Cold-Water Soft Corals (Cnidaria: Nephtheidae) as Habitat for Juvenile Basket Stars (Echinodermata: Gorgonocephalidae)
- 1Northwest Atlantic Fisheries Center, Department of Fisheries and Oceans Canada, St. John’s, NL, Canada
- 2Freshwater Institute, Department of Fisheries and Oceans Canada, Winnipeg, MB, Canada
- 3ArcticNet, Québec Océan, Takuvik, Départment de Biologie, Université Laval, Québec, QC, Canada
Nephtheidae soft corals are a common component of benthic ecosystems in the Northwest (NW) Atlantic and in the Arctic, but little is known about their functional roles. Here we investigated their role as habitat for basket stars (Ophiuroidea). By examining over 4500 soft coral colonies of Drifa glomerata, Duva florida, Gersemia spp., and Pseudodrifa racemosa collected during trawl surveys in the regions of Newfoundland, Labrador and West Baffin Bay, we show that Nephtheidae soft corals are habitat for juvenile Gorgonocephalus sp. basket stars. Juvenile Gorgonocephalus sp. were found on all soft corals species, but Drifa glomerata was the soft coral species with the highest occurrence of associated juvenile basket stars (32%), with a maximum of 111 basket stars found on a single 4.8 cm tall (contracted state) colony. Individual basket star disc diameter did not change with colony height, but it varied with season for individuals found on D. glomerata. On the other hand, colony height was positively related with maximum disc diameter (D. glomerata), and presence and number of basket stars on a colony (D. glomerata and D. florida). One very young ophiuroid was found within one polyp of a D. florida colony collected in July. We also examined bottom trawl survey data from 2011 to 2017 from Newfoundland to Baffin Bay, and other locations in the Eastern Canadian Arctic and found that for our largest database, 45% of trawl sets that contained basket stars also contained corals, of which 67% were Nephtheidae soft corals. In this study we show that all four Nephtheidae soft coral taxa studied act as habitat for juvenile basket stars, which are conspicuous components of benthic communities in cold-water environments. Soft corals are commonly exposed and vulnerable to bottom fishing gear in the North Atlantic. We urge future studies evaluating the nature of this relationship, the potential role of soft corals as nurseries, and the potential degree of dependency/preference between basket stars and soft corals in relation to other organisms.
Introduction
The functional role of an organism can be defined as the functions provided to the ecosystem (Vaughn and Hakenkamp, 2008), such as ecosystem functions related to impacts on substrate, bentho-pelagic coupling, and associations with other organisms (e.g., provision of microhabitat) (Bell, 2008). The function of habitat provision might also be accompanied by other functions, such as protection against predation, substrate for settlement, and camouflage (Bell, 2008). Cold-water corals are well known for their habitat-provision potential, as they are often reported in association with other organisms. Gorgonians and black corals have drawn particular attention, as they can grow to large sizes and host a variety of organisms in symbiotic, parasitic, or simply epibiotic relationships (Wahl, 1999; Krieger and Wing, 2002; Buhl-Mortensen and Mortensen, 2004a,b, 2005; Mosher and Watling, 2009; Clippele et al., 2015; Girard et al., 2016). With the advent of imagery technologies, in situ evidence of conspicuous associations with cold-water corals have become more common. This is especially true for taxa that can attain large sizes like gorgonians, which are more noticeable from imagery than small taxa like Nephtheidae soft corals, which are relatively small (often < 15 cm tall), with flexible, soft bodies. In contrast to other octocorals, soft corals have even been considered unsuitable to host attached species (Buhl-Mortensen et al., 2010), and believed to not host symbionts, in comparison to their warm-water nephtheid counterparts (Watling et al., 2011).
Ophiuroids in the genus Gorgonocephalus are a good example of conspicuous organisms commonly reported in association with cold-water corals (Table 1), including the scleractinians Desmophyllum pertusum (published as Lophelia pertusa) (Jensen and Frederiksen, 1992; Rosenberg et al., 2005; Wheeler et al., 2005) and Madrepora oculata (Buhl-Mortensen et al., 2010), the stylasterid Errina antarctica (Försterra et al., 2017), the gorgonians Paragorgia arborea (Buhl-Mortensen and Mortensen, 2005; Metaxas and Davis, 2005; Buhl-Mortensen et al., 2010; Clippele et al., 2015), Paramuricea placomus (Rosenberg et al., 2005; Clippele et al., 2015), Primnoa spp. (Jensen and Frederiksen, 1992; Krieger and Wing, 2002; Wild et al., 2008; Clippele et al., 2015), and also sponges (Klitgaard, 1995). Adult Gorgonocephalus spp. can have disc diameters of 7–10 cm, and they can also be found as free-living organisms (Mortensen, 1927; Patent, 1970a). Juveniles, on the other hand, have been suggested to be strongly linked to cold-water Nephtheidae soft corals (hereinafter referred to as soft corals), as this association has been reported at several instances (e.g., Fedotov, 1924; Mortensen, 1927; Patent, 1970a; Haedrich and Maunder, 1984; Buhl-Mortensen and Mortensen, 2005; Buhl-Mortensen et al., 2017).
Fedotov (1924) and Patent (1970a) revealed that the relationship between juvenile basket stars and soft corals begins very early in the basket stars’ ontogeny. Fedotov (1924) observed juvenile Gorgonocephalus sp. (<0.5 mm disc diameter) protruding from polyps of live Drifa glomerata colonies from Kola Fjord (Barents Sea, published as Gersemia glomerata). Decades later, Patent (1970a) reported the presence of juvenile Gorgonocephalus eucnemis inside polyps of live Gersemia sp. colonies from Washington State (West coast of North America), in both August and September 1965. Patent (1970a) suggested that basket star embryos are passively ingested by the soft corals between spawning and 8–9 days of age, and develop within the polyps. Since Patent’s work, studies have focused on reporting the association: Haedrich and Maunder (1984) found Gorgonocephalus lamarckii individuals entangled with colonies of a Nephtheidae coral in Newfoundland, and young individuals of Gorgonocephalus sp. have been reported on D. florida (Buhl-Mortensen and Mortensen, 2005) and D. glomerata (Buhl-Mortensen et al., 2017). Young basket stars have also been observed in situ on colonies of Gersemia sp. from coastal areas in Labrador (∼50–60 m, personal communication B. M. Neves and R. Rangeley, Oceana Canada).
Both soft corals and basket stars are common in the Northwest (NW) Atlantic and the Eastern Canadian Arctic, being found at wide latitudinal ranges and depths of <10–1287 m (Wareham and Edinger, 2007; this study) and 40–1324 m (Neves and Wareham Hayes, 2019; this study), respectively. Soft coral colonies can be found in high densities, being often the most frequent coral taxa in bottom trawl bycatch samples in this region (Wareham and Edinger, 2007; Wareham, 2009; Murillo et al., 2011). Similarly, basket stars can be found in high densities at some locations (e.g., Neves and Wareham Hayes, 2019).
Despite commonly reported in the literature, the basket star-soft coral association has never been quantified. Considering the potential importance of soft corals in the early stages of basket star development, the objectives of this study are: (1) to describe the relationship between the two taxa in terms of epibiont basket star size ranges, occurrence and position on a colony, and (2) to investigate the potential influence of colony height and environmental variables on epibiont basket star occurrence and abundance by soft coral taxa. We examined over 4500 physical colonies from four soft coral taxa (Drifa glomerata, Duva florida, Gersemia spp., and Pseudodrifa racemosa) from the regions of Newfoundland, Labrador, and W Baffin Bay (NW Atlantic). Using 7 years of bottom trawl bycatch data we also investigated the co-occurrence of soft corals and basket stars in the region.
Materials and Methods
Sampling
Most soft coral samples were collected during multispecies trawl surveys conducted by the Department of Fisheries and Oceans Canada (DFO) between the years 2003–2015 in the NW Atlantic and Eastern Canadian Arctic (Table 2 and Figure 1A). These surveys are conducted by DFO’s Newfoundland and Labrador (NL) and Ontario and Prairie (OP, previously Central and Arctic) Regions. Surveys have a stratified random design, with tow speeds of 3 knots and duration of 15 minutes, although OP tows can range between 15 and 30 min.
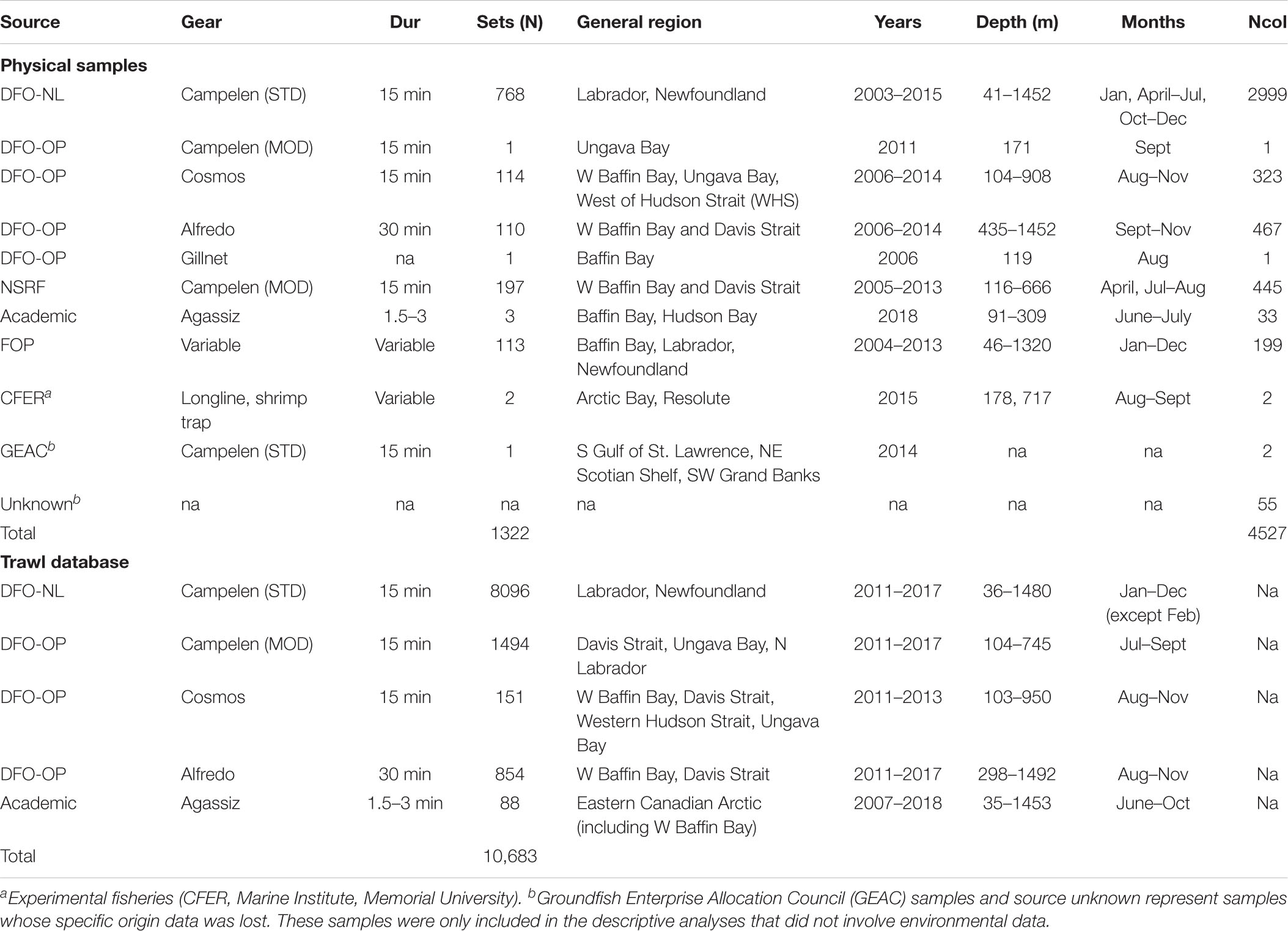
Table 2. Gear (mostly trawls), mean tow duration, number of sets/stations, regions surveyed, years, months, and number of colonies per survey (Ncol) for physical samples and trawl databases.
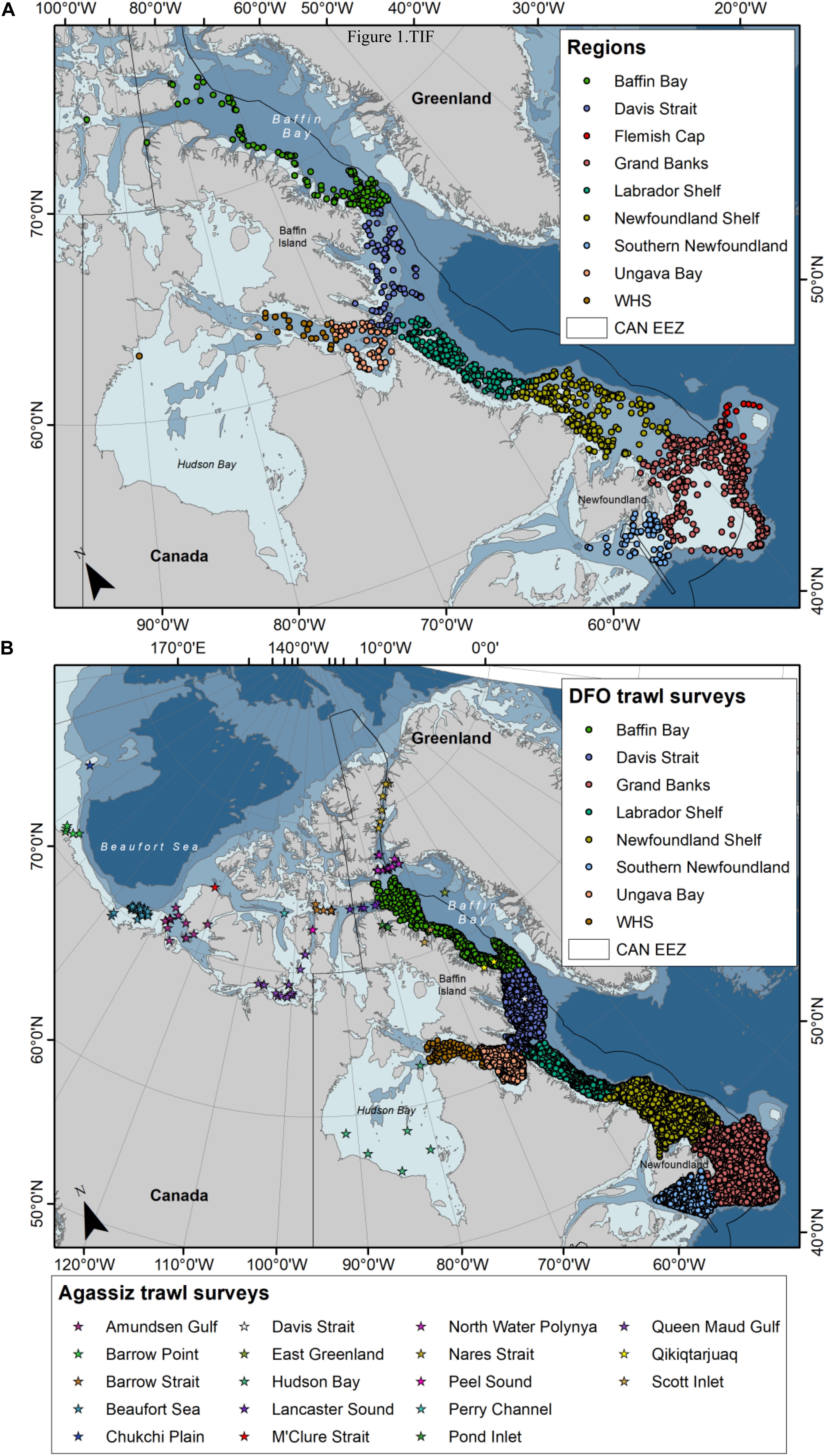
Figure 1. Distribution of (A) Nephtheidae soft coral colonies inspected for the presence of clinging basket stars (2003–2015), and (B) trawl databases examined for the presence of basket stars and Nephtheidae soft corals, where dots represent DFO trawl surveys (2011–2017) and stars represent Agassiz trawl surveys (2007–2018).
