Nitrogen addition enhances nitrogen but not carbon mineralization in aggregate size fractions of soils in a Pinus massonia plantation
- 1Key Laboratory of Forest Ecology and Environment of National Forestry and Grassland Administration, Ecology and Nature Conservation Institute, Chinese Academy of Forestry, Beijing, China
- 2Co-Innovation Center for Sustainable Forestry in Southern China, Nanjing Forestry University, Nanjing, China
Introduction: Atmospheric nitrogen (N) deposition can impact the levels of soil organic carbon (SOC) and total nitrogen (total N) by altering the soil N availability. However, the effect of N input on the mineralization of SOC and total N in various soil aggregate size fractions requires further clarification.
Methods: The soil samples were collected from a Pinus massoniana plantation situated in the Three Gorges Reservoir Area of China. Over a period of three years, the soils from the plantation were subjected to four different levels of nitrogen addition (0 [N0], 30 [N30], 60 [N60], and 90 [N90] kg N ha−1 yr−1). The impact of N addition on the mineralization of SOC and total N in aggregates was evaluated through an incubation experiment, encompassing four aggregate sizes (2000 − 8000, 1000 − 2000, 250 − 1000, and < 250 μm).
Results: The < 250 μm fraction showed the highest levels of cumulative C mineralization, while the lowest levels were observed in the 2000 − 8000 μm fraction. Compared to the < 250 um fraction, a drop of 9 − 21% in cumulative C mineralization was observed in the 2000 − 8000 μm fraction, indicating that soil aggregates enhance the stability of C in the soil. Cumulative N mineralization levels were consistently at their lowest in the 2000 − 8000 μm fraction, indicating aggregates reducing mineralization-related N loss. Adding N to forest soil samples led to a reduction in cumulative C mineralization. In contrast, an opposite trend was observed in the cumulative N mineralization after adding N in microaggregates. Nitrification was the main contributor to net N mineralization. SOC and total levels increased in response to N30 and N60. N addition leads to an increase in the weight ratio of the 1000 − 2000 μm fraction. Moreover, N90 was linked to decreases in microbial biomass C and N.
Discussion: These findings confirm that the structural characteristics of soil aggregates play a crucial role in sequestering organic carbon and total N sequestration in the presence of N deposition, while highlighting N loss from the soil caused by N input.
1 Introduction
In subtropical forest ecosystems, stability and primary productivity of the ecosystem are constrained by the effectiveness of nitrogen (N, Du et al., 2021), Over the past century, there has been an approximately tenfold increase in N input into these ecosystems by atmospheric deposition, significant augmenting N level in the soil (Galloway et al., 2004; Peñuelas et al., 2012). The process of N mineralization plays a crucial role in breaking down soil organic N into inorganic N, thereby critically regulating the N balance within forest ecosystems (Kaarakka et al., 2016). Atmospheric N deposition can modify soil organic N mineralization rates through its effect on inorganic N stocks (Baldos et al., 2015), and it can also impact overall N mineralization by impacting stoichiometric ratios (Gao et al., 2020). Studies have shown that artificial external N deposition leads to an initial increase in net N mineralization, but this effect diminishes over time (Baldos et al., 2015). While, some studies have found no impact of N deposition on forest soil N mineralization (Blaško et al., 2013; Chen et al., 2024). In the same time, other reports indicate that N addition may actually inhibit soil N mineralization (Gao et al., 2016). This is related to the amount of N deposition and ecosystem type. Therefore, considering local soil conditions and variations in N deposition levels is crucial when forecasting the impact of regional N deposition on forest soil N dynamics.
Terrestrial soil serves as the primary source and sink of carbon (C) pool on Earth, representing approximately 5–15% of total global annual C emissions sourced from soil C pool (Post et al., 1982; Lal, 2004). Consequently, even slight fluctuations in this soil C pool can exert a significant influence on the global C balance, thereby contributing to climate change on a worldwide scale (Ahirwal et al., 2022). Elevated levels of N input have the potential to impact the stability of soil organic C (SOC), in terrestrial ecosystems due to the interconnection between N and C (Mehnaz et al., 2018; Jing et al., 2021). Forest soils sustain a diverse array of ongoing biochemical and physiological processes (Bossio et al., 2020), and even minor fluctuations in C sinks within forest soil can have significant repercussions at the soil C pool level (Gao et al., 2020). Similar to N mineralization, C mineralization is a crucial process within the global C cycle (Wang and Zhong, 2016), highlighting the necessity for additional research into the impacts of atmospheric N deposition on soil C pool dynamics. Consequently, an increasing number of recent studies have investigated the correlation between soil N balance and C mineralization. Generally, it is believed that the rate of SOC mineralization decreases with higher N input (Wang et al., 2014), although these findings are not universally applicable. Some studies have indicated no significant relationship between N deposition and SOC mineralization (Prescott, 1995), while others have suggested a positive correlation between these processes (Huang et al., 2011). The underlying mechanisms for these observations require further clarification.
The physical structure of soil aggregates is a primary determinant of soil organic carbon (SOC) stability (García-Oliva et al., 2004; Tobiašová et al., 2016). It is conventionally believed that aggregates possess varying levels of porosity, which can influence the microbial population in the soil (Carolin et al., 2016; Wei et al., 2020). Consequently, this results in different rates of C and N mineralization due to the particle sizes of these aggregates. For example, microaggregates exhibit significantly lower rates of organic matter mineralization compared to macroaggregates due to reduced adsorption of organic matter particles and limited contact of microorganisms and extracellular enzymes with organic matter, caused by spatial constraints and a smaller specific surface area (Carolin et al., 2016). However, some studies on natural variations in 13C abundance and organic matter turnover time have indicated that the average turnover time for organic matter in aggregates with particle sizes >250 μm is approximately 15–50 years, while in aggregates with particles <250 μm, the turnover time is approximately 100–300 years (Besnard et al., 1996; John et al., 2005). The dense coordination bonds and interaction bridges in the microaggregates fraction that contribute to enhancing the stability of the organic matter within these aggregates (Cai et al., 2016). Consequently, the organic matter of microaggregates is classified as inert or neutral, whereas that in macroaggregates is considered reactive due to weaker bonding (Jha et al., 2012; Cao et al., 2021). Additionally, it has been suggested that fresh organic matter will enter the macroaggregates first and will also be mineralized first (Bucka et al., 2019). Despite these findings, the overall relationship between aggregate physical structure and C and N mineralization remains uncertain. Moreover, N addition exerts a significant impact on the mineralization of organic matter within soil aggregates; however, there is no uniform consensus in this area. For instance, Zhang and Shangguan (2022) demonstrated that N addition elevated the organic C levels and C mineralization rate in small marcoaggregates, while no such effect was observed in microaggregates. Similarly, Liao et al. (2021) observed that N addition increased the rate of N mineralization in macroaggregates, but did not cause any significant change in microaggregates. Conversely, Tao and Song (2014) pointed out that nitrogen deposition notably inhibited carbon mineralization in macroaggregates, but enhanced it in microaggregates. Furthermore, Li et al. (2020) reported that the rise in nitrogen mineralization due to nitrogen addition was mainly attributed to microaggregates. The addition of N increases the uncertainty faced by C and N mineralization in aggregates.
The area around the Three Gorges Reservoir area covers a 663 km long stretch between Hubei province and Chongqing municipality, experiencing high N deposition levels in China and is typical of more fragile ecological stability. Hence, there is an urgent need for analysis of its response to the local ecosystems to increasing N deposition. Pinus massoniana (P. massoniana) is a significant pioneer species in this region to be applied to ecological restoration. Therefore, this study aimed to assess the characteristics of the soil environment in P. plantation aggregates. Indoor incubation experiments were employed to further evaluate N and C mineralization rates within the aggregates. The primary goals of this study were (1) to assess whether aggregate particle size had an impact on the responses of total N and SOC mineralization to N addition, and (2) to evaluate whether different levels of N addition had the same consistent impact on total N and SOC mineralization. We hypothesized that (1) rates of C and N mineralization vary as a function of soil aggregate particle size, and that (2) the responses of C and N mineralization in forest soils under conditions of N deposition are distinct from one another and associated with the degree of N addition.
2 Materials and methods
2.1 General situation and study design
The P. massoniana plantations in the Three Gorges Reservoir Area in China were aerially seeded during the 1980s. For the present study, a single site located at 30°46′N, 110°55′E was selected in August of 2018. This site has a subtropical monsoon climate with a mean annual precipitation of 1,400 mm, mostly from June to September (~70%), and a mean annual temperature ranging from 14 to 22°C. The elevations range from 800 to 850 m, with an average slope of 20°. The soils at these sites were classified as Luvisols and were characterized by a loamy sandy texture. In total, three replicate plots measuring 20 × 20 m were set up.
A randomized block design was used, with four 3 m × 3 m sample quadrats used for N addition treatments in each replicate plot, totaling 12. A 10 m buffer strip was placed between treatment quadrats. Four different N addition rates were implemented within sample quadrats in each replicate plot, as illustrated in Figure 1: 0 kg N·ha−1 yr.−1 (N0), 30 kg N·ha−1 yr.−1 (N30), 60 kg N·ha−1 yr.−1 (N60), and 90 kg N·ha−1 yr.−1 (N90). These conditions were determined considering the local atmospheric N deposition baseline of 30 kg N·ha−1 yr.−1 (Ge et al., 2017). The N additions for treatments were evenly divided into four parts, with each part being applied in the initial month of every quarter throughout 1 year. In 2018, the respective baseline SOC, total N, total P, available N, available P, pH values and CEC at a soil depth of 0–20 cm were 18.33 g kg−1, 0.94 g kg−1, 0.25 g kg−1, 165.60 mg kg−1, 2.45 mg kg−1, 4.75, and 24.23 cmol kg−1. During the experimental period, neither logging, nor understory removal, nor any other management practice were performed.
