Non-indigenous Species in the Mediterranean Sea: Turning From Pest to Source by Developing the 8Rs Model, a New Paradigm in Pollution Mitigation
- Marine Biology Station Piran, National Institute of Biology, Piran, Slovenia
For mitigation of the effects of pollution, the media, policy makers and, in turn, the scientific community and industry each provide contributions through development of a sense of urgency, and with guidelines and solutions. For non-indigenous species (NIS) that can frequently have negative impacts on the native biota, this is often conveyed in an emotive way to the general public, who are typically keen to help and to get personally involved. This might be through organization of cleaning campaigns, influence on the media, or collaboration with scientists, to inform them of the local presence and abundance of NIS. Alternatively, they might proactively develop technological solutions themselves. To assess the current state of affairs, we reviewed the presence and effects of NIS in the Mediterranean Sea. As so often, any well-planned and successful activity is directly linked to financing, or a lack thereof, and this leads to sometimes untargeted and sporadic measures that are developed within a project or over a limited timeframe, without any sustainability measures. Therefore, we also assessed the activities and strategies that have been financed in this area of NIS mitigation. Based on this review of the presence and impact of NIS, and previous and ongoing activities, we propose a new paradigm to mitigate such pollution: the 8Rs model (i.e., Recognize, Reduce, Replace, Reuse, Recycle, Recover/Restore, Remove, and Regulate). This model extends from the more traditional 3Rs model (i.e., Reduce, Reuse, and Recycle) that is often used and promoted for innovative waste management strategies. Importantly, the 8Rs model can be applied sequentially, for either prevention of NIS introduction, or preparation of mitigation measures. The 8Rs model was constructed based on Mediterranean NIS, although we believe it can be applied to other sources of pollution and other geographic areas. Importantly, the 8Rs model represents a general framework to organize and categorize future pollution mitigation strategies. This approach is essential for development of any action plan to influence the administrative and financial decision makers who essentially enable these activities, and therefore who have important roles in the guarantee of sustainability of these actions, and the creation of innovative societies.
Introduction
Non-indigenous species (NIS, also known as non-native, alien, or exotic organisms) are species that have been introduced outside of their natural previous or present range by human activities. These might survive and subsequently reproduce in the new environment (CBD, 1993). If these species become established, and their spread threatens the local biodiversity, or causes economic damage, they are known as invasive species (Molnar et al., 2008). The pioneer studies on NIS date back to the 1970s (see the review in Ojaveer et al., 2018), and they become quite numerous in the 1990s (e.g., Carlton and Geller, 1993; Nolan, 1994; Gollasch and Leppäkoski, 1999). Since then, the global research in this field has grown very rapidly (Giakoumi et al., 2019).
Primary Pathways of Non-indigenous Species Introduction
Globally, the introduction of marine NIS can happen deliberately or accidentally. The primary introduction pathways are presented here according to the classification scheme proposed by the Convention on Biological Diversity (CBD, 2014; see also Tsiamis et al., 2018; Zenetos et al., 2018). Accidental introduction of NIS is typically mediated by ships, either through ballast water tanks or by hull biofouling (i.e., Transport – Stowaway). Other examples of the introduction of NIS in aquatic ecosystems include mariculture [i.e., Transport – Contaminant: contaminant on animals (except parasites, species transported by host/vector)], or escape or release due to aquarist activities (i.e., Escape from confinement), or also, for the Mediterranean Sea via the Suez Canal, as dispersal of Lessepsian migrants (i.e., Corridor: Interconnected waterways/basins/seas).
Non-indigenous Species in the Mediterranean Sea
The Mediterranean Sea is nowadays considered among the most impacted upon of the regional seas, due to the diverse anthropogenic pressures on the different ecosystems within the Mediterranean basin (Mannino et al., 2017). Among these, the most relevant are habitat loss and modification, climate change trends, eutrophication and pollution, coastal urbanization, overexploitation of natural resources, and intentional or unintentional introduction of NIS (Lejeusne et al., 2010; Zenetos et al., 2012; Katsanevakis et al., 2013; Marchini et al., 2015; Azzurro et al., 2019).
Over the last two decades, changes in the Mediterranean marine biodiversity related to introduction of NIS have been reported as the consequences of a number of specific actions: intense maritime traffic (Petrocelli et al., 2019; Sardain et al., 2019); opening of artificial channels (Galil, 2006; Galil et al., 2017); and aquaculture activities (Savini et al., 2010). In the last decade, the species richness of marine organisms in the Mediterranean Sea has been reported to have reached ∼17,000 taxa, among which some 820 can be considered NIS (Katsanevakis et al., 2013; Galil et al., 2016; Zenetos et al., 2017). These have included marine species across all taxa, from phytoplankton to fish. These numbers of NIS in the Mediterranean basin are continually evolving, as checklists of NIS are subjected to frequent changes due to morphological similarities and the consequent erroneous identification of taxa or insufficient historical records, and to data from new molecular high-throughput analyses and new phylogenetic studies. However, only around 12% of all of NIS in the Mediterranean are today considered as invasive, or potentially invasive (for review, see Bonanno and Orlando-Bonaca, 2019). Very few invasive aquatic species (IAS) have been recorded for offshore areas, which is probably because the majority of the pathways for their introduction (i.e., maritime traffic, aquaculture, Suez Canal) are related to shallow coastal waters. At the same time, this might also be because most of the sampling efforts have been focused on the coastal areas (Katsanevakis et al., 2016).
Impact of Non-indigenous Species
Human-mediated introduction of marine species to a region outside their natural range of distribution is widely considered to be the major threat to indigenous species diversity and community structures. This can cause habitat modifications, changes in ecosystem functioning, introduction of new diseases and parasites, and genetic modifications, such as hybridization with the native taxa (Cook et al., 2016). NIS, and especially marine IAS, have an overall impact on the provisioning, regulating, cultural, and supporting ecosystem services (Kettunen et al., 2008). Some studies have argued that the overall impact of invasive species is first related to the degree of biological diversity of the recipient habitats, which assumes that the higher the biodiversity, the lower the level of vulnerability of a habitat to NIS invasion (Occhipinti-Ambrogi and Savini, 2003; Bulleri et al., 2008). However, the overall ecological impact of NIS on the Mediterranean Sea remains relatively difficult to quantify, and is mainly qualitative; nevertheless, there have been some good attempts at quantification (Katsanevakis et al., 2014, 2016; Ojaveer et al., 2014, 2015; Gallardo et al., 2016). In particular, the analyses of Katsanevakis et al. (2014) have led to the conclusion that the majority of the recognized invasive species in the European seas (72%) have both positive and negative impacts on the native biota. Few have only positive effects (8%), while more (∼20%) have only negative effects on the host environment.
Environmental Impact and Impact on Human Health
Gallardo et al. (2016) stated that settlement of IAS can lead to ecological impacts that propagate along the food web. Their analysis suggested strong negative influences of IAS on the abundance of aquatic communities, and especially on native macrophytes, zooplankton, and fish, although not on the species diversity in the invaded habitat. Although some NIS impacts on native marine biota were well documented and summarized by Gallardo et al. (2016), there remains a large lack of knowledge in some scientific fields. These gaps are primarily related to biological traits (e.g., competition and space requirements) and biotic resistance (e.g., to pathogens, parasites, and native predators of NIS). This knowledge gaps need to be filled to incorporate such kind of data into a robust bioinvasion model (Ulman et al., 2019).
Some studies have shown strong relationships between the settlement of NIS and the extinction of native species (for reviews, see Bellard et al., 2016; Pyšek et al., 2017). However, as indicated above, a wide range of anthropogenic pressures is influencing worldwide ecosystems, with deleterious consequences on both species and habitats. These continuous changes need to be considered in any assessment of the roles of NIS in undergoing extinctions, as in many cases, a decline in the native species and an increase in abundance of NIS might be coincidental, as this might derive from simultaneous adaptations of native species and NIS to various human-induced disturbances, as was discussed by Pyšek et al. (2017).
Invasive macroalgae have the highest impact among all of the taxonomic groups, with Caulerpa cylindracea Sonder, Womersleyella setacea (Hollenberg) R.E. Norris, and Lophocladia lallemandii (Montagne) F. Schmitz indicated as the most invasive in the Mediterranean Sea (Katsanevakis et al., 2016). The green alga C. cylindracea is considered highly invasive also in the Adriatic Sea (for review, see Orlando-Bonaca et al., 2019), and specifically along the eastern Croatian coast. This seaweed was reported to have smothered live colonies of Mediterranean stony coral [Cladocora caespitosa (L., 1767)] in the Mljet National Park, which caused complete retraction of the corals, thus leaving the calyx rims deprived of tissue cover (Kružić et al., 2008). The C. cylindracea colonization of predominantly port areas and urbanized bays along the western Istrian coast led Iveša et al. (2015) to assume that human-induced activities might enhance the diffusion of this IAS. Ulman et al. (2019) concluded that as high levels of pollution are known to cause reduced biodiversity, the settlement of opportunistic NIS is favored in such impacted sites.
As for C. cylindracea, the red algae W. setacea and L. lallemandii have also been reported to reproduce predominantly vegetatively (Rindi et al., 1999; Lučić et al., 2017), to form extensive turfs in the southern Adriatic Sea (Battelli and Rindi, 2008) and along other parts of the Mediterranean coast (Deudero et al., 2010; Bitar et al., 2017), thus competing with the native algal species for the natural resources (Verlaque et al., 2015). Battelli and Rindi (2008) outlined that the rapid spread by vegetative fragmentation is a typical trait of many of the invasive macroalgae that have been introduced into the Mediterranean Sea.
Among the animal NIS, it appears that the invasive American comb jellyfish, Mnemiopsis leidyi A. Agassiz, 1865, is one of the invertebrates that is still spreading, and has not yet reached its maximum possible extension (Malej et al., 2017). M. leidyi was first detected in the Black Sea in the 1980s (Vinogradov et al., 1989), from where it has spread to diverse marine and transitional Mediterranean areas (Shiganova et al., 2004; Boero et al., 2009; Galil et al., 2009). From 2016, M. leidyi has been observed in several northern Adriatic areas, where it was also reported to successfully reproduce, which confirms its status as a successful invader (Malej et al., 2017).
When the Pacific oyster Magallana gigas (Thunberg, 1793) was intentionally introduced into northwestern Europe in the late 1960s, it was considered an ideal replacement for many of the stressed native oyster farms due to its rapid growth rates and resilience to disease (Kerckhof et al., 2007). As a culture species, this NIS was then translocated to over 60 countries outside its native range, and in the end it now accounts for >80% of the global oyster cultures (Kong et al., 2015). M. gigas has spread widely from the culture sites, thus also proving to be a successful invader (Troost, 2010), with competition with the native oyster species for food resources and space (Dankers et al., 2006). Among molluscs, for the Mediterranean Sea to date, only the increased abundance of the Red Sea mussel Brachidontes pharaonis (Fischer, 1870) has been reported to have led to local displacement (although without extinction) of the native mussel Mytilaster minimus (Poli, 1795) (Rilov et al., 2004).
According to Galil (2011) and Manfrin et al. (2018), among the diverse crustacean NIS so far detected in the Mediterranean Sea, around 70 decapods are known (i.e., crabs, crayfish, and prawns), which are believed to be both aggressive and adaptable to various environments. Among these, the Atlantic blue crab, Callinectes sapidus Rathbun, 1896, that is a native from the eastern coast of America, has high fecundity, strong swimming capacity, and pronounced aggressiveness (Garcia et al., 2018). C. sapidus has now been reported as already established in the Mediterranean Sea (Galil et al., 2002; Lipej et al., 2017), and it continues to spread up to the northern Adriatic (Dulčić et al., 2010). As C. sapidus also attacks fish and other crustaceans that are trapped in fishing gear, which can cause material damage, it is included in the list of the 100 worst invasive alien marine species of the Mediterranean Sea (Streftaris and Zenetos, 2006).