The NL Region conducts annual Multispecies Stock Assessment Surveys twice a year, between April-May and October-December. But in some cases, surveys took place during months outside of this range. In this region, surveys use a Campelen 1800 shrimp trawl equipped with rockhopper footgear, with tight rubber disks and spacers mounted along the footrope. The spring survey covers areas within NAFO Divisions 3PL, to a maximum depth of 750 m, while the fall survey covers deep and more northern areas (NAFO Divisions 2HJ, 3KLMNOP) to a maximum depth of 1500 m.
The OP Region conducts annual Groundfish Stock Assessment Surveys twice a year, in August and September, targeting Greenland halibut (Reinhardtius hippoglossoides) and shrimp (Pandalus spp.). These surveys use different trawl types depending on the area being surveyed. Cosmos and Alfredo trawls are used from Hatton Basin to Baffin Bay (NAFO Div. 0AB), to maximum depths of 1500 m. A Campelen trawl is used to survey SE Baffin Shelf - Hatton Basin area (NAFO Div. 0B).
Samples collected during Northern Shrimp Research Foundation (NSRF)/DFO Stock Assessment Surveys were also analyzed. These surveys are conducted from July to September (2006-present), and target Pandalus spp. in Hudson Strait and Ungava Bay, and Eastern Arctic Regions (NAFO Divisions 2G, 0B). This survey uses a modified Campelen 1800 shrimp trawl, sampling at depths < 750 m. A small number of samples from the Baffin Bay region were obtained from miscellaneous surveys, which include the Fisheries Observer Program (FOP), other fisheries surveys (e.g., GEAC), academic research surveys, and experimental fisheries (Table 2).
Over the years, coral samples collected during these surveys have been kept frozen at −20°C at the Northwest Atlantic Fisheries Center (St. John’s, Canada). For this study, soft coral samples were randomly selected from a number of bags containing a miscellaneous of specimens from different trips. Not all specimens from a trip were available, as not all samples caught are kept during the surveys. A total of 4527 soft coral colonies were examined for the presence of clinging epibiont ophiuroids. Samples include colonies of Duva florida (N = 1192), Drifa glomerata (N = 959), Gersemia spp. (N = 2154), and Pseudodrifa racemosa (N = 222) from Newfoundland, Labrador, and Baffin Bay (Figure 1A), from depths ranging between 41 and 1452 m, sampled between January and December. Only whole colonies were considered in this study. Due to challenges in the current taxonomy of Nephtheidae soft corals, we opted for pooling colonies of Gersemia, although we believe to have at least two taxa in this group (Gersemia rubiformis and Gersemia fruticosa), as per current classification (Utinomi, 1961).
Height was measured for each soft coral colony. Ophiuroid positions on the colony, their mass, and disc diameter were also determined. Ophiuroid position was standardized in relation to colony height due to variation in colony sizes. Position was measured as the distance from the center of the basket star’s disc to the colony distal extremity, with relative values of 100% representing individuals found at the very tip of the colony. Position of ophiuroid on a colony was not annotated for 11% of the specimens. This was merely circumstantial, as position was not a variable of interest in the early days of the project. Basket stars were photographed under a stereomicroscope and disc diameters were measured using the image analysis software Image-Pro Plus 7.0. Disc diameters were measured from the distal edge of the radial shields to the edge of the opposite interradial, following Stöhr et al. (2012). A total of 28 ophiuroids (1.4% of measured specimens) could not be measured, as they were deemed too damaged. These individuals were still counted. We provide the final N used in all analyses.
To investigate the presence of embryo or juvenile ophiuroids inside the corals, a subset of the studied colonies of D. glomerata (N = 178) and D. florida (N = 145) collected between April and December was also dissected and examined internally. A total of 10 polyps from three regions in each colony (i.e., top, middle, and base) were dissected and examined under a stereomicroscope (total 30 polyps per colony). Polyps of only a few Gersemia spp. (N = 13) and P. racemosa (N = 11) colonies were examined, as they were often retracted or in a poor physical condition due to their preservation method (i.e., freezing).
Due to the very small size of most ophiuroids, 19 randomly chosen specimens were submitted to the Natural Resources DNA Profiling and Forensic Centre (NRDPFC, Trent University, Canada) for DNA barcoding species identification. COI was the targeted gene, following Layton et al. (2016). Not all samples were amplified using Layton et al.’s primers, so additional primers produced by the NRDPFC (not published) and Kim and Shin (2015) were used.
To gain further insights into the relationship between soft coral and basket stars, we also performed δ13C and δ15N stable isotopes analyses and elemental C and N (%) on seven basket stars (disc diameters between 1.3 and 15 mm) and their soft coral hosts (D. florida and one P. racemosa). Sample preparation followed Sherwood et al. (2008) for both soft corals and basket stars. Briefly, randomly chosen individual soft coral polyps were removed to make a composite sample, while whole basket stars were processed due to their small size (one specimen had an arm sampled). Both dried and crushed basket stars and soft corals were acidified with 5% HCl prior to δ13C analyses to remove carbonates. Analysis of C/N ratios indicated that samples might not have been completely decalcified; therefore only results of the δ15N analyses are presented. Samples were analyzed at the Stable Isotopes Laboratory at Memorial University (St. John’s, Canada) and results are given in ‰.
Although the inspection of colonies for clinging basket stars was done carefully and diligently, we acknowledge that extremely small basket stars could have been missed during inspection, which could have led to false absences. Similarly, the loss of clinging juvenile basket stars during sampling (i.e., trawling) cannot be excluded. However, basket stars were found strongly intermingled with the corals, such that in some cases it was hard to remove them without breaking their arms. Ultimately, we believe that these potential false absences were not common and should not have largely influenced our analyses. In spite of that, we call for caution on the interpretation of absences.
Trawl Database
We have also examined DFO’s multispecies trawl survey and Agassiz trawl Arctic survey databases (Figure 1B) to investigate relationships between soft coral and basket star occurrence in trawl sets (Table 2). A total of 10595 trawl sets were analyzed for coral and basket star presence/absence using the DFO database (NL and OP), from mean depths ranging between 36 and 1492 m, between 2011 and 2017. For the Agassiz trawl Arctic database, data from 88 stations were analyzed, most of which have been resampled through the years during the months of June to October (2007–2018, with exception of 2012). Mean depth for these surveys ranged between 35 and 1453 m. For the trawl datasets we treated soft corals at the family level (i.e., Nephtheidae) due to difficulties with species identification at lower taxonomic levels in the field. Basket stars are not routinely measured in the field, so information on their ontogeny was not available for this study. Generally, the presence of juvenile basket stars on corals is also not accounted for as basket star presence in a trawl set, as soft corals are not inspected at sea.
Five different bottom trawls were used in the surveys included in the trawl database portion of this study: Alfredo, Campelen standard, Campelen modified, Cosmos, and Agassiz (Table 2). These trawls are routinely deployed in different regions, have different dimensions, and can be deployed for different periods of time. For instance, Campelen standard trawls are normally deployed for 15 min during DFO multispecies surveys in the Newfoundland Region, while the much smaller Agassiz trawls are usually deployed for less than 5 min in the Eastern Canadian Arctic as part of academic research surveys. However, our objective was to investigate the presence of soft corals and basket stars in a same set. In this sense, we refrained our analyses to descriptive statistics and considered that any emerging patterns should be relatively consistent across these trawls. Due to a more substantial difference in the gear dimensions, we present the data from the DFO trawls separately from the Agassiz trawl surveys.
Data Analysis
Data analyses were performed using RStudio 1.1.383 (RStudio Team, 2016). Pseudodrifa racemosa and Gersemia spp. were not included in the statistical analyses because of the small number of basket stars associated with them and/or very unbalanced number of observations per season (in some cases not enough to include co-variables). However, the raw data for these taxa is described and graphically presented. Analyses were performed separately for D. glomerata and D. florida, as descriptive statistics revealed that basket star occurrence rates between the two taxa were quite distinct.
Our main explanatory variables of interest were colony height and season. However, in the initial models we also included depth, latitude, year, and interactions (height:depth, height:latitude, season:latitude). We ran different models that included all or some of these variables, and performed model selection by comparing Akaike Information Criteria (AIC) values. Models with the lowest AIC values were selected, except for model 2b shown below, for which the diagnostic plots looked slightly improved than those for the model with the lowest AIC.
The relationship between individual basket star disc diameter (log-transformed) and colony height and season was investigated through Linear Mixed Effects Models (R package nlme, lme function in R, Pinheiro et al., 2020), with colony height and season as fixed effects, and colony id nested in trawl set (station) as random effects (Model 1). Basket star disc diameter was log-transformed to reduce its left-skewed impact on the analyses. We also examined the influence of colony height and season on basket star maximum disc diameter per colony. For D. glomerata the selected model also included depth and latitude (Model 2a), while for D. florida the simplest model (height and season) was selected (Model 2b). For this analysis, only trawl set was included as a random effect, as in this case there was only one disc diameter measurement per colony.
Basket star presence-absence (PA) on a colony was investigated through binomial Generalized Additive Mixed Models (GAMMs) with a clog-log link, with colony height and season as fixed effects, and trawl set (station) as a random effect (Model 3). The clog-log link function was chosen because there were considerably more zeros than ones (Zuur et al., 2009). We also used GAMMs with a Poisson family to investigate the relationship between number of basket stars per colony (in colonies with basket stars) and colony height and season as fixed effects, and trawl set (station) as a random effect (Model 4a). For D. glomerata the selected model also included depth and latitude (Model 4b). We did not include year or interactions in the initial GAMMs, as including these terms generated model convergence errors, likely due to the high complexity of the models for the available data. We used restricted maximum likelihood (REML) as the estimator for the mixed linear models (Zuur et al., 2009). The final models were:
Model 1: lme (logdiscdiam∼height + season, random = ∼1| station/colony, method = “REML”).
Model 2a (D. florida): lme (logmaxdd ∼ height + season, random = ∼1| station, method = “REML”).
Model 2b (D. glomerata): lme (logmaxdd ∼ height∗depth+latitude∗season+year, random = ∼1| station, method = “REML”).
Model 3: gamm(PA∼s(height)+season, random = list(station = ∼1), family = binomial (cloglog)).
Model 4a (D. florida): gamm(abundance∼s(height)+season, random = list (station = ∼1), family = poisson).
Model 4b (D. glomerata): gamm(abundance∼s(height)+season +depth+s(latitude), random = list (station = ∼1), family = poisson).
GAMMs were applied using the gamm function of the R package mgcv (Wood, 2011). When season was identified as a significant source of variation, we used the R function summary to obtain pair-wise comparisons (e.g., between seasons), with the function relevel used to change the order of the baseline, accordingly.
We tested for the presence of outliers and influential observations using Cook’s distance for linear mixed models (CookD function in predictmeans R package). Influential observations were removed as they can have too much influence in the models. For instance, for D. glomerata, analysis on the number of basket stars per colony excluded entries with > 100 basket stars (N = 2), and only considered basket stars < 15 mm in disc diameter (where disc diameter was included, both species). Assumptions of normality, independence, and homogeneity of residuals were assessed through the visualization of diagnostic plots for the linear mixed models (Kabacoff, 2015). For the GAMMs, validation tools include the examination of normalized residuals versus fitted values and explanatory variables (Zuur et al., 2009). The presence of spatial correlation was assessed by adding a spatial correlation structure to the models, and comparing these to those without a correlation structure using AIC values (Zuur et al., 2009). Significance level for all analyses was set at α < 0.05. We included season, depth, and latitude in two selected D. glomerata models (Models 2b and 4b); however since DFO trawl surveys per region depend on time of the year, effects of these variables might be confounded.
In terms of the trawl databases, in order to account for the unbalanced number of trawl sets per region and season, data on soft coral and basket star presence per trawl set is presented as relative proportions. For the Newfoundland and Labrador regions, the data is presented by Ecosystem Production Units (EPUs) (Pepin et al., 2014), and by general region where there is no defined EPU (e.g., Davis Strait, Ungava Bay, West of Hudson Bay, and Baffin Bay, Figure 1B). For the Agassiz trawl Arctic database, data was grouped in 18 main regions (Figure 1B).
Results
Ophiuroid DNA Barcoding
The molecular analysis of 19 basket star specimens yielded 99–100% GenBank matches to Gorgonocephalus arcticus. Two brittle stars were also identified: Ophiopholis aculeata and Ophiocten gracilis (99% matches). Since most specimens were not submitted for molecular analysis (N = 2003 specimens in total), we opted for treating the specimens in this study as Gorgonocephalus sp., but knowing that all specimens included in the molecular analysis yielded very close matches to G. arcticus. GenBank accession numbers for COI sequences of the analyzed specimens can be found in Supplementary Table S1.
General Description
A total of 2003 ophiuroids were found on 543 colonies (12% of all examined colonies), although of these only seven were brittle stars, which were found on only five colonies (in some cases both taxa on a same colony). For this reason, we focus the remaining of the study on basket stars only (N = 1996).
Basket stars were found clinging on colonies of all four studied soft coral taxa (e.g., Figure 2), collected from all studied regions (although limited in Baffin Bay), from depths ranging between 53 and 1317 m (Figure 3). Basket stars were present on colonies collected at every month of the year, except for February when 18 sets were deployed between 2005 and 2006 (one D. glomerata, 24 D. florida, and two Gersemia sp. colonies). No basket stars < 1 mm in disc diameter were found on colonies collected in February, March, or April. Basket stars were mainly found between the 2nd and 3rd quartile of colony height (upper half) in all four soft coral taxa (51–75%, Figure 4A).
