Connectivity Between Coastal and Oceanic Zooplankton From Rio Grande do Norte in the Tropical Western Atlantic
- 1Departamento de Oceanografia, Universidade Federal de Pernambuco, Recife, Brazil
- 2Departamento de Biologia, Universidade Federal Rural de Pernambuco, Recife, Brazil
- 3Departamento de Zootecnia, Universidade Federal Rural de Pernambuco, Recife, Brazil
- 4Instituto Socioambiental e dos Recursos Hídricos (ISARH), Universidade Federal Rural da Amazônia, Belém, Brazil
- 5Departamento de Educação, Campus VIII, Universidade do Estado da Bahia, Paulo Afonso, Brazil
- 6Departamento de Ciências Humanas e Tecnologia, Campus XXIV, Universidade do Estado da Bahia, Xique-Xique, Brazil
The zooplankton off the coast of Rio Grande do Norte, Brazil was studied during four oceanographic campaigns from July 2002 to May 2004. A Bongo net (mesh sizes of 64, 120, and 300 μm) was used for sample collections. A total of 462 zooplankton samples was studied. These samples were classified in four areas (Coastal, Inner Shelf, Shelf Break, Slope) according to local depth and distance from coast. Most of the studied environment is oligotrophic, under the Tropical Water mass influence. We recorded 199 zooplankton taxa, and Copepoda was the most numerous with the highest number of species. There were 93 species of copepods, of which calanoids comprised 62%. The most frequent zooplankton taxa were Undinula vulgaris, Nannocalanus minor, Oithona nana, Onychocorycaeus giesbrechti, Crustacea nauplius, Bivalvia, and Gastropoda veligers. The species diversity varied from 0.87±0.28 bits⋅ind-1 (Coastal) to 3.96±0.12 bits⋅ind-1 (Slope). The minimum density was 11.5 ind⋅m-3 (macrozooplankton, Inner Shelf), and the maximum density was 161.6 × 106 ind⋅m-3 (microzooplankton, Coastal). The biomass decreased sharply between the microzooplankton to macrozooplankton fractions. The community presented a general trend in species composition that included coastal eutrophic indicators and oceanic oligotrophic indicators, with low numerical abundance of zooplanktonic organisms. A higher density occurs in the coastal area that is near the littoral area due to the continental flux and benthic regeneration. The seasonal effect was also highlighted as an important factor responsible for the dissimilarity of zooplankton communities. Cluster analysis of the samples based on Copepoda presented differences among the four campaigns corresponding to the rainy and dry seasons. The copepod’s functional traits divided the community into three groups, and the reproduction and the trophic strategies were the main factors in structuring the community. We found a pelagic gradient for the zooplankton community varying from a low diverse eutrophic coastal area to a high diverse oligotrophic oceanic area, located over a varied, high biomass benthic habitat, which is mostly covered by calcareous algae functioning on the shallow shelf as a large reef system.
Introduction
Studies on community ecology have emphasized the local processes influencing species composition and abundance. However, the effects of regional processes upon local dynamics have been shown by some researchers (Ricklefs and Schluter, 1993; Hubbell, 1997; Forbes and Chase, 2002), including those processes that connect communities. Physic-chemical processes can play an important role in the connectivity among habitats and in defining the community attributes at different size scales (Ricklefs, 1987; Cowen et al., 2006). For example, dispersal by currents in marine areas connects coastal communities to oceanic ones and vice-versa, thus forming species patches along interconnected environs (metacommunities) (Watson et al., 2011; Niebuhr et al., 2015).
Coastal ecosystems are of great human interest (both ecological and socioeconomic); in these ecosystems, biotic and abiotic factors vary continuously along scales that oscillate from short to long term (Walsh, 1988). In Northeastern Brazil, an arid clime, extensive beaches and high dunes characterize the littoral area in the northern region of the Rio Grande do Norte State. The continental shelf is approximately 30 km wide, and calcareous algae primarily cover the bottom; this represents the largest area covered by limestone sediments on the planet (Santos, 2010). This shelf functions as a huge “coral reef” that supports a high benthic diversity and biological production. The oligotrophic South Equatorial Current (SEC) dominates the pelagic inner shelf waters (Stramma et al., 1990); thus, like that of a coral reef area, the plankton productivity is very low, but it eventually increases due localized enrichment events (Medeiros et al., 1999). In this “coral reef”-like area, regenerated production dominates (fueled by nutrients accumulated in the benthic realm biologically recycled). The continental slope starts at a depth of 80 m and extends until 1300–3600 m (Mabesoone and Coutinho, 1970; Gomes and Vital, 2010); further, the sea floor is far beneath the photic zone, so the influence of regenerating production can be ignored when budgeting for flow in the offshore oceanic pelagic ecosystem.
This variable scenario highly influences the lively populations flourishing in the littoral area, which can cause a misunderstanding of the annual biodiversity and abundance patterns. A cooperative research program was established between the Federal University of Pernambuco (Brazil) and the Brazilian Oil Company (PETROBRAS) to develop comprehensive biotic and abiotic characterizations of the north coast of Rio Grande do Norte State and to study the plankton community; this work took place from July 2002 to May 2004. In coastal ecosystems where local processes are linked to anthropogenic impacts the biological community are threatened and can present large-scale changes (Guichard et al., 2004).
Plankton studies in this area are scarce. In relation to the zooplankton, previous investigations of tropical Southwestern Atlantic (SWA) were restricted to certain groups (Copepoda: Björnberg, 1963; Chaetognatha: Gusmão, 1986; Cnidaria: Gusmão et al., 2015) or to a few samples evaluated on a macro-scale level that were obtained by international expeditions. A zooplankton atlas (Boltovskoy, 1981, 1999) is an important reference of the SWA; however, data from the Rio Grande do Norte littoral area and the connectivity among habitats remains fragmented (e.g., Neumann-Leitão et al., 1999, 2008), and the main functional groups are still incipient (Campos et al., 2017; Neumann-Leitão et al., 2018).
Elucidation of the biotic community is important, particularly the zooplankton, and involves sampling methods capable of obtaining a representative population; as marine zooplankton comprise different size classes of organisms, they serve as a pivotal group in the transference of mass and energy from primary producers to higher trophic levels in the food web (Clark et al., 2001). The size of the zooplankton animals and their main functional traits are powerful forcing factors outlining marine systems. Also, few researches have been devoted to the comparison of the spatial-temporal variableness of micro-, meso- and macrozooplankton densities in marine systems (Smeti et al., 2015).
Thus, the goal of the present study was to produce accurate and complete information on micro-, meso-, and macrozooplankton diversity, biomass, and density distribution in different seasons along a coastal-oceanic gradient.
Materials and Methods
Study Area
The continental shelf at the North of Rio Grande do Norte State (Figure 1) is divided in three sedimentary environments: the first (coastal and inner shelf) is characterized by siliciclastic sediments and huge longitudinal sandbanks with a depth around 15 m. The second (mid shelf) has a mixture of siliciclastic and carbonate sediments, and transversal sandbanks approximately on 20 m. The third (outer shelf) dominated by carbonates sediments, with a narrow shelf and depths varying from 25 to 50 m. A beachrock line at the isobath of 25 m delimits the boundary from mid- and outer shelf. The shelf extends seawards into a steep slope that spreads until 2000 m at the basin plain (Gomes and Vital, 2010). This basin has a high economic status because of the daily production of 80 thousand barrels of oil and 3 million⋅m3 of gas (2003 data, production on ocean and earth), and it is the second most productive oil basin offshore in Brazil (Soares et al., 2003).
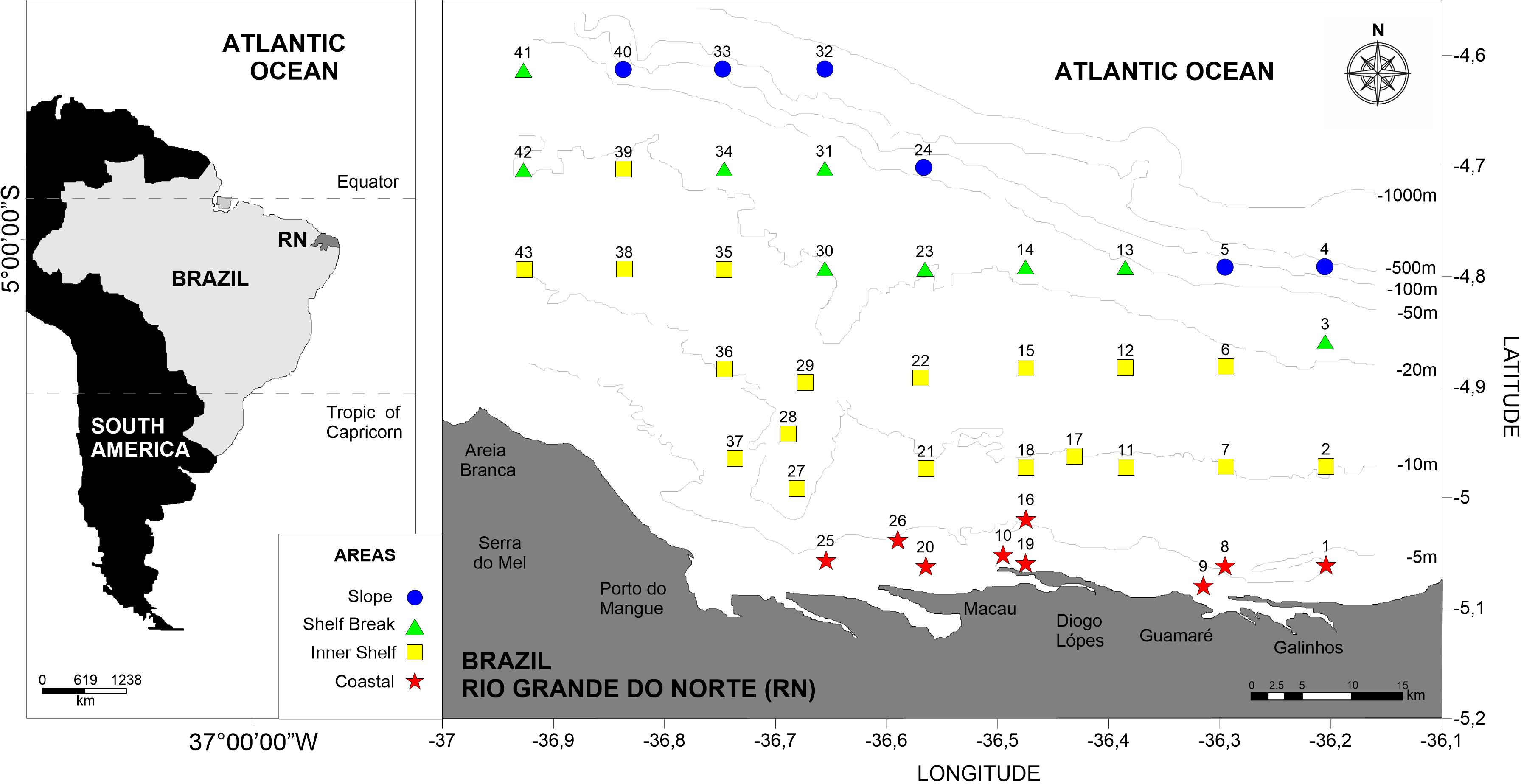
Figure 1. Study area and stations located at the coastal and oceanic areas off Rio Grande do Norte, Southwestern Atlantic.
The average monthly total rainfall of North of Rio Grande do Norte (data from 1962 to 20051) presents an annual average of 735.6 mm, with a higher rainfall from February to May, and a lower precipitation from September to November. The monthly average from 2001 to 2004 shows a warmer rainy season (January to May), and a dry season a bit cooler (July to November) (Figure 2)1.
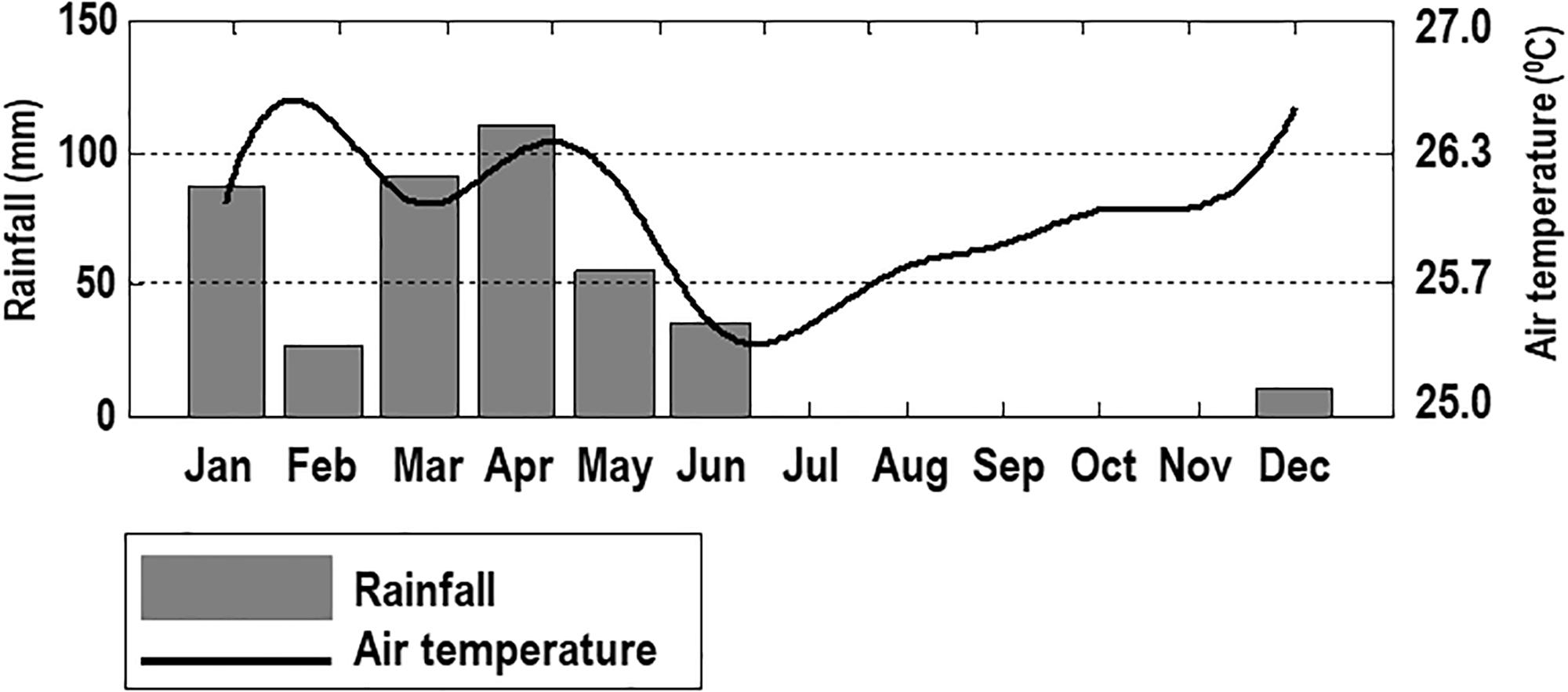
Figure 2. Rainfall and air temperature from 2001 to 2004 at the coastal area of Rio Grande do Norte, Southwestern Atlantic (Source: http://www.inmet.gov.br).
