Unveiling biomarkers for postharvest resilience: the role of canopy position on quality and abscisic acid dynamics of ‘Nadorcott’ clementine mandarins
- Plant Science Laboratory, Cranfield University, Cranfield, United Kingdom
Introduction: Clementine mandarins are categorised as non-climacteric fruit because they do not exhibit an increase in respiration rate and ethylene production during ripening. Recent studies have suggested that abscisic acid (ABA), a distinct ripening hormone, plays a pivotal role in the postharvest behaviour of this fruit. The present study aimed to identify biomarkers for enhancing the postharvest durability and flavour retention of imported ‘Nadorcott’ clementines (Citrus reticulata Blanco). To achieve this goal, an experiment was designed to investigate pre- and post-harvest factors influencing quality of clementine fruit, encompassing both physiological and biochemical aspects. Furthermore, the study investigated the role of ABA and ABA catabolites in the fruit senescence process.
Methods: Clementines from four different canopy positions, i.e. upper outside, upper inside, lower outside, and lower inside, were manually harvested at different horticultural maturity. The study was conducted over two harvest times, specifically in March (mid-season) and April (end of season). Harvested fruit samples were stored for six weeks in a cold room with temperature and relative humidity set at 8.5±0.5 °C and 85±2%, respectively. Various physiological (i.e. respiration rate), organoleptic (i.e. rind colour, total soluble solids, and titratable acidity) and biochemical (i.e. individual sugars and organic acids, abscisic acid and its catabolites) variables were measured bi-weekly. Sensory evaluation and consumer survey were conducted during the fourth week of storage.
Results and discussion: At the end of storage, fruit located on the inside of the canopy had a significantly higher respiration rate and lower concentration of sugars than outside fruit, resulting in a shorter shelf-life. At the same time, inside fruit showed a higher concentration of ABA and ABA catabolites than outside fruit, coinciding with a lower consumer acceptance score for external appearance, aroma, and flavour. For the first time, the key biomarkers affecting resilience and flavour retention, notably the higher ABA concentration and lower sugar levels in inner canopy fruit, resulting in a shorter shelf-life and reduced consumer acceptance were unveiled. This research not only provides valuable insights for the citrus industry but also unveils the intricate relationship between fruit quality, ABA, and ABA catabolites during senescence and consumer acceptance.
1 Introduction
Soft citrus, including mandarins such as tangerines, clementines, and satsumas are preferred by consumers for their ease of peeling, juiciness, and delightful balance of sweet and tangy citrus flavours (Bureš et al., 2023; Gupta et al., 2023). However, consumers are not able to evaluate these attributes at the moment of purchasing, and their decision is based on the external appearance and perceived freshness of the fruit (Magwaza and Opara, 2015; Guo et al., 2023). To maintain a consistent supply of fruit throughout the year, the citrus industry procures fruit from multiple growing locations spanning different seasons and climatic regions. As a result, effective management of the fruit within the canopy position, light interception, irrigation, harvest maturity, postharvest storage temperature and relative humidity become crucial in determining the final quality (Mditshwa et al., 2017; Lado et al., 2018). Citrus fruits are harvested using established commercial standards which are based on the concept of minimum physiological maturity. However, the horticultural maturity at which citrus is harvested can vary, depending on intended use and targeted markets. Hence, fruit harvested at different times during the season will exhibit varying quality categories (Thakre et al., 2015; Rokaya et al., 2016; Sun et al., 2021).
The position of the fruit within the canopy has been widely reported to have an effect on citrus rind quality and susceptibility to postharvest disorders (Cronje et al., 2011a., Cronje and Barry, 2013; Magwaza et al., 2013, 2019). Generally, fruit harvested from the outer, sun-exposed parts of the canopy have better colour (deep orange), higher rind sugar and organic acid concentration, higher juice content, and less susceptibility to postharvest rind disorders (Moon et al., 2011; Cronje et al., 2011a; Cronje and Barry, 2013; Ncama et al., 2018a; Ncama et al., 2018b; Olarewaju et al., 2017). However, opposing results have also been reported, with the fruit located on the inside position of the canopy showing the highest rind carbohydrate levels (Magwaza et al., 2013). These differences between canopy positions have been explained by the postharvest changes in hormonal content due to abiotic stresses (Rosa et al., 2009; Romero et al., 2013; Sawicki et al., 2015; Magwaza et al., 2019; Olarewaju et al., 2022) as well as the different microclimatic conditions within the canopy, mainly light exposure, temperature, and osmotic pressures (Moon et al., 2011; Cronje et al., 2011b; Cronje and Barry, 2013).
Although a significant amount of research has reported the effect of the canopy on citrus fruit quality, most of the studies have focused on rind quality during postharvest storage, rather than on internal biochemical properties. The information on how canopy position affects citrus fruit internal quality and consumer experience after harvest is limited (Ncama et al., 2018a, Ncama et al., 2018b; Olarewaju et al., 2018, 2022). This knowledge gap has been previously reported by many authors, who have identified the need for research relating to produce attributes, shelf-life, and consumer preferences (Poole and Baron, 1996; Gao et al., 2011; Terry et al., 2011; Goldenberg et al., 2015);. Therefore, the present study was designed with the objectives of (i) evaluating the quality of clementines from different canopy positions at different horticultural maturity stages during postharvest cold storage, (ii) determining if these differences can be perceived by consumers during the eating experience, and (iii) identifying the attributes that drive purchasing decisions and if these coincide with the preferences of citrus consumers.
2 Materials and methods
2.1 Plant material
The experiments were conducted on clementine fruits (Citrus reticulata Blanco) cv. ‘Nadorcott’ on Cleopatra (Citrus reshni hort.) rootstock from a commercial field in Lora del Rio, Seville (Southern Spain). The meteorological data for the orchard in the months preceding the harvest is presented in Supplementary Table 1. Three trees from three consecutive rows (spaced 6 x 5 m) (9 trees, n = 3) were selected for their good crop load and distribution of the fruit within the tree. A total of 2,880 clementine samples were manually harvested from four distinct canopy positions, namely, upper outside, upper inside, lower outside, and lower inside (Khan et al., 2009, Cronje et al., 2011a; Cronje et al., 2011b; Cronje and Barry, 2013) (Supplementary Figure 1). Fruit were harvested at different horticultural maturity according to commercial standards at two different times during the 2017 season (March - middle of the season and April – end of the season). The fruit were then transferred to the Plant Science Laboratory (PSL) at Cranfield University, UK, by refrigerated lorry (5.5 ± 0.5°C and 85 ± 2% relative humidity [RH]) within 5 days from harvest.
2.2 Experimental design
Upon arrival at the Plant Science Laboratory (PSL), samples with skin damage or decay were removed, and only sound fruit were placed in crates in cold storage (8.5 ± 0.5°C and 85 ± 2% RH) overnight to acclimatise. The experiment consisted of four canopy positions, two harvest times (March and April), and three replicates per canopy position, with six fruit per replicate. This led to a total of 18 fruit (n = 3 reps * 6 fruit per rep = 18) per canopy position. Sampling for physical, physiological, and biochemical parameters was done upon arrival and every two weeks during the six-week storage period.
2.3 Physiological analysis
2.3.1 Respiration rate and rind colour
Respiration rate (RR) was measured as described by Collings et al. (2018). Samples were placed in 3 L airtight glass jars and dry clean air (300 mL min-1) was passed through the jars into a Sable Respirometry System (model 1.3.8 Pro, Sable Systems International, Las Vegas, USA). Each sample jar (n = 3 replicates per treatment, 6 clementine fruit per replicate) was measured for 2 min to achieve a stable reading. An empty jar (continuous air for 1 min) was used as a baseline to avoid crosscontamination between treatment measurements. The rate of CO2 production was expressed in mL kg-1 h-1.
The external colour of the samples was determined using a hand-held tristimulus colourimeter with a D65 illuminant and 8 mm light aperture (model CR-400 Chroma Meter, Konica Minolta Inc., Cheshire, UK). The colourimeter was calibrated with a white plate, and it provided the lightness.
(L*), chroma index (C∗ = √(a∗)2 + (b∗)2), and Hue angle (H0 = tan−1(b∗⁄a∗)) values of the samples, where a* and b* are the CIELAB colour space coordinates. These colour parameters are widely used as a measure of ripeness (Conesa et al., 2016).
2.3.2 Total soluble solids, juice content and titratable acidity
The juice of six clementine fruit per replicate was prepared using a commercial citrus juicer and filtered through a stainless steel fine mesh sieve to obtain a clear juice. The total soluble solids (TSS) content of the clementine juice was measured at room temperature (21°C) using a digital refractometer with automatic temperature compensation (model PR-32α, Atago Ltd., Tokyo, Japan) and expressed as %. The refractometer was calibrated using deionised water, and the measurements were done in triplicate. The juice content was determined by weighing both the juice and the fruit, using the following formula: juice content = (juice mass ÷ fruit weight) *100.
Titratable acidity (TA) was determined using an automatic titrator (model Rondolino, Mettler Toledo Ltd., Leicester, UK). The calibration of the equipment was done using a 3-point calibration curve with pH buffers ranging from pH 4 to pH 10. Then, 5 mL of juice was homogenised with 50 mL of deionised water, and the mL of NaOH consumed to reach an endpoint of pH 8.2 were recorded. The TA of the clementine fruit juice (%) was calculated as mL NaOH * 0.1 N NaOH * milliequivalent factor * 100 * mL-1 juice. The milliequivalent factor for citric acid, which is the predominant acid in citrus juice, is 0.064.
2.4 Biochemical analysis
Before biochemical analysis, portions of clementine segments were cut, snap-frozen in liquid nitrogen and placed in a -50°C CoolSafe freeze-dryer (Scanvac, Labogene, Denmark) for seven to ten days, until a constant mass was reached. After this, the freeze-dried samples were ground and kept at -40°C until analysis (Garcia-Pastor et al., 2021).
2.4.1 Individual sugars
Extraction and analysis of individual sugars (glucose, fructose, and sucrose) were done following Davis et al. (2007). Briefly, 150 mg of freeze-dried fruit samples were extracted with 3 mL of 62.5% (v/v) aqueous High Performance Liquid Chromatography (HPLC)-grade methanol in a water bath at 55°C for 15 min, vortexing every 5 min for 30 s. The extract was then filtered through 0.2 μm PTFE filters and stored in 2 mL HPLC vials at -40°C until analysis. Before analysis, the extracts were diluted 1:4 with HPLC-grade water.
