Short Term CO2 Enrichment Increases Carbon Sequestration of Air-Exposed Intertidal Communities of a Coastal Lagoon
- 1ALGAE-Marine Plant and Ecology Research Group, Centre for Marine Sciences, University of Algarve, Faro, Portugal
- 2Marine Biology and Ecology Research Centre, University of Plymouth, Plymouth, United Kingdom
In situ production responses of air-exposed intertidal communities under CO2 enrichment are reported here for the first time. We assessed the short-term effects of CO2 on the light responses of the net community production (NCP) and community respiration (CR) of intertidal Z. noltei and unvegetated sediment communities of Ria Formosa lagoon, when exposed to air. NCP and CR were measured in situ in summer and winter, under present and CO2 enriched conditions using benthic chambers. Within chamber CO2 evolution measurements were carried out by a series of short-term incubations (30 min) using an infra-red gas analyser. Liner regression models fitted to the NCP-irradiance responses were used to estimate the seasonal budgets of air-exposed, intertidal production as determined by the daily and seasonal variation of incident photosynthetic active radiation. High CO2 resulted in higher CO2 sequestration by both communities in both summer and winter seasons. Lower respiration rates of both communities under high CO2 further contributed to a potential negative climate feedback, except in winter when the CR of sediment community was higher. The light compensation points (LCP) (light intensity where production equals respiration) of Z. noltei and sediment communities also decreased under CO2 enriched conditions in both seasons. The seasonal community production of Z. noltei was 115.54 ± 7.58 g C m−2 season−1 in summer and 29.45 ± 4.04 g C m−2 season−1 in winter and of unvegetated sediment was 91.28 ± 6.32 g C m−2 season−1 in summer and 25.83 ± 4.01 g C m−2 season−1 in winter under CO2 enriched conditions. Future CO2 conditions may increase air-exposed seagrass production by about 1.5-fold and unvegetated sediments by about 1.2-fold.
Introduction
The current atmospheric carbon dioxide (CO2) level is predicted to be doubled by the end of the twenty-first century (IPCC, 2007). Rising atmospheric CO2 will increase the input of CO2 levels in the oceans, as 30% of the anthropogenic CO2 emissions are absorbed in the surface oceans (IPCC, 2014). This raises increased concern on the various impacts, elevated CO2 will have on the marine ecosystem (Gattuso et al., 1998; Guinotte and Fabry, 2008; Hall-Spencer et al., 2008; Portner, 2008; Porzio et al., 2011). The increase of CO2 in the world oceans is changing the carbonate chemistry of the seawater, lowering the pH and thus resulting in ocean acidification. This pH levels are expected to decrease further by 0.3–0.4 units by the end of this century (Royal Society, 2005; IPCC, 2014). The low pH of seawater will alter the carbonate chemistry of oceans by increasing the levels of dissolved inorganic carbon (DIC) species [CO2, bicarbonate () and carbonate ()], which will result in a regime shift of the total DIC in the seawater favoring more toward and CO2 (aq) than (Riebesell et al., 2007). This shift is expected to favor those species that can use as a source for carbon for photosynthesis along with CO2 (Beer et al., 2002; Mercado et al., 2003).
Seagrass ecosystems are distributed worldwide and contribute significantly to the carbon cycle of coastal areas (Duarte and Chiscano, 1999; Hemminga and Duarte, 2000). They are important marine carbon sinks and contribute significantly to the total carbon storage of oceans (12%) (Duarte, 1990; McLeod et al., 2011; Fourqurean et al., 2012). Saying that, the response of seagrass ecosystems to the rising CO2 levels is important to understand, as seagrasses are predicted to benefit from the increasing CO2 levels by increasing their carbon uptake (Beer and Koch, 1996; Zimmerman et al., 1997; Invers et al., 2001) and overcome their limitation under the current carbon dioxide concentrations.
Most of the studies on the effects of elevated CO2 levels on seagrasses have been on the responses of productivity and light requirements (Beer and Koch, 1996; Thom, 1996; Zimmerman et al., 1997; Palacios and Zimmerman, 2007), photosynthetic and leaf growth rates (Alexandre et al., 2012) and the uptake rate of ammonium and nitrate (Short and Neckles, 1999; Alexandre et al., 2012; Ow et al., 2016). Results so far have suggested that, seagrass meadows can utilize the increased CO2 concentration from the water column and enhance their photosynthetic activity and community metabolism (Frankignoulle and Disteche, 1984; Frankignoulle and Bouquegneau, 1990; Invers et al., 2001). However, these experiments were carried out mostly on submerged seagrass meadows and data on intertidal meadows is yet scarce. The intertidal seagrass meadows are subjected to daily shifts in light and temperature, due to a combination of tidal cycles and daily irradiance (Short et al., 2001; Vermaat, 2009; Van der Heide et al., 2010). In these intertidal flats, the intensity of light is generally higher during air exposure than in submerged conditions, when the nutrient sources are reduced to sediment pore water (Ouisse et al., 2011). Intertidal seagrasses are exposed to various concentrations of gaseous CO2 during exposure to air and to various dissolved carbon forms (CO2, , and ) during submersion (Ouisse et al., 2011; Bahlmann et al., 2015). The seagrass Zostera noltei, that inhabits the low intertidal zone, experiences alternate daily periods of submerged and exposed condition to air for several hours especially during spring tides (Silva and Santos, 2003; Coyer et al., 2004; Ouisse et al., 2011). During low tide when exposed to air, the photosynthetic activity of Z. noltei may be higher than when submerged (Silva et al., 2005) or decrease due to desiccation (Leuschner et al., 1998).
In most of the intertidal macrophytes, community-level metabolism estimates have been assessed using infrared gas analysis (IRGA) of CO2 fluxes through gas exchange methods, where flow- through systems or closed chambers have been used for longer periods of time (Streever et al., 1998; Silva et al., 2005). Much of the community-productivity studies utilizing CO2 flux measurements have focused more on salt marsh than seagrass communities. However, in salt marsh communities, carbon flux measurements have been carried out, by incubation of individual leaves in special leaf chambers with artificial light and controlled temperature (Streever et al., 1998). There is a scarcity in utilization of IRGA for carbon flux measurements in the intertidal seagrasses. Most studies using carbon flux measurements on Z. noltei were performed in lab conditions (Leuschner and Rees, 1993; Perez-Loren and Niell, 1994; Leuschner et al., 1998), whereas few measurements have been performed in situ (Leuschner et al., 1998; Silva et al., 2005; Ouisse et al., 2010, 2011). In this context, we aimed to measure the short-term effects of high CO2 concentration (750 ppm) on the net community production (NCP) and community respiration (CR) of air exposed Z. noltei community in Ria Formosa Lagoon, Portugal, where the species dominates the intertidal zone. The effects of vegetation on the intertidal ecosystem metabolism were also assessed by comparison with unvegetated areas.