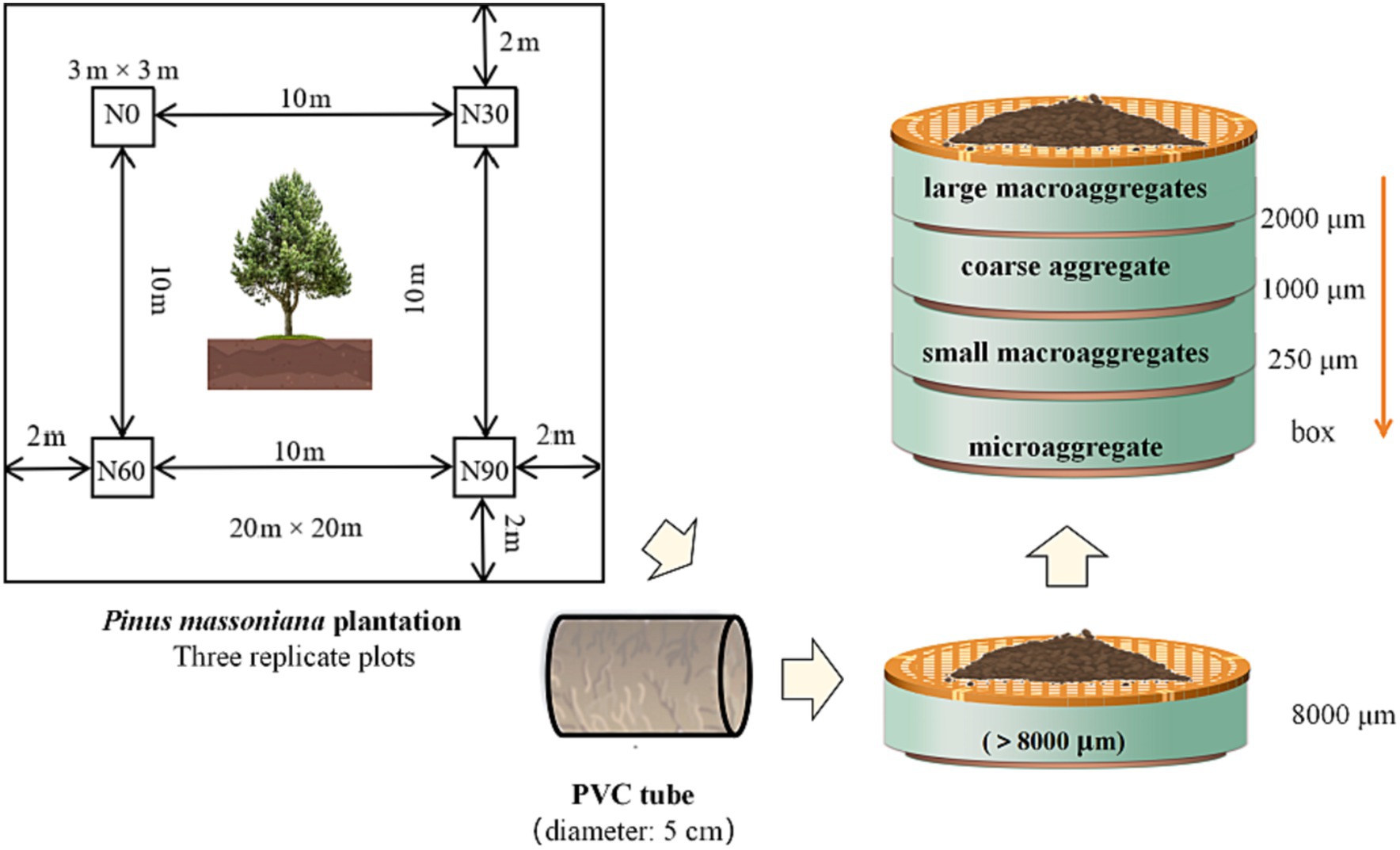
Figure 1. Schematic representation of the separation of different particle sizes aggregates. #Large macroaggregate: 8000–2000 μm, coarse aggregate: 1000–2000 μm, small macroaggregates: 250–1,000 μm, microaggregates: <250 μm.
2.2 Sampling and different particle sizes aggregates separation
In August 2021, litter residue was removed from the soil surface in each sampling quadrat. PVC tubes with a diameter of 5 cm as described by Piccolo et al. (1994), were utilized to sample the soil at 0–20 cm depths across 20–40 locations in each quadrat. The PVC tubes were sealed with plastic wrap and transported back to the laboratory in a refrigerator at 4°C.
Four classes of aggregate-sized materials were then separated from the soil samples. Initially, a series of sieves were used to separate soil after careful removal from the PVC tubes to facilitate aggregate preservation. The soil was gently broken along soil gaps to ensure that all samples could pass through an 8,000 μm sieve. This cut-off level was selected to preserve larger entities present within natural soil, and which were from the same sampling quadrats was mixed. Aggregates were isolated by utilizing a circular sieve shaker machine (type: AS 200 BASIC, Retsch Germany), referencing the procedure used by Bach and Hofmockel (2014). Approximately 200 g of soil was placed on a series of sieves including 2000, 1,000, and 250 μm mesh openings. The stack was shaken at approximately 200–250 rpm for 5 min. The soil was gently removed from each sieve and weighed to determine the aggregate distribution. Aggregates isolated from all methods are referred to by size: large macroaggregates (8000–2000 μm), coarse aggregate (1000–2000 μm), small macroaggregates (250–1,000 μm), and microaggregates (<250 μm).
Each sub-sample of the aggregate fraction was extracted and air-dried in a natural state for the determination of soil organic carbon (SOC), total N, total and available phosphorus (total P, available P), and pH was measured. A sub-sample remainder of each aggregate fraction was saved and immediately transported to a refrigerator at 4°C for soil microbial biomass quantification and rates of soil C and N mineralization determination. Negligible roots, stones, and other debris were removed (Figure 1).
2.3 Testing of soil properties
The sieved portion of the aggregates was naturally air-dried and passed through 2-mm and 0.149-mm sieves to be used for detecting basic soil properties. The soil NH4+-N and NO3−-N levels were quantified by the KCl extraction method. SOM level was determined using the high-temperature exothermic potassium dichromate oxidation–capacitance method, total N level using the Kjeldahl N determination method, total and available P level using the alkali fusion-molybdenum antimony colorimetric method, and hydrochloric acid-ammonium fluoride leaching-molybdenum antimony colorimetric method, respectively. Quantification of soil microbial biomass using the fumigation method.
2.4 Laboratory incubation
In the C mineralization experiment, the collection of CO2 released from the soil into the NaOH solution is essential for quantification. To ensure accurate measurements, it is imperative to tightly seal the incubation jars’ lids to prevent any air exchange between the internal and external environments. However, such sealing is not required for N mineralization studies (Reuland et al., 2022). In order to minimize any potential impact of this procedure on N mineralization results, both cultures were conducted concurrently using two separate systems (Figure 2).
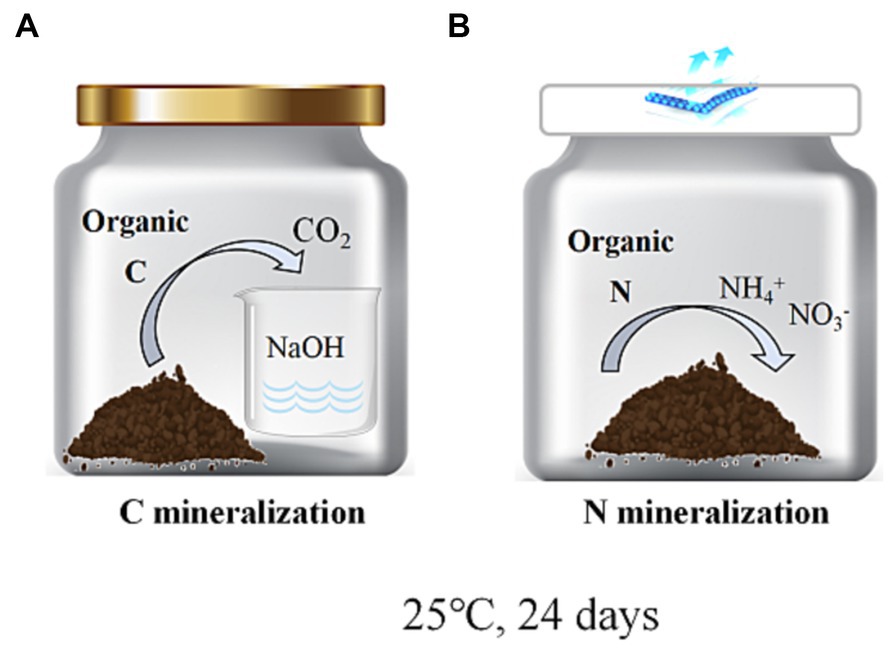
Figure 2. Schematic representation of the indoor culture setup for C mineralization (A) and N mineralization (B).
2.4.1 Carbon mineralization laboratory experiment
Aggregate samples with an equivalent to a dry soil weight of 25 g were initially incubated for a 7-day period in 200 mL jars containing 5 mL of distilled water at 25°C, to restore the activity of soil microorganisms. After this pre-incubation period, the aggregates with an equivalent to a dry soil weight of 25 g were added to a brown 200 mL jar containing an alkali trap (a 50 mL plastic cup filled with 20 mL of 0.25 M NaOH) and maintaining soil water content, with this process being performed for each soil fraction. Soil aeration was improved, and the uniformity of inoculum dispersion was ensured by loosening, stirring, and leveling samples (Figure 2A). Soil moisture was adjusted at 60% of the field capacity. Levels of CO2 absorbed in NaOH, as measured by total oxidizable C, were used to calculate Soil Organic Carbon (SOC) mineralization (Rabbi et al., 2014; Kaarakka et al., 2016). These soil fractions were incubated in an incubator at 25°C for 24 days. Measurements were conducted on days 3, 6, 12, and 24. First, the openings of reaction jars were sealed to prevent CO2 exchange and to maximize the NaOH absorption of all CO2 released from the soil. To mitigate potential issues, shorter sampling intervals were implemented to prevent carbon dioxide saturation in the NaOH solution and maintain adequate oxygen levels in the reaction chambers. Finally, three replicate treatments were established for each jar to maintain consistent reaction conditions, aimed at obtaining highly reliable data and attributing variations in CO2 absorption by the NaOH to the soil quality.
2.4.2 Nitrogen mineralization laboratory experiment
For N mineralization experiments, samples were processed in an identical manner to the methods used for C mineralization experiments. Soil aggregates were incubated for 24 days at 25°C in an incubator, jars were sealed with sterile breathable film. The difference between the final and initial levels of extractable inorganic N concentrations (NH4+-N + NO3−-N), after adjusting for soil fresh dry weight, was used to measure N mineralization. Water content was corrected once in 48 h (Figure 2B). Analyses were repeated in triplicate (Wei et al., 2016). The levels of soil NH4+-N and NO3−-N were quantified by the KCl extraction method.
2.5 Data analysis
The data analysis was conducted using SPSS 24.0 (SPSS Inc., IL, United States). One-way analysis of variance (ANOVA) and Duncan’s test for multiple comparisons (p < 0.05) were employed to analyze data related to soil organic matter (SOM), microbial biomass, and changes in aggregate proportions. Additionally, two-way ANOVAs were used to compare data related to SOC, Total N, and mineralization rates for these aggregates. The figures were created with Origin 2021 (OriginLab Corporation, MA, United States).
3 Results
3.1 Soil organic matter, organic carbon, total nitrogen, and microbial biomass in aggregates
Under conditions of N addition, the proportions of coarse aggregate increased whereas the proportion of small aggregates declined (Figure 3A). Soil organic matter (SOM) levels significantly declined with increasing particle size, as well as SOC and total N. With SOM levels for particles <2000 μm being 5.06–18.29% higher than N0 under N30 and N60 conditions, although N90 were associated with a 14.57–24.15% reduction in SOM levels for this fraction (Table 1). N addition additionally increased total N and SOC proportions in the microaggregates and coarse aggregate (Figures 3B,C). The highest levels of SOC and total N were observed in microaggregates irrespective of the level of N addition (Figures 4A,B), while the proportion of total N and SOC in aggregates ranked from highest to lowest irrespective being as follows: small macroaggregates, coarse aggregate, microaggregates, large macroaggregates (Figures 3B,C). The average soil C/N ratio showed no significant changes with levels of N addition regardless of particle size (Figure 4C). Relative to the control N0 treatment, SMBC and SMBN initially rose and then declined with increasing N addition, with no differences in SMBC/SMBN among different aggregate particle sizes (Table 1).
