Turmeric shortens lifespan in houseflies
- 1School of Biological Sciences, University of Aberdeen, Aberdeen, United Kingdom
- 2Institute of Mathematics, University of Aberdeen, King’s College, Aberdeen, United Kingdom
- 3Programa de Pós-graduação em Ecologia e Conservação, Universidade Federal do Paraná, Curitiba, Brazil
Climate change poses a significant threat to food security and global public health with the increasing likelihood of insect pest outbreaks. Alternative ways to control insect populations, preferably using environmental-friendly compounds, are needed. Turmeric has been suggested as a natural insecticide with toxicity properties in some insect groups. However, empirical evidence of the effects of turmeric – and their interaction with other ecological factors such as diet – on insect survival has been limited. Here, we tested the effects of turmeric and its interactions with diets differing in protein source in the common housefly, Musca domestica. We found that turmeric shortened lifespan independent of diet and sex. Females in turmeric diets were heavier at death, which was likely driven by a combination of relatively lower rates of body mass loss during their lifetime and a higher percentage of water content at death. Each sex responded differently to the protein source in the diet, and the magnitude of the difference in lifespan between sexes were greatest in diets in which protein source was hydrolysed yeast; individuals from both sexes lived longest in sucrose-milk diets and shortest in diets with hydrolysed yeast. There was no evidence of an interaction between turmeric and diet, suggesting that the toxicity effects are independent of protein source in the diet. Given the seemingly opposing effects of turmeric in insects and mammals being uncovered in the literature, our findings provide further evidence in support of turmeric as a potential natural insecticide.
Introduction
Insect pests inflict damage to agricultural crops resulting in yield loss which causes major economic losses worldwide. This damage is predicted to increase with climate change (1, 2), as insects are sensitive to the rise in global temperatures and will pose further threats to food security (3, 4). Pesticides are chemicals used to control insect outbreaks and have been extensively used in agriculture. Approximately two million tonnes of pesticides are used worldwide per year, which pollute ecosystems and pose serious health hazards for living organisms (5, 6). For instance, dichlorodiphenyltrichloroethane (DDT) can remain in soils and water for years after exposure posing health risks for humans and other living organisms (7, 8). Alternative ways to control insect pest outbreaks are needed, which are affordable and more environmental-friendly.
Natural compounds can have pesticide activity, making them attractive for further studies as environmental-friendly alternatives to chemical pesticides (9–11). Turmeric (Curcuma longa) is a spice that is native to southeast Asia and is used worldwide as a medicinal plant, food colouring agent, and an ingredient in supplements (12, 13). Studies have shown that turmeric is toxic to insects, likely in part due to its main compound, curcumin, making this spice a candidate as natural pest control agent (14–18). For example, a study by Muhammad et al. (19) found turmeric extracts to be effective against insect pests that were feeding on okra (Abelmoschus esculentus). The results showed turmeric significantly protected the okra plant from aphid (Aphis gossypii) attacks and was more effective than synthetic pesticides that had been previously used. Turmeric extracts have also shown to reduce the development of the red flour beetle Tribollium castaneum, which helps to stop the invasion of the beetle against wheat grains (20). Similarly, a study by De Souza Tavares et al. (18) extracted ar-turmerone from C. longa rhizomes to investigate its insecticidal effects against the cabbage looper. This study found ar-turmerone reduced larval weight and increased mortality of the cabbage looper which helped to stop the insect from feeding on agricultural crops. Other studies have used turmeric oils as a natural and effective insecticide to repel the grain borer pest Rhyzopertha dominica from feeding on agricultural crops (21, 22). These oils have been described as having insect repellent properties and have been used as an insecticide against other indoor pests including the acid flies Paederus fuscipes and the darkling beetles Luprops tristis (23). These essential oils from turmeric also show promising repellent activity against mosquito species (15). Turmeric crude oil extract can also help to control cucumber powdery mildew which is a toxic foliar disease caused by the plant pathogen Podosphaera xanthii. This disease damages the yield and reduces the quality of crops, but turmeric oils show successful fungicidal effects against P. xanthii (24). Turmeric is relatively cheap and more environmentally friendly alternative to chemical pesticides. Thus, turmeric use as pesticide can contribute towards more sustainable crop production that minimizes environmental contamination and human health risks (25).
Nutrition is essential for insect growth and development but can also act as – or modulate the effects of – toxic compounds (26, 27). For example, recent studies have shown that excess protein can be toxic and shorten adult lifespan in flies and crickets (28–33). Other studies have also shown that protein shortens lifespan in different insect species including ants (34–36) and honeybees (37). Diet also modulates insect survival and behaviour by playing an interactive role with toxic bait compounds. For example, in the German cockroach Blattella germanica, both protein-to-carbohydrate ratios (PC ratio) and sugar type (glucose versus fructose) interacted with the formulation of the bait containing insecticide hydramethylnon to reduce survival of adult males and first-instar nymphs (38). In fact, exposure to suboptimal, high-protein diets improved bait effectiveness, suggesting that a high-protein diet could potentialize the toxicity of the bait (38). Notably though, this study investigates the interaction of diet and a noxious compound in the context of baits. Recent studies have broadened this approach and investigated the interactive effects of diet and toxic natural compounds. For example, Morimoto (39) showed that urea was toxic for the development of Drosophila melanogaster larvae but had no effect on oviposition choices by egg-laying females. Moreover, it showed that high-sugar diets combined with low and intermediate concentrations of urea were potentially more detrimental to the development of the larvae than high-protein diets with similar urea concentrations (39). Collectively, these results suggest that (1) diet is a major ecological factor determining development and health of insects, (2) diet modulates the toxicity effects of chemical baits as well as (3) natural toxic compounds. To date, however, we still need a better understanding of how diet and natural toxic compounds interact in models other than D. melanogaster (see e.g. past work by 14–18). Simpson and Raubenheimer (40) showed that mortality was independent of the allelochemical compounds tannic acid when locusts Locusta migratoria were fed in near-optimum protein-to-carbohydrate (PC) ratios, but the toxicity effect increased as the PC ratio became more unbalanced. Insights into these sorts of interactions can be invaluable when applying allelochemicals to modulate survival and physiology of species with economic significance and to better understand how herbivores interact with and adapt to plant compounds (41).