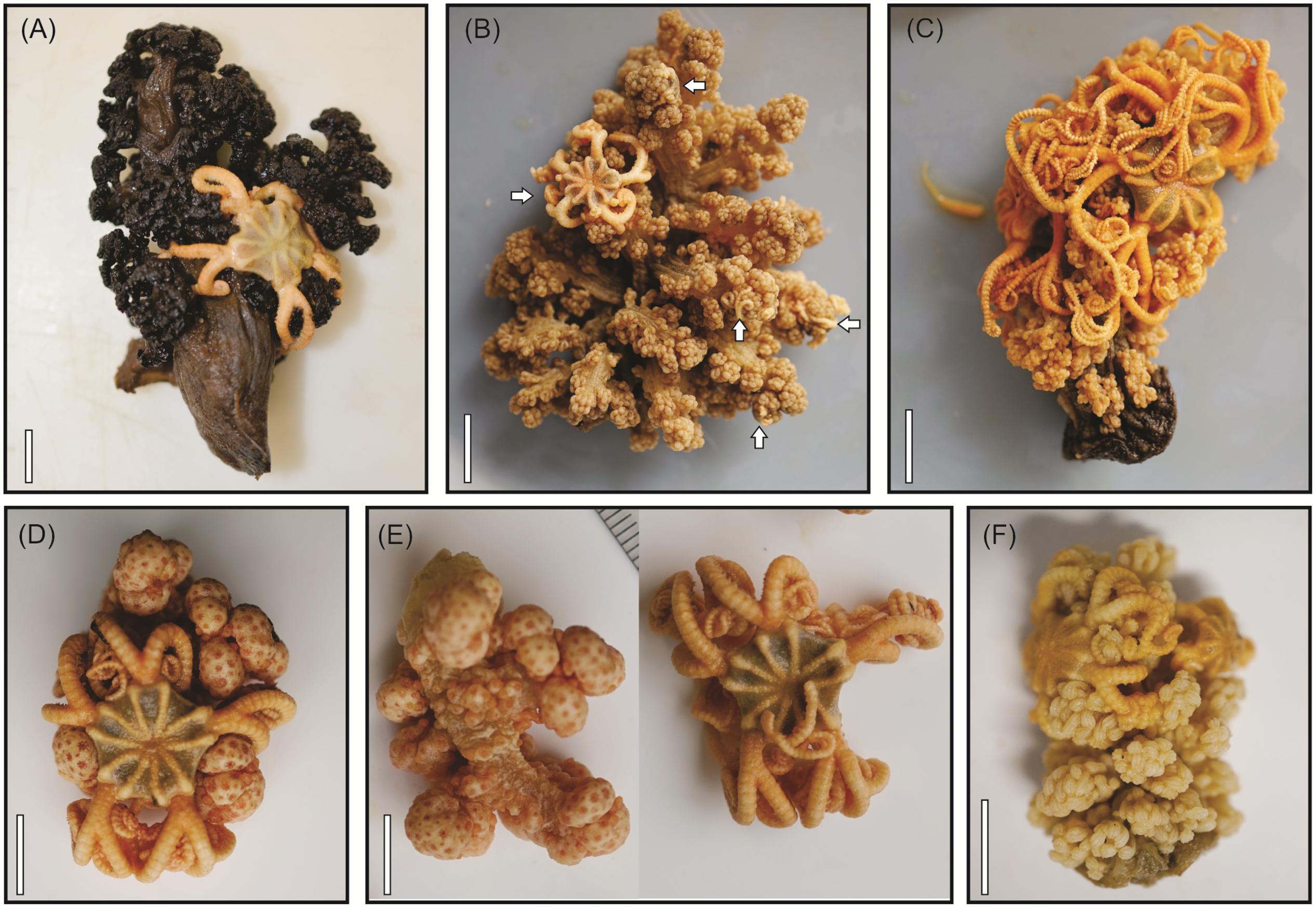
Figure 2. Cold-water Nephtheidae soft corals as hosts for juvenile basket stars (Gorgonocephalus sp.). (A) Duva florida, (B,C) Drifa glomerata, (D,E) Gersemia rubiformis, (F) Pseudodrifa racemosa. Arrows in (B) point to some of the individuals found on this colony, which contained 15 juvenile basket stars. (E) Shows the colony and basket star seen in (D) after being separated. Scale bars = 1 cm.
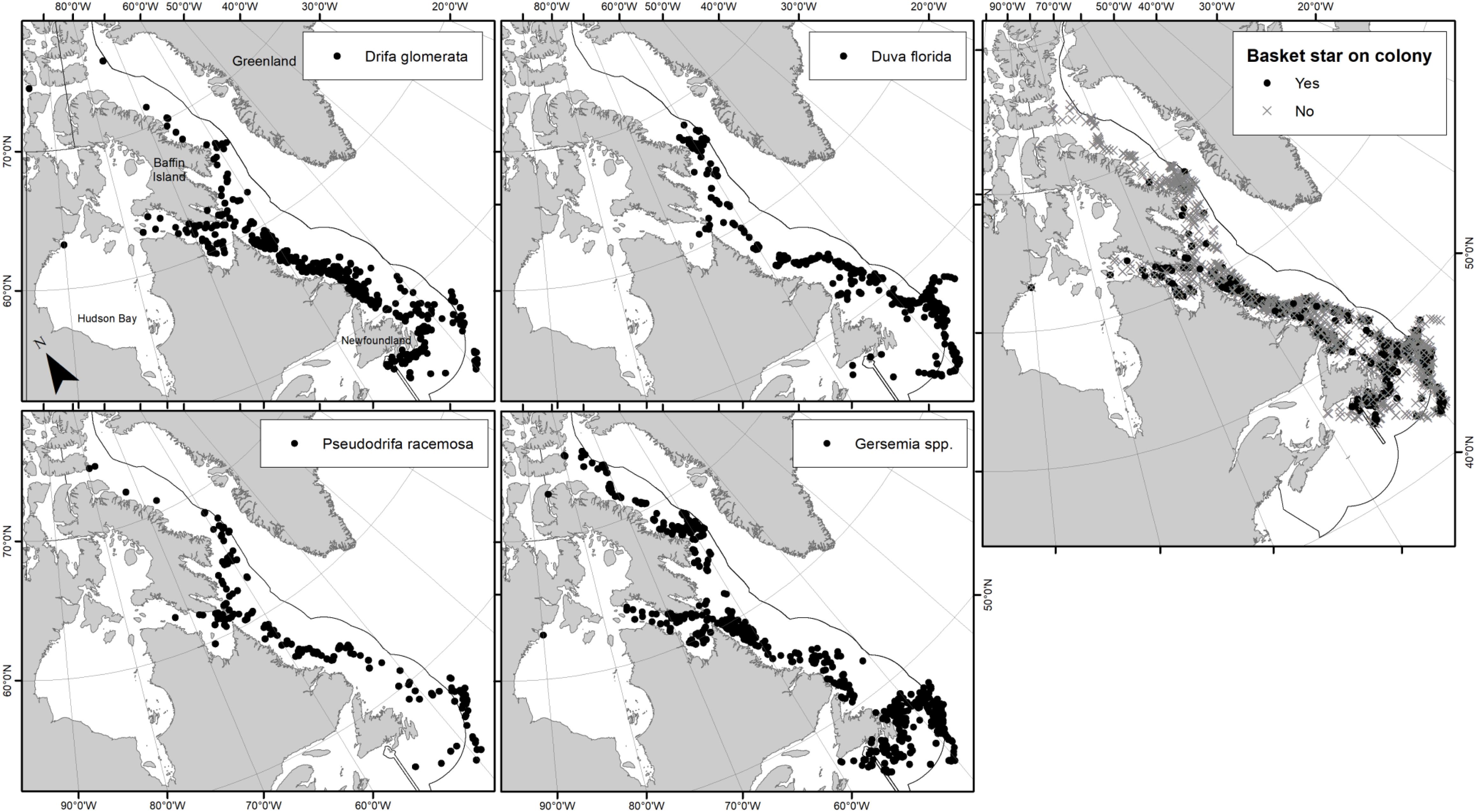
Figure 3. Distribution of examined soft coral samples per taxa, and overall presence-absence of juvenile basket stars on examined soft coral colonies (right panel). Dots on panels per taxa only indicate soft coral presence, not associated basket stars.
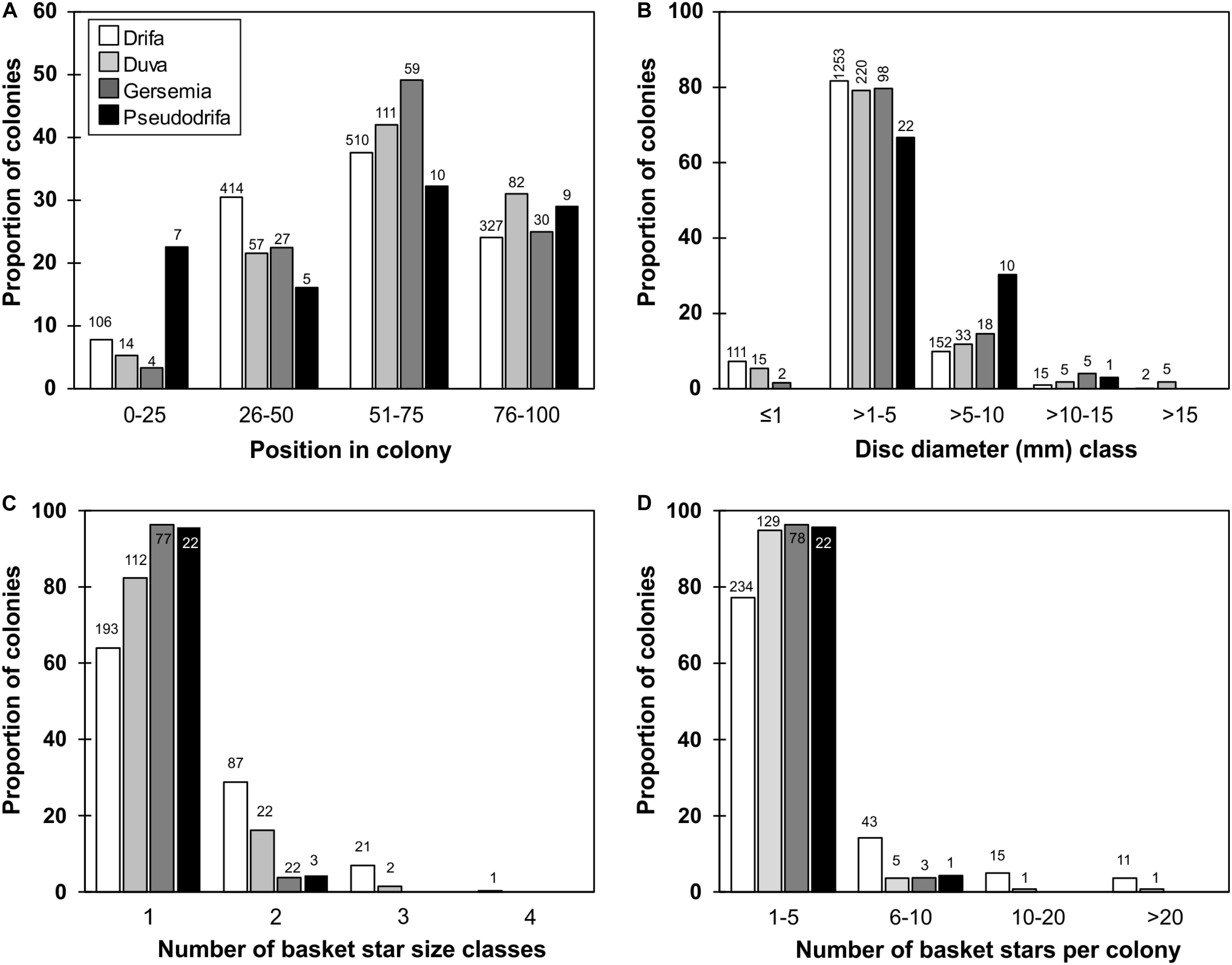
Figure 4. Proportion of soft coral colonies by taxa in relation to: (A) basket star position on colony by quartiles of colony height (0 closest to base, and 100 closest to distal extremity of colony), (B) basket star disc diameter class, (C) maximum number of basket star size classes found on a single colony, (D) number of basket stars per colony. Number of specimens is shown on top of bars.
Basket Star Disc Diameter
Disc diameters ranged between 0.42 and 19 mm, however, two basket stars 22.2 and 23.6 mm in disc diameter were found on broken D. florida and D. glomerata colonies, respectively, which were not included in the analyses. Disc diameters were classified and displayed in five size classes: ≤ 1, > 1–5, > 5–10, > 10–15, > 15 mm (Figure 4B). In most cases, disc diameters ranged between 1 and 5 mm for basket stars found on all soft coral species (Figure 4B), and for most colonies basket stars belonged to a single size class, although in D. florida and D. glomerata basket stars from up to three size classes on a same colony were identified (Figure 4C).
Individual basket star disc diameter did not significantly vary with colony height for both D. florida and D. glomerata, but it did for season in D. glomerata (Table 3). For this species, individual basket star disc diameters were significantly larger in the Spring than in the Summer and Fall (Figure 5A and Supplementary Table S2).
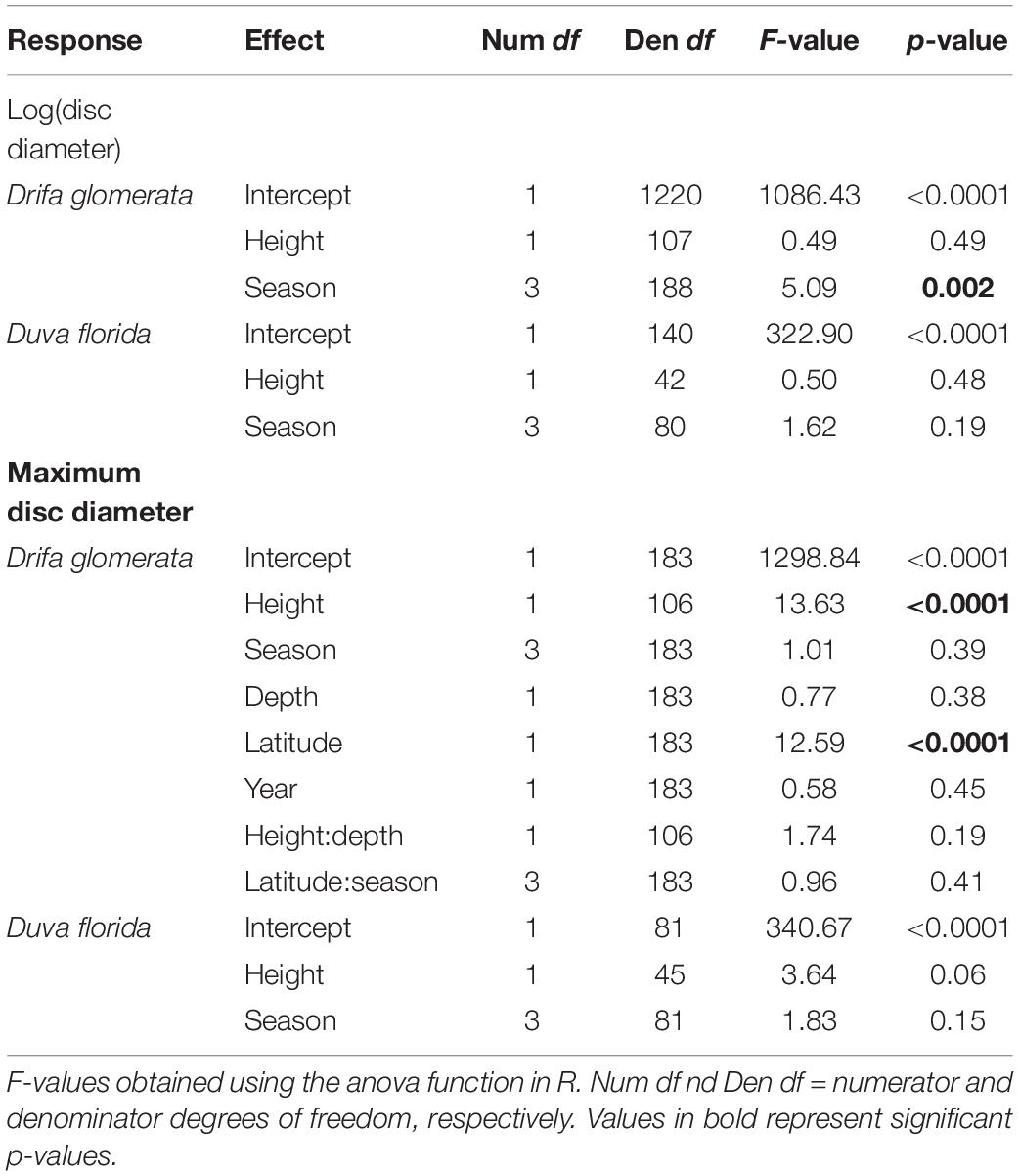
Table 3. ANOVA of fixed effects for linear mixed models estimating colony height and season effects on juveniles basket star disc diameter associated with the Nephtheidae soft corals Drifa glomerata and Duva florida in the Northwest Atlantic.