Materials and Methods
Study Site
The study site, Ria Formosa lagoon (37°00′ N, 7°58′ W) is in the Southern coast of Portugal. It is a mesotidal system characterized by large intertidal flats (Andrade, 1990). The seagrass Zostera noltei dominates the intertidal flats of Ria Formosa lagoon, it's distribution ranges up to 2 m from the mean tide level and plays a significant role in the carbon metabolism of the lagoon (Santos et al., 2004). The Z. noltei meadows regularly experience alternate daily periods of submersion and exposure (Site A, Figure 1) withstanding extended periods of air exposure of up to 4.5–6 h per tidal cycle. The unvegetated sediment flats are at the same bathymetric zone experiencing similar daily tidal fluctuations. The studied unvegetated flats are located on the north-west direction of seagrass beds approximately 600 m in distance (Site B, Figure 1).
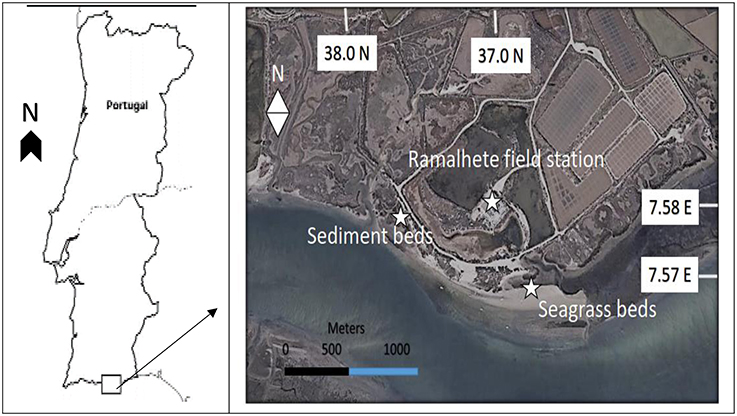
Figure 1. Study area showing Zostera noltei beds and unvegetated sediment flats at Ria Formosa Lagoon.
Gas Exchange Measurements
Measurements were made in situ, through continuous carbon dioxide flux monitoring (IRGA) in short-term incubations using benthic Plexiglas chambers. Incubations were conducted under the range of naturally available irradiance. The community response of Z. noltei was compared with that of adjacent un-vegetated sediments. This experiment was carried out in summer (June, July, August) 2014 and winter (November, December, January) 2014–2015 seasons. Gas-tight incubation chambers were made of UV-transparent Plexiglas, providing a closed environment for the incubations. These glass chambers comprised of two separate parts, a basal ring (30 cm in diameter) and a dome shaped lid. Sharpening of the basal ring was carried out, for easy 5–10 cm deep insertion into sediment. To secure both parts of the chamber power clamps were used. To confirm the chamber was sealed a special latex ring was installed in the flange. The chamber has three ports one in the ring (2), one on the top of the dome (1) and the third (3) on the base 5–10 cm above the sediment insertion line where CO2 gas is injected (Figure 2). All ports were fitted with Tygon® ST gas-impermeable tubing. The global operating volume of the chambers ranged between 22 and 26 L, depending on the insertion of ring into the sediment (Silva et al., 2005).
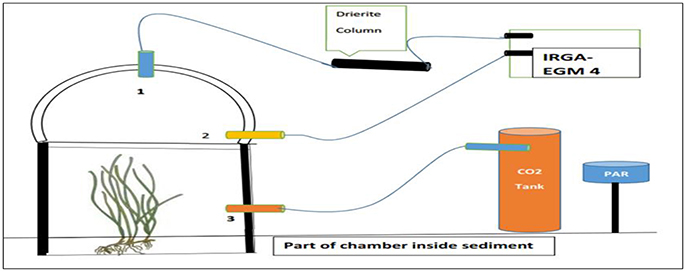
Figure 2. A diagrammatic representation of the incubation work. (1) Port one on top of dome. (2) Port two on ring. (3) Port three for CO2 injection.
Short-term field incubations using these chambers were conducted in Z. noltei meadows and bare sediment beds during several low tides at different times of the day along the season, so that the diel variation of light was captured. Each incubation lasted for a maximum of 30 min and under ambient atmospheric CO2 concentration (397–399 ppm) followed by CO2 enrichment to 750 ppm. During the incubation air entered the chamber through the ring port (2) and was out through the port (1), which allowed the air inside the chamber to be homogenized. An external non-dispersive infrared gas analyser (IRGA) (EGM-4, PP Systems, UK) was connected to the chamber, through a low volume (about 25 ml) closed air circuit. Measures of the evolution of CO2 concentrations within the chambers were done with accurate precision of 1 μatm. The IRGA has a pump installed in it, which gathered the air from the chamber, passed through a desiccation column (Anhydrous calcium sulfate, Drierite) to remove humidity from the air to avoid interferences in CO2 measurements when passed through the infrared cell. Then this air was send back to the chamber to keep continuous air flow between the chamber and gas analyser. The change in partial pressure of CO2 inside the chamber was continuously monitored (1 min steps) during the incubation period usually allowing a drop in the CO2 partial pressure for a 30–40 μatm. During this time the port (3) was clamped, so that no air passes through the tubes. After completion of the air incubation, the clamp was removed and CO2 gas was injected slowly into the chamber through the port (3), from a premixed air-CO2 tank until the CO2 concentration inside the chamber reached 750 ppm. The clamp was closed again. The CO2 partial pressure was measured, as described previously. Following each incubation, the seagrass was collected, washed and dried for 24 h at 60°C and the dry weight was recorded. Photosynthetic active radiation (PAR) inside the chambers was measured with a Li-Cor system (Li-Cor, 250 A meter, USA) at both the start and end of each incubation. Instantaneous PAR measurements were continuously done at the Ramalhete field station (Figure 1) every 30 min using the same sensor to record daily and seasonal light variation. There was approximately 10% of light attenuation inside the glass chambers. Temperature was recorded using a thermometer at the start and end of each air and CO2 incubation. Similar incubations were also performed for unvegetated sediment beds.
NCP and CR Measurements
Light and dark incubations were conducted for both NCP and CR respectively. The net flux (F) of CO2 (μmol C m−2 h−1) for both air and CO2 enriched incubations was computed as described in Silva et al. (2005):
where s (ppm CO2 min−1 = μmol CO2 mol air−1 min−1) represents the slope of the change in CO2 concentration during the incubation period,
V (L) = chamber volume,
A (m2) = benthic area,
mv (mol L−1) = molar volume or number of gas mole per volume unit, derived from the ideal gas law [mv = P/(R × T)],
where P (atm) = gas pressure,
T (K) = temperature, and R (0.082 atm LK−1 mol−1) = universal gas constant.
