Testing of human reactions during braking manoeuvres combining a physical test bed with virtual reality
- 1Game Laboratory Graz, Institute of Interactive Systems and Data Science, Graz University of Technology, Graz, Austria
- 2Vehicle Safety Institute, Graz University of Technology, Graz, Austria
For the development of restraint systems, the initial posture and kinematics of the occupants immediately before the crash are essential. To predict the behaviour of vehicle occupants in pre-crash manoeuvres, volunteer tests under controlled boundary conditions to calibrate and validate active human body models are needed. The challenge in such experiments is to evoke a natural behaviour of the volunteers. The aim of this study was to investigate the potential of distractions via virtual reality (VR) in volunteer tests to study pre-crash kinematics. For this purpose, it was investigated if the volunteers are able to get immersed into the virtual reality using head-mounted displays to an extent in which they forget the laboratory environment and feel as if they are really sitting in an autonomous vehicle. A total of 27 volunteers took part in the study, and 10 different trials were carried out with each of them. In the baseline trial, no VR was displayed and the volunteers were made aware that the sled is going to start moving by a countdown. In trials 3–10, the volunteers were instructed to perform various tasks in virtual reality, which we have developed for this specific purpose. The physical platform on which they were sitting on began to move mimicking a braking maneuver as they performed a task. The peak forward head excursions resulting from the different trials were compared and showed significant differences between the baseline trial and the trials where they were distracted with VR. In contrast to our expectations, peak forward head excursions were overall significantly lower for the volunteers distracted by VR compared to the baseline trial. However, volunteers, who had significantly lower peak forward head excursions in the baseline trials showed higher peak forward head excursions in the VR trials comparable in magnitude to the other volunteers. All the volunteers answered in the questionnaire that their experience had been good and they were willing to participate in the study again. Further research is needed to understand the effect of the performed activities on the volunteer muscle activation and head excursions.
1 Introduction
While autonomous vehicles have the potential to improve road safety by avoiding human errors, traffic accidents are still expected to occur. Since sensor information is already available before the crash, integrated safety measures will play an increasing role. As safety systems react already prior to the crash, it is important to be able to predict the kinematic behavior of the vehicle occupants before the actual crash.
This cannot be predicted with the current crash test dummies, as they are designed for much higher accelerations during the crash and do not have muscles included. Active human body models (HBMs) are therefore playing an increasingly important role in vehicle safety (Östh et al., 2013; Iwamoto et al., 2015; Öst et al., 2015; Erlinger et al., 2022). These are numerical finite element models of the human body equipped with active muscle models, which can be used to perform simulations of crashes in order to evaluate and improve safety concepts (e.g., new restraint systems) (Saito et al., 2016; Yamada et al., 2016; Östmann and Jakobsson, 2016). Experiments with volunteers are essential in order to be capable of calibrating and validating active HBMs and to obtain a better understanding of human behavior in the pre-crash phase. Kinematics and muscle activity are thereby examined in experiments in full vehicles (Behr et al., 2010; Olafsdottir et al., 2013; Östh et al., 2013; Huber et al., 2014, 2015; Kirschbichler et al., 2014; Reed et al., 2020, 2019; Fice et al., 2019; Ghaffari et al., 2019) or on simplified test beds in laboratories (Ejima et al., 2009; Beeman et al., 2011; Carlsson and Davidsson, 2011; Kirschbichler et al., 2011; Ito et al., 2013; Sandoz et al., 2019; Chan et al., 2021; Erlinger et al., 2022). Tests in laboratory environments have the advantage of better controllability and the possibility to add more measurements than in full vehicle tests (e.g., interaction forces and full body visibility). However, the behaviour of the volunteers is influenced by the laboratory environment. It is difficult for them to relax, which causes a higher spread of responses and also braced behavior. Tests in cars, might lead to more realistic behaviour of the volunteers, but on the other hand, are more complex, and are more challenging in terms of repeatably, measurement possibilities and visibility.
In the course of the past decade, the use of virtual reality (VR) has spread to many different purposes and in a wide range of fields (Potkonjak et al., 2016), such as education (Dede, 2009), health (Kim and Rizzo, 2005), and business (Muller Queiroz et al., 2018), in addition to it is main application as an entertainment medium. VR has proved to be particularly useful because of its ability to simulate situations that could be dangerous or life-threatening in the real world (Bailenson et al., 2008). For these reasons, it provides various opportunities for training, practice or experience that might be unthinkable, risky, or costly in the physical world (Bailenson, 2018). Such applications are for example the use of VR for driving simulators combined with hardware (e.g. gas pedals or steering wheels) to help people with spinal cord injuries learn to drive (Tudor et al., 2015), study advanced driver assisting functions (Brossmann et al., 2018; Nascimento et al., 2019) or improve human machine interaction (Lagoo et al., 2019). VR can be also used to distract persons from their current real situation. This is used for example in pain management (Lambert et al., 2020; Ohlrogge and Glaß, 2020).
The objective of the current study is to find a way to merge the more natural behaviour of volunteers when sitting in a featured autonomous vehicle with the advantages of volunteer tests performed on a test bed in a laboratory environment. The idea was that by using virtual reality, the volunteers should forget that they sit in a laboratory environment and feel as they actually sit in an autonomous vehicle. They were to be distracted by the VR and show a natural, surprising reaction when subjected to an emergency braking manoeuvre on a test bed. The aim was to compare peak forward head excursions of the volunteers exposed to different distraction tasks within the VR while being subjected to a braking acceleration. It was aimed to investigate the usefulness of VR distraction for volunteer studies and provide recommendations future study designs.
In this study, we aimed to answer the following research questions:
• Is there a significant effect of VR distraction on head excursions in volunteer tests mimicking braking manoeuvres?
• Is there a significant deviation of peak forward head excursion between different VR scenarios?
• Is the use of VR beneficial for volunteer tests, in which kinematics resulting from pre-crash related acceleration pulses are studied?
2 Methods
A volunteer study was performed to evaluate the effect of different VR scenarios on the response shown by these people during a pre-crash braking manoeuvre in a simplified lab environment. A head-mounted display was used to draw the attention of the volunteers to different tasks. While they were performing the task, the platform on which they were seated, began to move, mimicking a conventional braking manoeuvre. Peak forward head excursions from tests with different distractions in the VR were compared to excursions of the baseline tests, in which no VR was displayed. Ten different trials were performed with each volunteer in which five different scenarios were tested (four VR and one baseline scenario).
