Sensory Qualities of Oysters Unaltered by a Short Exposure to Combined Elevated pCO2 and Temperature
- 1Marine Biology and Ecology Research Centre, School of Biological and Marine Sciences, Plymouth University, Plymouth, United Kingdom
- 2Food, Health and Nutrition, School of Biological and Marine Sciences, Plymouth University, Plymouth, United Kingdom
- 3Shimoda Marine Research Centre, University of Tsukuba, Tsukuba, Japan
- 4Marine Conservation and Policy Research Centre, School of Biological and Marine Sciences, Plymouth University, Plymouth, United Kingdom
- 5UN Environment World Conservation Monitoring Centre, Cambridge, United Kingdom
- 6School of Geography, Earth and Environmental Science, Plymouth University, Plymouth, United Kingdom
Reliance on the marine environment for the provision of food is ever-increasing, but future climate change threatens production. Despite this concern, the impact on seafood quality and success of the seafood industry is unknown. Using a short-term study, we test these concerns using a major aquaculture species—Crassostrea gigas—exposing them to three acidification and warming scenarios: (1) ambient pCO2 (~400 ppm) & control temperature (15°C), (2) ambient pCO2 (~400 ppm) & elevated temperature (20°C), (3) elevated pCO2 (~1,000 ppm) & elevated temperature (20°C). Oyster quality was assessed by scoring appearance, aroma, taste, and overall acceptability. A panel of five experts was asked to score nine oysters—three from each treatment—according to agreed criteria. Results indicate that these levels of acidification and warming did not significantly alter the sensory properties of C. gigas, and notably the overall acceptability remained unchanged. Non-statistically supported trends suggest that several sensory attributes—opacity, mouthfeel, aspect of meat, shininess, meat resistance, meat texture, and creaminess—may improve under acidification and warming scenarios. These findings can be considered positive for the future of the aquaculture and food sectors. Crassostrea gigas therefore is expected to remain a key species for food security that is resilient to climate change, whilst retaining its valuable attributes.
Introduction
Seafood represents a significant contribution to the provision of animal protein globally—approximatively 17% of the global population animal protein intake in 2013 was from fish consumption (FAO, 2016). If the world population is to reach between 9.6 and 12.3 billion by the year 2100 as predicted (Gerland et al., 2014), demand for animal protein is unlikely to be met by terrestrial farming. Instead, increasing reliance on the marine environment for the provision of proteins and other products is expected (Delgado, 2003; Cooley et al., 2012).
The “Blue Revolution”—the emergence of aquaculture as an important alternative to meat production from land agricultural activity—is thought by many to be a solution to securing food provision in the future (Naylor et al., 2000; Tacon and Metian, 2013; Barange et al., 2014). In 2015, total aquaculture production volume was estimated at over 106 million tons and valued in excess of US$162.9 billion; ~15% of this total is attributed to molluscan aquaculture (over 16 million tons; worth over US$18 billion) (FAO data1) In comparison to other seafood species, the production cost of many molluscs is relatively low, making them ideal for aquaculture.
Due to pressure on natural fish stocks from overfishing and habitat destruction (Molfese et al., 2014; McCauley et al., 2015; Macura et al., 2016), a switch to more sustainable aquaculture practices, such as mollusc production, is timely. However, there is increasing concern over the fate of seafood harvesting and production under future climate scenarios (Cooley et al., 2015; Ekstrom et al., 2015; Lloret et al., 2016). To date, the main scientific focus has been on the biomass produced, with much of the evidence suggesting aquaculture production will be negatively impacted by climate change due to increased larvae and juvenile mortality, and changes in shell development and calcification rates (Hettinger et al., 2012; Guo et al., 2015). In addition, environmental stressors can also influence the physiology of marine organisms, altering the quality of the meat (Borderias and Sanchez-Alonso, 2011). The sustainability of the industry is, in part, driven by consumer demand. It is therefore crucial to detect the potential factors (e.g., seafood quality) that may negatively or positively affect public perception and consumer choice regarding molluscs, such that the aquaculture sector is better positioned to make informed decisions regarding the choice of cultured species and respond to consumer demand.
Seafood products are known for their high nutritional value, and are key to human health and well-being (Lloret et al., 2016). Oysters, especially, are consumed around the world and are a good example of highly nutritious seafood. It was estimated that in 2015 throughout the globe, over 5 million tons of oysters were produced, worth in excess of US$4 billion, of which 1,600 tons was produced in the UK, worth in excess of US$6 million FAO data1. The biological effects of ocean acidification and warming on individuals and the production industry are already well documented (Lemasson et al., 2017), with several hatcheries experiencing significant decline in production and considerable economic losses (Barton et al., 2012, 2015). However, less attention has been given to the quality of shellfish, including aesthetic aspects, taste, and nutritional properties, despite being a critical factor in consumer's food choice (Alfnes et al., 2006). To date, only one study has looked at the effects of ocean acidification on the eating quality of seafood, and showed that future climate scenarios negatively affect the quality of northern shrimps (Pandalus borealis) by altering their sensory quality (taste and texture) (Dupont et al., 2014). Given that the physiology of adult oysters is negatively impacted by warming and acidification (Scanes et al., 2017, but see Lemasson et al., under review), it is possible that their sensory quality will reflect such changes.
Here, we evaluate changes to the sensory properties of the Pacific oyster Crassostrea gigas using descriptive sensory analysis, to determine the effects of exposure to acidification and warming conditions on consumer perception.
Methods
Descriptive sensory analysis using parameters such as appearance, aroma, and taste, is commonly used to assess seafood freshness, shelf-life, and acceptability (Liu et al., 2010; Šimat et al., 2012; Azpeitia et al., 2017). This technique is considered an accurate quality predictor (Reineccius, 1991; Chang et al., 1998), and has been used extensively for oyster quality assessments (Aaraas et al., 2004; Cao et al., 2009; Buzin et al., 2011; Cochet et al., 2015).