Detection was performed using a refractive index detector (RID) coupled to an Agilent 1200 series HPLC system (Agilent Technologies, Germany) with a Phenomenex Rezex RCM monosaccharide Ca+2 (8%) ion exclusion column (300 mm x 7.8 mm), 8 μm particle size, fitted with a Phenomenex Carbo Ca+4 mm x 3 mm guard column (Phenomenex, CA). The column oven temperature was set at 80°C and the refractive index detector at 50°C. The mobile phase used was HPLC-grade water at a flow rate of 0.6 mL min-1, and the autosampler injection volume was 20 μL. A 7-point calibration curve ranging from 1 to 4 mg mL-1 was used for quantification, and the results were expressed as mg g-1 dry weight (DW).
2.4.2 Individual organic acids
Analysis of non-volatile organic acids was done as described by Terry et al. (2007) with slight modifications. Briefly, 150 mg of freeze-dried samples were dissolved in 6 mL of 3% aqueous metaphosphoric acid, kept at room temperature for 10 min, filtered through 0.2 μm cellulose filters into HPLC vials, and analysed immediately after extraction.
The concentration of organic acids was determined using an Agilent 1200 series HPLC system with an Alltech Prevail Organic Acid 250 mm x 4.6 mm, 5 μm particle size column fitted with an Alltech Prevail Organic Acid 7.5 mm x 4.6 mm, 5 μm particle size guard column (Alltech, CA, USA). The column oven temperature was set at 35°C, and the compounds were detected by a diode array detector (DAD) set at 210 nm. The mobile phase used was 25 mM KH2PO4 HPLC-grade water at a flow rate of 1.5 mL min-1, and the autosampler injection volume was 20 μL. An 8-point calibration curve ranging from 5 to 300 µg mL-1 with a mixture of tartaric and malic acids was used for quantification, and the results were expressed as mg g-1 DW.
2.4.3 Abscisic acid and its catabolites
The concentration of ABA and ABA catabolites was determined by following a method previously used by Serradilla et al. (2019) with modifications. Freeze-dried samples (5 mg) were mixed with 500 μL of methanol/formic acid/water (60:5:35, v/v/v) and 10 μL of the internal standards mix (100 ng mL-1) in a 1.5 mL Eppendorf tube, and shaken in a Star Beater (R&L Slaughter Ltd., Essex, UK) at 30 Hz for 2 min. The internal standard mix was made of the labelled forms of the compounds: (-)-
5,8ˊ8ˊ8ˊ-d4-abscisic acid (d4-ABA); (-)-7ˊ,7ˊ,7ˊ-d3-phaseic acid (d3-PA); (-)-7ˊ,7ˊ,7ˊ-d3dihydrophaseic acid (d3-DPA); (+)-4,5,8ˊ,8ˊ,8ˊd5-abscisic acid glucose ester (d5-ABA-GE); (±)5,8ˊ,8ˊ,8ˊ-d4-7ˊ-dihydroxy-ABA (d4−7-OH-ABA). The tubes were then placed in dry ice and kept in darkness for 20 min. Afterwards, the tubes were centrifuged at 4°C and 14 000 rpm for 10 min, then transferred to 15 mL falcon tubes and freeze-dried overnight. The extracts were reconstituted with 500 μL of acetonitrile/formic acid/water (10:0.1:89.9, v/v/v), vortexed for 1 min, sonicated for 1 min, and centrifuged at 4°C and 4 500 rpm for 1 min. The samples (20 μL) were then injected on a Phenomenex Luna C18 100 mm x 2 mm, 3 μm with guard column at 40 °C, and analysed by an Agilent 1200 series HPLC system coupled to a Q-Trap 6500 mass spectrometer (AB Sciex, MA, USA). The concentration of ABA and ABA metabolites was quantified based on Morris et al. (2018) methodology. A 6-point calibration curve ranging from 0.1 to 100 ng mL-1 was used for quantification, and the results were expressed as ng g-1 DW.
2.5 Consumer study
2.5.1 Sensory evaluation
The consumer tasting session was performed by an external company, Wirral Sensory Services (Wirral, UK), which recommended a minimum of 50 panellists to obtain useful results. The tasters were regular consumers of citrus fruits with a mixture of gender and socio-economic demographics. The session took place at week 4 (representing minimum commercial storage time) of storage for the fruit harvested in March. This was simulating commercial storage and consumers were presented with one fruit per canopy position in random order and asked to score them for appearance, easiness to peel, aroma, flavour, juiciness, texture, and overall acceptance using a 9-point hedonic scale, where “1” meant “Extremely dislike” and “9” meant “Extremely like”, according to Kemp et al. (2009).
2.5.2 Buying decision survey
A survey was carried out with the same 50 panellists to identify the most important citrus attributes for consumers and which ones were relevant for them at the moment of buying. The respondents ranked different citrus attributes (appearance, easiness to peel, aroma, flavour, sweetness, tanginess, juiciness, and texture) from 1 to 8, with 1 being the most important attribute and 8 the least important. The total attribute importance was calculated using Equation 1 (Kemp et al., 2009).
2.6 Statistical analysis
Data were subjected to normality and homoscedasticity tests; data transformations were carried out (e.g. sensory evaluation data) and outliers removed if necessary. An analysis of variance (ANOVA) was performed to test the experimental hypotheses. When significant differences were found (p < 0.05), the data were subjected to a Fisher’s post-hoc test. The least significant differences (LSD) were calculated for each analysis (p < 0.05) and shown on the graphs when the interaction was significant. When factors were significant, LSD values were included in the corresponding caption for comparison. The interactions (canopy position*harvest time*storage time) were studied. All statistical analyses were carried out using Statistica for Windows version 13 (Dell Inc., USA). Figures were plotted in SigmaPlot 14 (Systat Software Inc., USA).
3 Results
3.1 Respiration rate and rind colour
Fruit positioned on the upper part of the canopy presented a higher RR than fruit on the lower part, at both harvest dates (March and April) (Figure 1). For the clementine mandarin harvested in March, fruit located on the inside of the canopy (shadowed) had significantly higher (~48% more) RR at the end of storage than outside (sun-exposed) fruit. At the same time, a significant increase (31.5-90%) in RR was observed from week 4 to week 6 of storage for both outside and inside fruit. Interestingly, this increase was observed in fruit harvested in April two weeks earlier, from weeks 2 to 4. However, as seen in March, all the positions reached similar RR values at the end of storage.
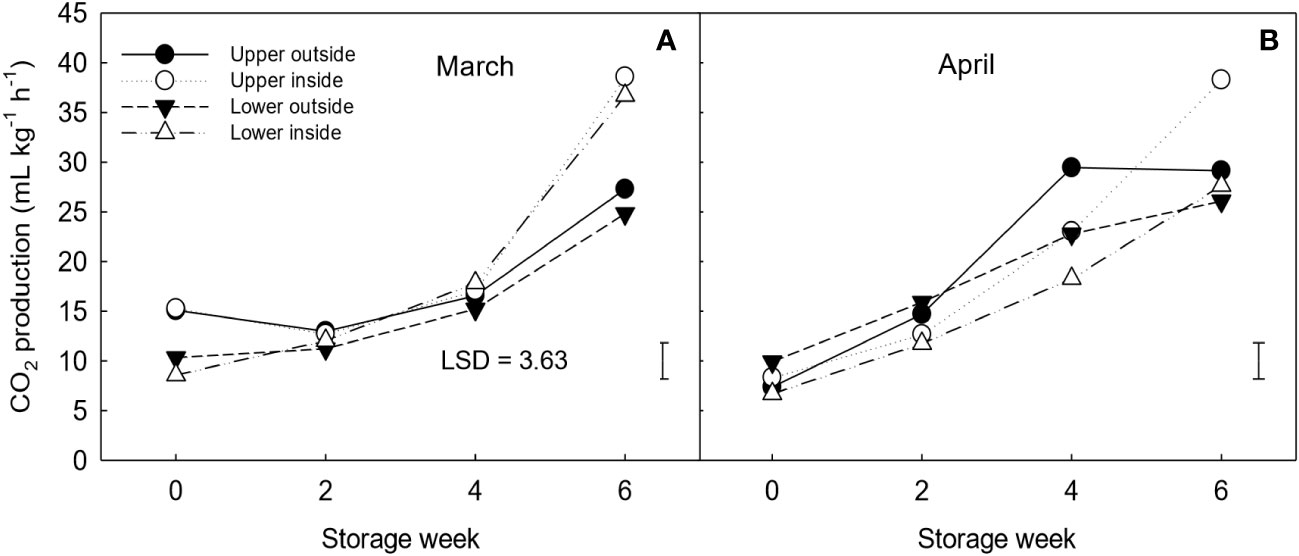
Figure 1 Respiration rate (RR) expressed as CO2 production (mL kg-1 h-1) of ‘Nadorcott’ clementines harvested in March (A) and April (B) 2017. The fruit were harvested from different canopy positions (upper outside, upper inside, lower outside and lower inside) and stored for six weeks at 8.5 ± 0.5°C and 85 ± 2% RH. Data represents means (n = 3 reps * 6 fruit per rep = 18) ± standard error of the means. The LSD0.05 for canopy position*harvest time*storage time interaction is shown.
Regarding rind colour, fruit positioned on the lower outside part of the canopy and harvested in March presented lower lightness, chroma index, and hue angle values than the rest of the samples (Figure 2). While all the positions showed similar colour values during March, a higher variability within positions was seen for the fruit harvested in April, when inside fruit showed significantly higher lightness and higher hue angle values than outside fruit during the first two weeks of storage.