2.1 Virtual reality implementation
2.1.1 Development environment
The virtual environment was developed using Unreal Engine 4.24. This game engine provides a realistic rendering environment, a collection of predefined and free to use assets, a simplified VR example, and multiplatform deployment of the project. The game logic in the Unreal Engine can be defined using the C++ programming language and the “Blueprint” visual scripting tool. The simplicity of visual scripting allows even non-programmers in the team to extend the development of the projects with ease. Therefore, we decided to use Blueprint for the implementation of the game logic.
2.1.2 Setup
The second generation of Oculus Quest was used in this study, which has been released with several improvements in processing power, screen resolution, and interaction system. This results in a lightweight, portable and affordable VR device that can be used by general users. In this study, we used Oculus Quest 2 as a head-mounted display (HMD). The cameras on this HMD can track the position of the device and the controller (or hand in the case of hand tracking). This means that no additional light sensors are needed, as they are on other HMDs like HTC Vive or Valve Index. In general, there are three ways to run VR applications on the Oculus Quest 2: 1. create a standalone “apk” file 2. connect the device to a computer via cable 3. connect the device to a computer via AirLink (wireless connection). The first option provided the ability to run the application without connecting to the PC, but is limited by the Oculus Quest 2 processor. The second option could run the VR application on the HMD with the full calculation power of the computer, but limits the user with the wired connection. The third option had the advantages of the second option, but was limited by the speed and stability of the wireless connection. For this study, we chose the second option to achieve more reliable experience with full calculation power. A long cable ensured that the volunteers were not limited by the wired connection.
Environment design and interactions: As shown in Figure 1, the voluteers were virtually placed in an autonomous vehicle with a 360° view. The seats were based on a freely available Unreal Engine template Automotive Configurator sample. The volunteer interacted through the virtual environment with the help of virtual hands, which are controlled with Oculus Quest 2 controllers.
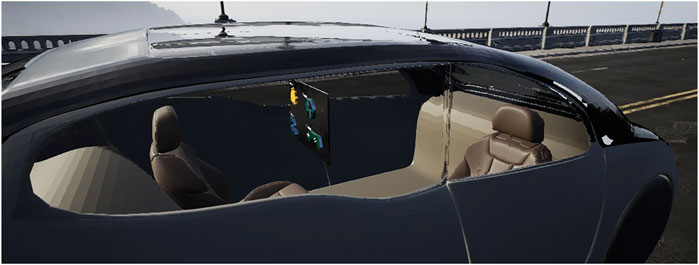
FIGURE 1. The VR Scenario–an autonomous vehicle with display for interaction shown on the HMD to the volunteers while they were seated on the test bed.
The main user interface in this virtual environment is the menu where the icons Weather, News, Phone and Game are shown as holograms in the player view (Figure 2). These icons are touchable and are activated when they are touched with the forefinger of the virtual hand. The selection of an icons started an animation that moved and shrinked the main menu. At the same time, the selected application started and was visualized. Each application could be stopped again by clicking the red “X” in the upper left or right corner. The “Weather” icon lead to an interface with an selection for two cities (Graz and Vienna). On touching one of the city names, the weather description and temperature appeared. When the “News” icon was touched, the volunteers could read four different made up news headlines and articles after selecting one of the headlines. Touching the “Phone” icon called a user interface displaying a numeric keypad. A number could be entered and modified. Interaction with the “Game” icon opened a TicTacToe game. It was possible to play the game as well as to restart a game after all fields have been filled in with “X” or “O”. Visualizations of all scenarios can be found in the Supplemental Material.
2.2 Test bed
The volunteers were seated on the in-house developed “LowG” test bed, shown in Figure 3, which was designed to physically mimic a braking manoeuvre in volunteer tests. The test bed consists of a guided sled with an adjustable seat and offers several redundant safety measures for a high safety standard during volunteer tests. To ensure the safety of the volunteers, a comprehensive safety concept based on a FMEA (failure mode and effect analysis) was integrated and strict checklists were followed when executing the tests. The LowG sled is driven with a controlled electric motor, capable of precisely following highly dynamic acceleration profiles up to 1 g. The maximum travel distance of the sled is 4 m with a peak velocity of 4.3 m/s. In the conducted tests within this study, a sine-shaped pulse with a maximum acceleration of 0.5 g was used, mimicking the medium emergency braking of a car (Ito et al., 2013; Graci et al., 2021). A conventional 3-point seat belt was used in combination with a generic padded seat and the volunteers were seated in an upright position.
2.3 Volunteers
Twenty-seven (13 male and 14 female) volunteers participated in the study. Volunteers were not selected based on specific anthropometry measurements. For recruiting of volunteers, university staff and students were informed verbally about the study and asked to participate in experiments simulating a vehicle braking manoeuvre under simplified conditions using a HMD. Self-disclosures of rheumatic diseases, changes of the spine caused by accidents, or other injuries, acute and chronic spinal complaints of any other kind, acute neurological or psychiatric diseases would have led to exclusion. The volunteers received no remuneration for their participation.
In Table 1, the age and experiences (in gaiming, VR and vehicle safety research) of the volunteers who participated in the study are shown. 77% of the volunteers reported to have experience in computer gaming and 40% with VR. The majority (59%) also had some background in the field of vehicle safety. One male volunteer was excluded from further analyses due to outlying behaviour (much higher head excursions than other participants), which might have been caused by too much knowledge about the study, as he was involved in some discussions on the study design.
