Food in the Sea: Size Also Matters for Pelagic Fish
- 1MARBEC (University of Montpellier, Ifremer, CNRS, IRD), Séte, France
- 2Ifremer (Institut Francais de Recherche pour l’Exploitation de la MER), Palavas-les-Flots, France
Small pelagic fish are key components of marine ecosystems and fisheries worldwide. Despite the absence of recruitment failure and overfishing, pelagic fisheries have been in crisis for a decade in the Western Mediterranean Sea because of a marked decline in sardine size and condition. This situation most probably results from bottom-up control and changes in the plankton community toward smaller plankton. To understand such an unusual phenomenon, we developed an original and innovative experimental approach investigating the mechanisms induced by a reduction in the quantity and size of sardine prey. While experimentations offer the unique opportunity to integrate behavior and ecophysiology in understanding key demographic processes, they remain rarely used in fisheries science, even more so on small pelagics due to the notorious difficulty to handle them. The results revealed that food size (without any modification of its energy content) is as important as food quantity for body condition, growth and reserve lipids: sardines that fed on small particles had to consume twice as much as those feeding on large particles to achieve the same condition and growth. Such a strong impact of food size (based on 100 vs. 1200 μm pellets) was unexpected and may reflect a different energy cost or gain of two feeding behaviors, filter-feeding vs. particulate-feeding, which would have to be tested in further study. As increasing temperature favors planktonic chains of smaller size, climate change might actually accelerate and amplify such phenomenon and thus strongly affect fisheries.
Introduction
Small pelagic fish are a key component of marine ecosystems, modulating population dynamics of both lower and upper trophic levels, especially in highly productive marine upwelling systems (Cury et al., 2000; Frederiksen et al., 2006; Taylor et al., 2008; Brochier et al., 2011). Small pelagics also represent 25% of worldwide landings (in tons), predominantly through anchovy, sardinella, sardine, mackerel and herring (Food and Agriculture Organization [FAO], 2018). Fluctuations in populations of small pelagics can, therefore, have critical ecological, economic and social consequences, such that understanding their underlying mechanisms is extremely important. The population dynamics of these species could be strongly impacted by natural environmental fluctuations (bottom-up control) and mortality (top-down control) (Checkley et al., 2017). On the one hand, the crucial significance of plankton production and availability for recruitment dynamics of small pelagics has been known since seminal work by Hjort (1914), and is central to the “match-mismatch” and “ocean triad” hypotheses (Cushing, 1990; Bakun, 1996). On the other hand, (over)fishing has also been identified as a key factor in the collapse of several stocks, often in conjunction with environmental fluctuations (Toresen and Ostvedt, 2000), which may be exacerbated by ongoing global change (Shannon et al., 2009; Brochier et al., 2013). Nevertheless, the relative importance of bottom-up vs. top-down controls remains complicated to assess since they are often entangled (Hunt and McKinnell, 2006; Rouyer et al., 2014).
The Gulf of Lions is one of the most productive areas of the Mediterranean Sea due to wind-driven coastal upwelling, the western Mediterranean mesoscale circulation and fresh water inputs from the Rhone River (Millot, 1990; Petrenko et al., 2005). Until the mid-2000s, sardine (Sardina pilchardus) and anchovy (Engraulis encrasicolus) represented up to 50% of the total annual landings (around 15,000 tons) in the Gulf of Lions (Bǎnaru et al., 2013). Landings of sardines and anchovies have, however, decreased sharply since 2008, reaching the lowest levels recorded in 150 years (around 3,000 tons), although population abundance remains high (Van Beveren et al., 2016a; General Fisheries Commission for the Mediterranean [GFCM], 2017b; Saraux et al., 2019). Declines in landings of small pelagic fishes are typically a result of recruitment failure and/or overfishing (Schwartzlose et al., 1999). In the Gulf of Lions, however, the dramatic drop in catches has been due to a severe decrease in individual body size and condition (mean weight ± SE: 24.3 ± 0.3 g between 1993 and 2007 and 11.4 ± 0.2 g between 2008 and 2018), which has rendered the sardines and anchovies unattractive for fisheries (Van Beveren et al., 2014, 2016a; Saraux et al., 2019). The decrease in size of sardines is the combined outcome of lower growth and the disappearance, from the population, of the oldest and largest individuals (Van Beveren et al., 2014; Saraux et al., 2019). This situation was not due to recruitment failure and/or overfishing, which is, as mentioned above, unusual. The fishing pressure and selectivity were (and still are) very low and could not explain the disappearance of the large individuals (General Fisheries Commission for the Mediterranean [GFCM], 2017b). Sardine recruitment remained stable and even increased during the last decade in this area (Saraux et al., 2019). Recent studies further showed that this was neither due to emigration, top-down controls (natural predators) or diseases (Van Beveren et al., 2016b, 2017; Queiros et al., 2018; Saraux et al., 2019). The major remaining hypothesis is therefore bottom-up control, linked in some way to the quantity and quality of plankton production in the Gulf of Lions (Van Beveren et al., 2014; Brosset et al., 2015b; Saraux et al., 2019).
Sardines are both filter and particulate feeders, depending on the size of their prey and switch easily between these feeding modes (Garrido et al., 2007, 2008). In the Gulf of Lions, sardines feed on a size range of plankton ranging from 0.1 to 1.4 mm in length (Le Bourg et al., 2015). Interestingly, the diet of sardines has shifted from large size prey (with a high contribution of cladocerans > 1 mm) before 2008 to smaller prey (copepods < 1 mm suspected to be less nutritious) in the most recent years (Evjemo et al., 2003; Zarubin et al., 2014; Brosset et al., 2016). Thus, bottom-up control might explain the poor condition of sardines, with knock-on effects on survival rates of adults after reproduction (Brosset et al., 2016). This remains, however, to be validated (Saraux et al., 2019).
In this study, we developed an experimental approach to investigate the potential mechanisms for this bottom-up control on sardines. To investigate whether the changes we observed in the wild might derive from a change in prey, we studied the long-term consequences of feeding on different food sizes and quantities on individual body condition and growth through a 7-month experiment on captive adult sardines. While long-term experiments in captivity are rarely performed to inform on fisheries science, especially with difficult-to-maintain small pelagics, we believe that this is an important step to test for hypotheses and better understand the behavioral or ecophysiological mechanisms involved in natural processes. We coupled our experiment with laboratory analyses of physiological parameters, such as muscle constituents (lipids and proteins) and blood oxidative balance. This latter provides insight into levels of metabolism and plays a central role in aging processes by acting as a mediator in trade-offs between growth, maintenance and reproduction (Kirkwood and Rose, 1991; Finkel and Holbrook, 2000; Metcalfe and Alonso-Alvarez, 2010).
Materials and Methods
From Capture to Maintenance in Experimental Tanks
Sardines were captured in October 2016 (2 trips on the 10th and 18th of October) by a commercial purse-seiner operating from Sète (South of France). Fishing and acclimation procedures are detailed in Supporting Information.
Experimental Design
449 sardines were distributed homogeneously into 8 experimental tanks of 300 L each (56–57 sardines per tank), so that both the mean and the range in length and weight were comparable among tanks and treatments (Figure 1 and Table 1). Prior to transfer, sardines were anesthetized (benzocaine at 140 ppm), total body length and total wet weight recorded to the nearest 0.1 mm and the nearest 0.01 g, respectively. A tiny RFID tag (Biolog-id, Bernay, France, 0.03 g i.e., <0.2% of sardine lowest body mass) was implanted in the dorsal muscle using a specific injector and allowed individual identification. This procedure caused less than 1% mortality and did not affect their behavior. Tanks were supplied with water pumped directly from the sea and filtered through sand filter (30–40 μm). The photoperiod was adjusted each week to follow the natural cycle and sea water temperature was not controlled except to maintain a minimum of 10°C or a maximum of 25°C (Supplementary Figure S1). After 10 days of acclimation to these new tanks (sardines fed with the same pellet mix as the one used during acclimation but at 0.3% of the biomass), the experiment started on November 14, 2016 (t0) and continued until June 15, 2017 (reproduction period between November and March). Biometries were performed every 4 weeks, with all sardines measured individually (tag read, total body length, and body weight recorded). There was very rarely any mortality following the biometries. Relative body condition of each sardine was calculated with the Le Cren index Kn as estimated by Brosset et al. (2015a):
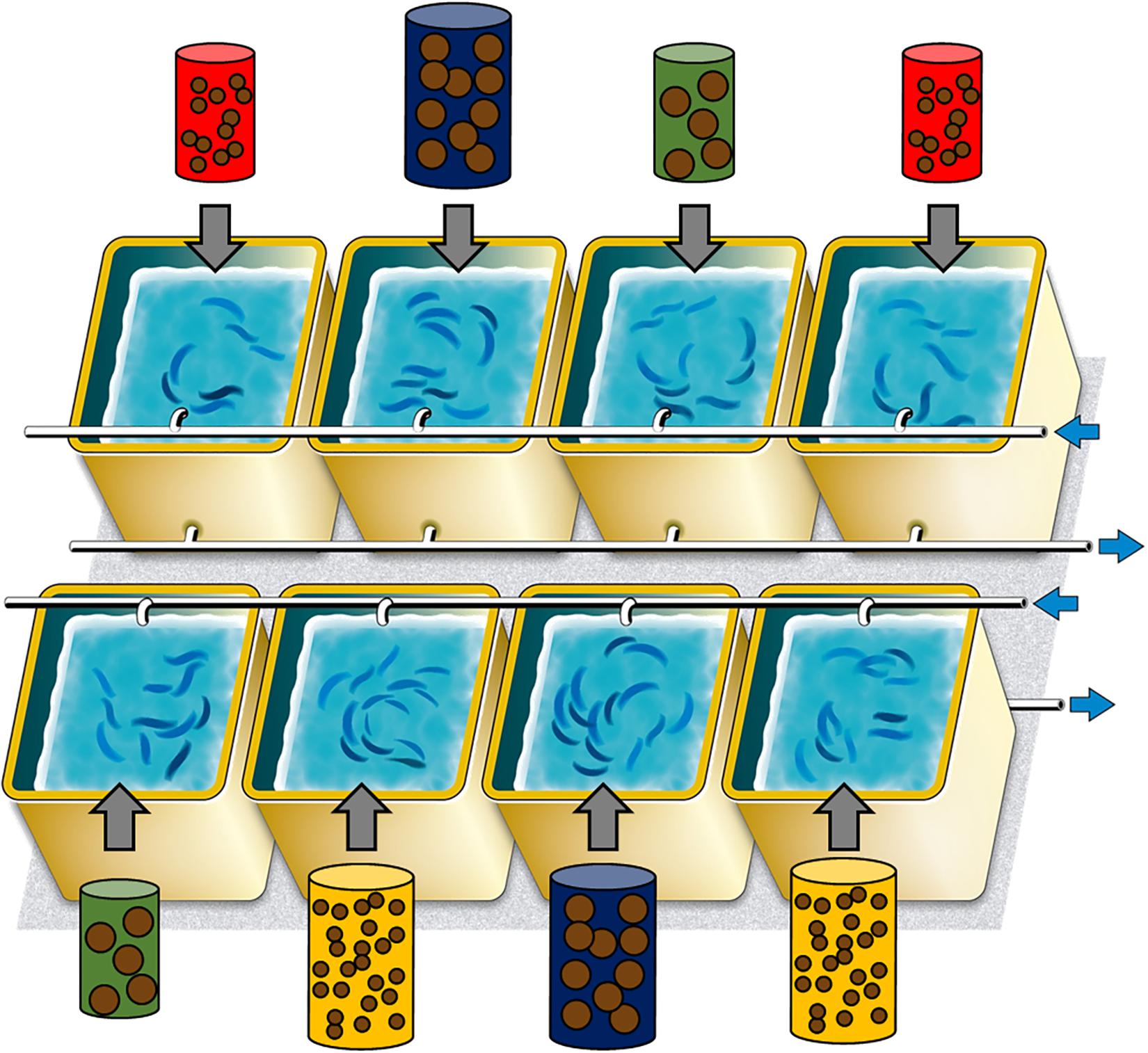
Figure 1. Experimental design to study the impact of food treatment, comprising the 8 tanks and their feeding treatment (red: pellet size of 0.1 mm and pellet quantity of 0.3%; green: 0.1 mm and 0.6%; yellow: 1.2 mm and 0.1% and blue: 1.2 mm and 0.6%). Blue arrows represented input and output sea water.