Non-indigenous species have also been reported for the phytoplankton and benthic microalgal communities across the Mediterranean Sea, although they appear to be far less numerous compared to macroalgae. Native phytoplankton species can sometimes be deleterious for the environment, such as for their high biomass blooms and all the consequences of these, or due to their toxic potential against humans and marine fauna (e.g., Lassus et al., 2016). Therefore, introduced microalgal species might be accompanied by the same threats. Many factors contribute to the difficulty of assessing the NIS status of any microalgal species, including difficulties in sampling and identification, and poor knowledge of their particular biological and ecological characteristics, such as patchy distributions and heteromorphic life cycles (Gómez, 2008, 2019; Corriero et al., 2016). In his recent work, Gómez (2019) expressed doubt on the non-indigenous nature of all 52 microalgal species in European seas reported by Tsiamis et al. (2019). This would also exclude 10 NIS found in the Mediterranean Sea. Similar doubts on the origin of several cryptic species have led experts from Mediterranean sub-basins to perform a rigorous survey, which resulted in a much shorter list of microalgal NIS, i.e., two dinoflagellates [Alexandrium catenella (Whedon & Kofoid) Balech, Ostreopsis cf. ovata Y.Fukuyo] and three diatoms [Chaetoceros bacteriastroides Karsten, Pseudo-nitzschia multistriata H.Takano (H.Takano), Skeletonema tropicum Cleve] (Zingone, 2015; Corriero et al., 2016; Mozetič et al., 2019). Apart from the diatoms Skeletonema tropicum and Chaetoceros bacteriastroides, the other three species can be considered as harmful to the environment and to human health (Lassus et al., 2016).
Toward the Establishment of Mitigation Measures
According to González-Moreno et al. (2019), to identify the most harmful NIS on a larger trans-regional scale, standardized tools are needed, as well as highly skilled experts, to whom adequate training and clear guidelines have been given. In parallel, the mapping and quantification of NIS impacts on a Mediterranean scale, combined with better knowledge about the anthropogenic pressures that have led to the majority of the invasions, would significantly help scientists and policy makers in the preparation of prevention or mitigation actions. In terms of legislation, the Marine Strategy Framework Directive (MSFD; 2008/56/EC) (EC, 2008) is the most comprehensive environmental legislation in the European Union that requires the Member States to report on the ecosystem characteristics, pressures and impacts (Borja et al., 2016). NIS are covered within the Descriptor 2 that provides basis to develop indicators for the evaluation of the environmental status. Moreover, there are regional legislative initiatives that provide requirements and standards in terms of NIS monitoring. In the Mediterranean area, the United Nations Environment Programme (UNEP-MAP) Convention for the protection of the Mediterranean Sea against pollution (Barcelona convention) and its Action Plan identify the potential impact of NIS and establish the objective of maintaining the levels of NIS, introduced by human activities at levels that do not adversely alter the ecosystem (UNEP-MAP, 2012). However, a lack of consistency/reliability of the information related to NIS has been reported (Palialexis et al., 2014). Moreover, these legislative provisions describe the need for establishing proactive measures but fail to develop a strategic framework that might offer a starting point for the implementation of mitigation measures. One of the reasons for this could be the communication and collaboration gap between scientists and policy makers where the increasing monitoring requirements are often set against a backdrop of decreasing budgets (Borja et al., 2016). On the other hand, there is a lack of adoption of good practice examples from other regional strategic frameworks, such as HELCOM Monitoring and Assessment Strategy, adopted for the Baltic Sea Region (HELCOM, 2018). Importantly, both regional strategies highlight the need of developing mitigation measures against NIS introduction, without providing concrete measures.
Economic Impact
The oceans, and especially the coastal ecosystems, have been estimated to contribute >75% of the global economic value of the biosphere (Martínez et al., 2007; Katsanevakis et al., 2014). Estimations of the costs of the damage of harmful IAS have ranged from 1.4 to 12% of gross domestic product (Marbuah et al., 2014). This is up to sevenfold more than the proportion of the global gross domestic product that was spent on research and development, at 1.7% in 2017 (UNESCO Institute for Statistics, Supplementary Table S1). The concerns for the need to reduce the impact of NIS species are thus of significant importance to economists. However, due to the lack of understanding between the fields of ecology and economy, experts from these areas rarely work together to understand and mitigate the adverse effects of NIS (Keller et al., 2011).
The present article reviews the current situation of NIS spreading in the Mediterranean Sea and confirms the need for the definition of structured approaches for mitigation of such pollution, especially in the case of those species for which their invasive nature has already been recognized. The aim of the manuscript is to propose and introduce the 8Rs model (Recognize, Reduce, Replace, Reuse, Recycle, Recover/Restore, Remove, and Regulate) for the NIS mitigation, with the explanation of each of the eight Rs, including real-life examples of previously established solutions. The formal 3-step prevention/mitigation strategy is then proposed, where each of the eight Rs are included with a set of recommendations on how to efficiently tackle NIS presence in the Mediterranean. Finally, a case study with two non-indigenous and invasive macroalgae in the Mediterranean Sea, the brown Undaria pinnatifida and the green Caulerpa taxifolia, is presented.
A New Paradigm in Mitigation of Non-Indigenous Species
Marine pollution can be categorized into physical (e.g., light, sound), chemical or biological (macro and microorganisms). The introduction and/or successful colonization of NIS and IAS has been regarded as biological pollution in the past (Elliott, 2003; Olenin et al., 2011). It is, however, important to differentiate between NIS and IAS. The latter can automatically be considered as biological pollutants, as their adverse effects on the environment have been proven. An example is Caulerpa taxifolia, a green alga that was accidentally introduced to the Mediterranean Sea from ornamental aquaria and attributed to the decline in native species richness and abundance (Keller et al., 2011). On the other hand, NIS should not automatically be considered as biological pollutants before their effects on the ecosystem and their invasiveness potential are monitored and confirmed. This necessarily implies a structured approach for determining strategies to first establish the effects of NIS and mitigate them in the second instance. Hence, to develop a new paradigm toward mitigation of NIS, we started by upgrading the 3Rs model (Reduce, Reuse, and Recycle) that was originally defined to help to improve global waste management. We have thus defined an 8Rs model for NIS management, as illustrated in Figure 1. The eight steps proposed are Recognize, Reduce, Replace, Reuse, Recycle, Recover/Restore, Remove, and Regulate, which are now initially defined.
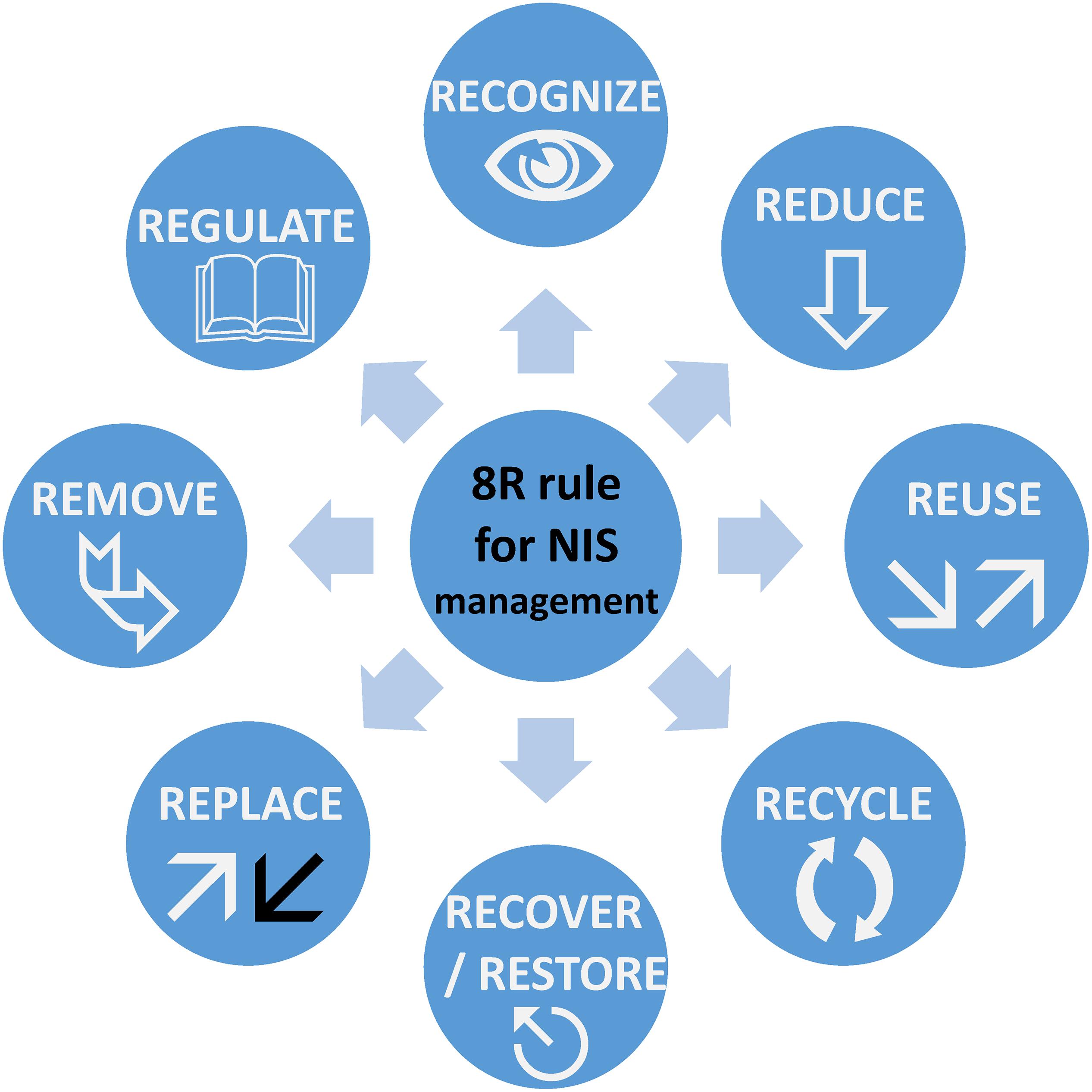
Figure 1. Representation of the 8Rs model for NIS management. This model can be adapted for use for other sources of biological or chemical pollution.
Recognize
Correct identification of NIS is of extreme importance for the scientific and government sectors. Recognition of a NIS is typically through dedicated collaborative projects or long-term monitoring campaigns, which in Europe should follow the requirements of ‘Descriptor 2’ of the Marine Strategy Framework Directive (MSFD; 2008/56/EC) (EC, 2008). However, monitoring campaigns often provide only static information of the absence or presence of a species. Between any two monitoring campaigns, and particularly in cases where there is a lack of financial support, researchers are not able to dedicate time and effort to recognize any dynamic changes in NIS abundance. Therefore, it is of strategic importance to educate citizens, to more professionally prepare them for individual citizen science campaigns. These surveys can then serve as important sources of information (e.g., geolocation, time, and abundance) for the detection of important changes and dynamics in NIS richness and abundance. In the case of macroorganisms, it is relatively simple to organize citizen science campaigns nowadays, providing that there is a constructive strategy and financing for the resources needed (e.g., app development for real-time feedback, motivation workshops for future citizen scientists).
Reduce
The most effective method in NIS reduction is to prevent the entry and spread, and finally the establishment, of any NIS in any specific area. In the marine environment, where the introduction of NIS mainly occurs through ballast water discharges from transport ships, the reduction of NIS might be achieved by focusing on the primary pathways of their introduction; i.e., the appropriate treatment of the ballast waters. For the introduction of NIS by mariculture activities (i.e., species transported by a host or vector), we recommend examination of, e.g., imported molluscs in the search for non-indigenous accompanying algal species.
Reuse
These activities encompass the use of complete organisms for another goal. For example, larger NIS can be removed from the affected areas and used for recreational purposes (e.g., for home use, in spa salons, such as jellyfish or fish in aquaria), in “high-level” culinary products, or as organic fertilizers, as a bio-based approach where side stream/waste (as NIS can be seen as such) is used to produce valuable products, thus also helping the global economy.
Recycle
The compounds and metabolites in NIS can be valorized for use as high value-added products. Indeed, this is the innovative area with the highest potential for exploitation. Marine biotechnology is an emerging field, where synthesis of the metabolites that are produced by marine (micro)organisms can be optimized to maximize their yield. These metabolites can then be used in various applications, such as medicine, pharmacology, nutraceuticals, food, feed, and other industries.
