Conservation of shibire and RpII215 temperature-sensitive lethal mutations between Drosophila and Bactrocera tryoni
- 1School of BioSciences, University of Melbourne, Melbourne, VIC, Australia
- 2School of Biological Sciences, University of Adelaide, Adelaide, SA, Australia
The sterile insect technique can suppress and eliminate population outbreaks of the Australian horticultural pest, Bactrocera tryoni, the Queensland fruit fly. Sterile males mate with wild females that produce inviable embryos, causing population suppression or elimination. Current sterile insect releases are mixed sex, as the efficient removal of unrequired factory-reared females is not yet possible. In this paper, we assessed the known Drosophila melanogaster temperature-sensitive embryonic lethal alleles shibire (G268D, shits1) and RNA polymerase II 215 (R977C, RpII215ts) for potential use in developing B. tryoni genetic sexing strains (GSS) for the conditional removal of females. Complementation tests in D. melanogaster wild-type or temperature-sensitive genetic backgrounds were performed using the GAL4–UAS transgene expression system. A B. tryoni wild-type shibire isoform partially rescued Drosophila temperature lethality at 29°C by improving survivorship to pupation, while expressing B. tryoni shits1 failed to rescue the lethality, supporting a temperature-sensitive phenotype. Expression of the B. tryoni RpII215 wild-type protein rescued the lethality of D. melanogaster RpII215ts flies at 29°C. Overexpressing the B. tryoni RpII215ts allele in the D. melanogaster wild-type background unexpectedly produced a dominant lethal phenotype at 29°C. The B. tryoni shibire and RpII215 wild-type alleles were able to compensate, to varying degrees, for the function of the D. melanogaster temperature-sensitive proteins, supporting functional conservation across species. Shibire and RpII215 hold potential for developing insect strains that can selectively kill using elevated temperatures; however, alleles with milder effects than shits1 will need to be considered.
1 Introduction
Insect pests pose a constant and significant threat to the agriculture sector and animal livestock industries, as they can cause serious negative impacts on productivity, impose market access restrictions, and threaten food security. Integrated pest management strategies aim to keep insect pest populations below levels that cause economic loss by combining environmentally acceptable control methods and sustainable farming techniques (1–3). These strategies help reduce the use of insecticides, which often have adverse effects on non-target animals and result in the development of insecticide resistance in pest populations (4, 5). The sterile insect technique (SIT) is an efficient species-specific insect biocontrol strategy that is used as a component of integrated pest management (3). SIT involves the intentional release of large numbers of sterile insects into regions with low to moderate pest density to decrease or eliminate pest populations (6). Radiation exposure to factory-reared pupae causes irreparable chromosomal damage and sterility. Once development is complete, the sterile insects are released, and males that mate with wild females produce inviable embryos, causing reproductive failure and population suppression. SIT was first developed to control New World screwworm (7) and has since successfully been used to combat numerous pest species worldwide, including several species of tephritid fruit flies (8), moths (9), tsetse flies (10), and mosquitoes (10, 11).
The Queensland fruit fly, Bactrocera tryoni (Diptera: Tephritidae), can infest more than 200 fruits and vegetables and is the most significant pest threat to Australian horticultural industries (12). Females oviposit into soft and ripening host fruit, resulting in puncture wounds that are susceptible to microbial infection, and larvae feed on internal flesh, causing severe damage (12). Controlling B. tryoni has traditionally relied on trapping systems containing attractants or broad-spectrum insecticides that have posed environmental and human health concerns (13). SIT has been applied in the management of B. tryoni to restrict reproduction and eliminate outbreaks (14). The current SIT approach for B. tryoni releases both sterile males and females, although females are not required. Developing strategies for sterile male-only release will significantly enhance the efficacy and cost-effectiveness of the program, which has been proven in another tephritid species, the Mediterranean fruit fly (Medfly), Ceratitis capitata (15).
Medfly SIT programs have benefited from genetic sexing strains (GSS) that enable male-only releases. GSS females are homozygous for a temperature-sensitive lethal (tsl) mutation causing embryonic death when treated at 34°C for 24 h, whereas males survive heat treatment as they carry a wild-type chromosomal genetic region translocated to the Y chromosome (16, 17). The Medfly GSS has taken decades to develop (8), and the tsl was generated by random mutagenesis, with its genetic nature remaining unknown (18). This female conditional lethal strategy has the potential to be replicated in other tephritid species using precise CRIPSR/Cas9 genome editing technology (19); however, this would require identification of the Medfly tsl mutation and its ortholog in the target species. An alternative strategy is the utilization of known temperature-sensitive mutations that have been characterized in the vinegar fly, Drosophila melanogaster (20–22).
Three key requirements exist for temperature-sensitive mutations to be considered as potential candidates for the development of an ideal GSS: i) Lethality should occur at early developmental stages, when embryos are treated at a restrictive temperature; ii) homozygous mutant females must be viable and fecund at permissive rearing temperatures for large-scale production; and iii) the conditional mutation should be recessive so that male fitness can be rescued using a wild-type allele translocated to the Y chromosome. A number of temperature-sensitive mutations have been identified in D. melanogaster (20, 23–25), yet only a few of them meet these three important requirements (26), e.g., shibire (shi) and the RNA polymerase II 215 (RpII215) genes (Table 1).
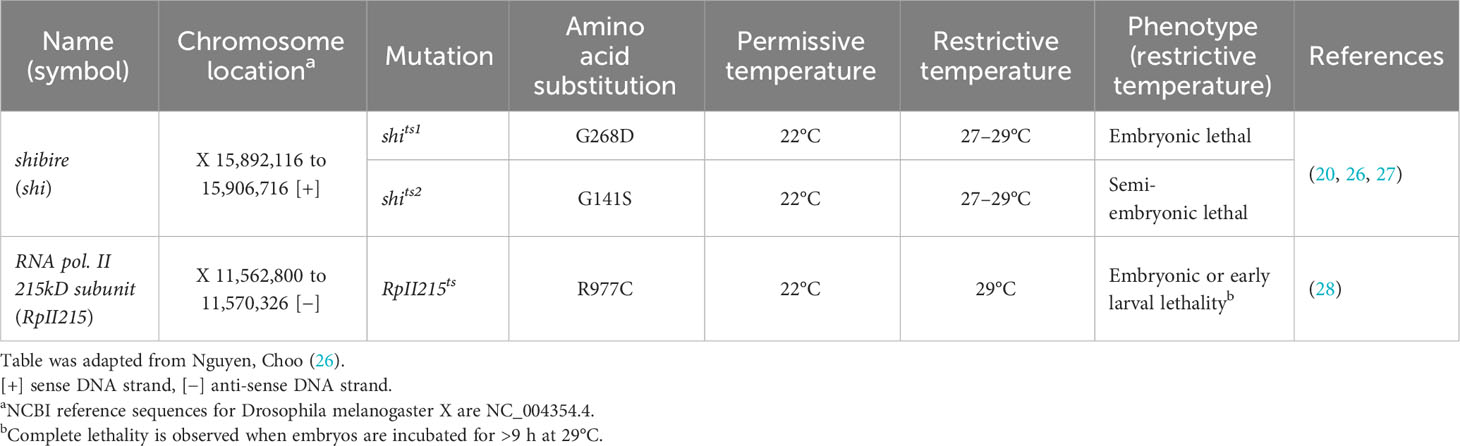
Table 1 Characterized Drosophila melanogaster temperature-sensitive mutations with potential to cause embryonic lethality when introduced into other insect species.