To address this, we manipulated protein source in the diet and turmeric levels to investigate how the toxicity of turmeric interacts with diet to modulate housefly Musca domestica lifespan and body composition. The common housefly, Musca domestica, is a cosmopolitan species closely associated with humans, and is perhaps one of the most common insect disease vectors of our societies (42–46). With our changing climate, houseflies could become an increasing threat to public health through the increase risks of disease transmission (47, 48). Here, we tested whether turmeric interacted with diet to shorten male and female lifespan, and then investigated individuals’ body mass at death, loss of body mass over lifespan and body water content at death. We predicted that turmeric would shorten individual lifespan due to its known toxic effects. We also predicted that flies feeding on sucrose alone would have a longer lifespan, based on the findings in the literature for other insect species (28, 31). There was not enough a priori information to conceptualise predictions about the interaction between turmeric and diet. We predicted that body mass at death, weight loss, and water content would be lower in flies in turmeric diets, likely because of feeding avoidance due to the presence of turmeric. Our results reveal how a noxious compound and diet can be used to modulate lifespan and body traits in a cosmopolitan insect species of public health interest.
Materials and methods
Fly stock and experimental flies
Houseflies were maintained in a large stock population (>700 individuals) at 25°C and 35% humidity, with a 12:12 hour light:dark cycle. Flies were obtained as pupae from a commercial supplier (Blades Biologics Ltd) and were maintained in the laboratory for 5 generations prior to the experiments. Stock colonies were maintained inside mesh cages (32cmx32cmx32cm) (BugDorm-4E4545; MegaView science Co. Limited). Adults were given ad libitum access to Hydrolysed Yeast (MP Biomedicals, Cat no: 103304) and commercial sucrose (Tate and Lyle White Granulated Sugar). Water was provided using a 500ml lidded Plastic Container with a slit cut into the centre of the lid which gave access to water through a moist surface and minimised drowning. We collected eggs to generate experimental flies by introducing a plastic container (14cm x 9cm x 5cm) into the stock cage containing the larval diet used to maintain our stocks. Larval diet recipe included 70g Organic Wheat Bran, 6g Nestle Instant Full Cream Milk Powder, 15g Alfalfa meal from Supreme Science Selective Guinea Pig Pellets (blended) and 300ml water. Females were allowed to lay eggs freely for 12 hours, after which containers were placed onto 1-2 cm of ground wheat bran within a larger 3 litre container (23cm x 16cm x 8cm) and reserved at controlled temperature (see above) to complete development. Larval diet was monitored daily, and water was added to the container to avoid desiccation. After 10 days, the larval diet was flooded with approximately 200ml of water to encourage pupation. Pupae for the experiments were retrieved from bran using a commercial sieve, reserved in Petri dishes at controlled temperatures for 7 days, and assigned to one of the experimental diets.
Experimental diets and turmeric
We randomly sampled 280 pupae collected and placed each pupa in one of the experimental treatments. From those, 213 pupae were weighted and randomly allocated to plastic vials (SARSTEDT, Ref: 58.631, 75 x 23.5mm) containing 5mL of each of the four experimental diets (henceforth ‘diets’) which varied in the protein source that was available to the flies: Sucrose, Brewer’s Yeast (BY) (MP Biomedicals, Cat no: 903312), hydrolysed yeast (HY) (MP Biomedicals, Cat no: 103304) and whole milk (Nestle Instant Full Cream Milk Powder, Nestlé) (M) diets. From manufacturer specifications, HY was the diet with the highest protein content (ca. 60%), followed by BY (ca 40%) and M (ca. 25.7%). Table 1 summarises the diet recipes. Each diet had two turmeric levels: control (0%) and treatment (5%) turmeric (w/v) (Turmeric from Sevenhills Wholefoods®). The product has a reported average curcumin content of 2.5%.We selected 5% turmeric concentration for this study to test whether similar toxicity levels were observed in houseflies as we found in D. melanogaster (14). Our final sample sizes per diet treatment with and without turmeric, respectively, were for Sucrose (n = 30, 26), M (n = 34, 33), HY (n = 28, 24) and BY (n = 19, 15), for a total of 213 data points. The sample sizes were uneven due to the availability of consumables.
Survival and body water content
We investigated how adult diet composition and the presence of turmeric affected survival time and body composition. Pupae were weighted and allocated to treatments as mentioned above. Adults in the experimental treatments were maintained in isolation for the entire experiment and were monitored daily which allowed us to measure lifespan. Once the individuals died, they were frozen at -20°C within 24 h. We then processed the individuals by measuring their weight at death and water content at death. Water content at death was obtained by subtracting the weight at death from the weight after individuals were maintained at 65°C for 2 days; water content at death was the difference between wet body weight at death minus dry body weight at death, divided by wet body weight at death x 100 (for percentage). All individuals were weighed in a SECURA124-1S, Secura® Laboratory Balances, Sartorius (precision: 0.00001g).
Statistical analysis
All statistical analyses were conducted in RStudio version 4.2.2. We used the ‘ggplot2’ package version 3.4.3 (49) for data visualisation (50). We wrangled the data using ‘stringr’ version 1.5.0 (51); ‘car’ version 4.0.0 (52); ‘dplyr’ version 1.1.3 (53); ‘survival’ version 3.5-7 (54); ‘ggfortify’ version 0.4.16 (55) and ‘tidyr’ packages version 2.0.0 (56). We fitted a survival model with Weibull distribution to assess the effects of diet, turmeric, and sex on fly survival. This model was selected for having lower AIC values compared with a model with exponential (Gompertz) distribution (Weibul: 1471.2 vs Exponential: 1804.36). We fitted linear models with either adult weight at death, weight loss from pupae to adult at death, and water content at death as response variables, and the main, two-way and three-way interactions of turmeric, diet and sex as explanatory variables. We also included individual fly lifespan as a covariate to control for differential survival effects on body weight and composition estimates. To improve model fit, we transformed adult weight at death (weight at death^2) and water content (square-root). P-values were obtained from F-statistics using the inbuilt anova function in R for all models except survival, where p-values were obtained from a log-rank test using the ‘Anova’ function of the ‘car’ package.