where WW the wet weight in g and TL is the total length in cm.
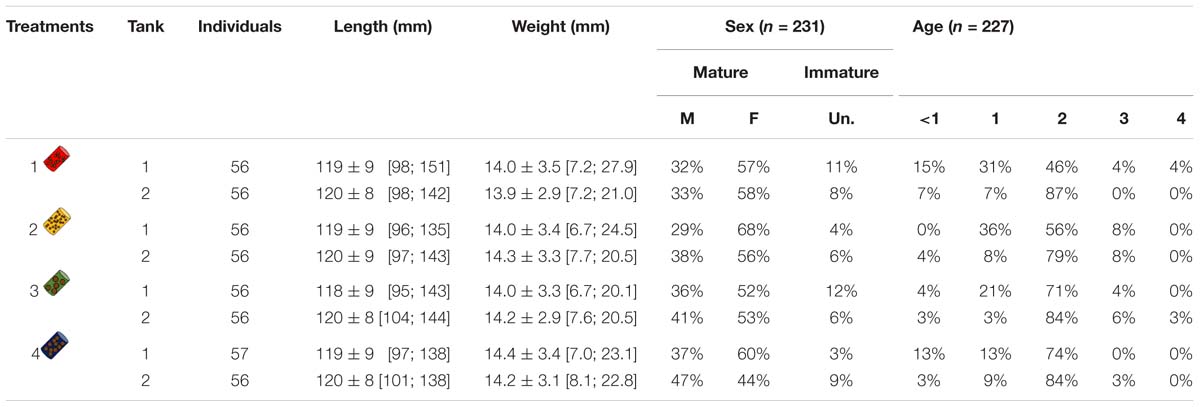
Table 1. Number of individuals, mean length (±SD) and length range (95% CI), mean weight (±SD) and weight range (95% CI), sex ratio (in %) of males (M), females (F) or unidentified (Un.), age class ratio (in %) for each tank and each treatment. Sex ratios and age class ratios are based on samples of March and June (sample size is given by n).
Feeding Conditions
For feeding conditions to be as realistic as possible, pellet quantities were selected to mimic weight gains measured in the wild under contrasting conditions (see Supplementary Figure S2 and Supplementary Movie S1). That is, we estimated daily weight gain in the two different periods of good (2005–2006) and bad conditions (after 2010) defined by Van Beveren et al. (2014), using data on weight and age of ∼24,000 individuals compiled by Brosset et al. (2015a). Assuming constant growth throughout the year, daily weight gain was estimated between two consecutive ages by:
where μage is the mean weight of sardines at a specific age. We found daily weight gain in the wild corresponded to 0.2 and 0.1% of their weight under good and bad conditions, respectively. Using preliminary tests in tanks, these daily weight gains corresponded to daily feeding rates of 0.6 and 0.3% of the total biomass of fish, respectively.
Stomach content analyses indicated that Gulf of Lions sardines were feeding on larger prey during the period of good conditions and smaller ones during poor conditions (Le Bourg et al., 2015; Brosset et al., 2016), so we also tested for the impact of food size. Ideally, experiments should have been conducted on a continuous range of pellets size, e.g., 100, 300, 600, 900, and 1200 μm, which was, unfortunately, infeasible for several logistic and cost reasons, so that a choice had to be made. Our goal was then to compare two contrasting food sizes within the natural range of sardine diet, which elicited two different feeding modes (i.e., filtering vs. catching). In the Gulf of Lions, prey sizes exhibited a bimodal distribution: between 100 and 600 μm and around 1200 μm (Le Bourg et al., 2015). Further, prey smaller than 200 μm could represent until 88% of the numerical contribution of prey to their diet (Nikolioudakis et al., 2012). Finally, sardines are known to switch feeding behaviors depending on prey size; filter-feeding for small prey but particulate-feeding for larger prey (Garrido et al., 2007). The change in their feeding behavior was observed under 300 μm in the Mediterranean Sea (pers. comm.). According to the range of prey size in their diet (Le Bourg et al., 2015), the effects of food size were tested using two contrasting pellet sizes: 0.1 mm (actually ranging between 80 and 250 μm) and 1.2 mm (ranging between 900 and 1500 μm) to avoid any overlap in prey size distributions while ensuring a shift in feeding modes (i.e., filtering vs. catching).
The combination of two food sizes (0.1 and 1.2 mm) and two quantities (0.3 and 0.6% of the total fish mass in tanks) resulted in four treatments with two replicas each (Figure 1): (i) small size and small quantity (0.1 mm and 0.3%, represented in red in the following – treatment 1), (ii) small size and large quantity (0.1 mm and 0.6%, in yellow – treatment 2), (iii) large size and small quantity (1.2 mm and 0.3%, in green – treatment 3), (iv) large size and large quantity (1.2 mm and 0.6%, in blue – treatment 4). Both sizes of pellet had similar proximate compositions, being 62 and 57% of proteins and 14 and 12% of lipids, for 0.1 and 1.2 mm pellets, respectively (lipid class contents are also given in the Supplementary Table S1). Sardines were fed four times a day, at the same time, to limit non-ingested food. The total biomass of each tank was estimated weekly (based on linear mass gain between monthly biometries) to re-adjust food intake to account for mass gain.
Estimation of Food Loss
To quantify loss of non-ingested food, we performed an additional 2-week experiment described in the Supplementary Figure S3.
Biochemical Analyses
The physiological consequences of the diets were further investigated by measuring both lipid and protein content of dorsal epaxial muscle and blood oxidative stress status (d-ROMs and OXY).
Samplings were done at two different times: (i) on the 15th of March 2017 at the mid-experiment (15 sardines per tank) and (ii) on the 15th of June 2017 at the end of the experiment (depending on survival rate, but at least 20 sardines per treatment). Sampled sardines were selected by random draws according to normal distributions fitted on body mass within each tank and anesthetized by benzocaine at 140 ppm. Blood was collected from the caudal vein with a 26G needle into a heparinized syringe and transferred to 1.5 mL Eppendorf tubes. Blood samples were centrifuged at 3000 g for 10 min at 4°C to separate plasma, which was collected and stored at −80°C until further analyses. Sardines were then sacrificed by a lethal dose of benzocaine at 1000 ppm. Sex and maturity were determined by gonad observation and sagittal otolith pairs were removed from their otic cavity and read to estimate age (International Council for the Exploration of the Sea [ICES], 2011). This showed that sex, maturity and ages were comparable among tanks and treatments (Table 1). Portions of dorsal epaxial muscle were removed, frozen in liquid nitrogen and stored at −80°C for later lipid and protein content analyses.
Muscle Energy Resources
The energy status was first examined by analyzing lipid and protein content of dorsal muscle. The content of each lipid class was measured in muscle as described by Brosset et al. (2015a). Structural lipids were assumed to comprise phospholipids (PL), sterols (ST), acetone-mobile polar lipids (AMPL), and alcohols (ALC) whereas reserve lipids comprised triacylglycerols (TAG), diacylglycerols (DAG as precursors of TAG), and free fatty acids (FFA) (Zhol et al., 1995; Tocher, 2003; Moltschaniwskyj and Johnston, 2006; Lloret et al., 2013). Proportions of free fatty acids (FFA) were checked to ensure that lipids had not been degraded during sample conservation.
