Enhanced Production of Astaxanthin by Co-culture of Paracoccus haeundaensis and Lactic Acid Bacteria
- 1Department of Microbiology, College of Natural Sciences, Pukyong National University, Busan, South Korea
- 2Cbs Bioscience Co., Ltd., Daejeon, South Korea
- 3Biomedical Engineering & Biotechnology Major, Division of Applied Bioengineering, College of Engineering, Dong-Eui University, Busan, South Korea
- 4Department of Smart-Biohealth, Dong-Eui University, Busan, South Korea
This study aimed to determine the increase in astaxanthin production from Paracoccus haeundaensis by optimizing the medium conditions and co-culturing with lactic acid bacteria. In order to select a suitable strain to co-culture with P. haeundaensis, several strains were co-cultured with P. haeundaensis. Lactobacillus fermentum was selected as the optimal strain. Further to optimize the astaxanthin production in the co-culture, various medium conditions were tested and the PMF medium developed in this study was selected as the optimum medium. For the co-culture under optimal conditions, the dried cell mass and astaxanthin concentration were 1.84 ± 0.09 g/L and 821.09 ± 30.98 μg/g-dried cell weight, respectively, which was 2.5 times higher than that of the P. haeundaensis strain in the Luria-Bertani broth medium. In order to confirm the transcription level of the carotenoid biosynthesis genes such as crtE, crtB, crtI, crtY, crtZ, and crtW of P. haeundaensis in the co-culture, real-time qPCR was performed. Among the six carotenoid biosynthesis genes, crtI, crtY, and crtZ showed a significant difference in relative RNA levels. The mass culture was performed using a 5 L jar fermenter and the astaxanthin concentration was the same as that at the laboratory scale. These results may be used as reference for co-culture and astaxanthin production in carotenoid biosynthesis microorganisms.
Introduction
Carotenoids are fat-soluble pigments that are widely present in nature and have red, orange, and yellow colors. These pigments are biosynthesized from bacteria, algae, yeast, fungi, and plants, and play essential roles in photosynthesis, such as electron transport and photoprotection (Domonkos et al., 2013). Also, carotenoids have essential physiological activities, such as antioxidant, anti-inflammatory, and anticancer activities in mammalian cells (An et al., 1991; Kobayashi et al., 1993; Castelblanco-Matiz et al., 2015). Carotenoids mainly have 40 carbon atoms backbones and are classified as either carotenes, which consist of only carbon and hydrogen atoms, and xanthophylls with cyclic or acyclic functional groups containing oxygen (Emmerstorfer-Augustin et al., 2016). Currently, more than 1,000 different carotenoids are known (Yabuzaki, 2017), and these carotenoids are listed on the website http://carotenoiddb.jp. Among the carotenoids, astaxanthin has excellent physiological activity in humans including antioxidant and anti-inflammatory effects (Fassett and Coombes, 2009, 2011), and is used in cosmetic additives, dietary supplements, and aquaculture feed. In order to produce astaxanthin industrially, Haematococcus pluvialis and Phaffia rhodozyma have been used for decades (Miller et al., 1976; Bubrick, 1991), and research has been conducted to produce astaxanthin from various microorganisms (Ide et al., 2012; Watanabe et al., 2018; Jhang et al., 2020).
Many studies have reported an increase in the production of carotenoids from carotene-producing microorganisms. The methods used to increase carotenoid production include mutations of microorganisms by chemical and physical mutagens (Seo et al., 2017), stress during microbial culture (Li et al., 2019), and the co-culture of microorganisms with other microorganisms. Co-culture methods have been used to produce a variety of valuable chemicals from microorganisms (Jones and Wang, 2018). Some studies have revealed that carotenoid production is increased when different microorganisms are co-cultured with carotenoid-producing microorganisms. Studies on carotenoid production using co-culture include Rhodotorula glutinis and Debaryomyces castellii in corn syrup (Buzzini, 2001), R. glutinis and Chlorella vulgaris in starch waste water (Zhang et al., 2019), R. rubra, and Lactobacillus casei in whey ultrafiltrate (Simova et al., 2003), R. rubra and yogurt starter in whey ultrafiltrate (Simova et al., 2004) and H. pluvialis and B. subtilis in starch-containing waste water (Bohutskyia et al., 2018). According to these studies, the cell mass and carotenoid production increased in the raw materials and byproducts of agro-industrial origin, such as corn syrup, whey, and starch wastewaters. These studies show that the production of carotenoids in carotenoid-producing microorganisms is increased by interactions with other bacteria, which aid in the use of nutrients in the media (such as starch and whey) that are not available to carotenoid-producing microorganisms.