Experimental Design and Set-Up
Here, Pacific oysters (C. gigas) under 2 years of age and of UK market size (10–12 cm long and 80–100 g) were obtained from Menai Oysters and Mussels Ltd. (Llanfairpwllgwyngyll, Wales, UK) and exposed to ocean acidification and warming scenarios predicted for the UK coastline for 2100 [temperature estimates based on the medium emission scenario IPCC SRES A1B and UKCP09 predictions2; pCO2 estimates based on predictions by the Marine Ecosystem Evolution in a Changing Environment (MEECE)3 for 2080–2099]. Two levels of pCO2 (ambient ~400 ppm, elevated ~1,000 ppm) and two temperatures (control 15°C, elevated 20°C) were used to create three distinct climate scenarios (Figure 1). The first treatment, hereafter referred to as ACT, replicated the current climate (ambient pCO2 & control temperature). The other two treatments corresponded to future scenarios of ocean warming and acidification; one simulating warming alone, hereafter referred to as AET (ambient pCO2 & elevated temperature), due to local geological conditions allowing the buffering of acidification (such as the occurrence of limestone), and the other simulating acidification and warming simultaneously, hereafter referred to as EET (elevated pCO2 & elevated temperature; Humphreys, 2016). No “acidification alone” treatment was used, as it does not reflect a predicted (realistic) scenario for the region (see discussions in Reum et al., 2015; Humphreys, 2016).
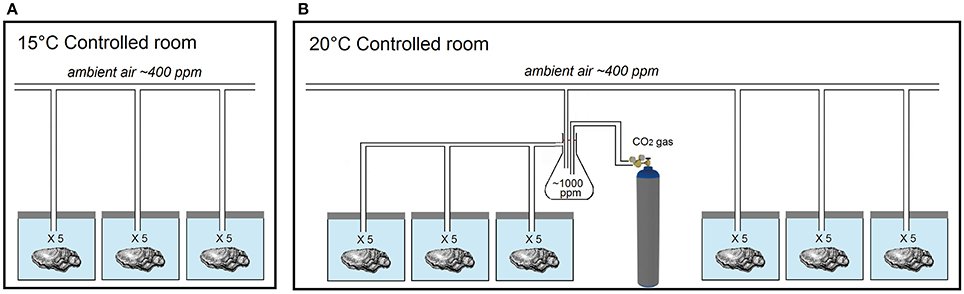
Figure 1. Experimental design used to expose oysters to three acidification and warming treatments. (A) temperature-controlled room kept at 15°C and holding oysters supplied with ambient air at ~400 ppm. (B) temperature-controlled room kept at 20°C and holding oysters supplied with ambient air at ~400 ppm and oysters supplied with ~1,000 ppm pCO2. Each tank contained 5 oysters, one for each of the 5 panelists, such that there were 15 oysters per experimental treatment, and 9 oysters per panelist.
Three replicate tanks (33 × 20 × 19 cm) filled with 9 L of filtered seawater were established per treatment, with each tank containing five randomly allocated individual oysters of similar age and size class. Oysters were exposed to treatment conditions for 5 days, as longer exposure duration while unfed would have caused physiological stress from starvation and confounded the effects of the treatment. Each replicate tanks were cleaned and a third of the water partially renewed daily, with either seawater or deionized water as needed to maintain stable salinity levels. Oxygen concentration was monitored to insure adequate aeration (air saturation >90%). Throughout the duration of the experiment, oysters were subjected to a 12 h light/12 h darkness cycle. Oysters were not fed, so as not to alter the natural taste of the Menai variety attributed to the site-specific seawater characteristics (Krijnen, S. Menai Oysters and Mussels Ltd. pers. communication).
Replicate tanks were aerated with either ambient air (pCO2 ~400 ppm) or CO2-enriched air (pCO2 ~1,000 ppm). CO2-enriched air was created by a controlled release of CO2 into a Buchner flask using a multistage CO2 regulator (EN ISO 7291; GCE, Worksop, UK) and mixed with ambient air until a concentration of ~1,000 ppm pCO2 was achieved. The CO2 level in the ambient and CO2-enriched pipes were recorded using a CO2 analyser (LI-820; LI-COR, Lincoln, NE, USA). Tanks were maintained at control (~15°C) and elevated (20°C) temperatures using controlled-temperature rooms.
Measurements of Seawater Parameters
At least once a day throughout the exposure duration, temperature, salinity, and pH were measured in all replicate tanks (Table 1). Salinity was measured using a handheld refractometer (D&D The Aquarium Solution Ltd, Ilford, UK). Temperature was measured using a digital thermometer (TL; Fisher Scientific, Loughborough, UK). pH was measured using a microelectrode (InLab® Expert Pro-ISM; Mettler- Toledo Ltd, Beaumont Leys, UK) coupled to a pH meter (S400 SevenExcellence™; Mettler-Toledo Ltd, Beaumont Leys, UK) after calibration with NIST traceable buffers. One hundred and twenty-five milliliters of water samples were taken once on day 5 for Total Alkalinity (AT) measurements from each of the replicate tanks, and directly analyzed by automatic Gran titration (Titralab AT1000© Hach Company/Hach Lange GmbH). Partial pressure of carbon dioxide (pCO2), and saturation states of calcite and aragonite (Ωca and Ωar), were calculated at the end of the experiment (Table 1), using CO2 SYS (Pierrot et al., 2006) employing constants from Mehrbach et al. (1973) refitted to the NBS pH scale by Dickson and Millero (1987) and the KSO4 dissociation constant from Dickson (1990).
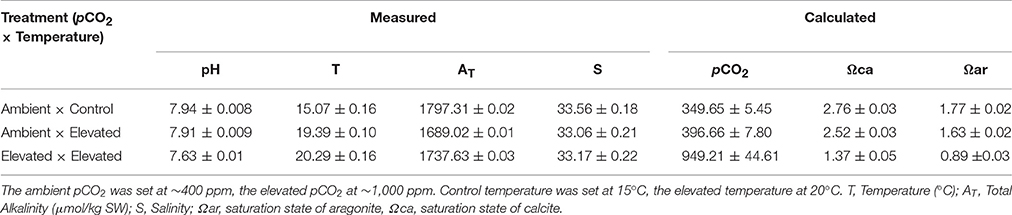
Table 1. Physical and chemical characteristics of seawater in the three experimental treatments for Crassostrea gigas [presented as mean values over the duration of the experiment ± standard deviation (s.d.)].