Portions of the same samples were lyophilized and grinded using a ball mill (MM400, Retsch GmbH, Germany). 10 mg of dry muscle powder was then immersed in 1.5 mL of a 10% SDS (Sigma Aldrich, France), 1.5% Protease inhibitor Cocktail (cOmplete, Sigma Aldrich, France), miliQ water solution (lysis solution adapted from Campus et al., 2010) and subjected to four cycles of 15 min in a ultrasonic bath (300 Ultrasonik, Ney Company, United States) alternatively with 3 min vortex. Extracts were then clarified for 10 min at 3000 g at 4°C, and protein content of 25 μL of the upper liquid fraction was quantified by the BCA method (Pierce, Thermo Fisher Scientific, France). Intra- and inter-plate protein variations (based on the same sample repeated over plates) were 6.4 and 16.7% respectively.
Sample sizes associated with these tests varied depending on the amount of muscle in each tissue sample and are indicated in each figure.
Oxidative Stress Balance
Reactive oxygen metabolites (d-ROMs) and plasma antioxidant defense (OXY) were estimated in plasma using the d-ROMs test and the OXY Adsorbent test, respectively (Diacron International©, Grosseto, Italy) in accordance with Costantini and Dell’Omo (2006). Experimental protocols were modified such as (i) each well of 96-wells microplate was filled with 8 μL of serum for the d-ROMs estimation; (ii) 4 μL of serum was diluted 1:100 for OXY estimation; and (iii) samples were duplicated when possible (depending on available plasma). After incubation, absorbance was measured at 555 nm by microplate automatic reader. The oxidant ability (d-ROMs) was expressed in mg of H2O2 equivalent L−1 and the antioxidant defense capacity (OXY) in μmol HClO mL−1. Intra- and inter-plate variations were 4.5 and 3.1% respectively for d-ROMs estimations, and 9.4 and 7.7% respectively for OXY estimations. Sample sizes associated with these tests varied due to plasma quantity and some sample loss due to hemolysis and are indicated in all figures.
Data Analyses
Mixed-effects models were applied to test for the impact of the different treatments on body condition and length. As body condition index and length distributions on November 2016 were approximated by normal distributions, we built a series of linear mixed effect models where body condition or length were dependent on two fixed effects: time (months) and food treatment, as well as their interaction. Because of variability among individuals and/or tanks within each treatment, we also introduced a random individual intercept effect (to take into account variations in individual condition/length), a random individual slope effect (to take into account variations among individual slopes in condition/length through time) and a random tank intercept effect (to take into account variations between tanks). We applied the model procedure recommended by Zuur et al. (2009) and the selection of the final model was done using the AIC criterion (Burnham and Anderson, 2002). Body composition and oxidative stress differences between treatments (for the same month) were investigated using parametric (one-way ANOVA) or non-parametric (Kruskal–Wallis) tests and associated post-hoc (Tukey or Dunn test), depending on residual normality and homoscedasticity. Finally, a Principal Component Analysis (PCA) was performed using the individual sardines as objects and terminal body condition index, total length, lipid and protein contents, d-ROMs and OXY as descriptors to summarize all the information and describe the relationships between descriptors.
All statistical analyses were performed in R (R Core Team, 2018) using the FactoMineR (Lê et al., 2008), the FSA (Ogle, 2018), the nlme (Pinheiro et al., 2018), the lsmeans (Lenth, 2016), and the factoextra (Kassambara and Mundt, 2017) packages.
Results
Among the 449 individuals distributed within the 8 tanks, length and weight initially varied between 95 and 151 mm and between 6.7 and 27.9 g, respectively (Figure 1 and Table 1). Females were in general more abundant (56% F, 37% M, and 7% unknown), while age 2 was the dominant age (Table 1).
Estimation of the non-ingested food was low to very low in all experiments; the mean ( ± SD) food loss was 0.3% (±0.6%) and 1.2% (±1.1%) for the 1.2 and 0.1 mm pellets, respectively.
Body Condition and Total Length
Treatment 4 led to the highest body condition and total length at the end of experimental design, whereas treatment 1 led to the lowest ones (Figure 2). Body condition and total length exhibited similar dynamics through time in treatments 2 and 3, and were intermediate compared to treatments 1 and 4 (Figure 2).
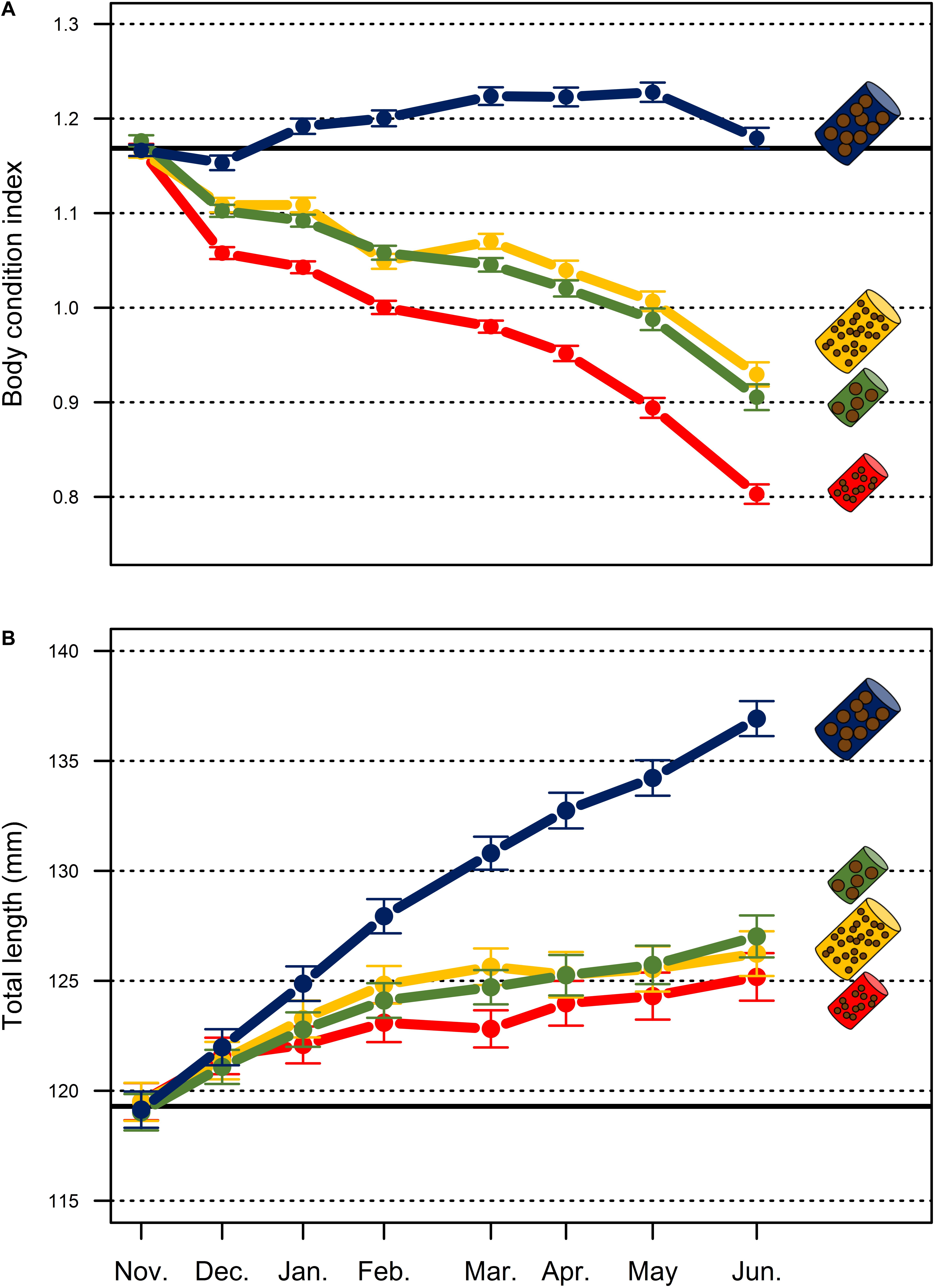
Figure 2. Time series of the mean (±se) body condition (A) and total length (B) of all sardines in each feeding treatment: red: pellet size of 0.1 mm and pellet quantity of 0.3%; green: 0.1 mm and 0.6%; yellow: 1.2 mm and 0.1% and blue: 1.2 mm and 0.6%. Black lines represent the mean body condition and total length at the beginning of the experiments.
The best linear mixed-effect model (Supplementary Figure S4) explaining both body condition and total length, as selected based on the AIC criterion, both included fixed effects (dates, treatments, and their interaction) as well as the random slope and intercept effects for individuals. The random tank effect was never retained in any selection, suggesting no difference between the 2 tanks of the same treatment for body condition and length over time. The diagnostic plots of the final models were satisfactory and residuals were mostly normally distributed (see Supplementary Figures S5, S6). Treatment effects were significant in the body condition model (p ≤ 0.05) but not in the total length model (p > 0.05, see Supplementary Tables S2, S3). Slope of body condition and total length over time exhibited significant differences between all treatments except for intermediate treatments 2 and 3. Body condition of all treatments decreased over time, except treatment 4 (large quantity of large pellets) at +0.01 month−1. The steepest decrease occurred in treatment 1, at −0.04 month−1 (Supplementary Table S2). Sardines from treatment 4 grew at a rate of 2.5 mm month−1, twice as fast as treatments 2 and 3 (1.2 and 1.0 mm month−1, respectively) and 5 times faster than treatment 1 (0.5 mm month−1) (Supplementary Table S3).