Recover
These are organized activities aimed to recover and/or restore an invaded area to its original state. However, before any efficient recovery activities can be carried out, it is necessary to remove the impacting organisms, or at least to reduce their severity, intensity and/or duration (Smith et al., 2017). Ideally, restore/recovery activities are conducted in regions where the ecosystem has high recovery potential (Smith et al., 2017).
Replace
Replacement strategies can be categorized into (a) species replacement or (b) surface replacement. In the first case, the aim of replacement strategies is to intentionally introduce competing species that will eventually out-number and out-compete the NIS. On the other hand, surface replacement strategies can be considered to decrease the likelihood of accidental NIS introduction by hull biofouling. In fact, when not coated with anti-fouling additives, ships, buoys, floating oil platforms, and other shipping infrastructure represent an excellent substrate for settlement of NIS. Hence, ship bottoms are often coated with anti-fouling systems to prevent the attachment of marine organisms. By adopting the International Convention on the Control of Harmful Antifouling Systems on Ships in 2001 (IMO, 2001) that completely prohibited the use of organotin compounds in anti-fouling systems in 2008, there was a need to find alternatives (e.g., copper-based, tin-free paints, non-stick coatings, periodic cleaning of the hull, electricity, prickly coatings, and natural biocides). Therefore, by using bio-based anti-fouling systems, these surfaces can be replaced by environmentally acceptable and efficient options, thus reducing the surface area to which NIS can attach.
Remove
Removal activities can be organized as citizen science events (in the case of the macroorganisms that are easy to detect and remove), or in the form of innovative research and actions. These would typically be financed as collaborative projects where removal strategies are developed. In the case of microorganisms, e.g., harmful algal blooms of either native or non-indigenous species, several mitigation actions have been proposed, which are likely to be field demonstration ready in the near future or are already in use. These actions include physical (flocculation, sediment resuspension, and cell harvesting and removal), chemical (use of biosurfactants, barley straw, hydrogen peroxide, copper) and biological control (algistatic and algicidal properties of macroalgae, viruses, and bacteria) for mitigating harmful algal blooms (Kidwell, 2015).
Regulate
Essentially, the aim of the establishment of a regulatory framework for NIS in the Mediterranean Sea is to safeguard the environment from any critical increases in the numbers of individual NIS before they can have adverse effects on local ecosystems. Moreover, regulation is often directly linked to stable financing, which at a minimum enables constant monitoring of the environmental status to detect any introductions, and the local abundance and effects of NIS.
The 8Rs Model in Current Practices
We initially collected together the available information on the efforts that have been (or are currently being) undertaken in the Mediterranean Sea to better manage marine NIS. We searched for finalized and ongoing EU projects, as well as scientific papers that have provided mitigation solutions. The CORDIS and keep.eu databases were used for EU-funded research (mainly Framework Programmes 7, Horizon 2020) and Interreg/cross-border projects, and Web of Science for scientific articles, using a range of keywords: non-indigenous, non-native, alien, invasive species, ballast water management, citizen science, and biotechnology. These past and current activities were then arranged into the categories of the newly constructed 8Rs model for NIS management, and are presented in Table 1, as Recognize, Reduce, Reuse, Recycle, Recover/Restore, Replace, Remove, and Regulate. Table 1 provides an overview of the current strategies and enables better identification of strategies and recommendations for future NIS pollution mitigation.
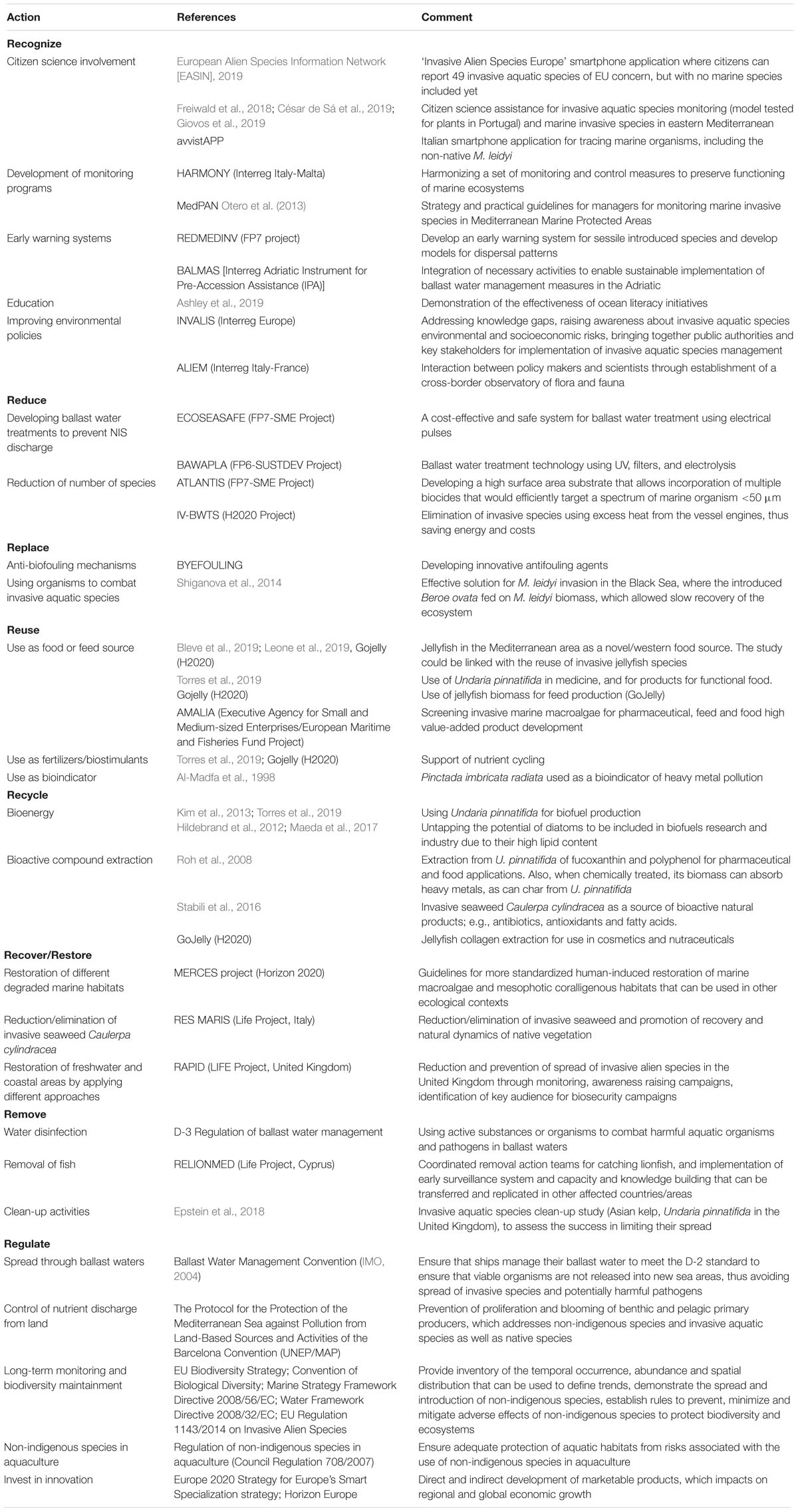
Table 1. Innovative solutions under the 8Rs categories that are designed to mitigate the problems of non-indigenous species.
Recognize
Different approaches that fit under this action were included, which are all crucial for prevention of the spread of NIS. Harmonization and implementation of standard monitoring programs at national, regional, and European levels represent one of these approaches. Some projects have focused on the harmonization of monitoring programs (e.g., HARMONY) and the development of early warning systems at a regional level (e.g., REDMEDINV). However, joint efforts need to be carried out to gain accurate estimations of NIS richness, abundance, and potential additional dispersal. On the other hand, there appears to be a lack of collaboration between public authorities, policy makers, stakeholders, and scientists, which needs to be established at national and cross-border levels to improve environmental policies (e.g., INVALIS and ALIEM). MedPAN associations have prepared guidelines for NIS monitoring in marine protected areas, where they have emphasized the need for site-specific NIS management. Recently, increased efforts have been observed in awareness-raising campaigns, and for citizen science inclusion and education programs (i.e., ocean literacy). Addressing this problem with the general public, and especially with the younger generations, is of tremendous importance. Studies have shown that the inclusion of citizen science in the monitoring of IAS (e.g., using smartphone apps, online platforms) can significantly improve the database and also the knowledge of the overall IAS abundance and spread (Freiwald et al., 2018; César de Sá et al., 2019; Giovos et al., 2019). Ocean literacy initiatives have been shown to be effective and to have positive effects on behavior changes of citizens, who then take direct and sustainable actions toward sustainable solutions for marine environments (Ashley et al., 2019).
Reduce
A lot of research has been carried out into ballast water treatment within EU projects (e.g., FP6, FP7, and H2020), for the development of cost-effective technologies (e.g., using UV, filters, electrical pulses, and electrolysis) that can be used to successfully destroy, remove or reduce the organisms before or during the ballast water discharge, to thus reduce the quantities of NIS that enter the new environment with the discharged water. Since 2017, ships have been required to manage their ballast water according to the D-1 and D-2 standards defined by the Ballast Water Management Convention (IMO, 2004). All ships must conform to the D-1 standard, whereby the ballast water must be discharged at least 200 miles from the coast at a minimum depth of 200 m. New ships must also conform to the D-2 standard, where a specific number of organisms is allowed in the discharged water. Although the implementation of these two standards minimizes the survival and spread of harmful aquatic organisms and pathogens, they can still be discharged, thus still allowing the expansion of IAS.
Replace
In some cases, the removal of IAS by replacement of the species has been reported to be effective. In the late 1980s, the invasive Mnemiopsis leidyi devastated the Black Sea economy, and also its ecology. M. leidyi reached high abundance and greatly affected the fish stock, as it was feeding on fish larvae and competing with adult fish for food resources. Another ctenophore Beroe ovata arrived in the Black Sea ecosystem from North American coasts through ballast waters (Shiganova et al., 2014), which then preyed on and replaced M. leidyi. This action turned out to be effective in the reduction of M. leidyi abundance (Kideys, 2002). Although this action allowed recovery here, similar biocontrol systems should be considered very carefully, as all of the potential impacts of any native species in a new environment can be difficult to predict.
The replacement of standard antifouling agents can be achieved by finding a sustainable and eco-friendly anti-fouling agent that can cover or modify (i.e., replace) exposed surfaces, and thus prevent NIS attachment. Many EU projects have been financed for research in this field. For example, the recently concluded EU FP7 project BYEFOULING was aimed to design, develop, and upscale antifouling coatings through the use of innovative antifouling agents, protein adsorption inhibitors, quorum sensing inhibitors, natural biocides, and microorganisms with antifouling properties.
Reuse
Microalgae, seaweed and jellyfish have been studied and screened for potential product development in terms of the reuse of their biomass as fertilizers or biostimulants, or feed and food (Bleve et al., 2019; Leone et al., 2019; Torres et al., 2019). Seaweeds have already been used in the food processing industry (e.g., for production of agar, alginates), but many other applications are possible. They are rich in polysaccharides, phycocolloids, minerals, pigments, fatty acids, vitamins, and terpenoids, whereby their economic value can be relatively low or particularly high, depending on the costs of the application. Of the over 10,000 seaweed species worldwide, many are invasive in certain areas. Two invasive seaweed species in the Mediterranean, Undaria pinnatifida (Harvey) Suringar and C. cylindracea, have shown potential for biomass reuse as a source for feed, food, and fertilizers. An EU project was also financed for screening of invasive macroalgae for bioactive molecules, as well as their testing as feed and food ingredients (i.e., AMALIA). As well as marine flora, marine animals that are invasive or create problems might be reused as a source for industrial processing. Jellyfish biomass has been recently recognized as a novel food source from the Mediterranean Sea (Bleve et al., 2019; Leone et al., 2019), particularly as it has been known as a delicacy in eastern Asia for hundreds of years. Even though the jellyfish body is composed of 95% water, the remaining biomass is rich in proteins and bioactive compounds. An ongoing EU H2020 project called GoJelly is focused on using jellyfish biomass for food, feed, and fertilizer (Emadodin et al., 2020). The above-mentioned ctenophore M. leidyi will also be taken into consideration for applications. Interestingly, an invasive pearl-oyster (Pinctada imbricata radiata) has been used as a bioindicator for metal pollution in the waters of Quatar (Al-Madfa et al., 1998). This species can thus be used as a bioindicator in metal pollution monitoring programs, which is already being established.