In the studied area the Tropical Water (TW: ≥ 20°C, ≥36.0) was present with relatively warm and salty waters, occurring from surface down to 70 m depth, and the South Atlantic Central Water (SACW: ≤18°C, ≤36.0) occurred below the TW and extended down to nearly 450 m. At depths between 70 and 150 m, there was also the Maximum Salinity Water (MSW), with temperatures varying from 18.0 to 26.0°C and salinity ≥37.0 (Schott et al., 1998).
The sea surface temperature average was 27.17 ± 0.7°C and ranged from 27.11°C (dry season, Slope) to 28.41°C (rainy season, Coastal). Salinity average was 36.2 ± 0.27, with a minimum of 35.90 (rainy season, Slope) and a maximum of 36.77 (dry season, Inner Shelf). Chlorophyll-a average was 0.52 ± 0.33 mg⋅m-3, fluctuating from 0.23 mg⋅m-3 (dry season, Slope) to 1.13 mg⋅m-3 (dry season, Coastal) (Figure 3, PETROBRAS, 2005).
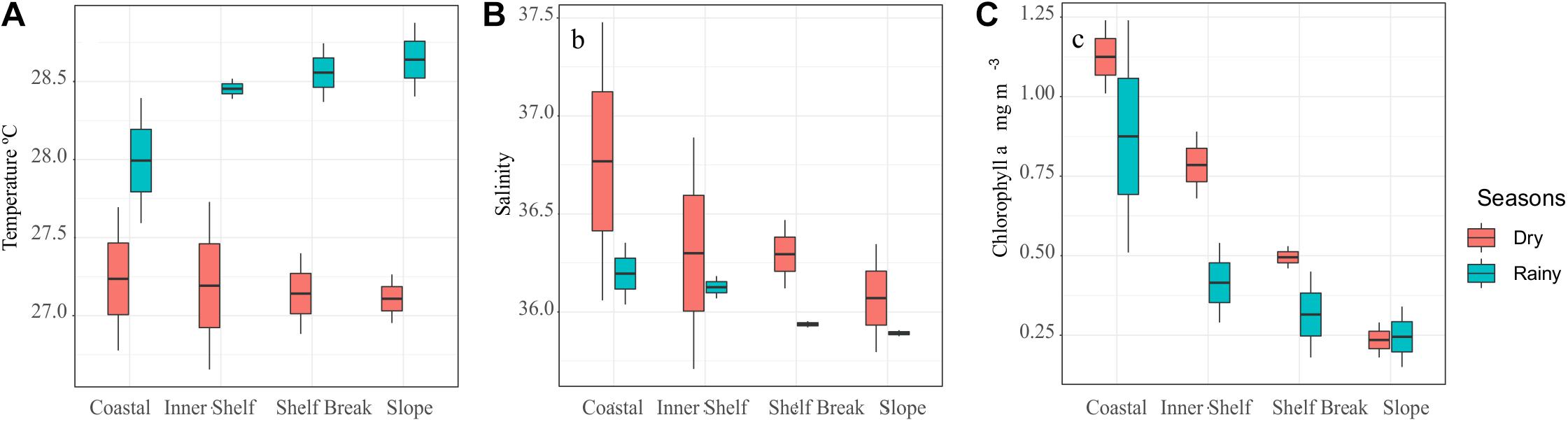
Figure 3. Box-plot of the temperature (A), salinity (B), and chlorophyll-a (C) at the coastal and oceanic areas off Rio Grande do Norte, Southwestern Atlantic (Source: Petrobras, Bacia Potiguar-RN 2005).
Sampling Strategy
The survey was performed during four campaigns from July 21 to 30, 2002 (Campaign 1, dry season), May 12 to 30, 2003 (Campaign 2, rainy season), November 14 to 23, 2003 (Campaign 3, dry season), and May 17 to 31, 2004 (Campaign 4, rainy season). The zooplankton was sampled during daytime at 43 stations, along nine transects from the coast to the offshore north of Rio Grande do Norte (latitude 4° to 5° S, longitude 36° to 37° W) (Figure 1). A total of 462 samples was collected; these samples were classified into four areas (Coastal: < 10 m, Inner Shelf: 10–20 m, Shelf Break: 20–50 m, and Slope: >50 m) according to local depth and distance from coast.
Field Sampling and Laboratory Analyses
The zooplankton sampling was carried out by bongo net hauls. The analyzed nets had the following mesh sizes and diameters: 64 μm/30 cm; 120 μm/30 cm; and 300 μm/60 cm. The terminology micro-, meso-, and macrozooplankton will be used throughout the text to refer to the net’s mesh sizes, which were 64, 120, and 300 μm, respectively (although in the literature these sizes corresponded to micro- and mesozooplankton, see Omori and Ikeda, 1984). Oblique hauls were made at a speed of 2 to 2.5 knots from a depth of 14 m (nearshore) and 150 m (Shelf Break and Slope) to the surface. A flowmeter (Hydrobios, Kiel, Germany) was used to measure the water filtered volume. A 4% buffered formalin-seawater solution was used to preserve the samples. Seston biomass (mg⋅m-3) was assessed by using the Omori and Ikeda (1984) wet-weight method. To identify the species, we used the Trégouboff and Rose (1957) and Boltovskoy (1981, 1999) manuals, among others. Taxa enumeration and identification considered the lowest taxonomic unit of each phylum. Taxon density was calculated from subsamples of 1 mL (for microzooplankton using a Sedgwick-Rafter chamber) and 8 mL (meso- and macrozooplankton using a Bogorov plate) taken of the whole sample (standardized to 500 mL). For each sample, three subsamples (with at least of 300 individuals) were counted and the mean calculated for abundance valuation (ind⋅m-3).
Data Analysis
The Shannon diversity index H’ was applied to Copepoda for the diversity evaluation (Shannon, 1948), as this was the most diverse taxon and the most identified species. Evenness was calculated according to Pielou (1977).
To investigate for trends in spatial distribution, total density (log10 transformed), biomass and ecological indexes (diversity and evenness) of the micro-, meso- and macrozooplankton were compared among the coastal, inner shelf, shelf break and slope areas during both dry and rainy season. As even after data transformation, the assumptions of the ANOVA model could not be satisfied for total density and evenness, we applied Kruskal–Wallis tests to compare zooplankton total density and evenness among the areas in both seasons. When significant p values were found, the Dunn’s Multiple Comparison test was applied. Diversity was compared by means of two-way ANOVA models, after checking the validity of the assumptions of normality in distribution of the errors and homoscedasticity by means of the Shapiro-Wilk and Bartlett tests, respectively.
A Permutational multivariate analysis of variance (PERMANOVA) was used to test the micro-, meso- and macrozooplankton community structure changes in response to the factors area, season, and to investigate possible interactions among these two factors. The calculation of pseudo-F and p values was based on 999 random permutations. Pairwise test between different levels of significant factor(s) was performed in case of significant differences identified by the PERMANOVA. The non-metric multi-dimensional scaling (MDS) was used to represent the data in a bi-dimensional space. The PERMANOVA and the nMDS were performed with basis on a Bray–Curtis dissimilarity matrix constructed on the log (X + 1) transformed abundance of species with relative abundance >2%. The SIMPER (SIMilarity of PERcentages) routine was used to identify the taxa that contributed most to the Bray–Curtis dissimilarity of areas within the identified seasons. The SIMPER test was set at ≥50% cumulative contribution.
A Redundancy Analysis (RDA) was applied to identify whether there were different size communities that could be explained by the temporal and spatial data variability. We used the total micro-, meso-, and macrozooplankton abundance and biomass data. Zooplankton groups were log (X + 1) transformed and compared with an abiotic factor matrix (Temperature, Salinity, Chlorophyll a). The spatio-temporal factors (Coastal, Inner Shelf, Shelf Break, Slope, Dry season and Rainy season) were transformed into dummy variables, represented by 1 and 0. Non-significant variables (p > 0.05) were excluded from the matrix of environmental data in a step by step procedure, using Monte Carlo permutation tests with 9999 permutations to remove collinearities between variables (Ter Braak and Smilauer, 2002). The analyses were performed using the CANOCO software (version 4.5; Ter Braak and Smilauer, 2002). All analyses were conducted considering p < 0.05 (Zar, 1996).
Hierarchical cluster analyses in Q mode using Ward’s method (Legendre and Legendre, 1998) were performed with basis on a Bray–Curtis dissimilarity matrix calculated using Copepoda density data. The goodness of fit of the data was assessed by means of the cophenetic correlation coefficient (Rohlf and Fisher, 1968).
The functional trait matrix of copepods was built using the available literature (mainly Boltovskoy, 1999 and Benedetti et al., 2016). We included seven traits with 23 trait categories: (1) Average adult female body length (0.5–1.5, 1.5–2.5, 2.5–3.5, 3.5–4.5, >4.5 mm); (2) Trophic group (herbivore, carnivore, omnivore, detritivore); (3) Feeding type (active ambush, passive ambush, filter, cruise, mixed); (4) Mode of reproduction (broadcast-spawner, sac-spawner); (5) Vertical distribution preference (epipelagic, mesopelagic, bathypelagic); (6) Diel vertical migration behavior (Yes, No); and (7) Habitat type (estuarine, neritic, oceanic). These traits are functionally important, as they relate to the life history and ecology of species and are expected to be constant through time and space (Pomerleau et al., 2015). The Sorensen index was applied to a presence/absence species-by-trait matrix, and the cluster dendrogram was formed through the average linkage method. The Calinski and Harabasz (1974) criteria were used to establish the number of functional groups. This analysis was applied only to Copepoda at the species level (see Supplementary Table S1).
Results
Species Composition
We identified 199 taxa in the three zooplankton size fractions composed of Foraminifera, Ciliophora, Radiozoa, Cnidaria, Aschelminthes, Mollusca, Annelida, Arthropoda, Echinodermata, Chaetognatha, Bryozoa, Cephalochordata, and Chordata (Tables 1, 2). A total of 88 taxa occurred in the microzooplankton fraction, 115 taxa in the mesozooplankton fraction, and 102 taxa in the macrozooplankton fraction. In the three fractions, 69 taxa cooccurred and consisted mostly of juveniles in the microzooplankton fraction and adults in the meso- and macrozooplankton fractions. Holoplankton comprised 87, 67, and 61% in the micro- meso-, and macrozooplankton fractions, respectively. Most zooplankton species (91) were oceanic indicators and were obtained mainly from the North Brazil Current. Several neritic taxa were larval stages of benthic organisms that normally occur in the continental shelf.
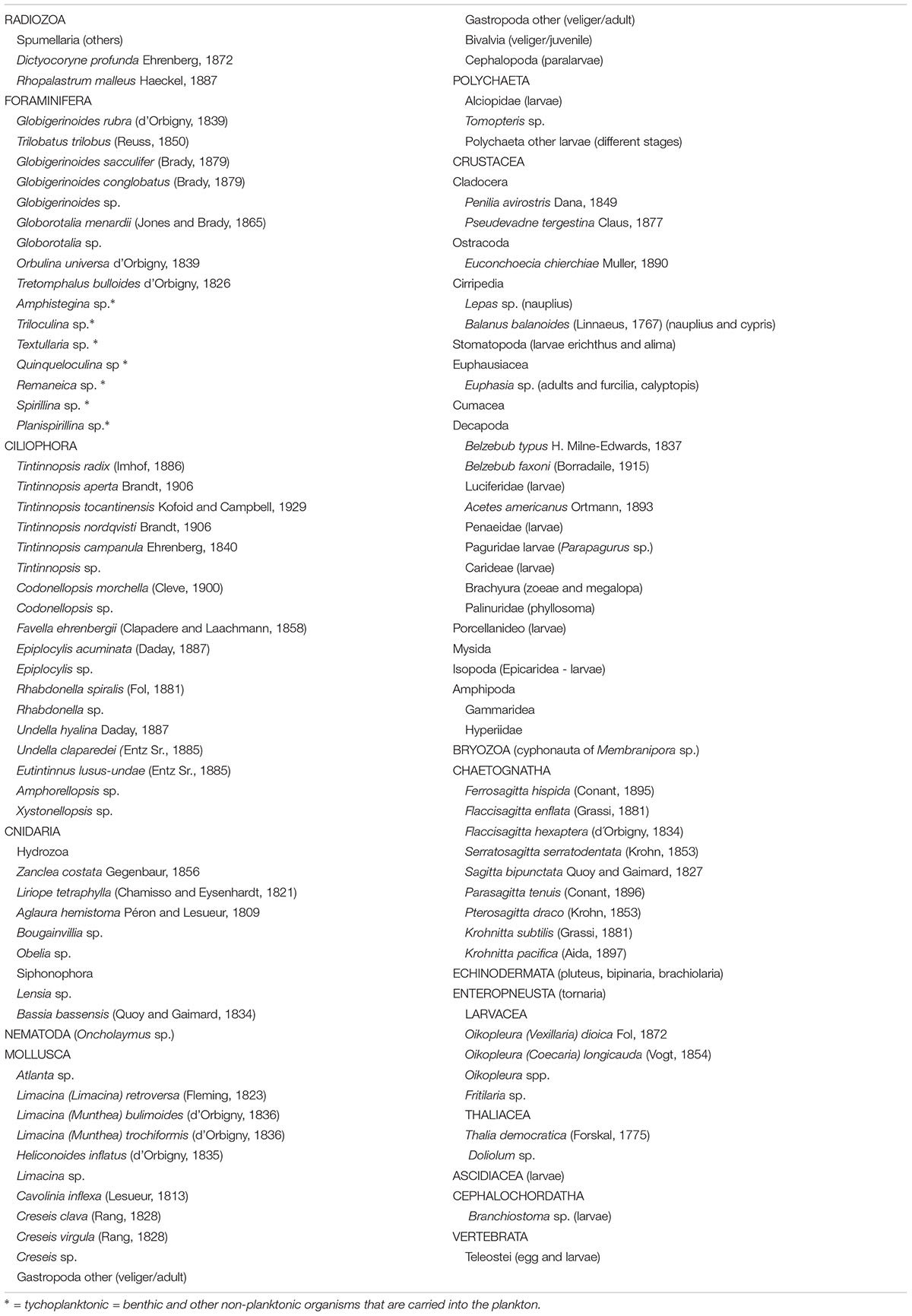
Table 1. List of zooplankton taxa (without Copepoda) from coastal and oceanic areas of Rio Grande do Norte, Northeastern Brazil.