2.4 Procedure
The experimental procedure was evaluated by the Ethics Committee of the Medical University of Graz and a positive ethics vote was issued (33–552 ex 20/21). As the first step, the volunteers were informed of the possible risks, the procedure and the exclusion criteria. Volunteers who agreed to participate, signed a consent form and were informed that they could end their participation at any time without specifying a reason. The genders and ages of all the volunteers were documented. Following these formalities, the volunteers were then asked to sit on the generic seat in the position comfortable for them and belted with the 3-point belt. The seatback angle, seatbelt d-ring position or foot test was not adjusted between volunteers, prescribing the initial posture to some extent. As the next step, the volunteers were instructed on how to use the HMD and how to stop using it. Furthermore, they were informed that they could ask for assistance at any time during the experiment. They were equipped with headphones and heard easy-listening music (rhythm, blues and jazz). The test matrix executed for each volunteer is shown in Table 2. The same sequence was applied to all participants, in order to avoid mixing up different factors. As it was shown in previous studies (Erlinger et al., 2022), that peak forward head excursion may be highly subject-specific. The sled on which the seat was mounted, was then accelerated and decelerated via the sinusoidal acceleration pulse (shown in red in Figure 4). The sled movement took place in a purely longitudinal direction (in backward direction from the volunteer’s point of view). The duration of one pulse was in total 2 seconds, whereby the first second caused the head excursion by the acceleration and the second second was only needed to decelerate the sled and come to a halt. For trials T01 and T02, the volunteers saw the real environment not the VR via the HMD. In trial 1, it was intended to make the volunteers fully aware of what was about to happen and with this in mind also an acoustical countdown was presented, indicating when the acceleration onset. This trial was named as the “baseline test”, meaning that the participants were not distracted in this trial and our hypothesis was therefore that they would have the lowest head excursions as they might have been able to pre-tense their muscles. In the trials 3–10 volunteers were instructed to perform one of the four activities of the VR environment and complete a certain task in each trial. Except for the first trial, the volunteers were not aware of the exact onset of the acceleration pulse. The triggering times, when the sled starts to move after the volunteers initiated the task, were varied according to the test matrix. Each VR scenario was tested twice in order to investigate learning and acclimation effects. After completing all trials, the volunteers were asked to fill in a questionnaire in paper form.
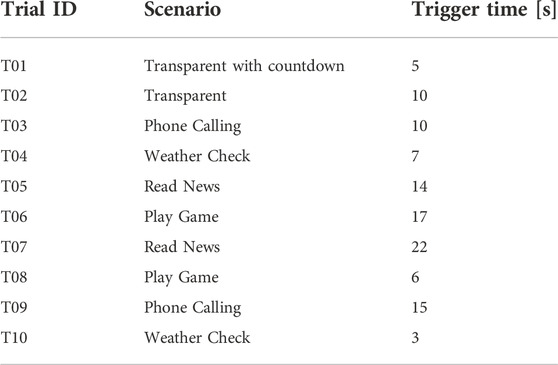
TABLE 2. Test matrix, showing the 10 trials differing in terms of the VR task and the trigger time which were performed by each volunteer.
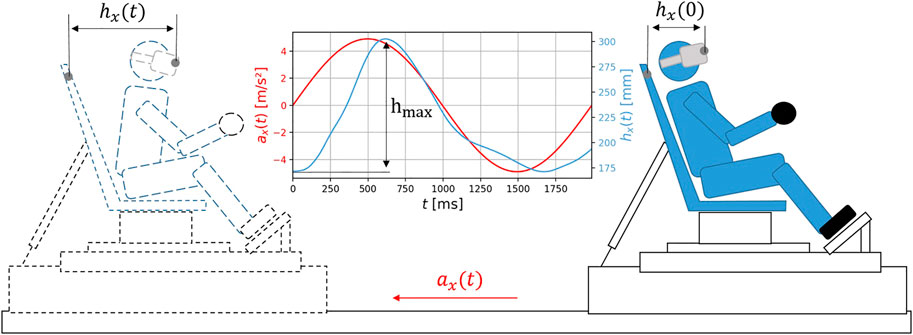
FIGURE 4. Schematic representation of the simplified braking manoeuvre on the test bed. The sled acceleration pulse ax(t) with a peak of 0.5 g is represented in red, the volunteer’s head excursion hx(t) in blue. hmax is the difference of hx (0) and the absolute maximum
2.5 Data collection
From the experiments, the head motion and the answers in the questionnaire were analysed to compare the volunteers and different trials.
2.5.1 Head motion
Three-dimensional motion of the volunteer head and the seat was captured using a Qualisys motion-tracking system with ten infrared cameras (Miqus, 6 x M3 and 4 x M5 and a sampling frequency of 100 Hz) and retroreflective markers attached to the HMD. Qualisys Track Manager (QTM) software was used to post-process the kinematic data, with defined rigid bodies for the seat and the HMD.
2.5.2 Questionnaire
The aim of the questionnaires was to evaluate the immersion and physical demand of the VR tasks. Furthermore, we aimed to find out when the volunteers were most distracted and which VR task they have enjoyed the most. We also asked how the volunteers were feeling after the test and whether they would be willing to participate in a similar study again. They were also encouraged to provide feedback on further improvements and bring in their own ideas.
The questionnaire was structured into four parts:
• Demographic questions
• Igroup Presence Questionnaire (IPQ): The IPQ has three different presence components. These components are used to analyze the presence in VR. The first one is the general “Sense of being there”, the second one is the “Spatial Presence”, the third one is “Involvement and Experienced Realism” (Regenbrecht et al., 2016).
• NASA Task Load Index (TLX) is a type of questionnaire that is used to measure the mental, physical and temporal demand as well performance, effort, and frustration during the test (Hart, 1986).
• Study-specific questions designed by the authors of this study
The full questionnaire can be found in the Supplemental Material.
2.6 Data processing
The maximum head excursion was used as a parameter to quantify the reaction of the volunteers in the different trials. The 3D head kinematics
The data was processed using Python and the Python libaries Scipy.Stats, Pingouin, Pandas and Matplotlib. To test for significant differences between the maximum head excursions of different trials, a repeated measures analysis of variance (ANOVA) with the function rm_anova was conducted. ANOVA is commonly used for comparison of multiple observations obtained from the same volunteer. Since statistical tests showed that the sphericity and normality assumptions for repeated measures ANOVA are violated a square root transformation was applied to the dependent variable hmax. The unique distractions of trials T01 to T06 were chosen, to be excluded, as levels of the within-subject factor. To gain further insights, multiple pairwise paired t-tests were performed and the p-values were then adjusted using the Bonferroni correction method.