Recycle
Recycling covers the use of the IAS biomass for extraction of specific compounds, as applied for added value product development. Under this aspect, application to the bioenergy, cosmetic, nutraceutical and pharmaceutical industries has been considered. Microalgae and seaweed have also been studied for value-added products through biotechnological processing, such as for bioenergy (e.g., methane, hydrogen, ethanol, acetone, butanol, and biodiesel) and for extraction of bioactive compounds for high value-added products (Roh et al., 2008; Kim et al., 2013; Torres et al., 2019). For example, the invasive species U. pinnatifida has been studied for biofuel production (Kim et al., 2013; Torres et al., 2019). Screening for bioactive compounds has also been carried out for invasive seaweeds in the Mediterranean Sea, with U. pinnatifida and C. cylindracea showing promising data (Roh et al., 2008; Stabili et al., 2016). U. pinnatifida extracts specifically are already used in Europe and worldwide in various products for skin care with anti-aging, moisturizing or anti acne benefits1. Under the EU H2020 project GoJelly, the extraction and further processing of jellyfish collagen and other bioactive compounds is ongoing, to use the products for cosmeceutical/nutraceutical applications.
Recover/Restore
Two EU Programs for the Environment and Climate Action (LIFE) projects investigated the restoration of marine areas affected by invasive species (i.e., RESMARIS and RAPID). Their main goal was to reduce and/or eliminate the invasive species, to promote recovery of the natural vegetation. They used different approaches, including monitoring, awareness-raising campaigns, and identification of key actors for biosecurity measures.
Remove
A LIFE project in Cyprus focused on the removal of the lionfish Pterois miles by coordinated removal action teams, as well as by implementation of early warning systems, with capacity building replicated in other affected areas (i.e., RELIONMED). Ballast water management conventions follow the D-3 regulation, which allows the use of active substances and organisms to act against harmful aquatic organisms and pathogens. The substances might also remove and thus prevent the entrance of these NIS. Importantly, removal campaigns are more relevant for large organisms, such as fish (e.g., lionfish), as the likelihood for success is more realistic and does not include measures that might have adverse effects on the environment and local biodiversity (e.g., disinfectants). In addition, studies have been carried out on seaweed clean-up activities, although these have turned out to be less effective for their reduction (Epstein et al., 2018). For example, in the Mediterranean Sea various control efforts, such manual removals of Caulerpa taxifolia (M.Vahl) C.Agardh were taken in marine waters of four countries, but the invasive green alga is still present in the basin, since it was impossible to eradicate it from all colonized areas (Meinesz et al., 2001).
Regulate
The invasion of NIS is not affected by any geopolitical borders. Thus, it necessarily needs international cooperation where regulatory initiatives are needed to define and influence NIS management strategies. The international legislative measures that are designed to help to monitor and control the spread of NIS include the Ballast Water Management Convention for control and management of ship ballast water and sediments (IMO, 2004). However, despite this convention being finalized in 2004, and despite the widespread consensus that its implementation can reduce rates of invasion by IAS, it entered into force only in 2017. The Convention on Biological Diversity (CBD, 1993) entered into force in 1993, and this included provisions for conservation of biological diversity, sustainable use of its components, and fair and equitable sharing of the benefits arising out of the use of genetic resources. However, there is no clear control of (inter)national compliance with legally binding measures and defined sanctions for violation of these provisions that has been established internationally. For example, there is no history of nations being sanctioned for not following the guidance on prevention, introduction, and mitigation of the impacts of alien species, and there is little evidence that the CBD has led to improved NIS management (Keller et al., 2011). Finally, there is no centralized legislation that covers NIS, which makes the coordination of mitigation measures difficult (Keller et al., 2011). Long-term NIS monitoring programs in ports and Marine Protected Areas need to be implemented and harmonized, rather than just providing monitoring of a target species (Ojaveer et al., 2014; Lehtiniemi et al., 2015). According to MSFD requirements, European Member States have to establish the good environmental status of their marine waters, and should subsequently monitor them, also in relation to Descriptor 2 (see section “Recognize”). However, to date, organized monitoring programs for NIS have not been started in all EU countries (Tsiamis et al., 2019), even if proposals for national Descriptor 2 monitoring programs were developed before July 2014, as requested by the MSFD. Nonetheless, there are other mechanisms within EU financing schemes that can provide the additional funds needed to achieve a good environmental status based on MSFD: European Maritime and Fisheries Fund; EU Regional Funds (European Regional Development Funds and Cohesion Funds); EU Program for the Environment and Climate Action (LIFE); and EU Framework Programme for Research and Innovation (Horizon 2020).
As categorized in Table 1, Figure 2 illustrates the summarized EU funding contributions per each of the eight Rs. Here, funding of scientific articles was not taken into account, nor Regulate, because there is no information available on dedicated funds per country for NIS monitoring. To date, most of the EU contributions have been dedicated to NIS introduction (Recognize, 35%; Reduce, 17%; Replace, 21%) which is perhaps not surprising, as this is the first important step toward tackling the NIS problem. There are already regulations that are designed to prevent the entry and spread of NIS, as well as to minimize their impact, although there remains the need for harmonized, long-term, and innovative approaches. Also, there is still room for solutions to Recover, Recycle, and Reuse NIS that are already established in a new environment.
Discussion
Three-Step Mitigation Strategy
Based on the results from our systematic review and analysis and the current and past 8Rs initiatives (Table 1), Figure 3 provides a conceptual diagram of the impact of NIS and the possible mitigation measures in the Mediterranean Sea. The invasion flow is represented by the rounded blue squares. Once NIS enter into their new environment and establish themselves, their impact on the ecosystem services, health and the overall economy can be estimated. The mitigation strategies that are in the process of being developed and are routinely implemented are indicated in the conceptual diagram for the 8Rs model. This three-step mitigation strategy is as follows:
Step 1: Mitigation before NIS enter into the Mediterranean Sea. As the demonstration of successful marine NIS removal is rare, the pragmatic approach to marine NIS management is to focus on precautionary activities (Ojaveer et al., 2015). By increasing the knowledge of the scientific community and by educating the general public (e.g., through proactive communication campaigns, citizen science approaches), the environmental changes can be recognized early enough to enforce the monitoring of potential areas for NIS introduction (e.g., reducing ballast water exchange locations), and replace the previous anti-fouling paints with newly developed bio-based anti-fouling compounds.
Step 2: Establishment of global regulatory mechanisms (by national and joint international monitoring campaigns) that will provide for detection of new NIS and/or changes in their spatiotemporal abundance. This will allow definition of the situation and the issue of early warning mechanisms. In particular, when propagated by the media, these can lead to rapid responses in terms of organized activities (e.g., citizen science campaigns) or to increased budgets [e.g., research programs, (inter)governmental financing] that can be dedicated to mitigation of the potentially devastating impacts of a NIS.
Step 3: Definition of several possible mitigation measures that can come into force after NIS establishment in a new environment. Ideally, this step will generate a plethora of new innovative approaches in the near future. We envision the organization of removal campaigns that will include citizens. With the development of high-throughput technologies in molecular biology, future collaborations will result in new ways to recycle NIS biomass and use their metabolic compounds in new products in the cosmetics, pharmaceutical and energy fields. NIS might also be reused as an alternative food or feed source.
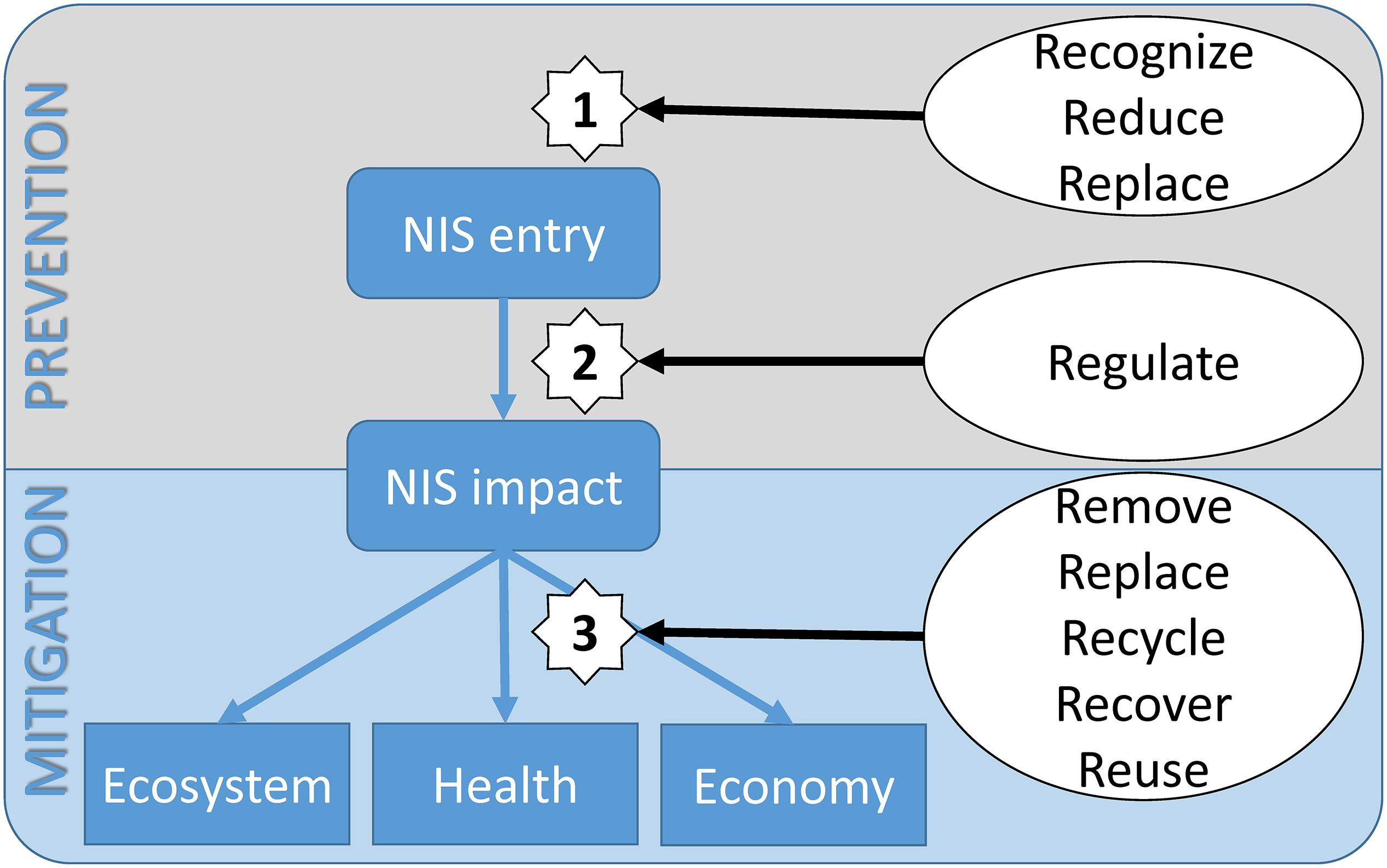
Figure 3. Conceptual diagram of non-indigenous species invasion flow (rounded boxes), their impact (boxes), and the possible steps (1–3) of the 8Rs prevention and mitigation measures (in ellipses).
Recommendations for the Efficient Adoption of 8Rs Model
Finally, we provide here a set of recommendations to search for and find the diverse alternative uses of marine NIS.