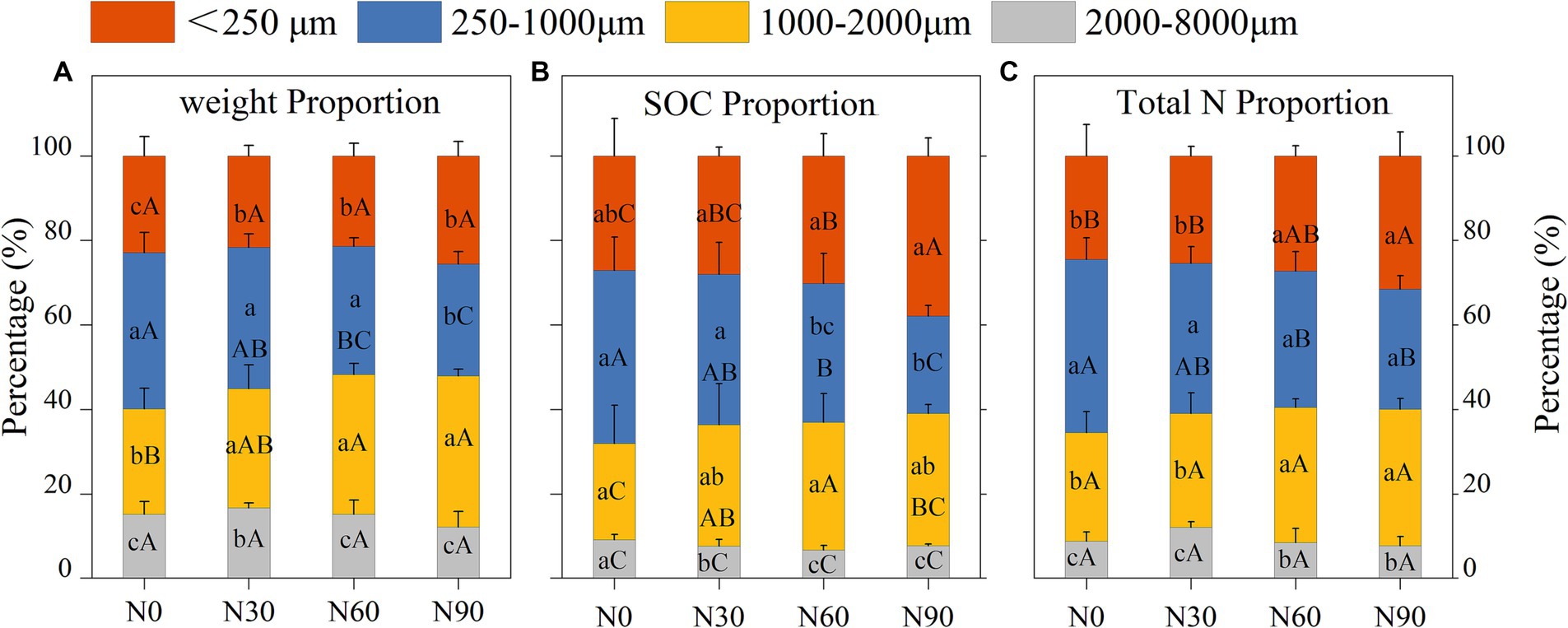
Figure 3. Percentage of weight (A), soil organic carbon (SOC, B), and total nitrogen (total N, C) levels in aggregates (dry soil) after N addition. #The treatments N0, N30, N60, and N90 represent the additional N rates of 0, 30, 60, and 90 kg N ha−1·yr.−1, respectively. Different lowercase letters indicate significant differences in test level (p < 0.05) between particle sizes; different uppercase letters indicate significant differences in test level (p < 0.05) between N addition treatments.
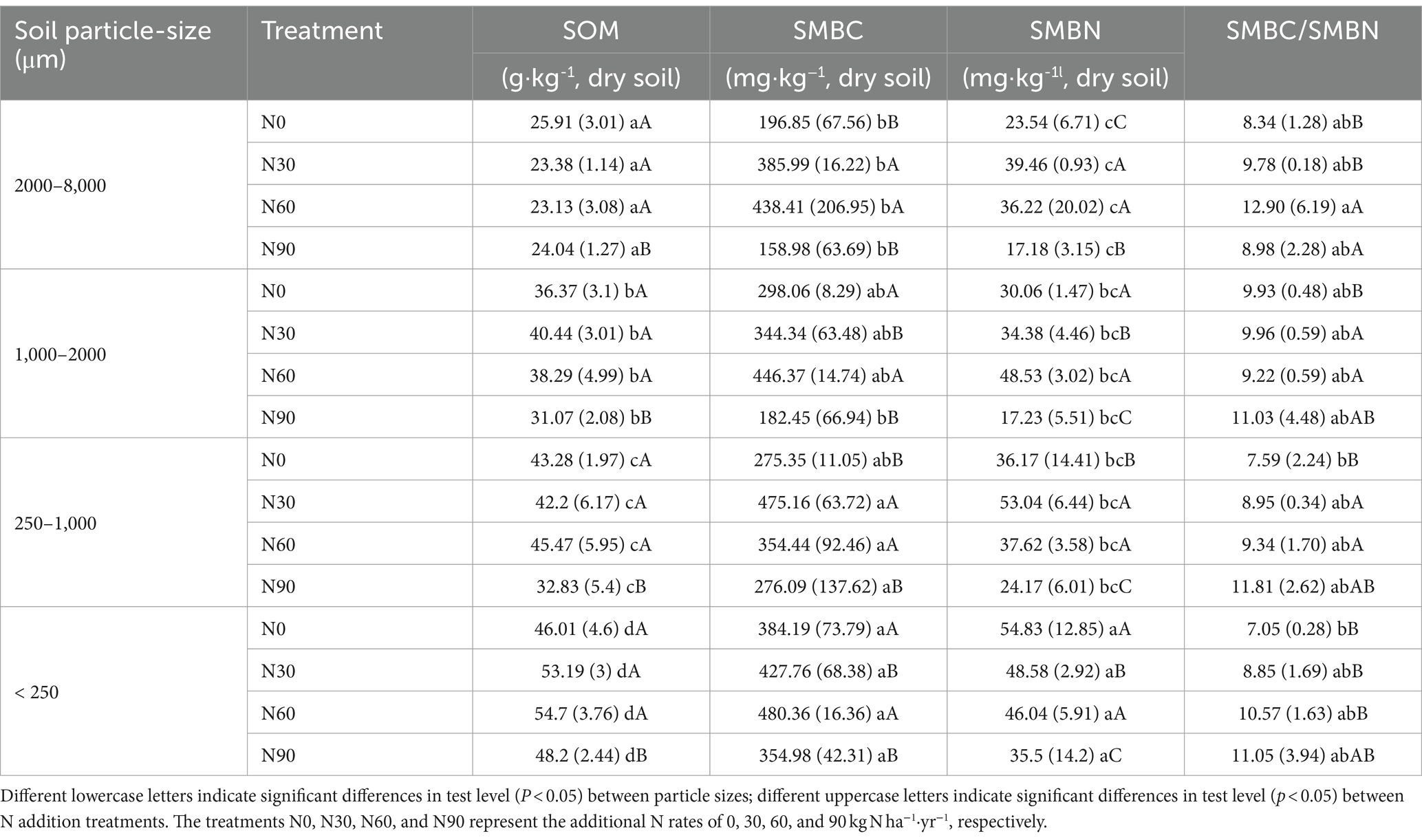
Table 1. Aggregates soil organic matter (SOM), soil microbial biomass carbon (SMBC), soil microbial biomass nitrogen (SMBN), and SMBC/SMBN levels after N addition treatments.
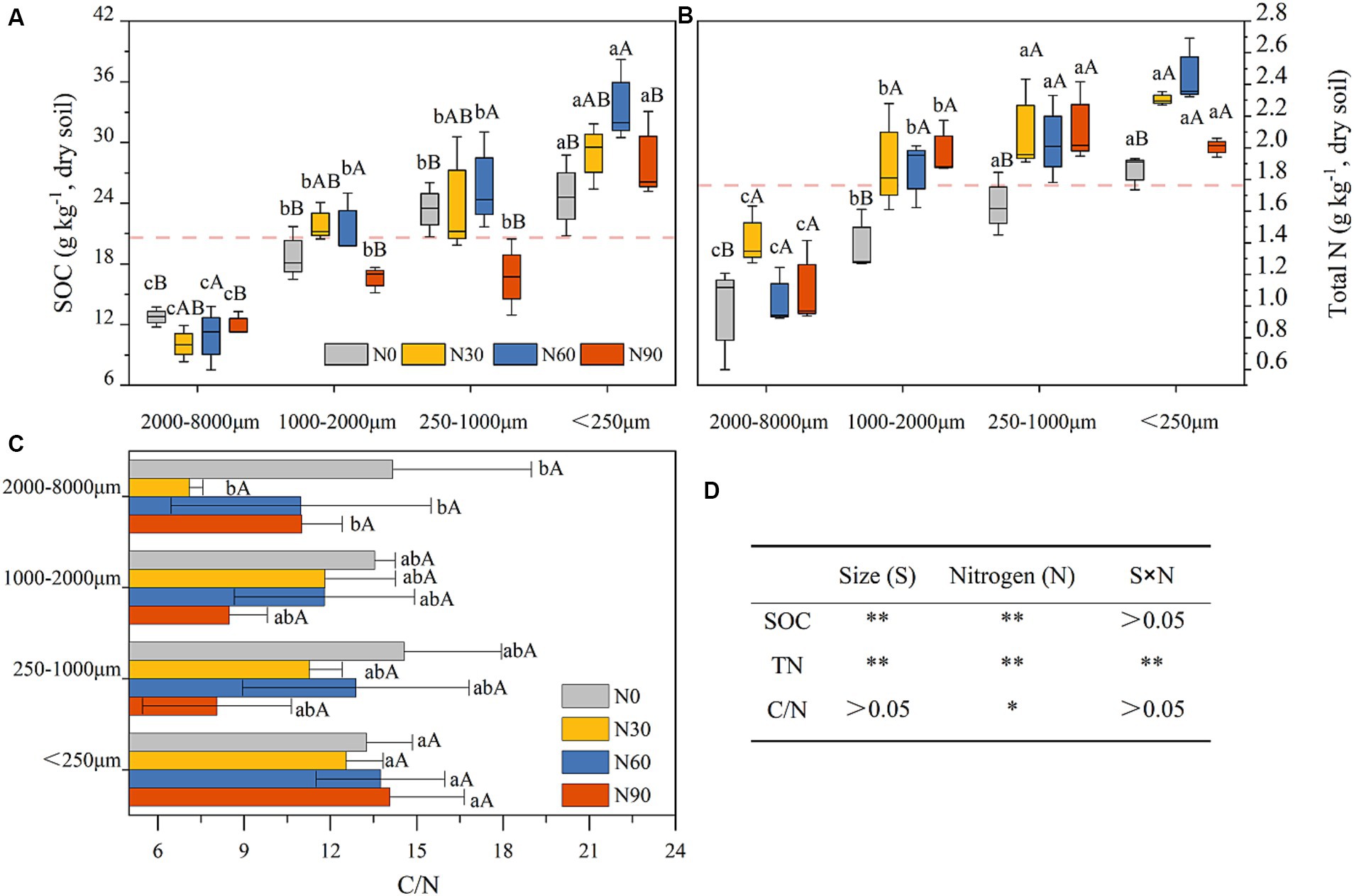
Figure 4. Soil organic carbon (SOC, A), total nitrogen (total N, B), and carbon-nitrogen ratio in values (C / N, C) in aggregates (dry soil) after N addition. #The treatments N0, N30, N60, and N90 represent the additional N rates of 0, 30, 60, and 90 kg N ha−1·yr.−1, respectively. Different lowercase letters indicate significant differences in test level (p < 0.05) between particle sizes; different uppercase letters indicate significant differences in test level (p < 0.05) between N addition treatments. The mean value is represented by the dashed reference line. N, nitrogen; S, size. * P<0.05; ** P<0.01.