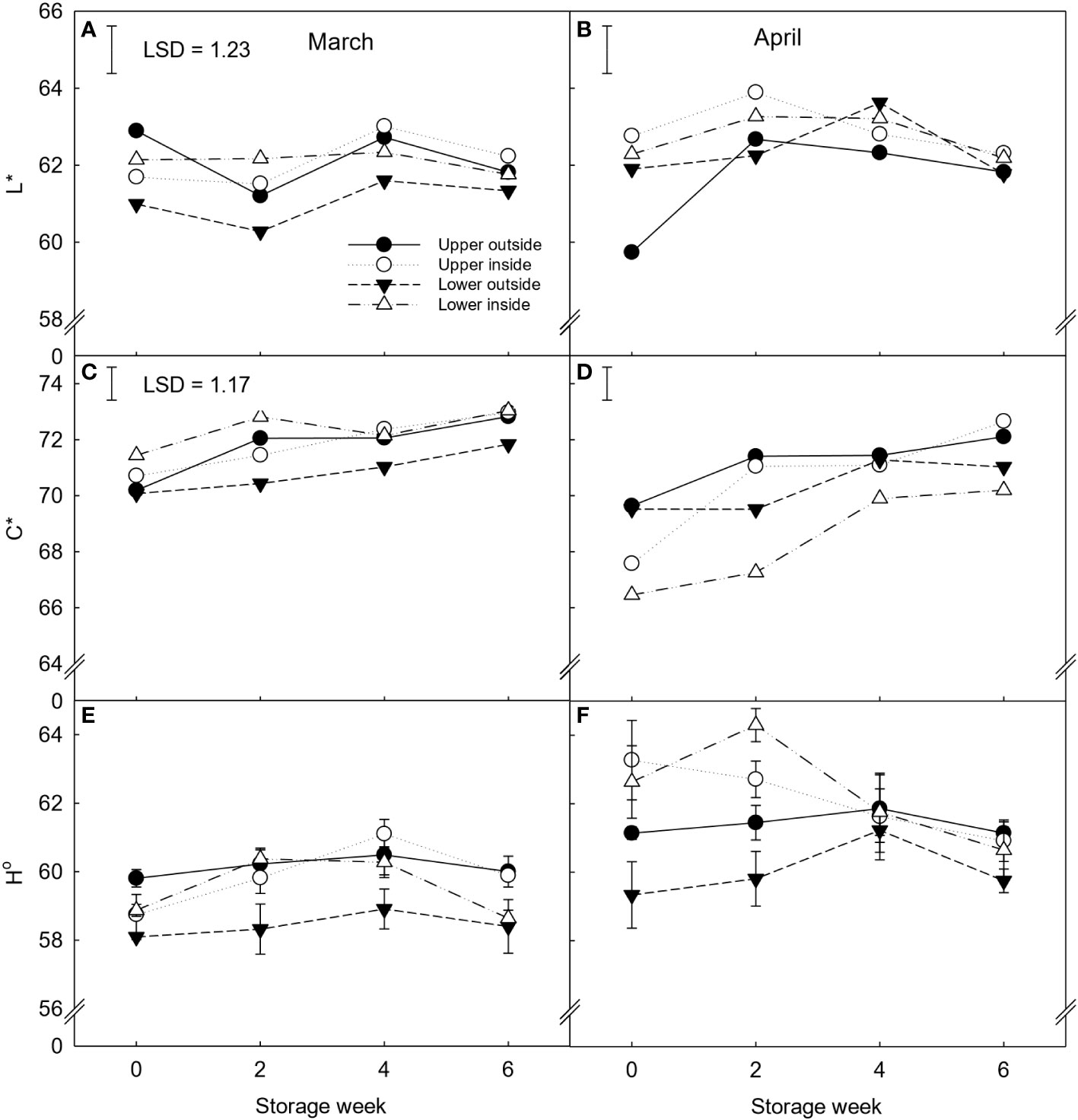
Figure 2 CIELab colour parameters {Lightness (L*) (A, B), Chroma index (C*) (C, D), and Hue angle (H°) (E, F) of ‘Nadorcott’ clementines harvested in March (left) and April (right) 2017. The fruit were harvested from different canopy positions (upper outside, upper inside, lower outside and lower inside) and stored for six weeks at 8.5 ± 0.5°C and 85 ± 2% RH. Data represents means (n = 3 reps * 6 fruit per rep = 18) ± standard error of the mean. For L* and C*, the LSD0.05 for canopy position*harvest time*storage time interaction was 1.23 and 1.17, respectively. For H°, the canopy position*harvest time*storage time interaction was not significant, hence, LSD0.05 not shown.
3.2 Juice content, total soluble solids, and titratable acidity
The interaction of canopy position, harvest time, and storage week significantly affected juice content, TSS, and TA (Figure 3). Fruit harvested in March maintained similar TSS and TA values throughout cold storage, while the April harvest showed higher variability within positions in TSS and TA values. Nevertheless, clear trends were observed during this month, when outside fruit had higher TSS and TA levels than inside fruit. Moreover, significant differences in TSS were observed between weeks 2 and 4 of storage for fruit harvested in April, with TSS increasing more than 1% for all positions studied. By the end of storage, fruit located on the upper part of the canopy respectively showed 10 and 17% higher TSS and TA values than on the lower part, and the highest values (14.3% and 0.7% TA) were observed for the upper outside fruit. However, this position had 5% lower juice content at the end of storage independently of the harvest month.
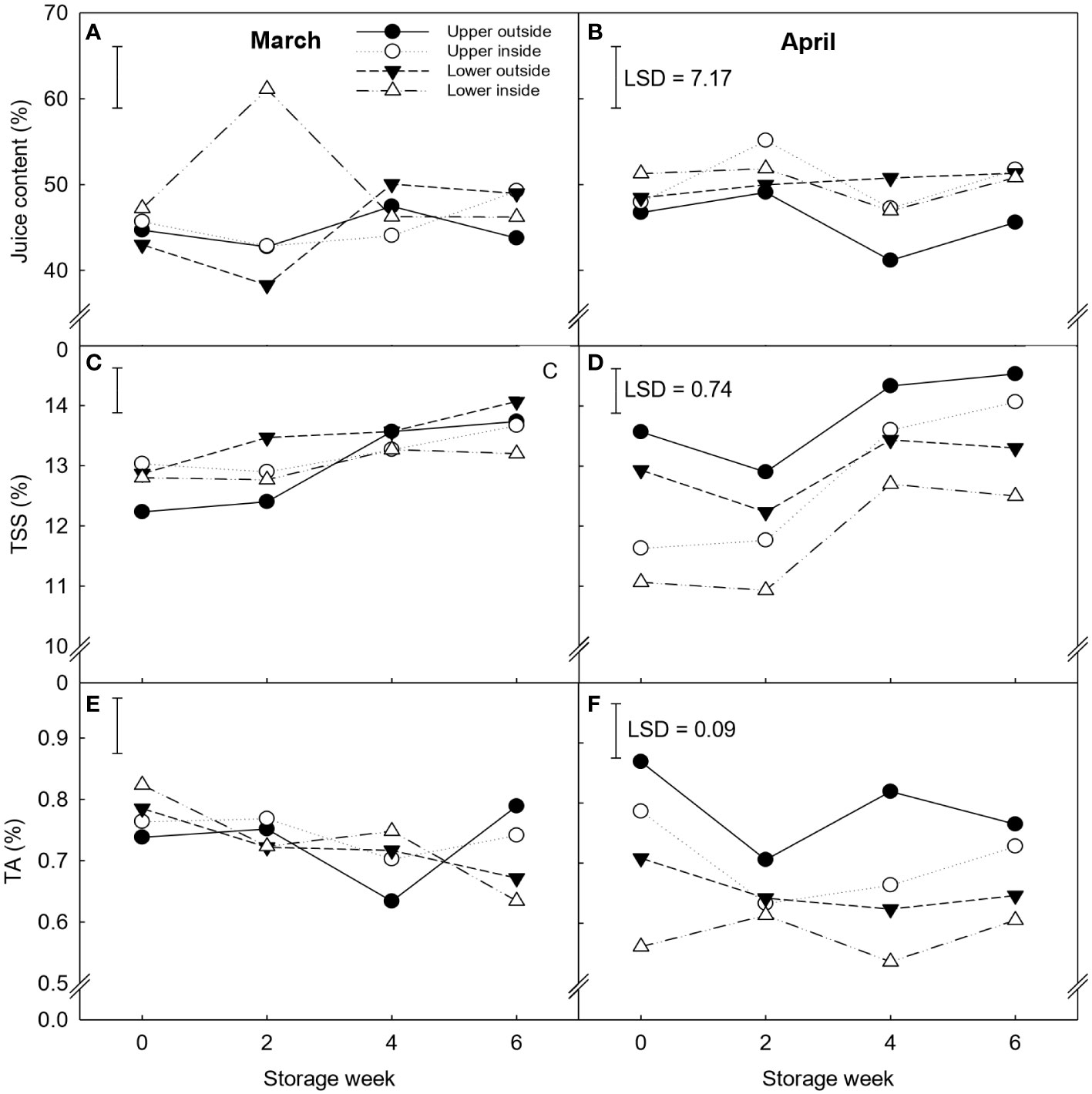
Figure 3 Juice content (A, B), Total soluble solids (TSS) (C, D) and titratable acidity (TA) (E, F) expressed in percentage (%) of ‘Nadorcott’ clementines harvested in March (left) and April (right) 2017. The fruit were harvested from different canopy positions (upper outside, upper inside, lower outside and lower inside) and stored for six weeks at 8.5 ± 0.5°C and 85 ± 2% RH. Data represents means (n = 3 reps * 6 fruit per rep = 18) ± standard error of the mean. For juice content, TSS and TA, the LSD0.05 for canopy position*harvest time*storage time interaction was 7.7, 0.74, and 0.09, respectively.
3.3 Individual sugars
Sucrose was the primary sugar found in ‘Nadorcott’ clementines, followed by fructose and glucose (Figure 4). As observed for TSS, the concentration of sugars on the fruit harvested in March was similar between the different canopy positions throughout storage, except for week 2, when upper outside and lower inside fruit had the highest and the lowest sucrose levels, respectively. However, lower inside fruit showed a significant increase in the sucrose content from week 2 to week 4 of storage. Besides that, the fructose and glucose levels significantly increased from week 0 until the end of storage. Significant differences in the fructose and glucose content were observed between canopy positions. On the other hand, the fructose and glucose content of fruit harvested in April was higher for upper outside fruit and lower for lower inside fruit independently of the storage week. However, at the end of storage, there was a significant decrease in all sugars (8, 9 and 12% for fructose, glucose, and sucrose, respectively) in fruit located on the lower inside position of the tree.