Recommendation 1: Establishment and Maintenance of Regular Monitoring Programs
Regular and early detection, elaboration of mitigation measures, and harmonization of national and international legislation help to avoid the need for later damage control (Marbuah et al., 2014; Galil et al., 2016). Therefore, countries should establish national monitoring programs that include ballast water sampling, fouling surveys, and targeted sampling of aquaculture sites. These programs provide longitudinal data that can be used to quantify changes in the native species composition, assess the rate of introduction and spread of a NIS, provide trends in the NIS richness and abundance, detect their negative impacts, and release early warnings. Data from monitoring campaigns are also valuable sources of information for planning mitigation measures for removal of NIS, and the possible novel uses of the compounds that these species synthesize. The establishment of monitoring areas should ideally be carried out near the port areas that receive the greatest number of ships, and are therefore at the greatest risk of NIS introduction (Chan et al., 2015). However, current monitoring programs mostly rely only on traditional taxonomic identification of species based on their morphological traits, which is a lengthy and error-prone system due to the varying individual taxonomic expertise of the analysts (Leese et al., 2016). Moreover, monitoring field sampling, although well suited for larger organisms such as macrofauna or macroalgae (von Ammon et al., 2018), is subjected to imperfect detectability, as not all of the target species can be caught by classical sampling strategies and devices, which can lead to underestimation of a species population size and extent, or even to total failure to detect a NIS (Katsanevakis and Moustakas, 2018). Therefore, high-throughput technologies are increasingly being used for biodiversity assessment and marine biosecurity surveillance programs for a range of aquatic ecosystems, such as the use of metabarcoding (von Ammon et al., 2018). However, there are challenges that prevent the application of the genomic tools into routine monitoring campaigns: standardization of sampling protocols, establishment of DNA barcode reference databases, selection of gold standard sequencing platform and protocols, data analysis and storage pipelines, as well as the lack of ecogenomic indicators (Leese et al., 2016). Ideally, metabarcoding will in future be endorsed by the monitoring authorities and used as an additional screening method to detect NIS, followed by the required validation methods that combine more targeted molecular methods or in-depth morphological analysis (von Ammon et al., 2018). This way we will understand and provide explanation on the ecology of the invaders, their native and introduced ranges and the control of their spread (Douglas et al., 2009).
Recommendation 2: Education of the New Generation of Researchers for Communication of Their Science
Nowadays, the research itself is being carried out in transdisciplinary communities. This allows the work of basic biology scientists, who are typically in charge of providing knowledge of the species presence and abundance, and of changes in their trends, to be merged with the outcomes of applied scientists, who typically aim to exploit compounds that might be synthesized by these organisms. As information is readily accessible and new technologies are emerging on almost a daily basis, the science is rapidly evolving. There is a demonstrated need for awareness raising about NIS and their negative impacts, and for an understanding of the methods for their control (Dehnen-Schmutz et al., 2018). Even the CBD has identified the need to raise public awareness of IAS, which is seen as crucial to their successful management and any introduction and mitigation activities. Future scientific collaborations will necessarily consist of researchers skilled in their basic scientific expertise, and also able to communicate well with the media and across various disciplines. Hence, knowledge of communication, economy, entrepreneurship and project teamwork are all subjects that need to find their way into the obligatory student curricula.
Recommendation 3: Innovation as a Result of Multisectoral Collaboration
As society is becoming more interactive, contributions to innovation are a result of the sharing of knowledge and the transfer of know-how (Cavallini et al., 2016). This implies the need for collaboration between different entities with the global aim of creating an innovative society. The potential use of NIS as a source of new compounds is perfectly encapsulated using the quadruple helix model, where transdisciplinarity, rather than disciplinarity, is the dominant system for creating and organizing knowledge (Yawson, 2009). Hence, any mitigation measure should be an iterative result of transdisciplinary collaboration between the policy makers and the scientific community. Scientists are experts that can provide the know-how and propose mitigation strategies (possibly guided by the 8Rs model), while policy makers have the authoritative power over the legislative and financial barriers. Figure 4 shows how we envision the quadruple helix approach in practice. Often the initial demand for providing solutions for NIS removal/reuse will be created by the media and non-governmental organizations, who will inform the general public about the problem. This creates a demand for providing solutions, which is directed toward the experts (i.e., scientific and academic institutions). However, innovative solutions cannot be found by scientific collaboration alone, although collaborative networks need to be established. These should include other key actors from the quadruple helix: the policy makers who can directly change the legal framework, which can enable financing or dictate monitoring activities (i.e., either through national monitoring campaigns or through national and international ecological scientific collaborations). Policy makers also provide the legal basis and the financial support to tailor solutions (e.g., usually by financing relevant collaborative projects), as well as the constraints (e.g., quality assurance and trademarking) before any products enter the target markets. Importantly, any innovation inherently includes industrial partners who are in need of fresh ideas and innovative knowledge, for prototypes/ideas that can eventually be used and commercialized. This will directly contribute to (international) economic development and prosperity. Last but not least, we are stepping into the age of proactive involvement of citizens, and hence closing the circle of innovative society, as illustrated in Figure 4 (full black lines as the first step; dashed blue lines as the second step). Citizens can directly contribute to scientific knowledge through their involvement as individuals or within environmental non-governmental organizations. By organizing awareness raising and educational campaigns, the scientific community can empower citizens with knowledge and tools to monitor and report on sightings of individual NIS. Their feedback is typically invaluable for data for statistical models and to increase knowledge of the abundance and seasonal trends of NIS. Moreover, citizens can be included in large clean-up campaigns, and give their potential user feedback on new products, which represents a milestone in pre-commercialization stages of product development. Importantly, the involvement of each of the individual quadruple helix actors is not mutually exclusive; i.e., a representative from the industrial sector can commercialize recycled items and be included in the NIS recognition activities as a citizen scientist.
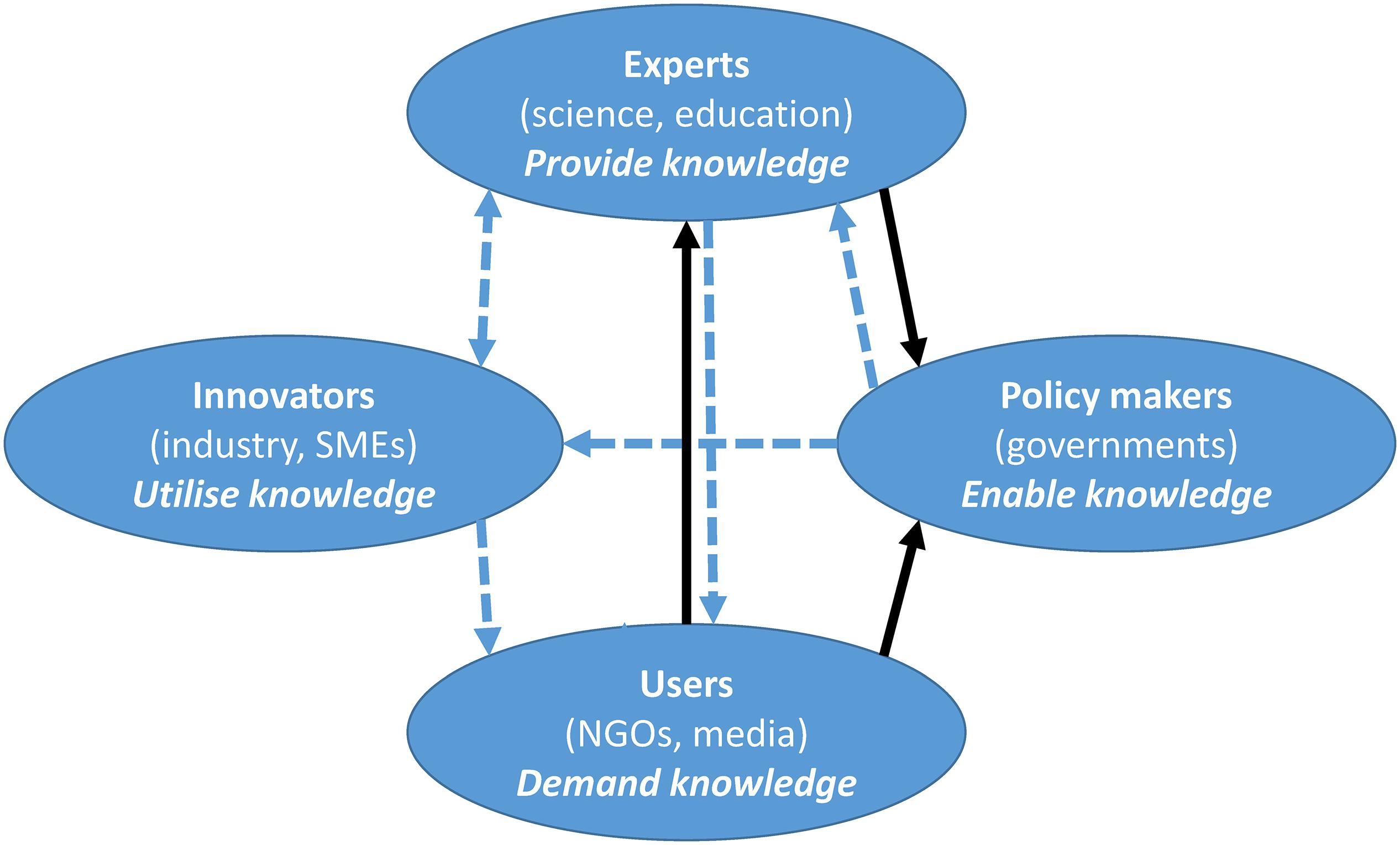
Figure 4. The quadruple helix approach for using non-indigenous species as a source is a two-step process. The first step: black arrows; second step: blue arrows. Communication flow (from-to) is represented by the direction of the arrows. SMEs, small and medium-sized enterprises; NGOs, non-governmental organizations.
Recommendation 4: Possible Mitigation Strategies for Non-indigenous Species in the Mediterranean
An important result of this study introduces the 8Rs model for NIS management, with the definition of possible uses and preventive actions to reduce the introduction and/or diffusion of NIS (Figure 1). There are two levels of the possible intervention measures (Figure 3, left): prevention measures (which encompass the first half of the 8Rs model), and mitigation measures (which encompass the second half of the 8Rs model). While the first step (i.e., Recognize, Reduce, and Replace) has traditionally been given the most amount of financing (73% overall; Table 1 and Figure 2), real innovations are expected to arise from innovative collaborations for the NIS mitigation activities (i.e., Reuse, Recycle, Recover/Restore, and Remove). Currently about a quarter of the overall financing (27%; Table 1 and Figure 2) has enabled mitigation ideas to emerge. We are, however, convinced that in the next 10 years, financed collaborative efforts will emerge that will use NIS to provide and subsequently commercialize prototypes, or to identify new removal strategies that will be economically viable and environmentally sustainable. The future Framework Program (Horizon Europe, the main European international scientific financing mechanism) is indeed allocating a significant proportion of resources to build an innovative and competitive Europe (i.e., with the Mediterranean Sea included). Importantly, our proposed 8Rs model can be extended and iterated to offer solutions for other modern challenges (e.g., plastic pollution, where Reduce, Reuse, Recycle, Remove, Recover, Regulate and additionally Refill, Return, and Repair Rs can be added as mitigation strategies). This strategic 8Rs framework can therefore directly contribute to many of the global challenges and strategies, and in particular to the UN 2030 agenda for sustainable development, which addresses societal challenges (e.g., environmental protection) and promotes resource efficiency by financing and implementing innovative actions that will in the long run build toward global economic growth.
Case Study – Potential Application of 8Rs Model on a Realistic Example
We selected two well-known non-indigenous macroalge in the Mediterranean Sea to provide example of conducted mitigation strategies in relation to the unexploited potential of the 8Rs model: the brown Undaria pinnatifida and the green Caulerpa taxifolia. In both instances, the common mitigation strategy was the manual removal. However, in both cases, the removal strategy was not 100% efficient and eventually these species recolonized the affected sites. Importantly, as seen in Figure 3, the mitigation strategies began at step 3. In absence of organized mitigation frameworks, this step was not effective. If our 8Rs model should be implemented as a standard regulatory framework, the mitigation would have started earlier, at steps 1 and 2 – Recognize and Regulate, which could potentially prevent the environmental and economic damage of these invasive species. Regular monitoring campaigns, which are compliant with legislations (such as MSFD, Ballast Water Management Convention and national legislations) should be enhanced in areas that are under threat for establishment of NIS. Besides removal strategies, step 3 also offers other potential mitigation measures – Reuse and Recycle. C. taxifolia was reused as a fertilizer for oil crops, pulses and cereals (Misal and Sabale, 2016), while U. pinnatifida could be used as a functional food (Cherry et al., 2019). Both species can be also recycled. C. taxifolia synthesizes caulerpenyne, an acetylenic sesquiterpene with antifouling activity against bacteria and could be applied for Removal strategies as well (Barzkar et al., 2019). U. pinnatifida is rich in natural compounds such as polyphenols, peptides, and polysaccharides, all of which have anticancer, antioxidant, anti-inflammatory, and anti-diabetes properties (Wang et al., 2018).