Using the mutagen ethyl methanesulfonate (EMS), Grigliatti et al. (20) isolated six temperature-sensitive mutations in the D. melanogaster X-linked shibire locus (shits1 to shits6), which encodes a GTPase dynamin protein involved in synaptic vesicle recycling in nerve terminals (29). Four of these mutations (shits1, shits2, shits3, and shits6) had been reported to have partial or complete embryonic lethality at 29°C and are unaffected when reared at 22°C (20). The D. melanogaster shits1 (G268D) and shits2 (G141S) alleles are point mutations resulting in amino acid substitutions at the boundary of the shibire GTPase domain (30) and also cause paralysis at the larval and adult stages at 29°C (20, 27). The mutations underlying shits3 and shits6 have never been reported.
Heat treatment of shits2 embryos at 29°C results in a less severe phenotype than shits1 (26). Individually expressing six different shibire wild-type isoforms in a shits2 background revealed that specific isoforms were able to rescue some of the temperature-sensitive phenotypes—this includes rescuing the lethality of shits2 larvae subjected to heat pulses late in development (at 32°C, 34°C, and some at 36°C) and adult paralysis at 27°C (31). The rescue of adult paralysis was considered partial as the rescue flies were still paralyzed at higher temperatures of 30°C and 32°C, suggesting that a single isoform of the gene may not be sufficient to rescue completely (31).
The X-linked gene RpII215 encodes for a subunit of RNA polymerase II, a multi-subunit enzyme catalyzing the transcription process to synthesize various types of RNA (32). The RpII215 temperature-sensitive (RpII215ts) mutation was induced using EMS by Mortin and Kaufman (28). Initial reports showed that the RpII215ts mutants were viable at the permissive temperature of 22°C, whereas shifting embryos to a high temperature of 29°C resulted in lethality at the late embryo or first larval instar stages (23, 28). Nguyen et al. (26) found that 25°C is also a suitable permissive temperature for rearing RpII215ts homozygous mutants. The RpII215ts mutation is a single-base substitution resulting in an amino acid replacement (R977C) located in domain 6, which forms part of the shelf module (33, 34).
Here, we used the D. melanogaster GAL4-UAS system to perform complementation tests by expressing B. tryoni transgenes for shibire or RpII215 in Drosophila temperature-sensitive genetic backgrounds to determine whether phenotypic rescue could be achieved. We hypothesized that phenotypic rescue would occur at 29°C through the expression of B. tryoni wild-type alleles and that synthetic alleles containing shits1 (G268D) or RpII215ts (R977C) substitutions would fail to rescue. This work advances our understanding of temperature-sensitive alleles in B. tryoni and provides new candidates for developing GSS for SIT.
2 Materials and methods
2.1 Drosophila melanogaster strains
The fly strains used in this study are listed in Supplementary Table S1. All stocks were maintained on standard cornmeal media at 25°C with 65% relative humidity at a 12:12 light/dark cycle unless described otherwise. Crosses investigating shibire temperature sensitivity were performed in the shits2 (DGRC 106754) background, as attempts to obtain a D. melanogaster shits1 stock (DGRC 106278) that survived the shipping process from the Bloomington Drosophila Stock Center (BDSC) or Kyoto Stock Center (DGRC) were unsuccessful.
2.2 Cloning of full-length Bactrocera tryoni shi+, shits1, RpII215+, and RpII215ts cDNAs into P-element transformation vectors
The D. melanogaster shibire (shi) and RNA polymerase II 215 (RpII215) proteins were queried against the B. tryoni genome (GCA_000695345.1) using the tBLASTn algorithm in Geneious (v7.1.7) to identify their orthologs. The predicted coding sequence was obtained from a “gff3” genome annotation file (35). The NCBI Conserved Domain Search (CD-search) was used to identify signature motifs in B. tryoni orthologs. Codon optimization was performed on the coding sequence using the Integrated DNA Technologies (IDT, Coralville, IA, USA) online Codon Optimization Tool (http://sg.idtdna.com/CodonOpt) to account for D. melanogaster codon bias.
A Kozak sequence (CAAAATG) was placed in front of the start codon to facilitate translation initiation, and the stop codon “TAA” was added at the end of the coding sequence. gBlocks for B. tryoni shi, shits1, RpII215+, and RpII215ts were obtained from IDT as dried oligos (1,000 ng), which were then resuspended in 20 μL of TE (10 mM Tris, pH 7.5–8.0, 1 mM EDTA) to 50 ng/μL stock solutions and stored at −20°C. gBlock fragments were cloned into the EcoRI site of the pUAST-attB vector using Gibson Assembly Master Mix (NEB, Ipswich, MA, USA). Cloned products were analyzed using Sanger sequencing to ensure they did not contain non-synonymous mutations. Validated constructs were transformed into D. melanogaster by BestGene Inc. (Chino Hills, CA, USA).
2.3 Expression of Bactrocera tryoni orthologs under Drosophila melanogaster temperature-sensitive mutant backgrounds
The prefix Q distinguishes between B. tryoni (Queensland fruit fly) transgenes and D. melanogaster alleles or genotypes. The UAS-Qshi+ and UAS-Qshits1 transgenic lines and da-GAL4 and nSyb-GAL4 driver lines were crossed into the genetic background of the D. melanogaster shits2 strain (DGRC 106754). The D. melanogaster transgenic lines carrying UAS-QRpII215+ and UAS-QRpII215ts and the da-GAL4 driver line were crossed into the genetic background of the D. melanogaster RNA polymerase temperature-sensitive strain RpII215ts (BDSC 34755) (Supplementary Tables S2–S4). The presence of UAS transgenes and D. melanogaster mutant backgrounds was confirmed by PCR and Sanger sequencing using the primers listed in Supplementary Table S5. GAL4 driver expression was verified by crossing the respective line to UAS-mCD8-GFP, then confirming the green fluorescent protein (GFP) expression in the progeny (data not shown).