Development of Sensory Terms and Evaluation Method
This study was carried out in accordance with the recommendations of the University of Plymouth's policy on the Ethical Principles for Research Involving Human Participants, and approved by the University of Plymouth Research Ethics Committee, with written informed consent from all subjects. All subjects gave written informed consent in accordance with the Declaration of Helsinki. The protocol was approved by the University of Plymouth Research Ethics Committee. A sensory panel was selected according to criteria IV.4.1.2 (Codex Standards, 1999) and consisted of five panelists (A–E), each of them expert in the field of food sensory evaluation, oyster production, or oyster meroir—the effect of seawater environment on the taste and texture of oysters—and was trained during group sessions following standard methods (ISO 6658, 1985). A focus group activity aimed to: (i) develop the attributes and descriptors to be used in the descriptive sensory analysis, (ii) obtain a consensus on their definition, and (iii) develop a standardized method of evaluation. The panelists agreed on three major categories of attributes: Appearance, Aroma, and Degustation. Each main category comprised several attributes (see Table 2), whilst Overall Acceptability was also chosen as a key attribute, adding up to 17 attributes in total. The panel agreed on the following method of evaluation. First, the appearance of the meat was visually assessed and scores for attributes including shell-to-meat ratio, aspect, shininess, and opacity of the meat were recorded. Then, the oyster (in its shell and liquor) was brought to the nose to assess the aroma, with liquor and without liquor. Finally, the degustation was assessed in four steps: liquor (saltiness and depth), meat texture (resistance, juiciness, texture), meat taste and flavor (sweetness, creaminess, meatiness), and aftertaste (aftertaste intensity and mouthfeel). Panelists were required to score each attribute using a 4 or 5-point scale semantically anchored with descriptors on the extremes and the midpoint. Each oyster was then finally scored for overall acceptability (likeness) using a hedonic scale (Table 2). The scales were linked to demerit scales ranging from 0 (optimum score) to 3 (least favorable score; Table 2).
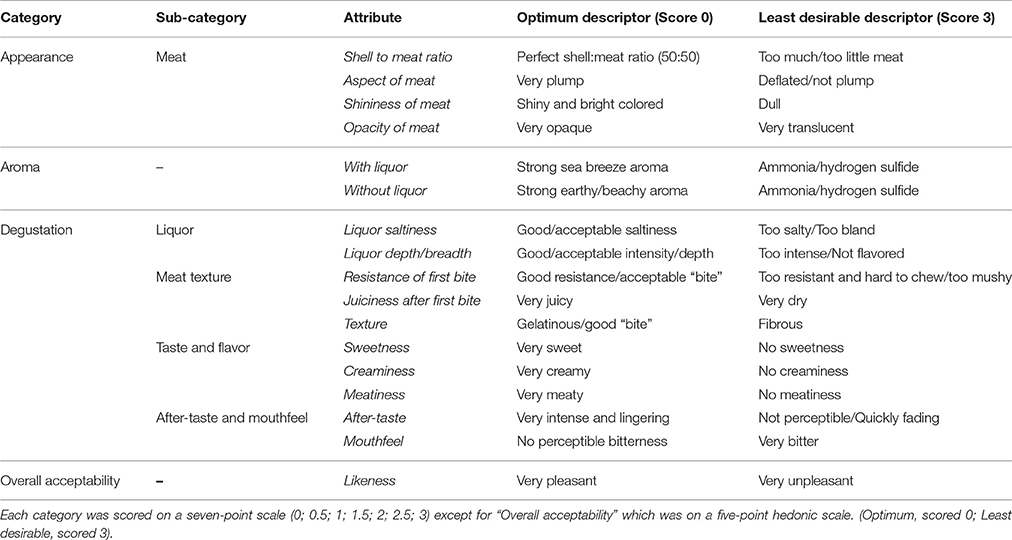
Table 2. Assessment categories and attributes selected during the focus session and used for the sensory analysis, along with the extreme descriptors.
Sample Preparation and Presentation
All oysters were blind-coded by assigning them a randomly generated 3-digit number. At the end of the exposure period, oysters were brought to the Food and Nutrition Unit at Plymouth University, and prepared for tasting by the same highly experienced practitioner. They were manually shucked by cutting the adductor muscle with an oyster knife after 1 h at room temperature. The meat was left inside the shell, with the liquor, taking care to avoid spillage. The oysters were individually labeled and presented to each panelist on aluminum trays. Each panelist (5) was presented with an oyster from each replicate tank (9 oysters in total per panelist; see Figure 1). The order of presentation was randomized across panelists. The assessments were carried out in individual partitioned booths, as recommended in standard methods (ISO 6658, 1985).
Statistical Analyses
Data for each attribute were analyzed using two-way nested Analysis of Variance, with “Treatment” as a fixed factor, and “Panelist” as a random factor nested within “Treatment”. This type of model is commonly used for analysis of sensory studies data (Aaraas et al., 2004; Buzin et al., 2011; Cochet et al., 2015). Homogeneity of variance was checked using Cochran's C test. When data did not meet this assumption, they were transformed using ln(x+1). Where significant differences were present, post-hoc Student-Newman-Keuls (SNK) multiple comparison of means was performed to determine which treatment levels differed. All data were analyzed using the GMAV 5 software (University of Sydney, Australia).
Results
The sensory attributes of oysters were not significantly impacted by the treatment alone (p > 0.05 in all instances; Figures 2, 3). However, there were significant differences in the scores allocated to five of the attributes between panelists within treatment levels: shell-to-meat ratio [F(12, 44) = 3.39; p < 0.001], opacity [F(12, 44) = 2.10; p < 0.05], aroma without liquor [F(12, 44) = 2.54; p < 0.05], aftertaste [F(12, 44) = 3.01; p < 0.01], and mouthfeel [F(12, 44) = 4.41; p < 0.001; Figure 4].