Thus, the net CO2 flux represents directly the NCP for the given light and temperature conditions. Similarly, the net flux of CO2 in dark represents the community respiration, CR.
A linear regression model was used to fit the NCP vs. PAR data,
Where Y is NCP, −b represent the rate of CO2 uptake by the community, X is the PAR value and “a” intercept, representing CR. The daily PAR variation reaching the Z. noltei community when exposed to the air, was used to estimate the daily NCP variation using the above model. Tidal variations (exposed period) for the study site (Z. noltei meadows and sediment beds) for each season (summer and winter) was estimated using a tidal model (Carrasco et al., submitted). The exposed period derived from the model for each day along the month and seasons for seagrass meadows and sediment beds was used to calculate the daily NCP, GCP and CR for each season.
Linear regression was used to model the effects of PAR on NCP. These models were then used to calculate NCP from PAR, which was measured continuously along the year. The daily NCP and CR during exposed period (g C m−2 day−1) was estimated by adding every 30 min NCP and CR values along the day when the seagrass beds were exposed to the air. GCP was obtained by adding the NCP and CR during light hours. Seasonal budgets of NCP, CR and GCP were obtained by considering the variability of exposure times related to the alternation of spring and neap tidal cycles and the daily cycle of irradiance.
Statistics
Results are expressed as mean ± Standard error (SE). Significant differences of NCP responses to light were tested using the difference between slopes (Cohen et al., 2003; Soper, 2017). Significant differences between daily and seasonal CR was detected using t-tests. Data was tested for normality and constant variance before testing for significant difference. Significant levels were considered at p < 0.05.
Results
The mean plant biomass of Z. noltei was 293.25 ± 8.08 g DW m−2 in summer and 189.66 ± 3.45 g DW m−2 in winter. The slopes of the linear responses of the NCP of Zostera noltei and unvegetated sediment were higher under CO2 enriched conditions than ambient conditions in both summer and winter seasons (Figure 3). The differences were only apparent at light intensities above the light compensation point for community production (about 200 μmol m−2 s−1), i.e., when production is higher than respiration. The NCP of unvegetated sediments was lower than Z. noltei within PAR ranges of 800–1,600 μmol m−2 s−1, which was most evident in the summer (Figures 4A,B). In the summer season under higher light (1,680 μmol m−2 s−1) the NCP of sediments was higher than Z. noltei under ambient conditions, whereas under CO2 enriched conditions the NCP of Z. noltei was higher than unvegetated sediment community (Figure 4).
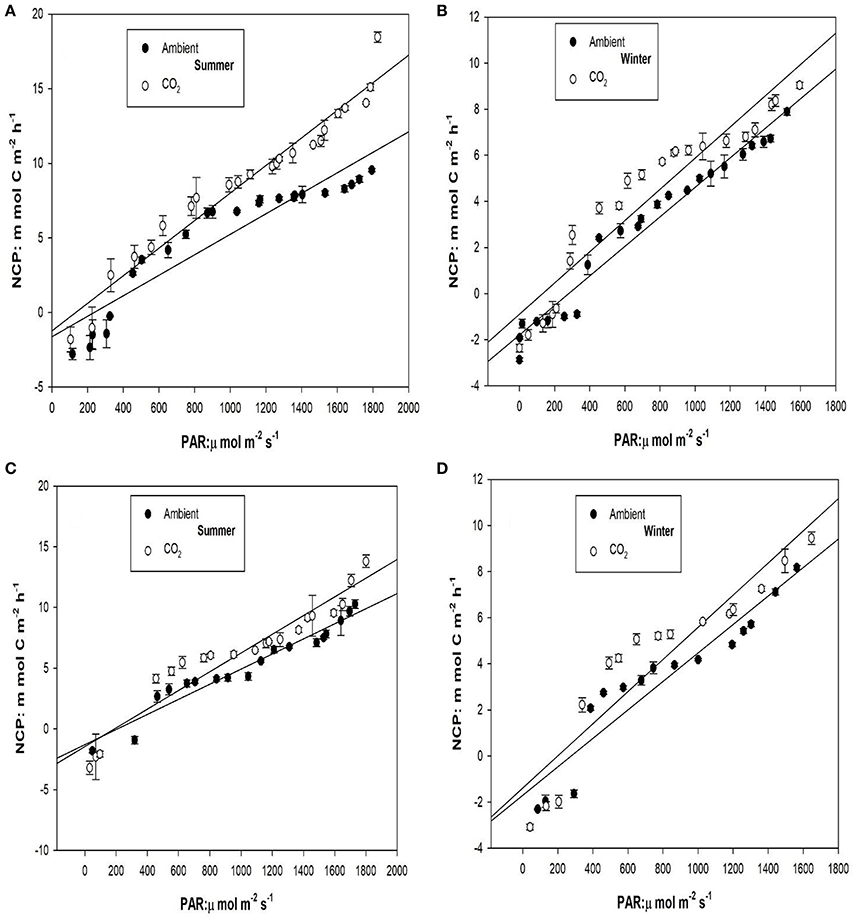
Figure 3. Response of net community production (NCP) to irradiance (PAR) under ambient and CO2 enriched conditions in summer and winter for Z. noltei, (A,B) and sediments (C,D). Error bars represent standard errors.
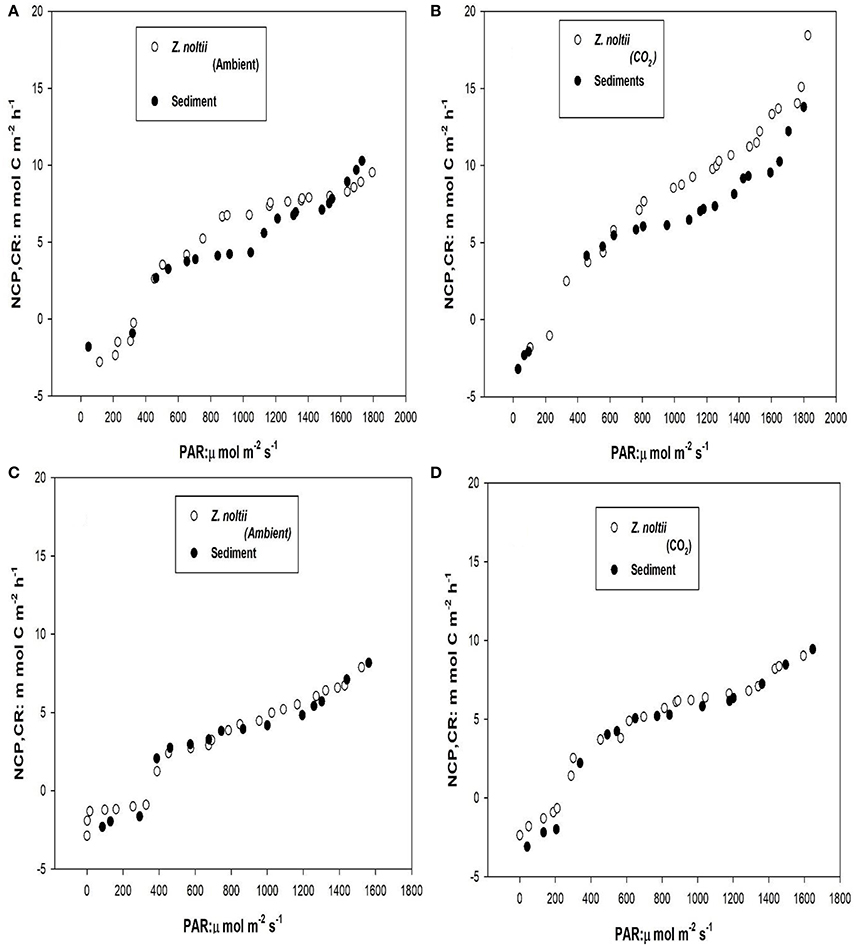
Figure 4. Comparison of net community production (NCP) and respiration (NCP at 0 light level) of Z. noltei and unvegetated sediment in summer (A,B) and winter seasons (C,D) under ambient and CO2 enriched conditions.