3 Results
3.1 Peak head excursiont
Figure 5 shows the peak forward head excursion hmax in boxplots for each trial over all volunteers. The repeated measures ANOVA test showed with a p-value less than 0.001 that the null hypothesis of equal means of the maximum head excursion hmax was rejected. The pairwise statistical analysis of the effect of the different scenarios is shown in Table 3. The pairwise t-test showed significant (p ≤ 0.05) differences between the baseline trial T01 (“transparent with countdown”) and T04 (“weather check”), T01 and T05 (“read news”) and T01 and T06 (“play game”). All other pairwise comparisons did not show significant differences.
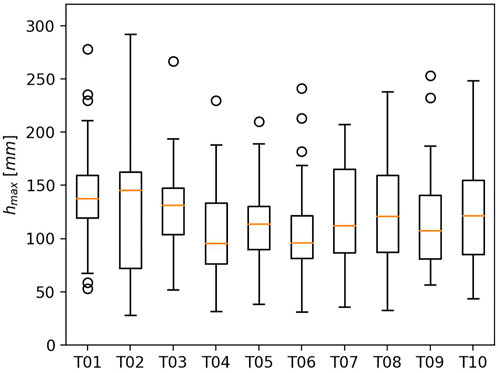
FIGURE 5. Peak forward head excursion hmax in boxplots. The box shows the inter-quartile range (IQR), with the whiskers being at 1.5 x IQR. Outliers are represented by circles and the median by the orange line.
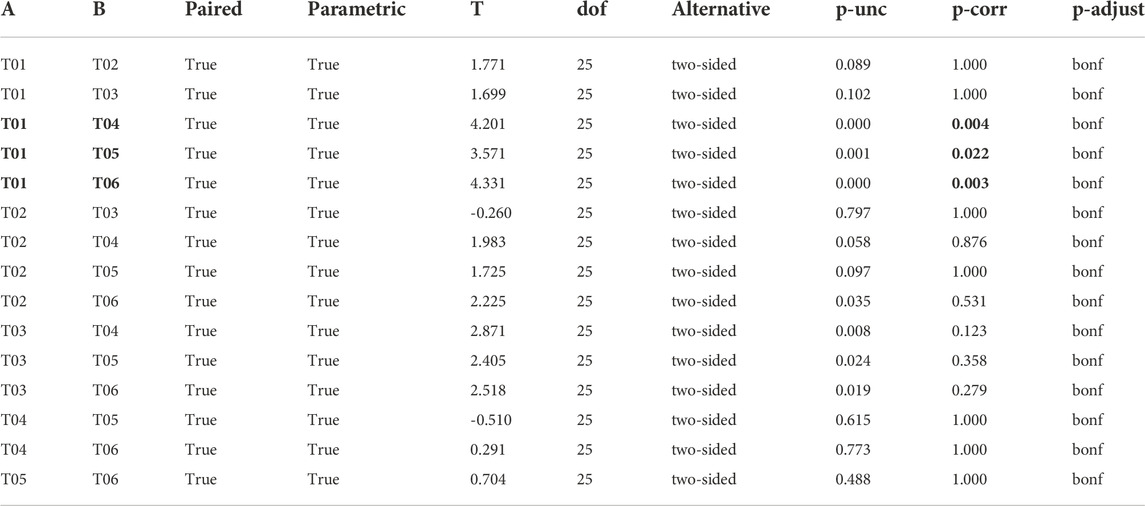
TABLE 3. Results of the pairwise t-tests of the peak forward head excursion of the different trials. Significant differences between peak head excursions of the different trials are shown in bold.
Furthermore, the data was analysed in more detail to determine whether the volunteers could be classified into groups. Therefore, we have compared hmax in the first trial with hmax over all trials. It was observed that for those four volunteers where hmax in the baseline trial was lower than 100 mm, head excursions increased in the VR trials. This difference in these volunteer groups for the different trials is shown in Figure 6. In the VR scenarios, head excursions for these volunteers were in the range of the other volunteer. For T03 (“phone calling”) and T08 (“play game”), highest head excursions were observed for theses originally “tensed” volunteers. For the other group of volunteers, the mean hmax values were very similar over all trials with high scatter in results.
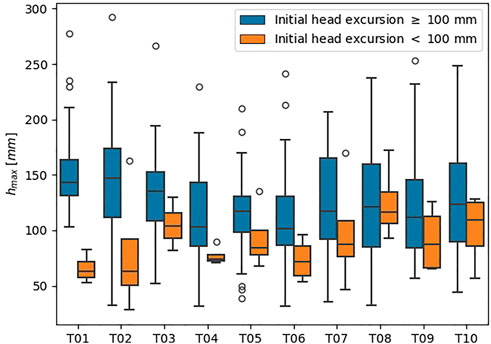
FIGURE 6. Boxplots for each trial (T01-T10) for two groups of volunteers based on hmax in the baseline trial. The box shows the inter-quartile range (IQR), with the whiskers being at 1.5 x IQR. Outliers are represented by circles and the median by the horizontal line.
In addition, we took into account whether or not the volunteers were already experienced with VR. Both classifications are shown in Figure 7. In the highlighted box, the response of the volunteers with a maximum head excursion in T01 smaller than 100 mm is shown. The colours indicate the different trials and filled symbols represent volunteers with previous VR experience while unfilled symbols represent volunteers who were exposed to VR for the first time. No significant effects have been observed.
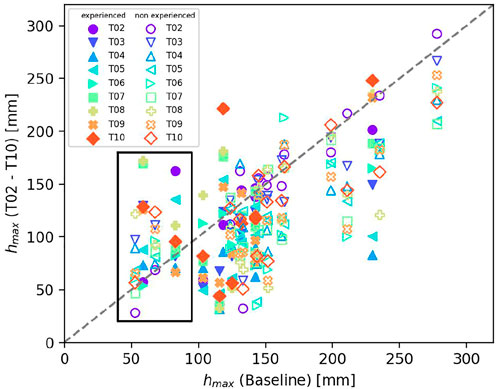
FIGURE 7. Comparison of the maximum peak forward head excursion hmax of the baseline trial and the trials 02 to 10. Filled symbols mark volunteers with prior VR experience, unfilled, those without. The black frame indicates the group of “tensed” participants, who had a peak forward head excursion hmax < 100 [mm] in the baseline trial.On the dashed grey line, head excursions are equal to the baseline and above they were higher.