Results
Turmeric shortens lifespan in a diet- and sex-independent fashion
We first tested whether turmeric addition to diets with varying protein sources shortened individual lifespan. There was a statistically significant two-way interaction between diet and sex (χ2 = 62.849, p < 0.001). This was driven by the fact that males had substantially shorter lifespan than females when the protein source was hydrolysed yeast (HY), but this effect disappeared in sucrose only diets and diets with brewers’ yeast (BY) or whole milk (M) as protein sources (Figure 1A). There were also statistically significant effects of diet (χ2 = 105.318, p < 0.001), turmeric (χ2 = 15.884, p < 0.001) and sex (χ2 = 16.949, p < 0.001). These effects were driven by the fact that, on average, turmeric addition to the diet or HY as protein source shortened lifespan of both males and females, whereas males were on average shorter lived than females (Figure 1A; Supplementary Table S1). The three-way interaction between diet, turmeric, and sex was not statistically significant (χ2 = 6.347, p = 0.096), neither were the two-way interactions between turmeric and sex (χ2 = 0.026, p = 0.872) and diet and turmeric (χ2 = 6.412, p = 0.093). These results showed that turmeric addition shortened lifespan in a diet- and sex-independent fashion, corroborating the potential role of turmeric as noxious compound to shorten insect lifespan. Moreover, the results also showed that protein source in the diet can have sex-specific effects on lifespan despite the presence of turmeric.
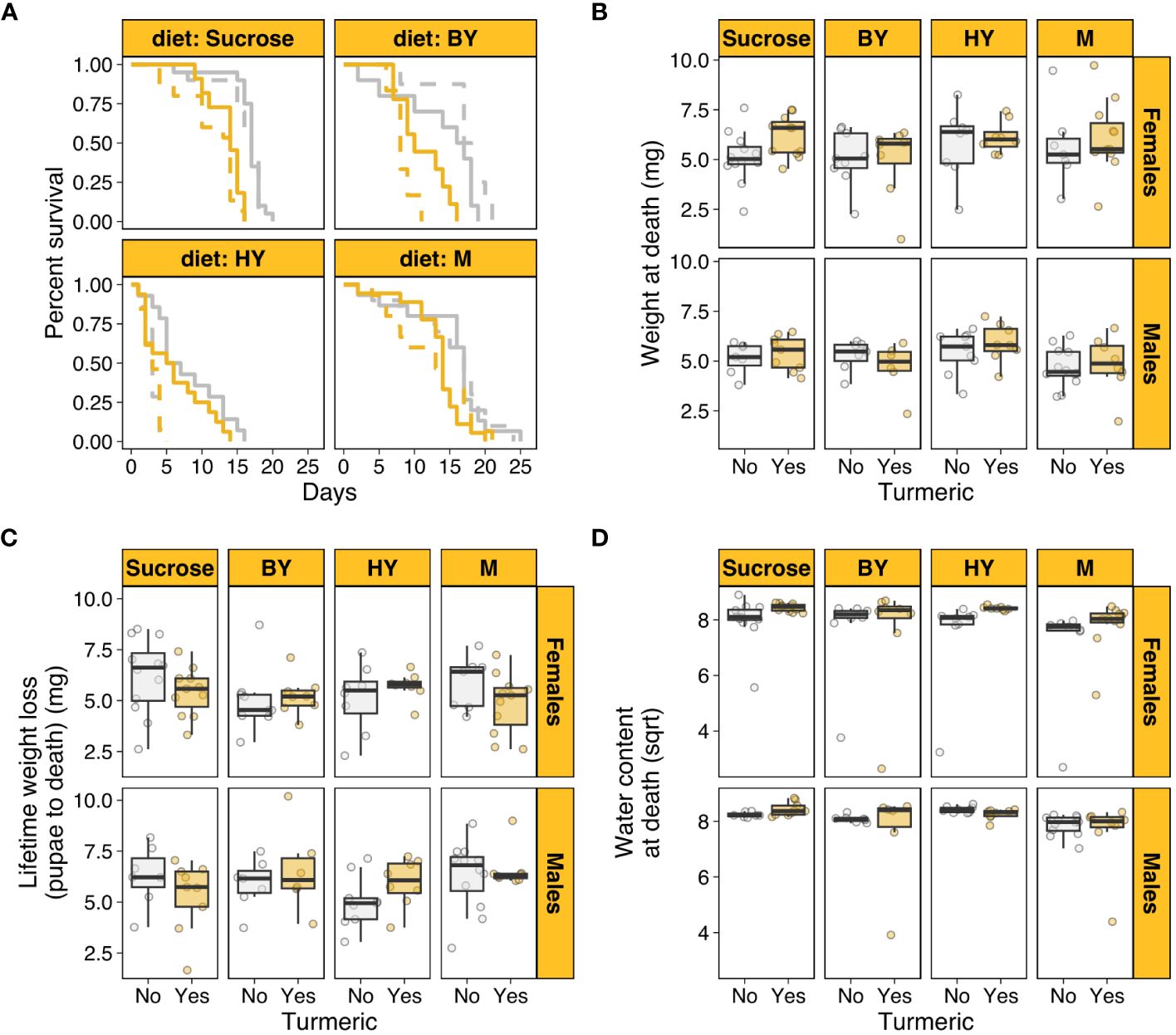
Figure 1 Turmeric shortens lifespan and affect body traits in houseflies. (A) Female (solid) and male (dashed) survival across diets varying in protein source with (yellow) and without turmeric (grey). (B) Body weight at death across diets with and without turmeric. (C) Lifetime weight loss (from pupae to adult death) across diets with and without turmeric. (D) Water content at death (square root-transformed) across diets with and without turmeric. M, Whole Milk as protein source; BY, Brewers’ yeast as protein source; HY, Hydrolysed yeast as protein source.