The NCP of Z. noltei and unvegetated sediments responded linearly to the range of PAR observed when the community was exposed to the air, both in summer and winter seasons (Table 2). The slope, intercept and R2-values of the linear regression models are presented in Table 2. The high coefficients of determination (R2) both under ambient and CO2 enriched conditions (Table 1) indicate a good fit of the models to data. The light compensation points (LCP) were always lower under CO2 enriched conditions for both Z. noltei and sediment communities (Table 2). The LCP of Z. noltei community was higher than the unvegetated sediment community under ambient conditions as opposed to CO2 enriched conditions (Table 2).
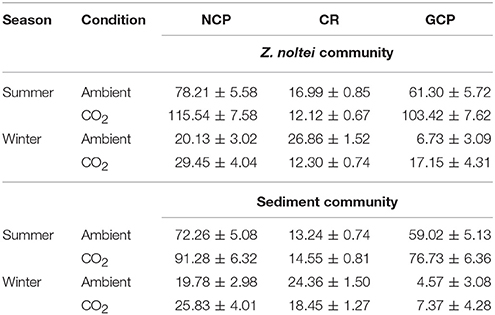
Table 1. Seasonal budget (Mean ± SE, n = 90 days) of NCP (g C m−2 season−1), CR (g C m−2 season−1) and GCP (g C m−2 season−1) of Z. noltei and unvegetated sediment community under ambient and CO2 enriched conditions in summer and winter seasons.
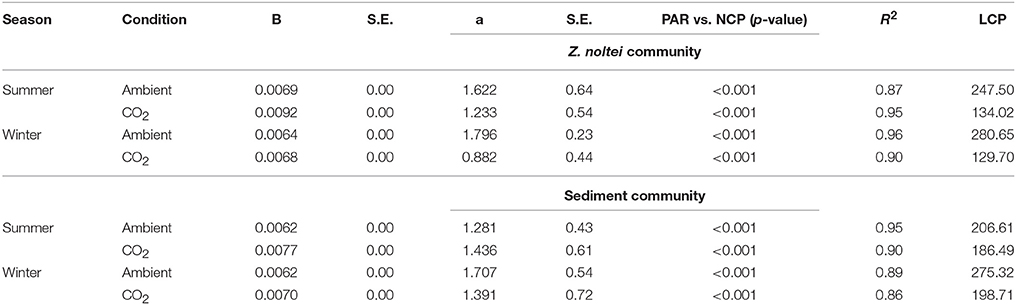
Table 2. Slope (b), intercept (a), p, R2, standard error (S.E.) and light compensation point (LCP, μmol m−2 s−1) values derived from linear regression model between PAR and NCP under ambient and CO2 enriched conditions for Z. noltei community and unvegetated sediments community.
The daily NCP of Z. noltei was 1.5-fold and 1.3-fold higher in both seasons under CO2 enriched conditions and CR was lower (Figures 5A,B). Consequently, the daily gross community production (GCP) was even higher under CO2 enriched conditions than ambient conditions (Figure 5A). Similarly, under CO2 enriched conditions the daily sediment NCP was higher in both seasons, whereas the daily CR was higher in summer and lower in winter (Figures 5C,D). The daily GCP of sediment community was higher in summer under CO2 enriched conditions and there was no significant difference in winter.
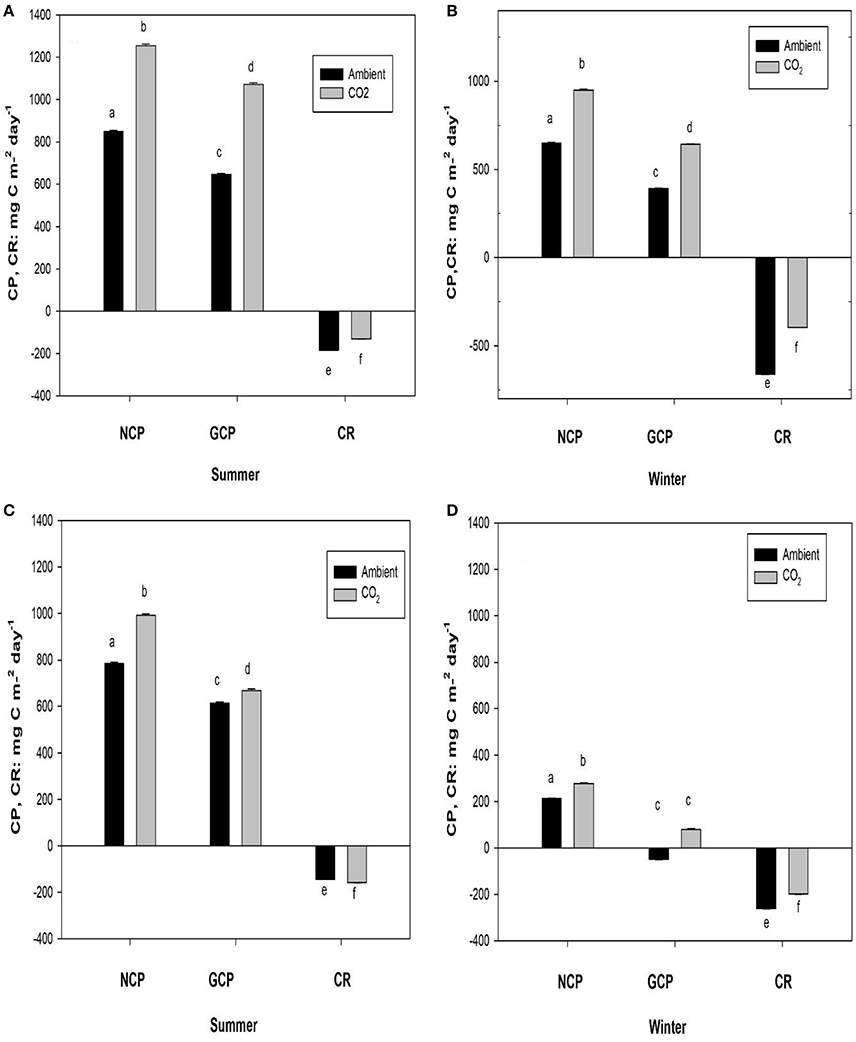
Figure 5. Mean (± SE) daily net community production and respiration (NCP at 0 light level) of Z. noltei (A,B) and unvegetated sediment (C,D) in summer and winter seasons under ambient and CO2 enriched conditions. Different letters indicate significant difference between ambient (air) and CO2 enriched conditions. Error bars represent standard errors derived from regression models for NCP and GCP and error bars for CR are derived from field measurements.