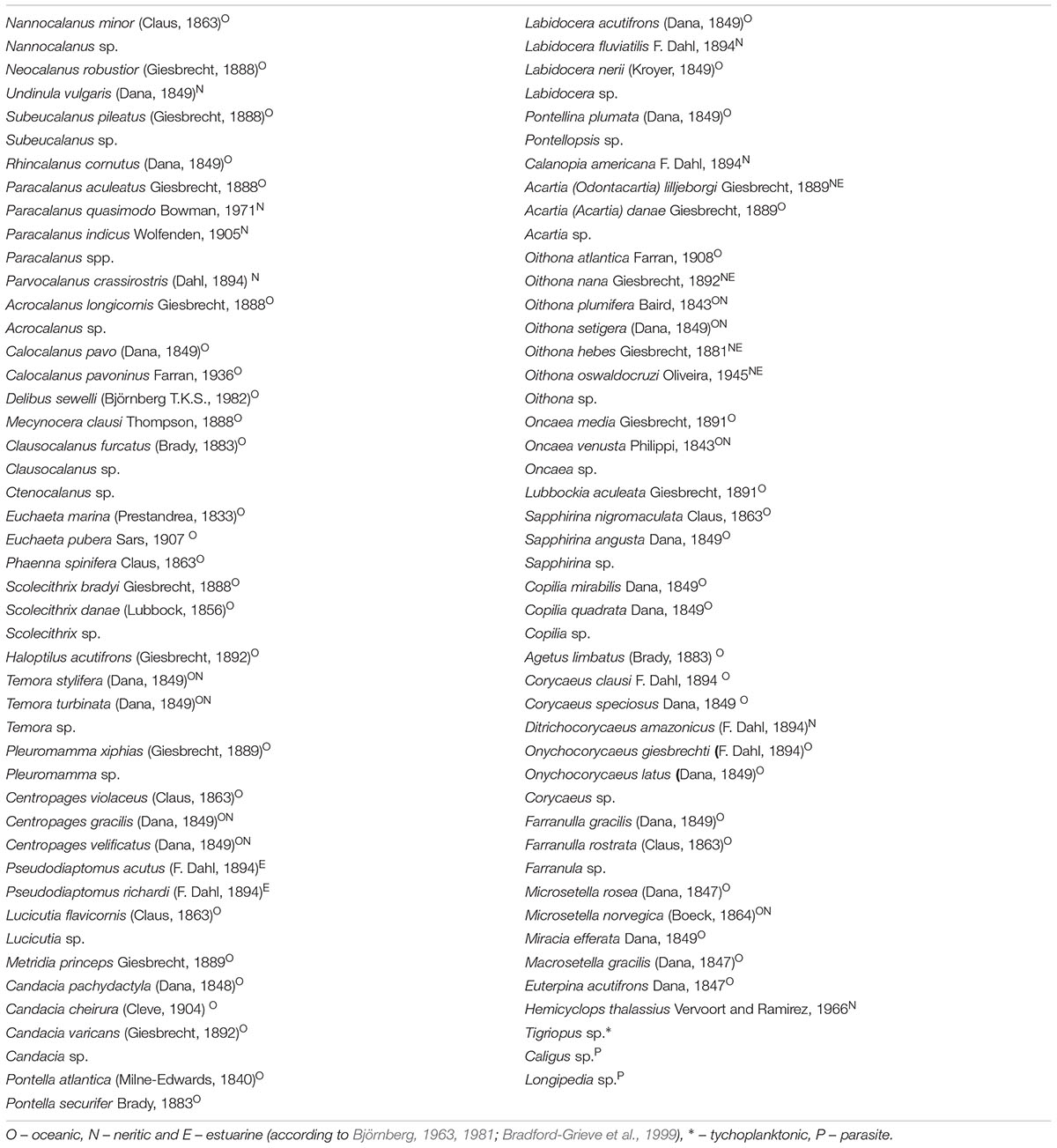
Table 2. List of Copepoda species from coastal and oceanic areas of Rio Grande do Norte, Northeastern Brazil.
The most important group was Copepoda, both in richness and abundance. There were 93 copepod species, of which 62% were calanoids. In total, 54 species occurred in the microzooplankton fraction, 69 species in the mesozooplankton fraction, and 68 species in the macrozooplankton fraction. An important group found in the Coastal area was the estuarine plume indicators, which consisted of Acartia (Odontacartia) lilljeborgi, Parvocalanus crassirostris, Oithona hebes, Oithona oswaldocruzi and Euterpina acutifrons.
In the microzooplankton fraction, there was a high frequency of occurrence of Crustacea nauplius (94%), Bivalvia veliger (92%), Gastropoda veliger (82%), and Oithona nana (76%); furthermore, nearly 51% of the species occurred at frequencies of less than 10%. Tintinnina was observed only in the microzooplankton fraction with 18 species. The higher frequency of occurrence recorded in the mesozooplankton fraction were of Bivalvia larvae (90%), O. nana, and Undinula vulgaris (81%), as well as Nannocalanus minor (74%); nearly 50% of the taxa occurred at frequencies of less than 10%. In the macrozooplankton fraction, the most frequent group was composed of Onychocorycaeus giesbrechti (80%) and U. vulgaris (79%), and nearly 68% of the community occurred at a frequency of occurrence of less than 10%.
Total Density (ind⋅m-3)
At the dry season, the total microzooplankton density varied from 904 ind⋅m-3 (Slope, Campaign 3) to 161.6 × 106 ind⋅m-3 (Coastal, Campaign 1). At the rainy season, total microzooplankton densities ranged from 2,985 ind⋅m-3 (Inner Shelf, Campaign 2) to 34 × 105 ind⋅m-3 (Inner Shelf, Campaign 4). During both the dry and rainy seasons significant differences among the areas were found (Kruskal–Wallis, p > 0.05). During the dry season, the coastal area was different from all the other, and the inner shelf was different from the slope area (Dunn, p < 0.05). A decreasing pattern in total density was observed during this season. During the rainy season the coastal area was different from all other areas, presenting higher total density values, and no further differences were detected (Dunn, p < 0.05) (Figure 4).
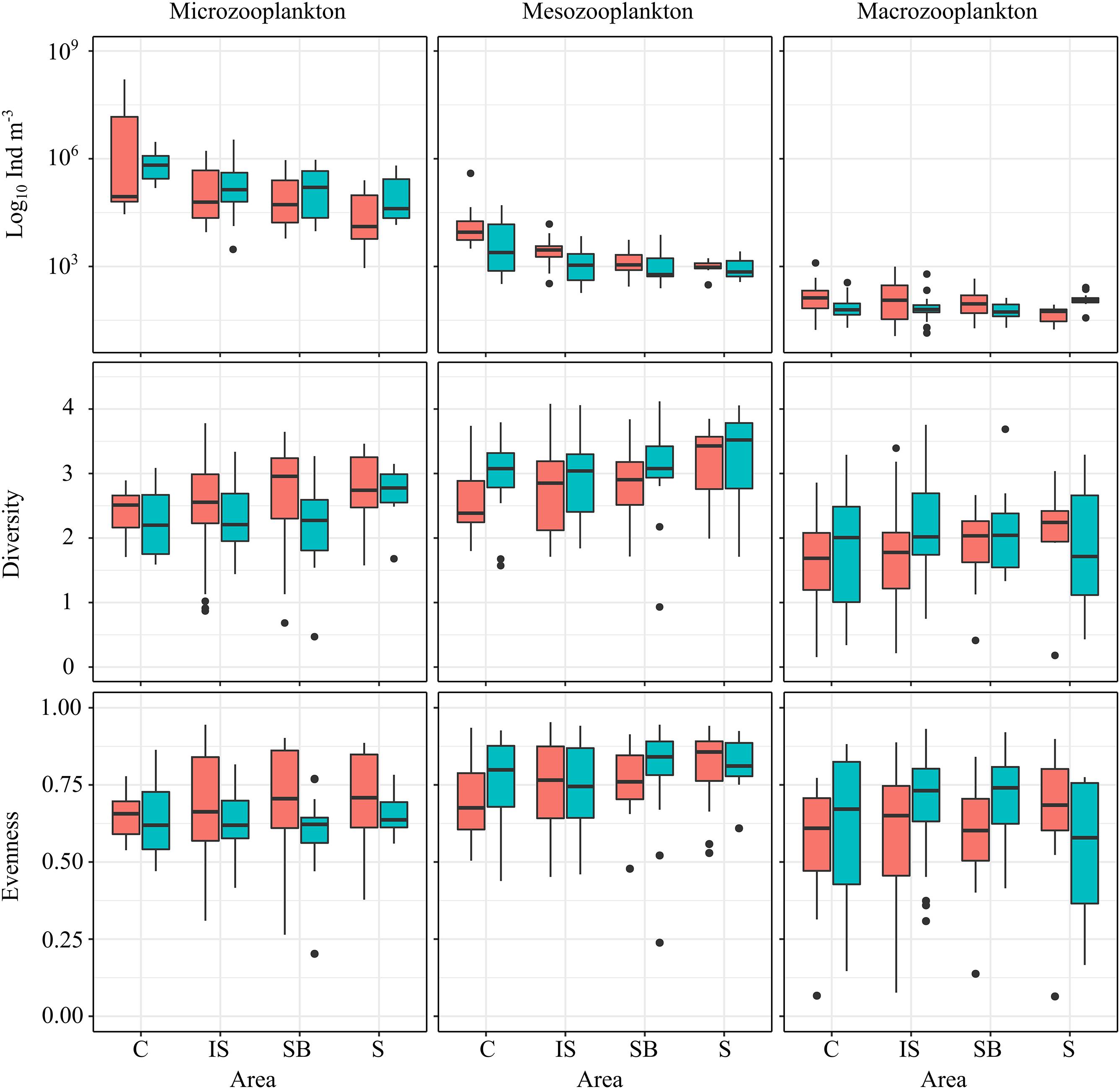
Figure 4. Box-plot of zooplankton density, diversity and evenness at the coastal and oceanic areas off Rio Grande do Norte, Southwestern Atlantic. Dots = outliers.
The total mesozooplankton density varied from 275 ind⋅m-3 (Shelf Break, Campaign 1) to 39.2 × 104 ind⋅m-3 (Coastal, Campaign 1) during the dry season, and from 183 ind⋅m-3 (Inner shelf, Campaign 2) to 50.9 × 103 ind⋅m-3 (Coastal, Campaign 4) during the rainy season. During the rainy season no difference among the areas was found (Kruskal–Wallis, p > 0.05), but during the dry season a gradient in the distribution of the total density was detected in which the Coastal area presented densities significntly higher than all the other areas, and the Inner Shelf presented higher densities than the Shelf break and Slope (Dunn, p < 0.05). No difference between the Shelf break and Slope was detected (Dunn, p > 0.05) (Figure 4).
The total macrozooplankton density varied from 11.5 ind⋅m-3 (Inner shelf, Campaign 3) to 1,251 ind⋅m-3 (Coastal, Campaign 3) during the dry season, and from 14 ind⋅m-3 (Inner shelf, Campaign 4) to 613 ind⋅m-3 (Inner shelf, Campaign 4) during the rainy season. During the dry season no evidence for differences among the areas was detected (Kruskal–Wallis, p > 0.05). During the rainy season differences were detected (Kruskal–Wallis, p < 0.05), and the Slope area was different from all the other areas (Dunn, p < 0.05), with higher density values (Figure 4).
Total Copepoda density varied from 3 ind⋅m-3 (Inner Shelf, Campaign 3) to 480 ind⋅m-3 (Inner Shelf, Campaign 1) during the dry season, and from 4 ind⋅m-3 (Shelf Break, Campaign 2) to 582 ind⋅m-3 (Inner Shelf, Campaign 4) during the rainy season. The statistical results were the same as for the total macrozooplankton density, since Copepods constituted most of the organisms within the macrozooplankton size class.
Fish eggs varied from 0 (several samples) to 53 eggs⋅m-3 (Coastal, Campaign 1) during the dry season, and from 0 (several samples) to 13 larvae⋅m-3 (Inner Shelf, Campaign 4) during the rainy season. During the dry season, a decreasing pattern in total density of fish eggs m-3 was detected (Kruskal–Wallis, p < 0.05), with higher values at the coastal area, and lower values at the slope (Dunn, p < 0.05). No evidence for differences in fish larvae total density was detected across the areas during the dry season (Kruskal–Wallis, p > 0.05). During the rainy season, no difference in fish eggs density m-3 was observed (Kruskal–Wallis, p > 0.05). However, fish larvae density m-3 was higher at the Slope when compared to all other areas (Dunn, p < 0.05).
Copepods Diversity
The Shannon diversity index for copepods ranged from 0.155 bits⋅ind-1 (Coastal, Rainy season, macrozooplankton) to 4.12 bits⋅ind-1 (Shelf break, Dry season, mesozooplankton) (Figure 4). Although an increasing trend from coastal to offshore stations in Copepoda diversity was observed, this pattern was not statistically confirmed, nor any interaction effect among seasons and spatial areas was detected (two-way ANOVA, p > 0.05). The minimum evenness was 0.064 (Slope, Rainy season, macrozooplankton), and the maximum evenness was 0.953 (Inner shelf, Rainy season, mesozooplankton) (Figure 4). No spatial gradient was detected on evenness in any season (Kruskal–Wallis test, p > 0.05).
Seston Biomass (mg⋅m-3)
The total seston biomass decreased sharply from the microzooplankton to macrozooplankton fractions (Figure 5). Mesozooplankton biomass was significantly lower than the microzooplankton biomass (Kruskal–Wallis, p < 0.05), with values lower than 300 mg⋅m-3. This pattern occurred during all campaigns, except in Campaign 2, when the macrozooplankton fraction had a higher biomass than the mesozooplankton. The biomass spatial distribution pattern varied over time, which demonstrated the importance of collecting different size classes of zooplankton. In all campaigns, microzooplankton biomass peaks were registered near coastal areas, and significant differences (Kruskal–Wallis, p < 0.05) were found between the Coastal and other areas. Higher macrozooplankton biomass occurred at the stations over the slope; however, the biomass was low in comparison to other fractions.