3.2 The impact of N addition on rates of C and N mineralization
There was an increase with N addition in ammonification in all fractions except the large macroaggregates, while net nitrification increased with N addition in all fractions but small macroaggregates (Figures 5A,B). Ammoniation per unit N weight showed a “V” shape with an increasing N addition rate, reaching its maximum value at N90 and the lowest value in large aggregates. Nitrification per unit N weight was not significantly associated with particle size reduction but showed a strong stoichiometric dependence on N addition rate (Table 2). Over 80% of net N mineralization rates were attributable to net nitrification. Consistent trends were observed in the curves of net N mineralization and net nitrification rate (Figure 5C). As the incubation period lengthened, there was a decrease in the rates of CO2-C evolution in aggregates. The rate was higher in microaggregates than in macroaggregates, and the addition of N generally led to a decrease in rates of SOC mineralization. This effect was mainly observed for the initial rate (Figure 6).
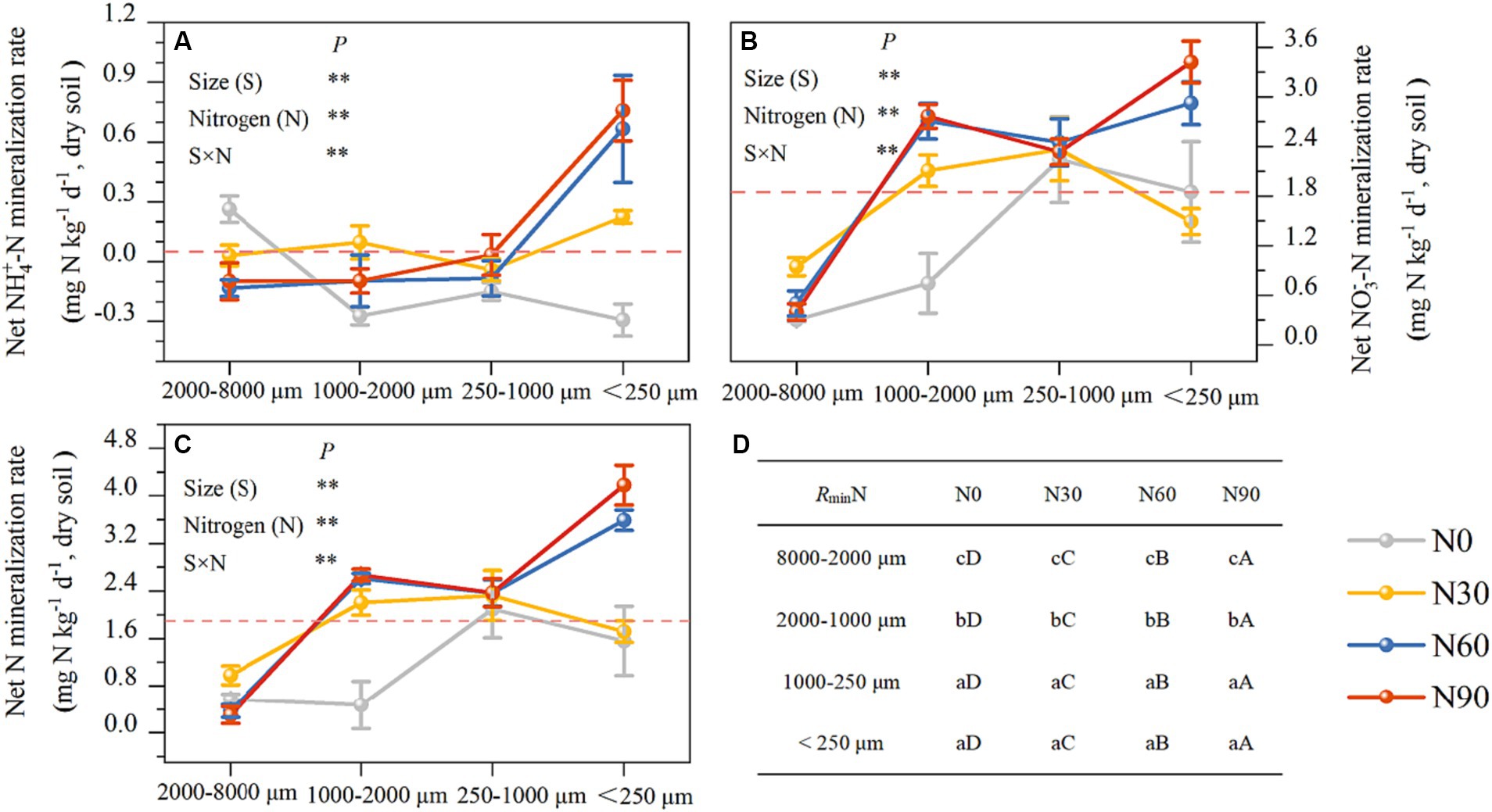
Figure 5. Aggregates (dry soil) N mineralization rate responses to N addition. Net ammoniation mineralization rate (A), net nitrate mineralization rate (B), net N mineralization rate (C), and the significant differences labels (D). #The mean value is represented by the dashed reference line. #The treatments N0, N30, N60, and N90 represent the additional N rates of 0, 30, 60, and 90 kg N ha−1·yr.−1, respectively. N: Nitrogen; S: Size. * P<0.05; ** P<0.01. Different lowercase letters indicate significant differences in test level (p < 0.05) between particle sizes; different uppercase letters indicate significant differences in test level (p < 0.05) between N addition treatments.
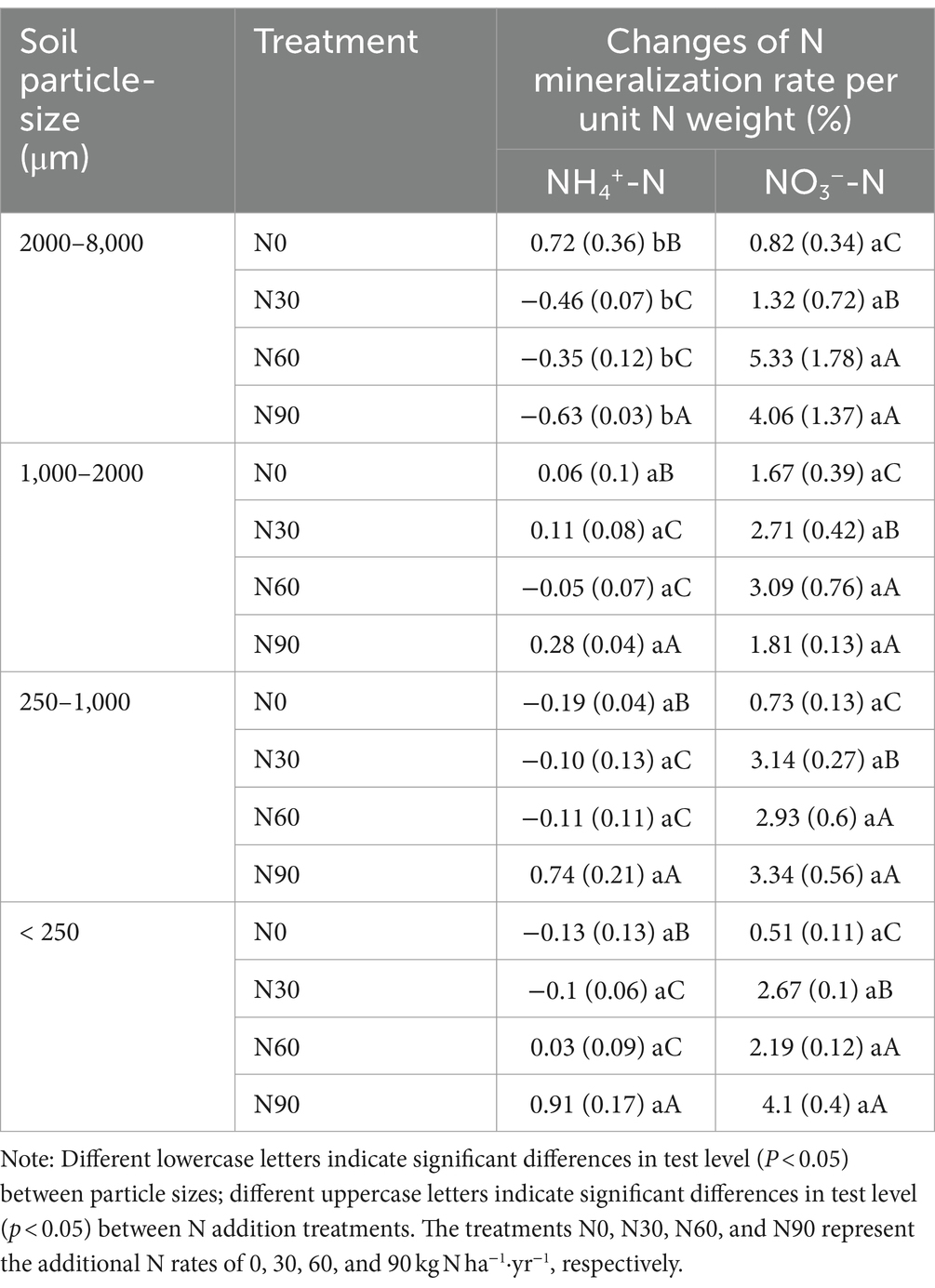
Table 2. Changes of N mineralization rate per unit N weight after N addition treatments in aggregates.