3.2 Results from questionnaires
In Figure 8, the results of the IPQ questionnaire and in Figure 9 the results of the NASA TLX are plotted. The values for volunteers with previous experience with VR are colored gray and volunteers without previous experience blue. As the scatter among volunteers was very high, they were further subdivided into those with and those without VR experience. In Table 4 the mean value, standard deviation and p-values when comparing experienced and non-experienced volunteers are summarisied for both questionnaires. The mean values for general presence, involvement, and realism are all higher for a participant without experience in VR, but differences are not significant. For the spatial presence, the results of both groups are almost the same. For the Nasa TLX questionnaires, the mean and maximum values for all tasks except the temporal demand are higher for non-experienced than the participants that have already experience with VR. Significant differences were observed for the effort, which was lower for those with VR experience.
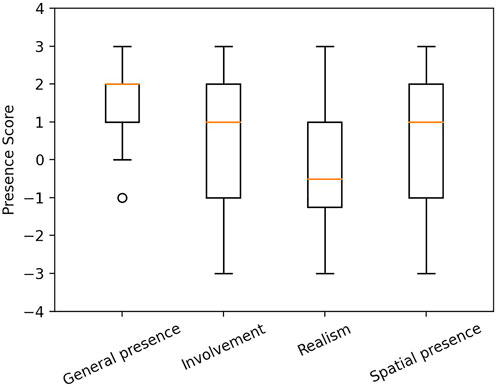
FIGURE 8. Boxplots of the four IPQ subgroups, as answered by the volunteers. The score indicates the sense of presence experienced in a virtual environment (-4 not being present to 4 being fully present). The box shows the inter-quartile range (IQR), with the whiskers being at 1.5 x IQR. Outliers are represented by circles, the median score by the orange line.
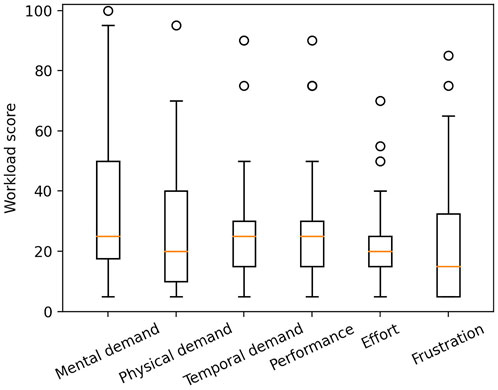
FIGURE 9. Boxplots of the NASA-TLX questionnaire, as answered by the volunteers. The score rates the perceived workload of individual tasks (0 being of very low demand, 100 being of high demand). The box shows the inter-quartile range (IQR), with the whiskers being at 1.5 x IQR. Outliers are represented by circles, the median score by the orange line.
Most of the volunteers (N = 20) stated to feel happy after the tests, five volunteers were surprised, one felt sick and one was happy and surprised. In Figure 10, the subjective evaluation of the volunteers on the different tasks within the VR environment is shown. The majority of volunteers liked the scenario “play game” most, but indicated that they were most surprised about the acceleration onset during the “phone calling” scenarios.
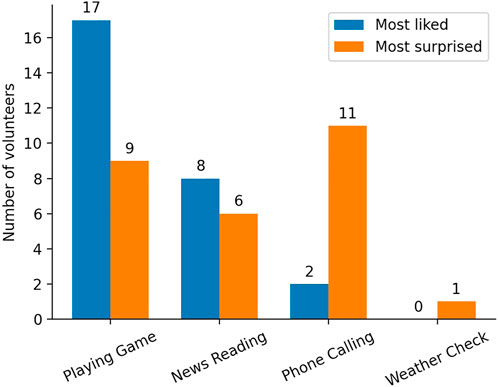
FIGURE 10. Subjective assessment of the four different scenarios. Those indicated by the volunteers as “most liked” scenarios are represented as blue bars, those where they indicated that they were “most surprising” in orange.
The average duration of the experiment was 17 min. One volunteer estimated the time spent in virtual reality to be less than 5 min, seven estimated it to be less than 10 min, and 19 volunteers felt they spent between 10 and 20 min in VR.
4 Discussion
Large test-scatter, make it generally difficult to interpret the observed results. We have tried to identify post-hoc groups based on their peak forward head excursion of the baseline trial, which was a help for understanding some of the pattern differences. In future studies this hypothesis should be further investigated by measuring muscle activity to facilitate the identification of pre-tensed volunteers.
It appeared that the group of volunteers that was initially showing low head excursions (hmax below 100 mm) might have been initially tensed. They seemed to become more relaxed in subsequent trial, probably caused by the distraction with VR. The mean peak forward excursions had the highest magnitude for volunteers in trials T03 (“phone calling”, chronologically the first trial) and T08 (“play game”, chronologically the second trial).This indicates, that the distraction “play game” showed more effect in the second trial, when the movement of the test bed started earlier. Therefore, in future studies, longer-lasting tasks should be developed to avoid having the volunteers finalize the task already, while waiting for the sled to start moving.
It seems that we managed to relax the group of volunteers that were very tensed (hmax below 100 mm) in the baseline by distracting them with VR. Mean values were highest for these volunteers in T03 and T08, which were the scenarios “phone calling” (and also the very first VR trial) and “play game” (in the second trial series). This indicates, that the distraction “play game” worked better in the second trial, when the triggering time was shorter and therefore the movement of the test bed started earlier. This indicates, that in the future, longer-lasting tasks should be developed, to avoid that volunteers have finalized the task already, waiting for the sled to start moving.
VR showed the potential in general to relax the volunteers, which was indicated by the maximum head excursions and their feedback. This was not possible in a previous studies (Erlinger et al., 2022), where the initial groups of volunteers remained significantly different throughout the test series and three out of six volunteers consistently showed head excursions below 100 mm. The two studies are not directly comparable, because both the setup and pulse differed.
The volunteers stated in the questionnaires that they had fun and liked the tests. All of them indicated that they would be happy to participate again. The use of VR thus appears to be beneficial for future volunteer tests. However, the distraction of the volunteers needs to be better controlled and the scenarios need to be further enhanced to make sure that the volunteers are immersed at time of acceleration onset.