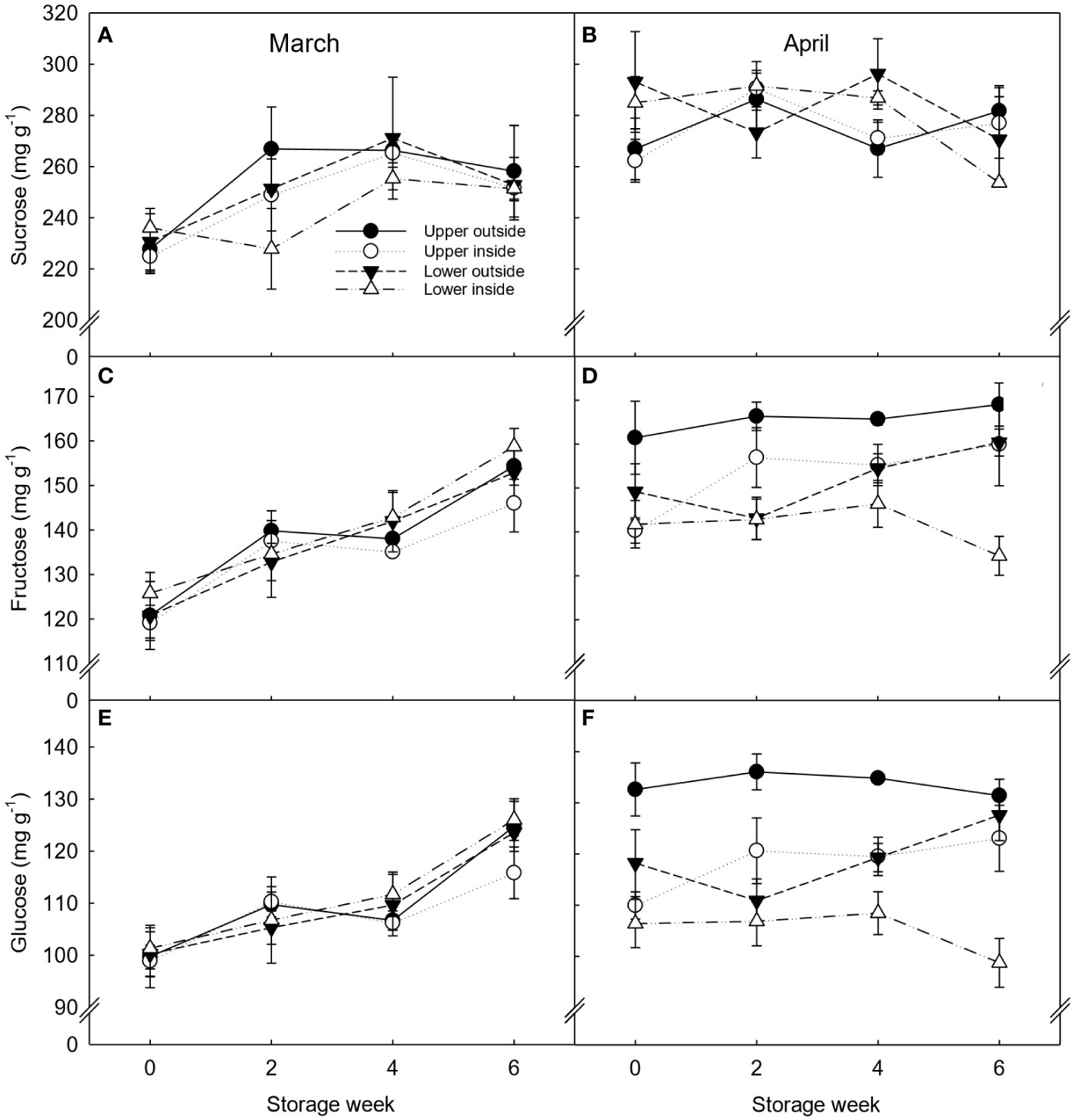
Figure 4 Individual sugars (sucrose (A, B), fructose (C, D), and glucose (E, F)) expressed as mg g-1 dry weight (DW) of ‘Nadorcott’ clementines harvested in March (left) and April (right) 2017. The fruit were harvested from different canopy positions (upper outside, upper inside, lower outside and lower inside) and stored for six weeks at 8.5 ± 0.5°C and 85 ± 2% RH. Data represents means (n = 3 reps * 6 fruit per rep = 18) ± standard error of the mean. The canopy position*harvest time*storage time interaction was not significant, hence, LSD0.05 was not shown.
3.4 Individual organic acids
The primary organic acids found in clementines were citric and ascorbic acid. No significant differences within canopy positions were observed in fruit harvested in March (Figure 5). In contrast, fruit harvested in April and located on the upper part of the canopy showed significantly higher (17%) citric acid content throughout storage than on the lower part. Similarly, fruit located on the inside part of the canopy had higher ascorbic acid levels than fruit on the outside.
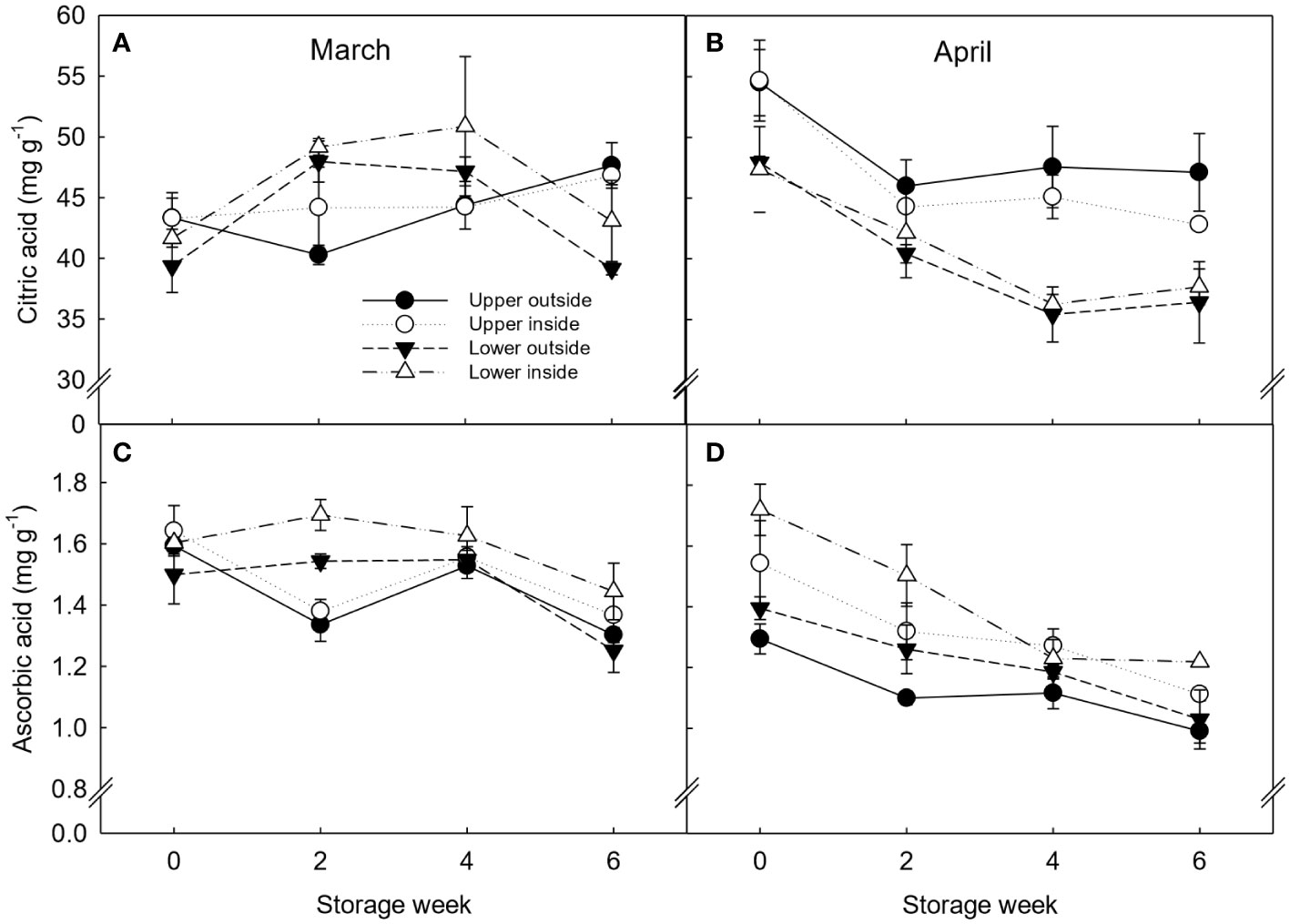
Figure 5 Organic acids (citric acid (A, B) and ascorbic acid (C, D)) expressed as mg g-1 dry weight (DW) of ‘Nadorcott’ clementines harvested in March (left) and April (right) 2017. The fruit were harvested from different canopy positions (upper outside, upper inside, lower outside and lower inside) and stored for six weeks at 8.5 ± 0.5°C and 85 ± 2% RH. Data represents means (n = 3 reps * 6 fruit per rep = 18) ± standard error of the mean. The canopy position*harvest time*storage time interaction was not significant, hence, LSD0.05 not shown.
3.5 Abscisic acid and its catabolites
ABA-GE was the main ABA catabolite found in clementines during storage, with ten times higher content than ABA ranging from 6 to 12 μg g-1 (Figure 6). Compared to April, there was greater variability in hormonal concentration between the different canopy positions for fruit harvested in March. At the same time, fruit located on the inside of the canopy exhibited two-fold higher concentration of ABA and its catabolites than fruit on the outside at the beginning of storage. In contrast, fruit harvested in April and located on the outside had more than 30% higher ABA and ABA-GE concentration than fruit on the inside part of the canopy. At the end of storage and independent of the harvest month, upper outside fruit showed the highest level of ABA-GE (11.1 µg g-1), while lower inside fruit had the lowest values (5.5 µg g-1).