For the same food quantity (in terms of mass), sardines that fed on large pellet size showed higher body condition and grew twice as much as those feeding on small pellets (Figure 2 and Supplementary Tables S2, S3). Similarly, for the same pellet size, sardines that fed on large quantity exhibited higher body condition and grew 2.5 times higher than those feeding on low quantity (Figure 2 and Supplementary Tables S2, S3). Furthermore, body condition and growth of sardines that fed on large quantity of small pellets were similar to those that fed on low quantity of large pellets.
Muscle Constituents
Reserve lipids for treatment 4 were 2 to 5 times higher than those of the three other treatments, which did not differ significantly (p > 0.05) in both March and June (Figure 3A; see Supplementary Table S4). By contrast, structural lipids were similar among treatments in March and June (p > 0.05, Figure 3B; see Supplementary Table S4), Note, however, that the medians exhibited a positive trend in March according to size and quantity of food (Figure 3B). Protein content exhibited no significant difference between treatments in either March or June (p > 0.05), but showed the same pattern as structural lipids in March (Figure 3C; see Supplementary Table S4).
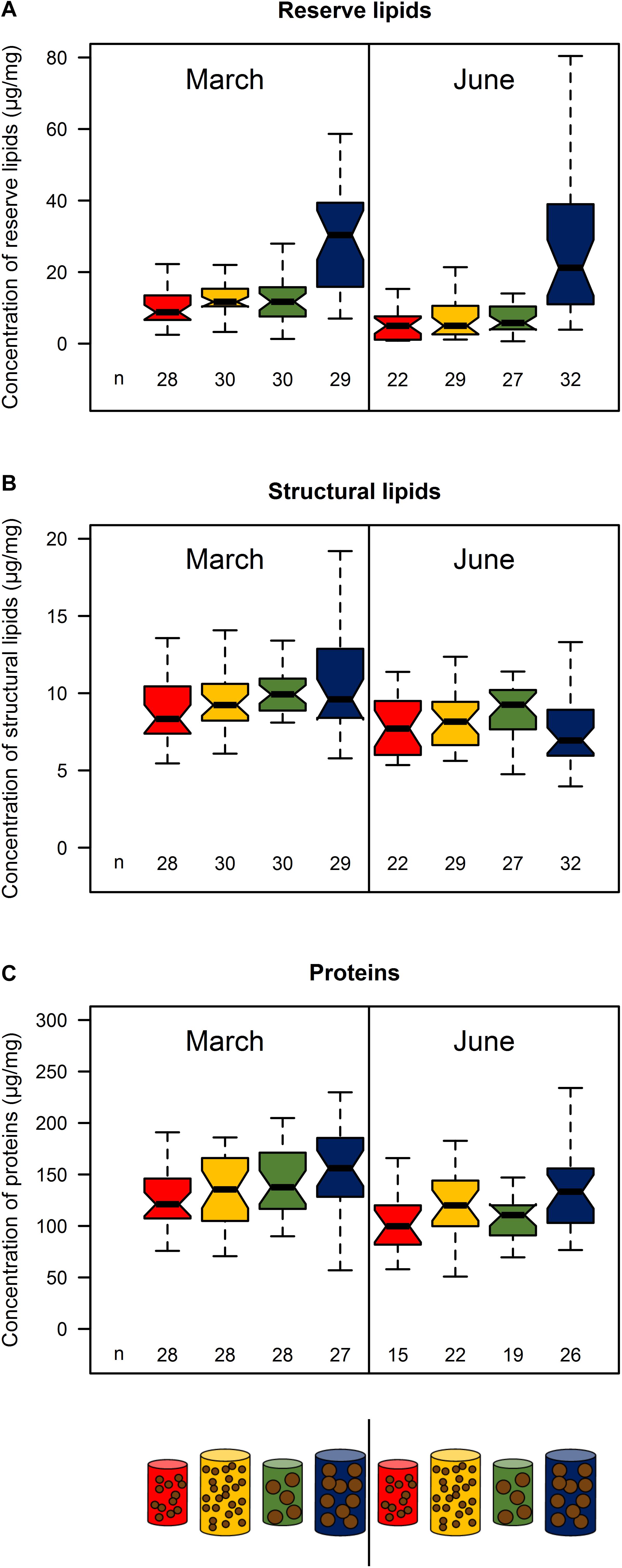
Figure 3. Reserve lipids (A), structural lipids (B), and proteins (C) of sardine muscle from the four feeding treatments (red: pellet size of 0.1 mm and pellet quantity 0.3%; green: 0.1 mm and 0.6%; yellow: 1.2 mm and 0.1% and blue: 1.2 mm and 0.6%) in March and June 2017. Concentrations are given relative to muscle wet weight. Sample size for each treatment is given by n below the boxes. Boxplots are presented without outliers for clarity purposes.
Oxidative Stress Balance
In March, there were no significant differences in d-ROMs and OXY among treatments (p > 0.05, Figure 4; see Supplementary Table S4). In June, there were increasing levels of d-ROMs and OXY with food size and quantity: treatment 1 exhibited the lowest values, treatments 2 and 3 intermediate ones and treatment 4 the highest (Figure 4). Only d-ROMs concentrations were significantly different between treatments 1 and 4 (medians: 20.5 and 61.5 mg of H2O2 equivalent L−1 for treatments 1 and 4, respectively) (Figure 4; see Supplementary Table S4).
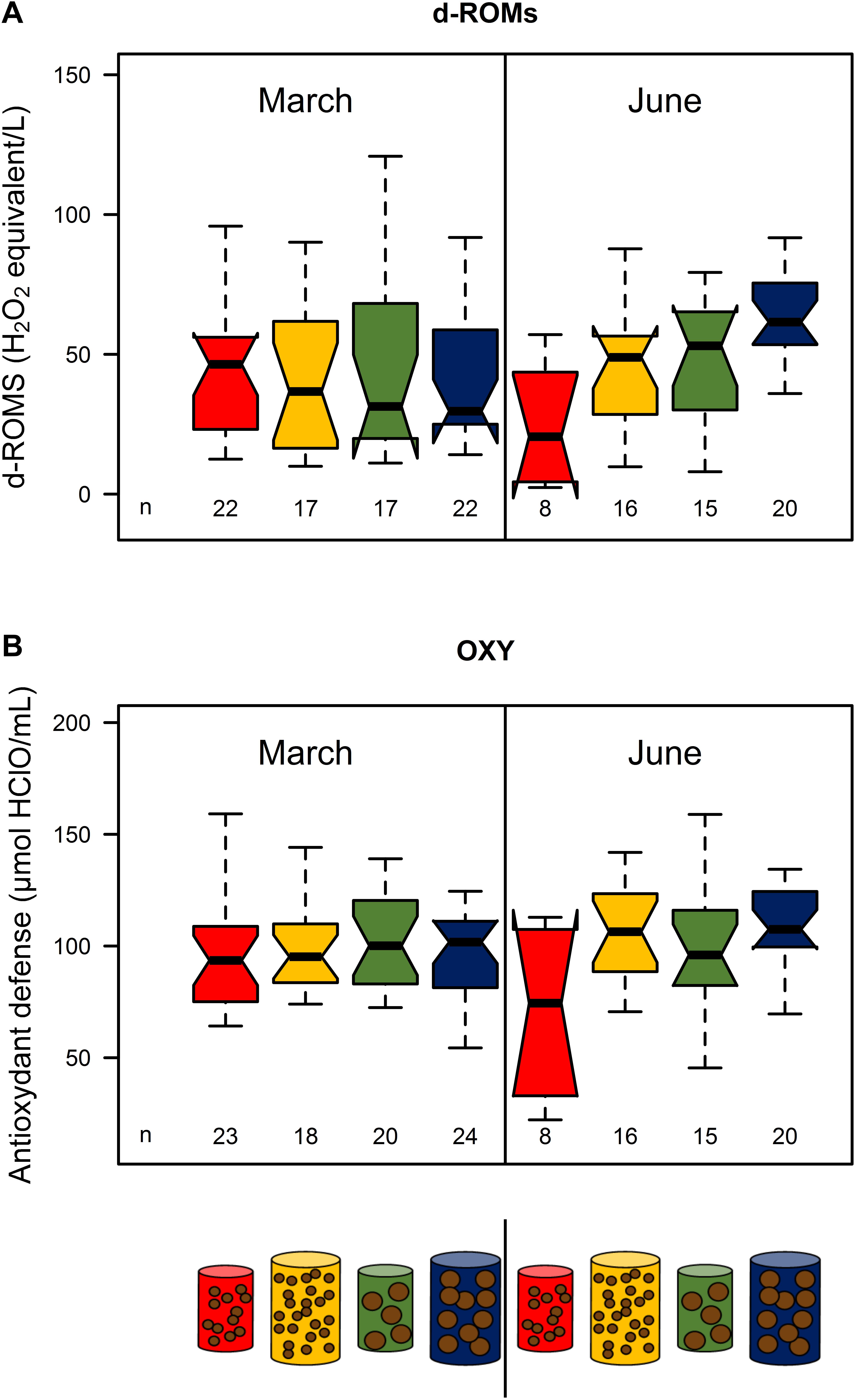
Figure 4. d-ROMs (A) and OXY (B) of the four feeding treatments (red: pellet size of 0.1 mm and pellet quantity 0.3%; green: 0.1 mm and 0.6%; yellow: 1.2 mm and 0.1% and blue: 1.2 mm and 0.6%) in March and June 2017. Sample size for each treatment is given by n below the boxes. Boxplots are presented without outliers for clarity purposes.