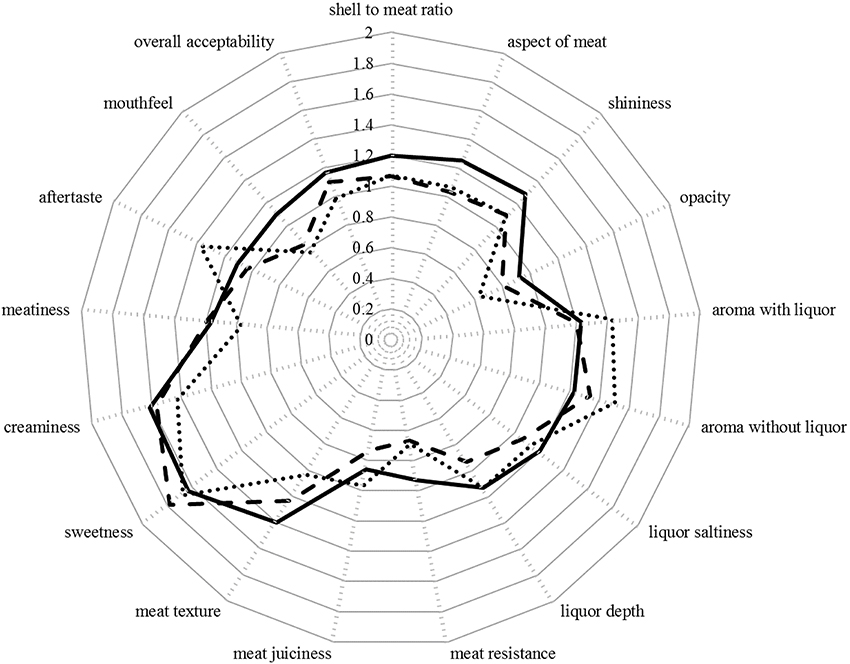
Figure 2. Mean score attributed by the panelists to each of the 17 oyster attributes (n = 9 oysters per treatment). Continuous line: Ambient pCO2 & control temperature; Dashed line: Ambient pCO2 & elevated temperature; Dotted line: Elevated pCO2 & elevated temperature. The scales ranged from 0 (optimum score 0) to 3 (least favorable score).
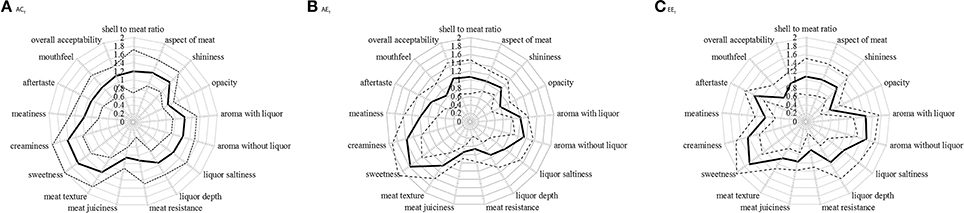
Figure 3. Score attributed by the panelists to each of the 17 oyster attributes. (A) Ambient pCO2 & control temperature (ACT), (B) Ambient pCO2 & elevated temperature (AET), (C) Elevated pCO2 & elevated temperature (EET). Mean (n = 9) scores (continuous line) with confidence interval (dotted lines), on scales ranging from 0 (optimum score) to 3 (least favorable score).
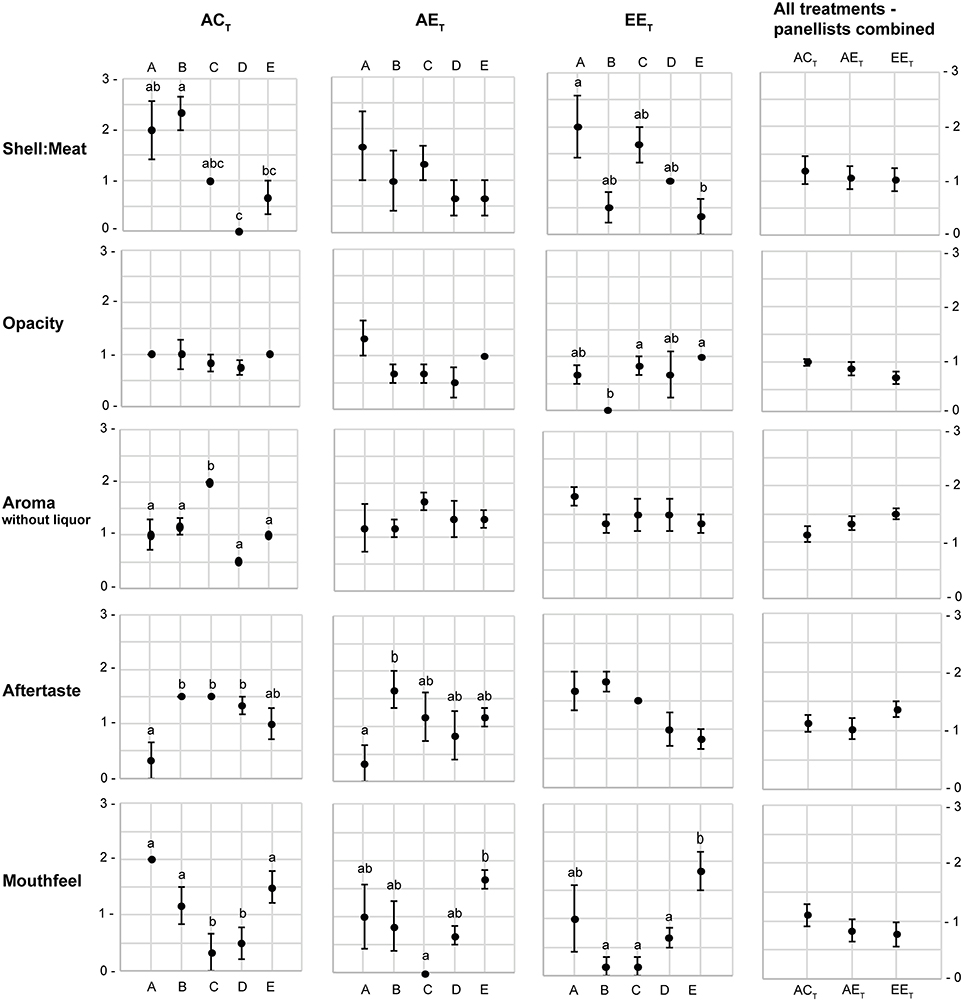
Figure 4. Mean score (±S.E.) of the attributes that displayed significant interaction between “panelist” and “treatment”. Letters A–E represent the five panelists. ACT, ambient pCO2and control temperature; AET, ambient pCO2and control temperature; EET, elevated pCO2 and elevated temperature. All attribute scores ranged from 0 to 3 (0 representing the optimum score, and 3 the least favorable score). Treatment levels that do not share a letter are significantly different.