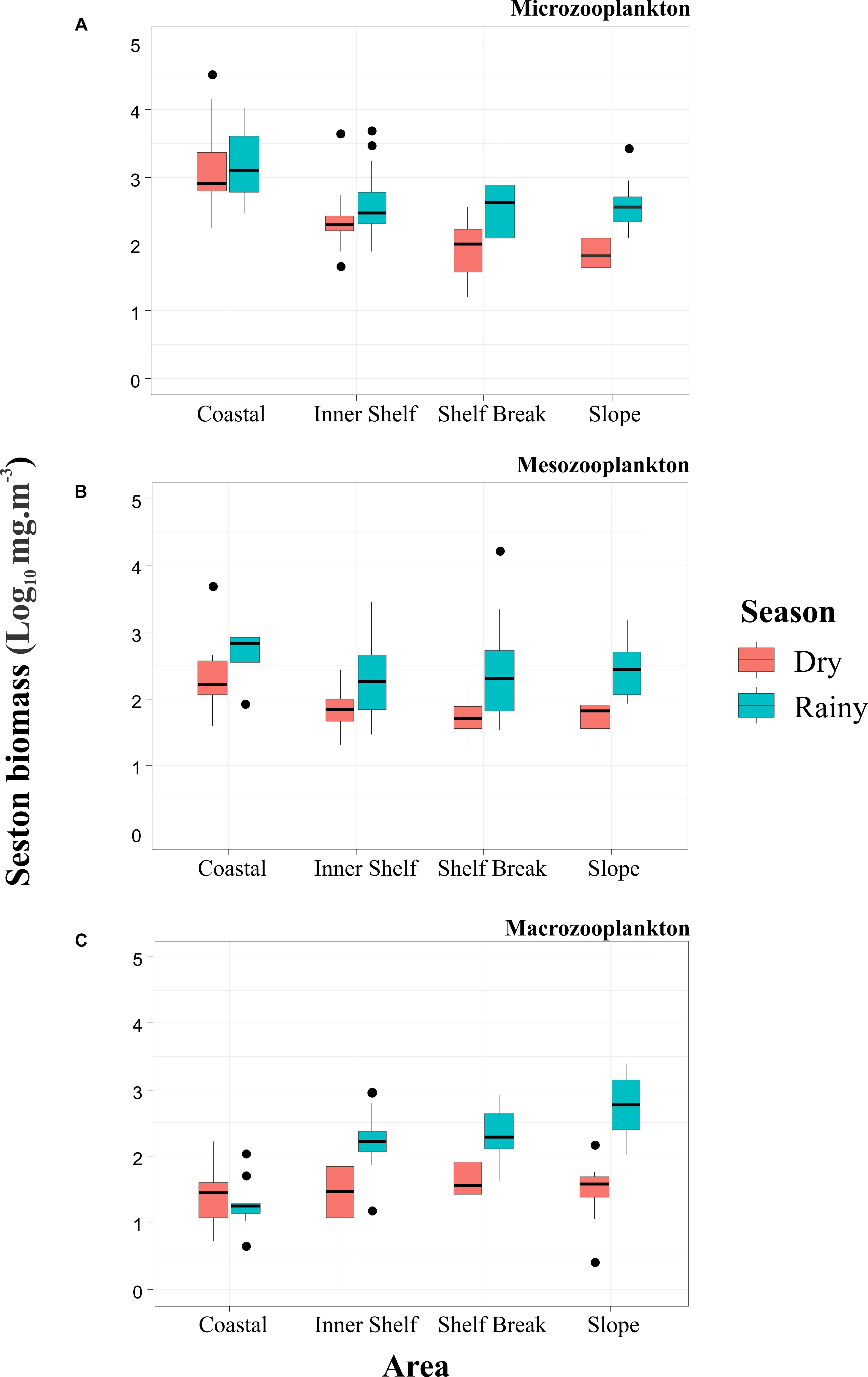
Figure 5. Box-plot of seston biomass (Log10 mg⋅m-3) of microzooplankton (A), mesozooplankton (B), and macrozooplankton (C) at the coastal and oceanic areas off Rio Grande do Norte, Southwestern Atlantic. Dots = outliers.
The minimum and maximum values of seston biomass for the microzooplankton community occurred in the campaign 1, varying from 16.35 mg⋅m-3 in the Shelf Break to 34,798.30 mg⋅m-3 in the Coastal region. The minimum recorded for the mesozooplankton community occurred in the Slope in the campaign 3 (18.67 mg⋅m-3) and the maximum (17,285.5 mg⋅m-3) in the Shelf Break in the campaign 4. The minimum and maximum biomass for the macrozooplankton community varied between 0.06 mg⋅m-3 in the Inner Shelf (Campaign 3) to 2434 mg⋅m-3 in the Slope in the campaign 2.
The spatial effect over the seston biomass of the microzooplankton community was verified in both sampled seasons (ANOVA Kruskal–Wallis, p < 0.05) (Figure 5). A decreasing trend from Coastal to Slope occurred for the microzooplankton in the dry season (Figure 5). From the post hoc test we found that the average biomass recorded during the dry season in the Coastal region (4666.9 ± 9498.9 mg⋅m-3) was significantly higher than those recorded in the Inner Shelf (347.3 ± 776.9 mg⋅m-3), Shelf Break (121.2 ± 98.8 mg⋅m-3) and Slope (92.9 ± 68.4 mg⋅m-3) (Dunn, p < 0.05). In the rainy season, the same pattern was observed. The coastal region (2929.5 ± 3274.5 mg⋅m-3) recorded a significantly higher biomass than the Inner Shelf (617.1 ± 938.5 mg⋅m-3), Shelf Break (728.2 ± 898.7 mg⋅m-3) and the Slope (560 ± 695.2 mg⋅m-3) (Dunn, p < 0.05).
The seston biomass of mesozooplankton differed among the marine areas only during the dry season (ANOVA Kruskal–Wallis, p < 0.05) (Figure 5). The Coastal area presented a biomass of 871.5 ± 1,734.6 mg⋅m-3 and this value was significantly higher than the Inner Shelf (84.3 ± 55.4 mg⋅m-3), Shelf Break (63.3 ± 42.1 mg⋅m-3) and Slope (67.2 ± 41.6 mg⋅m-3) (Dunn, p < 0.05). During the rainy season the average values were 637.3 ± 456.6 mg⋅m-3, 464.8 ± 728.6 mg⋅m-3, 1,221.2 ± 3,813 mg⋅m-3 and 407 ± 411.4 mg⋅m-3, respectively recorded to the Coastal, Inner Shelf, Shelf Break and Slope areas.
The seston biomass of the macrozooplankton community differed significantly only in the rainy season (ANOVA Kruskal–Wallis, p < 0.05) (Figure 5). During this season, an increase in biomass toward the Slope was recorded (Figure 5), with values in the Coastal (28.6 ± 33.3 mg⋅m-3) significantly lower than that observed in the Inner Shelf (259.1 ± 242.7 mg⋅m-3), Shelf Break (307.1 ± 242.8 mg⋅m-3) and Slope (876.8 ± 745.7 mg⋅m-3) (Dunn, p < 0.05). For the dry season, the averages recorded in the Coastal, Inner Shelf, Shelf Break and Slope areas were, respectively 37.6 ± 42.5 mg⋅m-3, 42.6 ± 40.6 mg⋅m-3, 59.1 ± 55.2 mg⋅m-3 and 46 ± 46 mg⋅m-3.
Zooplankton Community Structure
The nMDS analyses indicated differences in the structure of micro- (Figure 6A), meso- (Figure 6B) and macrozooplankton (Figure 6C) community between areas, as well as marked differences among the seasonal periods sampled (Figures 6D–F). The micro-, meso- and macrozooplankton community differences across a coastal-oceanic gradient was verified through the PERMANOVA test (Table 3). The seasonal effect was also highlighted as an important factor responsible for the dissimilarity of zooplankton investigated communities (Table 3). We recorded a significant interaction between the tested factors (area and season) only for the macrozooplankton community (Table 3C).
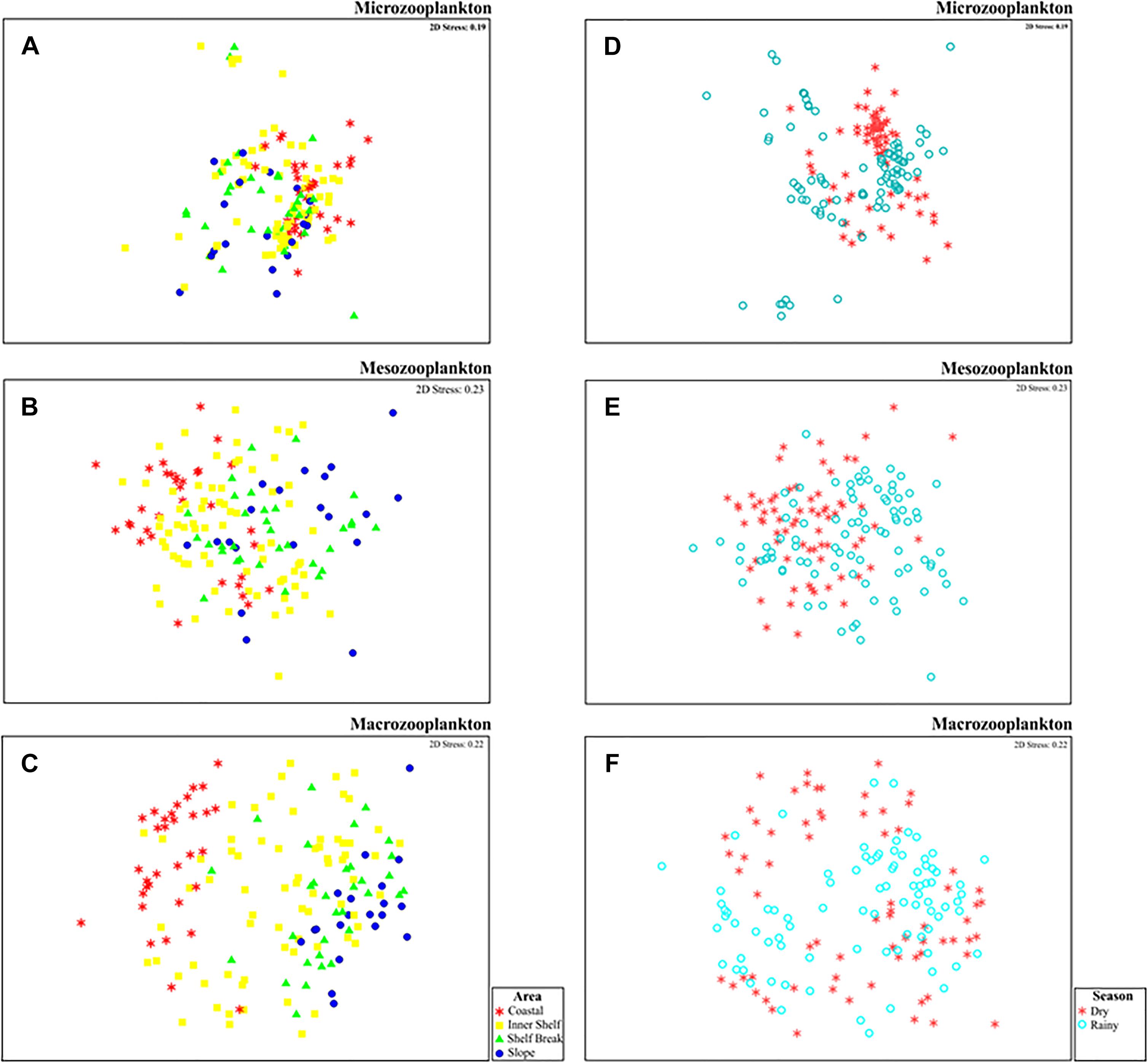
Figure 6. (A–C) MDS ordination of the micro-, meso- and macrozooplankton (respectively) in response to the area (Coastal-red asterisks; Inner Shelf-Yellow squares; Shelf Break-Green triangles; and Slope-Blue circles). (D–F) MDS ordination of the micro-, meso- and macrozooplankton (respectively) in response to seasonality (dry season-red asterisks; and rainy season-empty blue circles).
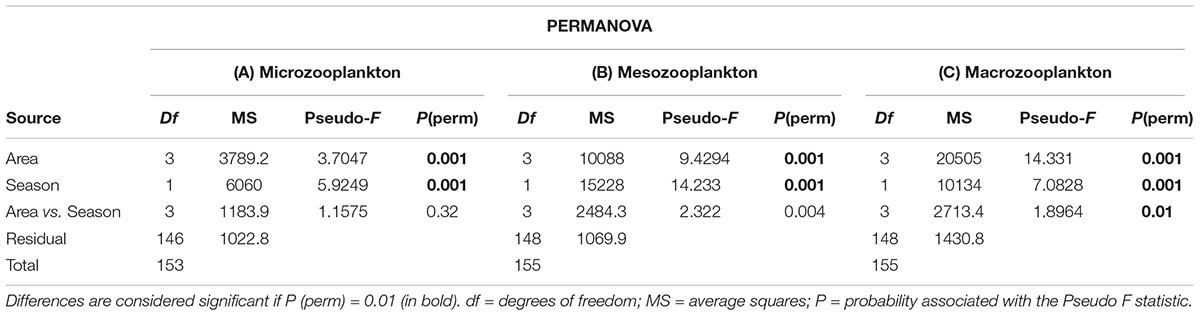
Table 3. Summary: PERMANOVA, this analysis tests differences in quantitative taxonomic composition of the micro- meso- and macrozooplankton community considering area and season as factors.
Results of pairwise t tests indicate that the coastal area differs from the Inner Shelf, Shelf Break and Slope in terms of the taxonomic composition of micro-, meso- and macrozooplankton community (Table 4). However, the greatest difference was recorded between the coastal area and the slope for the micro- (Table 4A) and mesozooplankton (Table 4B). The significant interaction between the area and season for the macrozooplankton showed that in the dry season the greatest dissimilarity in the taxonomic composition occurred between the Coastal area and the Shelf Break (Table 4C), while in the rainy season occurred between Coastal area and the Slope (Table 4D).