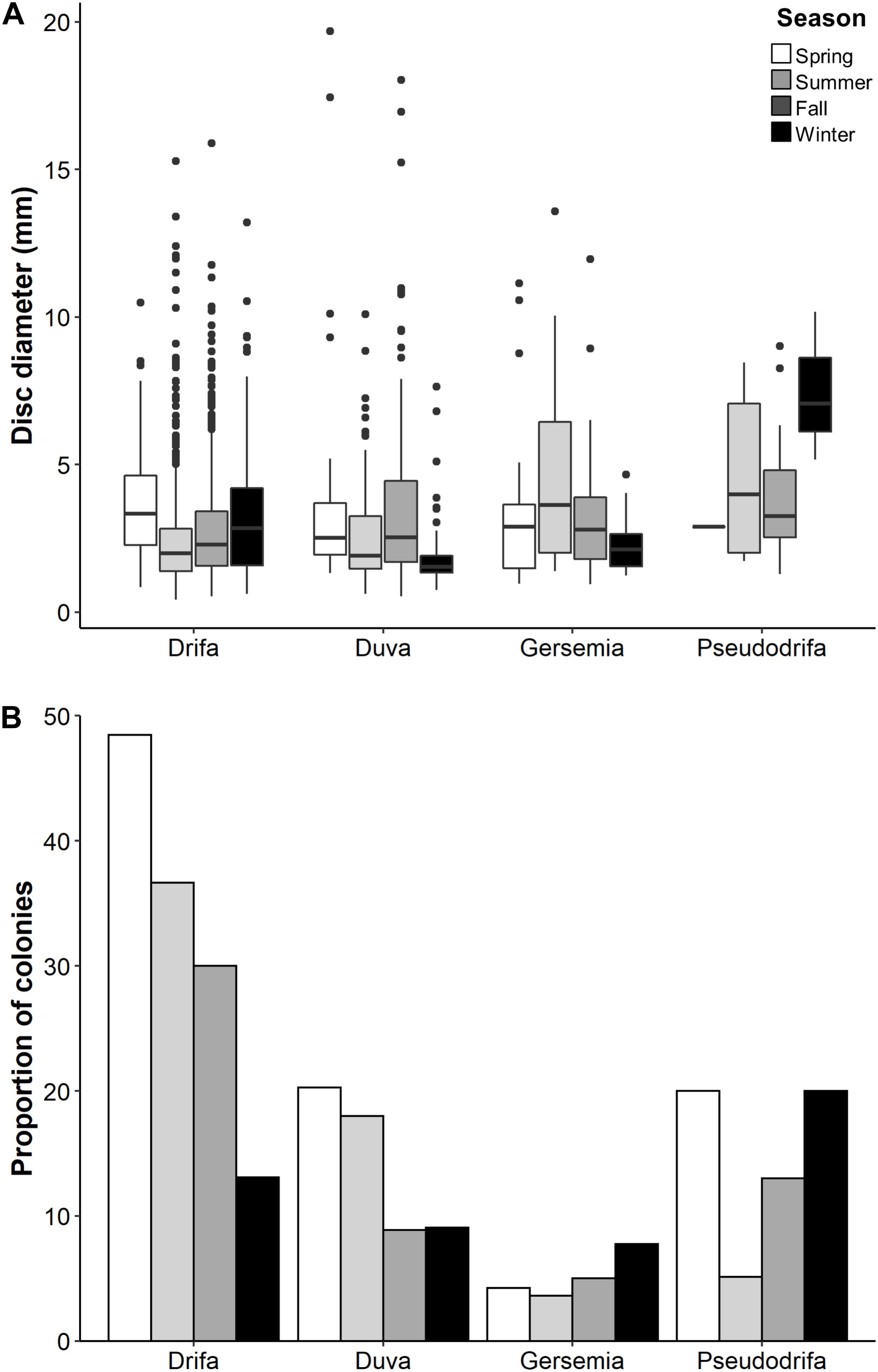
Figure 5. (A) Basket star disc diameter by taxa (shown as genus) per season. (B) Relative proportion of colonies with basket stars per season and host species (number of colonies with basket stars relative to total number of colonies available per season). Pseudodrifa racemosa winter is represented by one colony.
On the other hand, maximum disc diameter per colony was significantly related to colony height, and latitude in D. glomerata (Table 3). For D. florida, maximum disc diameter was not significantly related to colony height or season (Table 3).
Basket Star Presence-Absence on Colonies
Basket stars were present on colonies ranging between 10.5 and 147 mm in height (Figure 7). They were more frequent in larger colonies for both D. glomerata, D. florida, and Gersemia spp. (Table 4 and Figure 7). The binomial GAMM indicated that for D. glomerata and D. florida the probability of having associated basket stars increases with colony height up to ∼70 mm for both taxa, when it starts to level off for the former and to slightly decrease for the latter (Figures 6A,B). Descriptive statistics corroborate these observations, as the inter-quartile range of colony height – for colonies where basket stars were present – was 38.8–65.6 mm for D. glomerata and 44.3–69.9 mm for D. florida.
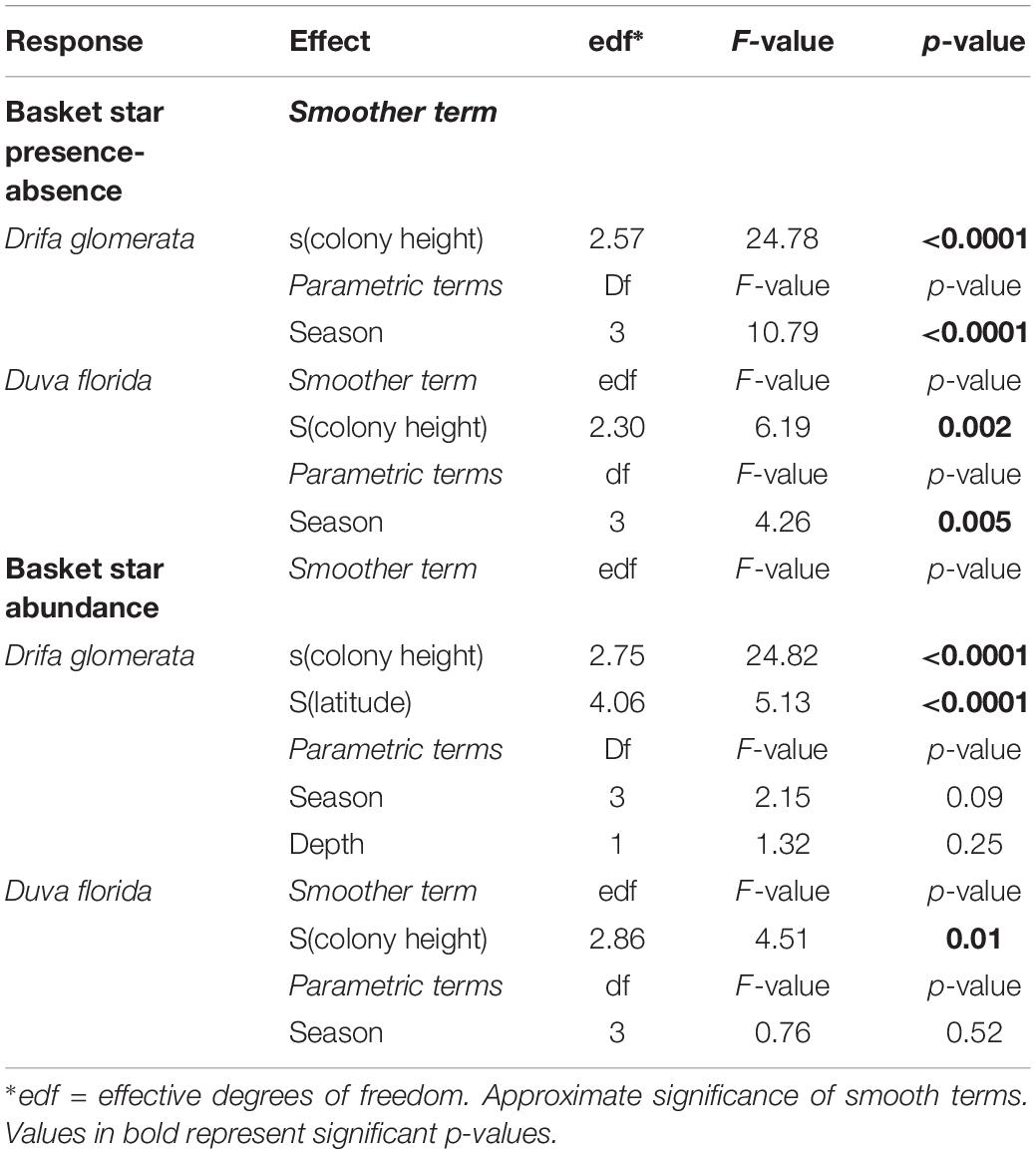
Table 4. Statistical outputs of GAMM models on the effects of soft coral colony height (smoother) and season on juvenile basket star abundance and presence-absence on Nephtheidae soft corals in the Northwest Atlantic.
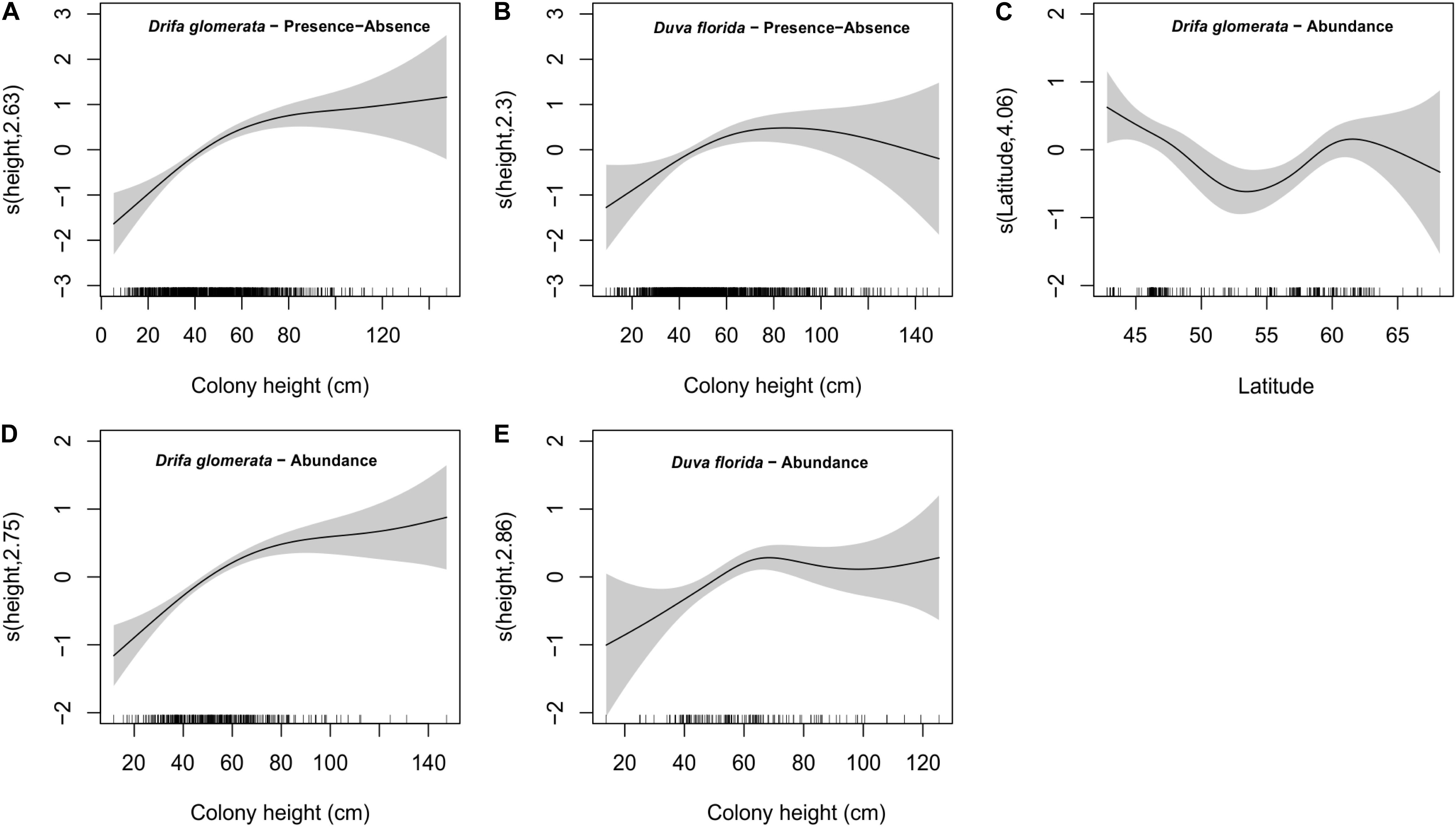
Figure 6. Estimated smoothing curves for colony height obtained by the GAMMs on basket star presence-absence for Drifa glomerata (A) and Duva florida (B), latitude (C) and colony height on abundance for Drifa glomerata (D) and colony height on abundance for Duva florida (E). Solid lines and gray bars represent the smoothers and 95% confidence bands, respectively. Scale for the x-axis looks slightly different for presence-absence versus abundance because the number of samples available for these analyses was not the same.
Colonies with basket stars were more frequent during the Spring for D. glomerata and D. florida, and during the Winter for Gersemia spp. and P. racemosa (Figure 5B). Basket star presence-absence on D. glomerata colonies significantly changed across seasons (Table 4), except for the Summer-Fall pair (Supplementary Table S3 and Figure 5B). For D. florida, basket star presence-absence on a colony was also significantly different across seasons (Table 4), except for the pairs Fall-Winter and Spring-Summer (Supplementary Table S3 and Figure 5B).
Examination of at least 10410 dissected polyps from 347 colonies yielded the presence of one juvenile ophiuroid (0.34 mm disc diameter) within a D. florida polyp (Figure 8), whose colony was collected in July in the Newfoundland Shelf region, at 306 m. This colony had no epibiont basket stars, and there was no record of a basket star collected in that trawl set. The closest set with a basket star reported was at ∼34 km from this site.