Variable Covariation
The first 2 components of the PCA explained 48.5% of the total variance observed. The main contributing variables to the first axis were body condition, reserve lipids and total length, whereas d-ROMs and OXY were the two main contributors to the second axis. We super-imposed the treatment for each individual and the projection of the barycenters showed a clear separation between treatment 4 and the three other treatments, especially on the first axis (Figure 5). While the PCA therefore confirms all the above results, it also revealed rather marked individual variation within each treatment, especially treatment 1.
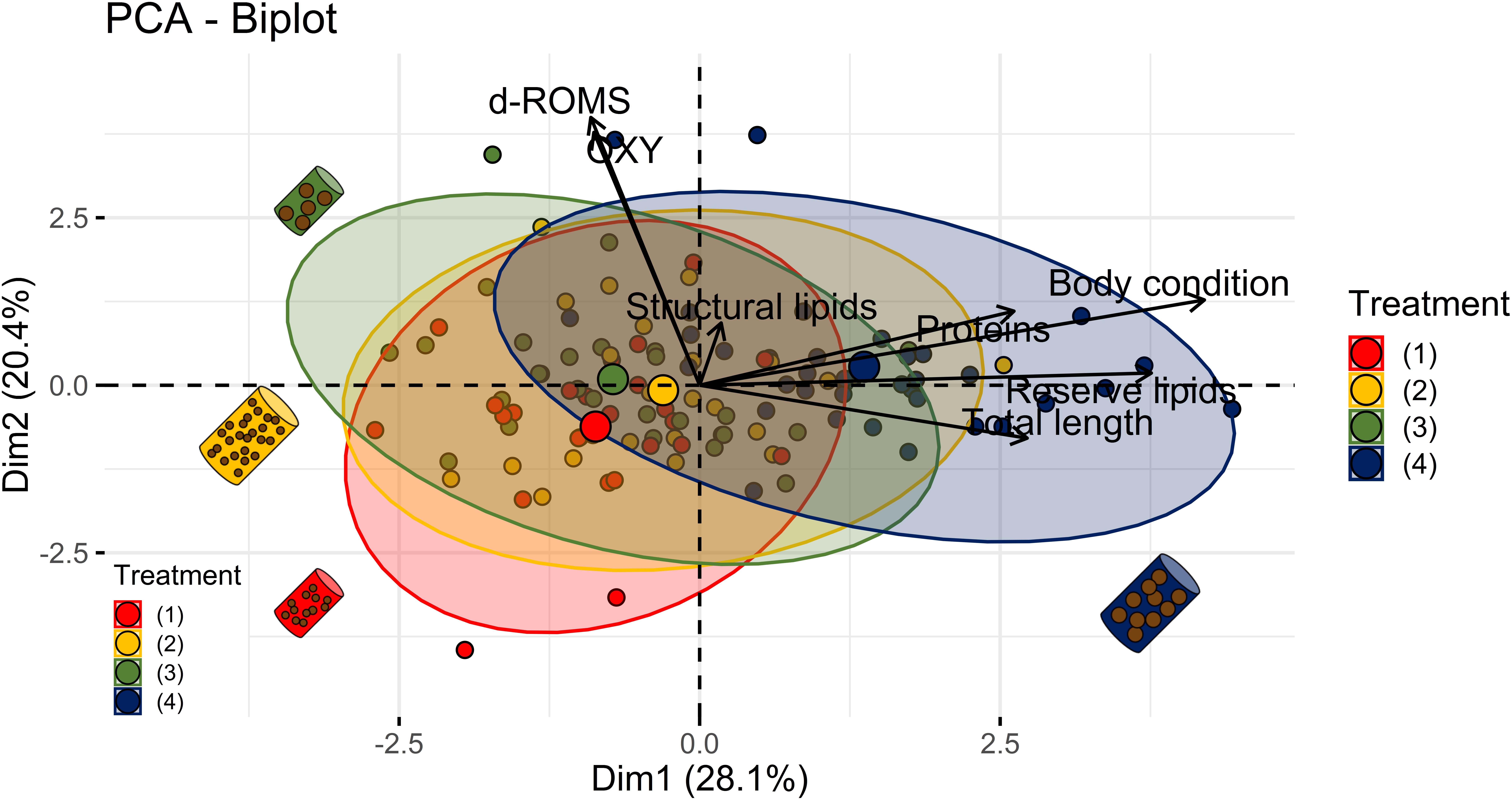
Figure 5. Biplot of the PCA built using body condition, total length, reserve and structural lipids and proteins contents, d-ROMs and OXY as explanatory variables. Ellipses correspond to 95% confidence level for each treatment. The large circles represent the barycenter of the individuals for a given treatment.
Discussion
Experimentation on marine fish species are often performed in a context of aquaculture and fundamental biology, but more rarely in applied and fisheries ecology. Nonetheless, controlled experimentation is one of the most useful approach for studying population dynamics (Hunt and McKinnell, 2006). Here, we developed a novel experimental approach on sardines, a species that is notoriously difficult to maintain and handle. We performed a long-lasting experiment (7 months) on a large number of adult sardines (449 individuals) to validate a specific bottom-up control hypothesis: does food size significantly affect the growth, condition and physiological status of sardines?
Size and Quantity Effects
Sardines use particulate feeding on large prey but rely on filter-feeding to capture small prey (Garrido et al., 2008). Both sardine and anchovy are able to switch between the two feeding modes, but the well-developed gill raker apparatus of sardines (larger and less spaced gill rakers than anchovies (Engraulis encrasicolus) and denticles on their gill rakers) provides for higher filtration capacity than anchovies (Rykaczewski, 2009; Collard et al., 2017). As a result, in upwelling systems the communities of small pelagics are often dominated by sardines when prey size falls (van der Lingen, 1994; Schwartzlose et al., 1999; Chavez et al., 2003). In light of this, the reduction of planktonic prey sizes in the Gulf of Lions (Brosset et al., 2016) was expected to be less detrimental for sardines than anchovies. Surprisingly, sardine populations are in a more alarming situation than anchovies, with more striking declines in body condition, growth and adult survival (see Van Beveren et al., 2014). Our experimental results confirmed the detrimental effect of small prey for sardines of the Mediterranean Sea. To achieve the same growth and body condition, sardines fed on small pellets had to eat twice as much as those feeding on large pellets. Additionally, sardines had to eat large quantity of large pellets in order to store reserve lipids. Knowing that the amount of food ingested was similar (i.e., there was no loss of food in the experiments), how could size have such a marked effect on growth, condition and reserve lipids? It is possible that food is assimilated differently and sardines gain less energy from the smaller particles. Retention efficiency of Atlantic sardines would be estimated at around 70% for 0.1 mm pellets in the filtration mode (using equation in Garrido et al., 2007) against 100% for large pellets in particulate mode. This could be even worse in Mediterranean sardines, which appear to have lower numbers and densities of gill rakers (for a given body length) than their Atlantic relatives (Costalago et al., 2015) suggesting they might be less adapted to catch small prey. However, an ongoing study of the density of the gill rakers indicated that the filtering efficiency of sardines in the Gulf of Lions may actually be higher than those of the Atlantic sardines nowadays owing to higher gill raker density (unpublished data). Thus, current Mediterranean sardines are likely to catch small prey such as 100 μm prey, even if they correspond to the lower range of sardine prey sizes. It is also possible that they derive the same energy but spend more, because the filter-feeding mode has high energetic costs. This would have to be investigated directly but information from the literature and our own observations provide support for some speculation. In this study, the two food sizes elicited a marked and visible change in sardine feeding behavior, from particulate feeding (1.2 mm) to filter feeding (0.1 mm). Feeding duration seemed to be about 10 times longer for filtration than particulate feeding (up to 20 min for filtration against 2 min for particulate). The energy requirements of sustained aerobic swimming during filter-feeding might be significantly greater than those of rapid bouts of swimming to capture particles (Costalago and Palomera, 2014). Metabolic costs of the filter-feeding mode were higher than those of particulate feeding for the Cape anchovy Engraulis capensis (James and Probyn, 1989), but the reverse was observed for the pilchard Sardinops sagax (van der Lingen, 1995). In the absence of coherent conclusions on other small pelagic species, we can only hypothesize for Mediterranean sardines that filter feeding may provide lower net gain (so constraining energy allocation to growth and reserves, as was observed in the present study). Nonetheless, this hypothesis remains to be validated by oxygen consumption experiments.
An alternative hypothesis to explain changes in body condition and growth is associated to different energetic and nutritional needs depending on maturity processes and/or engagement in gametogenesis. However, 93% of the fish in our experiment were mature discarding maturity processes as an explanation. Further, our experiment lasted 7 months encompassing both the reproduction period and the period following reproduction and the differences in growth and condition between treatments were observed and of similar importance in both periods, indicating that our results were not biased by potential differences in nutritional needs due to maturity or reproduction.
Structural lipids, proteins and the blood oxidative balance (d-ROMs and OXY) were less or not affected by the feeding treatments compared to body condition, growth and reserve lipids. The fact that structural lipid and protein contents remained steady across treatments suggests that diet provided enough energy for individuals to maintain the basal energetic costs of their structure. The increase in d-ROM levels for treatments 2, 3, and 4 between March and June suggests that individuals might pay short-term costs when displaying higher growth rates (Monaghan et al., 2009). Similar patterns have been shown in species facing dietary restriction during early development and displaying important compensatory growth later in life when food abundance was retrieved (Metcalfe and Monaghan, 2001). OXY levels followed similar trends to a lesser extent suggesting sardines from treatment 4 were not completely able to buffer d-ROM increase leading to oxidative stress (imbalance toward d-ROMs). On the other hand, treatment 1 displayed lower level of both d-ROMs and OXY, suggesting that sardines might cope with “poor feeding conditions” by lowering their metabolic rates to limit their living costs (O’Connor et al., 2000). Furthermore, starvation is suggested to have negative consequences on immune functions, although, such effects seem to differ between species; starvation has no or positive effect on immune responses in pacu (Gimbo et al., 2015), European eel (Caruso et al., 2010) and Chinese Sturgeon (Feng et al., 2011) but decreases the ability to cope with infection in Atlantic salmon (Martin et al., 2010) and Jade perch (Luo et al., 2013). Whether such relationship is found in sardines facing “poor feeding condition” remains to be explored, but it raises concern on the possible additive effect of long-term fasting on individual survival through immune functions impairment.