Importantly, Figure 2 and the lower amount of investment confirms that we are at the early stages of turning the pests into opportunities and solutions (Recycle and Reuse). However, as globally there is the trend to stimulate innovations, we are confident that the Recycle and Reuse steps will have a more significant share among the 8 Rs.
Conclusion
Any innovation is an iterative collaboration between industry, research, and academia, which is also often applied through public–private partnerships that link basic and applied research to the market, via technology transfer and commercialization mechanisms (Carayannis and Campbell, 2012). Only a team of transdisciplinary actors will have the joint capacity to promote global sustainable development by orchestrating innovative collaborations, possibly taking into consideration this 8Rs model. Both experts and innovators should constantly inform, educate and update potential users on the status of their research, and get feedback from the same potential users. Importantly, the way that research is communicated by the media influences every innovation system, to highlight new discoveries and innovations that will improve the eco-innovation toward its final commercialization (Yawson, 2009; Carayannis and Campbell, 2012).
Data Availability Statement
All datasets generated for this study are included in the article/Supplementary Material.
Author Contributions
AR conceived the study and wrote the first draft of the manuscript. MO-B, KK, PM, and JF iterated the study concept, based on their individual areas of expertise, and wrote sections of the manuscript. All authors contributed to the manuscript revision, and have read and approved the submitted version.
Funding
The publication is part of a project that has received funding from the European Union’s Horizon 2020 Research and Innovation Programme under grant agreement No. 774499 – GoJelly project. The authors also acknowledge financial support from the Slovenian Research Agency (Research Core Funding No. P1-0237).
Conflict of Interest
The authors declare that the research was conducted in the absence of any commercial or financial relationships that could be construed as a potential conflict of interest.
Supplementary Material
The Supplementary Material for this article can be found online at: https://www.frontiersin.org/articles/10.3389/fmars.2020.00178/full#supplementary-material
TABLE S1 | Gross domestic expenditure on R&D (GERD) as a percentage of GDP. Data taken from the database available from the UNESCO Institute for Statistics.
Footnotes
- ^https://cosmetics.specialchem.com/inci/undaria-pinnatifida-extract; https://centifoliabio.fr/en/content/187-undaria-pinnatifida-undaria-pinnatifida
References
Al-Madfa, H. M., Abdel-Moati, A. R., and Al-Gimaly, F. H. (1998). Pinctada radiata (pearl oyster): a bioindicator for metal pollution monitoring in the qatari waters. Bull. Environ. Contam. Toxicol. 60, 245–251. doi: 10.1007/s001289900617
Ashley, M., Pahl, S., Glegg, G., and Fletcher, S. (2019). A change of mind: applying social and behavioral research methods to the assessment of the effectiveness of ocean literacy initiatives. Front. Mar. Sci. 6:288. doi: 10.3389/fmars.2019.00288
Azzurro, E., Sbragaglia, V., Cerri, J., Bariche, M., Bolognini, L., Ben Souissi, J., et al. (2019). Climate change, biological invasions, and the shifting distribution of Mediterranean fishes: a large−scale survey based on local ecological knowledge. Global Change Biol. 25, 2779–2792. doi: 10.1111/gcb.14670
Barzkar, N., Tamadoni Jahromi, S., Bolooki Poorsaheli, H., and Vianello, F. (2019). Metabolites from marine microorganisms, micro, and macroalgae: immense scope for pharmacology. Mar. Drugs 17:464. doi: 10.3390/md17080464
Battelli, C., and Rindi, F. (2008). The extensive development of the turf-forming red alga Womersleyella setacea (Hollenberg) R. E. Norris (Rhodophyta, Ceramiales) in the Bay of Boka Kotorska, Montenegro (southern Adriatic Sea). Plant Biosyst. 142, 120–125. doi: 10.1080/11263500701872747
Bellard, C., Cassey, P., and Blackburn, T. M. (2016). Aliens species as a driver of recent extinctions. Biol. Lett. 12:20150623. doi: 10.1098/rsbl.2015.0623
Bitar, G., Ramos-Esplá, A. A., Ocaña, O., Sghaier, Y. R., Forcada, A., Valle, C., et al. (2017). The introduced marine macroflora of Lebanon and its distribution on the Levantine coast. Mediterr. Mar. Sci. 18, 138–155.
Bleve, G., Ramires, F. A., Gallo, A., and Leone, A. (2019). Identification of safety and quality parameters for preparation of jellyfish-based novel food products. Foods 8:263. doi: 10.3390/foods8070263
Boero, F., Putti, M., Trainito, E., Prontera, E., Piraino, S., and Shiganova, T. A. (2009). First records of Mnemiopsis leidyi (Ctenophora) from the Ligurian, Tyrrhenian and Ionian Seas (western Mediterranean) and first record of Phyllorhiza punctata (Cnidaria) from the western Mediterranean. Aquat. Invas. 4, 675–680. doi: 10.3391/ai.2009.4.4.13
Bonanno, G., and Orlando-Bonaca, M. (2019). Non-indigenous marine species in the Mediterranean Sea – myth and reality. Environm. Sci. Policy 96, 123–131. doi: 10.1016/j.envsci.2019.03.014
Borja, A., Elliott, M., Snelgrove, P. V. R., Austen, M. C., Berg, T., Cochrane, S., et al. (2016). Bridging the gap between policy and science in assessing the health status of marine ecosystems. Front. Mar. Sci. 3:175. doi: 10.3389/fmars.2016.00175
Bulleri, F., Bruno, J. F., and Benedetti-Cecchi, L. (2008). Beyond competition: incorporating positive interactions between species to predict ecosystem invasibility. PLoS Biol. 6:e162. doi: 10.1371/journal.pbio.0060162
Carayannis, E. G., and Campbell, D. F. J. (2012). “Mode 3 knowledge production in quadruple helix innovation systems,” in Mode 3 knowledge Production in Quadruple Helix Innovation Systems, eds E. G. Carayannis and D. F. J. Campbell (New York, NY: Springer), 1–63. doi: 10.1007/978-1-4614-2062-0_1
Carlton, J. T., and Geller, J. B. (1993). Ecological roulette: the global transport of non-indigenous marine organisms. Science 261, 78–82. doi: 10.1126/science.261.5117.78
Cavallini, S., Soldi, R., Friedl, J., and Volpe, M. (2016). Using the Quadruple Helix Approach to Accelerate the Transfer of Research and Innovation Results to Regional Growth. European Union – Committee of the Regions. Available online at: https://cor.europa.eu/en/engage/studies/Documents/quadruple-helix.pdf (accessed August 1, 2019).
CBD (1993). Convention on Biological Diversity (With Annexes), Vol. 1760. (Rio de Janeiro: United Nations–Treaty Series), 142–382. (accessed June 5, 1992).
CBD (2014). Convention on Biological Diversity. Pathways of Introduction of Invasive Species, Their Prioritization and Management. UNEP/CBD/SBSTTA/18/9/Add.1, Subsidiary Body on Scientific, Technical and Technological Advice. Available online at: https://www.cbd.int/doc/meetings/sbstta/sbstta-18/official/sbstta-18-09-add1-en.pdf (accessed September 24, 2019).
César de Sá, N., Marchante, H., Marchante, E., Cabral, J. A., Honrado, J. P., et al. (2019). Can citizen science data guide the surveillance of invasive plants? A model-based test with Acacia trees in Portugal. Biol. Invas. 21, 2127–2141. doi: 10.1007/s10530-019-01962-6
Chan, F. T., MacIsaac, H. J., and Bailey, S. A. (2015). Relative importance of vessel hull fouling and ballast water as transport vectors of non-indigenous species to the Canadian Arctic. Can. J. Fish. Aquat. Sci. 72, 1–13.
Cherry, P., O’Hara, C., Magee, P. J., McSorley, E. M., and Allsopp, P. J. (2019). Risks and benefits of consuming edible seaweeds. Nutr. Rev. 77, 307–329. doi: 10.1093/nutrit/nuy066
Cook, E. J., Payne, R. D., Macleod, A. K., and Brown, S. F. (2016). Marine biosecurity: protecting indigenous marine species. Res. Rep. Biodiv. Stud. 5, 1–14.
Corriero, G., Pierri, C., Accoroni, S., Alabiso, G., Bavestrello, G., Barbone, E., et al. (2016). Ecosystem vulnerability to alien and invasive species: a case study on marine habitats along the Italian coast. Aquat. Conserv. Mar. Freshw. Ecosys. 26, 392–409.
Dankers, N., Meijboom, A., De, Jong M, Dijkman, E., Cremer, J., Fey, F., et al. (2006). “The Development of the Japanese Oyster in The Netherlands (Wadden Sea and Oosterschelde) IMARES Rapport”. Report, No. (C040/06). Wageningen: Wageningen IMARES Institute for Marine Resources and Ecosystem Studies.
Dehnen-Schmutz, K., Boivin, T., Essl, F., Groom, Q. J., Harrison, L., Touza, J. M., et al. (2018). Alien futures: what is on the horizon for biological invasions? Divers. Distr. 24, 1149–1157. doi: 10.1111/ddi.12755
Deudero, S., Blanco, A., Box, A., Mateu-Vicens, G., Cabanellas-Reboredo, M., and Sureda, A. (2010). Interaction between the invasive macroalga Lophocladia lallemandii and the bryozoan Reteporella grimaldii at seagrass meadows: density and physiological responses. Biol. Invas. 12, 41–52. doi: 10.1007/s10530-009-9428-1
Douglas, H., Dang, P. T., Gill, B. D., Huber, J., Mason, P. G., Parker, D. J., et al. (2009). The importance of taxonomy in responses to invasive alien species. Biodiversity 10, 92–99. doi: 10.1080/14888386.2009.9712850
Dulčić, J., Dragičević, B., and Lipej, L. (2010). New record of the blue crab. Callinectes sapidus Rathbun, 1896, (Decapoda: Brachyura) in the Adriatic Sea. Annal. Ser. Hist. Nat. 20, 23–28.
EC (2008). Directive 2008/56/EC of the European Parliament and of the Council of 17 June 2008 establishing a framework for community action in the field of marine environmental policy (Marine Strategy Framework Directive). Off. J. Eur. Union L 164, 19–40.
Elliott, M. (2003). Biological pollutants and biological pollution–an increasing cause for concern. Mar. Pollut. Bull. 46, 275–280. doi: 10.1016/s0025-326x(02)00423-x
Emadodin, I., Reinsch, T., Rotter, A., Orlando-Bonaca, M., Taube, F., and Javidpour, J. (2020). A perspective on the potential of using marine organic fertilizers for the sustainable management of coastal ecosystem services. Environ. Sustain. doi: 10.1007/s42398-020-00097-y
Epstein, G., Hawkins, S. J., and Smale, D. A. (2018). Removal treatments alter the recruitment dynamics of a global marine invader – implications for management feasibility. Mar. Environ. Res. 140, 322–331. doi: 10.1016/j.marenvres.2018.06.022
European Alien Species Information Network [EASIN] (2019). European Commission – Joint Research Centre. European Alien Species Information Network. Available online at: https://easin.jrc.ec.europa.eu/ (accessed August 2, 2019).
Freiwald, J. Meyer, R., Caselle, J. E., Blanchette, C. A., Hovel, K., Neilson, D., et al. (2018). Citizen science monitoring of marine protected areas: Case studies and recommendations for integration into monitoring programs. Mar. Ecol. 39:e12470. doi: 10.1111/maec.12470
Galil, B., Froglia, C., and Noël, P. (2002). “CIESM atlas of exotic species in the mediterranean,” in Crustaceans: Decapods and Stomatopods, Vol. 2, ed. F. Briand (Monaco: CIESM Publishers), 192.