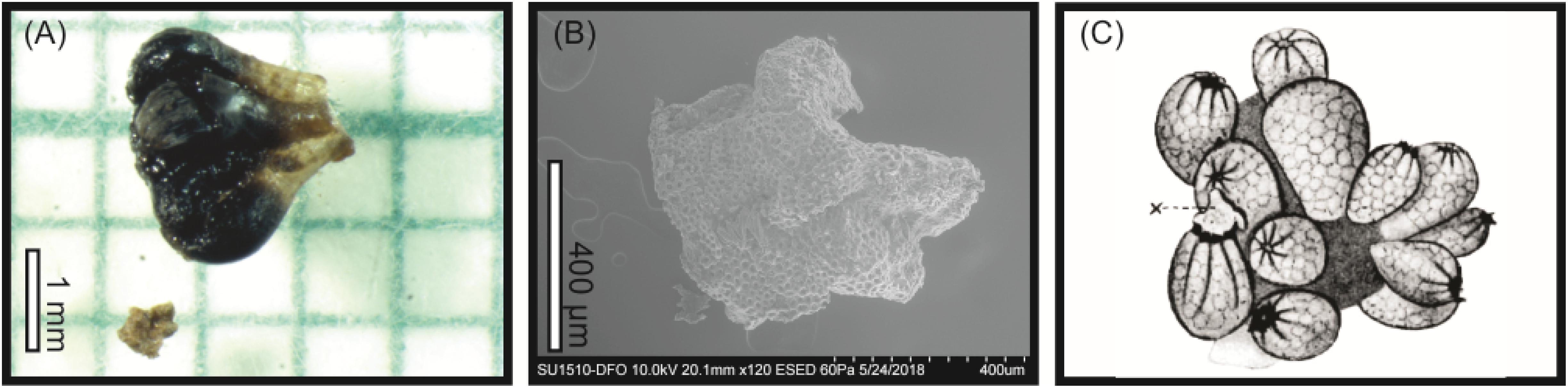
Figure 8. (A) Piece of branch of Duva florida colony showing three large polyps and juvenile ophiuroid found within one of the polyps, (B) Scanning Electronic Microscopy (SEM) of the ophiuroid specimen seen in (A), (C) reproduction of Fedotov (1924)’s sketch of a juvenile basket star leaving the polyp of a live Drifa glomerata (published as G. glomerata) colony in Russia.
Basket Star Abundance
Most colonies had between 1 and 5 clinging basket stars, and only D. glomerata and D. florida had > 20 individuals on a single colony (Figure 4D). Drifa glomerata had both the highest ophiuroid occurrence (31.5%) and maximum number of ophiuroids per colony (N = 111), followed by D. florida and P. racemosa (11 and 10.5%, N = 28 and 6, respectively), and Gersemia spp. (3.9%, N = 8).
Number of basket stars per colony (in colonies with basket stars) significantly varied with colony height, but not with season for both D. glomerata and D. florida (Table 4). There was also an effect of latitude for basket star abundance on D. glomerata (Figure 6C), but not of depth (variables not tested for D. florida). The GAMM for both species indicated that, similarly, to the observed for presence-absence, the number of basket stars per colony increases with colony height up to ∼70 mm, when it levels off (Figures 6D,E).
Trawl Data
When examining the DFO-RV database pooled (2011–2017, Figure 9), 45% of trawl sets containing basket stars (N = 2624) also had corals (N = 1186), of which 67% were Nephtheidae soft corals only (N = 796), followed by a mix of coral taxa that could also include soft corals (16%). The total percentage of trawl sets with basket stars that contained soft corals was 35%. Overall, Labrador shelf was the region with the highest co-occurrence of basket stars and soft corals per trawl set (54% of sets, N = 166), followed by Newfoundland Shelf (48%, N = 206), Grand Banks (36%, N = 275), and southern Newfoundland (31%, N = 120, Figure 10A). Ungava Bay, Davis Strait, and W Baffin Bay had the lowest overall co-occurrence of basket stars and soft corals (18, 19, and 28%, respectively, Figure 10A). Maximum co-occurrence across years took place in the Labrador Shelf region in 2012 (84%, N = 50), and the lowest co-occurrence was identified in Baffin Bay, Davis Strait, and Ungava Bay, where in some years no soft corals were reported in sets with basket stars.
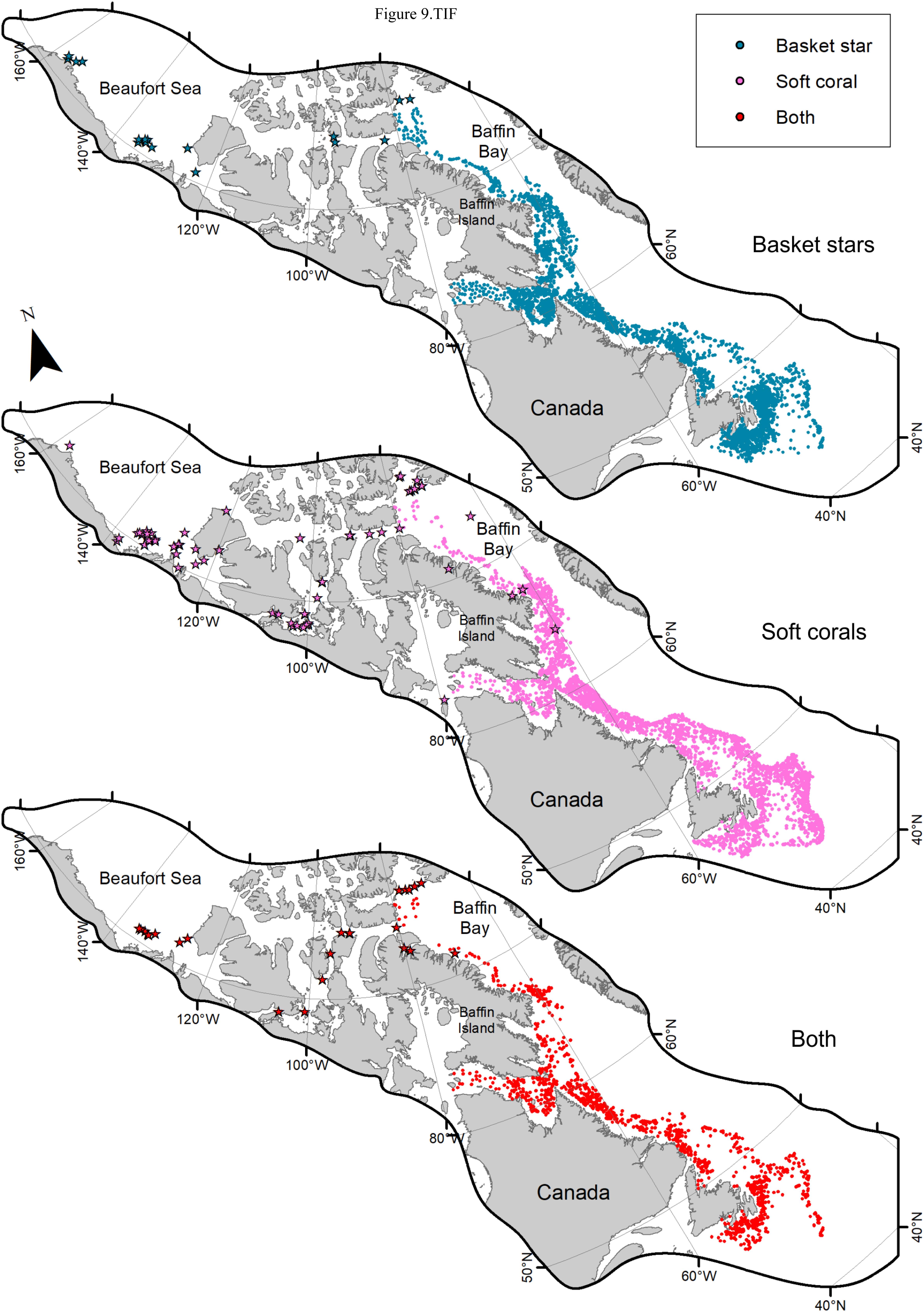
Figure 9. Trawl data on presence of basket stars, Nephtheidae soft corals, and both per trawl set (2011–2017). Dots represent DFO RV trawl surveys, while stars represent Agassiz trawl surveys. The maps were cropped to limit the view to the distribution of samples.
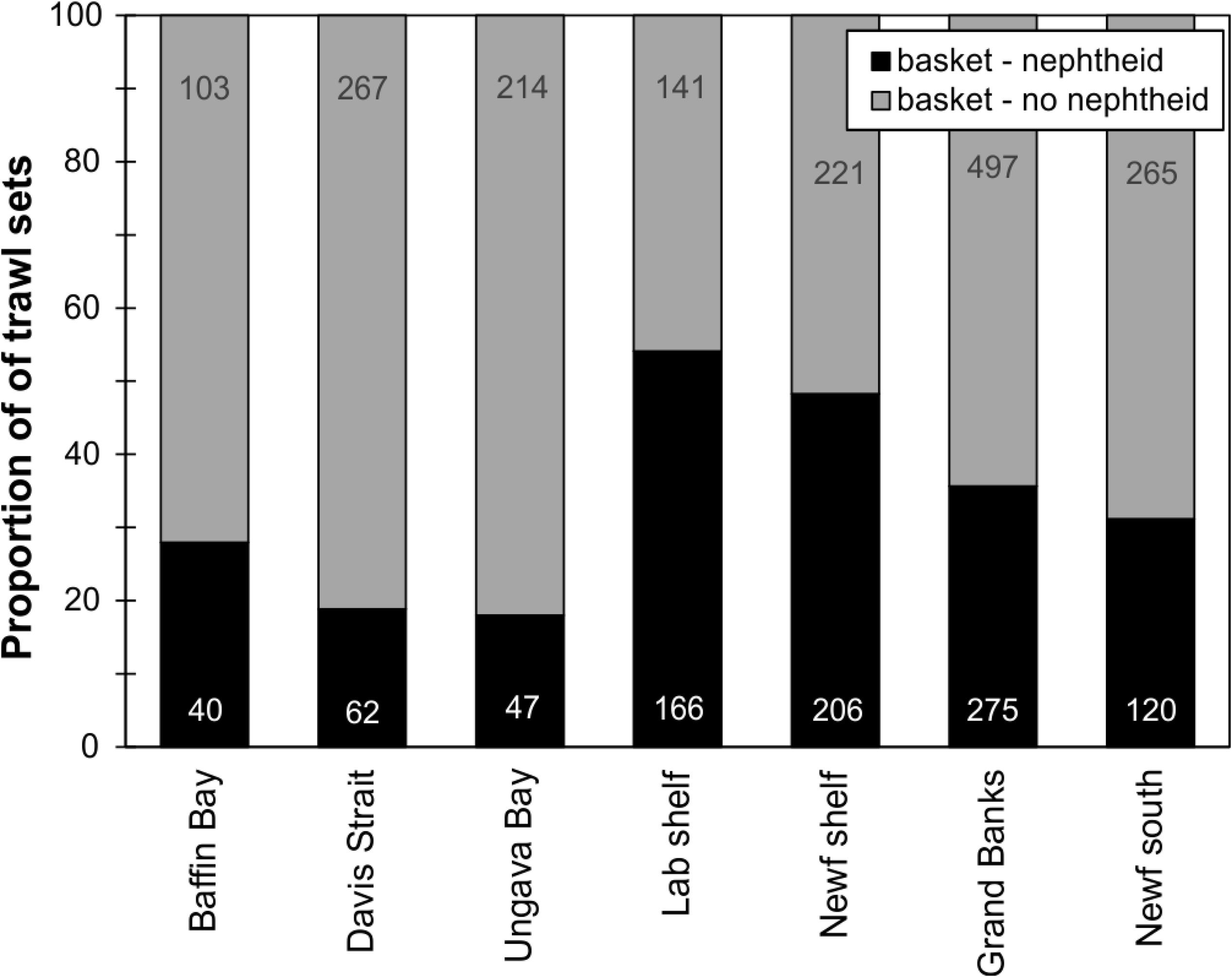
Figure 10. Relative proportion of coral presence-absence in DFO RV trawl sets containing basket stars by region (number of trawl sets with/without corals relative to total number of sets per region – including basket star absences). Number of sets per region is shown.
Since DFO trawl surveys in the different regions are directed by time of the year (e.g., Southern Newfoundland only during Spring), we could not assess the influence of season on basket star-soft coral co-occurrence per region. But in the Grand Banks region, for which there were sets deployed in every season, basket star presence at depths > 100 m was more frequent in the Summer, followed by Winter, Fall, and Spring (Figure 11). However, basket star-soft coral co-occurrence was more frequent in the Fall, followed by Winter, Summer, and Spring (Figure 11).
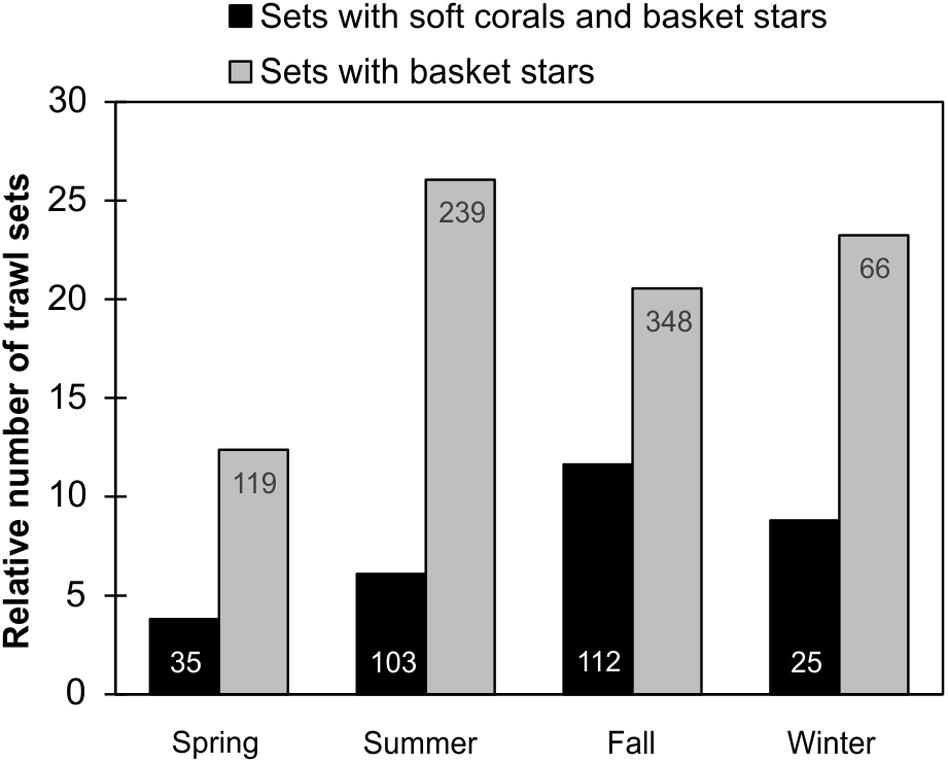
Figure 11. Relative proportion of DFO trawl sets with basket stars and Nephtheidae soft corals, and sets with basket stars per season in the Grand Banks region (pooled 2011–2017). Total number of sets with basket star and coral co-occurrence is shown on bottom of bars, and number of sets with basket stars is shown on top of bars.