Sardines are known to be primarily capital breeders (Ganias, 2009; McBride et al., 2015); they store energy during summer and use reserves to invest in breeding during winter. The fact that energetic reserves were so impacted by both food size and quantity may be a cause of significant mortality of the oldest individuals after reproduction: a low reserve storage at the beginning of the reproductive period would impose a trade-off between survival and reproduction, in favor for the latter in oldest sardines (Brosset et al., 2015b; Saraux et al., 2019). Furthermore, seasonal fluctuations of plankton (Costalago et al., 2012; Nikolioudakis et al., 2012) could act synergistically to these declines and punctually reduce even more sardine condition as observed during the winter reproduction period in the Gulf of Lions (Brosset et al., 2015b). Such a difference in reproductive seasonality with income breeder anchovies might help explaining why sardine condition and size have decreased even more than anchovy’s.
Finally, the PCA while confirming previous results with treatment 4 easily distinguishable from all others also showed a high variability within each treatment. Such interindividual variability might result from differences in coping abilities under adverse conditions or from competition. For a given quantity of food, the number of pellets was much lower when using large pellets than small ones, thus generating a potential source of competition. As such, it is interesting to note that particulate-feeding on 1.2 mm pellets did not trigger higher competition as the CV was minimal for fish fed of large pellets in large quantities (treatment 4 – blue) both in terms of body condition and length and fish fed with large pellets in small quantities (treatment 3 – green) displayed a lower CV than fish fed on small particles for length and a similar one for body condition (see Supplementary Figure S7). Rather, the increase in length or condition variability seemed linked to an increase in interindividual differences when facing adverse conditions. The PCA first two components were less affected by sex or age (Supplementary Figures S8, S9). Furthermore, the inter-individual variability observed in March in all physiological variables was similar to the one observed in June, when fish were out of the reproduction season and their maturity levels all equal to 1 (e.g., in Figures 3, 4), suggesting that their reproductive status was not the source of interindividual variability. Interindividual variability might thus translate differences in other endogenous variables, often viewed as “individual quality” despite the ambiguity of this term (Wilson and Nussey, 2010).
Amplifying Factors and Their Consequences
Although we have demonstrated that smaller prey size (as of 0.1 mm) can act as a key factor in limiting sardine growth, this raises the question of why planktonic prey sizes are falling in the Gulf of Lions. The reduction in prey size might result from a change in community, with implications that extend beyond size to include nutritional quality (Zarubin et al., 2014). In the present study, the composition of both sizes of aquaculture pellets was similar but a reduction in energetic value of smaller prey would presumably exacerbate the effects we have revealed. Oceanographic modifications, induced by global warming, coupled with pollution, are two potential candidates that may explain reduced prey sizes for sardines. Up to 77% of total marine pollution is from terrestrial sources (Williams, 1996) and contaminants such as pesticides, fertilizers, heavy metals and synthetic organic chemicals are all known to affect plankton communities, reducing their diversity and causing irreversible changes in marine ecosystems (Shahidul Islam and Tanaka, 2004; Pérez et al., 2011; Smith et al., 2018). For example, the insecticide Malathion and the herbicide Roundup®significantly decreased copepod abundance, but favored smaller and lower energetic zooplankton (Geyer et al., 2016; Smith et al., 2018). Even in low concentrations, mixture of pesticides had similar effects (Relyea, 2009). Global warming enhances stratification of the water column in the Mediterranean (Coma et al., 2009), which seems to favor population dynamics of small phytoplankton and zooplankton (van der Lingen et al., 2006). The combination of pollution and global change might, therefore, result in a long-lasting domination of small plankton in the Mediterranean Sea, which could be extremely detrimental to sardine populations in the Gulf of Lions, in terms of fish growth and population demography. More research and more high-resolution observations on the plankton compartment of the Gulf of Lions are needed to assess potential changes in species composition that have affected energy flows through the pelagic community. If this is confirmed, then oceanographic, atmospheric, hydrological parameters and pollutant concentration should be further analyzed to identify the origins of this potential ecosystem regime shift.
Finally, the consequences of such a change in small pelagic populations might have further important repercussions for fisheries and entire ecosystem function. As stated earlier, there has been a major decline in sardine landings in the French Mediterranean fisheries. Fisheries have therefore turned toward other stocks, such as the European hake (Merluccius merluccius), which has been overexploited for decades (General Fisheries Commission for the Mediterranean [GFCM], 2017a). Top predators such as large tunas, sharks, marine mammals and birds could suffer from a potential long-term collapse of small pelagic populations (Shannon et al., 2009). In summary, long-term changes in the marine pelagic ecosystem of the Gulf of Lions may lead to critical economic, societal and ecological situations. Sardines may display plasticity or adaptation to cope with smaller prey but this remains to be investigated.
Experimentation on small pelagics is rarely exploited to study ecosystem dynamics, but essential information on trophic controls can be derived from such studies. Our study provides evidence that food size matters as much as food quantity for sardines, which might have repercussions on the entire ecosystem. As increasing temperature favors planktonic chains of smaller size (van der Lingen et al., 2006), climate change might actually accelerate and amplify such phenomenon and thus strongly affect fisheries worldwide.
Ethics Statement
All procedures were in accordance with the French and the EU legislation regarding animal experimentation (APAFIS, Permission No. 7097-2016093008412692).
Author Contributions
QQ, J-MF, EG, GD, CH, DM, and CS designed and carried out the experimentation. LM and LL analyzed the lipid and protein contents of the muscle. QQ and QS analyzed the d-ROMs and OXY levels in the blood. QQ, J-MF, and CS analyzed the data. QQ, J-MF, CS, QS, and DM wrote and reviewed the manuscript.
Funding
This study was funded through the MONALISA project, financed by the European Union and the French Ministry of Agriculture through the European Maritime and Fisheries Fund (EMFF) as well as the France Filière Pêche. QQ acknowledges doctoral fellowship support from the French Ministry of Higher Education of Research and Innovation.
Conflict of Interest Statement
The authors declare that the research was conducted in the absence of any commercial or financial relationships that could be construed as a potential conflict of interest.
Acknowledgments
The authors express their thanks to the IPHC team (Institut Pluridisciplinaire Hubert Curien) for their welcome and access to their laboratories for biochemical analyses.
Supplementary Material
The Supplementary Material for this article can be found online at: https://www.frontiersin.org/articles/10.3389/fmars.2019.00385/full#supplementary-material
References
Bakun, A. (1996). Patterns in the Ocean: Ocean Processes and Marine Population Dynamics. La Jolla, CA: California Sea Grant College System, National Oceanic and Atmospheric Administration, in cooperation with Centro de Investigaciones Biológicas del Noroeste.
Brochier, T., Echevin, V., Tam, J., Chaigneau, A., Goubanova, K., and Bertrand, A. (2013). Climate change scenarios experiments predict a future reduction in small pelagic fish recruitment in the humboldt current system. Glob. Chang. Biol. 19, 1841–1853. doi: 10.1111/gcb.12184
Brochier, T., Lett, C., and Fréon, P. (2011). Investigating the “northern humboldt paradox” from model comparisons of small pelagic fish reproductive strategies in eastern boundary upwelling ecosystems. Fish Fish. 12, 94–109. doi: 10.1111/j.1467-2979.2010.00385.x
Brosset, P., Fromentin, J.-M., Ménard, F., Pernet, F., Bourdeix, J.-H., Bigot, J.-L., et al. (2015a). Measurement and analysis of small pelagic fish condition: a suitable method for rapid evaluation in the field. J. Exp. Mar. Bio. Ecol. 462, 90–97. doi: 10.1016/j.jembe.2014.10.016
Brosset, P., Ménard, F., Fromentin, J.-M., Bonhommeau, S., Ulses, C., Bourdeix, J.-H., et al. (2015b). Influence of environmental variability and age on the body condition of small pelagic fish in the gulf of lions. Mar. Ecol. Prog. Ser. 529, 219–231. doi: 10.3354/meps11275
Brosset, P., Le Bourg, B., Costalago, D., Bǎnaru, D., Van Beveren, E., Bourdeix, J.-H., et al. (2016). Linking small pelagic dietary shifts with ecosystem changes in the Gulf of Lions. Mar. Ecol. Prog. Ser. 554, 157–171. doi: 10.3354/meps11796
Burnham, K. P., and Anderson, D. R. (2002). Model Selection and Multimodel Inference: A Practical Information-Theoretic Approach, 2nd Edn. New York, NY: Springer.