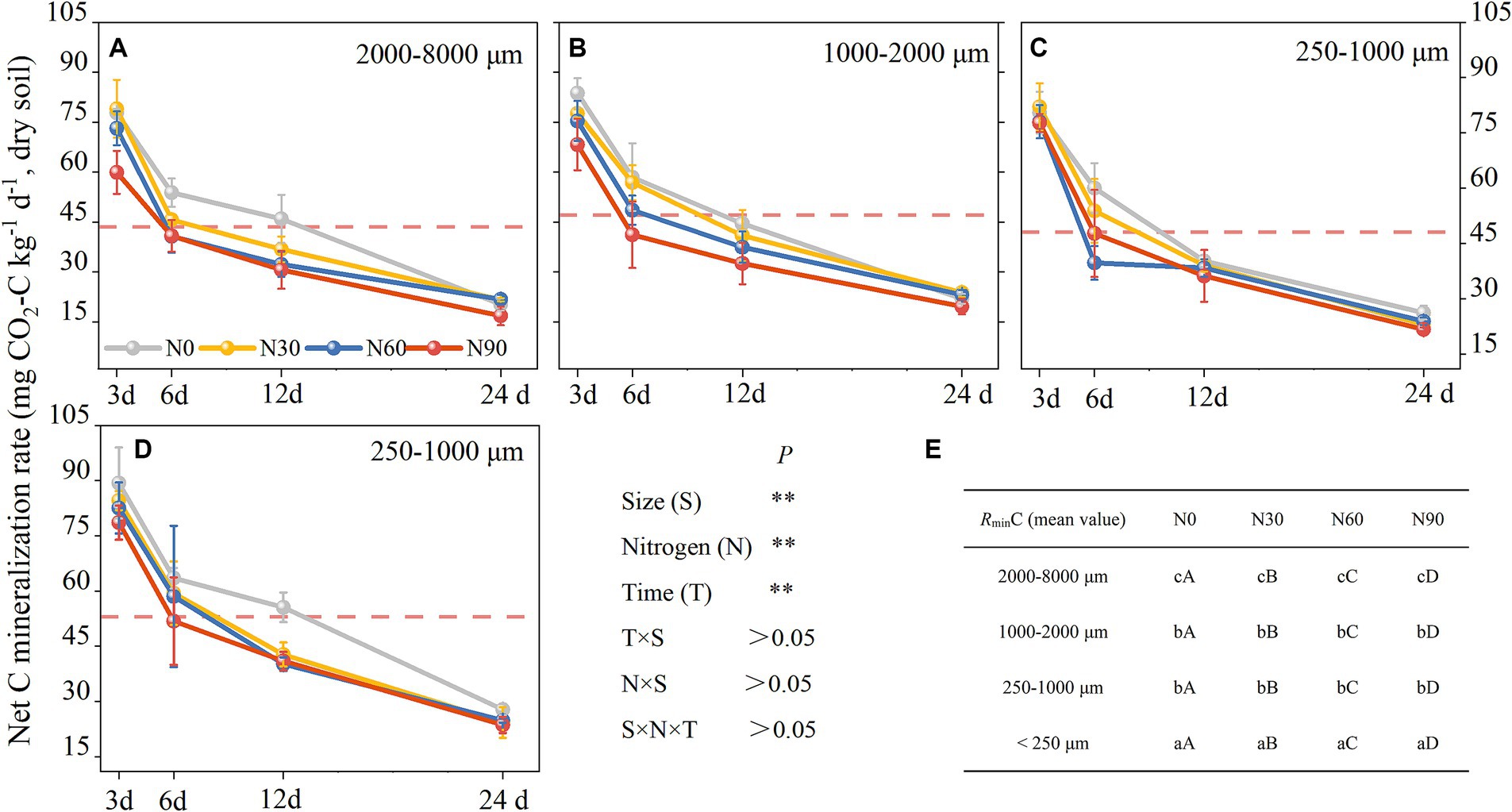
Figure 6. Aggregates (dry soil) C mineralization rates responses to N addition. 2000–8,000 μm fraction (A), 1,000–2000 μm fraction (B), 250–1,000 μm fraction (C), <250 μm fraction (D), and the significant differences labels (E). #The mean value is represented by the dashed reference line. #The treatments N0, N30, N60, and N90 represent the additional N rates of 0, 30, 60, and 90 kg N ha−1·yr.−1, respectively. The mean value is represented by the dashed reference line. N: Nitrogen; S: Size. * P<0.05; ** P<0.01. Different lowercase letters indicate significant differences in test level (p < 0.05) between particle sizes; different uppercase letters indicate significant differences in test level (p < 0.05) between N addition treatments.
3.3 The impact of N addition on cumulative C and N mineralization
N addition resulted in a significant increase in cumulative N mineralization for aggregates relative to N0, with cumulative N mineralization rates under N0 conditions being, on average, 0.52 times, 1.37–6.55 times, and 0.76–12.90 times lower than under N30, N60, and N90 conditions, respectively, across the four analyzed particle sizes (Figure 7A). Cumulative C mineralization in microaggregates were 14.70–33.87%, 14.91–21.31%, and 6.01–15.33% higher than in large macroaggregates, coarse aggregate, and small macroaggregates, respectively, under N0, N30, N60, and N90 (Figure 7B). N addition also reduced mineralized C accumulation, with overall cumulative C mineralization under N0 conditions being 2.32–12.93%, 8.64–14.47%, and 12.66–24.69% higher than N30, N60, and N90, respectively, in large macroaggregates, coarse aggregate, small macroaggregates, and microaggregates (Figure 7B).
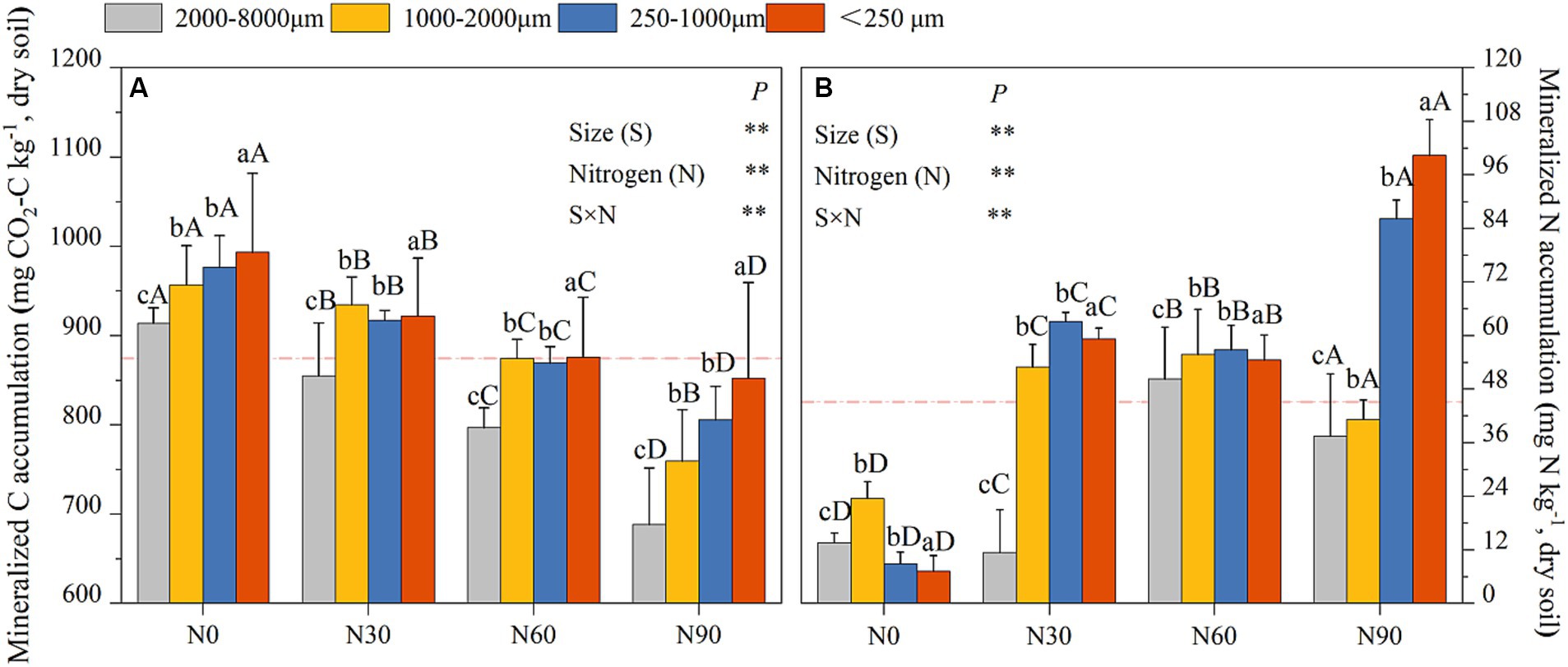
Figure 7. Aggregates (dry soil) cumulative mineralization responses to N addition. C cumulative mineralization (A), N cumulative mineralization (B). #The mean value is represented by the dashed reference line. #The treatments N0, N30, N60, and N90 represent the additional N rates of 0, 30, 60, and 90 kg N ha−1·yr.−1, respectively. Different lowercase letters indicate significant differences in test level (p < 0.05) between particle sizes; different uppercase letters indicate significant differences in test level (p < 0.05) between N addition treatments. The mean value is represented by the dashed reference line. N: Nitrogen; S: Size. * P<0.05; ** P<0.01.
4 Discussion
4.1 N addition influences aggregate SOC and total N levels as well as aggregates stability
N addition at levels N30 - N60 significantly increased SOC and total N levels in fractions <2000 μm, while no effect was observed with excessively high N levels (N90) added (Figures 4A,B). This suggests that appropriate levels of N addition can facilitate the effective accumulation of SOM in this plantation (Simon et al., 2021). The level of SOC is attributed to the dynamic balance between C input and output. A global meta-analysis revealed that SOC gain from increased plant biomass at N addition rates of ≤60 kg N exceeds SOC loss from litter decomposition and mineralization, leading to a net increase in inputs of SOC over microbially mediated outputs (Yang et al., 2022). This is consistent with our results (Figure 4A). In addition, microbial residual C contributes more than 30% to SOC, despite the fact that SMBC accounts for ≤5% of SOC (Miltner et al., 2011; Liang et al., 2019; Wang et al., 2021). Compared with N0, N30 and N60 increased SMBC by 0.11 to 0.96-fold and 0.25 to 1.22-fold, respectively (Table 1). This finding partly explains the increase in SOC levels due to N addition. The increase in total N after the N30 and N60 treatments (Figures 4A,B) indicates that soil absorption of added NH4+ and NO3− surpassed the combined utilization by plants, microorganisms, and N losses caused by leaching, gaseous loss, etc. (Hao et al., 2020). Our findings indicate that smaller aggregate sizes are associated with higher levels of SOC and total N (Figures 4A,B). Previous studies have highlighted the significance of soil aggregates in sequestration SOC (Goebel et al., 2009; Xu et al., 2021), with aligns with our findings. This is smaller aggregate particles with a higher specific surface area can adsorb more SOM (Polláková et al., 2018). Previous research by Nicola et al. (2020) found higher levels of SOC in aggregates with a clay loam texture, corroborating the interpretation of our present results. N90 led to a decrease in soil microbial biomass compared with N0 (Table 1), consequently, the observed decline in aggregate SOM levels at this N added rate, aligns with findings from prior research (Yang and Zhu, 2015; Niu et al., 2021).
Coarse aggregates play a crucial role in maintaining soil aggregate stability, as higher proportions of these aggregates are linked to increased stability. In this study, we observed an increase in the percentage of coarse aggregate weight and a decrease in small macroaggregates due to N addition (Figure 1A). This suggests that N addition improved soil aggregate stability (Liu et al., 2020).
4.2 N addition reduces rates of aggregate C but not N mineralization
N addition reduced rates of cumulative C mineralization and effect sizes in all aggregates (Figure 6). These findings align with previous studies conducted in temperate (Janssens et al., 2010), subtropical (Wang et al., 2017), and tropical forest soils (Mo et al., 2008). This relationship between N addition and SOC mineralization can be elucidated through multiple mechanisms. Firstly, N addition has consistently been demonstrated to reduce microbial biomass, fungal biomass, and overall microbial abundance (Treseder, 2008; Zhou et al., 2019). In this study, high levels of N addition (N90) led to substantial decreases in soil microbial biomass (Table 1). The decrease in soil microbial biomass may explain the reduced mineralization of SOC in aggregates due to N addition (Lu et al., 2021), considering that soil microbes are the primary regulators of SOC decomposition rates (Zhou et al., 2019). Secondly, soil N levels strongly influence SOC mineralization. In N-deficient conditions, nitrogen may act as a stimulus for microbe-mediated SOC mineralization (Moorhead and Sinsabaugh, 2006; Hu et al., 2022). This is due to the need for mineral N in microorganisms, and microbial activities that acquire N require C as an energy source to access difficult-to-obtain N (Bernard et al., 2022; Sokol et al., 2022). In this study, N addition was achieved by spraying study plots with a solution of low-molecular-weight NH4NO3. Once the demand for N was met, economically, microorganisms would no longer maintain a high demand for SOC mineralization. In addition, exogenously applied N was immobilized in a stable soil organic pool following the addition of low-molecular-weight N compounds and through the stabilization of heavy SOC fractions to further reduce mineralized SOC (Neff et al., 2002).