Turmeric and diet modulate body traits, although not necessarily interactively
Next, we investigated the effects of turmeric and protein source in the diets on organismal traits such as body weight at death and water content at death, which were used here as proxies for individual condition. After controlling for differential survival across treatments, there was a statistically significant effect of sex (F1,123 = 7.159, p = 0.008) and turmeric (F1,123 = 6.536, p = 0.011), whereby female flies on turmeric diets were on average heavier at death (Figure 1B). This effect could have emerged from two factors. First, female flies on turmeric diet could be heavier because they lost relatively less weight over their lifespan. Indeed, after controlling for differential survival, males lost more weight from pupae to adult death (F1,123 = 5.163, p = 0.024), but turmeric had no effect on weight loss (F1,123 = 0.035, p = 0.851; Supplementary Table S1; Figure 1C). As a result, weight loss alone could not fully explain the results the effects of turmeric and sex on adult weight at death.
A second explanation for the effects observed for weight at death could be due to differential water loss. Insect adult body composition is primarily related to water content (57, 58). Thus, turmeric could have affected individual hydration, which in turn influenced their weight at death. After controlling for differential survival, there was a statistically significant interaction between turmeric and sex (F1,123 = 3.983, p = 0.048; Supplementary Table S1), which was driven primarily by the fact that females on turmeric diets had on average higher water content at death (Figure 1D). There was also a small but statistically significant effect of diet (F1,123 = 3.055, p = 0.0310), driven by lower water content at death in M diets for both sexes and irrespective of turmeric (Figure 1D). These results showed that body weight at death was higher for females in turmeric diets likely due to a combination of lower body weight loss over lifespan and a relatively higher water content at death for females in turmeric diets. There were no other statistically significant three-way, two-way or main effects in the models of weight loss and water content (Supplementary Table S1).
Discussion
We set out to test the additive and interactive effects of turmeric in diets with varying protein sources in houseflies. Our data showed that turmeric shortened lifespan in a diet- and sex-independent fashion. This confirms our prediction that turmeric is a toxic compound which could potentially be used to control the development of houseflies. We also found that turmeric and diet independently modulated organism traits such as body mass loss during their lifetime and a higher percentage of water content at death. There was no evidence that turmeric interacted with diet. We also found that females had longer lifespan than males across all diets with and without turmeric, but the magnitude of the difference was diet-dependent but turmeric-independent. Together, our findings show that a natural compound such as turmeric can be used as an insecticide in a cosmopolitan fly species.
Our findings corroborate the role of turmeric in shortening lifespan from previous studies (Figure 1A). For instance, Uysal et al. (17) found that turmeric shortened lifespan in D. melanogaster adults. Similarly, von Hellfeld et al. (14) found that increasing turmeric concentration in the diet decreased oviposition in adult females and subsequently reduced egg-to-adult viability in the developing larvae in D. malanogaster. In fact, the study showed that high turmeric levels (>5%) could lead to complete developmental arrest (14). The molecular mechanisms through which turmeric – or compounds therein – leads to toxicity in insects is uncertain but Rahman et al. (59) found that higher concentrations of turmeric (>1%) in the diet decreased β-tubulin levels in the brain and affected a range of physiological traits of D. melanogaster. The study also found that an optimal dosage of 0.5% of turmeric could maintain healthy aging by increasing β-tubulin expression. Moreover, 0.5% turmeric also improved survivability, locomotor activity, fertility, tolerance to oxidative stress, and eye health (59). Taken together, these results suggest that turmeric has a non-linear effect on insect health: at lower concentrations, turmeric might be beneficial by increasing β-tubulin and protecting the physiology of the individual up to a point, after which turmeric is toxic and leads to negative effects that decrease survival. This could occur if turmeric has a hormesis-like effect in the individuals (i.e. beneficial effect of low exposure to a toxic compound). Hormesis-like benefits upon exposure to stressors have been described in Drosophila species in terms of temperature (60–62), radiation (63) and larval crowding (64, 65), showing that hormesis-like responses can be ubiquitous. As turmeric can be used as an insecticide, and hormesis is known to occur in response to insecticides in other species (66), future studies should investigate the molecular underpinnings of the responses described here to ascertain hormesis-like molecular profiles. This leads us to hypothesise that the relationship between turmeric concertation in the diet and β-tubulin expression in the brain is a concave parabola. Having said that, von Hellfeld et al. (14) conducted a discrete dose-response study and found that turmeric was harmful for the developing larvae at concentrations above 1% but found no evidence of beneficial effects. Dose-response studies for turmeric in other species are needed to uncover the nuances of the toxic vs beneficial effects of turmeric in insects.
Curcumin, one of the main components of turmeric, was found to extend the lifespan in D. melanogaster (67–69). Curcumin prolongs lifespan in D. melanogaster by enhancing superoxide dismutase (SOD) activity. Similarly, Chen et al. (68) also found that under heat stress conditions, adding curcumin to diet eased the effects of increased oxidative stress by increasing SOD expression and resulting in longer lifespan. Shen et al. (69) found that D. melanogaster fed diets with either 0.5 or 1.0mg/g of curcumin in the diet also increased the enzyme activity of SOD in both adult male and females. Curcumin extended the lifespan and improved the physiological traits that are related to aging. It is therefore possible that turmeric effects on survival are modulated directly by curcumin, and that curcumin might itself be beneficial while other compounds in turmeric may be triggering toxicity. The benefits of curcumin are likely observed due to its antioxidant role. It is unclear why an excess of curcumin could lead to toxicity, and this is an important area for future studies, which should conduct a dose-response experiment with both curcumin and turmeric to disentangle the roles of each of these compounds in the beneficial vs toxic effects in insects.