Bǎnaru, D., Mellon-duval, C., Roos, D., Bigot, J.-L., Souplet, A., Jadaud, A., et al. (2013). Trophic structure in the Gulf of Lions marine ecosystem (north-western Mediterranean Sea) and fishing impacts. J. Mar. Syst. 111–112, 45–68. doi: 10.1016/j.jmarsys.2012.09.010
Campus, M., Addis, M. F., Cappuccinelli, R., Porcu, M. C., Pretti, L., Tedde, V., et al. (2010). Stress relaxation behaviour and structural changes of muscle tissues from Gilthead Sea Bream (Sparus aurata L.) following high pressure treatment. J. Food Eng. 96, 192–198. doi: 10.1016/j.jfoodeng.2009.07.013
Caruso, G., Maricchiolo, G., Micale, V., Genovese, L., Caruso, R., and Denaro, M. G. (2010). Physiological responses to starvation in the European eel (Anguilla anguilla): effects on haematological, biochemical, non-specific immune parameters and skin structures. Fish Physiol. Biochem. 36, 71–83. doi: 10.1007/s10695-008-9290-6
Chavez, F. P., Ryan, J., Lluch-Cota, S. E., and Niquen, M. C. (2003). From anchovies to sardines and back: multidecal change in the Pacific Ocean. Science 299, 217–221. doi: 10.1126/science.1075880
Checkley, D. M., Asch, R. G., and Rykaczewski, R. R. (2017). Climate, anchovy, and sardine. Ann. Rev. Mar. Sci. 9, 469–493. doi: 10.1146/annurev-marine-122414-133819
Collard, F., Gilbert, B., Eppe, G., Roos, L., Compère, P., Das, K., et al. (2017). Morphology of the filtration apparatus of three planktivorous fishes and relation with ingested anthropogenic particles. Mar. Pollut. Bull. 116, 182–191. doi: 10.1016/j.marpolbul.2016.12.067
Coma, R., Ribes, M., Serrano, E., Jimenez, E., Salat, J., and Pascual, J. (2009). Global warming-enhanced stratification and mass mortality events in the Mediterranean. Proc. Natl. Acad. Sci. U.S.A. 106, 6176–6181. doi: 10.1073/pnas.0805801106
Costalago, D., Garrido, S., and Palomera, I. (2015). Comparison of the feeding apparatus and diet of European sardines Sardina pilchardus of Atlantic and Mediterranean waters: ecological implications. J. Fish Biol. 86, 1348–1362. doi: 10.1111/jfb.12645
Costalago, D., Navarro, J., Álvarez-Calleja, I., and Palomera, I. (2012). Ontogenetic and seasonal changes in the feeding habits and trophic levels of two small pelagic fish species. Mar. Ecol. Prog. Ser. 460, 169–181. doi: 10.3354/meps09751
Costalago, D., and Palomera, I. (2014). Feeding of European pilchard (Sardina pilchardus) in the northwestern Mediterranean: from late larvae to adults. Sci. Mar. 78, 41–54. doi: 10.3989/scimar.03898.06D
Costantini, D., and Dell’Omo, G. (2006). Effects of T-cell-mediated immune response on avian oxidative stress. Comp. Biochem. Physiol. A Mol. Integr. Physiol. 145, 137–142. doi: 10.1016/j.cbpa.2006.06.002
Cury, P. M., Bakun, A., Crawford, R. J. M., Jarre, A., Quiñones, R. A., Shannon, L. J., et al. (2000). Small pelagics in upwelling systems: patterns of interaction and structural changes in “wasp-waist” ecosystems. ICES J. Mar. Sci. 57, 603–618. doi: 10.1006/jmsc.2000.0712
Cushing, D. H. (1990). Plankton production and year-class strength in fish populations: an update of the match/mismatch hypothesis. Adv. Mar. Biol. 26, 249–293. doi: 10.1016/S0065-2881(08)60202-3
Evjemo, J. O., Reitan, K. I., and Olsen, Y. (2003). Copepods as live food organisms in the larval rearing of halibut larvae (Hippoglossus hippoglossus L.) with special emphasis on the nutritional value. Aquaculture 227, 191–210. doi: 10.1016/S0044-8486(03)00503-9
Feng, G., Shi, X., Huang, X., and Zhuang, P. (2011). Oxidative stress and antioxidant defenses after long-term fasting in blood of Chinese sturgeon (Acipenser sinensis). Procedia Environ. Sci. 8, 469–475. doi: 10.1016/j.proenv.2011.10.074
Finkel, T., and Holbrook, N. J. (2000). Oxidants, oxidative stress and the biology of ageing. Nature 408, 239–247. doi: 10.1038/35041687
Food and Agriculture Organization [FAO] (2018). The State of World Fisheries and Aquaculture 2018 - Meeting the Sustainable Development Goals. Rome: FAO.
Frederiksen, M., Edwards, M., Richardson, A. J., Halliday, N. C., and Wanless, S. (2006). From plankton to top predators: bottom-up control of a marine food web across four trophic levels. J. Anim. Ecol. 75, 1259–1268. doi: 10.1111/j.1365-2656.2006.01148.x
Ganias, K. (2009). Linking sardine spawning dynamics to environmental variability. Estuar. Coast. Shelf Sci. 84, 402–408. doi: 10.1016/j.ecss.2009.07.004
Garrido, S., Ben-Hamadou, R., Oliveira, P. B., Cunha, M. E., Chícharo, M. A., and van der Lingen, C. D. (2008). Diet and feeding intensity of sardine Sardina pilchardus: correlation with satellite-derived chlorophyll data. Mar. Ecol. Prog. Ser. 354, 245–256. doi: 10.3354/meps07201
Garrido, S., Marçalo, A., Zwolinski, J., and van der Lingen, C. D. (2007). Laboratory investigations on the effect of prey size and concentration on the feeding behaviour of Sardina pilchardus. Mar. Ecol. Prog. Ser. 330, 189–199. doi: 10.3354/meps330189
General Fisheries Commission for the Mediterranean [GFCM] (2017a). Report of the Working Group on Stock Assessment of Demersal Species. Rome: GFCM.
General Fisheries Commission for the Mediterranean [GFCM] (2017b). Report of the Working Group on Stock Assessment of Small Pelagic Species. Rome: GFCM.
Geyer, R. L., Smith, G. R., and Rettig, J. E. (2016). Effects of Roundup formulations, nutrient addition, and Western mosquitofish (Gambusia affinis) on aquatic communities. Environ. Sci. Pollut. Res. 23, 11729–11739. doi: 10.1007/s11356-016-6381-2
Gimbo, R. Y., Fávero, G. C., Franco Montoya, L. N., and Urbinati, E. C. (2015). Energy deficit does not affect immune responses of experimentally infected pacu (Piaractus mesopotamicus). Fish Shellfish Immunol. 43, 295–300. doi: 10.1016/j.fsi.2015.01.005
Hjort, J. (1914). Fluctuations in the Great Fisheries of Northern Europe Viewed in the Light of Biological Research. Copenhagen: ICES.
Hunt, G. L., and McKinnell, S. (2006). Interplay between top-down, bottom-up, and wasp-waist control in marine ecosystems. Prog. Oceanogr. 68, 115–124. doi: 10.1016/j.pocean.2006.02.008
International Council for the Exploration of the Sea [ICES] (2011). Report of the Workshop on Age Reading of European Atlantic Sardine (WKARAS). Lisbon: ICES.
James, A. G., and Probyn, T. (1989). The relationship between respiration rate, swimming speed and feeding behaviour in the cape anchovy engraulis capensis Gilchris. J. Exp. Mar. Bio. Ecol. 131, 81–100. doi: 10.1016/0022-0981(89)90001-4
Kassambara, A., and Mundt, F. (2017). factoextra: Extract and Visualize the Results of Multivariate Data Analyses. Available at: https://cran.r-project.org/package=factoextra (accessed August 22, 2017).
Kirkwood, T. B. L., and Rose, M. R. (1991). Evolution of senescence: late survival sacrificed for reproduction. Philos. Trans. R. Soc. Lond. Ser. B Biol. Sci. 332, 15–24. doi: 10.1098/rstb.1991.0028
Lê, S., Josse, J., and Husson, F. (2008). FactoMineR: an R package for multivariate analysis. J. Stat. Softw. 25, 1–18. doi: 10.18637/jss.v025.i01
Le Bourg, B., Bǎnaru, D., Saraux, C., Nowaczyk, A., Le Luherne, E., Jadaud, A., et al. (2015). Trophic niche overlap of sprat and commercial small pelagic teleosts in the Gulf of Lions (NW Mediterranean Sea). J. Sea Res. 103, 138–146. doi: 10.1016/j.seares.2015.06.011
Lenth, R. V. (2016). Least-squares means: the R package lsmeans. J. Stat. Softw. 69, 1–33. doi: 10.18637/jss.v069.i01
Lloret, J., Shulman, G., and Love, R. M. (2013). Condition and Health Indicators of Exploited Marine Fishes. Oxford: John Wiley & Sons.
Luo, G., Liu, G., and Tan, H. X. (2013). Effects of stocking density and food deprivation-related stress on the physiology and growth in adult Scortum barcoo (McCulloch & Waite). Aquac. Res. 44, 885–894. doi: 10.1111/j.1365-2109.2012.03093.x
Martin, S. A. M., Douglas, A., Houlihan, D. F., and Secombes, C. J. (2010). Starvation alters the liver transcriptome of the innate immune response in Atlantic salmon (Salmo salar). BMC Genomics 11:418. doi: 10.1186/1471-2164-11-418
McBride, R. S., Somarakis, S., Fitzhugh, G. R., Albert, A., Yaragina, N. A., Wuenschel, M. J., et al. (2015). Energy acquisition and allocation to egg production in relation to fish reproductive strategies. Fish Fish. 16, 23–57. doi: 10.1111/faf.12043
Metcalfe, N. B., and Alonso-Alvarez, C. (2010). Oxidative stress as a life-history constraint: the role of reactive oxygen species in shaping phenotypes from conception to death. Funct. Ecol. 24, 984–996. doi: 10.1111/j.1365-2435.2010.01750.x
Metcalfe, N. B., and Monaghan, P. (2001). Compensation for a bad start: grow now, pay later? Trends Ecol. Evol. 16, 254–260. doi: 10.1016/S0169-5347(01)02124-3
Millot, C. (1990). The Gulf of Lions’ hydrodynamics. Cont. Shelf Res. 10, 885–894. doi: 10.1016/0278-4343(90)90065-T
Moltschaniwskyj, N., and Johnston, D. (2006). Evidence that lipid can be digested by the dumpling squid Euprymna tasmanica, but is not stored in the digestive gland. Mar. Biol. 149, 565–572. doi: 10.1007/s00227-006-0246-z
Monaghan, P., Metcalfe, N. B., and Torres, R. (2009). Oxidative stress as a mediator of life history trade-offs: mechanisms, measurements and interpretation. Ecol. Lett. 12, 75–92. doi: 10.1111/j.1461-0248.2008.01258.x
Nikolioudakis, N., Isari, S., Pitta, P., and Somarakis, S. (2012). Diet of sardine Sardina pilchardus: an “end-to-end” field study. Mar. Ecol. Prog. Ser. 453, 173–188. doi: 10.3354/meps09656
O’Connor, K. I., Taylor, A. C., and Metcalfe, N. B. (2000). The stability of standard metabolic rate during a period of food deprivation in juvenile Atlantic salmon. J. Fish Biol. 57, 41–51. doi: 10.1006/jfbi.2000.1280
Pérez, G. L., Vera, M. S., and Miranda, L. (2011). “Effects of herbicide glyphosate and glyphosate-based formulations on aquatic ecosystems,” in Herbicides and Environment, ed. A. Kortekamp (London: InTech).