Paracoccus haeundaensis is known as a strain that produces astaxanthin. However, it is difficult to use commercially due to low astaxanthin productivity. For this reason, in order to increase the production of astaxanthin from Paracoccus sp. strains, mainly physicochemical mutation methods were studied (Ide et al., 2012; Seo et al., 2017). However, no studies were reported to increase the production of astaxanthin using co-culture of Paracoccus sp. strains. The purpose of this study was to determine the production of astaxanthin using a co-culture method to establish the optimal production conditions for P. haeundaensis and to increase astaxanthin production. In order to increase the production of carotenoids in the P. haeundaensis strain, lactic acid bacteria and Bacillus subtilis were co-cultured with P. haeundaensis by changing the composition of the medium. This medium referred to the composition of LB medium (Luria-Bertani medium) known as nutrient medium and PPES-II medium for culturing marine bacteria. After optimizing the medium composition, lactic acid bacteria strains were selected for co-culture with P. haeundaensis. P. haeundaensis has been reported to possess the crtE, crtB, crtI, crtY, crtZ, and crtW genes required for the biosynthesis of astaxanthin (Figure 1), and astaxanthin production is directly related to the expression of these genes. To analyze the cause of the increase in astaxanthin production, The cell mass and astaxanthin produced were analyzed after the medium composition and strain were selected, and expression patterns of the carotenoid biosynthesis genes were analyzed using real-time quantitative polymerase chain reaction (qPCR). Paracoccus haeundaensis and lactic acid bacteria were co-cultured in a 5 L jar fermenter, and the production of the cell mass and astaxanthin were confirmed.
Materials and Methods
Bacterial Strains and Growth Conditions
The P. haeundaensis (KCCM 10460) strain was cultured at 25°C for 24 h using LB medium. Lactobacillus sakei (KCCM 40264), L. casei (KCCM 35465), L. brevis (KCCM 11509), L. fermentum (KCCM 35461), L. helveticus (KCCM 11223), Enterococcus faecium (KCCM 12117), E. durans (KCCM 40711), E. lactis (KCTC 21015), and Bacillus subtilis (KCCM 11316) were cultured at 37°C for 24 h in MRS medium (10 g peptone, 10 g beef extract, 4 g yeast extract, 20 g glucose, 5 g sodium acetate trihydrate, 1 g polysorbate 80, 2 g dipotassium hydrogen phosphate, 2 g triammonium citrate, 0.2 g magnesium sulfate heptahydrate, 0.05 g manganese sulfate tetrahydrate, and distilled water 1 L). In a previous study, P. haeundaensis was found to have optimal growth and astaxanthin production at 25°C; thus, the co-culture of P. haeundaensis and lactic acid bacteria was performed at 25°C (Lee et al., 2004).
Co-culture of Paracoccus haeundaensis With Lactic Acid Bacteria
The co-culture of P. haeundaensis with lactic acid bacteria was performed to increase the production of astaxanthin. Paracoccus haeundaensis were pre-cultured for 24 h at 25°C in LB medium, and the lactic acid bacteria strains for the co-culture were pre-cultured for 24 h at 37°C in MRS medium. After pre-culture, 1 L of the LB medium was inoculated with 10% volume of the P. haeundaensis cultured medium and each of the lactic acid bacteria culture media, then incubated at 25°C for 72 h. After incubation, the cells cultured in each medium were centrifuged at 4,000 rpm for 30 min, and the supernatant was removed and washed three times with physiological saline. After the collected cells were dried using a freeze dryer (FDU-2200, Eyela, Japan), the weight of the dried cell mass was measured. The freeze-dried cell mass samples were stored at −20°C.