Eight volunteers had the highest peak forward head excursion of all trials in the baseline trial (chronologically the first trial). This could be caused by muscle tension when performing one the predefined tasks and should be investigated in more detail in future studies. When riding in a car it is very likely that a task is performed just before the crash occurs (interacting with the car display to change the music, call someone) and this might be of even higher relevance in autonomous vehicles. As the initial posture and the current activity of the occupant could be monitored by in-vehicle cameras, this information might be relevant and available for restraint system algorithms.
Taking a look at the mean values in the Nasa TLX questionnaire result shows that the virtual experience for the participant with no prior VR experiences is more mentally and physically demanding. They need more effort and performance to complete the task. This difference can affect the user experience in VR. Designing an onboarding session before the actual trial can help reduce this difference between volunteers with and without prior VR experience. However, it should be noted that the onboarding session should not interfere with the surprise effect of the main experience. In this way, the design of the onboarding session should include some interaction elements of the main experience, but differ in story and events. Another aspect that can be considered when designing the onboarding level is the duration of this learning session. The impact of this factor on the user experience and the study results can define appropriate design decisions for an onboarding environment. But in general when considering the scale ranges for NASA TLX (Nikulin et al., 2019), the effort for participants with no prior VR experience was low, and very low for the experienced. This means that the tasks were relatively easy, also for those without experience. On the other hand, future studies may also investigate whether these relatively low mental and physical demands are influenced by task difficulty. Accordingly, studies on different task demands with more interactions and difficulty levels may extend our current results.
Looking at the result of the IPQ, there was no statistically significant difference between volunteers with and without prior VR experience. There are two possibilities for this: either the environment provided enough presence that was accepted by both groups, or vice versa. When we analysed the “general presence”, “involvement” and “spatial presence” factors of the IPQ, the first idea emerged as the more dominant one. However, the factor “realism” showed an opposite result (which is consistent with our simplified design approach). Therefore, we can assume that further studies with a more realistic environmental design (aimed at probably increasing the realism factor) can help us investigate and compare the presence in both groups. In addition, we can use the IPQ first after instruction and then after actual experience to investigate the influence of braking manoeuvres on the reported sense of presence.
Most of the participants (N = 20) did not identify any irritating items during their VR experience. Seven participants reported difficulty with the hand control in VR and they preferred to appropriate the learning environment before the main experience. This issue is more crucial for the new user. As future work, this study can be extended by adding an onboarding environment before the main experience. The other reported issue was related to a visual representation of the 3D model, specific improvement in rendering of materials. It can be done by enhancing the 3D model with reading materials in future works. As we observed from the open questionnaire form at the end of the survey, participants preferred the gaming activity (Figure 10) more than others. This can be interpreted as the volunteers enjoying the environment more when further entertaining and immersive activities are added. The participants agreed that more complex tasks, where more attention is needed, might improve their engagement. As all participants indicated in the questionnaire that they would be willing to participate in the study again, it appeared clear that they had a good time, a factor which has already emerged as very important for tests with volunteers. The good user experience is therefore another argument for using VR in future similar experiments.
5 Limitation and future work
The experiments performed within this study had the purpose aim to investigate the effect of VR distraction on the peak forward head excursions of the participating volunteers. The recorded data is not sufficient for the calibration or validation of active HBMs, as only head excursions were recorded. The study should be understood as feasibility study and guide future experimental protocol developments.
It is unclear, why in some trials, the head excursion was even higher when volunteers performed the tasks than in the baseline. No EMG measurement was performed in the current tests, which would have shown if the volunteers have tensed muscles when performing the tasks. This should be investigated in future studies.
The number of volunteers with initially low head excursions in the baseline trial was very low (n = 4 with a head excursion
The minimum age of the volunteers was 21 and the maximum 48. No age-dependency was therefore investigated in the current study and it is unclear whether elderly volunteers would cope with the VR interactions and the HMD.
The volunteers indicated in their feedback, that the tasks were relatively easy and could be more complex and longer in the future.
Only one acceleration profile and one loading direction were investigated in the current study. The applied low-severity acceleration profile, however, is most challenging in terms of reproducible volunteer behavior (the higher the loads, the less controllable it gets for the volunteers). Additional loading directions and acceleration profiles should be tested in the future. Furthermore, in the future different sitting positions should be investigated to analyze this effect on pre-crash behaviour.
6 Conclusion
By combining VR and an acceleration test bed in a laboratory environment, we have attempted to bridge the gap between highly controlled boundary conditions and realistic volunteer behavior. A significant difference between different scenarios and of VR distraction compared to the baseline was observed leading to lower head excursions for the majority of volunteers, while increasing head excursions for initially tensed volunteers (peak head excursions below 100 mm in baseline trial).
The use of VR with HMD displays was shown to be beneficial when conducting volunteer tests in a laboratory environment to investigate human behaviour in pre-crash maneuvers. Tensed volunteers were able to relax by getting distracted with the VR. All the volunteers had a joyful experience, indicating that they would be happy to participate again.
Further research is needed to make the tests and the volunteer reaction more repeatable and to understand the reason for the reduced head excursions and muscle activation patterns in dependency on the individual task they are performing in the VR during braking maneuvers.
Data availability statement
The raw data supporting the conclusions of this article will be made available by the authors, without undue reservation.
Ethics statement
The studies involving human participants were reviewed and approved by Ethics Committee of the Medical University of Graz. The patients/participants provided their written informed consent to participate in this study.
Author contributions
SR has developed the VR environment supervised by JP and SS. MS processed the recorded data and developed the included visualisations. BS performed the statistical analyses. GG and DK supervised the volunteer study and executed the tests. NE supported the writing of the manuscript. CK designed the study and wrote the initial draft manuscript. All authors critically reviewed and revised the manuscript.
Funding
Open access funding provided by Graz University of Technology Open Access Publishing Fund.
Acknowledgments
The LowG testbed was developed by Philipp Rothmaier, Stefan Grollitsch and Christian Ellersdorfer.
Conflict of interest
The authors declare that the research was conducted in the absence of any commercial or financial relationships that could be construed as a potential conflict of interest.
Publisher’s note
All claims expressed in this article are solely those of the authors and do not necessarily represent those of their affiliated organizations, or those of the publisher, the editors and the reviewers. Any product that may be evaluated in this article, or claim that may be made by its manufacturer, is not guaranteed or endorsed by the publisher.