Our findings that females on turmeric diets were on average heavier at death contradicts a previous study by Rawal et al. (70) which showed that flies feeding on turmeric had no noticeable effect on body weight in D. melanogaster, although there was a slight decline in body weight in their experiment which was attributed to the process of aging. More studies are needed to corroborate whether turmeric feeding affect body traits in insects. Nevertheless, our data supports previous findings in nutritional research and showed that flies feeding on protein-rich diets had shortened lifespan. This is because amongst the protein sources used here, hydrolysed yeast contained the highest protein concentration (~60% according to manufacturer report). This corroborates previous findings in other flies that protein-rich diets shortened lifespan (28, 30–33), which has also been found in other insect species (e.g. 29, 34–37, 71). In fact, a recent comparative nutrition study showed that lifespan is maximized at lower protein-to-carbohydrate ratios, and that Dipterans often survive the longest in sugar-rich diets (72). It is important to note that, because there are no holidic diet developed for houseflies in the literature, we cannot interpret our results in relation to protein and carbohydrate ratios alone since our manipulation of protein source also varied other micronutrients which might be important for survival. Future studies manipulating these micronutrients will shed light into their relative contributions to the survival and body composition effects found here. Nonetheless, our results add to this body of literature by showing that houseflies also survive longer in sugar-rich diets.
Conclusion
Our study shows that turmeric can be an important ally to shorten lifespan of houseflies, a species with public health interest. This adds to the growing literature showing that turmeric can be used to manage insect pests of agriculture. Notably, turmeric has known health benefits to mammals and humans (e.g. 73, 74). This makes turmeric the perfect candidate to be used in agricultural insect pest control because it can have no – or even positive – effects on animals and humans that consume the food treated with turmeric. Overall, turmeric is emerging as a potent natural pesticide in both fundamental and applied entomology and our study corroborates the potential uses of turmeric for insect control.
Data availability statement
The original contributions presented in the study are included in the article/Supplementary Material. Further inquiries can be directed to the corresponding author.
Ethics statement
The manuscript presents research on animals that do not require ethical approval for their study.
Author contributions
SL: Conceptualization, Data curation, Funding acquisition, Investigation, Methodology, Writing – original draft, Writing – review & editing. LA: Formal Analysis, Investigation, Writing – original draft, Writing – review & editing. SM: Conceptualization, Funding acquisition, Investigation, Methodology, Resources, Supervision, Writing – review & editing. JM: Conceptualization, Data curation, Formal Analysis, Funding acquisition, Investigation, Methodology, Project administration, Resources, Software, Supervision, Validation, Visualization, Writing – original draft, Writing – review & editing.
Funding
The author(s) declare financial support was received for the research, authorship, and/or publication of this article. SL was funded by a Medical Research Scotland Undergraduate Vacation Scholarship VAC-1937-2023.
Acknowledgments
The authors would like to thank Elissavet Kaplaneli for the technical support in keeping the housefly colonies.
Conflict of interest
The authors declare that the research was conducted in the absence of any commercial or financial relationships that could be construed as a potential conflict of interest.
Publisher’s note
All claims expressed in this article are solely those of the authors and do not necessarily represent those of their affiliated organizations, or those of the publisher, the editors and the reviewers. Any product that may be evaluated in this article, or claim that may be made by its manufacturer, is not guaranteed or endorsed by the publisher.
Supplementary material
The Supplementary Material for this article can be found online at: https://www.frontiersin.org/articles/10.3389/finsc.2024.1376011/full#supplementary-material
Supplementary Table 1 | Complete model output for the analysis presented in the main text.
References
1. Gomez-Zavaglia A, Mejuto JC, Simal-Gandara J. Mitigation of emerging implications of climate change on food production systems. Food Res Int. (2020) 134:109256. doi: 10.1016/j.foodres.2020.109256
2. Tonnang HE, Sokame BM, Abdel-Rahman EM, Dubois T. Measuring and modelling crop yield losses due to invasive insect pests under climate change. Curr Opin Insect Sci. (2022) 50:100873. doi: 10.1016/j.cois.2022.100873
3. Lehmann P, Ammunét T, Barton M, Battisti A, Eigenbrode SD, Jepsen JU, et al. Complex responses of global insect pests to climate warming. Front Ecol Environ. (2020) 18:pp.141–150. doi: 10.1002/fee.2160
4. Skendžić S, Zovko M, Živković IP, Lešić V, Lemić D. The impact of climate change on agricultural insect pests. Insects. (2021) 12:440. doi: 10.3390/insects12050440
5. Damalas CA, Eleftherohorinos IG. Pesticide exposure, safety issues, and risk assessment indicators. Int J Environ Res Public Health. (2011) 8:1402. doi: 10.3390/IJERPH8051402
6. Sharma A, Kumar V, Shahzad B, Tanveer M, Sidhu GPS, Handa N, et al. Worldwide pesticide usage and its impacts on ecosystem. SN Appl Sci. (2019) 1:1–16. doi: 10.1007/S42452-019-1485-1
7. Turusov V, Rakitsky V, Tomatis L. Dichlorodiphenyltrichloroethane (DDT): ubiquity, persistence, and risks. Environ Health Perspect. (2002) 110:125–8. doi: 10.1289/ehp.02110125
8. Beard J. DDT and human health. Sci Total Environ. (2006) 355:78–89. doi: 10.1016/j.scitotenv.2005.02.022
9. Batish DR, Singh HP, Kohli RK, Kaur S. Eucalyptus essential oil as a natural pesticide. For Ecol Manage. (2008) 256:2166–74. doi: 10.1016/j.foreco.2008.08.008
10. Duke SO. (1990). Natural pesticides from plants, in: Microbial pathogenesis Advances in New Crops. Proceedings of the First National Symposium “New Crops: Research, Development, Economics”, Indianapolis, Indiana, USA (New York: Spinger), Vol. 23-26. pp. 511–7.