Crosses were performed using 3- to 6-day-old virgin flies with three males and five females per vial. Egg laying occurred for 24 h at 25°C and the flies were then removed. Vials containing eggs were maintained at either 18°C, 22°C, 25°C, or 29°C until pupal eclosion, and the number of adult flies, either the GAL4 driver or the balancer TM6B, was recorded. The percentage of progeny with the GAL4 driver (non-TM6B) was calculated for each treatment. Data were analyzed using RStudio (v1.2.1335).
3 Results
3.1 Generating Drosophila melanogaster transgenic lines carrying Bactrocera tryoni shi or RpII215 orthologs
We generated D. melanogaster transgenic lines carrying the B. tryoni wild-type (Qshi+ and QRpII215+) and putative temperature-sensitive (Qshits1 and QRpII215ts) transgenes. Expression was selectively controlled using the GAL4–UAS system (Supplementary Figure S1) (36). Complementation rescue experiments in the Drosophila temperature-sensitive lethal strains shits2 and RpII215ts were then attempted with the B. tryoni transgenes. Expressing wild-type allelic transgenes was expected to rescue Drosophila temperature-sensitive phenotypes, while putative temperature-sensitive alleles were not expected to rescue.
The B. tryoni shits1 (G268D) mutation was investigated as D. melanogaster shits1 has complete embryonic lethality. The B. tryoni ortholog of the D. melanogaster “short” isoform (Supplementary Figure S2), which has 49 amino acids absent at the carboxy terminal, was chosen for the transgenic complementation test. The D. melanogaster shibire “short” isoform previously appeared to have relatively consistent rescue phenotypes when expressed in the D. melanogaster shits2 temperature-sensitive background at high temperatures (31). Staples and Ramswami (1999) showed that a single isoform was unable to completely rescue the temperature-sensitive phenotypes across a broad range of restrictive temperatures, although rescue could occur at specific temperatures and a distinguishable level of rescue was still observable with each isoform (31). Insertion of the entire B. tryoni genomic region of shibire may have enabled alternate splicing of multiple isoforms; however, this was not feasible due to the size of the genomic region (30 kb). The coding sequence of B. tryoni shi “short” was obtained from a gff3 genome annotation file provided with the draft genome sequencing project (35). The shibire proteins from D. melanogaster and B. tryoni share 91% identity, including conserved glycine amino acids at positions 268 and 141, which are encoded by aspartate in shits1 (G268D) and serine in shits2 (G141S) mutants, respectively.
The D. melanogaster RpII215 has only one protein isoform (Supplementary Figure S3), which was used in a BLAST search against the B. tryoni genome (JHQJ00000000.1) to identify B. tryoni RpII215 on scaffold Btry263 (JHQJ01000312.1). The coding regions of the two alleles share 78% identity and their protein sequences share 93% identity. The amino acid that is mutated in D. melanogaster RpII215ts (R997C) is conserved in B. tryoni.
3.2 Assessing the temperature sensitivity of Bactrocera tryoni shi transgenes
The D. melanogaster shits2 strain was used as a temperature-sensitive lethal genetic background to test for complementation rescue with the B. tryoni shi+ wild-type construct. Complementation tests using the B. tryoni shits1 were also performed, but not expected to rescue the temperature-sensitive phenotype. According to modENCODE (implemented in FlyBase r2021_05) (37) and Chen et al. (38), the D. melanogaster shi is expressed in all life stages, and its major expression site is the nervous system. We obtained a stock of shi-GAL4 (BDSC stock ID 42738) with the intention to drive the expression of the UAS shibire constructs with the native promoter; however, we were unable to detect the expression of the UAS responder constructs with this specific driver. Consequently, the drivers da-GAL4 and nSyb-GAL4 were chosen due to their similar expression patterns in brain tissues according to single-cell transcriptomic analysis of D. melanogaster (39).
Interspecific functional complementation tests were carried out by crossing the UAS responder female lines with the male GAL4 driver lines to test the ability of B. tryoni transgenes to rescue the D. melanogaster shits2 temperature-sensitive effect at 29°C. The crossing strategy was designed with an internal control, meaning that ~50% of progeny would inherit the GAL4 driver and express the transgene and ~50% would inherit the visible phenotypic marker TM6B and not express the transgene.
Males with the genotype shits2/Y; +/+; nSyb-GAL4/TM6B were systematically crossed with six different homozygous female strains, and progeny were reared at four temperatures (18°C, 22°C, 25°C, or 29°C). Crossing males with w1118 control females confirmed that progeny survived under all experimental conditions and approximately 50% of progeny inherited the TM6B marker [Figure 1 (i)]. When males were crossed to shits2 female homozygotes, strong survivorship was recorded at all temperatures, except at 29°C where only 13 individuals developed to the pupal stage, which is consistent with a previous observation [Figure 1 (vi)] (20, 26). The UAS-Qshi+ and UAS-Qshits1 constructs were then expressed in D. melanogaster to assess any negative impacts of expressing the transgenes. Approximately 50% of individuals expressed the transgene at all temperatures, demonstrating that B. tryoni UAS-Qshi+ and UAS-Qshits1 do not negatively affect fly viability under the nSyb-GAL4 driver [Figure 1 (ii and iii)].