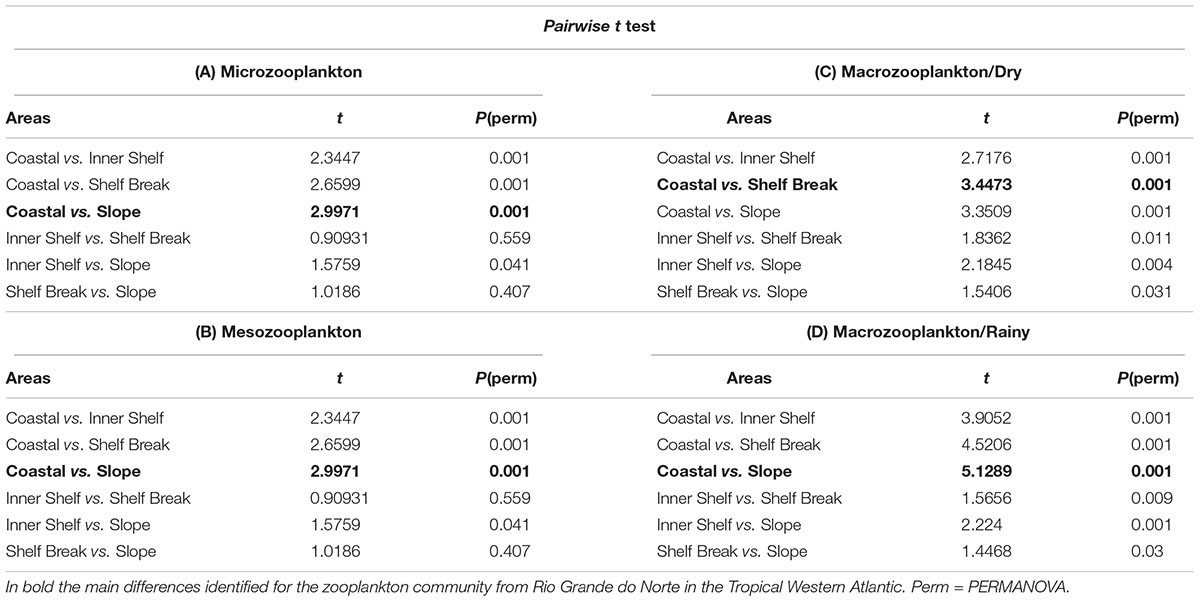
Table 4. Results of pairwise t test of the micro- meso- and macrozooplankton community structure considering area and season as factors.
The SIMPER analysis reinforced the greatest dissimilarity of the taxonomic composition of the microzooplankton occurring between the Coastal and the Slope areas (Average dissimilarity = 46.01), whose cumulative contribution of the taxa, O. hebes, E. acutifrons, P. crassirostris, Oikopleura dioica, Oithona (copepodite) and Oithona sp. corresponded to 51.86% of the dissimilarity (Table 5A). For the mesozooplankton the greatest dissimilarity (64.49) was also recorded between the Coastal area and the Slope (Table 5B). The taxa, O. nana, N. minor, Temora turbinata, O. hebes, P. crassirostris and Clausocalanus furcatus corresponded to 52.26% of the differences recorded between these areas (Table 5B).
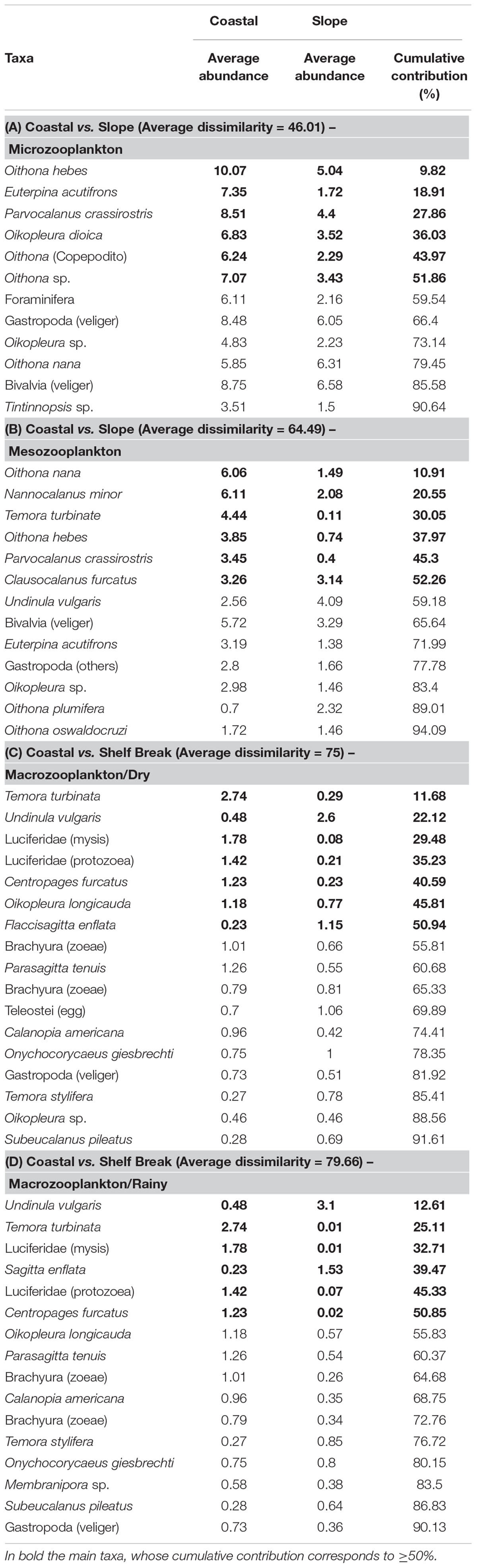
Table 5. Results of SIMPER analysis for micro-, meso- and macrozooplankton and the zooplankton taxa that contributed to the composition and their values in %, off Rio Grande do Norte, Southwestern Atlantic.
For the macrozooplankton, the greatest dissimilarity (average dissimilarity = 75) of the taxonomic composition observed in the dry season between the coastal area and the Shelf Break occurred as a response of the highest contribution of the taxa, T. turbinata, U. vulgaris, Lucifer sp. (mysis), Lucifer sp. (protozoea), Centropages furcatus, Oikopleura longicauda and Flaccisagitta enflata. These showed a cumulative contribution of 50.94%. (Table 5C). In the rainy season, the high difference between the Coastal area and the Slope (Average dissimilarity = 79.66) occurred due to the greater cumulative contribution of 50.85% of the taxa U. vulgaris, T. turbinata, Lucifer sp. (mysis), F. enflata, Luciferidae (protozoea) and C. furcatus (Table 5D).
The RDA explained 68% of the variance in the zooplankton community (Figure 7). Two vectors with significant values (RDA, p < 0.05) were highlighted for the community. One vector separated the coastal from the other areas (Figure 7) and other associated the zooplankton to temperature. The groups most associated with the coastal area were mainly the organisms of the microozooplankton (both sestonic biomass and abundance); however, the other zooplanktonic size fractions were also associated with the coastal area. The only group associated with temperature was the macrozooplankton biomass. Coastal and temperature vectors explained together 33% of the variability.
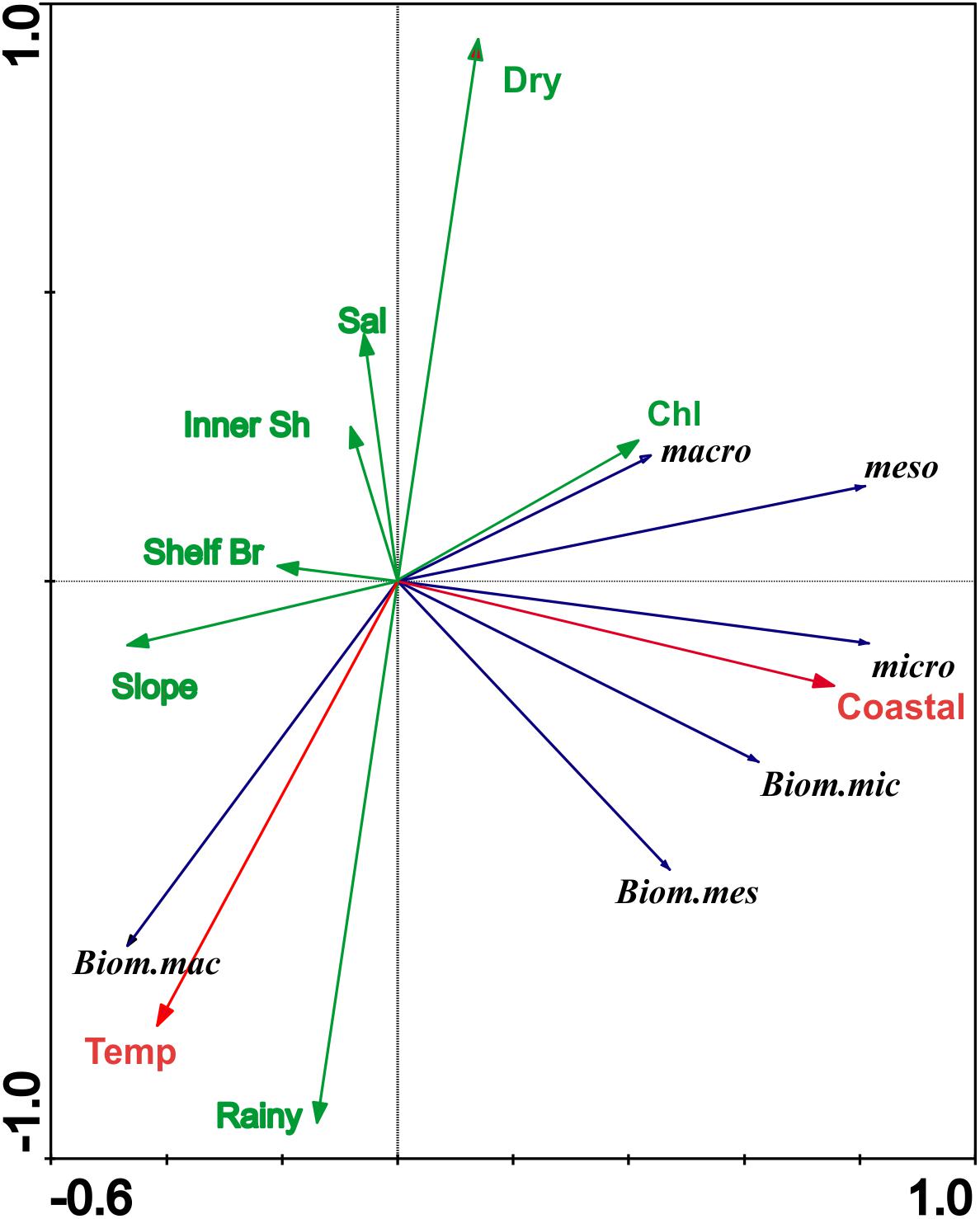
Figure 7. Ordination diagram of the Redundancy Analysis (RDA). Significant vectors of spatial-temporal are in red, non-significant vectors in green, and taxon classification in blue for Zooplankton at the coastal and oceanic areas off Rio Grande do Norte, Southwestern Atlantic. Areas: Coastal, Inner Shelf, Shelf Break, and Slope; Rainy: Rainy season; Dry: Dry season: Sal: Salinity; Chl: Chlorophyll a; macro: macrozooplankton; meso: mesozooplankton; micro: microzooplankton; Bio_macro: biomass macrozooplankton; Bio_meso: Biomass mesozooplankton, and Bio_micro: biomass microzooplankton.
A cluster analysis of the samples based on Copepoda presented four groups (Cophenetic r = 0.89), which corresponded to the four campaigns (Figure 8). In the groups corresponding to Campaigns 1 and 2, two subgroups were formed and consisted of Coastal and Inner Shelf samples at one subgroup, and Shelf Break and Slope samples at the other. In Campaigns 3 and 4, the Coastal stations were separated from the subgroups formed by Inner Shelf, Shelf Break, and Slope.
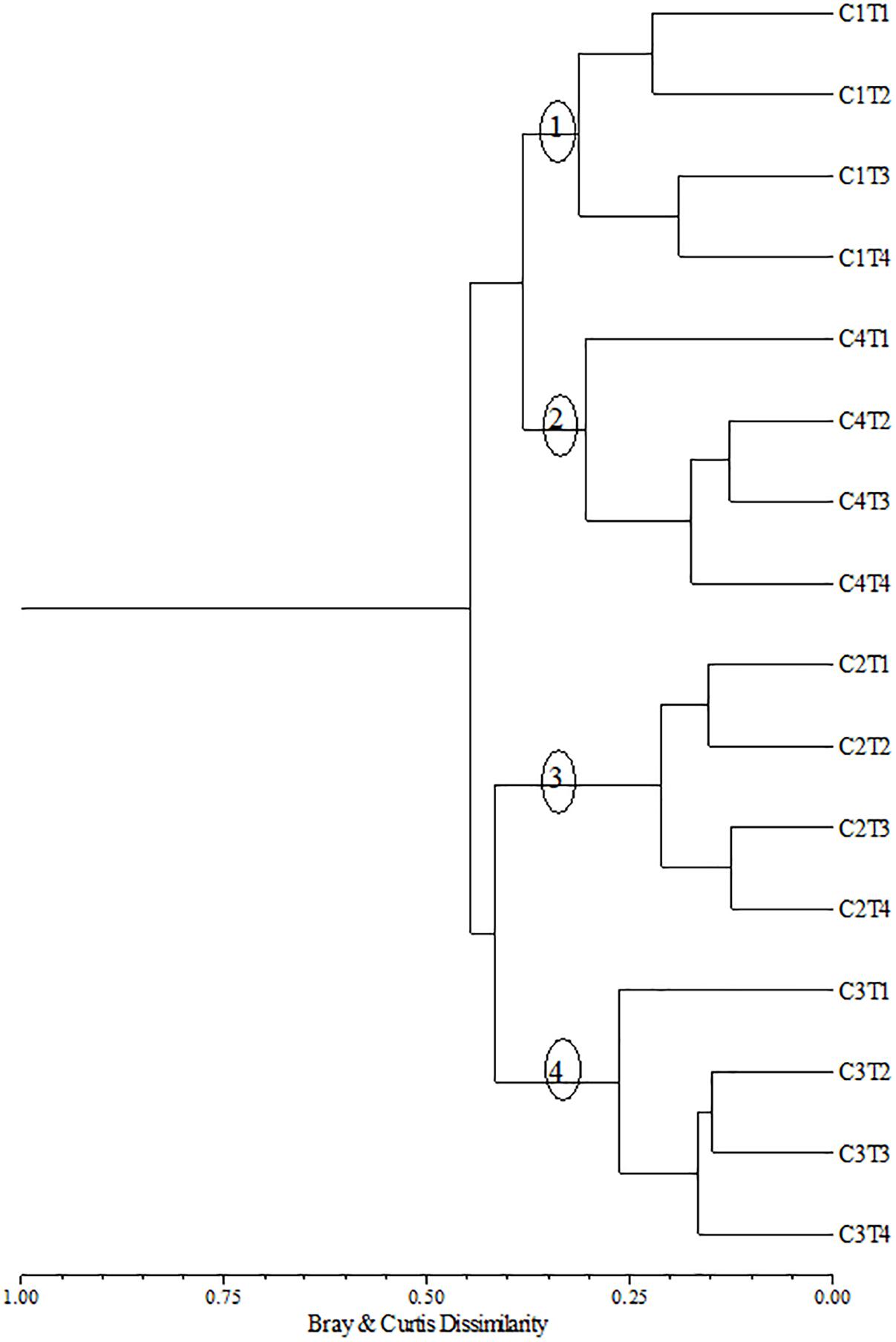
Figure 8. Dendrogram of samples based on Copepoda density from the coastal and oceanic areas off Rio Grande do Norte, Southwestern Atlantic. Bray–Curtis index and Ward linkage clustering. C = Campaign (C1 = July 21–30, 2002, C2: May 12–30, 2003, C3: November 14–23, 2003, C4: May 17–31, 2004). T = Transect (T1: <10 m, T2: 10–20 m, T3: 20–50 m, and T4: >50 m).