The net increase in NH4+-N and NO3−-N in the soil represents the net soil N mineralization, providing the most direct indication of the changes in inorganic N levels and reflecting the soil’s N supply capacity, which correlates with forest productivity development (Durán et al., 2012; Carolin et al., 2016). Discrepancies in the net N mineralization outcomes (representing the net accumulation of NH4+-N and NO3−-N) in soils subjected to various N additions under constant mineralization conditions are the focal point of our examination. Nitrification and nitrate reduction, the two reactions are a dynamic process of conversion of NH4+-N and NO3−-N (Kaur et al., 2010), therefore the rate of N transformation for this pathway was omitted from the calculation of net N mineralization. The experimental mineralization was set up to be carried out under well-gassed conditions, which ensured the mineralization of N and suppressed the degree of excessive denitrification. Consistent experimental procedures were employed across all samples. The N addition significantly increased net nitrification rates, which contribute to more than 80% of net N mineralization rates. Therefore, both responses to N addition are similar (Figures 5B,C). Net nitrification rates did not show a linear correlation with the amount of added N. The net nitrification rates of large aggregates and coarse aggregates decreased under the N90 treatment compared to the N60 treatment (Figures 3B,C). The decline may be attributed to the decrease in available phosphorus levels, which hinders microbial activities after an excess of soil N (Table 1). Net ammonification rates were negative in some cases. Net ammonification was the absolute increment of the amount of NH4+-N at the end of the incubation experiment minus the amount at the beginning. NH4+-N is a preferred source of N for soil microorganisms to utilize (Rice et al., 1989; Zhang et al., 2008). As the incubation proceeded, the microorganisms continued to consume both the original and newly mineralized NH4+-N in the soil to perform their activities (Janne et al., 1998). Further, NH4+-N is the substrate of nitrification reaction in ecosystems, and the majority of soil NH4+-N is nitrified in N-saturated ecosystems (Ouyang et al., 2016). The net ammonification rate became negative when consumption surpassed mineralization. The addition of nitrogen reduced the net ammonification rate in large macroaggregates compared to the N0 condition (Figure 5A). This reduction was a result of the stimulated microbial activity due to nitrogen addition and the increased net nitrification rate, as indicated in Figure 5B, both reflecting the accelerated consumption of NH4+-N from the soil. However, NH4+-N mineralization accumulated under the N90 treatment in microaggregates due to the significant input of exogenous NH4+-N to meet the microbial demand. Ultimately, N addition can enhance nitrogen mineralization in aggregates relative to the N0 condition, as illustrated in Figure 5B, consistent with our hypothesis and prior studies (Xue et al., 2005; Nave et al., 2009). We conducted measurements of net N transformation indices (net ammonification and net nitrification rate) in the soil (Figure 5) and calculated the changes in the N mineralization rate per unit N weight (Table 2). We found that the decrease in aggregate particle size was associated with a significant increase in the net nitrification rate, illustrating a dynamic correlation between these parameters. Interestingly, this enhancing effect was not evident when analyzing the nitrification rate per unit N weight, indicating a more intricate interplay within the soil ecosystem. The elevated levels of N found in the soil post-N application can be attributed to N accumulation surpassing both natural depletion and losses, a phenomenon extensively discussed by Hao et al. (2020) and Chen et al. (2024). This phenomenon is more pronounced in smaller particle sizes compared to larger aggregates, as depicted in Figure 4B. Consequently, smaller particles accumulate higher N levels, resulting in a minimal variation in the nitrification rate per unit N weight among different aggregate sizes. These findings highlight the complex interactions governing N cycling dynamics in the soil environment and emphasize the role of aggregate particle size in influencing N transformation processes.
In order to minimize CO2 exchange inside and outside the reaction jars, and to ensure that NaOH absorbs all and only the CO2 released from the aggregates, the mouths of the reaction jars were sealed throughout the experiments. For each jar, three replicated treatments were set up to ensure the maintenance of coincident culture conditions, facilitating the acquisition of highly dependable data and linking discrepancies in CO2 absorption by NaOH to soil quality. Consequently, separate reaction jars were utilized for quantifying the rates of N and C mineralization. It is important to acknowledge that the statements in the preceding discussion may be influenced by the examination of C and N mineralization in two distinct yet simultaneous experiments.
4.3 Aggregate structural properties reduce SOC and total N mineralization
As the number of days in culture increased, rates of SOC mineralization slowed until stabilizing at the end of the culture period (Figure 4). At the start of this culture period, high levels of active SOC were evident in the soil and microbes were able to rapidly decompose this material (Cao et al., 2021). Subsequently, as the levels of active soil SOC diminished, the microbes shifted to utilizing inert SOC, consequently leading to reduced SOC mineralization rates (Semenov et al., 2010; Rabbi et al., 2014). Various factors influence soil aggregation, such as plant roots, fauna, microbes, environmental and physical forces, as well as the inorganic and organic binding agents within a specific soil system (Šimanský and Bajčan, 2014). The analyses indicated a higher rate of C mineralization in the <250 μm fraction compared to the 250–1,000 μm fraction, consistent with the hypothesis that C mineralization rates increase with decreasing soil aggregate sizes (Figure 5A). The physical characteristics of macroaggregates contribute to less efficient interactions between the sequestered particulate matter and soil microbes (Galicia-Andres et al., 2021) such that the organic particles therein are less amenable to microbial decomposition (Carolin et al., 2016). This mechanism ultimately contributes to reduced rates of C mineralization in macroaggregates. Aggregate SOC content has been shown to be significantly linked to cumulative C mineralization (Wang et al., 2017). In this context, SOC content tended to increase with decreasing aggregate fraction size such that the <250 μm fraction, harbored SOC with a higher potential for mineralization (Figure 2A). C mineralization levels throughout the culture period were the result of the accumulation of the effects of daily mineralization such that large aggregate formation reduces aggregate turnover rates.
In this study, rates of N mineralization initially rose as soil aggregate particle size decreased before declining under N0 conditions (Figure 5B). The trends in aggregate N mineralization following N addition being similar to those for C. This implies that the formation of aggregates diminishes soil nitrogen turnover rates by protecting organic nitrogen within them from continual microbe-mediated decomposition (Figure 5B). There are three contributing explanations for these results. For one, even under identical N treatment conditions, varying soil N mineralization rates were observed that were largely dependent on mineralizable N reserves (Accoe et al., 2004; Zaman and Chang, 2004) such that N mineralization within these aggregates was ultimately positively correlated with total N level. Second, various physical and chemical environmental factors could break down larger aggregates into smaller microaggregates and other sticky particles, releasing microbial biomass compounds and contributing to the mineralization activity of microaggregate (Lehmann et al., 2007; Dai et al., 2015). This aligns well with previous theories that suggest higher rates of SOC mineralization in microaggregates. Organic particles are generally enclosed within layers of large aggregates. Meanwhile, the smaller surface area of microaggregates can expose these organic particles to microbial decomposition, contributing to higher rates of mineralization (Guidi et al., 2021; Yudina et al., 2022). Third, in the present study higher levels of microbial biomass were evident in smaller aggregates. Higher microbial load in microaggregate has been associated with increased levels of activities for hydrolases including urease, nitrate reductase, L-glutaminase, and beta-glucosidase, thus contributing to elevated rates of N mineralization such that following N input (Khorsandi and Nourbakhsh, 2007; Li et al., 2021), such N mineralization increased. The results indicated the highest net N conversion and nitrification rates in microaggregates under N90 treatment conditions (Figure 5B), indicating that high N input can raise effective soil N concentrations and intensify mineral N loss, reducing the soil’s N fixation capacity (Fang et al., 2009).
5 Conclusion
In summary, these results demonstrate the significant role of soil aggregates in determining the stability of soil organic carbon (SOC) through carbon sequestration. The structural properties of aggregates were associated with soil nitrogen (N) fixation through similar mechanisms. However, N addition has various effects on soil N and C mineralization in these aggregates, suppressing C mineralization while simultaneously accelerating the mineralization of N present therein. These effects are particularly evident in microaggregates. These data emphasize the role of soil aggregates as regulators of C and N cycling and sequestration within terrestrial ecosystems under conditions of N deposition. Considering the role of these aggregates as mediators of N and C sequestration, it is important to fully consider their effects when analyzing the release of inorganic substances in future studies of soil mineralization. Additionally, increases in N deposition led to a reduction in available phosphorus (P), offering another crucial consideration for future research.
Data availability statement
The original contributions presented in the study are included in the article/Supplementary material, further inquiries can be directed to the corresponding author.
Author contributions
TC and TR envisioned and wrote the manuscript. YS, LW, PS, MZ and JL did the experimental work, which was supervised by WX and LZ. All authors contributed to the article and approved the submitted version.
Funding
The Fundamental Research Fund of the Chinese Academy of Forestry (No. CAFYBB2021ZE003).
Acknowledgments
We would like to thank mjeditor (https://www.mjeditor.com) for English language editing.
Conflict of interest
The authors declare that they have no known competing financial interests or personal relationships that could have appeared to influence the work reported in this paper.
Publisher’s note
All claims expressed in this article are solely those of the authors and do not necessarily represent those of their affiliated organizations, or those of the publisher, the editors and the reviewers. Any product that may be evaluated in this article, or claim that may be made by its manufacturer, is not guaranteed or endorsed by the publisher.