The seasonal budgets of carbon sequestered by Z. noltei was 1.3-fold and 2.3-fold higher than unvegetated sediment under CO2 enriched conditions in both seasons (Table 1). Under CO2 enriched conditions, the carbon sequestered by Z. noltei was 4.9-fold higher in summer than winter season. The unvegetated sediment community sequestered 10-fold higher carbon in summer than winter season under CO2 enriched conditions (Table 1). Seasonal budget of CR was higher in sediment communities than Z. noltei under CO2 enriched conditions in winter season (Table 1).
Discussion
Ambient Conditions
NCP and CR of unvegetated sediment community were lower than Z. noltei community in both summer and winter under ambient conditions (Figure 5). This suggests Z. noltei communities are more diverse and include more autotrophic organisms (e.g., epiphytes and benthic microalgae) (Charpy-Roubaud and Sournia, 1990) contributing to the community production than sediment communities. Similar results of higher community production in Z. noltei than unvegetated sediment were observed at the Bay of Morlaix, France (Ouisse et al., 2010), and Banco de Mauritania (Clavier et al., 2014).
In the summer, the NCP of Z. noltei of Ria Formosa lagoon was 1.5-fold higher than previously reported for Z. noltei at Roscoff, France (Ouisse et al., 2011). As well the CR was 2-fold higher than Z. noltei community response observed by Ouisse et al. (2011). This resulted in higher GCP of Z. noltei of Ria Formosa lagoon than the GCP obtained for Z. noltei community at Roscoff (Ouisse et al., 2011).
The GCP and CR of Z. noltei observed in winter at Ria Formosa were also higher than previously reported for Z. noltei community by Clavier et al. (2011, 2014) and Ouisse et al. (2011). Higher community production (NCP, GCP) is due to higher irradiances (1,522–1,594 μmol m−2 s−1) at Ria Formosa than the irradiance (829 μmol m−2 s−1) level observed by Ouisse et al. (2011) at Roscoff. The higher respiratory demand of the extended rhizome network of Z. noltei community at Ria Formosa is probably due to higher temperature.
The summer NCP of unvegetated sediment in Ria Formosa lagoon (785 mg Cm−2 day−1) was higher than previously reported (681.5 mg Cm−2 day−1) for the Tagus estuary, Portugal in summer (Uthicke and Klumpp, 1998), whereas NCP (212.52 mg Cm−2 day−1) of sediment in winter was lower (255 mg Cm−2 day−1). Average NCP and CR observed in our results for sediment community in winter were higher than NCP and CR rates observed in the intertidal sediment community at Banco di Marituania (Clavier et al., 2014).
CO2 Enrichment
We observed positive responses of Z. noltei and sediment communities to CO2 enrichment. The short-term gas exchange measurements with CO2 enrichment conditions clearly indicated that Z. noltei community production at around the same irradiance level increased 1.5-fold. Higher community production under CO2 enrichment suggest that community production of Z. noltei are carbon limited under the current atmospheric CO2 concentration, confirming the same conclusions previously obtained for Z. noltei (Alexandre et al., 2012) and for Z. marina (Beer and Koch, 1996; Zimmerman et al., 1997; Invers et al., 2001). These reports are on the photosynthetic response of individual plants rather than whole community, but it is expected that the increase in photosynthetic rate of individual plants is reflected on the higher organizational level of the community production. On the other hand, Martínez-Crego et al. (2014) did not find a significant effect of CO2 on the community metabolism of Z. noltei, but this experiment was done in submerged conditions. The hypothesis that the future effects of CO2 on intertidal seagrass will be higher when they are exposed to the air should probably be tested.
The key factor during exposed conditions for higher production under CO2 enrichment can be higher light availability during exposure periods and abundant CO2 as substrate from the CO2 enrichment. Increase in CO2 as substrate resulting in higher production efficiency was observed in Z. marina where elevated CO2 levels increased plant production (Zimmerman et al., 1997). Secondly sediment microtopography of Z. noltei community plays an important role in maintaining hydration for the whole exposed period, due to numerous small depressions in the sediment retaining water (Silva et al., 2005). Thirdly during exposure, a thin layer of water is trapped between the leaves of Z. noltei due to higher leaf density (Silva and Santos, 2003), helping the plants remain moist throughout the exposed period.
The desiccations in Z. noltei meadows is thus not severe and the Z. noltei root system also supplies water to the leaves, which helps in maintaining a thin layer of water at the leaf surface (Leuschner et al., 1998). In these favorable conditions when CO2 enrichment happens, a rapid diffusion of CO2 into the thin film of water occurs around the seagrass leaves due to air-water CO2 gradient, making the CO2 readily available to the plants as they are consuming it (Leuschner et al., 1998). Therefore, high CO2 concentrations along with high irradiance levels during air exposure of the Z. noltei community, creates the ideal condition for high community production during exposure (Leuschner and Rees, 1993; Silva et al., 2005; Touchette and Burkholder, 2007).
NCP and CR of Z. noltei are lower during exposed than when submerged under ambient conditions (Ouisse et al., 2010; Clavier et al., 2011), but under CO2 enrichment conditions the community production is higher and CR lower as observed in our results. Lower CR during exposed condition may increase the overall community production of exposed Z. noltei compared to submerged conditions where CR rates are higher. As well, during submersion Z. noltei favors the utilization of bicarbonate directly or indirectly to increase its production (Hellblom et al., 2001), but this process is energy intensive (Burnell et al., 2014), whereas the air exposed community during exposure, because of its high affinity and high availability of CO2 in future conditions, can easily use the CO2 from the atmosphere for community production (Touchette and Burkholder, 2007). The other factors that can contribute to the higher production of air- exposed Z. noltei community under CO2 enriched conditions can be high light intensity and low turbidity.