While panelists agreed on the shell-to-meat ratio score in the AET treatment, there were large variations in scoring between panelists in the ACT and EET treatments (Figure 4). Moreover, panelists agreed on the opacity of the meat score for oysters in the ACT and AET treatments, whereas there were large variations in scoring in the EET treatment. There was an apparent improving trend in the opacity of oysters with stressful treatment [as depicted by a decreasing average score from ~1 (somewhat opaque) to ~0.5 (very opaque); Figure 4]. One panelist scored the aroma without liquor differently than all four others in the ACT treatment, but they all agreed on scores in the AET and EET treatments. There was an apparent trend for worsened aroma with stressful treatment, as depicted by an increasing average score from ~1.2 (distinctive earthy/beachy aroma) to ~1.5 (lack of aroma; Figure 4). One panelist also scored the aftertaste differently than the other assessors in the ACT and AET treatments, whereas no differences between panelists were observed in the EET treatment. Finally, there were significant differences in the scoring of the mouthfeel between panelists across all three treatments. Mouthfeel scores followed an apparent improving trend with stressful treatment, from ~1.1 (neutral/pleasant) to ~0.7 (very pleasant; Figure 4).
Several other attributes appeared to be improving (decreasing score) with the level of stress applied, including aspect of meat, shininess, meat resistance, meat texture, and creaminess (Figure 2).
Discussion
We investigated whether the sensory quality of Pacific oyster C. gigas would be affected by short-term exposure to seawater acidification and warming conditions. Sensory and cognitive perceptions have been recognized as key elements dictating consume attitudes toward seafood (Carlucci et al., 2015) and changes in environmental conditions have been predicted to negatively alter their taste and quality as a result of physiological stress (Borderias and Sanchez-Alonso, 2011; Dupont et al., 2014). Our work revealed that acidification and warming conditions did not significantly alter the sensory properties of C. gigas after a short exposure, with the overall acceptability of oysters remaining unchanged to a panel of seafood experts. In general, oysters exposed to future conditions scored as “pleasant,” irrespective of treatments.
Admittedly, the growth phase of oysters is much longer than the exposure duration used in this study, and previous environmental history during growth can have an impact on the final product characteristics. Physiological stress arising from changes in environmental conditions has been shown to reduce the quality and affect the sensory properties in other seafood species through physical, morphological, and biochemical ways (Borderias and Sanchez-Alonso, 2011; Dupont et al., 2014). Many species take months to acclimate to stressful conditions, if they acclimate at all, and thus it is possible that changes to the taste and sensory properties can only be discerned during that initial acclimation period. Although, no changes were found in this relatively short exposure study, acidification and warming has been shown to induce important physiological stress in oysters over longer exposure duration (Scanes et al., 2017; Lemasson et al., under review). While we concede that this limited exposure may not be sufficient to induce significant long-term physiological changes, some mechanisms of physiological responses, such as protein synthesis, oxygen supply, and acid-base regulation, are also involved in short-term exposures, but may not be detectable nor detrimental (Gazeau et al., 2007; Pörtner, 2008). The scenario used here reflects transient warming and acidification events—thought to increase and intensify in the future (Stocker et al., 2013; Helmuth et al., 2014; Fischer and Knutti, 2015)—occurring due to natural temporal variability in seawater conditions (Hauri et al., 2013; Artioli et al., 2014; Guyondet et al., 2014), but also events such as heat waves and coastal upwellings, rather than more prolonged and continuous environmental conditions (Ghedini et al., 2015). Such events have been shown to yield disproportionally high ecological change, and are exacerbated by additional local disturbances (Ghedini et al., 2015 and references therein; Wernberg et al., 2013). Thus, transient warming and acidification events have the potential to harm wild and aquaculture stocks, particularly those located near anthropogenically disturbed coastlines, if they occur close to the time of harvest. Studies have shown that pre-harvest conditions—even of relatively short-duration, known as “finishing”—can hold important implications for the quality of the final product by altering oysters physiology and consequently yielding different biochemical make-up, influencing the sensory attributes (Cruz-Romero et al., 2004; Fratini et al., 2013). Some of those sensory traits may or may not be detectable by panelists and may not demerit the overall acceptability of the product.
As explained by Cochet et al. (2015), the use of descriptive sensory techniques for oysters can be difficult considering that oysters reared under the same conditions display a wide range of different sensory profiles (Aaraas et al., 2004), making it difficult to decipher sensory changes due to experimental treatment. The batch of oysters used for our experiment was of consistent age and size class, however there were slight natural morphological variations between individuals, which could have had an influence on their sensory profiles. During training, panelists were aware of organism-to-organism variability, and how that could play a role in the eating experience. The extrinsic and intrinsic cues that determine eating quality are complex. The adopted methodology, including panel selection, training, and consensus, is expected to focus the measurements on intrinsic sensory attributes. Consumers can be expected to have a wide range of sensory attribute perception thresholds—due to age, gender, genetic makeup, previously acquired responsiveness—and inclusion criteria for this small panel did not discriminate for these. Our study revealed significant differences in absolute scores given to several attributes between panelists, which suggests variability in the initial perception of certain attributes by panelists, however, the magnitude of changes between treatments was perceived similarly by the panelists. Such variability, which could be due to a number of factors such as experience, gender, or tasting technique, would occur naturally within the population of consumers. While the sensory panel does not necessarily represent the expectations for the whole consumer population, these findings are useful to predict acceptability; in relation to consumption predictions over long periods of time, it could be expected that context, socio-demographic factors and product presentation would influence oyster acceptability (Debucquet et al., 2012).
The sensory properties of oysters have been linked to various quality attributes of the meat. For instance, taste and flavor profile are linked to the proximate composition and the type of amino acids they contain, while aroma is associated with fatty acids and diet composition, and texture, mouthfeel, chewiness, and juiciness are linked to glycogen content, fat content, and meat pH (see references in Cochet et al., 2015). It is little understood how climate change and other changes to the marine environment will affect the quality of seafood through changes in their proximate composition and nutritional characteristics. To our knowledge, only three studies to date have investigated this matter (Ab Lah, 2017; Tate et al., 2017). A study on the turban snail Turbo militaris (Ab Lah, 2017) did not detect any changes in nutritional properties (protein, lipids, minerals, fatty acids) with combined warming and acidification, whereas two studies on the edible dogwhelk Dicathais orbita (Valles-Regino et al., 2015; Tate et al., 2017) revealed that the nutritional properties (protein and lipids) were negatively affected by similar conditions. The findings of these three studies are in agreement with our recent work on the changes in the nutritional properties of oysters, which show a decrease in protein, lipid, and carbohydrate content in C. gigas and Ostrea edulis under warming and acidification (Lemasson et al., manuscript in preparation). Although, these studies are not directly comparable to the one presented here due to differences in exposure duration (38 days: Ab Lah, 2017; 35 days: Valles-Regino et al., 2015; Tate et al., 2017; 12 weeks: Lemasson et al., manuscript in preparation), it is possible that these longer-term physiological changes—in the form of nutritional alterations—could lead to decreased sensory quality of these species. Negative changes to the nutritional and sensory quality of seafood species, such as D. orbita, C. gigas, and O. edulis, may in turn influence consumer preferences by altering their perception as healthy and appealing food choices.