Galil, B., Kress, N., and Shiganova, T. A. (2009). First record of Mnemiopsis leidyi A. Agassiz, 1865 (Ctenophora; Lobata; Mnemiidae) off the Mediterranean coast of Israel. Aquat. Invas. 4, 357–360. doi: 10.3391/ai.2009.4.2.8
Galil, B., Marchini, A., Occhipinti-Ambrogi, A., and Ojaveer, H. (2017). The enlargement of the Suez Canal–Erythraean introductions and management challenges. Manag. Biol. Inv. 8, 141–152. doi: 10.3391/mbi.2017.8.2.02
Galil, B. S. (2006). “The marine caravan – the Suez Canal and the Erythrean invasion,” in Bridging Divides: Maritime Canals as Invasion Corridors. Monographiae Biologicae 83, eds S. Gollasch, B. S. Galil, and A. N. Cohen (Dordrecht: Springer), 207–300. doi: 10.1007/978-1-4020-5047-3_6
Galil, B. S. (2011). “The alien crustaceans in the Mediterranean Sea: a historical review,” in In the Wrong Place - Alien Marine Crustaceans: Distribution, Biology and Impacts, Invading Nature - Springer Series in Invasion Ecology, Vol. 6, eds B. S. Galil, P. F. Clark, and J. T. Carlton (Springer: Dordrecht), 377–402. doi: 10.1007/978-94-007-0591-3_13
Galil, B. S., Marchini, A., and Occhipinti-Ambrogi, A. (2016). East is east and West is west? Management of marine bioinvasions in the Mediterranean Sea. Estuar. Coast. Shelf Sci. 201, 7–16. doi: 10.1016/j.ecss.2015.12.021
Gallardo, B., Clavero, M., Sánchez, M. I., and Vilà, M. (2016). Global ecological impacts of invasive species in aquatic ecosystems. Glob. Chang. Biol. 22, 151–163. doi: 10.1111/gcb.13004
Garcia, L., Pinya, S., Colomar, V., París, T., Puig, M., Rebassa, M., et al. (2018). The first recorded occurrences of the invasive crab Callinectes sapidus Rathbun, 1896 (Crustacea: Decapoda: Portunidae) in coastal lagoons of the Balearic Islands (Spain). Bioinvas. Rec. 7, 191–196. doi: 10.3391/bir.2018.7.2.12
Giakoumi, S., Katsanevakis, S., Albano, P. G., Azzurro, E., Cardoso, A. C., Cebrian, E., et al. (2019). Management priorities for marine invasive species. Sci. Total Environ. 688, 976–982. doi: 10.1016/j.scitotenv.2019.06.282
Giovos, I., Kleitou, P., Poursanidis, D., Batjakas, I., Bernardi, G., Crocetta, F., et al. (2019). Citizen-science for monitoring marine invasions and stimulating public engagement: a case project from the eastern Mediterranean. Biol. Invas. 21, 3707–3721. doi: 10.1007/s10530-019-02083-w
Gollasch, S., and Leppäkoski, E. (1999). Initial Risk Assessment of Alien Species in Nordic Coastal Waters. Copenhagen: Nordic Council of Ministers, 1–124.
Gómez, F. (2008). Phytoplankton invasions: comments on the validity of categorizing the non-indigenous dinoflagellates and diatoms in European Seas. Mar. Pollut. Bull. 56, 620–628. doi: 10.1016/j.marpolbul.2007.12.014
Gómez, F. (2019). Comments on the non-indigenous microalgae in the European seas. Mar. Pollut. Bull. 148, 1–2. doi: 10.1016/j.marpolbul.2019.07.048
González-Moreno, P., Lazzaro, L., Vila, M., Preda, C., Adriaens, T., Bacher, S., et al. (2019). Consistency of impact assessment protocols for non-native species. Neobiota 44, 1–25.
HELCOM (2018). Trends in Arrival of New Non-Indigenous Species. HELCOM Core Indicator Report. Online. Available online at: https://helcom.fi/wp-content/uploads/2019/08/Trends-in-arrival-of-new-non-indigenous-species-HELCOM-core-indicator-2018.pdf (accessed February 25, 2020).
Hildebrand, M., Davis, A. K., Smith, S. R., Traller, J. C., and Abbriano, R. (2012). The place of diatoms in the biofuel industry. Biofuels 3, 221–240. doi: 10.4155/bfs.11.157
IMO (2001). International Convention on the Control of Harmful Anti-Fouling Systems on Ships. London: International Maritime Organization.
IMO (2004). International Convention for the Control and Management of Ships’ Ballast Water and Sediments. London: International Maritime Organization.
Iveša, L., Djakovac, T., and Devescovi, M. (2015). Spreading patterns of the invasive Caulerpa cylindracea Sonder along the west Istrian coast (northern Adriatic Sea, Croatia). Mar. Environ. Res. 107, 1–7. doi: 10.1016/j.marenvres.2015.03.008
Katsanevakis, S., and Moustakas, A. (2018). Uncertainty in marine invasion science. Front. Mar. Sci. 5:38. doi: 10.3389/fmars.2018.00038
Katsanevakis, S., Tempera, F., and Teixeira, H. (2016). Mapping the impact of alien species on marine ecosystems: the Mediterranean Sea case study. Divers. Distrib. 22, 694–707. doi: 10.1111/ddi.12429
Katsanevakis, S., Wallentinus, I., Zenetos, A., Leppäkoski, E., Çinar, M. E., Oztürk, B., et al. (2014). Impacts of marine invasive alien species on ecosystem services and biodiversity: a pan-European review. Aquat. Invas. 9, 391–423. doi: 10.3391/ai.2014.9.4.01
Katsanevakis, S., Zenetos, A., Belchior, C., and Cardoso, A. C. (2013). Invading European seas: assessing pathways of introduction of marine aliens. Ocean Coast. Manag. 76, 64–74. doi: 10.1016/j.ocecoaman.2013.02.024
Keller, R. P., Geist, J., Jeschke, J. M., and Kühn, I. (2011). Invasive species in Europe: ecology, status, and policy. Environm. Sci. Eur. 23:23.
Kerckhof, F., Haelters, J., and Gollasch, S. (2007). Alien species in the marine and brackish ecosystem: the situation in Belgian waters. Aquat. Invas. 2, 243–257. doi: 10.3391/ai.2007.2.3.9
Kettunen, M., Genovesi, P., Gollasch, S., Pagad, S., Starfinger, U., ten Brink, P., et al. (2008). Technical Support to EU Strategy on Invasive Species (IAS) – Assessment of the Impacts of IAS in Europe and the EU (Final Module Report for the European Commission). Brussels: Institute for European Environmental Policy (IEEP), 44.
Kideys, A. E. (2002). Fall and rise of the Black Sea ecosystem. Science 297, 1482–1484. doi: 10.1126/science.1073002
Kim, H., Ra, C. H., and Kim, S.-K. (2013). Ethanol production from seaweed (Undaria pinnatifida) using yeast acclimated to specific sugars. Biotech. Bioproc. Engin. 18, 533–537. doi: 10.1007/s12257-013-0051-8
Kong, N., Li, Q., Yu, H., and Kong, L.-F. (2015). Heritability estimates for growth-related traits in the Pacific oyster (Crassostrea gigas) using a molecular pedigree. Aquac. Res. 46, 499–508. doi: 10.1111/are.12205
Kružić, P., Žuljević, A., and Nikolić, V. (2008). The highly invasive alga Caulerpa racemosa var. cylindracea poses a new threat to the banks of the coral Cladocora caespitosa in the Adriatic Sea. Coral Reefs 27:441. doi: 10.1007/s00338-008-0358-7
Lassus, P., Chomérat, N., Hess, P., and Nézan, E. (2016). Toxic and Harmful Microalgae of the World Ocean. IOC Manuals and guides, 68. International Society for the Study of Harmful Algae. Denmark: Intergovernmental Oceanographic Commission of UNESCO.
Leese, F., Altermatt, F., Bouchez, A., Ekrem, T., Hering, D., Meissner, K., et al. (2016). DNAqua-Net: developing new genetic tools for bioassessment and monitoring of aquatic ecosystems in Europe. Res. Ideas Outc. 2:e11321. doi: 10.3897/rio.2.e11321
Lehtiniemi, M., Ojaveer, H., David, M., Galil, B., Gollasch, S., Mckenzie, C., et al. (2015). Dose of truth – monitoring marine non-indigenous species to serve legislative requirements. Mar. Pol. 54, 26–35. doi: 10.1016/j.marpol.2014.12.015
Lejeusne, C., Chevaldonne, P., Pergent-Martini, C., Boudouresque, C. F., and Perez, T. (2010). Climate change effects on a miniature ocean: the highly diverse, highly impacted Mediterranean Sea. Trends Ecol. Evol. 25, 250–260. doi: 10.1016/j.tree.2009.10.009
Leone, A., Lecci, R. M., Milisenda, G., and Piraino, S. (2019). Mediterranean jellyfish as novel food: effects of thermal processing on antioxidant, phenolic, and protein contents. Eur. Food Res. Technol. 245, 1611–1627. doi: 10.1007/s00217-019-03248-6
Lipej, L., Acevedo, I., Akel, E. H. K., Anastapoulou, A., Angelidis, A., Azzurro, E., et al. (2017). New Mediterranean biodiversity records (March 2017). Mediterr. Mar. Sci. 18, 179–201. doi: 10.12681/mms.2068
Lučić, P., Žuljević, A., and Antolić, B. (2017). “First record of the alien red alga Lophocladia lallemandii in Croatian part of Adriatic Sea,” in Proceedings of the Abstract book of the 52nd European Marine Biology Symposium, ed. A. Ramšak et al. (Piran: National Institute of Biology, Marine Biology Station), 160.
Maeda, Y., Nojima, D., Yoshino, T., and Tanaka, T. (2017). Structure and properties of oil bodies in diatoms. Phil. Trans. R. Soc. B 372:20160408. doi: 10.1098/rstb.2016.0408
Malej, A., Tirelli, V., Lučić, D., Paliaga, P., Vodopivec, M., Goruppi, A., et al. (2017). Mnemiopsis leidyi in the northern Adriatic: here to stay? J. Sea Res. 124, 10–16. doi: 10.1016/j.seares.2017.04.010
Manfrin, C., Souty-Grosset, C., Anastácio, P., Reynolds, J., and Giulianini, P. G. (2018). “The apparently relentless spread of the major decapod alien species in the mediterranean basin and european inland waters,” in Histories of Bioinvasions in the Mediterranean. Environmental History, Vol. 8, eds A. Queiroz and S. Pooley (Cham: Springer), 51–86. doi: 10.1007/978-3-319-74986-0_3
Mannino, A. M., Balistreri, P., and Deidun, A. (2017). “The marine biodiversity of the Mediterranean Sea in a changing climate: the impact of biological invasions,” in Mediterranean Identities – Environment, Society, Culture, ed. B. Fuerst-Bjelis (London: Intech), 101–127.
Marbuah, G., Gren, I. M., and McKie, B. (2014). Economics of harmful invasive species: a review. Diversity 6, 500–523. doi: 10.3390/d6030500
Marchini, A., Ferrario, J., Sfriso, A., and Occhipinti-Ambrogi, A. (2015). Current status and trends of biological invasions in the Lagoon of Venice, a hotspot of marine NIS introductions in the Mediterranean Sea. Biol. Invas. 17, 2943–2962. doi: 10.1007/s10530-015-0922-3
Martínez, M. L., Intralawan, A., Vázquez, G., Pérez-Maqueo, O., Sutton, P., and Landgrave, R. (2007). The coasts of our world: ecological, economic and social importance. Ecol. Econo. 63, 254–272. doi: 10.1016/j.ecolecon.2006.10.022
Meinesz, A., Belsher, T., Thibaut, T., Antolic, B., Ben Mustapha, K., Boudouresque, C.-F., et al. (2001). The introduced green alga Caulerpa taxifolia continues to spread in the Mediterranean. Biol. Inva. 3, 201–210.
Misal, B. N., and Sabale, A. B. (2016). Influence of seaweed liquid fertilizer (SLF) on seed germination and seedling growth of some crop plants. Indian J. Appl. Res. 6, 505–508.