Sediment community NCP were also higher with CO2 enrichment incubations in summer and winter than ambient incubations, which can be related to the presence of micro-phytobenthos in the sediments during intertidal exposure (Uthicke and Klumpp, 1998; Hubas et al., 2006; Clavier et al., 2014). The intertidal desiccation of the sediments was avoided due to the presence of a thin film of water trapped by the benthos community and water trapped by intertidal burrows (Michael and McIntir, 1983).
PAR Responses
NCP of both Z. noltei and unvegetated sediment communities responded linearly to PAR, when the communities are exposed to air both under ambient and CO2 enriched conditions. This suggests no photoinhibition of the whole community production at highest levels of PAR, which was also observed elsewhere for Z. noltei communities under ambient conditions (Ouisse et al., 2010, 2011; Clavier et al., 2011). Under CO2 enriched conditions both communities increase their production even further without signs of saturation. Even though the photosynthetic production of individual autotrophic species does saturate at high light levels the combination of the responses of individual species that occupy the whole microhabitats of the community results is non-saturating response. In the case of non-vegetated sediments, the vertical migration of microphytobenthos that optimize the whole community production may justify it.
Under CO2 enrichment, the light use capacity of both Z. noltei and unvegetated sediment communities increased as highlighted by the observed lower LCP in relation to ambient conditions. This was also observed in Z. marina (Zimmerman et al., 1997 and Z. noltei (Alexandre et al., 2012) where increase in CO2 concentrations increased the light using capacity of individual plants.
The lower LCP obtained from the NCP/PAR models suggest that intertidal communities of coastal ecosystems may become more efficient at low light levels in a future high CO2 scenario. This may result from a reduction of CR under high CO2 as observed here for both Z. noltei and unvegetated sediment communities and by an increase of the carboxylation rate mediated by RUBISCO as the CO2/O2 ratio will increase. The LCP observed in our results were higher than previously reported for Z. noltei for both seasons under ambient conditions (Gacia et al., 2005; Ouisse et al., 2010) and lower for CO2 enriched conditions. The LCPs of sediment community of both seasons under ambient conditions, were also higher than previously observed for sediment communities (Uthicke and Klumpp, 1998; Clavier and Garrigue, 1999). The LCP for CO2 enriched experiments during exposed conditions are presented for the first time for Z. noltei and sediment community.
The lower LCP under CO2 enriched conditions and higher NCP of Z. noltei in summer suggests that the community is more efficient in using low light (Silva and Santos, 2003) and can start using CO2 for production in low light conditions without community production compensating for CR. In the case of unvegetated sediment community, the LCP was higher than Z. noltei under CO2 enriched conditions, but the NCP was not, which suggests that even with higher CO2 concentrations the microphytobenthos production is not higher than CR until a certain level of irradiance level is reached.
In situ production responses of air-exposed intertidal communities under CO2 enrichment were reported here for the first time. The CO2 enrichment method based on in situ short-term incubations of intertidal communities using benthic chambers is a valuable tool for intertidal lagoon metabolic estimates to assess the future trends of carbon cycling in tidal systems. It provides fast and valuable estimation of community carbon metabolism responses during exposed periods. The use of short-term incubations is an important feature in these experiments as the temperature and humidity levels remain constant. More importantly, short-term incubations prevent saturation of photosynthesis due to an increase of O2 in relation to CO2, which inhibits the RUBISCO carboxylase activity favoring oxygenase allowing repeatable carbon uptake measurements with minimum disturbance to communities (Olivé et al., 2016).
The good correspondence between carbon metabolism responses to light of the Z. noltei community and unvegetated sediment communities across summer and winter provides evidence that community production rates derived from CO2 enriched incubations are a reflection of photosynthetic activity at ecologically relevant scales for future conditions of carbon dioxide concentrations. Positive production responses, negative respiration responses and lower light requirements under elevated CO2 levels as observed in our results strongly suggest that the carbon sequestration of intertidal communities, when air-exposed, will increase in the future.
Author Contributions
RS, JS, and AM have conceived the idea and the design of the experiments. AM has carried out the field work, data collection and writing of the manuscript. AM and RS carried out the interpretation of results. Both RS and JS have helped in revising and finalizing the final version of the manuscript. RS, JS, and AM approve the final version to be published. RS, JS, and AM agree to be accountable for all aspects of the work to maintain the scientific integrity and the research ideas are appropriately investigated and resolved.
Funding
This work was part of MARES “Future Oceans” project (MARES_12_14) and was funded through a MARES Grant. MARES is a Joint Doctorate programme selected under Erasmus Mundus coordinated by Ghent University (FPA 2011-0016). Check http://mares-eu.org/ for extra information. This study was also supported by Fundação para a Ciência e Tecnologia (FCT), through the strategic projects UID/Multi/04326/2013 granted to CCMAR.
Conflict of Interest Statement
The authors declare that the research was conducted in the absence of any commercial or financial relationships that could be construed as a potential conflict of interest.
Acknowledgments
We are grateful for the support of Ricardo Guerreiro with field sampling at Ria Formosa Lagoon, Portugal and laboratory works at CCMAR. We are thankful to Dr. Rita Carrasco and Dr. Carmen Santos for helping us with their tidal model data.
References
Alexandre, A., Silva, J., Buapet, P., Bjork, M., and Santos, R. (2012). Effects of CO2 enrichment on photosynthesis, growth and nitrogen metabolism of the seagrass Zostera noltii. Ecol. Evol. 2, 2620–2630. doi: 10.1002/ece3.333
Andrade, C. F. (1990). O Ambiente Barreira Da Ria Formosa, Algarve, Portugal. Ph.D., thesis, University of Lisbon, Lisbon.
Bahlmann, E., Weinberg, I., Lavric, V. J., Eckhardt, T., Michaelis, W., Santos, R., et al. (2015). Tidal controls on trace gas dynamics in a seagrass meadow of the Ria Formosa lagoon (Southern Portugal). Biogeosciences 12, 1683–1696. doi: 10.5194/bg-12-1683-2015
Beer, S., and Koch, E. (1996). Photosynthesis of marine macroalgae and seagrasses in globally changing CO2 environments. Mar. Ecol. Prog. Ser. 141, 199–204. doi: 10.3354/meps141199
Beer, S., Bjork, M., Hellblom, F., and Axelsson, L. (2002). Inorganic carbon utilization in marine angiosperms (seagrasses). Funct. Plant Biol. 29, 349–354. doi: 10.1071/PP01185
Burnell, O. W., Connell, S. D., Irving, A. D., Watling, J. R., and Russell, B. D. (2014). Contemporary reliance on bicarbonate acquisition predicts increased growth of seagrass Amphibolis antarctica in a high-CO2 world. Conserv. Physiol. 2, 1–11. doi: 10.1093/conphys/cou052
Charpy-Roubaud, C., and Sournia, A. (1990). The comparative estimation of phytoplankton microphytobenthic production in the oceans. Mar. Microb. Food Webs. 4, 31–57.