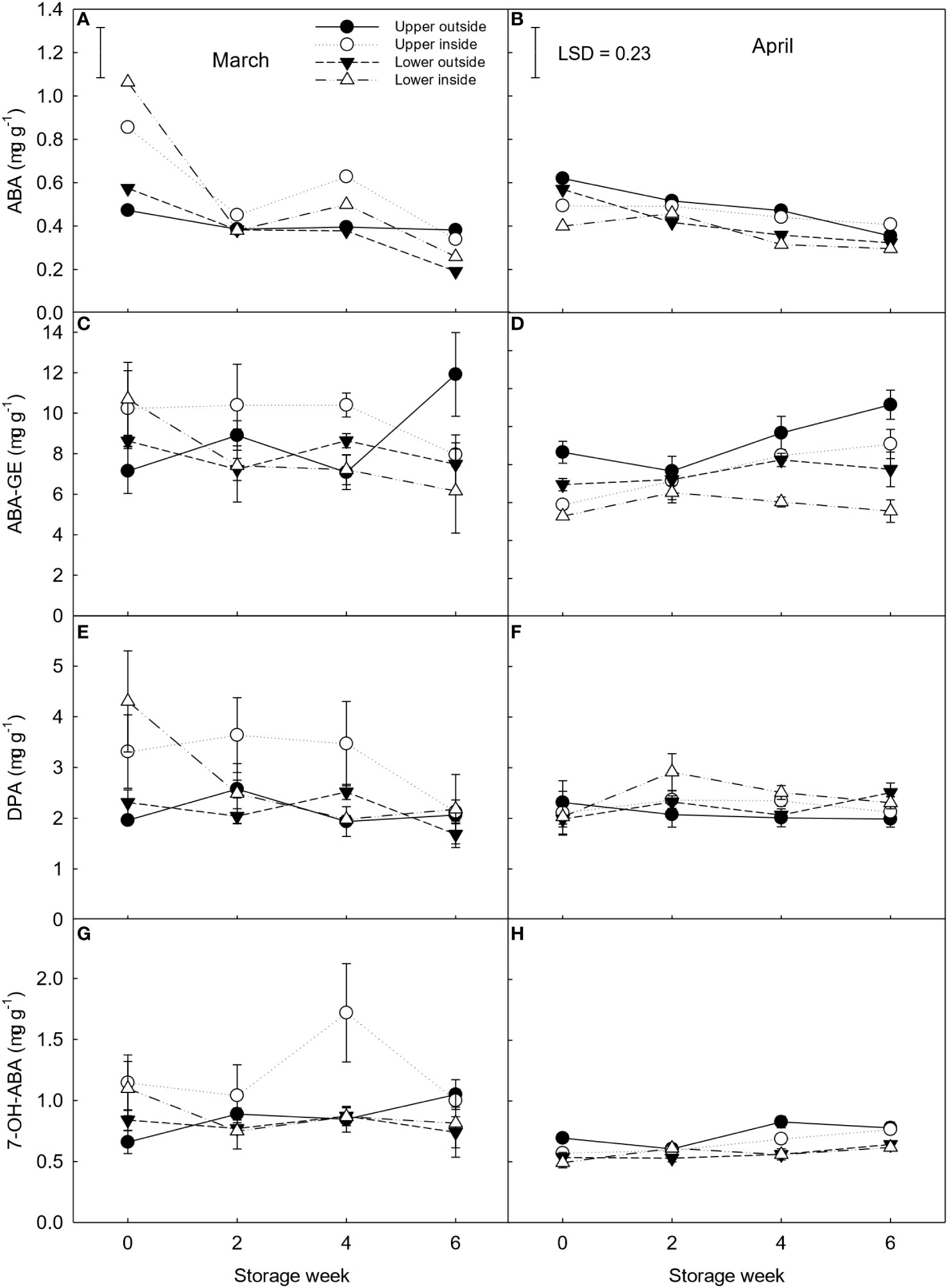
Figure 6 ABA (A, B) and ABA catabolites {ABA-GE (C, D), DPA (E, F) and 7-OHABA (G, H) expressed as μg g-1 DW of ‘Nadorcott’ clementines harvested in March (left) and April (right) 2017. The fruit were harvested from different canopy positions (upper outside, upper inside, lower outside and lower inside) and stored for six weeks at 8.5 ± 0.5°C and 85 ± 2% RH. Data represents means (n = 3 reps * 6 fruit per rep = 18) ± standard error of the means. For ABA, the LSD0.05 for canopy position*harvest time*storage time interaction was 0.66. For all other ABA metabolites, the canopy position*harvest time*storage time interaction was not significant, hence, LSD0.05 was not shown.
3.6 Sensory evaluation
The consumer tasting session showed that the canopy position significantly affected the overall acceptance of the fruit (Figure 7). Clementines located on the lower part of the canopy were preferred over the upper fruit among the consumers. Accordingly, the upper fruit obtained the lowest scores for flavour, juiciness, texture, and overall acceptance. Specifically, the upper inside fruit scored significantly lower (6-11%) for appearance than the rest of the samples and obtained the lowest scores for peelability, flavour, and overall acceptance.
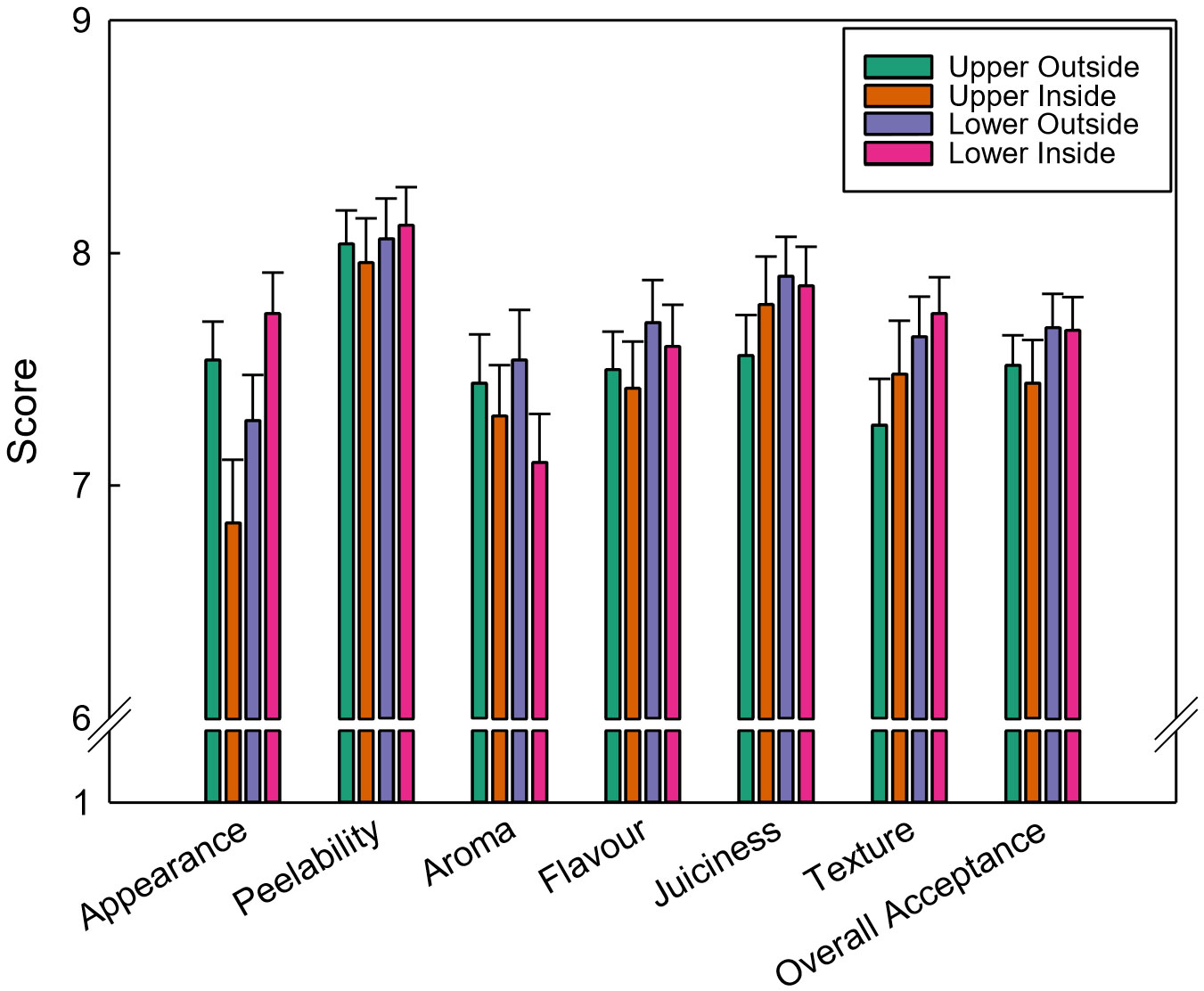
Figure 7 Results of the tasting session of ‘Nadorcott’ clementines carried out in March 2017. Consumers tasted one fruit per position and scored the individual eating quality attributes from 1 “Extremely dislike” to 9 “Extremely like”. Bars represent the standard error of the mean scores. The canopy position was not significant as main factor, hence, LSD0.05 has not been shown.
3.7 Buying decision survey
The results of the buying decision survey can be found in Table 1. The most important attribute for the panellists was flavour, followed by juiciness and sweetness. In contrast, the least important attributes were aroma and texture. Interestingly, appearance obtained the 5th position in importance. However, when consumers were asked to identify the attributes, they consider when buying citrus fruits, skin colour, appearance, and size had the highest number of responses (Supplementary Table 2).
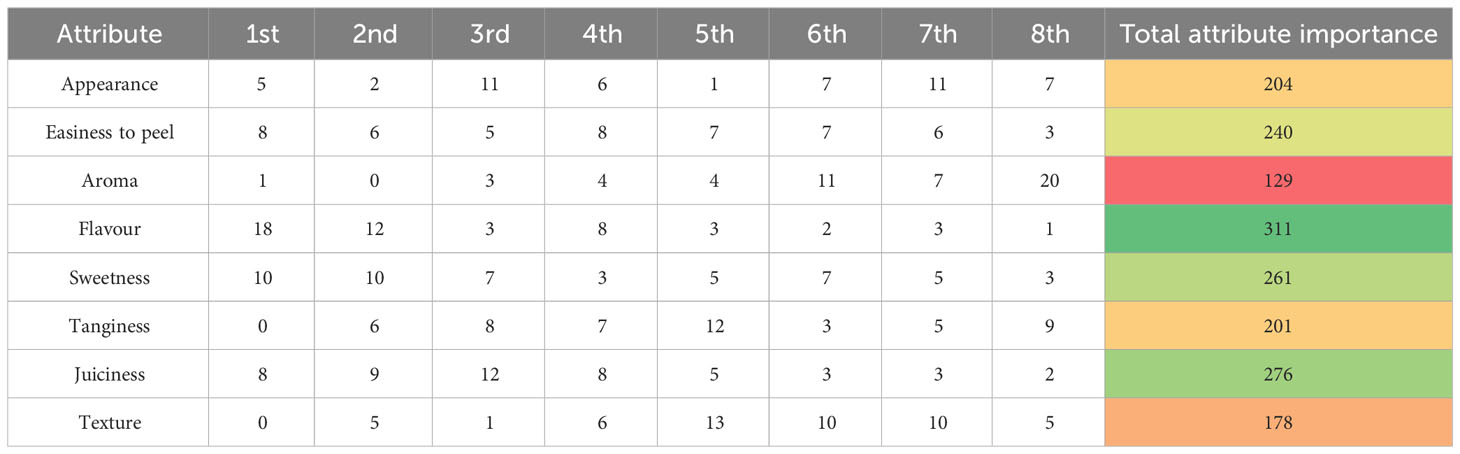
Table 1 Ranking of citrus attributes. ‘Nadorcot’ clementines were evaluated by a testing panel in March 2017. A total of 50 consumer panellists scored eight different mandarin attributes by order of importance. A final score per attribute was obtained ('Total attribute importance') which has been depicted in this table by a colour code from green to orange to red; where dark green represents the most important attribute and red the less important attribute.
