Elevation, aspect, and habitat heterogeneity determine plant diversity and compositional patterns in the Kashmir Himalaya. Front. For. Glob. Change 6:1019277. doi: 10.3389/ffgc.2023.1019277
Wei, T., and Simko, V. (2021). R package ‘corrplot’: Visualization of a Correlation Matrix (Version 0.92). Available online at: https://github.com/taiyun/corrplot (accessed September 18, 2023).
Wei, Y., Li, M., Chen, H., Lewis, B. J., Yu, D., Zhou, L., et al. (2013). Variation in carbon storage and its distribution by stand age and forest type in boreal and temperate forests in northeastern China. PLoS One 8:e72201. doi: 10.1371/journal.pone.0072201
Wieser, G., Holtmeier, F. K., and Smith, W. K. (2014). “Treelines in a changing global environment,” in Trees in a Changing Environment. Plant Ecophysiology, eds M. Tausz and N. Grulke (Dordrecht: Springer), doi: 10.1007/978-94-017-9100-7_10
Xu, X., Zhang, H., Zhang, D., Tian, W., Huang, H., and Ma, A. (2017). Altitudinal patterns of plant species richness in the Honghe region of China. Pak. J. Bot. 49, 1039–1048.
Yuan, Z., Wang, S., Ali, A., Gazol, A., Ruiz-Benito, P., Wang, X., et al. (2018). Aboveground carbon storage is driven by functional trait composition and stand structural attributes rather than biodiversity in temperate mixed forests recovering from disturbances. Ann. For. Sci. 75:67. doi: 10.1007/s13595-018-0745-3
Keywords: carbon stock, climate change, Western Himalayas, forest types, structural attributes
Citation: Kumar P, Kumar A, Patil M, Hussain S and Singh AN (2024) Factors influencing tree biomass and carbon stock in the Western Himalayas, India. Front. For. Glob. Change 6:1328694. doi: 10.3389/ffgc.2023.1328694
Received: 27 October 2023; Accepted: 18 December 2023;
Published: 09 January 2024.
Edited by:
Gopal Shukla, Uttar Banga Krishi Viswavidyalaya, IndiaReviewed by:
Prashant Sharma, Dr. Yashwant Singh Parmar University of Horticulture and Forestry, IndiaBhupendra Singh, VCSG Uttarakhand University, India
Copyright © 2024 Kumar, Kumar, Patil, Hussain and Singh. This is an open-access article distributed under the terms of the Creative Commons Attribution License (CC BY). The use, distribution or reproduction in other forums is permitted, provided the original author(s) and the copyright owner(s) are credited and that the original publication in this journal is cited, in accordance with accepted academic practice. No use, distribution or reproduction is permitted which does not comply with these terms.
*Correspondence: Anand Narain Singh, dranand1212@gmail.com; ansingh@pu.ac.in