Supplementary material
The Supplementary material for this article can be found online at: https://www.frontiersin.org/articles/10.3389/ffgc.2024.1240577/full#supplementary-material
References
Accoe, F., Boeckx, P., Busschaert, J., Hofman, G., and Van-Cleemput, O. (2004). Gross n transformation rates and net n mineralisation rates related to the c and n contents of soil organic matter fractions in grassland soils of different age. Soil Biol. Biochem. 36, 2075–2087. doi: 10.1016/j.soilbio.2004.06.006
Ahirwal, J., Gogoi, A., and Sahoo, U. K. (2022). Stability of soil organic carbon pools affected by land use and land cover changes in forests of eastern Himalayan region, India. Catena 215:106308. doi: 10.1016/j.catena.2022.106308
Bach, E. M., and Hofmockel, K. S. (2014). Soil aggregate isolation method affects measures of intra-aggregate extracellular enzyme activity. Soil Boil Biochem. 69, 54–62. doi: 10.1016/j.soilbio.2013.10.033
Baldos, A. P., Corre, M. D., and Veldkamp, E. (2015). Response of N cycling to nutrient inputs in forest soils across a 1000–3000 m elevation gradient in the Ecuadorian Andes. Ecology 96, 749–761. doi: 10.1890/14-0295.1
Bernard, L., Basile-Doelsch, I., Derrien, D., Fanin, N., Fontaine, S., Guenet, B., et al. (2022). Advancing the mechanistic understanding of the priming effect on soil organic matter mineralisation. Funct. Ecol. 36, 1355–1377. doi: 10.1111/1365-2435.14038
Besnard, E., Chenu, C., Balesdent, J., Puget, P., and Arrouays, D. (1996). Fate of particulate organic matter in soil aggregates during cultivation. Eur. J. Soil Sci. 47, 495–503. doi: 10.1111/j.1365-2389.1996.tb01849.x
Blaško, R., Hogberg, P., Bach, L. H., and Hogberg, M. N. (2013). Relations among soil microbial community composition, nitrogen turnover, and tree growth in N-loaded and previously N-loaded boreal spruce forest. For. Ecol. Manag. 302, 319–328. doi: 10.1016/j.foreco.2013.02.035
Bossio, D. A., Cook-Patton, S. C., and Griscom, B. W. (2020). The role of soil carbon in natural climate solutions. Nat. Sustain. 3, 391–398. doi: 10.1038/s41893-020-0491-z
Bucka, F. B., Kölbl, A., Uteau, D., Peth, S., and Kögel-Knabne, I. (2019). Organic matter input determines structure development and aggregate formation in artificial soils. Geoderma 354:113881. doi: 10.1016/j.geoderma.2019.113881
Cai, A. D., Xu, H., Shao, X. F., Zhu, P., Zhang, W. J., Xu, M. G., et al. (2016). Carbon and nitrogen mineralization in relation to soil particle-size fractions after 32 years of chemical and manure application in a continuous maize cropping system. PLoS One 11:e0152521. doi: 10.1371/journal.pone.015252
Cao, R. R., Chen, L. C., Hou, X. C., Lu, X. T., and Li, H. M. (2021). Nitrogen addition reduced carbon mineralization of aggregates in forest soils but enhanced in paddy soils in South China. Ecol. Process. 10:45. doi: 10.1186/s13717-021-00319-z
Carolin, B., Olivia, K., Angelika, K., Margit-Von, L., and Ingrid, K. K. (2016). Carbon and nitrogen mineralization in hierarchically structured aggregates of different size. Soil Tillage Res. 160, 23–33. doi: 10.1016/j.still.2015.12.011
Chen, T., Cheng, R., Xiao, W., Shen, Y. F., Wang, L. J., Sun, P. F., et al. (2024). Nitrogen addition enhances soil nitrogen mineralization through an increase in Mineralizable organic nitrogen and the abundance of functional genes. J. Soil Sci. Plant Nutr., 1–13. doi: 10.1007/s42729-023-01600-0
Dai, J., Hu, J. L., Zhu, A. N., Bai, J. F., Wang, J. H., and Lin, X. G. (2015). No tillage enhances arbuscular mycorrhizal fungal population, glomalin-related soil protein content, and organic carbon accumulation in soil macroaggregates. J. Soils Sediments 15, 1055–1062. doi: 10.1007/s11368-015-1091-9
Du, Z. G., Wang, J. W., Zhou, G. Y., Bai, S. H., Zhou, L. Y., Fu, Y. L., et al. (2021). Differential effects of nitrogen vs. phosphorus limitation on terrestrial carbon storage in two subtropical forests: a Bayesian approach. Sci. Total Environ. 795, 148485–148412. doi: 10.1016/j.scitotenv.2021.148485
Durán, J., Morse, J. L., and Groffman, P. M. (2012). Comparison of in situ methods to measure N mineralization rates in forest soils. Soil Boil Biochem. 46, 145–147. doi: 10.1016/j.soilbio.2011.12.005
Fang, Y. T., Zhu, W. X., Gundersen, P., Mo, J. M., Zhou, G. Y., and Yoh, M. (2009). Large loss of dissolved organic nitrogen from nitrogen-saturated forests in subtropical China. Ecosystems 12, 33–45. doi: 10.1007/s10021-008-9203-7
Galicia-Andres, E., Escalona, Y., Oostenbrink, C., Tunega, D., and Gerzabek, M. H. (2021). Soil organic matter stabilization at molecular scale: the role of metal cations and hydrogen bonds. Geoderma 401:115237. doi: 10.1016/j.geoderma.2021.115237
Galloway, J. N., Dentener, F. J., Capone, D. G., Boyer, E. W., Howarth, R. W., Seitzinger, S. P., et al. (2004). Nitrogen cycles: Past, present, and future. Biogeochemistry 70, 153–226. doi: 10.1007/s10533-004-0370-0
Gao, F., Cui, X. Y., Sang, Y., and Song, J. F. (2020). Changes in soil organic carbon and total nitrogen as affected by primary forest conversion. For. Ecol. Manag. 463:118013. doi: 10.1016/j.foreco.2020.118013
Gao, W., Kou, L., Zhang, J., Müller, C., Yang, H., and Li, S. (2016). Ammonium fertilization causes a decoupling of ammonium cycling in a boreal forest. Soil Boil Biochem. 101, 114–123. doi: 10.1016/j.soilbio.2016.07.007
García-Oliva, F., Oliva, M., and Sveshtarova, B. (2004). Effect of soil macroaggregates crushing on C mineralization in a tropical deciduous forest ecosystem. Plant Soil 259, 297–305. doi: 10.1023/B:PLSO.0000020978.38282.dc
Ge, X. G., Xiao, W. F., Zeng, L. X., Huang, Z. L., Zhou, B. Z., Schaub, M., et al. (2017). Relationships between soil-litter interface enzyme activities and decomposition in Pinus massoniana plantations in China. J. Soils Sediments 17, 996–1008. doi: 10.1007/s11368-016-1591-2
Goebel, M. O., Woche, S. K., and Bachmann, J. (2009). Do soil aggregates really protect encapsulated organic matter against microbial decomposition? Biologia 64, 443–448. doi: 10.2478/s11756-009-0065-z
Guidi, P., Falsone, G., Wilson, C., Cavani, L., Ciavatta, C., and Marzadori, C. (2021). New insights into organic carbon stabilization in soil macroaggregates: An in situ study by optical microscopy and SEM-EDS technique. Geoderma 397:115101. doi: 10.1016/j.geoderma.2021.115101
Hao, T. X., Zhang, Y. Y., Zhang, J. B., Muller, C., Li, K. H., Zhang, K. P., et al. (2020). Chronic nitrogen addition differentially affects gross nitrogen transformations in alpine and temperate grassland soils. Soil Boil Biochem. 149:107962. doi: 10.1016/j.soilbio.2020.107962
Hu, X. J., Gu, H. D., Liu, J. J., Wei, D., Zhu, P., Cui, X. A., et al. (2022). Metagenomics reveals divergent functional profiles of soil carbon and nitrogen cycling under long-term addition of chemical and organic fertilizers in the black soil region. Geoderma 418:115846. doi: 10.1016/j.geoderma.2022.115846
Huang, Z. Q., Clinton, P. W., Baisden, W. T., and Davis, M. R. (2011). Long-term nitrogen additions increased surface soil carbon concentration in a forest plantation despite elevated decomposition. Soil Boil Biochem. 43, 302–307. doi: 10.1016/j.soilbio.2010.10.015
Janne, K. O., Arne, O. S., and Magne, H. (1998). Effects of weekly nitrogen additions on N cycling in a coniferous forest catchment, Gårdsjön. Sweden. For. Ecol. Manag. 101, 227–249. doi: 10.1016/S0378-1127(97)00140-0
Janssens, I. A., Dieleman, W., Luyssaert, S., Subke, J. A., Reichstein, M., Ceulemans, R., et al. (2010). Reduction of forest soil respiration in response to nitrogen deposition. Nat. Geosci. 3, 315–322. doi: 10.1038/ngeo844
Jing, H., Li, J. J., Yan, B. S., Wei, F. R., Wang, G. L., and Liu, G. B. (2021). The effects of nitrogen addition on soil organic carbon decomposition and microbial C-degradation functional genes abundance in a Pinus tabulaeformis forest. For. Ecol. Manag. 489:119098. doi: 10.1016/j.foreco.2021.119098
John, B., Yamashita, T., Ludwig, B., and Flessa, H. (2005). Storage of organic carbon in aggregate and density fractions of silty soils under different types of land use. Geoderma 128, 63–79. doi: 10.1016/j.geoderma.2004.12.013
Kaarakka, L., Hyvonen, R., Stromgren, M., Palviainen, M., Persson, T., Olsson, B. A., et al. (2016). Carbon and nitrogen pools and mineralization rates in boreal forest soil after stump harvesting. For. Ecol. Manag. 377, 61–70. doi: 10.1016/j.foreco.2016.06.042
Kaur, A. J., Ross, D. S., and Fredriksen, G. (2010). Effect of soil mixing on nitrification rates in soils of two deciduous forests of Vermont, USA. Plant Soil 331, 289–298. doi: 10.1007/s11104-009-0253-1
Khorsandi, N., and Nourbakhsh, F. (2007). Effect of amendment of manure and corn residues on soil N mineralization and enzyme activity. Agron. Sustain. 27, 139–143. doi: 10.1051/agro:2007001
Lal, R. (2004). Soil carbon sequestration impacts on global climate change and food security. Science 304, 1623–1627. doi: 10.1126/science.1097396
Lehmann, J., Kinyangi, J., and Solomon, D. (2007). Organic matter stabilization in soil microaggregates: implications from spatial heterogeneity of organic carbon contents and carbon forms. Biogeochemistry 85, 45–57. doi: 10.1007/s10533-007-9105-3
Li, Z. J., Reichel, R., Li, Z. M., Yang, K. J., Zhang, L., Tan, B., et al. (2021). Effects of snow absence on available N pools and enzyme activities within soil aggregates in a spruce forest on the eastern Tibetan plateau. Soil Ecol. Letters. 4, 376–382. doi: 10.1007/s42832-021-0117-z
Li, J. J., Yang, C., Liu, X. L., Ji, H. Z., and Shao, X. Q. (2020). Soil aggregate size influences the impact of inorganic nitrogen deposition on soil nitrification in an alpine meadow of the Qinghai-Tibet plateau. PeerJ. 8:e8230. doi: 10.7717/peerj.8230
Liang, C., Amelung, W., Lehmann, J., and Kästner, M. (2019). Quantitative assessment of microbial necromass contribution to soil organic matter. Glob. Chang. Biol. 25, 3578–3590. doi: 10.1111/gcb.14781
Liao, R. K., Han, Y. G., and Guo, Z. F. (2021). Assessing the impact of soil aggregate size on mineralization of nitrogen in different soils, China. Catena 203:105358. doi: 10.1016/j.catena.2021.105358
Liu, H. F., Wang, X. K., Liang, C. T., Ai, Z. M., Wu, Y., Xu, H. W., et al. (2020). Glomalin-related soil protein affects soil aggregation and recovery of soil nutrient following natural revegetation on the loess plateau. Geoderma 357:113921. doi: 10.1016/j.geoderma.2019.113921
Lu, X. K., Mao, Q. G., Wang, Z. H., Mori, T., Mo, J. M., Su, F. L., et al. (2021). Long-term nitrogen addition decreases soil carbon mineralization in an N-rich primary tropical Forest. Forests 12:734. doi: 10.3390/f12060734