4 Discussion
4.1 The effect of harvest time and canopy position on the postharvest quality and consumer experience of ‘Nadorcott’ clementines
The quality profile of citrus fruit is highly dependent on the horticultural maturity at harvest (Lado et al., 2018; Liu et al., 2021; Sun et al., 2021), and factors like fruit canopy position have been shown to affect the postharvest quality of citrus. Previous research has demonstrated the effect of these factors on the pre- and post-harvest quality of different citrus cultivars like ‘Kinnow’ mandarins (Thakre et al., 2015), ‘Nules’ clementines (Cronje et al., 2011a, 2011b; Magwaza et al., 2013; Ncama et al., 2018a, b), and ‘Iwasaki’, ‘Okitsu’, ‘Goku Wase’ satsumas (Sun et al., 2021) as well as ‘Navel’ and ‘Valencia’ oranges (Zacarías-García et al., 2022). However, to the best of our knowledge, this is the first time that the effect of fruit canopy position on consumer acceptance of ‘Nadorcott’ clementines has been studied.
‘Nadorcott’ is a seedless, mid to late-maturing clementine that is harvested from January to June in the Northern Hemisphere. This variety does not need degreening (postharvest treatment with ethylene to develop peel colour), since carotenoids, which give the orange colour to the citrus peel and juice, are developed naturally as the fruit ripens. Peel colour is therefore a determinant factor of both fruit maturity for the citrus growers and eating quality for the final consumer. Nawaz et al. (2020) recently studied the effect of colour break on the internal quality of ‘Kinnow’ mandarins at early ripening stages and demonstrated that green fruit had higher TA, ascorbic acid and lower TSS and sugars than yellow-coloured fruits. In this study, fruit harvested in March was brighter in colour, and had significantly lower juice content, TSS : TA ratio, individual sugars, and higher ascorbic acid than fruit harvested in April. However, the results showed that the rind of fruit harvested in March was redder and there was less variability in colour hue within canopy positions. This confirms previous studies where ‘Kinnow’ mandarins harvested in India during the last week of December had higher ascorbic acid and total carotenoid content in the peel and juice than fruit harvested two weeks later (Thakre et al., 2015). Similarly, early maturing cultivars of satsumas (‘Iwasaki’, ‘Okitsu’, and ‘Goku Wase’) had higher absorption of carotenoids in the juice vesicles and advanced colour changes in the flavedo than late-maturing (Sun et al., 2021).
Regarding canopy position, this study showed that outside (sun-exposed) fruit had higher TSS but lower juice content and colour lightness than inside (shadowed) fruit. This is in agreement with the results reported by Thakre et al. (2015) and Olarewaju et al. (2018), who found that fruit from the external canopy had higher TSS and total carotenoids than fruit from the internal canopy. From all the positions studied, fruit on the lower inside canopy showed the highest juice content and ascorbic acid, and the lowest TSS, TA, and fructose and glucose content, while the upper outside fruit had the opposite results. This means that, if harvested at the same time, upper outside fruit would be more ripe than lower inside fruit due to a more advanced degradation of ascorbic acid and sucrose, which may result in a shorter shelf-life (Khalid et al., 2017; Olarewaju et al., 2018).
This work has, for the first time, investigated the effect of harvest time and canopy position on the CO2 production of ‘Nadorcott’ clementines throughout the postharvest cold storage. The respiration rate and the potential postharvest life of fresh produce can depend on the content of respiratory substrates at the time of harvest (Khalid et al., 2017), as once detached from the mother plant the photosynthetic substrates no longer accumulate. Citrus fruits are generally considered non-climacteric since there is no peak in ethylene production and respiration at the beginning of fruit ripening (Alférez et al., 2021). However, some authors have reported a rise in ethylene production after harvest. For instance, Khalid et al. (2017) observed two ethylene peaks during the shelf-life of ‘Kinnow’ mandarins stored for seven days at ambient temperature, which were attributed to varietal effect, variation in fruit maturity, or even tree age. The same authors reported that CO2 production decreased throughout ambient storage, which contrasts with our results. Herein, although harvest time did not affect fruit RR during the first two weeks of storage, there was a significant increase in RR from week 4 to 6 in March. Interestingly, this change was observed two weeks earlier in fruit harvested in April, from week 2 to 4, and could be explained by differences in the climactic conditions before the two harvests. Meteorologic data for Lora del Rio, Seville (Supplementary Table 1), showed that the month preceding the second harvest (11th March – 7th April) had higher maximum temperature, solar radiation, and reference evapotranspiration values compared to the month before the first harvest (10th February – 10th March).
Cronje et al. (2011b) found that sunlight availability decreases within the first 10 cm into the canopy resulting in outside fruit having greater exposure to higher temperatures, and lower RH compared to the inside position. However, the authors stated that this would result in the outer fruit having a higher transpiration rate than the inside fruit due to higher atmospheric evaporative demand, which is contrary to our observations at the end of storage. Here, fruit located on the upper part of the canopy had significantly higher CO2 production on average than fruit located on the lower part. Moreover, inside (shadowed) fruit showed the highest RR at the end of storage. It could be thought that these fruits should be less stressed than those on the outside part of the canopy as they are not exposed to direct sunlight. Furthermore, the upper inside fruit showed the highest RR at the end of storage, probably caused by a higher osmotic pressure on the top of the canopy compared to the lower part and poor ventilation inside the canopy (Cronje et al., 2011a; Cronje and Barry, 2013).
The results of the tasting session carried out during the fourth week of the March harvest showed that consumers preferred the fruit located on the lower part of the canopy, which had higher juice content and TSS and lower TA than the upper fruit. This agrees with the survey results, which showed that consumers value flavour, juiciness, and sweetness more than other citrus attributes. When asked to rank the attributes they take into account at the moment of purchase, consumers positioned skin colour and appearance as the most important factors, demonstrating the mismatch between the attributes that citrus consumers consider for buying decisions and those for eating quality. This finding confirms the results reported by Poole and Baron (1996), where the most important characteristics for citrus consumers were juiciness, skin quality, sweetness, and texture, of which only skin quality can be perceived at the moment of purchase.
Overall, ‘Nadorcott’ clementines harvested in the middle of the season (March) had less physiological and biochemical variability between the different canopy positions throughout storage than fruit harvested in April. This suggests that, if there is no differentiation in canopy positions at the moment of harvest, fruit picked in March would have more consistent quality than in April. In contrast, when harvesting at the end of the season, the variability in fruit quality for the different canopy positions will be higher, making it challenging for the citrus industry to supply a product with the consistency of quality and flavour throughout the season. To mitigate these variations between the different canopy positions, the implementation of shading nets could be an effective solution. As reported by Lee et al. (2015) and later confirmed by Cronjé et al. (2020), the use of white shade nets in ‘Ponkan’ mandarin farms produced a significant reduction in fruit surface temperature and sunburn, higher juice percentage, and lower weight loss and decay than in non-shaded fruit while postharvest peel colour, TSS and TA were unaffected. Therefore, the use of shading nets could decrease the variability in temperature, sunlight exposure, and transpiration of the different canopy positions and, as a result, improve the consistency in fruit quality at harvest.
4.2 The role of ABA and ABA catabolites on clementine senescence
ABA is a phytohormone that is synthesised in higher plants from C40 epoxycarotenoids, natural pigments that give the red-orange colour to plants, through several oxidation steps regulated by the enzyme 9-cis-Epoxycarotenoid Dioxygenase (NCED) (Endo et al., 2014; Zacarías-García et al., 2022; Álvarez et al., 2023). Alongside ethylene, ABA regulates physiological processes such as fruit maturation, ripening, and stress adaptation in both climacteric and non-climacteric produce. However, previous studies have demonstrated that ABA has a greater role in regulating ripening and abscission than ethylene in non-climacteric fruit (Jia et al., 2016; Wang et al., 2016; Magwaza et al., 2019; Alférez et al., 2021; Garcia-Pastor et al., 2021; Navarro-Calderón et al., 2023). At the same time, several authors have confirmed the implication of ABA in citrus rind quality (Lafuente and Sala, 2002; Magwaza et al., 2019), response to water stress and dehydration (Romero et al., 2013), and mould infection (Lafuente et al., 2021). However, very few studies have focused their attention on the changes in endogenous ABA and ABA catabolites during the postharvest life of citrus fruit and their role in citrus pulp quality. In addition, and to the best of our knowledge, there are no published studies relating the endogenous ABA and catabolites concentration in the citrus pulp with fruit canopy position and consumer acceptance.
Wang et al. (2016) reported that preharvest application of exogenous ABA to ‘Ponkan’ mandarins significantly affected the ripening of citrus fruit by accelerating fruit colouring and decreasing the organic acid content. Moreover, ABA regulated the expression of most sugar-related genes, confirming the coordinated role of ABA and sugars in regulating citrus fruit ripening. The results presented herein supported these findings, since both the organic acid and the ABA content in the pulp declined throughout storage and season, while sugars demonstrated the opposite behaviour. Interestingly, the levels of the catabolite ABA-GE were ten times higher than those of ABA and increased over time. This coincides with the results reported by Magwaza et al. (2019), who observed that the ABA-GE content in citrus rind increased throughout postharvest storage. Moreover, the ABA-GE content in the pulp reported here was four times higher than those in the citrus rind observed by Magwaza et al. (2019). These observations could be explained by the fact that the maturation processes of the citrus rind and pulp are considered autonomous and not coordinated (Lado et al., 2018; Sadka et al., 2019; Zacarías-García et al., 2022), and higher ABA catabolites in the pulp than in the rind could indicate that the pulp is overmature and that senescence processes in the pulp could have started much earlier than in the rind.