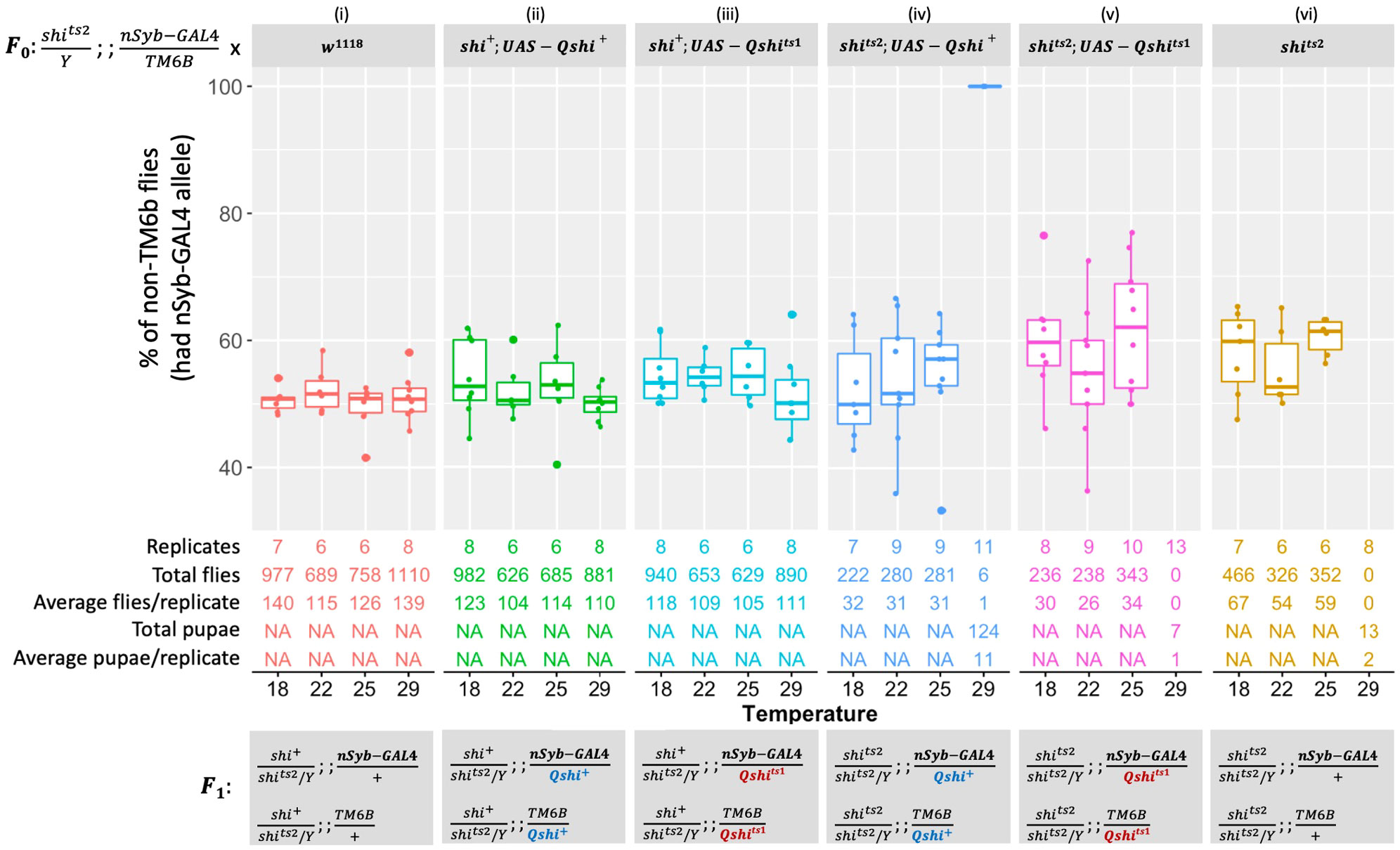
Figure 1 Expressing Bactrocera tryoni shi wild-type (UAS-Qshi+) and mutant (UAS-Qshits1) transgenes driven by the shits2; nSyb-GAL4/TM6B driver. In each standard food vial, F0 crosses were set up by placing three males that were hemizygous for the temperature-sensitive shits2 mutant allele on the X chromosome, heterozygous for nSyb-GAL4, and balancer on the third chromosome (shits2/Y; +/+; nSyb-GAL4/TM6B) and five females of either control wild type (w1118) (i), Qshi+ in the wild-type background (shi+; UAS-Qshi+) (ii), Qshits1 in the wild-type background (shi+; UAS-Qshits1) (iii), Qshi+ in the temperature-sensitive background (shits2; UAS-Qshi+) (iv), Qshits1 in the temperature-sensitive background (shits2; UAS-Qshits1) (v), or control temperature-sensitive (shits2) (vi). F0 parents were allowed to lay eggs for 24 h at 25°C, then eggs in vials were reared at either 18°C, 22°C, 25°C, or 29°C. In the F1, chromosomes are presented in order: sex determination chromosomes, chromosome 2, and chromosome 3. F1 flies with non-TM6B expressed UAS-Qshi+ or UAS-Qshits1, while TM6B flies did not express these transgenes. The number of replicates and total counted F1 flies for each cross at each temperature were indicated. Box plots represent the interquartile range, and the median value is indicated. Error bars represent 1.5 times the interquartile range.
A complementation cross was performed to determine whether the Drosophila shits2 temperature sensitivity could be rescued with the B. tryoni wild-type UAS-Qshi+ transgene. Flies expressing UAS-Qshi+ under the nSyb promoter or lacking expression (TM6B) were recovered in similar proportions at 18°C, 22°C, and 25°C. At 29°C, only six adults expressing UAS-Qshi+ emerged from 11 replicate vials, although there were 124 pupae that died in late-stage development (P12–P14, wings were varied from gray to completely black) (40). In comparison, only 13 pupae from 8 replicate vials were observed in the shits2 mutant background [Figure 1 (vi)]. Expressing the B. tryoni shibire “short” isoform, nSyb-GAL4:UAS-Qshi+, provided partial temperature-sensitive rescue at 29°C in a Drosophila shits2 background.
Expression of UAS-Qshits1 in the D. melanogaster temperature-sensitive shits2 background had no adult survival at 29°C. There were seven early-stage pupae that died prior to stage P8, as eyes were not visible [Figure 1 (vi)] (40). The B. tryoni shits1 allele differed in function from B. tryoni wild-type shi+ at a high temperature of 29°C [Figure 1 (v)]. These data indicate that amino acid G268 is required for function at elevated temperatures.
Expression of UAS-Qshits1 and UAS-Qshi+ was also assessed using the da-GAL4 driver. However, dominant lethal effects were observed when UAS-Qshi+ was expressed at 29°C, indicating that the driver was not suitable for the expression of this transgene (Supplementary Figure S4).
3.3 Assessing the temperature sensitivity of Bactrocera tryoni RpII215 transgenes
The D. melanogaster gene RpII215 is expressed in all life stages and tissues according to modENCODE (implemented in FlyBase r2021_05) (37). The R977C mutation (RpII215ts) is temperature-sensitive lethal at 29°C. We hypothesized that expressing B. tryoni UAS-QRpII215+ in temperature-sensitive Drosophila (RpII215ts) would rescue embryonic lethality at 29°C but that UAS-QRpII215ts would fail to rescue. The da-GAL4 driver was used as a ubiquitous driver to express UAS constructs, as RpII215-GAL4 drivers reproducing the complete endogenous expression pattern have not been described.
Interspecific functional complementation tests were performed by crossing experimental female lines with the same male driver line. Similar to the crosses described in Figure 1, 50% of progeny were expected to carry da-GAL4 and express the transgene, and 50% should carry TM6B and not express the transgene. Control crosses confirmed that da-GAL4 expression of the B. tryoni wild-type QRpII215+ protein did not have adverse effects [Figure 2 (i and ii)]. Homozygous and hemizygous RpII215ts Drosophila only survived at 29°C when the da-GAL4 allele drove the expression of UAS-QRpII215+, rescuing lethality and complementing loss of function [Figure 2 (iv)].