11. Schmutterer H. Properties and potential of natural pesticides from the neem tree, Azadirachta Indica. Annu Rev Entomol. (1990) 35(1):271–97. doi: 10.1146/Annurev.En.35.010190.001415
12. Gupta SC, Sung B, Kim JH, Prasad S, Li S, Aggarwal BB. Multitargeting by turmeric, the golden spice: From kitchen to clinic. Mol Nutr Food Res. (2013) 57:1510–28. doi: 10.1002/mnfr.201100741
13. Iweala EJ, Uche ME, Dike ED, Etumnu LR, Dokunmu TM, Oluwapelumi AE, et al. Curcuma longa (Turmeric): Ethnomedicinal uses, phytochemistry, pharmacological activities and toxicity profiles—A review. Pharmacol Res - Modern Chin Med. (2023) 6:100222. doi: 10.1016/j.prmcm.2023.100222
14. von Hellfeld R, Christie C, Derous D, Morimoto J. Super food or super toxic? Turmeric and spirulina as culprits for the toxic effects of food dyes in Drosophila. J Insect Physiol. (2024) 153:104600. doi: 10.1016/j.jinsphys.2023.104600
15. Damalas CA. Potential uses of turmeric (‘Curcuma longa’) products as alternative means of pest management in crop production. Plant Omics. (2011) 4:136–41. doi: 10.3316/informit.027888853397412
16. Raje KR, Hughes GP, Gondhalekar AD, Ginzel MD, Scharf ME. Toxicity of turmeric extracts to the termite reticulitermes flavipes (Blattodea: Rhinotermitidae). J Econ Entomol. (2015) 108:1479–85. doi: 10.1093/jee/tov109
17. Uysal H, Semerdöken S, Çolak DA, Ayar A. The hazardous effects of three natural food dyes on developmental stages and longevity of Drosophila melanogaster. Toxicol Ind Health. (2015) 31:624–9. doi: 10.1177/0748233713480206
18. De Souza Tavares W, Akhtar Y, Gonçalves GLP, Zanuncio JC, Isman MB. Turmeric powder and its derivatives from Curcuma longa rhizomes: Insecticidal effects on cabbage looper and the role of synergists. Sci Rep. (2016) 6:1–11. doi: 10.1038/srep34093
19. Muhammad U, Nawaz Khattak T, Rahman H, Daud MK, Murad W, Azizullah A. Effects of Neem (Azadirachta indica) seed and Turmeric (Curcuma longa) rhizome extracts on aphids control, plant growth and yield in okra. J Appl Bot Food Qual. (2018) 91:194–201. doi: 10.5073/jabfq.2018.091.026
20. Ali S, Sagheer M, ul Hassan M, Abbas M, Hafeez F, Farooq M, et al. Insecticidal activity of turmeric (Curcuma longa) and garlic (Allium sativum) extracts against red flour beetle, Tribolium castaneum: A safe alternative to insecticides in stored commodities. J Entomol. Zool. Stud. (2014) 2:201–5.
21. Jilani G, Saxena RC. Repellent and feeding deterrent effects of turmeric oil, sweetflag oil, neem oil, and a neem-based insecticide against lesser grain borer (Coleoptera: Bostrychidae). J Econ Entomol. (1990) 83:629–34. doi: 10.1093/jee/83.2.629
22. Visakh NU, Pathrose B, Chellappan M, Ranjith MT, Sindhu PV, Mathew D. Extraction and chemical characterisation of agro-waste from turmeric leaves as a source of bioactive essential oils with insecticidal and antioxidant activities. Waste Manage. (2023) 169:1–10. doi: 10.1016/j.wasman.2023.06.030
23. Vineesh PJ, Mathew A, Kavyamol PM, Vineetha VP, Rajagopal R, Alfarhan A, et al. Essential oils of cinnamon, turmeric and neem as potential control agents against home-invading acid flies (Paederus fuscipes) and darkling beetles (Luprops tristis). J King Saud Univ - Sci. (2023) 35:102363. doi: 10.1016/j.jksus.2022.102363
24. Fu WJ, Liu J, Zhang M, Li JQ, Hu JF, Xu LR, et al. Isolation, purification and identification of the active compound of turmeric and its potential application to control cucumber powdery mildew. J Agric Sci. (2018) 156:358–66. doi: 10.1017/S0021859618000345
25. Roy GC, Chakraborty K, Parthasarathi Nandy MN. Pros and cons of curcumin as bioactive phytocompound for effective management of insect pest. Am Sci Res J Eng. Technol. Sci. (2014) 7:31–43.