Molnar, J. L., Gamboa, R. L., Revenga, C., and Spalding, M. D. (2008). Assessing the global threat of invasive species to marine biodiversity. Front. Ecol. Env. 6:485–492. doi: 10.1890/070064
Mozetič, P., Cangini, M., Francé, J., Bastianini, M., Bernardi Aubry, F., Bužančić, M., et al. (2019). Phytoplankton diversity in Adriatic ports: lessons from the port baseline survey for the management of harmful algal species. Mar. Pollut. Bull. 147, 117–132. doi: 10.1016/j.marpolbul.2017.12.029
Nolan, C. (1994). Introduced Species in European Coastal Waters. Report on an International Workshop. EC, eds C. F. Boudouresque, F. Briand, and C. Nolan (Luxemburg: European Commissio), 1–3.
Occhipinti-Ambrogi, A., and Savini, D. (2003). Biological invasions as a component of global change in stressed marine ecosystems. Mar. Pollut. Bull. 46, 542–551. doi: 10.1016/s0025-326x(02)00363-6
Ojaveer, H., Galil, B. S., Campbell, M. L., Carlton, J. T., Canning-Clode, J., Cook, E. J., et al. (2015). Classification of non-indigenous species based on their impacts: considerations for application in marine management. PLoS Biol. 13:e1002130. doi: 10.1371/journal.pbio.1002130
Ojaveer, H., Galil, B. S., Carlton, J. T., Alleway, H., Goulletquer, P., Lehtiniemi, M., et al. (2018). Historical baselines in marine bioinvasions: implications for policy and management. PLoS One 13:e0202383. doi: 10.1371/journal.pone.0202383
Ojaveer, H., Galil, B. S., Minchin, D., Olenin, S., Amorim, A., Canning-Clode, J., et al. (2014). Ten recommendations for advancing the assessment and management of non-indigenous species in marine ecosystems. Mar. Policy 44, 160–165. doi: 10.1016/j.marpol.2013.08.019
Olenin, S., Elliott, M., Bysveen, B., Culverhouse, P. F., Daunys, D., Dubelaar, G. B. J., et al. (2011). Recommendations on methods for the detection and control of biological pollution in marine coastal waters. Mar. Pollut. Bull. 62, 2598–2604. doi: 10.1016/j.marpolbul.2011.08.011
Orlando-Bonaca, M., Lipej, L., and Bonanno, G. (2019). Non-indigenous macrophytes in Adriatic ports and transitional waters: Trends, taxonomy, introduction vectors, pathways and management. Mar. Pollut. Bull. 145, 656–672. doi: 10.1016/j.marpolbul.2019.06.065
Otero, M., Cebrian, E., Francour, P., Galil, B., and Savini, D. (2013). Monitoring Marine Invasive Species in Mediterranean Marine Protected Areas (MPAs): A Strategy and Practical Guide for Managers. Malaga: IUCN, 136.
Palialexis, A., Tornero, V., Barbone, E., Gonzalez, D., Hanke, G., Cardoso, A. C., et al. (2014). In-Depth Assessment of the EU Member States’ Submissions for the Marine Strategy Framework Directive under articles 8, 9 and 10. Report EUR 26473 EN. Brussels: European Union.
Petrocelli, A., Antolić, B., Bolognini, L., Cecere, E., Cvitković, I., Despalatović, M., et al. (2019). Port baseline biological surveys and seaweed bioinvasions in port areas: what’s the matter in the Adriatic Sea? Mar. Pollut. Bull. 147, 98–116. doi: 10.1016/j.marpolbul.2018.04.004
Pyšek, P., Blackburn, T. M., García-Berthou, E., Perglová, I., and Rabitsch, W. (2017). “Displacement and local extinction of native and endemic species,” in Impact of Biological Invasions on Ecosystem Services, eds M. Vilà and P. E. Hulme (Cham: Springer International Publishing), 157–175. doi: 10.1007/978-3-319-45121-3_10
Rilov, G., Benayahu, Y., and Gasith, A. (2004). Prolonged lag in population outbreak of an invasive mussel: a shifting-habitat model. Biol. Invas. 6, 347–364. doi: 10.1023/b:binv.0000034614.07427.96
Rindi, F., Guiry, M. D., and Cinelli, F. (1999). Morphology and reproduction of the adventive Mediterranean rhodophyte Polysiphonia setacea. Hydrobiologia 398/399, 91–100. doi: 10.1007/978-94-011-4449-0_11
Roh, M.-K., Uddin, M. S., and Chun, B.-S. (2008). Extraction of fucoxanthin and polyphenol from Undaria pinnatifida using supercritical carbon dioxide with co-solvent. Biotechnol. Bioproc. Engin. 13, 724–729. doi: 10.1007/s12257-008-0104-6
Sardain, A., Sardain, E., and Leung, B. (2019). Global forecasts of shipping traffic and biological invasions to 2050. Nat. Sustainab. 2, 274–282. doi: 10.1038/s41893-019-0245-y
Savini, D., Occhipinti-Ambrogi, A., Marchini, A., Tricarico, E., Gherardi, F., Olenin, S., et al. (2010). The top 27 animal alien species introduced into Europe for aquaculture and related activities. J. Appl. Ichthyol. 26, 1–7. doi: 10.1111/j.1439−0426.2010.01503.x
Shiganova, T. A., Christou, E. D., Bulgakova, J. V., Siokou-Frangou, I., Zervoudaki, S., and Siapatis, A. (2004). “Distribution and biology of Mnemiopsis leidyi in the northern Aegean Sea, and comparison with the indigenous Bolinopsis vitrea,” in Aquatic Invasions in the Black, Caspian, and Mediterranean Seas. Nato Science Series: IV: Earth and Environmental Sciences (IV: Earth and Environmental Science), Vol. 35, eds H. Dumont, T. A. Shiganova, and U. Niermann (Dordrecht: Springer), 113–135. doi: 10.1007/1-4020-2152-6_4
Shiganova, T. A., Legendre, L., Kazmin, A. S., and Nival, P. (2014). Interactions between invasive ctenophores in the Black Sea: assessment of control mechanisms based on long-term observations. Mar. Ecol. Prog. Ser. 507, 111–123. doi: 10.3354/meps10806
Smith, C. J., Dailianis, T., Papadopoulou, K.-N., Gerovasileiou, V., Sevastou, K., Grehan, A., et al. (2017). Current Marine Pressures and Mechanisms Driving Changes in Marine Habitats. Deliverable 1.2, MERCES Project. 102 pp, Incl. Two Annexes. Available online at: http://www.merces-project.eu/sites/default/files/MERCES_D1.2_Pressures_Final_25052017.pdf (accessed September 24, 2019).
Stabili, L., Fraschetti, S., Acquaviva, M. I., Cavallo, R. A., De Pascali, S. A., Fanizzi, F. P., et al. (2016). The potential exploitation of the Mediterranean invasive alga Caulerpa cylindracea: can the invasion be transformed into a gain? Mar. Drugs 14:210. doi: 10.3390/md14110210
Streftaris, N., and Zenetos, A. (2006). Alien marine species in the Mediterranean the 100 ‘worst invasives and their impact. Mediterr. Mar. Sci. 7, 87–118.
Torres, M. D., Kraan, S., and Domínguez, H. (2019). Seaweed biorefinery. Rev. Environm. Sci. Bio. Technol. 18:335. doi: 10.1007/s11157-019-09496-y
Troost, K. (2010). Causes and effects of a highly successful marine invasion: case-study of the introduced Pacific oyster Crassostrea gigas in continental NW European estuaries. J. Sea Res. 64, 145–165. doi: 10.1016/j.seares.2010.02.004
Tsiamis, K., Palialexis, A., Stefanova, K., Ničević Gladan, Ž, Skejić, S., Despalatović, M., et al. (2019). Non-indigenous species refined national baseline inventories: a synthesis in the context of the European Union’s Marine Strategy Framework Directive. Mar. Poll. Bull. 145, 429–435. doi: 10.1016/j.marpolbul.2019.06.012
Tsiamis, K., Zenetos, A., Deriu, I., Gervasini, E., and Cardoso, A. C. (2018). The native distribution range of the European marine non-indigenous species. Aquat. Invas. 13, 187–198. doi: 10.3391/ai.2018.13.2.01
Ulman, A., Ferrario, J., Forcada, A., Arvanitidis, C., Occhipinti-Ambrogi, A., and Marchini, A. (2019). A hitchhiker’s guide to Mediterranean marina travel for alien species. J. Environm. Manag. 241, 328–339. doi: 10.1016/j.jenvman.2019.04.011
UNEP-MAP (2012). Support to the Barcelona Convention for the Implementation of the Ecosystem Approach, Including the Establishment of MPAs in Open Seas Areas, Including Deep Sea. Contribution Agreement N°21.0401/2008/519114/SUB/D2. Available online at: https://ec.europa.eu/environment/marine/international-cooperation/regional-sea-conventions/barcelona-convention/pdf/SBCI_ECAP_FinalReport.pdf (accessed February 21, 2020).
Verlaque, M., Ruitton, S., Mineur, F., and Boudouresque, C. F. (2015). “CIESM atlas of exotic species in the mediterranean,” in Macrophytes, Vol. 4, ed. F. Briand (Monaco: CIESM Publisher), 364.
Vinogradov, M. E., Shushkina, E. A., Musaeva, E. I., and Sorokin, P. Y. (1989). Ctenophore Mnemiopsis leidyi (A. Agassiz) (Ctenophora: Lobata) – new settlers in the Black Sea. Oceanology 29, 293–298.
von Ammon, U., Wood, S. A., Laroche, O., Zaiko, A., Tait, L., Lavery, S., et al. (2018). Combining morpho-taxonomy and metabarcoding enhances the detection of non-indigenous marine pests in biofouling communities. Sci. Rep. 8:16290. doi: 10.1038/s41598-018-34541-1
Wang, L., Park, Y.-J., Jeon, Y.-J., and Ryu, B. (2018). Bioactivities of the edible brown seaweed. Undaria pinnatifida: a review. Aquaculture 495, 873–880. doi: 10.3390/md9101806
Yawson, R. M. (2009). “The ecological system of innovation: a new architectural framework for a functional evidence-based platform for science and innovation policy,” in Proceedings of the XXIV ISPIM 2009 Conference: The Future of Innovation, Vienna.
Zenetos, A., Çinar, M. E., Crocetta, F., Golani, D., Rosso, A., Servello, G., et al. (2017). Uncertainties and validation of alien species catalogues: the Mediterranean as an example. Estuar. Coast. Shelf Sci. 191, 171–187. doi: 10.1016/j.ecss.2017.03.031
Zenetos, A., Gofas, S., Morri, C., Rosso, A., Violanti, D., García Raso, J. E., et al. (2012). Alien species in the Mediterranean Sea by 2012. a contribution to the application of european union’s marine strategy framework directive (MSFD). Part 2. introduction trends and pathways. Mediterr. Mar. Sci 13, 328–352.
Zenetos, A. M., Corsini-Foka, F., Crocetta, V., Gerovasileiou, P. K., Karachle, N., Simboura, K., et al. (2018). Deep cleaning of alien and cryptogenic species records in the Greek Seas (2018 update). Manag. Biol. Invas. 9, 209–226. doi: 10.3391/mbi.2018.9.3.04
Keywords: non-indigenous species, pollution mitigation, 8Rs model, quadruple helix, Mediterranean Sea, citizen science, communication, sustainability
Citation: Rotter A, Klun K, Francé J, Mozetič P and Orlando-Bonaca M (2020) Non-indigenous Species in the Mediterranean Sea: Turning From Pest to Source by Developing the 8Rs Model, a New Paradigm in Pollution Mitigation. Front. Mar. Sci. 7:178. doi: 10.3389/fmars.2020.00178
Received: 13 November 2019; Accepted: 06 March 2020;
Published: 24 March 2020.
Edited by:
Juan José Alava, University of British Columbia, CanadaReviewed by:
Dimitris Poursanidis, Foundation for Research & Technology - Hellas, GreeceAgnese Marchini, University of Pavia, Italy
Copyright © 2020 Rotter, Klun, Francé, Mozetič and Orlando-Bonaca. This is an open-access article distributed under the terms of the Creative Commons Attribution License (CC BY). The use, distribution or reproduction in other forums is permitted, provided the original author(s) and the copyright owner(s) are credited and that the original publication in this journal is cited, in accordance with accepted academic practice. No use, distribution or reproduction is permitted which does not comply with these terms.
*Correspondence: Ana Rotter, ana.rotter@nib.si