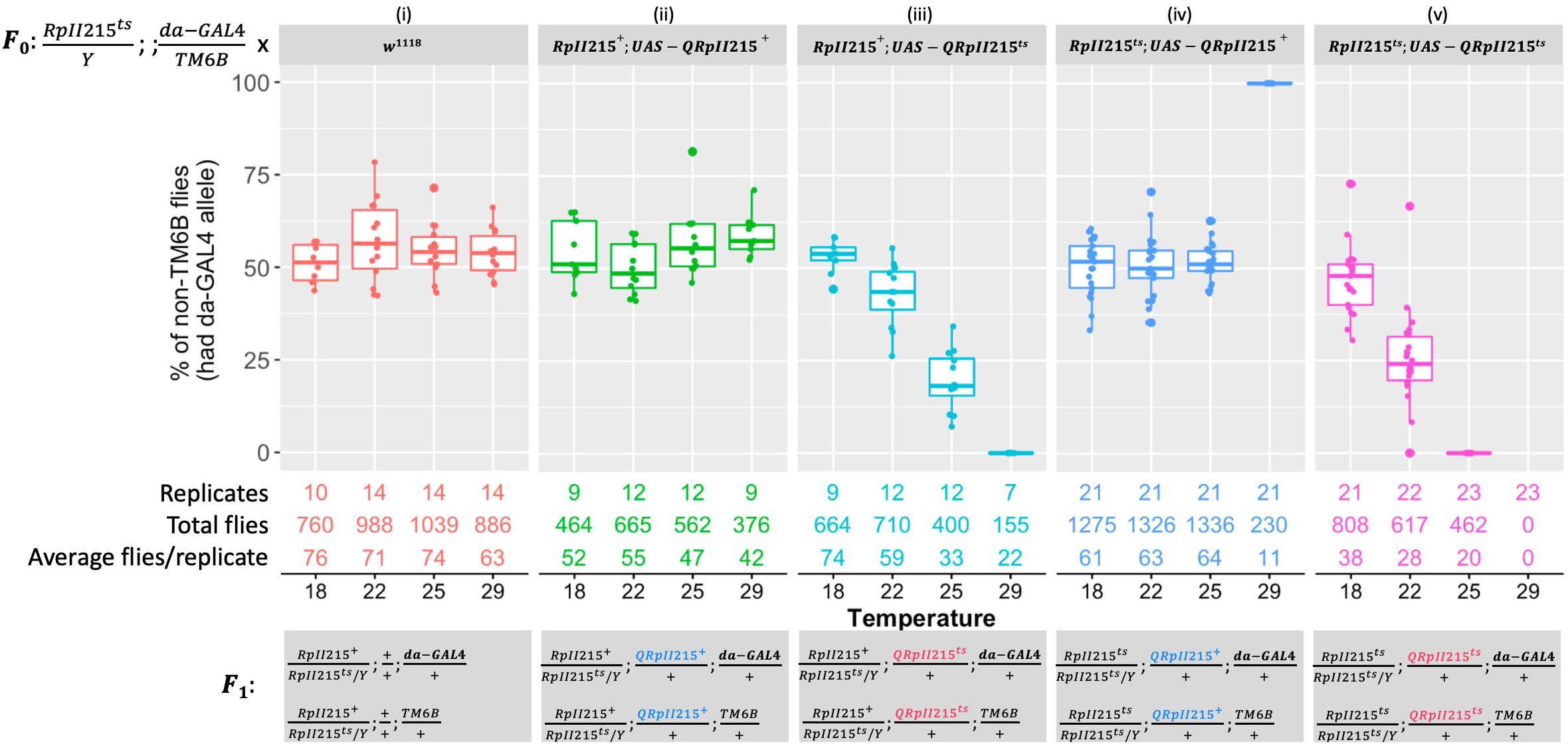
Figure 2 Expressing Bactrocera tryoni RpII215 wild-type (UAS-QRpII215+) and mutant (UAS-QRpII215ts) transgenes with the da-GAL4 driver. In each food vial, F0 crosses were set up by placing three males that were hemizygous for the temperature-sensitive RpII215ts mutant allele on the X chromosome, heterozygous for da-GAL4, and balancer on the third chromosome (RpII215ts/Y; +/+; da-GAL4/TM6B) and five females of either control wild type (w1118) (i), QRpII215+ in the wild-type background (RpII215+; UAS-QRpII215+) (ii), QRpII215ts in the wild-type background (RpII215+; UAS-QRpII215ts) (iii), QRpII215+ in the temperature-sensitive background (RpII215ts; UAS-QRpII215+) (iv), or QRpII215ts in the temperature-sensitive background (RpII215ts; UAS-QRpII215ts) (v). F0 parents were allowed to lay eggs for 24 h at 25°C, then eggs in vials were reared at either 18°C, 22°C, 25°C, or 29°C. In the F1, chromosomes are presented in order: sex determination chromosomes, chromosome 2, and chromosome 3. F1 flies with non-TM6B expressed UAS-QRpII215+ or UAS-QRpII215ts, while TM6B flies did not express these transgenes. The number of replicates and total counted F1 flies for each cross at each temperature were indicated. Box plots represent the interquartile range, and the median value is indicated. Error bars represent 1.5 times the interquartile range.
Surprisingly, the expression of the putative temperature-sensitive construct, UAS-QRpII215ts, showed a dominant lethal phenotype as temperature increased, regardless of genetic background. Drosophila carrying a wild-type RpII215+ allele were unable to survive at 29°C [Figure 2 (iii)]. In the Drosophila RpII215ts background, flies expressing the QRpII215ts transgene could not survive at the lower temperature of 25°C and none survived at 29°C [Figure 2 (v)].
In summary, the B. tryoni UAS-QRpII215+ was able to rescue the lethality associated with D. melanogaster RpII215ts at 29°C. A dominant negative effect occurred when expressing UAS-QRpII215ts in D. melanogaster with one wild-type allele, as expression of the RpII215ts itself resulted in reduced viability at 22°C and 25°C and complete lethality at 29°C. A more severe effect was observed with the expression of B. tryoni UAS-QRpII215ts in the D. melanogaster temperature-sensitive RpII215ts mutant background, which resulted in lethality at 25°C. Amino acid R977 in the B. tryoni RpII215 protein was required for viability at certain temperatures.
4 Discussion
Despite some success in genetic transformation and genome editing capabilities using molecular technologies, investigating temperature-sensitive lethal mutations in B. tryoni remain challenging (41, 42). Generating precise single-base substitutions in B. tryoni using CRISPR/Cas9 technology via the homology-directed repair (HDR) pathway is complex (19) and time-consuming, with the potential of off-target effects causing genetic variation. A minimum of three generations is required (which can take up to 3 months) to establish homozygous mutant strains. Therefore, using the D. melanogaster GAL4–UAS system can advance functional studies of B. tryoni orthologs with the advantage of performing experiments in the same genetic background across all lines in a relatively short time frame. Drosophila complementation tests were performed to determine whether B. tryoni wild-type alleles could rescue known temperature-sensitive lethal mutations for Drosophila shibire and RpII215. Substitution of specific amino acids, which are known to cause Drosophila temperature sensitivity, into B. tryoni alleles was not expected to rescue.