Our study showed that only limited negative alterations to the sensory profile of oysters take place under transient acidification and warming scenarios. Therefore, if the outcomes of this short-exposure remain valid over longer-term OAW, oysters are still likely to represent a key species for future food security, bringing together a degree of resilience to climate change, whilst retaining nutritional value and taste.
Conclusion and Future Directions
A limitation of our study is the short duration of exposure to future environmental conditions; changes to the sensory profile of oysters may require considerably more time. Our experimental scenario reflects abrupt and transient warming and acidification events, which are expected to increase and intensify in the future, rather than a chronic perturbation (Ghedini et al., 2015). When investigating factors that may influence the eating quality of oysters, over a defined but limited time scale, it is confirmed that acceptability is not significantly affected by the tested scenarios. At the time of writing, the time required for any changes in sensory profile to occur is unknown and future studies should determine over what time scale, if any, is required for changes to occur. In the absence of other studies, no significant change in eating quality of oysters as observed in this study, and particularly the absence of negative effects on the sensory attributes, can be considered positive for the future of the aquaculture and food sectors. While production numbers may decrease because of acidification and warming (Barton et al., 2015), these initial results suggest that the quality and demand for this important aquaculture product may well remain.
Author Contributions
AL designed the study with assistance from AK and VK. AL conducted the experiment. AL, AK, and VK analyzed and interpreted the data. AL wrote the main body of text. All authors commented and revised the manuscript before submission.
Funding
This research was supported by an internal grant provided by the University of Plymouth to AK, JH, RM and SF.
Conflict of Interest Statement
The authors declare that the research was conducted in the absence of any commercial or financial relationships that could be construed as a potential conflict of interest.
Acknowledgments
We wish to thank the technical staff in the Food and Nutrition Unit at Plymouth University for their help and assistance. Thanks also go to the five experts who took part in the study and shared their passion for oysters and enthusiasm toward science. Particularly, we are grateful to Katy Davidson—the Oyster Lady—for her involvement and support in the project from its early stages, and to Shaun Krijnen from Menai Oysters and Mussels Ltd. for supplying the oysters used in this study. Finally, we would like to thank Dr. Sam Dupont for encouraging and helpful discussions during the early conception of this study. We would like to extend our gratitude toward the two reviewers whose comments and suggestions greatly improved this manuscript.
Footnotes
References
Aaraas, R., Hernar, I., Vorre, A., Bergslien, H., Lunestad, B., Skeie, S., et al. (2004). Sensory, histological, and bacteriological changes in flat oysters, Ostrea edulis L., during different storage conditions. J. Food Sci. 69, 205–210. doi: 10.1111/j.1365-2621.2004.tb11006.x
Ab Lah, R. (2017). Biochemical Composition of Turbinid Snails and Its Sensitivity to Climate Change. Ph.D. thesis, Southern Cross University, Lismore, NSW.
Alfnes, F., Guttormsen, A. G., Steine, G., and Kolstad, K. (2006). ‘Consumers’ willingness to pay for the color of salmon: a choice experiment with real economic incentives. Am. J. Agric. Econ. 88, 1050–1061. doi: 10.1111/j.1467-8276.2006.00915.x
Artioli, Y., Blackford, J. C., Nondal, G., Bellerby, R. G. J., Wakelin, S. L., Holt, J. T., et al. (2014). Heterogeneity of impacts of high CO2 on the North Western European shelf. Biogeosciences 11, 601–612. doi: 10.5194/bg-11-601-2014
Azpeitia, K., Ríos, Y., Garcia, I., Pagaldai, J., and Mendiola, D. (2017). A sensory and nutritional validation of open ocean mussels (Mytilus galloprovincialis Lmk.) cultured in SE Bay of Biscay (Basque Country) compared to their commercial counterparts from Galician Rías (Spain). Int. Aquat. Res. 9, 89–106. doi: 10.1007/s40071-017-0159-0
Barange, M., Merino, G., Blanchard, J. L., Scholtens, J., Harle, J., Allison, E. H., et al. (2014). Impacts of climate change on marine ecosystem production in societies dependent on fisheries. Nat. Clim. Change 4, 211–216. doi: 10.1038/nclimate2119
Barton, A., Hales, B., Waldbusser, G. G., Langdon, C., and Feely, R. A. (2012). The Pacific oyster, Crassostrea gigas, shows negative correlation to naturally elevated carbon dioxide levels: implications for near-term ocean acidification effects. Limnol. Oceanogr. 57, 698–710. doi: 10.4319/lo.2012.57.3.0698
Barton, A., Waldbusser, G., Feely, R., Weisberg, S., Newton, J., Hales, B., et al. (2015). Impacts of coastal acidification on the Pacific Northwest shellfish industry and adaptation strategies implemented in response. Oceanography 25, 146–159. doi: 10.5670/oceanog.2015.38
Borderías, A. J., and Sánchez-Alonso, I. (2011). First processing steps and the quality of wild and farmed fish. J. Food Sci. 76, 1–5. doi: 10.1111/j.1750-3841.2010.01900.x
Buzin, F., Baudon, V., Cardinal, M., Barillé, L., and Haure, J. (2011). Cold storage of Pacific oysters out of water: biometry, intervalval water and sensory assessment. Int. J. Food Sci. Technol. 46, 1775–1782. doi: 10.1111/j.1365-2621.2011.02686.x
Cao, R., Xue, C.-H., Liu, Q., and Xue, Y. (2009). Microbiological, chemical, and sensory assessment of Pacific Oysters (Crassostrea gigas) stored at different temperatures. Czech J. Food Sci. 27, 102–108.