26. Deans CA, Behmer ST, Tessnow AE, Tamez-Guerra P, Pusztai-Carey M, Sword GA. Nutrition affects insect susceptibility to Bt toxins. Sci Rep. (2017) 7:1–9. doi: 10.1038/srep39705
27. Wada-Katsumata A, Schal C, Ozaki M, Pauchet Y. Salivary digestion extends the range of sugar-aversions in the German Cockroach. Insects. (2021) 12:263. doi: 10.3390/insects12030263
28. Lee KP, Simpson SJ, Clissold FJ, Brooks R, Ballard JWO, Taylor PW, et al. Lifespan and reproduction in Drosophila: New insights from nutritional geometry. Proc Natl Acad Sci United States America. (2008) 105:2498–503. doi: 10.1073/pnas.071078710
29. Maklakov AA, Simpson SJ, Zajitschek F, Hall MD, Dessmann J, Clissold F, et al. Report sex-specific fitness effects of nutrient intake on reproduction and lifespan. Curr Biol. (2008) 18:1062. doi: 10.1016/j.cub.2008.06.059
30. Prabhu V, Perez-Staples D, Taylor PW. Protein: carbohydrate ratios promoting sexual activity and longevity of male Queensland fruit flies. J Appl Entomol. (2008) 132:575–82. doi: 10.1111/j.1439-0418.2007.01265.x
31. Fanson BG, Weldon CW, Pérez-Staples D, Simpson SJ, Taylor PW. Nutrients, not caloric restriction, extend lifespan in Queensland fruit flies (Bactrocera tryoni). Aging Cell. (2009) 8:514–23. doi: 10.1111/j.1474-9726.2009.00497
32. Fanson BG, Fanson K, Taylor PW. Cost of reproduction in the Queensland fruit fly: Y-model versus lethal protein hypothesis. Proc R Soc B: Biol Sci. (2012) 279:4893. doi: 10.1098/rspb.2012.2033
33. Jensen K, McClure C, Priest NK, Hunt J. Sex-specific effects of protein and carbohydrate intake on reproduction but not lifespan in Drosophila melanogaster. Aging Cell. (2015) 14:605–15. doi: 10.1111/acel.12333
34. Dussutour A, Simpson SJ. Ant workers die young and colonies collapse when fed a high-protein diet. Proc R Soc B: Biol Sci. (2012) 279:2402–8. doi: 10.1098/rspb.2012.0051
35. Kay AD, Taylor Z, Heinen JL, Marsh TG, Holway DA. Nutrition and interference competition have interactive effects on the behavior and performance of Argentine ants. Ecology. (2010) 91:57–64. doi: 10.1890/09-0908.1
36. Dussutour A, Poissonnier L-A, Buhl J, Simpson SJ. Resistance to nutritional stress in ants: when being fat is advantageous. J Exp Biol. (2016) 219:pp.824–833. doi: 10.1242/jeb.136234
37. Pirk CWW, Boodhoo C, Human H, Nicolson SW. The importance of protein type and protein to carbohydrate ratio for survival and ovarian activation of caged honeybees (Apis mellifera scutellata). Apidologie. (2010) 41:62–72. doi: 10.1051/apido/2009055
38. Ko AE, Schal C, Silverman J. Diet quality affects bait performance in German cockroaches (Dictyoptera: Blattellidae). Pest Manage Sci. (2016) 72:1826–36. doi: 10.1002/PS.4295
39. Morimoto J. Uric acid metabolism modulates diet-dependent responses to intraspecific competition in Drosophila larvae. ISCIENCE (2022) 25:105598. doi: 10.1016/j.isci.2022.105598
40. Simpson SJ, Raubenheimer D. The geometric analysis of nutrient–allelochemical interactions: a case study using locusts. Ecology. (2001) 82:422–39. doi: 10.1890/0012-9658(2001)082[0422:TGAONA]2.0.CO;2
41. Slansky F. Allelochemical–nutrient interactions in herbivore nutritional ecology. In: Rosenthal GA, Berenbaum MR, editors. Herbivores: Their Interaction with Secondary Plant Metabolites (San Diego: Academic Press Inc.) (1992). p. 135–74.
42. Cervelin V, Fongaro G, Pastore JB, Engel F, Reimers MA, Viancelli A. Enterobacteria associated with houseflies (Musca domestica) as an infection risk indicator in swine production farms. Acta Tropica. (2018) 185:13–7. doi: 10.1016/j.actatropica.2018.04.024
43. Neupane S, Nayduch D, Zurek L. House flies (Musca domestica) pose a risk of carriage and transmission of bacterial pathogens associated with bovine respiratory disease (BRD). Insects. (2019) 10:358. doi: 10.3390/insects10100358
44. Poudel A, Hathcock T, Butaye P, Kang Y, Price S, Macklin K, et al. Multidrug-resistant escherichia coli, Klebsiella pneumoniae and staphylococcus spp. in houseflies and blowflies from farms and their environmental settings. Int J Environ Res Public Health. (2019) 16:3583. doi: 10.3390/ijerph16193583
45. Raele DA, Stoffolano JG, Vasco I, Pennuzzi G, La Porta MCN, Cafiero MA. Study on the role of the common house fly, Musca domestica, in the spread of ORF virus (poxviridae) DNA under laboratory conditions. Microorganisms. (2021) 9:2185. doi: 10.3390/microorganisms9112185
46. Toto NA, Elhenawy HI, Eltaweil AS, El-Ashram S, El-Samad LM, Moussian B, et al. Musca domestica (Diptera: Muscidae) as a biological model for the assessment of magnetite nanoparticles toxicity. Sci Total Environ. (2022) 806:151483. doi: 10.1016/j.scitotenv.2021.151483
47. Cousins M, Sargeant JM, Fisman D, Greer AL. Modelling the transmission dynamics of Campylobacter in Ontario, Canada, assuming house flies, Musca domestica, are a mechanical vector of disease transmission. R Soc Open Sci. (2019) 6. doi: 10.1098/rsos.181394
48. Meraz Jiménez AdeJ, López Santos A, García Munguía CA, Torres González JA, García Munguía AM. Potential distribution of Musca domestica in Jesús María Municipality, Aguascalientes, Mexico, based on climate change scenarios. Rev Mexicana Cienc Pecuarias. (2019) 10:14–29. doi: 10.22319/rmcp.v10i1.4241
49. Wickham H. ggplot2: Elegant Graphics for Data Analysis. New York: Springer-Verlag New York (2016). Available at: https://ggplot2.tidyverse.org.
50. Wickham H, Averick M, Bryan J, Chang W, D’Agostino L, McGowan A, et al. Welcome to the tidyverse. J Open Source Softw. (2019) 4:1686. doi: 10.21105/joss.01686
51. Wickham H. stringr: Simple, Consistent Wrappers for Common String Operations (2022). Available online at: https://stringr.tidyverse.orghttps://github.com/tidyverse/stringr.
52. Fox J, Weisberg S. An R Companion to Applied Regression, Third edition. 411 Thousand Oaks CA: Sage (2019). Available at: https://socialsciences.mcmaster.ca/jfox/Books/Companion/.
53. Wickham H, François R, Henry L, Müller K, Vaughan D. dplyr: A grammar of data manipulation (2023). Available online at: https://CRAN.R-project.org/package=dplyr.