Mehnaz, K. R., Keitel, C., and Dijkstra, F. A. (2018). Effects of carbon and phosphorus addition on microbial respiration, N2O emission, and gross nitrogen mineralization in a phosphorus-limited grassland soil. Biol. Fertil. Soils 54, 481–493. doi: 10.1007/s00374-018-1274-9
Miltner, A., Bombach, P., Schmidt-Brücken, B., and Kästner, M. (2011). SOM genesis: microbial biomass as a significant source. Biogeochemistry 111, 41–55. doi: 10.1007/s10533-011-9658-z
Mo, J. M., Zhang, W., Zhu, W. X., Gundersen, P., Fang, Y., Li, D., et al. (2008). Nitrogen addition reduces soil respiration in a mature tropical forest in southern China. Glob. Chang. Biol. 14, 403–412. doi: 10.1111/j.1365-2486.2007.01503.x
Moorhead, D. L., and Sinsabaugh, R. L. (2006). A theoretical model of litter decay and microbial interaction. Ecol. Monogr. 76, 151–174. doi: 10.1890/0012-9615(2006)076[0151:ATMOLD]2.0.CO;2
Nave, L. E., Vance, E. D., Swanston, C. W., and Curtis, P. S. (2009). Impacts of elevated N inputs on north temperate forest soil C storage, C/N, and net N-mineralization. Geoderma 153, 231–240. doi: 10.1016/j.geoderma.2009.08.012
Neff, J. C., Townsend, A. R., Gleixner, G., Lehman, S. J., Turnbull, J., and Bowman, W. D. (2002). Variable effects of nitrogen additions on the stability and turnover of soil carbon. Nature 419, 915–917. doi: 10.1038/nature01136
Nicola, D. F., Ilaria, P., Antonio, B., Riccardo, P., and Francesco, M. (2020). Organic carbon storage potential in deep agricultural soil layers: evidence from long-term experiments in Northeast Italy. Agricul. Ecosyst Environ. 300:106967. doi: 10.1016/j.agee.2020.106967
Niu, G. X., Hasi, M., Wang, R. Z., Wang, Y. L., Geng, Q. Q., Hu, S. Y., et al. (2021). Soil microbial community responses to long-term nitrogen addition at different soil depths in a typical steppe. Appl. Soil Ecol. 167:104054. doi: 10.1016/j.apsoil.2021.104054
Ouyang, Y., Norton, J. M., Stark, J. M., Reeve, J. R., and Habteselassie, M. Y. (2016). Ammonia-oxidizing bacteria are more responsive than archaea to nitrogen source in an agricultural soil. Soil Boil Biochem. 96, 4–15. doi: 10.1016/j.soilbio.2016.01.012
Peñuelas, J., Sardans, J., Rivas-Ubach, A., and Janssens, I. A. (2012). The human-induced imbalance between C, N and P in Earth's life system. Glob. Chang. Biol. 18, 3–6. doi: 10.1111/j.1365-2486.2011.02568.x
Piccolo, M. C., Neill, C., and Cerri, C. C. (1994). Net nitrogen mineralization and net nitrification along a tropical forest-to-pasture chronosequence. Plant Soil 162, 61–70. doi: 10.1007/BF01416090
Polláková, N., Šimanský, V. V., and Kravka, M. (2018). The influence of soil organic matter fractions on aggregates stabilization in agricultural and forest soils of selected Slovak and Czech hilly lands. J. Soils Sediments 18, 2790–2800. doi: 10.1007/s11368-017-1842-x
Post, W. M., Emanuel, W. R., Zinke, P. J., and Stangenberger, A. G. (1982). Soil carbon pools and world life zones. Nature 298, 156–159. doi: 10.1038/298156a0
Jha, P., Garg, N., Lakaria, B. L., Biswas, A. K., and Rao, A. S. (2012). Soil and residue carbon mineralization as affected by soil aggregate size. Soil Tillage Res. 121, 57–62. doi: 10.1016/j.still.2012.01.018
Prescott, C. E. (1995). Does nitrogen availability control rates of litter decomposition in forests? Plant Soil 168-169, 83–88. doi: 10.1007/BF00029316
Rabbi, S. M. F., Wilson, B. R., Lockwood, P. V., Daniel, H., and Young, I. M. (2014). Soil organic carbon mineralization rates in aggregates under contrasting land uses. Geoderma 216, 10–18. doi: 10.1016/j.geoderma.2013.10.023
Reuland, G., Sigurnjak, I., Dekker, H., Sleutel, S., and Meers, E. (2022). Assessment of the carbon and nitrogen mineralisation of Digestates elaborated from distinct feedstock profiles. Agronomy-Basel 12:456. doi: 10.3390/agronomy12020456
Rice, C. W., and Tiedje, J. M. (1989). Regulation of nitrate assimilation by ammonium in soils and in isolated soil microorganisms. Soil Boil Biochem. 21, 597–602. doi: 10.1016/0038-0717(89)90135-1
Semenov, V. M., Ivannikova, L. A., Semenova, N. A., Khodzhaeva, A. K., and Udal'tsov, S. N. (2010). Organic matter mineralization in different soil aggregate fractions. Eurasian Soil Sc. 43, 141–148. doi: 10.1134/S1064229310020031
Šimanský, V., and Bajčan, D. (2014). Stability of soil aggregates and their ability of carbon sequestration. Soil Water Res. 9, 111–118. doi: 10.17221/106/2013-SWR
Simon, L., Andrea, W., Markus, G., Ika, D., and Thomas, D. (2021). Functional response of an Austrian forest soil to N addition. Environ. Res. 3:025001. doi: 10.1088/2515-7620/abe8c7
Sokol, N. W., Slessarev, E., Marschmann, G. L., Nicolas, A., Blazewicz, S. J., Brodie, E. L., et al. (2022). Life and death in the soil microbiome: how ecological processes influence biogeochemistry. Nat. Rev. Microbiol. 20, 415–430. doi: 10.1038/s41579-022-00695-z
Tao, B. X., and Song, C. C. (2014). Temperature sensitivity of carbon dioxide production in aggregates and their responses to nitrogen addition in a freshwater marsh, Sanjiang Plain. Soil Sci Plant Nutr. 59, 953–960. doi: 10.1080/00380768.2013.857579
Tobiašová, E., Barančíková, G., Gömöryová, E., Makovnikova, J., Skalsky, R., Halas, J., et al. (2016). Labile forms of carbon and soil aggregates. Soil Water Res. 11, 259–266. doi: 10.17221/182/2015-SWR
Treseder, K. K. (2008). Nitrogen additions and microbial biomass: a meta-analysis of ecosystem studies. Ecol. Lett. 10, 1111–1120. doi: 10.1111/j.14610248.2008.01230.x
Wang, B. R., An, S. S., Liang, C., Liu, Y., and Kuzyakov, Y. (2021). Microbial necromass as the source of soil organic carbon in global ecosystems. Soil Boil Biochem. 162:108422. doi: 10.1016/j.soilbio.2021.108422
Wang, J., Liao, L., Ye, Z. C., Liu, H. F., Zhang, C., Zhang, L., et al. (2022). Different bacterial co-occurrence patterns and community assembly between rhizosphere and bulk soils under N addition in the plant–soil system. Plant Soil 471, 697–713. doi: 10.1007/s11104-021-05214-2
Wang, Q. K., Wang, S. L., He, T. X., Liu, L., and Wu, J. B. (2014). Response of organic carbon mineralization and microbial community to leaf litter and nutrient additions in subtropical forest soils. Soil Boil Biochem. 71, 13–20. doi: 10.1016/j.soilbio.2014.01.004
Wang, Q., Zhang, W., Sun, T., Chen, L. C., Pang, X. Y., Wang, Y. P., et al. (2017). N and P fertilization reduced soil autotrophic and heterotrophic respiration in a young Cunninghamia lanceolata forest. Agric. For. Meteorol. 232, 66–73. doi: 10.1016/j.agrformet.2016.08.007
Wang, Q., and Zhong, M. (2016). Composition and mineralization of soil organic carbon pools in four single-tree species forest soils. J. For. Res. 27, 1277–1285. doi: 10.1007/s11676-016-0244-z
Wei, X. R., Ma, T., Wang, Y. H., Wei, Y. C., Hao, M. D., Shao, M. G., et al. (2016). Long-term fertilization increases the temperature sensitivity of OC mineralization in soil aggregates of a highland agroecosystem. Geoderma 272, 1–9. doi: 10.1016/j.geoderma.2016.02.027
Wei, X., Zhu, Z., Liu, Y., Luo, Y., Deng, Y. W., Xu, X. L., et al. (2020). C: N: P stoichiometry regulates soil organic carbon mineralization and concomitant shifts in microbial community composition in paddy soil. Biol. Fertil. Soils 56, 1093–1107. doi: 10.1007/s00374-020-01468-7
Xu, P. D., Zhu, J., Wang, H., Shi, L., Zhuang, Y., Fu, Q. L., et al. (2021). Regulation of soil aggregate size under different fertilizations on dissolved organic matter, cellobiose hydrolyzing microbial community and their roles in organic matter mineralization Sci. Total Environ. 755:142595. doi: 10.1016/j.scitotenv.2020.142595
Xue, J. M., Sands, R., Clinton, P. W., Payn, T. W., and Skinner, M. F. (2005). Priming effect of biuret addition on native soil N mineralisation under laboratory conditions. Soil Boil Biochem. 37, 1959–1961. doi: 10.1016/j.soilbio.2005.02.034
Yang, Y., Li, T., Pokharel, P., Liu, L. X., Qiao, J. B., Wang, Y. Q., et al. (2022). Global effects on soil respiration and its temperature sensitivity depend on nitrogen addition rate. Soil Boil Biochem 174:108814. doi: 10.1016/j.soilbio.2022.108814
Yang, K., and Zhu, J. J. (2015). The effects of N and P additions on soil microbial properties in paired stands of temperate secondary forests and adjacent larch plantations in Northeast China. Soil Boil Biochem. 90, 80–86. doi: 10.1016/j.soilbio.2015.08.002
Yudina, A. V., Klyueva, V. V., Romanenko, K. A., and Dokuchaev, D. S. F. V. V. (2022). Micro- within macro: how micro-aggregation shapes the soil pore space and water-stability. Geoderma 415:115771. doi: 10.1016/j.geoderma.2022.115771
Zaman, M., and Chang, S. X. (2004). Substrate type, temperature, and moisture content affect gross and net N mineralization and nitrification rates in agroforestry systems. Biol. Fertil. Soils 39, 269–279. doi: 10.1007/s00374-003-0716-0
Zhou, J. C., Liu, X. F., Xie, J. S., Lyu, M. K., Zheng, Y., You, Z. T., et al., (2019). Nitrogen Addition Affects Soil Respiration Primarily through Changes in Microbial Community Structure and Biomass in a Subtropical Natural Forest. Forests 10:435. doi: 10.3390/f10050435.
Zhang, Y., and Shangguan, Z. P. (2022). Long-term N addition accelerated organic carbon mineralization in aggregates by shifting microbial community composition. Agric. Ecosyst. Environ. 342:108249. doi: 10.1016/j.agee.2022.108249
Zhang, N., Wan, S., Li, L., Bi, J., Zhao, M. M., and Ma, K. P. (2008). Impacts of urea N addition on soil microbial community in a semi-arid temperate steppe in northern China. Plant Soil 311, 19–28. doi: 10.1007/s11104-008-9650-0
Keywords: aggregates, microbial biomass, mineralization, nitrogen addition, soil organic carbon, total nitrogen
Citation: Chen T, Cheng R, Xiao W, Zeng L, Shen Y, Wang L, Sun P, Zhang M and Li J (2024) Nitrogen addition enhances nitrogen but not carbon mineralization in aggregate size fractions of soils in a Pinus massonia plantation. Front. For. Glob. Change. 7:1240577. doi: 10.3389/ffgc.2024.1240577
Edited by:
Rudong Zhao, Chinese Academy of Sciences, ChinaReviewed by:
Jörg Luster, Snow and Landscape Research (WSL), SwitzerlandZheng Wang, Hebei Agricultural University, China
Copyright © 2024 Chen, Cheng, Xiao, Zeng, Shen, Wang, Sun, Zhang and Li. This is an open-access article distributed under the terms of the Creative Commons Attribution License (CC BY). The use, distribution or reproduction in other forums is permitted, provided the original author(s) and the copyright owner(s) are credited and that the original publication in this journal is cited, in accordance with accepted academic practice. No use, distribution or reproduction is permitted which does not comply with these terms.
*Correspondence: Ruimei Cheng, cafcheng@sina.com