Functional Traits
The major functional traits dividing the Copepoda community was the reproductive and trophic attributes, which formed three main groups: herbivorous and broadcaster (Group 1), omnivorous-detritivorous, omnivorous-herbivorous, carnivorous and sac-spawner (Group 2), and carnivorous-omnivorous and sac-spawner (Group 3) (Figure 9). These groups each included different lengths, costal distances and vertical migration.
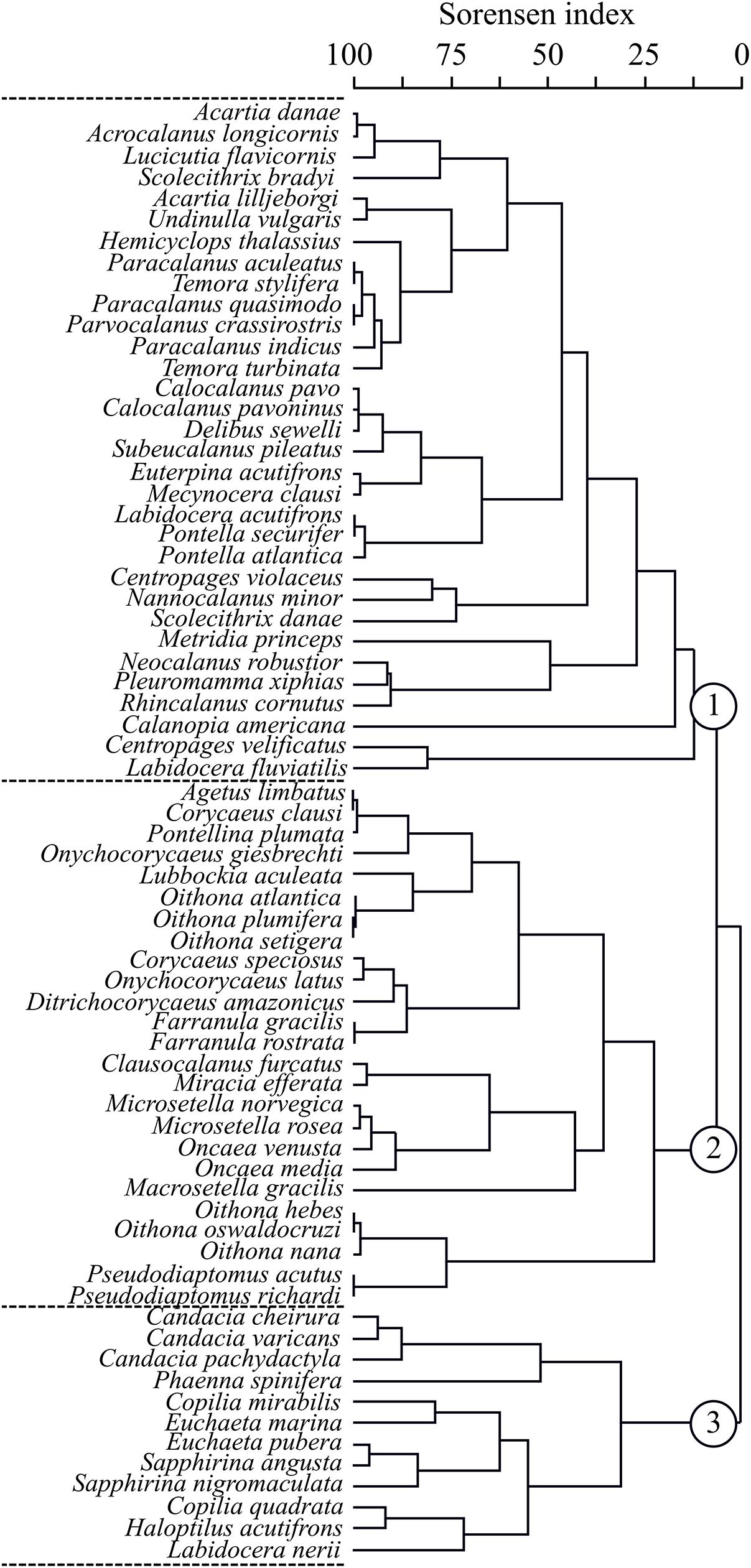
Figure 9. Dendrogram of the Copepoda species that have been clustered by functional traits identified from July 2002 to May 2004 in coastal and oceanic areas off Rio Grande do Norte, Southwestern Atlantic. The numbers 1, 2 and 3 represent the main groups. Sorensen index and average linkage clustering.
Group 1 comprised mostly species with broadcaster reproduction, with epipelagic offshore distribution, herbivorous and filter feeding, non-migrant behavior and smaller sizes varying from 500 to 1500 μm. This group contained mainly calanoids of the genera Acartia, Calocalanus, Temora, Paracalanus, Calanopia, Undinula and Centropages. Group 2 consisted mostly of sac-spawner species, neritic or neritic-oceanic, epi-meso-bathipelagic and presented three sub-groups, sub-group 1: carnivorous, sub-group 2: omnivorous-herbivorous and sub-group 3: omnivorous-detritivorous. All Oithona spp., which exhibit active ambush behavior belonged to sub-group 2. Group 3 also consisted of sac-spawners and included the large (2,500–6,500 μm) oceanic calanoids Euchaeta, Candacia, Labidocera and Haloptilus, and cyclopoids Saphirina and Copilia which exhibit omnivorous or carnivorous feeding habits, cruising or active ambush behavior types, and vertical diel migration that varies from weak to strong.
Discussion
The study of the zooplankton community structure and function between coastal and oceanic regions from Rio Grande do Norte in the Tropical Western Atlantic showed the great spatial diversity, seasonal variations and the productive potential, chiefly of the coastal region (<10 m depth), possibly caused by continental influence. The coastal zone is extremely complex and is affected by a variety of oceanographic processes (Walsh, 1976; Sousa et al., 2016) that are influenced by diverse anthropic impacts related to continental sources (Mee, 2012). In general, the seasonal distribution presented higher densities for the communities in the rainy season, when fertilization and discharge of nutrients from the continent are more intense.
Our study revealed a high number of taxa occurring in the mesozooplankton community and a greater numerical importance of the microzooplankton community. These results show that net mesh selectivity is an important factor to be considered in studies of the zooplankton community structure. The mesh size effect has already been investigated on the copepod assemblage in the South China Sea (Tseng et al., 2011) and on planktonic cnidarians from neritic and oceanic habitats in the equatorial Atlantic Ocean (Tosetto et al., 2019). The results of the mentioned studies demonstrate that the mesh size directly affects important descriptors (abundance and diversity) which are particularly useful for the management of marine resources, mostly of the marine regions studied (Coastal, Inner Shelf, Shelf Break and Slope).
Spatial differences were observed for the zooplanktonic communities among the marine regions investigated with a Coastal and Inner Shelf group (related to higher chlorophyll-a, shallow area and higher meroplanktonic forms) and Shelf Break and Slope group (linked to lower chlorophyll-a, deeper areas and higher percentage of holoplanktonics). However, greater dissimilarity was registered between the Coastal and the Slope areas (e.g., microzooplankton extremely high density was associated with the shallower coastal area). This Coastal region is enriched by rivers discharges from the continent (including outflow from the intensive shrimp culture ponds), delivering nutrients to the marine system. These nutrients stimulate the phytoplankton growth, favoring most zooplankton species, which are characteristically herbivores or omnivores (Johnson and Allen, 2005). This pattern is commonly found in Continental shelves (Neumann-Leitão et al., 1999, 2008; Dias et al., 2015; Bueno et al., 2017) and oceanic islands (Campelo et al., 2018; Santana et al., 2018) in Brazil and reflect the typical coast-ocean gradient.
An increase in macrozooplankton density was observed near the Shelf Break, caused by local topographic upwellings (Stramma et al., 1990). However, the condition of each location may strongly affect the distribution of the zooplankton, such as reefs currents, canyons, ravines, eddies and vortices (Regner, 1985), and alter the general pattern of the studied area. For instance, the Rio Grande do Norte coast has a narrow continental shelf; therefore, the zooplankton community has a high degree of influence from oceanic intrusions. Thus, a mixture of oceanic and neritic micro-, meso-, and macrozooplankton was observed, which resulted in difficulties separating the communities. As expected, diversity increased from inshore to offshore. This pattern is usual for SWA (Boltovskoy, 1981; Neumann-Leitão et al., 1999, 2008; Dias and Bonecker, 2009; Dias et al., 2015); however, due to the reproductive requirements, some species prefer shallow waters.
The high biomass of micro- and mesozooplankton was mainly recorded in the Coastal area in both studied seasonal periods, being able to consider that these fractions of the community are key elements in the planktonic food chain of this region. In addition, it is important to consider the large amount of particulate matter from the estuaries and bays (Lopes, 2007). These particulates matter (plankton, marine snows and detritus) are important components of the pelagic system because they are a food source for the zooplankton, being sampled more efficiently by smaller mesh sizes (Silva et al., 2019). In general, the lowest values of biomass observed for the macrozooplankton community occurred in the coastal area and the largest on the slope. This is a result of a coast-ocean size gradient (Marcolin et al., 2013), implying a change in the taxonomic composition of the organisms, with a greater contribution of siphonophores and fish larvae in the slope, considered organisms of larger body size, better sampled with the a net of 300 μm mesh size. A maximum of zooplankton biomass occurs in neritic waters of SWA (Boltovskoy, 1981; Dias et al., 2015), with 100 mg⋅m-3 in the top layer (200 m).
Copepoda were the most diverse group in all fractions, accounting for 93 species. This result is expected for the SWA tropical waters (Boltovskoy, 1981, 1999; Neumann-Leitão et al., 1999, 2008; Lopes et al., 1999; Bueno et al., 2017). Highest Copepoda diversity were registered in the mesozooplankton fraction during this study and most of them were of small size. In oligotrophic areas, species occur with smaller sizes and in developmental stages that are important in planktonic communities and these areas are dominated by microbial components (Calbet et al., 2001; Turner, 2004; Calbet, 2008). Small-sized copepods are a pivotal link between the classical and microbial food webs (Nakamura and Turner, 1997; Melo et al., 2014); they present a much higher growth rate (Peterson et al., 1991; Hopcroft et al., 1998) and influence the effectiveness of the trophic connection between primary producers/protozooplankton and larger species (Zervoudaki et al., 2007). One of the most oligotrophic oceans in the world is the Southwest Atlantic Ocean (Andrade et al., 2007); and, Dias et al. (2015) mention that oligotrophic areas present higher production than formerly supposed and have an extraordinary amount of biotic complexity, encompassing nearly 75% of marine surfaces.
Young forms (copepodites) of N. minor, Temora stylifera, C. furcatus, Centropages velificatus, and O. giesbrechti and adults of Microsetella norvegica and Macrosetella gracilis predominated the microzooplankton fraction. The species M. norvegica and M. gracilis were generally associated with the cyanobacterium Trichodesmium eritheum. Pelagic harpacticoids can also be found in close association with floating substrates, such as Trichodesmium (Calef and Grice, 1966; O’Neil and Roman, 1994; O’Neil, 1998; Uye et al., 2002). These blue-green colonies offer both a firm substratum and food for the accompanying plankton in tropical and subtropical oligotrophic oceans, thus supporting multifaceted microenvironments (Sheridan et al., 2002; Bergman et al., 2013).
The copepod P. crassirostris is very important to the microzooplankton community of the Coastal stations; P. crassirostris commonly occurs in nearly all Brazilian estuaries and the estuarine plume (Björnberg, 1981; Eskinazi-Sant’Anna and Björnberg, 2006), including those that are severely anthropically impacted (Schwamborn et al., 2004; Silva et al., 2004). This species forages mainly on pico- and nanoplankton fractions, behaving as an r-strategist (opportunistic) particle feeder and revealing high ingestion rates of the most profuse cells (2–5 μm nanoplankton) (Calbet et al., 2000). P. crassirostris is dominant in eutrophic systems, evidencing the estuarine effect in the coastal studied area. In the offshore direction, a characteristic zooplanktonic community is commonly found in reef areas. The high abundance of the Oithonidae and Paracalanidae families is typical of the coastal waters of Brazil (Björnberg, 1981; Dias and Bonecker, 2009).
Epipelagic, oceanic, and widespread species were the dominant groups in this region, and they occur in tropical and subtropical oceans (Atlantic, Pacific, and Indian), except for U. vulgaris and O. nana, which demonstrate neritic occurrence (Björnberg, 1981; Bradford-Grieve et al., 1999). There is a reported relation between U. vulgaris and coastal areas due to its occurrence in neritic environs; U. vulgaris exhibits a high abundance in many inshore plankton hauls in the surface layer (Razouls et al., 2005). However, U. vulgaris dominated among copepods in samples from oceanic provinces in the economic exclusive zone (EEZ) of Northeastern Brazil (Cavalcanti and Larrazábal, 2004; Melo et al., 2014; Campelo et al., 2018). In our study, U. vulgaris was also abundant at young stages under oceanic influence, which suggested that it also inhabits oceanic regions with an epi-mesopelagic distribution.
The wide distribution of numerous Copepoda species in our study is due their passive transportation by currents (Calocalanus pavo, U. vulgaris, Oithona plumifera, Oncaea venusta, M. gracilis, Microsetella rosea, and Corycaeus speciosus), Chaetognatha (F. enflata and Serratosagitta serratodentata), and Appendicularia (Oikopleura spp.) results in circumglobal distribution patterns. This occurs much more commonly than endemism for pelagial plankton (Boltovskoy et al., 2002; Pierrot-Bults and Angel, 2012). Species with high proportions of neritic organisms and benthopelagic forms are excluded from this pattern (Angel, 1993).