Clavier, J., and Garrigue, C. (1999). Annual sediment primary production and respiration in a large coral reef lagoon. Mar. Ecol. Prog. Seri. 191, 79–89. doi: 10.3354/meps191079
Clavier, J., Chauvaud, L., Amice, E., Lazure, P., Geest, M., Osse, P. L., et al. (2014). Benthic metabolism in shallow coastal ecosystems of the Banc d'Arguin, Mauritania. Mar. Ecol. Prog. Ser. 501, 11–23. doi: 10.3354/meps10683
Clavier, J., Chauvaud, L., Carlier, A., Amice, E., Geest, M., Labrosse, P., et al. (2011). Aerial and underwater carbon metabolism of a Zostera noltii seagrass bed in the Banc d' Arguin, Mauritania. Aquat. Bot. 1, 24–30. doi: 10.1016/j.aquabot.2011.03.005
Cohen, J., Cohen, P., West, S. G., and Aiken, L. S. (2003). Applied Multiple Regression/Correlation Analysis for the Behavioral Sciences, 3rd Edn. Mahwah, NJ: Lawrence Earlbaum Associates.
Coyer, J. A., Diekmann, O. E., Serrao, E. A., Procaccini, G., Milchakova, N., Pearson, G. A., et al. (2004). Population genetics of dwarf eelgrass Zostera noltii throughout its biogeographic range. Mar. Ecol. Prog. Ser. 281, 51–62. doi: 10.3354/meps281051
Duarte, C. M. (1990). Seagrass nutrient content. Mar. Ecol. Prog. Ser. 67, 201–207. doi: 10.3354/meps067201
Duarte, C. M., and Chiscano, C. L. (1999). Seagrass biomass and production: a reassessment. Aquat. Bot. 65, 159–164. doi: 10.1016/S0304-3770(99)00038-8
Fourqurean, J. W., Duarte, C. M., Kennedy, H., Marba, N., Holmer, M., Mateo, M. A., et al. (2012). Seagrass ecosystem as a globally significant carbon stock. Nat. Geosci. 5, 505–509. doi: 10.1038/ngeo1477
Frankignoulle, M., and Bouquegneau, J. M. (1990). Daily and yearly variations of total inorganic carbon in a productive coastal area, Estuar. Coast. Shelf Sci. 30, 79–89. doi: 10.1016/0272-7714(90)90078-6
Frankignoulle, M., and Disteche, A. (1984). CO2 chemistry in the water column above a Posidonia seagrass bed and related air-sea exchanges. Oceanol. Acta 7, 209–219.
Gacia, E., Kennedy, H., Duarte, C. M., Terrados, J., Marba, N., Papadimitriou, S., et al. (2005). Light dependence of the metabolic balance of a highly productive Philippine seagrass community. J. Expt. Mar. Biol. Ecol. 316, 55–67. doi: 10.1016/j.jembe.2004.10.008
Gattuso, J. P., Frankignoulle, M., Bourge, I., Romaine, S., and Buddemeier, R. W. (1998). Effect of calcium carbonate saturation of seawater on coral calcification. Glob. Planet. Change 18, 37–46. doi: 10.1016/S0921-8181(98)00035-6
Guinotte, J. M., and Fabry, V. J. (2008). Ocean acidification and its potential effects on marine ecosystems. Ann. N.Y. Acad. Sci. 1134, 320–342. doi: 10.1196/annals.1439.013
Hall-Spencer, J. M., Rodolfo-Metalpa, R., Martin, S., Ransome, E., Fine, M., Turner, S. M., et al. (2008). Volcanic carbon dioxide vents show ecosystem effects of ocean acidification. Nature 454, 96–99. doi: 10.1038/nature07051
Hellblom, F., Beer, S., Bjork, M., and Axelsson, L. (2001). A buffer sensitive inorganic carbon utilization system in Zostera marina. Aquat. Bot. 69, 55–62. doi: 10.1016/S0304-3770(00)00132-7
Hubas, C., Davoult, T., and Cariou, T. (2006). Factors controlling benthic metabolism during low tide along a granulometric gradient in an intertidal bay (Roscoff Aber Bay, France). Mar. Ecol. Prog. Ser. 316, 53–68. doi: 10.3354/meps316053
Invers, O., Zimmerman, R. C., Alberte, R. S., Perez, M., and Romero, R. (2001). Inorganic carbon sources for seagrass photosynthesis: an experimental evaluation of bicarbonate use in species inhabiting temperate waters. J. Exp. Mar. Biol. Ecol. 265, 203–217. doi: 10.1016/S0022-0981(01)00332-X
IPCC (Intergovernmental Panel on Climate Change) (2007). Climate Change 2007 Synthesis Report. New York, NY: Cambridge University Press.
IPCC (2014). “Climate change 2014: synthesis report,” in Contribution of Working Groups I, II and III to the Fifth Assessment Report of the Intergovernmental Panel on Climate Change, eds Core Writing Team, R. K. Pachauri, and L. A. Meyer (Geneva: IPCC), 151.