Shibire is an essential gene for D. melanogaster development as homozygous null mutants are non-viable (43). Multiple isoforms are produced and are likely to be expressed within different tissues at different development stages (31, 38). Staples and Ramaswami (31) reported that temperature-sensitive paralysis in shits2 flies occurred at 27°C, and partial phenotypic rescue was achieved through transgenic expression-specific shibire isoforms. A series of isoforms described as “short” lacked a 49-amino acid region at the carboxy terminus and prevented paralysis up to 30–32°C. B. tryoni is also known to express multiple shibire isoforms in RNA-seq transcriptome datasets (Dr. Stephen Pierce, CSIRO, personal communication). Expression of an equivalent “short” isoform of the B. tryoni wild-type shibire partially rescued the D. melanogaster shits2 temperature-sensitive phenotype when expressed using the nSyb-GAL4 driver. Insertion of the entire shibire genomic region would have been ideal to enable the transcription of all isoforms and may have then resulted in improved rescue efficiency; however, plasmid construction and genomic integration were not feasible due the insert size (approximately 30 kbp).
Expression of the shits1 mutant allele failed to show any level of rescue of the associated temperature-sensitive lethality, supporting the G268D amino acid substitution as an important site for viability at restrictive temperatures. Choo et al. (19) have successfully introduced this equivalent shits1 mutation into the B. tryoni genome using CRIPSR/Cas9-mediated homology directed repair. However, the mutation was found to be homozygous lethal at the low rearing temperature of 21°C (19). This shows reduced fitness compared to the equivalent mutation in Drosophila (27). The D. melanogaster shits2 had been shown to have a milder temperature-sensitive phenotype relative to shits1 (20, 27, 44). Although initial reports showed no embryonic lethality in Drosophila, Nguyen et al. (26) observed semi-embryonic lethality at 29°C (88% lethality), and no adult survival if continually treated at 29°C. This indicates that shits2 can also result in embryonic lethality, but appears less severe than shits1. The B. tryoni shits2 could be a potential target for future attempts at creating a GSS.
Dominant negative or semi-dominant effects have been reported for the Drosophila UAS-shits1 transgene when the construct was intentionally overexpressed at very high levels (45). We did not observe a poisoning effect when the B. tryoni UAS-Qshits1 was expressed via the da-GAL4 or nSyb-GAL4 drivers in Drosophila wild-type genetic backgrounds at any temperature. A dominant negative effect did occur when the ubiquitous da-GAL4 driver (but not nSyb-GAL4) expressed the B. tryoni shibire wild-type allele at 29°C (Supplementary Figure S4ii). The toxic effects appeared to be caused by the specific expression pattern of the da-GAL4 driver, which overexpressed the shibire “short” isoform in specific tissues. Alternatively, differences in the amino acid sequences between the D. melanogaster and B. tryoni shibire proteins may have functional consequences in specific tissues.
The D. melanogaster RpII215 gene encodes for a subunit of a multi-subunit enzyme, RNA polymerase II, involved in transcription catalysis (32). Expression of the B. tryoni wild-type RpII215 was sufficient to rescue the lethality of D. melanogaster RpII215ts at a high temperature of 29°C, indicating functional conservation across species. The B. tryoni RpII215ts expressed a dominant lethal effect in the D. melanogaster wild-type RpII215 background resulting in complete lethality at 29°C and had an even more severe effect in the temperature-sensitive RpII215ts background (when lethality was observed at 25°C). The reasons behind the B. tryoni RpII215ts temperature-sensitive dominant nature are unclear. Dominant negative effects have been observed expressing other temperature-sensitive alleles. Overexpression of D. melanogaster shits1 in different neuronal subsets using the GAL4–UAS system can result in dominant temperature-sensitive effects (41, 46). The D. melanogaster shits1 proteins block vesicle endocytosis at high restrictive temperatures (30, 44). In the case of overexpressing B. tryoni RpII215ts at 29°C, we propose two hypotheses. First, RpII215ts produces a thermolabile product that is inactivated or degraded at 29°C (23, 42). Alternatively, the assembly of the RpII215ts subunit into an active RNA polymerase II multi-subunit enzyme was temperature-sensitive (23). The B. tryoni RpII215ts may or may not have a dominant effect when introduced in the B. tryoni genome, which requires further experiments to confirm.
Expressing B. tryoni RpII215ts in a D. melanogaster RpII215ts temperature-sensitive lethal background caused complete lethality at 25°C, suggesting that wild-type amino acid R977 in the B. tryoni RpII215 protein has an important role in viability at certain temperatures. Determining whether the RpII215ts mutation has a dominant negative effect in B. tryoni will require transgenic modification and further experimentation.
5 Conclusions
We provide evidence for the partial rescue of D. melanogaster temperature-sensitive alleles of shibire, and strong rescue for RpII215, using interspecific complementation tests with B. tryoni wild-type orthologs. These results demonstrate a level of functional conservation across species. The B. tryoni equivalent of the temperature-sensitive alleles responded differently compared with the wild-type alleles at specific temperatures, supporting the replacement of amino acids (shibire G268D and RpII215 R977C) that failed to rescue temperature-sensitive phenotypes. Shibire and RpII215 hold some potential as candidate genes for studies aimed at developing B. tryoni temperature-sensitive GSS. Effective sex separation can be achieved when females homozygous for temperature-sensitive alleles are killed at elevated temperatures, while heterozygous males survive through a functional wild-type allele translocated or transgenically integrated onto the Y chromosome (16). While the shibire shits1 G268D allele has previously been shown to have a more severe effect in B. tryoni than observed in D. melanogaster, other temperature-sensitive shibire alleles can be explored.
Data availability statement
The original contributions presented in the study are included in the article/Supplementary Material. Further inquiries can be directed to the corresponding author.
Ethics statement
The manuscript presents research on animals that do not require ethical approval for their study.
Author contributions
SB and AC: Conceived the research; SB, AC, and TN: Designed experiments; AC and TN: Performed experiments. TN: Performed data analysis; TN, AC, and SB: Wrote the manuscript. All authors contributed to the article and approved the submitted version.
Funding
The author(s) declare financial support was received for the research, authorship, and/or publication of this article. This research was funded by the Hermon Slade Foundation grant number HSF 18-6. AC was funded by Horticulture Innovation Australia grant numbers MT13059 and FF18002, with re-search and development levy funds from the vegetable, apple and pear, citrus, strawberry, table grape, cherry and summerfruit industries, with co-investment from South Australian Research and Development Institute (SARDI) and Primary Industries and Regions South Australia (PIRSA).