Of the 88 stations sampled with the Agassiz trawl in the Eastern Canadian Arctic, 33% contained both basket stars and soft corals, which all together is comparable to the proportions found in Baffin Bay (28%) based on the larger trawls (see Figure 10). When examining this database by specific regions in the Arctic, stations (sometimes more than one set) with both basket stars and soft corals were identified in the Beaufort Sea (32%, N = 25), Amundsen Gulf (18%, N = 11), Peel Sound (N = 1), Queen Maud Gulf (37.5%, N = 8), Barrow Strait (100%, N = 3), Lancaster Sound (33%, N = 3), North Water Polynya (55.6%, N = 9), and Scott Inlet (50%, N = 2). Unfortunately, physical samples of soft coral colonies from these regions were not inspected for this study and could not be included in our analyzes. However, three physical samples from Hudson Bay/Strait area (not included in trawl database), where there was no co-occurrence between the two taxa based on the trawl database had juvenile basket stars associated to them.
Stable Isotope Analyses
Results of the stable isotope analysis indicate that absolute differences in δ15N content between basket star and soft coral host (i.e., D. florida and one P. racemosa) ranged between 0.28 and 1.1‰ (Supplementary Table S4). δ15N data show both cases of basket stars with higher and lower δ15N than the soft coral hosts (Supplementary Table S4).
Discussion
Basket Star and Soft Coral Co-occurrence
In this study we found juvenile basket stars associated with colonies of all four studied soft coral taxa, from Newfoundland to northern Baffin Bay, at occurrence rates ranging between 4 and 32%, depending on the host taxon. The presence of juvenile basket stars on soft corals is already evidence that the two taxa somehow spatially co-occur. Furthermore, examination of the pooled DFO-RV database showed that 45% of sets with basket stars also had corals, of which 67% were represented by soft corals only. In fact, 35% of the sets with basket stars contained soft corals, which is comparable to the highest proportion of basket star occurrence on physical samples (i.e., 32% in D. glomerata). Examination by region showed the highest relative co-occurrence in the Labrador Shelf Region, at 54%. Although this does not necessarily imply that basket star distribution depends on soft coral presence, our data does show a high degree of co-occurrence between the two taxa.
The northern regions (Ungava Bay, Davis Strait, Baffin Bay) had the lowest co-occurrence of soft coral and basket star in a trawl set (DFO trawls). Although we do not show distribution data by genus for the trawl datasets, examination of soft coral genera distribution based on the examined physical samples indicates that the taxa with the highest basket star occurrence on a colony (i.e., D. glomerata and D. florida) might have their distributions more limited in northern waters. The observation that basket star presence on a colony was limited in Baffin Bay (restricted to latitudes south of 68°N) might be related to the fact that most colonies available/examined from this region were Gersemia spp., which had the lowest basket star occurrence rate (i.e., 4%). In addition, for this taxon colonies with basket stars in the southern sites were more frequent during the Winter, when this region is rarely sampled. On the other hand, trawl sets with both basket stars and nephtheid soft corals were identified in Baffin Bay and elsewhere in the Eastern Canadian Arctic (although at lower rates), indicating that the two taxa do co-occur in this region (even northern of 68°N). The Agassiz trawl data pooled for all sampled Arctic stations indicate a comparable proportion of co-occurrence of the two taxa, in relation to the Baffin Bay data from the larger trawls.
Juvenile basket star occurrence rates on physical colonies were comparable to those found in a study that examined Gorgonocephalus spp. in situ occurrence (i.e., video data) with gorgonian corals in northern Norway (Clippele et al., 2015). In that study, Gorgonocephalus spp. occurrence rates on colonies of Paragorgia, Paramuricea, and Primnoa spp. were 18, 17, and 3%, respectively. These results are somehow comparable to our findings in terms of occurrence proportion for colonies of D. florida (11%), but colonies of D. glomerata held a much higher occurrence rate of juvenile basket stars than all three gorgonian species depicted by Clippele et al. (2015), and all other soft coral species included in the present study (i.e., 32%). Although there is no mention of basket star size in the study by Clippele et al. (2015), the authors have limited their analysis to megafauna > 1 cm; furthermore, two photographs indicate that those basket stars were considerably larger than the specimens described in the present study. Therefore, life stages of basket stars associated to soft corals versus other octocorals are likely different.
The preference of juvenile basket stars for colonies of D. glomerata might be associated with different factors. First, our trawl database indicates that basket stars are mainly found along the continental shelf. Unpublished information on soft coral distribution (at the species level) in the region indicates that D. glomerata seems to follow a more similar distribution to that of basket stars, while D. florida is mainly found along the slope break (in preparation, V. Wareham Hayes, personal communication). Therefore, the higher occurrence of basket stars associated with D. glomerata could be related to a higher co-occurrence in the distribution of these two taxa.
Colonies of D. glomerata have the tissue heavily covered with club-shaped spinous CaCO3 sclerites (Utinomi, 1961), whose roughness might increase attachment capacity (Dodou et al., 2011), which could be an advantage to juvenile ophiuroids with very short arms. Conversely, the scarce number of basket stars found on colonies of the other soft coral species (especially Gersemia spp.) could be related to the production of secondary metabolites by these soft corals, which might function as anti-fouling compounds (Williams et al., 1987; Slattery et al., 1995). To our knowledge, there are no published studies on secondary metabolites produced by D. glomerata, D. florida, or P. racemosa. It should be noted that any potential deterrence produced by these soft corals is obviously not enough to completely repel basket stars, as they were indeed found on all four soft coral taxa.
Basket stars were noticeably blended in color with colonies of D. glomerata. The similarity in color between host and basket star could be an advantage for the latter (e.g., camouflage against predators), and protective coloration was also suggested by Patent (1970a). However, colonies of Gersemia spp. and Primnoa resedaeformis gorgonians are also orange in tone, and had the lowest occurrence rate of Gorgonocephalus spp. among the studied colonies in this study and in the study by Clippele et al. (2015). Therefore, protective coloration alone does not seem to be the reason for Gorgonocephalus sp. to choose D. glomerata more frequently, although it might be an advantage.
Interestingly, young basket stars were commonly observed in situ on live colonies of Gersemia sp. from coastal areas in Labrador, where other soft coral species were not observed (∼50–60 m, personal communication B. M. Neves, and R. Rangeley – Oceana Canada). However, these colonies were observed in situ. It is possible that the difference between alive and dead in the case of this taxon is a factor influencing occurrence rates, since colonies of Gersemia spp. can contract considerably more than the other taxa. This might also corroborate our trawl data observations that co-occurrence rates vary regionally.
We found that most colonies had between 1 and 5 clinging basket stars per colony. Patent (1970a) found a maximum of five basket stars per colony on Gersemia sp., which is comparable to our numbers for this taxon (N = 8). On the other hand, we found a maximum of 111 juvenile basket stars on a single D. glomerata colony. Buhl-Mortensen and Mortensen (2005) found 15 G. lamarckii individuals on a single large P. arborea colony, and up to > 20 individuals per colony have been counted on P. arborea colonies collected as trawl bycatch in the Newfoundland region (Wareham-Hayes, unpublished). Clippele et al. (2015) reported a maximum of three basket stars on P. arborea and P. resedaeformis, and four on P. placomus, noting that on colonies of P. arborea, single basket stars were more common.
Soft Corals as a Platform for Recruitment and Nature of the Relationship
In this study we showed that most Gorgonocephalus sp. specimens found associated with soft corals had disc diameters < 5 mm (86%), characterizing them as juveniles. Our largest specimen was 23.6 mm in disc diameter, but these large individuals were not common, and likely still constitute juvenile basket stars, as Patent (1970a) indicated that most adults are usually between 700 and 850 mm in disc diameter. Our observations indicate that soft corals represent an important host for basket stars in the early stages of their lives.
The observation that for most colonies basket stars were in the same size class indicates that these individuals came from recruitment events that occurred close in time. For instance, in the D. glomerata colonies with 111 and 104 individuals, 84 and 76% of them, respectively, had disc diameters between 1 and 5 mm. Fedotov (1924) and Patent (1970a) suggested that basket star embryos are passively ingested by soft corals and develop within polyps, since these authors found very young individuals within live soft coral polyps. In this study we only found one juvenile ophiuroid (0.34 mm dd) within a D. florida polyp from a colony collected in July, from ∼300 m. Although we could not confirm the taxonomic identity of this individual, the mesh-like structure of its plates is consistent with that of a very young ophiuroid, although it cannot be determined whether it is an euryalid (Stöhrn, personal communication). Noticeably, this colony had no basket stars on it, and there was no record of a basket star present in the trawl set associated with that coral colony.
Our data show that basket stars find their coral hosts at a very young age, and we believe that soft coral passive ingestion and basket star development within polyps, as suggested in those early studies, is still a plausible hypothesis. It is possible that the fact that our samples were not alive when examined had an influence on our lack of success finding additional individuals within polyps. In fact, the trawl vibration, speed and overall stress could have had an influence on basket star expulsion/evacuation. Furthermore, very few colonies of Gersemia spp. or P. racemosa were inspected for endobiont basket stars. Gersemia sp. was the species studied by Patent (1970a). Soft corals are very small for adult Gorgonocephalus spp. to climb on, and it is hard to conceive that individuals as small as 0.4 mm in disc diameter with undeveloped arms would be able to actively swim or crawl from the seafloor to climb on soft corals. The embryology of Gorgonocephalus spp. is still not well understood, but Patent (1969) suggested that euryalids might not develop pelagic larvae.
Fedotov (1924) proposed that the stage on D. glomerata colonies was a necessary part of the life cycle of Gorgonocephalus. Patent (1970b) suggested that embryos of G. eucnemis kept in aquarium died < 10 days after spawning because soft corals were absent. If this is the case, it is possible that this relationship has an obligate aspect, as the basket star might depend on the soft coral. Whether the coral also benefits from the presence of juvenile basket stars is still unclear. Mutual benefits of faunal associations are not always evident. The brittle star Ophiocreas oedipus lives in a obligate, solitary relationship with the octocoral Metallogorgia melanotrichos. Despite this relationship being obligate for the brittle star, no benefits to the coral have been identified (Mosher and Watling, 2009). But in some cases, the meaning of these relationships might come to light under stress. For instance, the euryalid brittle star Asteroschema clavigerum appeared to limit impact of the Deepwater Horizon oil spill to colonies of the octocoral Paramuricea biscaya, and to have facilitated their recovery after impact (Girard et al., 2016).
Although Fedotov (1924) considered the nature of the juvenile basket star-soft coral relationship to be parasitic, Patent (1970a) noted that the fact that polyps looked healthy is an indication that the basket stars do not harm the coral, although she suggested that basket stars might feed on coral eggs. The case of the G. rubiformis colony depicted in Figure 2 shows a certain degree of “strangling” by the basket star, but we do not know whether/how that might impact the colony. Colonies of G. rubiformis have a significant power of contraction, and the “strangling” might therefore seem worse on dead individuals than what it was in fact when the colony was alive. Although the nature of the relationship is still uncertain, the consistent presence of juvenile basket stars on soft corals suggest that they are not ephemeral visitors. We believe that further studies including the monitoring of live organism in situ or in laboratory settings are necessary to investigate hypotheses regarding how basket stars reach their soft coral hosts, and the nature of the relationship.
Basket Star Size, Abundance, and Position
Colony height had an effect on maximum disc diameter (D. glomerata) and number of basket stars per colony (both species). Although coral size was found not to be a limiting factor in the size of individual juvenile basket stars that it can host, our data indicate that there is a maximum basket star size usually supported by a colony. As mentioned before, larger specimens were found at low frequencies, and our largest specimen was ∼24 mm in dd. According to Fedotov (1924), basket stars ∼5 mm in dd can leave the soft coral colonies and move to discs of adult Gorgonocephalus sp. Our observation that most individuals were between 1 and 5 mm dd seems to agree with this author in terms of timing (i.e., in ontogeny) when most juveniles leave the colony. The largest juvenile specimen per soft coral colony examined by Patent (1970a) was 3 mm dd, and one free-living individual with a 25 mm dd was also reported by this author. Buhl-Mortensen and Mortensen (2005) reported individuals of G. lamarckii ≥ 15 mm in dd on the gorgonian P. arborea. These reports show that young basket stars can be found on other habitats. However, it is unknown whether other corals or habitats also host basket stars in their very early stages, like soft corals do. Larvae of the brittle star Ophiothrix fragilis settle on the surface of sponges, with recruits doubling their sizes in 3 months (June–September), and leaving for an independent life on other surfaces when they are > 1 mm in disc diameter (Turon et al., 2000). In other cases, adult ophiuroids can host juvenile ophiuroids of different species. Juveniles have been reported to leave the adults at 4.5 mm (Hendler et al., 1999) and 6 mm dd (Fourgon et al., 2007).
Maximum disc diameter also significantly decreased with latitude. Despite the potential for confounding effects between latitude and season, in this case the effect of latitude on maximum disc diameter seems dissociated from season, since there was no significant interaction between latitude and season. Since we do not know whether our Gorgonocephalus spp. represent more than one species, we cannot infer on whether changes in disc diameter with latitude might be related to species-specific differences or to other variables.
Number of basket stars per colony was not influenced by seasonality, but rather by colony height (both species) and latitude (D. glomerata only). For both taxa, number of basket stars and the probability of basket star presence on a colony increased with colony height up to heights of ∼70 mm. Maximum D. glomerata height among the studied colonies was 147 mm, and the two colonies hosting > 100 basket stars were approximately 100 and 120 mm in height. These colonies were not included in the statistical analyses, as they were rare and had too much influence on the data. The GAMM plot indicated that number of basket stars per colony in D. glomerata reaches the lowest point at a latitude of ∼55°N (Labrador, Makkovik area). However, in this analysis, season was weakly not significant (p = 0.09). Considering that borderline p-values should be taken with extra caution in these types of models (Zuur et al., 2009), it is possible that the effect detected for latitude in this case could be associated to season, because of the survey design previously mentioned (location depends on time of the year).
The presence of more basket stars on larger colonies might be a response to space availability, but also increased distance from the seafloor, as most basket stars were found on the upper half of the colonies (as discussed below), indicating a preference for taller surfaces. However, colonies > 70 mm might not necessarily host more basket stars. Furthermore, no data is available on colony height variation between expanded and contracted states (studied species). A better understanding of these variations, which can be significant in soft corals (e.g., Hellström and Benzie, 2011), might enlighten our interpretation on the role played by colony height on basket star abundance.
Individuals of Gorgonocephalus sp. were also found more at the top on the gorgonians P. placomus and P. reseadaeformis, although nearer the base on P. arborea (Clippele et al., 2015). The presence of ophiuroids clinging on corals or other tall structures has been associated with the advantage of being higher in the water column, therefore hiding from predators, and of increasing access to food by benefiting from stronger currents (Buhl-Mortensen and Mortensen, 2005; Clippele et al., 2015; Buhl-Mortensen et al., 2017). The arms of Gorgonocephalus spp. individuals start to branch when they are larger than 1 mm in dd (Fedotov, 1924), and individuals > 20 mm in dd are already capable of capturing plankton (Patent, 1970a). Individuals < 20 mm in dd might feed on smaller particles and still benefit from being higher on the water column. Protection against predation is also a plausible hypothesis on their preference for relatively taller portions of the soft coral.