Petrenko, A., Leredde, Y., and Marsaleix, P. (2005). Circulation in a stratified and wind-forced Gulf of Lions, NW Mediterranean Sea: in situ and modeling data. Cont. Shelf Res. 25, 7–27. doi: 10.1016/j.csr.2004.09.004
Pinheiro, J., Bates, D., DebRoy, S., Sarkar, D., and R Core Team (2018). nlme: Linear and Nonlinear Mixed Effects Models. Available at: https://cran.r-project.org/package=nlme (accessed May 12, 2019).
Queiros, Q., Fromentin, J.-M., Astruc, G., Bauer, R. K., and Saraux, C. (2018). Dolphin predation pressure on pelagic and demersal fish in the northwestern Mediterranean Sea. Mar. Ecol. Prog. Ser. 603, 13–27. doi: 10.3354/meps12672
R Core Team (2018). R: A Language and Environment for Statistical Computing. Vienna: R foundation for statistical computing.
Relyea, R. A. (2009). A cocktail of contaminants: how mixtures of pesticides at low concentrations affect aquatic communities. Oecologia 159, 363–376. doi: 10.1007/s00442-008-1213-9
Rouyer, T., Fromentin, J.-M., Hidalgo, M., and Stenseth, N. C. (2014). Combined effects of exploitation and temperature on fish stocks in the Northeast Atlantic. ICES J. Mar. Sci. 71, 1554–1562. doi: 10.1093/icesjms/fsu042
Rykaczewski, R. R. (2009). Influence of Oceanographic Variability on the Planktonic Prey and Growth of Sardine and Anchovy in the California Current Ecosystem. San Diego: Scripps Institution of Oceanography Technical Report, Scripps Institution of Oceanography.
Saraux, C., Van Beveren, E., Brosset, P., Queiros, Q., Bourdeix, J.-H., Dutto, G., et al. (2019). Small pelagic fish dynamics: a review of mechanisms in the Gulf of Lions. Deep Sea Res. Part II Top. Stud. Oceanogr. 159, 52–61. doi: 10.1016/j.dsr2.2018.02.010
Schwartzlose, R. A., Alheit, J., Bakun, A., Baumgartner, T. R., Cloete, R., Crawford, R. J. M., et al. (1999). Worldwide large-scale fluctuations of sardine and anchovy populations. South Afr. J. Mar. Sci. 21, 289–347. doi: 10.2989/025776199784125962
Shahidul Islam, M., and Tanaka, M. (2004). Impacts of pollution on coastal and marine ecosystems including coastal and marine fisheries and approach for management: a review and synthesis. Mar. Pollut. Bull. 48, 624–649. doi: 10.1016/j.marpolbul.2003.12.004
Shannon, L., Coll, M., Neira, S., Cury, P. M., and Roux, J.-P. (2009). “Impacts of fishing and climate change explored using trophic models,” in Climate Change and Small Pelagic Fish, eds D. Checkley, J. Alheit, Y. Oozeki, and C. Roy (Cambridge: Cambridge University Press), 158–190. doi: 10.1017/cbo9780511596681.010
Smith, G. R., Krishnamurthy, S. V. B., Burger, A. C., and Rettig, J. E. (2018). Effects of malathion and nitrate exposure on the zooplankton community in experimental mesocosms. Environ. Sci. Pollut. Res. 25, 9992–9997. doi: 10.1007/s11356-018-1311-0
Taylor, M. H., Tam, J., Blaskovic, V., Espinoza, P., Michael Ballón, R., Wosnitza-Mendo, C., et al. (2008). Trophic modeling of the Northern Humboldt Current Ecosystem, Part II: elucidating ecosystem dynamics from 1995 to 2004 with a focus on the impact of ENSO. Prog. Oceanogr. 79, 366–378. doi: 10.1016/j.pocean.2008.10.008
Tocher, D. R. (2003). Metabolism and functions of lipids and fatty acids in teleost fish. Rev. Fish. Sci. 11, 107–184. doi: 10.1080/713610925
Toresen, R., and Ostvedt, O. J. (2000). Variation in abundance of Norwegian spring-spawning herring (Clupea harengus, Clupeidae) throughout the 20th century and the influence of climatic fluctuations. Fish Fish. 1, 231–256. doi: 10.1046/j.1467-2979.2000.00022.x
Van Beveren, E., Bonhommeau, S., Fromentin, J.-M., Bigot, J.-L., Bourdeix, J.-H., Brosset, P., et al. (2014). Rapid changes in growth, condition, size and age of small pelagic fish in the Mediterranean. Mar. Biol. 161, 1809–1822. doi: 10.1007/s00227-014-2463-1
Van Beveren, E., Fromentin, J.-M., Bonhommeau, S., Nieblas, A.-E., Métral, L., Brisset, B., et al. (2017). Predator–prey interactions in the face of management regulations: changes in Mediterranean small pelagic species are not due to increased tuna predation. Can. J. Fish. Aquat. Sci. 74, 1422–1430. doi: 10.1139/cjfas-2016-0152
Van Beveren, E., Fromentin, J.-M., Rouyer, T., Bonhommeau, S., Brosset, P., and Saraux, C. (2016a). The fisheries history of small pelagics in the Northern Mediterranean. ICES J. Mar. Sci. J. Cons. 73, 1474–1484. doi: 10.1093/icesjms/fsw023
Van Beveren, E., Keck, N., Fromentin, J.-M., Laurence, S., Boulet, H., Labrut, S., et al. (2016b). Can pathogens alter the population dynamics of sardine in the NW Mediterranean? Mar. Biol. 163:240. doi: 10.1007/s00227-016-3015-7
van der Lingen, C. D. (1994). Effect of particle size and concentration on the feeding behaviour of adult pilchard Sardinops sagax. Mar. Ecol. Prog. Ser. 109, 1–13. doi: 10.3354/meps109001
van der Lingen, C. D. (1995). Respiration rate of adult pilchard Sardinops sagax in relation to temperature, voluntary swimming speed and feeding behaviour. Mar. Ecol. Prog. Ser. 129, 41–54. doi: 10.3354/meps129041
van der Lingen, C. D., Hutchings, L., and Field, J. (2006). Comparative trophodynamics of anchovy Engraulis encrasicolus and sardine Sardinops sagax in the southern Benguela: are species alternations between small pelagic fish trophodynamically mediated? Afr. J. Mar. Sci. 28, 465–477. doi: 10.2989/18142320609504199
Williams, C. (1996). Combatting marine pollution from land-based activities: Australian initiatives. Ocean Coast. Manag. 33, 87–112. doi: 10.1016/S0964-5691(96)00046-4
Wilson, A. J., and Nussey, D. H. (2010). What is individual quality? an evolutionary perspective. Trends Ecol. Evol. 25, 207–214. doi: 10.1016/j.tree.2009.10.002
Zarubin, M., Farstey, V., Wold, A., Falk-Petersen, S., and Genin, A. (2014). Intraspecific differences in lipid content of calanoid copepods across fine-scale depth ranges within the photic layer. PLoS One 9:e092935. doi: 10.1371/journal.pone.0092935
Zhol, S., Ackman, R. G., and Morrison, C. (1995). Storage of lipids in the myosepta of Atlantic salmon (Salmo salar). Fish Physiol. Biochem. 14, 171–178. doi: 10.1007/BF00002460
Keywords: experimentation, small pelagics, Sardina pilchardus, body condition, bottom-up control
Citation: Queiros Q, Fromentin J-M, Gasset E, Dutto G, Huiban C, Metral L, Leclerc L, Schull Q, McKenzie DJ and Saraux C (2019) Food in the Sea: Size Also Matters for Pelagic Fish. Front. Mar. Sci. 6:385. doi: 10.3389/fmars.2019.00385
Received: 17 January 2019; Accepted: 19 June 2019;
Published: 09 July 2019.
Edited by:
Stelios Katsanevakis, University of the Aegean, GreeceReviewed by:
David Costalago, University of British Columbia, CanadaStylianos Somarakis, Hellenic Centre for Marine Research (HCMR), Greece
Susana Garrido, Portuguese Institute of Ocean and Atmosphere (IPMA), Portugal
Copyright © 2019 Queiros, Fromentin, Gasset, Dutto, Huiban, Metral, Leclerc, Schull, McKenzie and Saraux. This is an open-access article distributed under the terms of the Creative Commons Attribution License (CC BY). The use, distribution or reproduction in other forums is permitted, provided the original author(s) and the copyright owner(s) are credited and that the original publication in this journal is cited, in accordance with accepted academic practice. No use, distribution or reproduction is permitted which does not comply with these terms.
*Correspondence: Quentin Queiros, quentin.queiros@ifremer.fr