Carlucci, D., Nocella, G., De Devitiis, B., Viscecchia, R., Bimbo, F., and Nardone, G. (2015). Consumer purchasing behaviour towards fish and seafood products. Patterns and insights from a sample of international studies. Appetite 84, 212–227. doi: 10.1016/j.appet.2014.10.008
Chang, K. L., Chang, J., Shiau, C.-Y., and Pan, B. S. (1998). Biochemical, microbiological, and sensory changes of sea bass (Lateolabrax japonicus) under partial freezing and refrigerated storage. J. Agric. Food Chem. 46, 682–686. doi: 10.1021/jf970622c
Cochet, M., Brown, M., Kube, P., Elliott, N., and Delahunty, C. (2015). Understanding the impact of growing conditions on oysters: a study of their sensory and biochemical characteristics. Aquacult. Res. 46, 637–646. doi: 10.1111/are.12210
Codex Standards (1999). “Guidelines for the sensory evaluation of fish and shellfish in laboratories,” in Codex Standards for Fish and Fishery Product. Codex Alimentarius CAC-GL 31-1999 (Rome: FAO/WHO).
Cooley, S. R., Lucey, N., Kite-Powell, H., and Doney, S. C. (2012). Nutrition and income from molluscs today imply vulnerability to ocean acidification tomorrow. Fish Fish. 13, 182–215. doi: 10.1111/j.1467-2979.2011.00424.x
Cooley, S. R., Rheuban, J. E., Hart, D. R., Luu, V., Glover, D. M., Hare, J. A., et al. (2015). An integrated assessment model for helping the United States sea scallop (Placopecten magellanicus) fishery plan ahead for ocean acidification and warming. PLoS ONE 10:e0124145. doi: 10.1371/journal.pone.0124145
Cruz-Romero, M., Smiddy, M., Hill, C., Kerry, J., and Kelly, A. (2004). Effects of high pressure treatment on physicochemical characteristics of fresh oysters (Crassostrea gigas). Innov. Food Sci. Emerg. Technol. 5, 161–169. doi: 10.1016/j.ifset.2004.01.002
Debucquet, G., Cornet, J., Adam, I., and Cardinal, M. (2012). Perception of oyster-based products by French consumers. The effect of processing and role of social representations. Appetite 59, 844–852. doi: 10.1016/j.appet.2012.08.020
Delgado, C. L. (2003). Fish to 2020: Supply and Demand in Changing Global Markets, Vol. 62. Penang: WorldFish.
Dickson, A. G. (1990). Thermodynamics of the dissociation of boric acid in synthetic seawater from 273.15 to 318.15 K. Deep Sea Res. Part A Oceanogr. Res. Pap. 37, 755–766.
Dickson, A. G., and Millero, F. J. (1987). A comparison of the equilibrium constants for the dissociation of carbonic acid in seawater media. Deep Sea Res. Part A Oceanogr. Res. Pap. 34, 1733–1743.
Dupont, S., Hall, E., Calosi, P., and Lundve, B. (2014). First evidence of altered sensory quality in a shellfish exposed to decreased pH relevant to ocean acidification. J. Shellfish Res. 33, 857–861. doi: 10.2983/035.033.0320
Ekstrom, J. A., Suatoni, L., Cooley, S. R., Pendleton, L. H., Waldbusser, G. G., Cinner, J. E., et al. (2015). Vulnerability and adaptation of US shellfisheries to ocean acidification. Nat. Clim. Change 5, 207–214. doi: 10.1038/nclimate2508
FAO (2016). The State of World Fisheries and Aquaculture 2016. Contributing to Food Security and Nutrition for All. Rome: Food and Agriculture Organization.
Fischer, E. M., and Knutti, R. (2015). Anthropogenic contribution to global occurrence of heavy-precipitation and high-temperature extremes. Nat. Clim. Change 5, 560–564. doi: 10.1038/nclimate2617
Fratini, G., Medina, I., Lupi, P., Messini, A., Pazos, M., and Parisi, G. (2013). Effect of a finishing period in sea on the shelf life of Pacific oysters (C. gigas) farmed in lagoon. Food Res. Int. 51, 217–227. doi: 10.1016/j.foodres.2012.12.012
Gazeau, F., Quiblier, C., Jansen, J. M., Gattuso, J.-P., Middelburg, J. J., and Heip, C. H. R. (2007). Impact of elevated CO2 on shellfish calcification. Geophys. Res. Lett. 34:L07603. doi: 10.1029/2006GL028554
Gerland, P., Raftery, A. E., Ševčíková, H., Li, N., Gu, D., Spoorenberg, T., et al. (2014). World population stabilization unlikely this century. Science 346, 234–237. doi: 10.1126/science.1257469
Ghedini, G., Russell, B. D., Falkenberg, L. J., and Connell, S. D. (2015). Beyond spatial and temporal averages: ecological responses to extreme events may be exacerbated by local disturbances. Clim. Change Res. 2, 6. doi: 10.1186/s40665-015-0014-8
Guo, X., Huang, M., Pu, F., You, W., and Ke, C. (2015). Effects of ocean acidification caused by rising CO2 on the early development of three mollusks. Aquat. Biol. 23, 147–157. doi: 10.3354/ab00615
Guyondet, T., Comeau, L. A., Bacher, C., Grant, J., Rosland, R., Sonier, R., et al. (2014). Climate change influences carrying capacity in a coastal embayment dedicated to shellfish aquaculture. Estuar. Coasts 38, 1593–1618. doi: 10.1007/s12237-014-9899-x
Hauri, C., Gruber, N., Vogt, M., Doney, S. C., Feely, R. A., Lachkar, Z., et al. (2013). Spatiotemporal variability and long-term trends of ocean acidification in the California current system. Biogeosciences 10, 193–216. doi: 10.5194/bg-10-193-2013
Helmuth, B., Russell, B., Connell, S., Dong, Y., Harley, C., and Lima, F. (2014). Beyond long-term averages: making biological sense of a rapidly changing world. Clim. Chan. Res. 1, 6. doi: 10.1186/s40665-014-0006-0
Hettinger, A., Sanford, E., Hill, T. M., Russell, A. D., Sato, K. N., Hoey, J., et al. (2012). Persistent carry-over effects of planktonic exposure to ocean acidification in the Olympia oyster. Ecology 93, 2758–2768. doi: 10.1890/12-0567.1
Humphreys, M. P. (2016). Climate sensitivity and the rate of ocean acidification: future impacts, and implications for experimental design. ICES J. Mar. Sci. 74, 934–940. doi: 10.1093/icesjms/fsw189
ISO 6658 (1985). “Sensory analysis—methodology—general guidance,” in International Organization for Standardization, Geneva (ISO 6658) (London: BSI).