Supplementary material
The Supplementary Material for this article can be found online at: https://www.frontiersin.org/articles/10.3389/ffutr.2022.991666/full#supplementary-material
Data Sheet 1.PDF | Questionnaire used in the study.
Image 1.png | Phone calling scenario.
Image 2.png | Wetaher check scenario.
Image 3.png | Play game scenario.
Image 4.png | Read news scenario.
References
Bailenson, J. (2018). Experience on demand: What virtual reality is, how it works, and what it can do. New York, NY, USA: WW Norton & Company.
Bailenson, J. N., Yee, N., Blascovich, J., Beall, A. C., Lundblad, N., and Jin, M. (2008). The use of immersive virtual reality in the learning sciences: Digital transformations of teachers, students, and social context. J. Learn. Sci. 17, 102–141. doi:10.1080/10508400701793141
Beeman, S. M., Kemper, A. R., Madigan, M. L., and Duma, S. M. (2011). Effects of bracing on human kinematics in low-speed frontal sled tests. Ann. Biomed. Eng. 39, 2998–3010. doi:10.1007/s10439-011-0379-1
Behr, M., Poumarat, G., Serre, T., Arnoux, P.-J., Thollon, L., and Brunet, C. (2010). Posture and muscular behaviour in emergency braking: An experimental approach. Accid. Analysis Prev. 42, 797–801. doi:10.1016/j.aap.2009.04.010
Brossmann, F., Haböck, U., Maier, S., and Schwenninger, J. (2018). Virtual reality als werkzeug für die entwicklung von assistenzsystemen. ATZ Automob. Z. 120, 30–35. doi:10.1007/s35148-018-0041-4
Carlsson, S., and Davidsson, J. (2011). “Volunteer occupant kinematics during driver initiated and autonomous braking when driving in real traffic environments,” in 2011 IRCOBI Conference Proceedings., ed. International Research Council on the Biomechanics of Injury (IRCOBI), 125–136.
Chan, H., Devane, K., Albert, D., Gayzik, S., and Kemper, A. (2021). “Comparisons of initial joint angles and test buck reaction forces for relaxed and braced 5th percentile female and 50th percentile male volunteers and analogous active human body models in a simulated drivers seat,” in 2021 IRCOBI Conference Proceedings. Eed. International Research Council on the Biomechanics of Injury (IRCOBI), 83–104.
Dede, C. (2009). Immersive interfaces for engagement and learning. Science 323, 66–69. doi:10.1126/science.1167311
Ejima, S., Zama, Y., Ono, K., Kaneoka, K., Shiina, I., and Asada, H. (2009). “Prediction of pre-impact occupant kinematic behavior based on the muscle activity during frontal collision,” in Proc. of 21st ESV Conference.
Erlinger, N., Kofler, D., Heider, E., and Klug, C. (2022). “Effects of boundary conditions and posture on simulations with human body models of braking events,” in 2022 IRCOBI Conference Proceedings, ed. International Research Council on the Biomechanics of Injury (IRCOBI), IRCOBI Conference Proceedings, 628–652.
Fice, J. B., mang, D. W., Ólafsdóttir, J. M., Brolin, K., Cripton, P. A., Blouin, J. S., et al. (2019). “Head/neck kinematics and muscle responses for relaxed drivers and passengers in frontal and rear impacts,” in 2019 IRCOBI Conference Proceedings, ed. International Research Council on the Biomechanics of Injury (IRCOBI), IRCOBI Conference Proceedings, 121–122.
Ghaffari, G., Brolin, K., Pipkorn, B., Jakobsson, L., and Davidsson, J. (2019). Passenger muscle responses in lane change and lane change with braking maneuvers using two belt configurations: Standard and reversible pre-pretensioner. Traffic Inj. Prev. 20, S43–S51. doi:10.1080/15389588.2019.1634265
Graci, V., Maltenfort, M., Schneider, M., Griffith, M., Seacrist, T., and Arbogast, K. B. (2021). Quantitative characterization of aeb pulses across the modern fleet. Traffic Inj. Prev. 22, S62–S67. doi:10.1080/15389588.2021.1961227
Huber, P., Kirschbichler, S., Prüggler, A., and Steidl, T. (2015). “Erkenntnisse zur insassenkinematik aus dem aktuellen om4is projekt,” in VDI-Tagung Fahrzeugsicherheit.
Huber, P., Kirschbichler, S., Prüggler, A., and Steidl, T. (2014). “Three-dimensional occupant kinematics during frontal, lateral and combined emergency maneuvers,” in IRCOBI Conference 2014, 668–669.
Ito, D., Ejima, S., Kitajima, S., Katoh, R., Ito, H., Sakane, M., et al. (2013). “Occupant kinematic behavior and effects of a motorized seatbelt on occupant restraint of human volunteers during low speed frontal impact: Mini–sled tests with mass production car seat,” in Proceedings of IRCOBI Conference, Gothenburg, Sweden, 2013.
Iwamoto, M., Nakahira, Y., and Kimpara, H. (2015). Development and validation of the total human model for safety (thums) toward further understanding of occupant injury mechanisms in precrash and during crash. Traffic Inj. Prev. 16 (1), 36–48. doi:10.1080/15389588.2015.1015000
Kim, G. J., and Rizzo, A. S. (2005). A swot analysis of the field of virtual reality rehabilitation and therapy. Presence. (Camb). 14, 119–146. doi:10.1162/1054746053967094
Kirschbichler, S., Huber, P., Prüggler, A., Steidl, T., Sinz, W., Mayer, C., et al. (2014). “Factors influencing occupant kinematics during braking and lane change maneuvers in a passenger vehicle,” in Proceedings of IRCOBI Conference 2014 (IRC-14-70), 614–625.
Kirschbichler, S., Sinz, W., Prüggler, A., Huber, P., and Steiner, K. (2011). “Detailed analysis of 3d occupant kinematics and muscle activity during the pre-crash phase as basis for human modeling based on sled tests,” in International Technical Conference on the Enhanced Safety of Vehicles.