Patent (1970a) suggested that basket stars might be feeding on detritus or food from the polyps, as well as on coral eggs, as Gislén (1924, apud Patent, 1970a) found remnants of soft corals in stomach contents of G. caputmedusae. Although limited, our nitrogen stable isotope data indicate that maximum difference in δ15N between basket star and host was 1.1‰, indicating that the two organisms share the same trophic level. Average fractionation per trophic level is 3.4‰ for δ15N (Vander Zanden and Rasmussen, 2001). A relationship with trophic benefits for the associates has been suggested for other organisms. For instance, juveniles of the brittle star Ophiothrix fragilis are believed to take advantage of the currents produced by their sponge hosts (Turon et al., 2000).
Our stable isotopes data showed no indication that increasing size in juvenile basket stars leads to an increase in trophic level. However, this might be the result of our limited sample size, and it might also just indicate that juvenile basket stars of different sizes feed on similar sources. Other studies have yielded enriched δ15N values for individuals of Gorgonocephalus spp. in Arctic waters, in comparison to our results (Hobson et al., 2002; Iken et al., 2010; Feder et al., 2011). However, it is quite possible that this reflects larger differences in ontogeny, as juveniles and adults live in different habitats. Despite Gorgonocephalus spp. usually being considered suspension feeders (Hobson et al., 2002; Iken et al., 2010; Feder et al., 2011), G. arcticus has been considered a predatory suspension feeder (Emson et al., 1991), and G. chilensis a predator (Mauna et al., 2011), because they extend their arms in a typical suspension feeder position, but trap prey by looping their arms.
Effects of Seasonality
When evaluating the potential effects of seasonality here, it is important to consider that the location of DFO trawl surveys is dependent on time of the year. For instance, Spring surveys take place in southern Newfoundland, while Fall surveys take place in Labrador. Therefore, we cannot exclude that variables affected by season might also be affected by latitude-related dynamics. However, phytoplankton and zooplankton data clearly show the importance of seasonality in this region (Afanasyev et al., 2001; Pepin et al., 2011). Furthermore, considering that seasonality is expected to play a role on reproductive aspects of many organisms (Gili and Petraitis, 2009), the patterns identified here are discussed in light of this variable, rather than latitude.
Seasonality – with the constraint highlighted above – seems to play a role on the disc diameter of basket stars found on D. glomerata, as specimens were significantly larger in the Spring than in the Summer and Fall, but no difference was identified between Spring and Winter. Similarly, the relative number of D. glomerata colonies with basket stars peaked during the Spring, with the lowest number of occurrences during Winter. Since we have no information on early growth rates in these ophiuroids, we cannot infer on the timing when most basket stars (embryo/larvae) arrived to the colonies.
In the Grand Banks region, Fall was the season with the highest co-occurrence of basket stars-Nephtheidae soft corals in the trawl sets, followed by Winter, Summer, and Spring. Because soft corals are not mobile organisms, this variation on co-occurrence rates ought to be driven by the basket stars. Seasonality might have an influence on the distribution of adult basket stars, who might migrate seasonally, as do other echinoderms (e.g., Freeman et al., 2001).
In the NE Pacific, G. eucnemis (published as G. caryi) has a spawning season of 6 months, from June to November (Patent, 1969), but to our knowledge there is currently no published information on reproduction of Gorgonocephalus spp. in the NW Atlantic. Our observations that juvenile basket stars (<5 mm in dd) were found on coral colonies collected at every month of the year indicate that the spawning season for Gorgonocephalus in this region might be longer than in the Pacific. On the other hand, no basket stars < 1 mm dd were collected in February, March, or April. Reproduction of Gorgonocephalus spp. was not in the scope of this study, and therefore more dedicated studies are needed to shade light on the subject.
The lack of a statistically significant influence of seasonality on basket star metrics for D. florida (i.e., abundance, disc diameter) could be related to their lower basket star occurrence in comparison to D. glomerata. However, despite non-significance, the number of D. florida colonies with basket stars followed the same trend as observed for D. glomerata, with a peak during the Spring and a low in the Winter time. For Gersemia spp., the peak was during Winter time, with the other seasons showing similar trends to one another.
The divergence in co-occurrence per season between physical samples and the trawl sets might be related to the different ontogenetic stages of the basket stars. While physical coral samples contained only juveniles, individuals accounted for in trawl sets are usually large enough to be picked up by hand (Wareham Hayes, personal communication), with sizes not comparable to those found on soft corals. On the other hand, trawl data was considered at the family level, and specific differences by genus (e.g., season of highest frequency of co-occurrence) were not accounted for.
Conservation
Gorgonocephalus arcticus has been found in stomach contents of Greenland sharks (Somniosus microcephalus) from Cumberland Sound, Nunavut (McMeans et al., 2012), and Svalbard, Norway (Leclerc et al., 2012), as well as in stomachs of Northern Wolfish Anarhichas denticulatus (Albikovskaya, 1983; Simpson et al., 2013), which is a species protected under the Canadian Species at Risk Act (SARA). Although this does not necessarily mean that basket stars are a common prey item for these fish, stomach content data for these species is limited and additional studies are needed to fully describe their diet. In the Barents Sea, G. arcticus, G. eucnemis, and G. lamarckii are considered “high-risk” species to bottom trawling (Jørgensen et al., 2015). Both soft corals and Gorgonocephalus spp. have been considered potential indicators of Vulnerable Marine Ecosystems (VMEs) in that region (e.g., Nadtochy et al., 2017), and are listed as indicators in the South Atlantic (as basket stars) by the South East Atlantic Fisheries Organisation – SEAFO CM 30/15 (Food and Agriculture Organization of the United Nations [FAO], 2020). Currently, Nephtheidae soft corals are considered VME indicators in the Northeast Atlantic (i.e., Cauliflower coral fields), South Pacific, and Southern Ocean, but not in the Northwest Atlantic (Food and Agriculture Organization of the United Nations [FAO], 2020). However, recently Long et al. (2020) suggested the inclusion of soft coral gardens from West Greenland as a VME candidate, as they met several of the Food and Agriculture Organization (FAO) VME indicator criteria, such as the structural complexity of the habitat they form.
Basket stars might be relatively long-lived, as some ophiuroids can live for decades (Gage, 1990). Therefore, evidence of the potential impact of the removal of soft corals and their associated juvenile basket stars through fishing on adult basket star populations might be delayed; i.e., the potential impact of high soft coral biomass removal on local adult basket star populations might only be detected several years after the fact. In this sense, our trawl data provided information on distributional patterns between the two taxa. However, temporal synchronicity of catch biomass for basket stars and soft corals cannot be investigated without considering the temporal lag highlighted above.
In this study we showed that in the Northwest Atlantic and Eastern Canadian Arctic juvenile basket stars are consistently found on soft corals, and we believe that further studies are necessary to investigate this association; in particular the potential degree of dependency of basket stars in relation to soft corals and the potential role of the latter as nurseries. This information is paramount to assess the potential impacts that soft coral damage and/or removal (e.g., via bottom-contact fishing) might have on juvenile basket stars, their development, and the success of adult Gorgonocephalus populations. Cold-water Nephtheidae soft corals appear to play an essential role as habitat for juvenile basket stars.
Data Availability Statement
The datasets presented in this study can be found in online repositories. The names of the repository/repositories and accession number(s) can be found in the article/Supplementary Material. Data not present in tables is available upon request to the corresponding author.
Author Contributions
BMN and VW contributed to conception and design of the study. BMN performed laboratory work, organized the database, performed the statistical analysis, and wrote the manuscript. EH and VW performed laboratory work and literature review. KH, CG, and PA contributed the Arctic databases on trawl data. All authors contributed to manuscript revision, read and approved the submitted version.
Conflict of Interest
The authors declare that the research was conducted in the absence of any commercial or financial relationships that could be construed as a potential conflict of interest.
Acknowledgments
We thank the Canadian Coast Guard captains and crew, and the Fisheries and Oceans Canada crew involved in sample collection and processing in the field. Samples collected aboard CCGS Amundsen (Agassiz trawl samples and data) were covered by ArcticNet funding. Laura Wheeland and Brynn Devine provided CFER samples. Special thanks to all involved with the Fisheries Observer Program, which provided invaluable material. We also thank Paula Hawkins, Sarah Olson, and Rebecca Raymonds (DFO-NL) for assistance in the lab, Alison Pye (Memorial University) for the stable isotope analyses, Matthew Harnden for the DNA barcoding analysis, and Hannah Munro for GenBank submissions. Mr. Klaus Hansen for translating the paper by Fedotov (1924) and Dr. Sabine Stöhrn for her guidance regarding the juvenile ophiuroid found inside of a coral polyp. We also thank three reviewers for their useful comments. The Deep-Sea Biology Society is also thanked for a travel grant provided to BMN to present this study. This study was funded by a DFO International Governance Strategy (IGS) project through a Natural Sciences and Engineering Research Council of Canada (NSERC) Postdoctoral Visiting Fellowship (BMN).
Supplementary Material
The Supplementary Material for this article can be found online at: https://www.frontiersin.org/articles/10.3389/fmars.2020.547896/full#supplementary-material
References
Afanasyev, Y. D., Nezlin, N. P., and Kostianoy, A. G. (2001). Patterns of seasonal dynamics of remotely sensed chlorophyll and physical environment in the Newfoundland region. Remote Sens. Environ. 76, 268–282. doi: 10.1016/S0034-4257(00)00209-1
Albikovskaya, L. K. (1983). Feeding characteristics of wolffishes in the labrador-newfoundland region. NAFO Sci. Coun. Stud. 6, 35–38.
Barboza, C. A. M., Mendes, F. M., Dalben, A., and Tommasi, L. R. (2010). Echinodermata, Ophiuroidea, Gorgonocephalus Leach, 1815: first report of the genus for the Brazilian continental margin. Check List. 6, 289–291. doi: 10.15560/6.2.289
Bell, J. J. (2008). The functional roles of marine sponges. Estuar. Coast. Shelf Sci. 79, 341–353. doi: 10.1016/j.ecss.2008.05.002
Bruntse, G., and Tendal, O. S. (1999). Marine Biological Investigations and Assemblages of Benthic Invertebrates from the Faroe Islands. Faroe Islands: Kaldbak Marine Biological Laboratory.
Buhl-Mortensen, L., and Mortensen, P. B. (2004a). Crustaceans associated with the deep-water gorgonian corals Paragorgia arborea (L., 1758) and Primnoa resedaeformis (Gunn., 1763). J. Nat. Hist. 38, 1233–1247. doi: 10.1080/0022293031000155205
Buhl-Mortensen, L., and Mortensen, P. B. (2004b). Symbiosis in deep-water corals. Symbiosis 37, 33–61.
Buhl-Mortensen, L., and Mortensen, P. B. (2005). “Distribution and diversity of species associated with deep-sea gorgonian corals off Atlantic Canada,” in Cold-Water Corals and Ecosystems, eds A. Freiwald and J. M. Roberts, (Berlin: Springer), 849–879. doi: 10.1007/3-540-27673-4_44
Buhl-Mortensen, L., Vanreusel, A., Gooday, A. J., Levin, L. A., Priede, I. G., Buhl-Mortensen, P., et al. (2010). Biological structures as a source of habitat heterogeneity and biodiversity on the deep ocean margins. Mar. Ecol. 31, 21–50. doi: 10.1111/j.1439-0485.2010.00359.x
Buhl-Mortensen, P., Buhl-Mortensen, L., and Purser, A. (2017). “Trophic ecology and habitat provision in cold-water coral ecosystems,” in Marine Animal Forests: The Ecology of Benthic Biodiversity Hotspots, eds S. Rossi, L. Bramanti, A. Gori, and O. Covadonga, (Cham: Springer), 919–944. doi: 10.1007/978-3-319-21012-4_20
Burdon-Jones, C., and Tambs-Lyche, H. (1960). Observations on the fauna of the North Brattholmen stone-coral reef near Bergen. Årbok for Universitetet i Bergen, Matematisknaturvitenskaplig Serie 4, 1–24.
Clippele, L. H., Buhl-Mortensen, P., and Buhl-Mortensen, L. (2015). Fauna associated with cold water gorgonians and sea pens. Cont. Shelf Res. 105, 67–78. doi: 10.1016/j.csr.2015.06.007
Dodou, D., Breedveld, P., De Winter, J. C. F., Dankelman, J., and Van Leeuwen, J. L. (2011). Mechanisms of temporary adhesion in benthic animals. Biol. Rev. 86, 15–32. doi: 10.1111/j.1469-185X.2010.00132.x
Dons, C. (1944). Norges korallrev. Det Kongelige Norske Videnskabers Selskabs Forhandlinger 16, 37–82.
Emson, R. H., Mladenov, P. V., and Barrow, K. (1991). The feeding mechanism of the basket star Gorgonocephalus arcticus. Can. J. Zool. 69, 449–455. doi: 10.1139/z91-070
Feder, H. M., Iken, K., Blanchard, A. L., Jewett, S. C., and Schonberg, S. (2011). Benthic food web structure in the southeastern chukchi sea: an assessment using δ13C and δ15N analyses. Polar Biol. 34, 521–532. doi: 10.1007/s00300-010-0906-9
Fedotov, D. M. (1924). Einige beobachtungen tiber die biologie und metamorphose von Gorgonocephalus. Zool. Anz. 61, 303–311.
Food and Agriculture Organization of the United Nations [FAO] (2020). VME indicators, thresholds and encounter responses adopted by R(F)MOs in force during 2019. Available at: http://www.fao.org/in-action/vulnerable-marine-ecosystems/vme-indicators/en/ (accessed March 31, 2020).
Försterra, G., Häussermann, V., and Laudien, J. (2017). “Animal forests in the chilean fjords: discoveries, perspectives, and threats in shallow and deep waters,” in Marine Animal Forests: The Ecology of Benthic Biodiversity Hotspots, eds S. Rossi, L. Bramanti, A. Gori, and O. Covadonga, (Cham: Springer), 277–313. doi: 10.1007/978-3-319-21012-4_3
Fourgon, D., Jangoux, M., and Eeckhaut, I. (2007). Biology of a “babysitting” symbiosis in brittle stars: analysis of the interactions between Ophiomastix venosa and Ophiocoma scolopendrina. Invertebr. Biol. 126, 385–395. doi: 10.1111/j.1744-7410.2007.00108.x
Freeman, S. M., Richardson, C. A., and Seed, R. (2001). Seasonal abundance, spatial distribution, spawning and growth of Astropecten irregularis (Echinodermata?: Asteroidea). Estuar. Coast. Shelf Sci. 53, 39–49. doi: 10.1006/ecss.2000.0758
Gage, J. D. (1990). Skeletal growth markers in the deep-sea brittle stars Ophiura ljungmani and Ophiomusium lymani. Mar. Biol. 104, 427–435. doi: 10.1007/BF01314346
Girard, F., Fu, B., and Fisher, C. R. (2016). Mutualistic symbiosis with ophiuroids limited the impact of the Deepwater Horizon oil spill on deep-sea octocorals. Mar. Ecol. Prog. Ser. 549, 89–98. doi: 10.3354/meps11697
Haedrich, R., and Maunder, J. (1984). “The echinoderm fauna of the Newfoundland continental slope,” in Proceedings of the 5th International Echinoderm Conference, Galway, 37–46. doi: 10.1201/9781003079224-7
Häussermann, V., and Fösterra, G. (2007). Extraordinary abundance of hydrocorals (Cnidaria, Hydrozoa, Stylasteridae) in shallow water of the Patagonian fjord region. Polar Biol. 30, 487–492. doi: 10.1007/s00300-006-0207-5
Hellström, M., and Benzie, J. A. H. (2011). Robustness of size measurement in soft corals. Coral Reefs 30:787. doi: 10.1007/s00338-011-0760-4
Hendler, G., Grygier, M. J., Maldonado, E., and Denton, J. (1999). Babysitting brittle stars: heterospecific symbiosis between ophiuroids (Echinodermata). Invertebr. Biol. 118, 190–201. doi: 10.2307/3227060
Hendler, G., and Miller, J. E. (1984). Feeding behavior of Asteroporpa annulata, a gorgonocephalid brittlestar with unbranched arms. Bull. Mar. Sci. 34, 449–460.