54. Therneau T. A Package for Survival Analysis in R. R Package version (2023) 3.5-:536 7. https://CRAN.R-project.org/package=survival.
55. Horikoshi M, Tang Y. Data visualization tools for statistical analysis results (2018). Available online at: https://CRAN.R-Project.Org/Package=ggfortify.
56. Wickham H, Vaughan D, Girlich M. tidyr: Tidy Messy Data (2023). Available online at: https://github.com/tidyverse/tidyr.
57. Molleman F, Javoiš J, Esperk T, Teder T, Davis RB, Tammaru T. Sexual differences in weight loss upon eclosion are related to life history strategy in Lepidoptera. J Insect Physiol. (2011) 57:712–22. doi: 10.1016/j.jinsphys.2011.02.009
58. Fairbanks LD, Burch GE. Rate of water loss and water and fat content of adult Drosophila melanogaster of different ages. J Insect Physiol. (1970) 16:1429–36. doi: 10.1016/0022-1910(70)90141-1
59. Rahman MM, Al Noman MA, Hossain MW, Alam R, Akter S, Kabir MM, et al. Curcuma longa L. Prevents the Loss of β-Tubulin in the Brain and Maintains Healthy Aging in Drosophila melanogaster. Mol Neurobiol. (2022) 59:1819–35. doi: 10.1007/S12035-021-02701-6
60. Scannapieco AC, Sørensen JG, Loeschcke V, Norry FM. Heat-induced hormesis in longevity of two sibling Drosophila species. Biogerontology. (2007) 8:315–25. doi: 10.1007/s10522-006-9075-1
61. Sorensen JG, Sarup P, Kristensen TN, Loeschcke V. Temperature-induced hormesis in drosophila. Mild Stress Health. Aging: Appl. Hormesis Aging Res Interventions. (2008), 65–79. doi: 10.1007/978-1-4020-6869-0_5
62. Gomez FH, Bertoli CI, Sambucetti P, Scannapieco AC, Norry FM. Heat-induced hormesis in longevity as correlated response to thermal-stress selection in Drosophila buzzatii. J Thermal Biol. (2009) 34:17–22. doi: 10.1016/j.jtherbio.2008.09.003
63. Moskalev AA, Plyusnina EN, Shaposhnikov MV. Radiation hormesis and radioadaptive response in Drosophila melanogaster flies with different genetic backgrounds: the role of cellular stress-resistance mechanisms. Biogerontology. (2011) 12:253–63. doi: 10.1007/s10522-011-9320-0
64. Henry Y, Renault D, Colinet H. Hormesis-like effect of mild larval crowding on thermotolerance in Drosophila flies. J Exp Biol. (2018) 221:jeb169342. doi: 10.1242/jeb.178681
65. Lushchak OV, Karaman HS, Kozeretska IA, Koliada AK, Zabuga OG, Pisaruk AV, et al. Larval crowding results in hormesis-like effects on longevity in Drosophila: timing of eclosion as a model. Biogerontology. (2019) 20:191–201. doi: 10.1007/s10522-018-9786-0
66. Cutler GC. Insects, insecticides and hormesis: evidence and considerations for study. Dose-response. (2013) 11. doi: 10.2203/dose-response.12-008.Cutler
67. Suckow BK, Suckow MA. Lifespan extension by the antioxidant curcumin in Drosophila Melanogaster. Int J Biomed Sci. (2006) 2:402. doi: 10.59566/IJBS.2006.2401
68. Chen Y, Liu X, Jiang C, Liu L, Ordovas JM, Lai CQ, et al. Curcumin supplementation increases survival and lifespan in Drosophila under heat stress conditions. BioFactors (2018) 44(6):577–87. doi: 10.1002/biof.1454
69. Shen LR, Xiao F, Yuan P, Chen Y, Gao QK, Parnell LD, et al. Curcumin-supplemented diets increase superoxide dismutase activity and mean lifespan in Drosophila. Age. (2013) 35:1133–42. doi: 10.1007/S11357-012-9438-2
70. Rawal S, Singh P, Gupta A, Mohanty S. Dietary intake of curcuma longa and emblica officinalis increases life span in Drosophila melanogaster. BioMed Res Int. (2014) 2014:910290. doi: 10.1155/2014/910290
71. Despland E, Noseworthy M. How well do specialist feeders regulate nutrient intake? Evidence from a gregarious tree-feeding caterpillar. J Exp Biol (2006) 209(7):1301–9. doi: 10.1242/jeb.02130
72. Morimoto J. Optimum ratio of dietary protein and carbohydrate that maximises lifespan is shared among related insect species. Aging Cell. (2023) 00. doi: 10.1111/acel.14067
73. Labban L. Medicinal and pharmacological properties of Turmeric (Curcuma longa): A review. Int J Pharm BioMed Sci. (2014) 5:17–23.
Keywords: pesticide, environment, food security, contaminants, SDG
Citation: Laurie S, Ainslie L, Mitchell S and Morimoto J (2024) Turmeric shortens lifespan in houseflies. Front. Insect Sci. 4:1376011. doi: 10.3389/finsc.2024.1376011
Received: 24 January 2024; Accepted: 11 March 2024;
Published: 10 April 2024.
Edited by:
Ruth Archer, University of Ulm, GermanyReviewed by:
Kwang Pum Lee, Seoul National University, Republic of KoreaCandice Ann Owen, University of Chester, United Kingdom
Copyright © 2024 Laurie, Ainslie, Mitchell and Morimoto. This is an open-access article distributed under the terms of the Creative Commons Attribution License (CC BY). The use, distribution or reproduction in other forums is permitted, provided the original author(s) and the copyright owner(s) are credited and that the original publication in this journal is cited, in accordance with accepted academic practice. No use, distribution or reproduction is permitted which does not comply with these terms.
*Correspondence: Juliano Morimoto, juliano.morimoto@abdn.ac.uk