Lemasson, A. J., Fletcher, S., Hall-Spencer, J. M., and Knights, A. M. (2017). Linking the biological impacts of ocean acidification on oysters to changes in ecosystem services: a review. J. Exp. Mar. Biol. Ecol. 492, 49–62. doi: 10.1016/j.jembe.2017.01.019
Liu, S., Fan, W., Zhong, S., Ma, C., Li, P., Zhou, K., et al. (2010). Quality evaluation of tray-packed tilapia fillets stored at 0°C based on sensory, microbiological, biochemical and physical attributes. Afr. J. Biotechnol. 9, 692–701. doi: 10.5897/AJB09.1369
Lloret, J., Rätz, H.-J., Lleonart, J., and Demestre, M. (2016). Challenging the links between seafood and human health in the context of global change. J. Mar. Biol. Assoc. U.K. 96, 29–42. doi: 10.1017/S0025315415001988
Macura, B., Lönnstedt, O. M., Byström, P., Airoldi, L., Eriksson, B. K., Rudstam, L., et al. (2016). What is the impact on fish recruitment of anthropogenic physical and structural habitat change in shallow nearshore areas in temperate systems? A systematic review protocol. Environ. Evid. 5, 1–8. doi: 10.1186/s13750-016-0061-z
McCauley, D. J., Pinsky, M. L., Palumbi, S. R., Estes, J. A., Joyce, F. H., and Warner, R. R. (2015). Marine defaunation: animal loss in the global ocean. Science 347:1255641. doi: 10.1126/science.1255641
Mehrbach, C., Culberson, C. H., Hawley, J. E., and Pytkowicx, R. M. (1973). Measurement of the apparent dissociation constants of carbonic acid in seawater at atmospheric pressure. Limnol. Oceanogr. 18, 897–907.
Molfese, C., Beare, D., and Hall-Spencer, J. M. (2014). Overfishing and the replacement of demersal finfish by shellfish: an example from the English channel. PLoS ONE 9:e101506. doi: 10.1371/journal.pone.0101506
Naylor, R. L., Goldburg, R. J., Primavera, J. H., Kautsky, N., Beveridge, M. C., Clay, J., et al. (2000). Effect of aquaculture on world fish supplies. Nature 405, 1017–1024. doi: 10.1038/35016500
Pierrot, D., Lewis, E., and Wallace, D. W. R. (2006). MS Excel Program Developed for CO2 System Calculations. Oak Ridge, TN: Carbon Dioxide Information Analysis Center, Oak Ridge National Laboratory, US Department of Energy.
Pörtner, H. (2008). Ecosystem effects of ocean acidification in times of ocean warming: a physiologist's view. Mar. Ecol. Prog. Ser. 373, 203–217. doi: 10.3354/meps07768
Reineccius, G. (1991). Off-flavors in foods. Crit. Rev. Food Sci. Nutr. 29, 381–402. doi: 10.1080/10408399109527534
Reum, J. C. P., Alin, S. R., Harvey, C. J., Bednaršek, N., Evans, W., Feely, R. A., et al. (2015). Interpretation and design of ocean acidification experiments in upwelling systems in the context of carbonate chemistry co-variation with temperature and oxygen. ICES J. Mar. Sci. 73, 582–595. doi: 10.1093/icesjms/fsu231
Scanes, E., Parker, L. M., O'Connor, W. A., Stapp, L. S., and Ross, P. M. (2017). Intertidal oysters reach their physiological limit in a future high-CO2 world. J. Exp. Biol. 220(Pt 5), 765–774. doi: 10.1242/jeb.151365
Šimat, V., Bogdanović, T., KrŽelj, M., Soldo, A., and Maršić-Lučić, J. (2012). Differences in chemical, physical and sensory properties during shelf life assessment of wild and farmed gilthead sea bream (Sparus aurata, L.). J. Appl. Ichthyol. 28, 95–101. doi: 10.1111/j.1439-0426.2011.01883.x
Stocker, T., Qin, D., Plattner, G., Tignor, M., Allen, S., Boschung, J., et al. (2013). IPCC, 2013: Climate Change: the Physical Science Basis. Contribution of Working Group I to the Fifth Assessment Report of the Intergovernmental Panel on Climate Change, IPCC.
Tacon, A. G. J., and Metian, M. (2013). Fish matters: importance of aquatic foods in Human nutrition and global food supply. Rev. Fish. Sci. 21, 22–38. doi: 10.1080/10641262.2012.753405
Tate, R. D., Benkendorff, K., Ab Lah, R., and Kelaher, B. P. (2017). Ocean acidification and warming impacts the nutritional properties of the predatory whelk, Dicathais orbita. J. Exp. Mar. Biol. Ecol. 493, 7–13. doi: 10.1016/j.jembe.2017.03.006
Valles-Regino, R., Tate, R., Kelaher, B., Savins, D., Dowell, A., and Benkendorff, K. (2015). Ocean warming and CO2-induced acidification impact the lipid content of a marine predatory gastropod. Mar. Drugs 13, 6019–6037. doi: 10.3390/md13106019
Keywords: aquaculture, seafood, climate change, multi-stressors, Crassostrea gigas, sensory evaluation
Citation: Lemasson AJ, Kuri V, Hall-Spencer JM, Fletcher S, Moate R and Knights AM (2017) Sensory Qualities of Oysters Unaltered by a Short Exposure to Combined Elevated pCO2 and Temperature. Front. Mar. Sci. 4:352. doi: 10.3389/fmars.2017.00352
Received: 01 August 2017; Accepted: 18 October 2017;
Published: 01 November 2017.
Edited by:
Lyne Morissette, M–Expertise Marine, CanadaReviewed by:
Pedro M. Costa, Centro de Ciências do Mar e do Ambiente (MARE), PortugalCarlos Rosas, Universidad Nacional Autónoma de México, Mexico
Copyright © 2017 Lemasson, Kuri, Hall-Spencer, Fletcher, Moate and Knights. This is an open-access article distributed under the terms of the Creative Commons Attribution License (CC BY). The use, distribution or reproduction in other forums is permitted, provided the original author(s) or licensor are credited and that the original publication in this journal is cited, in accordance with accepted academic practice. No use, distribution or reproduction is permitted which does not comply with these terms.
*Correspondence: Anaëlle J. Lemasson, anaelle.lemasson@plymouth.ac.uk