Chaetognatha predominated in the macrozooplankton fraction and comprised species from coastal and oceanic provinces; oceanic origin was predominantly represented. F. enflata, S. serratodentata, Flaccisagitta hexaptera, Sagitta bipunctata, and Krohnitta subtilis are indicators of the Tropical Water, and Parasagitta tenuis a coastal-water indicator. Pterosagitta draco have a wide distribution recorded in both oceanic and costal water masses (Boltovskoy, 1981). High densities of F. enflata are common to this area (Boltovskoy, 1981, 1999; Gusmão, 1986; Neumann-Leitão et al., 1999, 2008).
The zooplankton species indicator classified by Björnberg (1963, 1981) and Bradford-Grieve et al. (1999) showed that the most relevant Copepoda group in our analysis included a mixture of neritic and oceanic species from the North Brazil Current, which is under the Shelf Break influence that presents the general scenario of a huge reef system. Campos et al. (2017) found a similar result to a coastal area north of the present studied area. Also, holoplanktonic organisms, mainly the oceanic Copepoda, predominated the entire studied region, including the inshore area. This pattern is a consequence of the weak continental freshwater runoff over the shelf due to arid climate and geomorphology. Thus, freshwater flux fertilizes the very coastal area under 10 m depth. Other authors for Northeastern Brazil (e.g., Gusmão et al., 1998; Neumann-Leitão et al., 1999, 2008; Campos et al., 2017) registered this same pattern. The constant northeast tradewinds and arid climate in this area permits for the establishment of widespread beaches and dunes, which are in continuous movement due to the absence of anchoring vegetation (Mabesoone and Coutinho, 1970). These dunes prevent the free flux of rivers to the sea during dry season, and many freshwater lakes form behind the dunes. The changes recorded along the shelf seem to be chiefly driven by the rain and periodic blooms of benthic invertebrate larvae.
The role of zooplankton through functional groups involves information that precisely capture their complex dynamics (Everett et al., 2017). The zooplankton are represented by many functional groups, that oscillate in size from small (>0.002 μm) to large (>5000 μm) organisms. Zooplankton also presents vast trophic malleability that can modify considerably along a time interval as the community structure changes. Many external factors (e.g., nutrients, temperature, phytoplankton, competition, predation) regulate the growth of an organism and/or of a population, that by its turn is strongly connected to life cycles, such as reproduction with generations fluctuating (from days to years) (Williams and Conway, 1982). Zooplankton species modify its diet preferences throughout different periods or life stages, or substitute a food item behavior (Stibor et al., 2004; Sommer and Sommer, 2006). To complicate the dynamics of the zooplankton community, several species of microzooplankton are mixotrophic (Caron, 2016), the phytoplankton succession is seasonal (Pingree et al., 1976; O’Boyle and Silke, 2010) and many meroplanktonic species emerge in the shelf (Williams and Collins, 1986). Thus, zooplankton within a specified size class (e.g., microzooplankton, mesozooplankton, macrozooplankton) are thus unlikely to behave reliably over a spatial-temporal scale.
The main traits separating the Copepoda groups were the reproductive and trophic strategies, and a very similar outcome was found by Campos et al. (2017) to a shelf area located North of the present studied area; and, by Neumann-Leitão et al. (2018) for the reef system in the Amazonas coastal area. Trophic stratagems and reproduction are normally controlled by female weight, ecosystem temperature, and diet (Blaxter et al., 1998; Bunker and Hirst, 2004; Brun et al., 2017). In terms of feeding, Kiørboe and Sabatini (1995) presented three strategies in copepods: filtering, active ambushing and cruising; and, Kiørboe (2011) presented the following not necessarily exclusive feeding strategies: Passive (Ambush feeding, particle feeding) Active (Feeding currents, cruise feeding) Mixed (Combination of active and passive modes) and Other (Parasitic).
In conclusion, the study area presents spatial heterogeneity, with low diversity limited to Coastal stations; high diversity and low abundance occur along the Inner Shelf, Shelf Break, and Slope. This is a pelagic oligotrophic habitat located off the coast of tropical Brazil over a diverse, high biomass benthic habitat, which is mostly covered by calcareous algae functioning on the shelf as a large reef system. The narrow and shallow shelf is responsible for the strong connectivity between nearshore enriched coastal water and the oceanic oligotrophic Tropical Water mass, which results in a complex mixed system that is critical to the food web structure and forms a peculiar pattern of zooplankton diversity and distribution.
Author Contributions
SNL and RS coordinated the project. SNL, RS, AS, PM, and VP designed and coordinated the fieldwork. SNL, FPN, MMJ, RS, AS, and LF wrote and reviewed the manuscript. SNL, RS, MMJ, FPN, TS, DNV, PM, XD, AS, VP, LF, AC, RC, JS, and SL contributed with data and data analyses.
Funding
This research was supported by the PETRÓLEO BRASILEIRO S.A – PETROBRAS, the Brazilian Oil Company (Cooperation Program No. 2500.0056584.10.2 between PETROBRAS and UFPE-FADE).
Conflict of Interest Statement
The authors declare that the research was conducted in the absence of any commercial or financial relationships that could be construed as a potential conflict of interest.
Acknowledgments
We would like to thank the Department of Oceanography of the Federal University of Pernambuco for the laboratory support. We thank Márcia França Rocha, Guarani de Holanda Cavalcanti and Breno Frias Dutra from Petrobras for making this cooperation program possible and for the important suggestions. SNL would like to thank the CNPq for the scholarship support (Process 307649/2016-4). We also thank American Journal Experts for the English editing services.
Supplementary Material
The Supplementary Material for this article can be found online at: https://www.frontiersin.org/articles/10.3389/fmars.2019.00287/full#supplementary-material
Footnotes
References
Andrade, L., Gonzalez, A. M., Rezende, C. E., Suzuki, M., Valentin, J. L., and Paranhos, R. (2007). Distribution of HNA and LNA bacterial groups in the Southwest Atlantic Ocean. Braz. J. Microbiol. 38, 330–336. doi: 10.1590/s1517-83822007000200028
Angel, M. V. (1993). Biodiversity in the pelagic ocean. Conserv. Biol. 7, 760–772. doi: 10.1046/j.1523-1739.1993.740760.x
Benedetti, F., Gasparini, S., and Ayata, S. D. (2016). Identifying copepod functional groups from species functional traits. J. Plankton Res. 38, 159–166. doi: 10.1093/plankt/fbv096
Bergman, B., Sandh, G., Lin, S., Larsson, J., and Carpenter, E. J. (2013). Trichodesmium – a widespread marine cyanobacterium with unusual nitrogen fixation properties. FEMS Microbiol. Ver. 37, 286–302. doi: 10.1111/j.1574-6976.2012.00352.x
Björnberg, T. K. S. (1963). On the marine free-living copepods off Brazil. Bol. Inst. Oceanogr. 13, 3–142.
Björnberg, T. K. S. (1981). “Copepoda,” in Atlas del Zooplancton del Atlantico Sudoccidental y Métodos de Trabajos com el Zooplancton Marino, ed. D. Boltovskoy (Mar del Plata: INIDEP), 587–679.
Blaxter, M. L., De Ley, P., Garey, J. R., Liu, L. X., Scheldeman, P., Vierstraete, A., et al. (1998). A molecular evolutionary framework for the phylum Nematoda. Nature 392, 71–75. doi: 10.1038/32160
Boltovskoy, D. (1981). Atlas Del Zooplancton Del Atlantico Sudoccidental y Métodos De Trabajos com el Zooplancton Marino. Mar del Plata: INIDEP.
Boltovskoy, D., Correa, N., and Boltovskoy, A. (2002). Marine zooplankton diversity: a view from the South Atlantic. Oceanol. Acta 25, 271–278. doi: 10.1016/s0399-1784(02)01199-4
Bradford-Grieve, J. M., Markhaseva, E. L., Rocha, C. E. F., and Abiahy, B. (1999). “Copepoda,” in South Atlantic Zooplankton, ed. D. Boltovskoy (Leiden: Backhuys Publishers), 869–1098.
Brun, P., Payne, M. R., and Kiørboe, T. (2017). A trait database for marine copepods. Sys. Sci. Data 9, 99–113. doi: 10.5194/essd-9-99-2017
Bueno, M., Alberto, S. F., Carvalho, R., Costa, T. M., Ciotti, A. M., and Christofoletti, R. A. (2017). Plankton in waters adjacent to the Laje de Santos state marine conservation park. Brazil: spatio-temporal distribution surveys. Braz. J. Oceanogr. 65, 564–575. doi: 10.1590/s1679-87592017129006504
Bunker, A. J., and Hirst, A. I. G. (2004). Fecundity of marine planktonic copepods: global rates and patterns in relation to chlorophyll a, temperature, and body weight. Mar. Ecol. Prog. Ser. 279, 161–181. doi: 10.3354/meps279161
Calbet, A. (2008). The trophic roles of microzooplankton in marine systems. ICES J. Mar. Sci. 65, 325–331. doi: 10.1093/icesjms/fsn013
Calbet, A., Garrido, S., Saiz, E., Alcaraz, M., and Duarte, C. M. (2001). Annual zooplankton succession in coastal NW Mediterranean waters, the importance of the smaller size fractions. J. Plankton Res. 23, 319–331. doi: 10.1093/plankt/23.3.319
Calbet, A., Landry, M. R., and Scheinberg, R. D. (2000). Copepod grazing in a subtropical bay: species-specific responses to a midsummer increase in nanoplankton standing stock. Mar. Ecol. Prog. Ser. 193, 75–84. doi: 10.3354/meps193075
Calef, G. W., and Grice, G. D. (1966). Relationship between the bluegreen alga Trichodesmium thiebautii and the copepod Macrosetella gracilis in the plankton of South America. Ecology 47, 855–856. doi: 10.2307/1934274
Calinski, R. B., and Harabasz, J. (1974). A dendrite method for cluster analysis. Communic. Stat. 3, 1–27. doi: 10.1080/03610927408827101
Campelo, R. P. S., Diaz, X. F. G., Santos, G., Melo, P. A. M. C., Melo Junior, M., Figueiredo, L. G. P., et al. (2018). Small-scale distribution of the mesozooplankton in a tropical insular system. Braz. J. Oceanogr. 66, 15–29. doi: 10.1590/s1679-87592018147306601
Campos, C. C., Garcia, T. M., Neumann-Leitão, S., and Soares, M. O. (2017). Ecological indicators and functional groups of copepod assemblages. Ecol. Ind. 83, 416–426. doi: 10.1016/j.ecolind.2017.08.018
Caron, D. A. (2016). Mixotrophy stirs up our understanding of marine food webs. Proc. Natl. Acad. Sci. U.S.A. 113, 2806–2808. doi: 10.1073/pnas.1600718113
Cavalcanti, E. A. H., and Larrazábal, M. E. (2004). Macrozooplâncton da zona econômica exclusiva do nordeste do Brasil (segunda expedição oceanográfica -REVIZEE/NE II) com ênfase em copepoda (Crustacea). Rev. Bras. Zool. 21, 467–475. doi: 10.1590/s0101-81752004000300008
Clark, D. R., Aazem, K. V., and Hays, G. C. (2001). Zooplankton abundance and community structure over a 4000 km transect in the North-east Atlantic. J. Plankton Res. 23, 365–372. doi: 10.1093/plankt/23.4.365
Cowen, R. K., Paris, C. B., and Srinivasan, A. (2006). Scaling of connectivity in marine populations. Science 311, 522–527. doi: 10.1126/science.1122039
Dias, C. O., Araujo, A. V., Vianna, S. C., Loureiro Fernandes, L. F., Paranhos, R., Suzuki, M. S., et al. (2015). Spatial and temporal changes in biomass, production and assemblage structure of mesozooplanktonic copepods in the tropical south-west Atlantic Ocean. J. Mar. Biol. Assoc. U.K. 95, 483–496. doi: 10.1017/s0025315414001866
Dias, C. O., and Bonecker, S. L. C. (2009). The copepod assemblage (Copepoda: Crustacea) on the inner continental shelf adjacent to camamu Bay, northeast Brazil. Zoologia 26, 629–640. doi: 10.1590/s1984-46702009000400007
Eskinazi-Sant’Anna, E., and Björnberg, T. K. S. (2006). Seasonal dynamics of microzooplankton in the são sebastião channel (SP, Brazil). Braz. J. Biol. 66, 221–231. doi: 10.1590/s1519-69842006000200006
Everett, J. D., Baird, M. E., Buchanan, P., Bulman, C., Davies, C., Downie, R., et al. (2017). Modeling what we sample and sampling what we model: challenges for zooplankton model assessment. Front. Mar. Sci. 4:77. doi: 10.3389/fmars.2017.00077
Forbes, A. E., and Chase, J. M. (2002). The role of habitat connectivity and landscape geometry in experimental zooplankton metacommunities. Oikos 96, 433–440. doi: 10.1034/j.1600-0706.2002.960305.x
Gomes, M. P., and Vital, H. (2010). Revisão da compartimentação geomorfológica da plataforma continental norte do rio grande do norte. Brasil. Rev. Bras. Geoc. 40, 321–329. doi: 10.25249/0375-7536.2010403321329
Guichard, F., Levin, S. A., Hastings, A., and Siegel, D. (2004). Toward a dynamic metacommunity approach to marine reserve theory. BioScience 54, 1003–1011.
Gusmão, L. M. O. (1986). Chaetognatha planctônicos de províncias nerítica e oceânica do Nordeste do Brasil. MSc Thesis, Recife: Federal University of Pernambuco, 1–160.
Gusmão, L. M. O., Diaz, X. F. G., Melo Junior, M., Schwamborn, R., and Neumann-Leitão, S. (2015). Jellyfish diversity and distribution patterns in the tropical Southwestern Atlantic. Mar. Ecol. 36, 93–103. doi: 10.1111/maec.12119
Gusmão, L. M. O., Neumann-Leitão, S., Nascimento-Vieira, D. A., Silva, T. A., Silva, A. P., Porto-Neto, F. F., et al. (1998). Zooplâncton oceânico entre os estados do ceará e pernambuco-Brasil. Trab. Oceanogr. Univ. Fed. PE. 25, 17–30.