Acknowledgments
We thank Prof. Robert Richards (University of Adelaide) for access to a Drosophila insectary, Stuart Gilchrist for providing a gff3 annotation file of the B. tryoni draft genome sequence and Trent Perry (University of Melbourne) for discussions regarding Drosophila crosses.
Conflict of interest
The authors declare that the research was conducted in the absence of any commercial or financial relationships that could be construed as a potential conflict of interest.
The authors AC declared that they were an editorial board member of Frontiers, at the time of submission. This had no impact on the peer review process and the final decision.
Publisher’s note
All claims expressed in this article are solely those of the authors and do not necessarily represent those of their affiliated organizations, or those of the publisher, the editors and the reviewers. Any product that may be evaluated in this article, or claim that may be made by its manufacturer, is not guaranteed or endorsed by the publisher.
Supplementary material
The Supplementary Material for this article can be found online at: https://www.frontiersin.org/articles/10.3389/finsc.2024.1249103/full#supplementary-material
References
1. Kogan M, Bajwa W. Integrated pest management: A global reality? Anais da Sociedade Entomológica do Brasil. (1999) 28(1):1–25. doi: 10.1590/S0301-80591999000100001
2. Mau RFL, Jang EB, Vargas RI. The Hawaii area-wide fruit fly pest management programme: influence of partnerships and a good education programme. In: Area-Wide Control of Insect Pests. Springer Netherlands, Dordrecht (2007).
3. Vargas RI, Pinero JC, Leblanc L. An overview of pest species of Bactrocera fruit flies (Diptera: tephritidae) and the integration of biopesticides with other biological approaches for their management with a focus on the Pacific region. Insects. (2015) 6:297–318. doi: 10.3390/insects6020297
4. Beketov MA, Kefford BJ, Schäfer RB, Liess M. Pesticides reduce regional biodiversity of stream invertebrates. Proc Natl Acad Sci. (2013) 110:11039. doi: 10.1073/pnas.1305618110
5. Nicolopoulou-Stamati P, Maipas S, Kotampasi C, Stamatis P, Hens L. Chemical pesticides and human health: the urgent need for a new concept in agriculture. Front Public Health. (2016) 4:148. doi: 10.3389/fpubh.2016.00148
6. Knipling E. Possibilities of insect control or eradication through use of sexually sterile males. J Economic Entomol. (1955) 48:459–62. doi: 10.1093/jee/48.4.459
7. Wyss JH. Screwworm eradication in the Americas. Ann New York Acad Sci. (2000) 916:186–93. doi: 10.1111/j.1749-6632.2000.tb05289.x
8. Enkerlin W, Gutiérrez-Ruelas JM, Cortes AV, Roldan EC, Midgarden D, Lira E, et al. Area freedom in Mexico from Mediterranean fruit fly (Diptera: tephritidae): A review of over 30 years of a successful containment program using an integrated area-wide sit approach. Florida Entomologist. (2015) 98:665–81. doi: 10.1653/024.098.0242
9. Marec F, Vreysen MJB. Advances and challenges of using the sterile insect technique for the management of pest lepidoptera. Insects. (2019) 10:371. doi: 10.3390/insects10110371
10. Bourtzis K, Lees RS, Hendrichs J, Vreysen MJB. More than one rabbit out of the hat: radiation, transgenic and symbiont-based approaches for sustainable management of mosquito and tsetse fly populations. Acta Tropica. (2016) 157:115–30. doi: 10.1016/j.actatropica.2016.01.009
11. Lees RS, Gilles JRL, Hendrichs J, Vreysen MJB, Bourtzis K. Back to the future: the sterile insect technique against mosquito disease vectors. Curr Opin Insect Sci. (2015) 10:156–62. doi: 10.1016/j.cois.2015.05.011
12. Plant Health Australia. The Australian Handbook for the Identification of Fruit Flies. Canberra, ACT: Plant Health Australia (2018).
13. Mangan RL. Priorities in formulation and activity of adulticidal insecticide bait sprays for fruit flies. In: Shelly T, Epsky N, Jang EB, Reyes-Flores J, Vargas R, editors. Trapping and the Detection, Control, and Regulation of Tephritid Fruit Flies: Lures, Area-Wide Programs, and Trade Implications. Springer Netherlands, Dordrecht (2014). p. 423–56.
14. Suckling DM, Kean JM, Stringer LD, Cáceres-Barrios C, Hendrichs J, Reyes-Flores J, et al. Eradication of tephritid fruit fly pest populations: outcomes and prospects. Pest. Manag. Sci. (2016) 72:456–65. doi: 10.1002/ps.3905
15. Rendón P, McInnis D, Lance D, Stewart J. Medfly (Diptera : tephritidae) genetic sexing: large-scale field comparison of males-only and bisexual sterile fly releases in Guatemala. J Economic Entomol. (2004) 97:1547–53. doi: 10.1603/0022-0493-97.5.1547
16. Franz G. Development and application of genetic sexing systems for the Mediterranean fruit fly based on a temperature sensitive lethal. In: McPheron BA, Steck GJ, editors. Fruit Fly Pest, A World Assessment of Their Biology and Management. Delray Beach, FL, USA: Saint Lucie Press (1996). pp. 185–91.