Lagoo, R., Charissis, V., and Harrison, D. K. (2019). Mitigating driver’s distraction: Automotive head-up display and gesture recognition system. IEEE Consum. Electron. Mag. 8, 79–85. doi:10.1109/mce.2019.2923896
Lambert, V., Boylan, P., Boran, L., Hicks, P., Kirubakaran, R., Devane, D., et al. (2020). Virtual reality distraction for acute pain in children. Cochrane Database Syst. Rev. 2020, CD010686. doi:10.1002/14651858.cd010686.pub2
Muller Queiroz, A. C., Moreira Nascimento, A., Tori, R., Brashear Alejandro, T., Veloso de Melo, V., de Souza Meirelles, F., et al. (2018). Immersive virtual environments in corporate education and training.
Nascimento, A. M., Queiroz, A. C. M., Vismari, L. F., Bailenson, J. N., Cugnasca, P. S., Camargo, J. B., et al. (2019). “The role of virtual reality in autonomous vehicles’ safety,” in Proceedings - 2019 IEEE International Conference on Artificial Intelligence and Virtual Reality, AIVR 2019, 50–57. doi:10.1109/AIVR46125.2019.00017
Nikulin, C., Lopez, G., Piñonez, E., Gonzalez, L., and Zapata, P. (2019). Nasa-tlx for predictability and measurability of instructional design models: Case study in design methods. Educ. Technol. Res. Dev. 67, 467–493. doi:10.1007/s11423-019-09657-4
Ohlrogge, C., and Glaß, F. (2020). Virtual-reality in der schmerztherapie. Pflegez. 73, 52–55. doi:10.1007/s41906-020-0718-7
Olafsdottir, J., Östh, J., Davidsson, J., and Brolin, K. (2013). “Passenger kinematics and muscle responses in autonomous braking events with standard and reversible pre–tensioned restraints,” in Proceedings of IRCOBI Conference, Gothenburg, Sweden, 2013.
Öst, J., Brolin, K., and Brase, D. (2015). A human body model with active muscles for simulation of pretensioned restraints in autonomous braking interventions. Traffic Inj. Prev. 16, 304–313. doi:10.1080/15389588.2014.931949
Östh, J., Ólafsdóttir, J. M., Davidsson, J., and Brolin, K. (2013). Driver kinematic and muscle responses in braking events with standard and reversible pre-tensioned restraints: Validation data for human models. Stapp Car Crash J. 57, 1–41. doi:10.4271/2013-22-0001
Östmann, M., and Jakobsson, L. (2016). “An examination of pre-crash braking influence on occupant crash response using an active human body model,” in International Research Council on the Biomechanics of Injury (Hg.): 2016 IRCOBI Conference Proceedings. IRCOBI Conference, Malaga, Spain, 275–283.
Potkonjak, V., Gardner, M., Callaghan, V., Mattila, P., Guetl, C., Petrović, V. M., et al. (2016). Virtual laboratories for education in science, technology, and engineering: A review. Comput. Educ. 95, 309–327. doi:10.1016/j.compedu.2016.02.002
Reed, M. P., Ebert, S. M., Jones, M. L. H., and Hallman, J. J. (2020). Prevalence of non-nominal seat positions and postures among front-seat passengers. Traffic Inj. Prev. 1, S7–S12. doi:10.1080/15389588.2020.1793971
Reed, M. P., Ebert, S. M., Jones, M. L. H., and Park, B.-K. D. (2019). Comparison across vehicles of passenger head kinematics in abrupt vehicle maneuvers. Traffic Inj. Prev. 20, S128–S132. doi:10.1080/15389588.2019.1689566
Regenbrecht, H., Schubert, T., Kohlhaas, M., Friedmann, F., Beetz, J., Schmidt-Tjarksen, T., et al. (2016). Igroup presence questionnaire (ipq).
Saito, H., Matsushita, T., Pipkorn, B., and Boström, O. (2016). “Evaluation of frontal impact res traint system in integrated saf ety scenario using human body model with pid controlled active muscles,” in International Research Council on the Biomechanics of Injury (Hg.): 2016 IRCOBI Conference Proceedings. IRCOBI Conference, Malaga, Spain, 248–260.
Sandoz, B., Sugiyama, T., and Weber, J. (2019). “Upper body in vivo kinematics during moderate lateral accelerations – towards active finite element human body model validation,” in 2019 IRCOBI Conference Proceedings, ed. International Research Council on the Biomechanics of Injury (IRCOBI), IRCOBI Conference Proceedings, 129–130.
Tudor, S., Carey, S., and Dubey, R. (2015). “Development and evaluation of a dynamic virtual reality driving simulator,” in Proceedings of the 8th ACM International Conference on PErvasive Technologies Related to Assistive Environments, 1–5.
Yamada, K., Gotoh, M., Kitagawa, Y., and Yasuki, T. (2016). “Simulation of occupant posture change during autonomous emergency braking and occupant kinematics in frontal collision,” in 2016 IRCOBI Conference Proceedings, ed. International Research Council on the Biomechanics of Injury (IRCOBI), IRCOBI Conference Proceedings, 261–274.
Keywords: virtual reality, precrash kinematics, volunteer tests, occupant safety, distraction
Citation: Rajinovic S, Safikhani S, Schachner M, Gstrein G, Kofler D, Schneider B, Erlinger N, Pirker J and Klug C (2022) Testing of human reactions during braking manoeuvres combining a physical test bed with virtual reality. Front. Future Transp. 3:991666. doi: 10.3389/ffutr.2022.991666
Received: 11 July 2022; Accepted: 24 October 2022;
Published: 16 November 2022.
Edited by:
Jaehyun Park, Incheon National University, South KoreaReviewed by:
David Kidd, Insurance Institute for Highway Safety (IIHS), United StatesPeter Burns, Transport Canada, Canada
Duane S. Cronin, University of Waterloo, Canada
Copyright © 2022 Rajinovic, Safikhani, Schachner, Gstrein, Kofler, Schneider, Erlinger, Pirker and Klug. This is an open-access article distributed under the terms of the Creative Commons Attribution License (CC BY). The use, distribution or reproduction in other forums is permitted, provided the original author(s) and the copyright owner(s) are credited and that the original publication in this journal is cited, in accordance with accepted academic practice. No use, distribution or reproduction is permitted which does not comply with these terms.
*Correspondence: Johanna Pirker, johanna.pirker@tugraz.at; Corina Klug, corina.klug@tugraz.at