The citrus rind has previously been regarded as a modified leaf (Cronje et al., 2011a). As such, photoassimilates, mainly sucrose, are synthesised in the chloroplasts during the early stages of development when the fruit is still green. Then, chloroplasts in the citrus fruit epicarp are converted to chromoplasts in order to develop external colour through the synthesis of carotenoids, which are the precursors of ABA. This chloroplast to chromoplast conversion is stimulated by sucrose accumulation (Iglesias et al., 2007; Tadeo et al., 2020), which is in turn exported from the leaves to the vacuoles of the juice sacs in the citrus pulp through the transport phloem (Lado et al., 2018). However, the juice sacs are disconnected from the fruit vascular system ending in the albedo (Sadka et al., 2019), therefore a translocation of sucrose and other solutes back to the citrus rind would be unlikely. At the same time, it has been reported that ABA can induce its biosynthesis in the juice sacs (Lado et al., 2018). These facts may explain the higher nutrient and hormonal content in the citrus pulp compared to the rind.
Previously, a higher level of ABA catabolites has been related to a higher moisture loss and rind breakdown disorder (RBD) development in the citrus rind (Magwaza et al., 2019), which could explain the low score in the external appearance of the upper inside fruit. When analysing the hormonal content in the pulp of fruit from different canopy positions, the upper inside samples showed significantly higher levels of ABA catabolites than the rest, and this position, interestingly, scored significantly lower than the others for external appearance during the consumers’ tasting session. Apart from this, no significant differences were observed for any of the quality attributes evaluated by the consumers between the different canopy positions.
5 Conclusions
This study has demonstrated that canopy position has an effect on the postharvest quality of ‘Nadorcott’ clementines, specifically on external appearance, which is one of the main citrus attributes that consumers take into account at the moment of purchasing. Fruit located on the upper inside part of the canopy obtained the lowest consumer score for external appearance and had the highest levels of ABA catabolites.
ABA-GE has been shown to have a greater role in the citrus senescence processes and the external quality of ‘Nadorcott’ clementine mandarin fruit than ABA, demonstrating that ABA catabolites could be used as potential biomarkers for assessing postharvest resilience and consumer acceptance. Establishing whether changes in endogenous ABA catabolites and sugar concentration are triggers of the different senescence mechanisms would require additional experimental evidence. Therefore, it is recommended for subsequent studies to concentrate on elucidating the changes in ABA-GE levels during the ripening and senescence of citrus fruit to establish the hormonal content mechanism at different stages of citrus shelf-life and its correlation with consumer acceptance.
At the same time, canopy position should be considered a determining factor of quality at harvest and be included in agricultural practices for two reasons. Firstly, to improve the consistency of citrus flavour and quality throughout the season; and secondly, to meet retail specifications and consumer needs. For this, the implementation of shading nets in the field needs further testing.
Data availability statement
The original contributions presented in the study are included in the article and Supplementary Files. The datasets generated and analysed in this study can be found in CORD using the following link: http://doi.org/10.17862/cranfield.rd.24600321. Further inquiries can be directed to the corresponding author.
Author contributions
ÁN: Conceptualization, Investigation, Data curation, Formal analysis, Methodology, Software, Visualization, Writing – original draft. LM: Writing – review & editing. LT: Writing – review & editing, Conceptualization, Funding acquisition, Project administration, Resources, Supervision. MA: Conceptualization, Project administration, Resources, Supervision, Writing – review & editing, Investigation.
Funding
The author(s) declare financial support was received for the research, authorship, and/or publication of this article. We thank AMFRESH Group and Cranfield University for sponsoring this project through the Cranfield Industrial Partnership PhD Scheme.
Acknowledgments
The Authors would like to thank Sam Franklin for his technical support during the project.
Conflict of interest
The authors declare that the research was conducted in the absence of any commercial or financial relationships that could be construed as a potential conflict of interest.
The authors declare that this study received funding from AMFRESH. The funder was not involved in the study design, collection, analysis, interpretation of data or the writing of this article.
Publisher’s note
All claims expressed in this article are solely those of the authors and do not necessarily represent those of their affiliated organizations, or those of the publisher, the editors and the reviewers. Any product that may be evaluated in this article, or claim that may be made by its manufacturer, is not guaranteed or endorsed by the publisher.
Supplementary material
The Supplementary Material for this article can be found online at: https://www.frontiersin.org/articles/10.3389/fhort.2024.1353070/full#supplementary-material
References
Alférez F., de Carvalho D. U., Boakye D. (2021). Interplay between abscisic acid and gibberellins, as related to ethylene and sugars, in regulating maturation of nonclimacteric fruit. Int. J. Mol. Sci. 22, 1–10. doi: 10.3390/ijms22020669
Álvarez F., Moya M., Rivera-Mora C., Zúñiga P. E., Jara-Cornejo K., Muñoz P., et al. (2023). Abscisic acid synthesis and signaling during the ripening of raspberry (Rubus idaeus 'Heritage') fruit. Plants (Basel) 12, 1882. doi: 10.3390/plants12091882
Bureš M. S., Bandić L. M., Vlahoviček-Kahlina K. (2023). Determination of bioactive components in mandarin fruits: A Review. Crit. Rev. Anal. Chem. 53, 1489–1514. doi: 10.1080/10408347.2022.2035209
Collings E. R., Alamar Gavidia M. C., Cools K., Redfern S., Terry L. A. (2018). Effect of UV-C on the physiology and biochemical profile of fresh Piper nigrum berries. Postharvest Biol. Technol. 136, 161–165. doi: 10.1016/j.postharvbio.2017.11.007
Conesa M. R., Falagán N., de la Rosa J. M., Aguayo E., Domingo R., Pérez Pastor A. (2016). Post-veraison deficit irrigation regimes enhance berry coloration and health-promoting bioactive compounds in ´Crimson Seedless´ table grapes. Agric. Water Manage. 163, 9–18. doi: 10.1016/j.agwat.2015.08.026
Cronje P. L. R., Barry G. H. (2013). Canopy position affects pigment expression and accumulation of flavedo carbohydrates of ‘Nules Clementine’ mandarin fruit, thereby affecting rind condition. J. Am. Soc Hortic. Sci. 138, 217–224. doi: 10.21273/JASHS.138.3.217
Cronje P. L. R., Barry G. H., Huysamer M. (2011a). Postharvest rind breakdown of ‘Nules Clementine’ mandarin is influenced by ethylene application, storage temperature and storage duration. Postharvest Biol. Technol. 60, 192–201. doi: 10.1016/j.postharvbio.2011.01.009
Cronje P. L. R., Barry G. H., Huysamer M. (2011b). Fruiting position during development of ‘Nules Clementine’ mandarin affects the concentration of K, Mg and Ca in the flavedo. Sci. Hortic. 130, 829–837. doi: 10.1016/j.scienta.2011.09.011
Cronjé P. J. R., Botes J., Prins D. M., Brown R., North J., Stander O. P. J., et al. (2020). The influence of 20% white shade nets on fruit quality of 'Nadorcott' mandarin. Acta Hortic. 1268, 279–284. doi: 10.17660/ActaHortic.2020.1268.36
Davis F., Terry L. A., Chope G. A., Faul C. F. J. (2007). Effect of extraction procedure on measured sugar concentrations in onion (Allium cepa L.) bulbs. J. Agric. Food Chem. 55, 42994306. doi: 10.1021/jf063170p
Endo A., Okamoto M., Koshiba T. (2014). “ABA biosynthetic and catabolic pathways,” in Abscisic Acid: Metabolism, Transport and Signaling. Ed. Zhang D. P. (Springer, Dordrecht), 21–45.
Gao Z., House L. O., Gmitter F. G. Jr., Valim M. F., Plotto A., Baldwin E. A. (2011). Consumer preferences for fresh citrus: impacts of demographic and behavioural characteristics. Int. Food Agribus. Manage. Rev. 14 (1), 23–40.
Garcia-Pastor M. E., Falagan N., Gine-Bordonaba J., Wojcik D. A., Terry L. A., Alamar M. C. (2021). Cultivar and tissue-specific changes of abscisic acid, its catabolites and individual sugars during postharvest handling of flat peaches (Prunus persica cv. platycarpa). Postharvest Biol. Technol. 181, 111688. doi: 10.1016/j.postharvbio.2021.111688
Goldenberg L., Yaniv Y., Kaplunov T., Doron-Faigenboim A., Carmi N., Porat R. (2015). Diversity in sensory quality and determining factors influencing mandarin flavour liking. J. Food Sci. 80, 418–425. doi: 10.1111/1750-3841.12742
Guo H., Zheng Y.-J., Wu D.-T., Du X., Gao H., Ayyash M., et al. (2023). Quality evaluation of citrus varieties based on phytochemical profiles and nutritional properties. Front. Nutr. Sec. Food Chem. 10. doi: 10.3389/fnut.2023.1165841
Gupta A. K., Dhua S., Thakur R., Ncama K., Sithole N. J., Magwaza L. S., et al. (2023). “Orange,” in Fruits and Their Roles in Nutraceuticals and Functional Foods, 1st ed. Eds. Ahmad Wani S., Kour J., Haq R.-U., Elshikh M. S. (Boca Raton, Florida, USA: CRC Press). doi: 10.1201/9781003259213
Iglesias D. J., Cercós M., Colmenero-Flores J. M., Naranjo M. A., Ríos G., Carrera E., et al. (2007). Physiology of citrus fruiting. Braz. J. Plant Physiol. 19, 333–362. doi: 10.1590/S1677-04202007000400006
Jia H., Jiu S., Zhang C., Wang C., Tariq P., Liu Z., et al. (2016). Abscisic acid and sucrose regulate tomato and strawberry fruit ripening through the abscisic acid-stress-ripening transcription factor. Plant Biotechnol. J. 14, 2045–2065. doi: 10.1111/pbi.12563
Kemp S. E., Hollowood T., Hort J. (2009). Sensory evaluation, a practical handbook (Chichester, UK: WileyBlackwell). 196 pp. doi: 10.1002/9781118688076
Khalid S., Malik A. U., Khan A. S., Khan M. N., Ullah M. I., Abbas T., et al. (2017). Tree age and fruit size in relation to postharvest respiration and quality changes in ‘Kinnow’ mandarin fruit under ambient storage. Sci. Hortic. 220, 183–192. doi: 10.1016/j.scienta.2017.03.042
Khan A. S., Malik A. U., Pervez M. A., Saleem B. A., Rajwana I. A., Shaheen T., et al. (2009). Foliar application of low-biuret urea and fruit canopy position in the tree influence the leaf nitrogen status and physico-chemical characteristics of Kinnow mandarin (Citru reticulata Blanco). Pak. J. Bot. 41 (1), 73–85.