17. Franz G, Gencheva E, Kerremans P. Improved stability of genetic sex-separation strains for the Mediterranean fruit fly, Ceratitis capitata. Genome. (1994) 37:72–82. doi: 10.1139/g94-009
18. Sim SB, Ruiz-Arce R, Barr NB, Geib SM. A new diagnostic resource for Ceratitis capitata strain identification based on qtl mapping. G3 (Bethesda). (2017) 7:3637–47. doi: 10.1534/g3.117.300169
19. Choo A, Fung E, Chen IY, Saint R, Crisp P, Baxter SW. Precise single base substitution in the shibire gene by crispr/cas9-mediated homology directed repair in Bactrocera tryoni. BMC Genet. (2020) 21:127. doi: 10.1186/s12863-020-00934-3
20. Grigliatti TA, Hall L, Rosenbluth R, Suzuki DT. Temperature-sensitive mutations in Drosophila melanogaster. Mol Gen Genet. (1973) 120:107–14. doi: 10.1007/BF00267238
21. Suzuki DT. Temperature-sensitive mutations in Drosophila melanogaster. Science. (1970) 170:695–706. doi: 10.1126/science.170.3959.695
22. Suzuki DT, Grigliatti T, Williamson R. Temperature-sensitive mutations in Drosophila melanogaster, vii. A mutation (Parats) causing reversible adult paralysis. Proc Natl Acad Sci. (1971) 68:890. doi: 10.1073/pnas.68.5.890
23. Mortin MA, Kaufman TC. Development effects of a temperature-sensitive rna polymerase ii mutation in Drosophila melanogaster. Dev Biol. (1984) 103:343–54. doi: 10.1016/0012-1606(84)90323-3
24. Pendleton RG, Rasheed A, Sardina T, Tully T, Hillman R. Effects of tyrosine hydroxylase mutants on locomotor activity in Drosophila: A study in functional genomics. Behav Genet. (2002) 32:89–94. doi: 10.1023/A:1015279221600
25. Shellenbarger DL, Mohler JD. Temperature-sensitive periods and autonomy of pleiotropic effects of L(1)Nts1, a conditional notch lethal in Drosophila. Dev Biol. (1978) 62:432–46. doi: 10.1016/0012-1606(78)90226-9
26. Nguyen TNM, Choo A, Baxter SW. Lessons from Drosophila: engineering genetic sexing strains with temperature-sensitive lethality for sterile insect technique applications. Insects. (2021) 12:243. doi: 10.3390/insects12030243
27. Poodry CA, Hall L, Suzuki DT. Developmental properties of shibirets1: A pleiotropic mutation affecting larval and adult locomotion and development. Dev Biol. (1973) 32:373–86. doi: 10.1016/0012-1606(73)90248-0
28. Mortin MA, Kaufman TC. Developmental genetics of a temperature sensitive rna polymerase ii mutation in Drosophila melanogaster. Mol Gen Genet. (1982) 187:120–5. doi: 10.1007/BF00384394
29. Chen MS, Obar RA, Schroeder CC, Austin TW, Poodry CA, Wadsworth SC, et al. Multiple forms of dynamin are encoded by shibire, a Drosophila gene involved in endocytosis. Nature. (1991) 351:583–6. doi: 10.1038/351583a0
30. van der Bliek AM, Meyerowitz EM. Dynamin-like protein encoded by the Drosophila shibire gene associated with vesicular traffic. Nature. (1991) 351:411–4. doi: 10.1038/351411a0
31. Staples RR, Ramaswami M. Functional analysis of dynamin isoforms in Drosophila melanogaster. J Neurogenet. (1999) 13:119–43. doi: 10.3109/01677069909083470
32. Ingles CJ, Biggs J, Wong JK, Weeks JR, Greenleaf AL. Identification of a structural gene for a rna polymerase ii polypeptide in Drosophila melanogaster and mammalian species. Proc Natl Acad Sci USA. (1983) 80:3396–400. doi: 10.1073/pnas.80.11.3396
33. Cramer P, Bushnell DA, Kornberg RD. Structural basis of transcription: rna polymerase ii at 2.8 ångstrom resolution. Science. (2001) 292:1863. doi: 10.1126/science.1059493
34. Severinov K, Mustaev A, Kukarin A, Muzzin O, Bass I, Darst SA, et al. Structural modules of the large subunits of rna polymerase. Introducing archaebacterial and chloroplast split sites in the beta and beta’ Subunits of Escherichia coli rna polymerase. J Biol Chem. (1996) 271:27969–74. doi: 10.1074/jbc.271.44.27969
35. Gilchrist AS, Shearman DCA, Frommer M, Raphael KA, Deshpande NP, Wilkins MR, et al. The draft genome of the pest tephritid fruit fly Bactrocera tryoni: resources for the genomic analysis of hybridising species. BMC Genomics. (2014) 15:1153. doi: 10.1186/1471-2164-15-1153
36. Brand AH, Perrimon N. Targeted gene expression as a means of altering cell fates and generating dominant phenotypes. Development. (1993) 118:401–15. doi: 10.1242/dev.118.2.401
37. Roy S, Ernst J, Kharchenko PV, Kheradpour P, Negre N, Eaton ML, et al. Identification of functional elements and regulatory circuits by Drosophila modencode. Science. (2010) 330:1787–97. doi: 10.1126/science.1198374
38. Chen MS, Burgess CC, Vallee RB, Wadsworth SC. Developmental stage- and tissue-specific expression of shibire, a Drosophila gene involved in endocytosis. J Cell Sci. (1992) 103:619–28. doi: 10.1242/jcs.103.3.619
39. Davie K, Janssens J, Koldere D, De Waegeneer M, Pech U, Kreft L, et al. Single-cell transcriptome atlas of the aging Drosophila brain. Cell. (2018) 174:982–+. doi: 10.1016/j.cell.2018.05.057
40. Bainbridge SP, Bownes M. Staging the metamorphosis of Drosophila melanogaster. Development. (1981) 66:57–80. doi: 10.1242/dev.66.1.57
41. Kitamoto T. Conditional modification of behavior in Drosophila by targeted expression of a temperature-sensitive shibire allele in defined neurons. J Neurobiol. (2001) 47:81–92. doi: 10.1002/neu.1018
42. Coulter DE, Greenleaf AL. Properties of mutationally altered rna polymerases ii of Drosophila. J Biol Chem. (1982) 257:1945–52. doi: 10.1016/S0021-9258(19)68130-5
43. Poodry CA. Shibire, a neurogenic mutant of Drosophila. Dev Biol. (1990) 138:464–72. doi: 10.1016/0012-1606(90)90212-2
44. Kim Y-T, Wu C-F. Allelic interactions at the shibire locus of Drosophila: effects on behavior. J Neurogenetics. (1990) 7:1–14. doi: 10.3109/01677069009084149
45. Pfeiffer BD, Truman JW, Rubin GM. Using translational enhancers to increase transgene expression in Drosophila. Proc Natl Acad Sci. (2012) 109:6626–31. doi: 10.1073/pnas.1204520109
Keywords: temperature sensitivity, embryo lethality, transgenic complementation test, shibire, RNA polymerase II 215
Citation: Nguyen TNM, Choo A and Baxter SW (2024) Conservation of shibire and RpII215 temperature-sensitive lethal mutations between Drosophila and Bactrocera tryoni. Front. Insect Sci. 4:1249103. doi: 10.3389/finsc.2024.1249103
Received: 28 June 2023; Accepted: 31 January 2024;
Published: 04 March 2024.
Edited by:
Ping Wang, Cornell University, United StatesReviewed by:
Jayendra Nath Shukla, Central University of Rajasthan, IndiaZach N. Adelman, Texas A and M University, United States
Copyright © 2024 Nguyen, Choo and Baxter. This is an open-access article distributed under the terms of the Creative Commons Attribution License (CC BY). The use, distribution or reproduction in other forums is permitted, provided the original author(s) and the copyright owner(s) are credited and that the original publication in this journal is cited, in accordance with accepted academic practice. No use, distribution or reproduction is permitted which does not comply with these terms.
*Correspondence: Simon W. Baxter, simon.baxter@unimelb.edu.au