Herrero-Pérezrul, M. A. D., Granja-Fernández, R., Hoyos-Padilla, M., and Reyes-Bonilla, H. (2014). New record of the basket star Gorgonocephalus eucnemis (Ophiuroidea: Gorgonocephalidae) at the Pacific coast of Mexico. Mar. Biodivers. Rec. 7:e33. doi: 10.1017/S1755267214000323
Hobson, K. A., Fisk, A., Karnovsky, N., Holst, M., Gagnon, J.-M., and Fortier, M. (2002). A stable isotope (δ13C, δ15N) model for the North Water food web: implications for evaluating trophodynamics and the flow of energy and contaminants. Deep Sea Res. Part II Top. Stud. Oceanogr. 49, 5131–5150. doi: 10.1016/S0967-0645(02)00182-0
Iken, K., Bluhm, B., and Dunton, K. (2010). Benthic food-web structure under differing water mass properties in the southern Chukchi Sea. Deep Sea Res. Part II Top. Stud. Oceanogr. 57, 71–85. doi: 10.1016/j.dsr2.2009.08.007
Jensen, A., and Frederiksen, R. (1992). The fauna associated with the bank-forming deepwater coral Lophelia pertusa (Scleractinaria) on the Faroe shelf. Sarsia 77, 53–69. doi: 10.1080/00364827.1992.10413492
Jørgensen, L. L., Planque, B., Thangstad, T. H., and Certain, G. (2015). Vulnerability of megabenthic species to trawling in the Barents Sea. ICES J. Mar. Sci. J. du Cons. 73, i84–i97. doi: 10.1093/icesjms/fsv107
Kabacoff, R. (2015). R in Action: Data Analysis and Graphics with R, 2nd Edn. Shelter Island, NY: Manning.
Kim, D., and Shin, S. (2015). A newly recorded basket star of genus Gorgonocephalus (Ophiuroidea: Euryalida: Gorgonocephalidae) from the East Sea, Korea. Anim. Syst. Evol. Diversity 31:311. doi: 10.5635/ased.2015.31.4.311
Klitgaard, A. B. (1995). The fauna associated with outer shelf and upper slope sponges (Porifera, Demospongiae) at the Faroe Islands, northeastern Atlantic. Sarsia 80, 1–22. doi: 10.1080/00364827.1995.10413574
Krieger, K. J., and Wing, B. L. (2002). Megafauna associations with deepwater corals (Primnoa spp.) in the Gulf of Alaska. Hydrobiologia 471, 83–90. doi: 10.1023/A:1016597119297
Layton, K. K. S., Corstorphine, E. A., and Hebert, P. D. N. (2016). Exploring Canadian echinoderm diversity through DNA barcodes. PLoS One 11:e0166118. doi: 10.1371/journal.pone.0166118
Leclerc, L.-M. E., Lydersen, C., Haug, T., Bachmann, L., Fisk, A. T., and Kovacs, K. M. (2012). A missing piece in the Arctic food web puzzle? Stomach contents of Greenland sharks sampled in Svalbard, Norway. Polar Biol. 35, 1197–1208. doi: 10.1007/s00300-012-1166-7
Long, S., Sparrow-Scinocca, B., Blicher, M. E., Hammeken Arboe, N., Fuhrmann, M., Kemp, K. M., et al. (2020). Identification of a soft coral garden candidate Vulnerable Marine Ecosystem (VME) using video imagery, Davis Strait, West Greenland. Front. Mar. Sci. 7:460. doi: 10.3389/fmars.2020.00460
Mauna, A. C., Botto, F., Franco, B., Schwartz, J. M., Acha, E. M., Lasta, M. L., et al. (2011). Shifts in an epibenthic trophic web across a marine frontal area in the Southwestern Atlantic (Argentina). J. Sea Res. 66, 248–255. doi: 10.1016/j.seares.2011.08.005
McMeans, B. C., Arts, M. T., and Fisk, A. T. (2012). Similarity between predator and prey fatty acid profiles is tissue dependent in Greenland sharks (Somniosus microcephalus): implications for diet reconstruction. J. Exp. Mar. Bio. Ecol. 429, 55–63. doi: 10.1016/j.jembe.2012.06.017
Metaxas, A., and Davis, J. (2005). Megafauna associated with assemblages of deep-water gorgonian corals in Northeast Channel, off Nova Scotia, Canada. J. Mar. Biol. Assoc. U.K. 85, 1381–1390. doi: 10.1017/S0025315405012567
Mortensen, T. H. (1927). Handbook of the Echinoderms of the British Isles. Humphrey Milford: Oxford University Press.
Mosher, C. V., and Watling, L. (2009). Partners for life: a brittle star and its octocoral host. Mar. Ecol. Prog. Ser. 397, 81–88. doi: 10.3354/meps08113
Murillo, F. J., Durán, M. P., Altuna, A., and Serrano, A. (2011). Distribution of deep-water corals of the Flemish Cap, Flemish Pass, and the Grand Banks of Newfoundland (Northwest Atlantic Ocean): interaction with fishing activities. ICES J. Mar. Sci. J. du Cons. 68, 319–332. doi: 10.1093/icesjms/fsq071
Nadtochy, V. A., Kolpakov, N. V., and Korneichuk, I. A. (2017). The distribution of macrozoobenthos taxa, as potential indicators of Vulnerable Marine Ecosystems in the Western Bering Sea: 1. Anadyr Bay area. Russ. J. Mar. Biol. 43, 555–567. doi: 10.1134/S1063074017070033
Neves, B. M., and Wareham Hayes, V. (2019). “Distribution patterns of basket stars (Gorgonocephalidae) in the Northwest Atlantic,” in Proceedings of the Benthic Ecology Meeting, St. John’s.
Patent, D. H. (1969). The reproductive cycle of Gorgonocephalus caryi (Echinodermata; Ophiuroidea). Biol. Bull. 136, 241–252. doi: 10.2307/1539817
Patent, D. H. (1970a). Life history of the basket star, Gorgonocephalus eucnemis (Müller & Troschel) (Echinodermata; Ophiuroidea). Ophelia 8, 145–159. doi: 10.1080/00785326.1970.10429556
Patent, D. H. (1970b). The early embryology of the basket star Gorgonocephalus caryi (Echinodermata, Ophiuroidea). Mar. Biol. 6, 262–267. doi: 10.1007/BF00347235
Pepin, P., Colbourne, E., and Maillet, G. (2011). Seasonal patterns in zooplankton community structure on the Newfoundland and Labrador Shelf. Prog. Oceanogr. 91, 273–285. doi: 10.1016/j.pocean.2011.01.003
Pepin, P., Higdon, J., Koen-Alonso, M., Fogarty, M., and Ollerhead, N. (2014). Application of Ecoregion Analysis to The Identification of Ecosystem Production Units (EPUs) in the NAFO Convention Area. NAFO SCR Doc. 14/069. Dartmouth: NAFO.
Pinheiro, J., Bates, D., DebRoy, S., Sarkar, D., and R Core Team. (2020). nlme: Linear and Nonlinear Mixed Effects Models. R Package Version 3. 1–149. Available online at: https://CRAN.R-project.org/package=nlme
Portela, J., Acosta, J., Cristobo, J., Muoz, A., Parra, S., Ibarrola, T., et al. (2012). “Management strategies to limit the impact of bottom trawling on VMEs in the High Seas of the SW Atlantic,” in Marine Ecosystems, ed. J. Acosta, (Rijeka: IntechOpen).
Rosenberg, R., Dupont, S., Lundälv, T., Sköld, H. N., Norkko, A., Roth, J., et al. (2005). Biology of the basket star Gorgonocephalus caputmedusae (L.). Mar. Biol. 148:43. doi: 10.1007/s00227-005-0032-3
RStudio Team (2016). RStudio: Integrated Development Environment for R. Available at: http://www.rstudio.com/
Sherwood, O. A., Jamieson, R. E., Edinger, E. N., and Wareham, V. E. (2008). Stable C and N isotopic composition of cold-water corals from the Newfoundland and Labrador continental slope: examination of trophic, depth and spatial effects. Deep Sea Res. Part I Oceanogr. Res. Pap. 55, 1392–1402. doi: 10.1016/j.dsr.2008.05.013
Simpson, M. R., Sherwood, G. D., Mello, L. G. S., Miri, C. M., and Kulka, D. W. (2013). Feeding Habits and Trophic Niche Differentiation in Three Species of Wolffish (Anarhichas sp.) Inhabiting Newfoundland and Labrador Waters. DFO Can. Sci. Advis. Sec. Res. Doc. 2013/056. Canada: DFO.
Slattery, M., McClintock, J. B., and Heine, J. N. (1995). Chemical defenses in Antarctic soft corals: evidence for antifouling compounds. J. Exp. Mar. Bio. Ecol. 190, 61–77. doi: 10.1016/0022-0981(95)00032-M
Stöhr, S., O’Hara, T. D., and Thuy, B. (2012). Global diversity of brittle stars (Echinodermata: Ophiuroidea). PLoS One 7:e0031940. doi: 10.1371/journal.pone.0031940
Storm, V. (1901). “Oversigt over Throndheimsfjordens fauna (med et kort),” in Trondhjems Biologiske Station, Meddelelser fra stationsanleggets arbeidskomite, ed. H. Moe’s Bog (Trondhjem: Accidentstrykkeri), 20.
Turon, X., Codina, M., Tarjuelo, I., and Uriz, M. J. (2000). Mass recruitment of Ophiothrix fragilis (Ophiuroidea) on sponges: settlement patterns and post-settlement dynamics. Mar. Ecol. Prog. Ser. 200, 201–212. doi: 10.3354/meps200201
Utinomi, H. (1961). A revision of the nomenclature of the family Nephtheidae (Octocorallia: Alcyonacea) II. The boreal genera Gersemia, Duva, Drifa and Pseudodrifa. Publ. Seto Mar. Biol. Lab. 9, 229–246.
Vander Zanden, M. J., and Rasmussen, J. B. (2001). Variation in δ15N and δ13C trophic fractionation: implications for aquatic food web studies. Limnol. Oceanogr. 46, 2061–2066. doi: 10.4319/lo.2001.46.8.2061
Vaughn, C. C., and Hakenkamp, C. C. (2008). The functional role of burrowing bivalves in freshwater ecosystems. Freshw. Biol. 46, 1431–1446. doi: 10.1046/j.1365-2427.2001.00771.x
Wahl, M. (1999). The predominantly facultative nature of epibiosis: experimental and observational evidence. Mar. Ecol. Prog. Ser. 187, 59–66.
Wareham, V. E. (2009). “Updates on deep-sea coral distributions in the Newfoundland and Labrador and Arctic Regions, Northwest Atlantic,” in The Ecology of Deep-Sea Corals of Newfoundland and Labrador Waters: Biogeography, Life History, Biogeochemistry, and Relation to Fishes, eds K. Gilkinson and E. Edinger, (London: Canadian Technical Report on Fisheries Aquatic Sciences).
Wareham, V. E., and Edinger, E. N. (2007). Distribution of deep-sea corals in the Newfoundland and Labrador region, Northwest Atlantic Ocean. Bull. Mar. Sci. 81, 289–313.
Watling, L., France, S. C., Pante, E., and Simpson, A. (2011). “Biology of deep-water octocorals,” in Advances in Marine Biology Advances in Marine Biology, ed. C. Sheppard, (Cambridge, MA: Academic Press), 41–122.
Wheeler, A. J., Beck, T., Thiede, J., Klages, M., Grehan, A., and Monteys, F. X. (2005). “Deep-water coral mounds on the Porcupine Bank, Irish Margin: preliminary results from the Polarstern ARK-XIX/3a ROV cruise,” in Cold-Water Corals and Ecosystems, eds A. Freiwald and J. M. Roberts, (Berlin: Springer), 393–402.
Wild, C., Mayr, C., Wehrmann, L., Schöttner, S., Naumann, M., and Hoffmann, F. (2008). Organic matter release by cold water corals and its implication for fauna–microbe interaction. Mar. Ecol. Prog. Ser. 372, 67–75.
Williams, D., Andersen, R. J., Van Duyne, G. D., and Clardy, J. (1987). Cembrane and pseudopterane diterpenes from the soft coral Gersemia rubiformis. J. Org. Chem. 52, 332–335. doi: 10.1021/jo00379a002
Wood, S. N. (2011). Fast stable restricted maximum likelihood and marginal likelihood estimation of semiparametric generalized linear models. J. R. Stat. Soc. Ser. B Stat. Methodol. 73, 3–36. doi: 10.1111/j.1467-9868.2010.00749.x
Keywords: cold-water corals, Nephtheidae, functional role, habitat, basket stars, Gorgonocephalus, juveniles
Citation: Neves BM, Wareham Hayes V, Herder E, Hedges K, Grant C and Archambault P (2020) Cold-Water Soft Corals (Cnidaria: Nephtheidae) as Habitat for Juvenile Basket Stars (Echinodermata: Gorgonocephalidae). Front. Mar. Sci. 7:547896. doi: 10.3389/fmars.2020.547896
Received: 01 April 2020; Accepted: 25 August 2020;
Published: 09 October 2020.
Edited by:
Santiago Herrera, Lehigh University, United StatesReviewed by:
Steven Y. Litvin, Monterey Bay Aquarium Research Institute (MBARI), United StatesLes Watling, University of Hawai‘i at Mānoa, United States
Fanny Girard, Institut Français de Recherche pour l’Exploitation de la Mer (IFREMER), France
Copyright © 2020 Neves, Wareham Hayes, Herder, Hedges, Grant and Archambault. This is an open-access article distributed under the terms of the Creative Commons Attribution License (CC BY). The use, distribution or reproduction in other forums is permitted, provided the original author(s) and the copyright owner(s) are credited and that the original publication in this journal is cited, in accordance with accepted academic practice. No use, distribution or reproduction is permitted which does not comply with these terms.
*Correspondence: Bárbara de Moura Neves, barbara.neves@dfo-mpo.gc.ca