Lado J., Gambetta G., Zacarias L. (2018). Key determinants of citrus fruit quality: Metabolites and main changes during maturation. Sci. Hortic. 233, 238–248. doi: 10.1016/j.scienta.2018.01.055
Lafuente M. T., Ballester A. R., Holland N., Cerveró J., Romero P. (2021). Interrelation between ABA and phospholipases D, C and A2 in early responses of citrus fruit to Penicillium digitatum infection. Postharvest Biol. Technol. 175, 111475. doi: 10.1016/j.postharvbio.2021.111475
Lafuente M. T., Sala J. M. (2002). Abscisic acid levels and the influence of ethylene, humidity and storage temperature on the incidence of postharvest rindstaning of ‘Navelina’ orange (Citrus sinensis L. Osbeck) fruit. Postharvest Biol. Technol. 25, 49–57. doi: 10.1016/S0925-5214(01)00162-4
Lee T.-C., Zhong P.-J., Chang P.-T. (2015). The effects of preharvest shading and postharvest storage temperatures on the quality of ‘Ponkan’ (Citrus reticulata Blanco) mandarin fruits. Sci. Hortic. 188, 57–65. doi: 10.1016/j.scienta.2015.03.016
Liu X., Wang Y., Kang F., Yue Y., Zheng Y. (2021). Canopy Parameter Estimation of Citrus grandis var. Longanyou Based on LiDAR 3D Point Clouds. Remote Sens. 13, 1859. doi: 10.3390/rs13091859
Magwaza L. S., Carmen Alamar M., Tesfay S. Z., Mditshwa A., Opara U. L., Terry L. A. (2019). Investigating the involvement of ABA, ABA catabolites and cytokinins in the susceptibility of ‘Nules Clementine’ mandarin to rind breakdown disorder. J. Sci. Food Agric. 99, 4142–4149. doi: 10.1002/jsfa.9644
Magwaza L. S., Opara U. L. (2015). Analytical methods for determination of sugars and sweetness of horticultural products - A review. Sci. Hortic. 184, 179–192. doi: 10.1016/j.scienta.2015.01.001
Magwaza L. S., Opara U. L., Nieuwoudt H. H., Cronjé P. J. R., Landahl S., Terry L. A. (2013). Quantifying the effects of fruit position in the canopy on physical and biochemical properties and predicting susceptibility to rind breakdown disorder of ‘Nules Clementine’ mandarin (Citrus reticulate Blanco) using Vis/NIR spectroscopy. Acta Hortic. 1007, 83–92. doi: 10.17660/ActaHortic.2013.1007.6
Mditshwa A., Magwaza L. S., Tesfay S. Z., Opara U. L. (2017). Postharvest factors affecting vitamin C content of citrus fruits: A review. Sci. Hortic. 218, 95–104. doi: 10.1016/j.scienta.2017.02.024
Moon D. G., Joa J. H., Moon Y. E., Seong K. C., Kim C. H., Ahn Y. K. (2011). Plant growth and fruit quality as affected by canopy locations in ‘Shiranuhi’ mandarin. Hortic. Environ. Biotechnol. 52, 443–447. doi: 10.1007/s13580-011-0004-0
Morris W. L., Alamar M. C., López-Cobollo R. M., Castillo J. C., Bennett M., van der Kaay J., et al. (2018). A member of the TERMINAL FLOWER1/CENTRORADIALIS gene family controls sprout growth in potato tubers. J. Exp. Bot. 70, 835–843. doi: 10.1093/jxb/ery387
Navarro-Calderón Á., Falagán N., Terry L. A., Alamar M. C. (2023). Biomarkers of postharvest resilience: unveiling the role of abscisic acid in table grapes during cold storage. Front. Plant Sci. 14. doi: 10.3389/fpls.2023.1266807
Nawaz R., Abbasi N. A., Ahmad Hafiz I., Khalid A. (2020). Impact of climate variables on fruit internal quality of ‘Kinnow’ mandarin (Citrus nobilis Lour x Citrus deliciosa Tenora) in ripening phase grown under varying environmental conditions. Sci. Hortic. 265, 109235. doi: 10.1016/j.scienta.2020.109235
Ncama K., Magwaza L. S., Fawole O. A., Tesfay S. Z., Opara U. L. (2018a). Investigating pre-symptomatic biochemical markers related to 'Marsh' grapefruit (Citrus × paradisi Macfad) susceptibility to chilling injury and rind pitting disorders. Acta Hortic. 1201, 131–138. doi: 10.17660/ActaHortic.2018.1201.18
Ncama K., Tesfay S. Z., Fawole O. A., Opara U. L., Magwaza L. S. (2018b). Non-destructive prediction of ‘Marsh’ grapefruit susceptibility to postharvest rind pitting disorder using reflectance Vis/NIR spectroscopy. Sci. Hortic. 231, 265–271. doi: 10.1016/j.scienta.2017.12.028
Olarewaju O. O., Magwaza L. S., Fajinmia O. O., Fawole O. A., Plačková L., Doležal K. (2022). Changes in cytokinins and auxins levels in the rind of ‘Nules Clementine’ mandarin as related to the fruit position on the tree and the susceptibility to non-chilling rind breakdown disorder. S. Afr. J. Bot. 151, 667–674. doi: 10.1016/j.sajb.2022.10.046
Olarewaju O. O., Magwaza L. S., Fawole O. A., Tesfay S. Z., Opara U. L. (2017). Role of canopy positions on rind biochemical concentrations and radical-scavenging activities in relation to rind breakdown of ‘Nules Clementine’ mandarins stored at non-chilling temperature. Sci. Hortic. 226, 231–240. doi: 10.1016/j.scienta.2017.08.048
Olarewaju O. O., Magwaza L. S., Fawole O. A., Tesfay S. Z., Opara U. L. (2018). Comparative effects of canopy position on physicochemical properties of ‘Marsh’ grapefruit during non-chilling postharvest cold storage. Sci. Hortic. 241, 1–7. doi: 10.1016/j.scienta.2018.06.074
Poole N., Baron L. (1996). Consumer awareness of citrus fruit attributes. Br. Food J. 98, 23–28. doi: 10.1108/00070709610111296
Rokaya P. R., Baral D. R., Gautam D. M., Shrestha A. K., Paudyal K. P. (2016). Effect of altitude and maturity stages on quality attributes of mandarin (Citrus reticulata Blanco). Am. J. Plant Sci. 07, 958–966. doi: 10.4236/ajps.2016.76091
Romero P., Gandía M., Alférez F. (2013). Interplay between ABA and phospholipases A2 and D in the response of citrus fruit to postharvest dehydration. Plant Physiol. Biochem. 70, 287–294. doi: 10.1016/j.plaphy.2013.06.002
Rosa M., Prado C., Podazza G., Interdonato R., González J. A., Hilal M., et al. (2009). Soluble sugars-metabolism, sensing and abiotic stress a complex network in the life of plants. Plant Signal. Behav. 4, 388–393. doi: 10.4161/psb.4.5.8294
Sadka A., Shlizerman L., Kamara I., Blumwald E. (2019). Primary metabolism in citrus fruit as affected by its unique structure. Front. Plant Sci. 10. doi: 10.3389/fpls.2019.01167
Sawicki M., Aït Barka E., Clément C., Vaillant-Gaveau N., Jacquard C. (2015). Crosstalk between environmental stresses and plant metabolism during reproductive organ abscission. J. Exp. Bot. 66, 1707–1719. doi: 10.1093/jxb/eru533
Serradilla M. J., Falagán N., Bohmer B., Terry L. A., Alamar M. C. (2019). The role of ethylene and 1-MCP in early-season sweet cherry ‘Burlat’ storage life. Sci. Hortic. 258, 108787. doi: 10.1016/j.scienta.2019.108787
Sun C., Aernouts B., Saeys W. (2021). Effects of harvest time, fruit size and cultivar on the bulk optical properties of Satsuma mandarin. Postharvest Biol. Technol. 175, 111412. doi: 10.1016/j.postharvbio.2020.111412
Tadeo F. R., Terol J., Rodrigo M. J., Licciardelo C., Sadka A. (2020). “Fruit growth and development,” in The genus citrus. Eds. Talón M., Caruso M., Gmitter F. (Woodhead Publishing, Cambridge, UK), 245–289.
Terry L. A., Chope G. A., Giné Bordonaba J. (2007). Effect of water deficit irrigation and inoculation with Botrytis cinerea on strawberry (Fragaria x ananassa) fruit quality. J. Agric. Food Chem. 55, 10812–10819. doi: 10.1021/jf072101n
Terry L., Mena C., Williams A., Jenney N., Whitehead P. (2011). Fruit and vegetable resource maps. Wrap, 1–94.
Thakre M., Verma M. K., Singh K., Awasthi O. P., Verghese E., Sharma V. K. (2015). Effect of nutrition, harvesting date and fruit canopy position on yield and quality of Kinnow Mandarin (Citrus nobilis × Citrus deliciosa). Indian J. Agric. Sci. 85, 1455–1460. doi: 10.56093/ijas.v85i11.53700
Wang X., Yin W., Wu J., Chai L., Yi H. (2016). Effects of exogenous abscisic acid on the expression of citrus fruit ripening-related genes and fruit ripening. Sci. Hortic. 201, 175–183. doi: 10.1016/j.scienta.2015.12.024
Keywords: ABA catabolites, citrus, ethylene, canopy position, respiration rate, senescence, sugars
Citation: Navarro-Calderón Á, Magwaza LS, Terry LA and Alamar MC (2024) Unveiling biomarkers for postharvest resilience: the role of canopy position on quality and abscisic acid dynamics of ‘Nadorcott’ clementine mandarins. Front. Hortic. 3:1353070. doi: 10.3389/fhort.2024.1353070
Received: 09 December 2023; Accepted: 19 February 2024;
Published: 13 March 2024.
Edited by:
Brian Farneti, Fondazione Edmund Mach, ItalyReviewed by:
María Emma García Pastor, Miguel Hernández University of Elche, SpainVasileios Ziogas, Hellenic Agricultural Organization DEMETER, Greece
Copyright © 2024 Navarro-Calderón, Magwaza, Terry and Alamar. This is an open-access article distributed under the terms of the Creative Commons Attribution License (CC BY). The use, distribution or reproduction in other forums is permitted, provided the original author(s) and the copyright owner(s) are credited and that the original publication in this journal is cited, in accordance with accepted academic practice. No use, distribution or reproduction is permitted which does not comply with these terms.
*Correspondence: M. Carmen Alamar, m.d.alamargavidia@cranfield.ac.uk