Leuschner, C., and Rees, U. (1993). CO2 gas exchange of two intertidal seagrass species, Zostera marina L. and Zostera noltii Hornem, during exposed condition. Aquat. Bot. 45, 53–62. doi: 10.1016/0304-3770(93)90052-X
Leuschner, C., Landwehr, S., and Mehlig, U. (1998). Limitation of carbon assimilation of intertidal Zostera noltii and Zostera marine by desiccation at low tide. Aquat. Bot. 62, 171–176. doi: 10.1016/S0304-3770(98)00091-6
Martínez-Crego, B., Olivé, I., and Santos, R. (2014). CO2 and nutrient-driven changes across multiple levels of organization in Zostera noltii ecosystems. Biogeosciences 11, 7237–7249. doi: 10.5194/bg-11-7237-2014
McLeod, E., Chmura, G., Bouillon, S., Salm, R., and Bjork, M. (2011). A blueprint for carbon: toward an improved understanding of the role of vegetated costal habitats in the sequestering CO2. Front. Ecol. Environ. 10, 552–560. doi: 10.1890/110004
Mercado, J. M., Niell, F. X., Silva, J., and Santos, R. (2003). Use of light and inorganic carbon acquisition by two morphotypes of Zostera noltii Hornem. J. Exp. Mar. Biol. Ecol. 297, 71–84. doi: 10.1016/S0022-0981(03)00368-X
Michael, W. D., and McIntir, D. (1983). Effects of physical gradients on the production dynamics of sediment- associated algae. Mar. Ecol. Prog. Ser. 13, 103–114. doi: 10.3354/meps013103
Olivé, I., Silva, J., Coasta, M. M., and Santos, R. (2016). Estimation of seagrass community metabolism using benthic chambers: effects of incubation duration. Est. Coasts. 39, 138–144. doi: 10.1007/s12237-015-9973-z
Ouisse, V., Migné, A., and Davoult, D. (2010). Seasonal variations of community production, respiration and biomass of different primary producers in an intertidal Zostera noltii bed (Western English Channel, France). Hydrobiologia. 649, 3–11. doi: 10.1007/s10750-010-0254-3
Ouisse, V., Migné, A., and Davoult, D. (2011). Community level carbon flux variability over a tidal cycle in Zostera marina and Z. noltii beds. Mar. Ecol. Prog. Ser. 437, 79–87. doi: 10.3354/meps09274
Ow, Y. X., Vogel, N., Collier, C. J., Holtum, J. A. M., Flores, F., and Uthicke, S. (2016). Nitrate fertilisation does not enhance CO2 responses in two tropical seagrass species. Sci. Rep. 6:23093. doi: 10.1038/srep23093
Palacios, S., and Zimmerman, R. (2007). Response of eelgrass Zostera marina to CO2 enrichment: possible impacts of climate change and potential for remediation of coastal habitats. Mar. Ecol. Prog. Ser. 344, 1–3. doi: 10.3354/meps07084
Perez-Loren, J. L., and Niell, F. X. (1994). Photosynthesis in air: comparative responses to different temperatures of two morphotypes of Zostera noltii Hornem. from Palmones River estuary (southern Spain). Verh. Internat. Verein. Limnol. 25, 2265–2269.
Portner, H. O. (2008). Ecosystem effects of ocean acidification in times of ocean warming: a physiologist's view. Mar. Ecol. Prog. Ser. 373, 203–217. doi: 10.3354/meps07768
Porzio, L., Buia, M. C., and Hall-Spencer, J. M. (2011). Effects of ocean acidification on macroalgal communities. J. Exp. Mar. Biol. Ecol. 400, 278–287. doi: 10.1016/j.jembe.2011.02.011
Riebesell, U., Schulz, K. G., Bellerby, R. G. J., Botros, M., Fritsche, P., Meyerhöfer, M., et al. (2007). Enhanced biological carbon consumption in a high CO2 ocean. Nature 450, 545–548. doi: 10.1038/nature06267
Royal Society (2005). Ocean Acidification Due to Increasing Atmospheric Carbon Dioxide. London, The Royal Society U.K.
Santos, R., Silva, J., Alexandre, A., Navarro, R., Barron, C., and Duarte, C. M. (2004). Ecosystem metabolism and carbon fluxes of a tidally-dominated coastal lagoon. Estuaries 27, 977–985. doi: 10.1007/BF02803424
Short, F. T., and Neckles, H. A. (1999). The effects of global climate change on seagrasses. Aquat. Bot. 63, 169–196. doi: 10.1016/S0304-3770(98)00117-X
Short, F. T., Coles, R. G., and Pergent-Martini, C. (2001). “Global seagrass distribution,” in Global Seagrass Research Methods, eds F. T. Short and R. G. Coles (Amsterdam: Elsevier), 5–31.
Silva, J., and Santos, R. (2003). Daily variation patterns in seagrass photosynthesis along a vertical gradient. Mar. Ecol. Prog. Ser. 257, 37–44. doi: 10.3354/meps257037
Silva, J., Santos, R., Calleja, M. L., and Duarte, C. (2005). Submerged versus air exposed intertidal macrophyte productivity: from physiological to community level assessments. J. Exp. Mar. Biol. Ecol. 317, 87–95. doi: 10.1016/j.jembe.2004.11.010
Soper, D. S. (2017). Significance of the Difference between Two Slopes. Available online at: http://www.danielsoper.com/statcalc
Streever, W. J., Genders, A. J., and Cole, M. A. (1998). A closed chamber CO2 flux method for estimating marsh productivity. Aquat. Bot. 62, 33–44. doi: 10.1016/S0304-3770(98)00080-1
Thom, R. M. (1996). CO2 enrichment effect on eelgrass (Zostera marina L.) and bull kelp (Nereocystis luetkeana (MERT.) P. & R.). Water Air Soil Pollut. 88, 383–391. doi: 10.1007/BF00294113
Touchette, B. W., and Burkholder, J. M. (2007). Carbon and nitrogen metabolism in the seagrass, Zostera marina L.: environmental control of enzymes involved in carbon allocation and nitrogen assimilation. J. Exp. Mar. Biol. Ecol. 350, 216–233. doi: 10.1016/j.jembe.2007.05.034
Uthicke, S., and Klumpp, D. W. (1998). Microphytobenthos community production at a near shore coral reef: seasonal variation and response to ammonium recycled by holothurians. Mar. Ecol. Prog. Ser. 169, 1–11. doi: 10.3354/meps169001
Van der Heide, T., Bouma, T. J., van Nes, E. H., van de Koppel, J., Scheffer, M., Roelofs, J. G., et al. (2010). Spatial self-organized patterning in seagrasses along a depth gradient of an intertidal ecosystem. Ecology 91, 362–369. doi: 10.1890/08-1567.1
Vermaat, J. E. (2009). Linking clonal growth patterns and ecophysiology allows the prediction of meadow-scale dynamics of seagrass beds. Perspect. Plant Ecol. Evol. Syst. 11, 137–155. doi: 10.1016/j.ppees.2009.01.002
Keywords: intertidal communities, Zostera noltei, CO2 effects, community metabolism, incubation chambers
Citation: Mishra AK, Silva J and Santos R (2018) Short Term CO2 Enrichment Increases Carbon Sequestration of Air-Exposed Intertidal Communities of a Coastal Lagoon. Front. Mar. Sci. 4:439. doi: 10.3389/fmars.2017.00439
Received: 01 September 2017; Accepted: 22 December 2017;
Published: 30 January 2018.
Edited by:
Nuria Marba, Consejo Superior de Investigaciones Científicas (CSIC), SpainReviewed by:
Mikael K. Sejr, Aarhus University, DenmarkJoanna Carey, Marine Biological Laboratory, United States
Copyright © 2018 Mishra, Silva and Santos. This is an open-access article distributed under the terms of the Creative Commons Attribution License (CC BY). The use, distribution or reproduction in other forums is permitted, provided the original author(s) and the copyright owner are credited and that the original publication in this journal is cited, in accordance with accepted academic practice. No use, distribution or reproduction is permitted which does not comply with these terms.
*Correspondence: Rui Santos, rosantos@ualg.pt