Hopcroft, R. R., Roff, J. C., and Lombard, D. (1998). Production of tropical copepods in Kingston Harbor, Jamaica: the importance of small species. Mar. Biol. 130, 593–604. doi: 10.1007/s002270050281
Hubbell, S. P. (1997). A unified neutral theory of biogeography and relative species abundance and its application to tropical rain forests and coral reefs. Coral Reefs 16(Suppl.), S9–S21.
Johnson, W. S., and Allen, D. M. (2005). Zooplankton of the Atlantic and Gulf coasts: a guide to their identification and ecology. Baltimore: The Johns Hopkins University Press, 379.
Kiørboe, T. (2011). How zooplankton feed: mechanisms, traits and trade-offs. Biol. Rev. 86, 311–339. doi: 10.1111/j.1469-185X.2010.00148.x
Kiørboe, T., and Sabatini, M. (1995). Scaling of fecundity, growth and development in marine planktonic copepods. Mar. Ecol. Prog. Ser. 120, 285–298. doi: 10.3354/meps120285
Legendre, P., and Legendre, L. (1998). Numerical Ecology Developments in Environmental Modelling 20. Amsterdam: Elsevier Science.
Lopes, R. M. (2007). Marine zooplankton studies in Brazil: a brief evaluation and perspectives. Anais da Academia Brasileira de Ciências 79, 369–379. doi: 10.1590/s0001-37652007000300002
Lopes, R. M., Brandini, F. P., and Gaeta, S. A. (1999). Distribution patterns of epipelagic copepods off rio de janeiro (SE Brazil) in summer 1991/1992 and winter 1992. Hydrobiol 411, 161–174.
Mabesoone, J. M., and Coutinho, P. N. (1970). Littoral and shallow marine geology of the Northern and Northeastern Brazil. Trab. Oceanogr. Univ. Fed. PE. 12, 1–124.
Marcolin, C. R., Schultes, S., Jackson, G. A., and Lopes, R. M. (2013). Plankton and seston size spectra estimated by the LOPC and zooscan in the abrolhos bank ecosystem (SE Atlantic). Cont. Shelf Res. 70, 74–87. doi: 10.1016/j.csr.2013.09.022
Medeiros, C., Macêdo, S. J., Feitosa, F. A. N., and Koening, M. L. (1999). Hydrology and phytoplankton biomass of the Northeastern Brazilian Waters. Arch. Fish. Mar. Res. 47, 133–151.
Mee, L. (2012). Between the devil and the deep Blue Sea: the coastal zone in an era of globalisation. Est. Coast. Shelf Sci. 96, 1–8. doi: 10.1016/j.ecss.2010.02.013
Melo, P. A. M. C., Melo Júnior, M., Macedo, S. J., Araujo Filho, M., and Neumann-Leitão, S. (2014). Copepod distribution and production in a mid-atlantic ridge archipelago. An. Acad. Bras. Cienc. 86, 1719–1733. doi: 10.1590/0001-3765201420130395
Nakamura, Y., and Turner, J. T. (1997). Predation and respiration by the small cyclopoid copepod Oithona similis; How important is the feeding on ciliates and heterotrophic flagellates? J. Plankton Res. 19, 1275–1288. doi: 10.1093/plankt/19.9.1275
Neumann-Leitão, S., Gusmão, L. M. O., Silva, T. A., Nascimento-Vieira, D. A., and Silva, A. P. (1999). Mesozooplankton biomass and diversity in coastal and oceanic waters off North-Eastern Brazil. Arch. Fish. Mar. Res. 47, 153–165.
Neumann-Leitão, S., Melo, P. A. M. C., Schwamborn, R., Diaz, X. F. G., Figueiredo, L. G. P., Silva, A. P., et al. (2018). Zooplankton from a reef system under the influence of the Amazon River plume. Front. Microbiol. 9:355. doi: 10.3389/fmicb.2018.00355
Neumann-Leitão, S., Sant′Anna, E. M. E., Gusmão, L. M. O., Nascimento-Vieira, D. A., Paranaguá, M. N., and Schwamborn, R. (2008). Diversity and distribution of the mesozooplankton in the tropical Southwestern Atlantic. J. Plankton Res. 30, 795–805. doi: 10.1093/plankt/fbn040
Niebuhr, B. B. S., Wosniack, M. E., Santos, M. C., Raposo, E. P., Viswanathan, G. M., Luz, M. G. E., et al. (2015). Survival in patchy landscapes: the interplay between dispersal, habitat loss and fragmentation. Sci. Rep. 5:11898, doi: 10.1038/srep11898
O’Boyle, S., and Silke, J. (2010). A review of phytoplankton ecology in estuarine and coastal waters around Ireland. J. Plankton Res. 32, 99–118. doi: 10.1093/plankt/fbp097
O’Neil, J. M. (1998). The colonial cyanobacterium Trichodesmium as a physical and nutritional substrate for the harpacticoid copepod Macrosetella gracilis. J. Plankton Res. 20, 43–59. doi: 10.1093/plankt/20.1.43
O’Neil, J. M., and Roman, M. R. (1994). Ingestion of the cyanobacterium Trichodesmium spp. by pelagic harpacticoid copepods Macrosetella. Miracia Oculosetella. Hydrobiol. 29, 235–240. doi: 10.1007/978-94-017-1347-4_31
Peterson, W. T., Tiselius, P., and Kiørboe, T. (1991). Copepod egg production, mouting and growth rates, and secondary production, in the Skagerrak in August 1988. J. Plankton Res. 13, 131–154. doi: 10.1093/plankt/13.1.131
PETROBRAS (2005). Relatório final das Atividades do Projeto de Monitoramento Ambiental da Bacia Potiguar: Oceanografia Física. Brazil: Petrobras.
Pierrot-Bults, A. C., and Angel, M. V. (2012). Pelagic biodiversity and biogeography of the oceans. Biol. Internat. 51, 9–35.
Pingree, R. D., Holligan, P. M., Mardell, G. T., and Head, R. N. (1976). The influence of physical stability on spring, summer and autumn phytoplankton blooms in the Celtic Sea. J. Mar. Biol. Ass. U.K. 56, 845–873.
Pomerleau, C., Sastri, A. R., and Beisner, B. E. (2015). Evaluation of functional trait diversity for marine zooplankton communities in the Northeast subarctic Pacific Ocean. J. Plankton Res. 37, 712–726. doi: 10.1093/plankt/fbv045
Razouls, C., Bovée, F., Kouwenberg, J., and Desreumaux, N. (2005). Diversity and geographic distribution of marine planktonic copepods. Sorbonne University, CNRS. Available at htt://copepods.obs-banyuls.fr/en (accessed May 24, 2019).
Regner, D. (1985). Seasonal and multiannual dynamics of copepods in the middle Adriatic. Acta Adriatica 26, 11–99.
Ricklefs, R. I. E., and Schluter, D. (1993). Species Diversity in Ecological Communities: Historical and Geographical Perspectives. Chicago: University of Chicago Press, 414.
Ricklefs, R. E. (1987). Community diversity: relative roles of local and regional processes. Science 9, 167–171. doi: 10.1126/science.235.4785.167
Rohlf, F. J., and Fisher, D. L. (1968). Test for hierarchical structure in random data sets. Systemat. Zool. 17, 407–412. doi: 10.1093/sysbio/17.4.407
Santana, C. S., Schwamborn, R., Neumann-Leitão, S., Montes, M. J. F., and Lira, S. M. A. (2018). Spatio-temporal variation of planktonic decapods along the leeward coast of the fernando de noronha archipelago. Brazil. Braz. J. Oceanogr. 66, 1–14. doi: 10.1590/s1679-87592018147206601
Santos, J. R. (2010). Caracterização Morfodinâmica e Sedimentologia da Plataforma Continental Rasa na APA Estadual dos Recifes de Corais - RN. 2010. 85 f. Dissertação (Mestrado em Geodinâmica; Geofísica). Natal: Universidade Federal do Rio Grande do Norte.
Schott, F. A., Stramma, L., and Fischer, J. (1998). Transports and pathways of the upper layer circulation in the western tropical Atlantic. J. Phys. Oceanogr. 28, 1904–1928. doi: 10.1175/1520-0485 (1998)028<1904:tapotu>2.0.co;2
Schwamborn, R., Bonecker, S. L. C., Galvão, I. B., Silva, T. A., and Neumann-Leitão, S. (2004). Mesozooplankton grazing under conditions of extreme eutrophication in Guanabara Bay. Brazil. J. Plankton Res. 26, 983–992. doi: 10.1093/plankt/fbh090
Sheridan, C. C., Steinberg, D. K., and Kling, G. W. (2002). The microbial and metazoan community associated with colonies of Trichodesmium spp.: a quantitative survey. J. Plankton Res. 24, 913–922. doi: 10.1093/plankt/24.9.913
Silva, A. P., Neumann-Leitão, S., Schwamborn, R., Gusmão, L. M. O., and Silva, T. A. (2004). Mesozooplankton of an impacted bay in North Eastern Brazil. Braz. Arch. Biol. Tech. 47, 485–493. doi: 10.1590/s1516-89132004000300020
Silva, N. L., Marcolin, C. R., and Schwamborn, R. (2019). Using image analysis to assess the contributions of plankton and particles to tropical coastal ecosystems. Estuar. Coast. Shelf Sci. 219, 252–261. doi: 10.1016/j.ecss.2019.02.010
Smeti, H., Pagano, M., Menkès, C. E., Lebourges-Dhaussy, A., Hunt, B. P. V., Allain, V., et al. (2015). Spatial and temporal variability of zooplankton off New Caledonia (Southwestern Pacific) from acoustics and net measurements. J. Geophys. Res. 120, 2676–2700. doi: 10.1002/2014jc010441
Soares, U. M., Rosetti, E. L., and Cassab, C. T. (2003). Bacia potiguar. Phoenix. Fundação Paleozóica Phoenix 56, 1–13.
Sommer, U., and Sommer, F. (2006). Cladocerans versus copepods: the cause of contrasting top-down controls on freshwater and marine phytoplankton. Oecologia 147, 183–194. doi: 10.1007/s00442-005-0320-0
Sousa, L. P., Sousa, A. I., Alves, F. L., and Lillebø, A. I. (2016). Ecosystem services provided by a complex coastal region: challenges of classification and mapping. Sci. Rep. 6:22782
Stibor, H., Vadstein, O., Diehl, S., Gelzleichter, A., Hansen, T., Hantzsche, F., et al. (2004). Copepods act as a switch between alternative trophic cascades in marine pelagic food webs. Ecol. Lett. 7, 321–328. doi: 10.1111/j.1461-0248.2004.00580.x
Stramma, L., Ikeda, Y., and Peterson, R. G. (1990). Geostrophic transport in the Brazil current region north of 20o S. Deep Sea Res. 37, 1875–1886. doi: 10.1016/0198-0149(90)90083-8
Ter Braak, C. J., and Smilauer, F. P. (2002). CANOCO Reference Manual and CanoDraw for Windows user’s Guide: Software for Canonical Community Ordination (version 4.5). New York, YK: Microcomputer Power, 500.
Tosetto, E. G., Neumann-Leitão, S., and Nogueira Júnior, M. (2019). Sampling planktonic cnidarians with paired nets: implications of mesh size on community structure and abundance. Estuar. Coast. Shelf Sci. 220, 48–53. doi: 10.1016/j.ecss.2019.02.027
Trégouboff, G., and Rose, M. (1957). Manuel de Planctonologie Mediterranéenne. Paris: Centre Nacional de la Recherche Scientifique.
Tseng, L. C., Dahms, H. U., Hung, J. J., Chen, Q. C., and Hwang, J. S. (2011). Can different mesh sizes affect the results of copepod community studies? J. Exp. Mar. Biol. Ecol. 398, 47–55. doi: 10.1016/j.jembe.2010.12.007
Turner, J. T. (2004). The importance of small planktonic copepods and their roles in pelagic marine food webs. Zool. Stud. 43, 255–266.
Uye, S., Aoto, I., and Onbé, T. (2002). Seasonal population dynamics and production of Microsetella norvegica, a widely distributed but little-studied marine planktonic harpacticoid copepod. J. Plankton Res. 24, 143–153. doi: 10.1093/plankt/24.2.143
Walsh, J. J. (1976). Herbivory as a factor in patterns of nutrient utilization in the sea. Limnol. Oceanogr. 21, 1–13. doi: 10.4319/lo.1976.21.1.0001
Watson, J. R., Siegel, D. A., Kendall, B. E., Mitarai, S., Rassweiller, A., and Gaines, S. D. (2011). Identifying critical regions in small-world marine metapopulations. PNAS 108, 907–913. doi: 10.1073/pnas.1111461108
Williams, R., and Collins, N. R. (1986). Seasonal composition of meroplankton and holoplankton in the Bristol channel. Mar. Biol. 92, 93–101. doi: 10.1007/bf00392751
Williams, R., and Conway, D. V. P. (1982). Population growth and vertical distribution of Calanus helgolandicus in the Celtic Sea. Nether. J. Sea Res. 16, 185–194. doi: 10.1016/0077-7579(82)90029-1
Zar, J. H. (1996). Biostatistical Analysis, 3th Edn. Upper Saddle River, N.J: Prentice Hall International, 662.
Keywords: zooplankton, Copepoda, connectivity, functional traits, Southwestern Atlantic
Citation: Neumann Leitão S, Melo Junior Md, Porto Neto FF, Silva AP, Diaz XFG, Silva TA, Nascimento Vieira DA, Figueiredo LGP, Costa AESF, Santana JR, Campelo RPS, Melo PAMC, Pessoa VT, Lira SMA and Schwamborn R (2019) Connectivity Between Coastal and Oceanic Zooplankton From Rio Grande do Norte in the Tropical Western Atlantic. Front. Mar. Sci. 6:287. doi: 10.3389/fmars.2019.00287
Received: 15 January 2019; Accepted: 17 May 2019;
Published: 07 June 2019.
Edited by:
Gabriel Machovsky-Capuska, University of Sydney, AustraliaReviewed by:
Ulisses Miranda Azeiteiro, University of Aveiro, PortugalPetra H. Lenz, University of Hawaii at Manoa, United States
Copyright © 2019 Neumann Leitão, Melo Junior, Porto Neto, Silva, Diaz, Silva, Nascimento Vieira, Figueiredo, Costa, Santana, Campelo, Melo, Pessoa, Lira and Schwamborn. This is an open-access article distributed under the terms of the Creative Commons Attribution License (CC BY). The use, distribution or reproduction in other forums is permitted, provided the original author(s) and the copyright owner(s) are credited and that the original publication in this journal is cited, in accordance with accepted academic practice. No use, distribution or reproduction is permitted which does not comply with these terms.
*Correspondence: Sigrid Neumann Leitão, sigridnl@uol.com.br