Cell Mass and Astaxanthin Production by Medium Composition
The media MLB (8 g tryptone, 5 g bacto yeast extract, 10 g NaCl, and 1 L distilled water), PPES-II (0.2 g polypeptone, 1.0 g bacto soytone, 1.0 g proteose peptone, 1.0 g bacto yeast extract, 30 g NaCl, 0.1 g ferric citrate, and 1 L distilled Water), MLB50 (5 g tryptone, 2.5 g bacto yeast extract, 10 g NaCl, and 1 L distilled water), PM (4 g tryptone, 0.2 g polypeptone, 1.0 g bacto soytone, 1.0 g proteose peptone, 3.5 g bacto yeast extract, 3% NaCl, 0.1% ferric citrate, and 1 L distilled water) were prepared. A co-culture of P. haeundaensis and L. fermentum was incubated for 72 h at 25°C in each of the prepared media. After freeze-drying, the weight of the dried cell mass was measured.
In some studies, there were reported that Fe2+ ions enhance the production of astaxanthin (Fraser et al., 1997; Zhou et al., 2015). Based on these studies, in order to confirm the production of the cell mass and astaxanthin depending to the concentration of ferric citrate, ferric citrate was added into the PM medium at final concentrations of 0, 0.1, 0.05, 0.01, 0.005, and 0.001%, respectively. Paracoccus haeundaensis and L. fermentum were inoculated in each medium followed by incubation at 25°C for 72 h. The cells cultured in each medium were centrifuged and washed three times with physiological saline. After the collected cells were dried, the weight of the dried cell mass was measured. The freeze-dried samples were stored at −20°C.
Cell Mass and Astaxanthin Production by the Inoculation Ratio
To confirm the ratio optimization of the inoculation for the co-culture, the amount of the culture solution of each strain was inoculated differently. Both cultures used for the inoculation were cultured for 24 h. To confirm the optimal inoculation conditions of the L. fermentum culture, the inoculation of the P. haeundaensis culture was kept at 10% of the volume of medium to be co-cultured, while L. fermentum cultures were inoculated with 0, 1, 5, 10, 15, and 20% of the volume of medium to be co-cultured. The optimum conditions for the P. haeundaensis culture were also confirmed by the same method. After washing with physiological saline and freeze-drying, the weight of the dried cell mass was measured.
Carotenoid Extraction From Bacteria and Astaxanthin Analysis by High Performance Liquid Chromatography
In order to analyze the production of astaxanthin produced from the collected cell samples, 0.1 g of the freeze-dried sample was treated with chloroform: methanol (v/v, 6:4), followed by vortexing at 3 h intervals for 24 h at room temperature in a dark room. After centrifugation at 4,000 rpm for 30 min, the upper solvent was collected and filtered through a 0.22 μm filter. The pretreated extract and standard sample (Sigma, USA) were subjected to high performance liquid chromatography (HPLC). The analysis was performed using an Alliance e2695 Separations Module (Waters, USA), and the column was Poroshell 120 EC-C18 (4.6 × 250 mm) (Agilent, USA). Methanol:acetonitrile (v/v, 80:20) mixed solvent was used as the mobile phase solvent, and the absorbance was measured at 470 nm.
Real-time Quantitative Polymerase Chain Reaction for the RNA Level of the Carotenoid Biosynthesis Genes
Real-time qPCR was performed with cDNA synthesized from P. haeundaensis total RNA. The primers used for the cDNA synthesis and real-time qPCR of carotenoid biosynthesis genes were designed using the astaxanthin biosynthesis gene cluster (AY957386.1) of P. haeundaensis, and the primers of rpoB gene for housekeeping gene was designed using genome sequence of Paracoccus marcusii (NZ_VDDD01000029.1). The primers are shown in Table 1. The equipment used for the real-time qPCR was QuantStudio 6 Flex (Thermo Fisher, US), and the reagents were AccuPower® 2X GreenStar™ qPCR Master Mix (Bioneer, Daejeon, Korea). The real-time qPCR was performed under conditions of 15 s at 95°C denaturation, 20 s at 58°C annealing, and 20 s at 72°C extension. The RNA levels of each gene were analyzed with Ct values and normalized to the rpoB gene transcript level (Dahllöf et al., 2000; Rodrigues and Tiedje, 2007).
Scale-Up With Optimum Production Conditions
To mass-produce astaxanthin from the P. haeundaensis strain, P. haeundaensis, and L. fermentum strains were co-cultured in a 5 L jar fermenter. For use as a seed culture, the P. haeundaensis strain was cultured for 24 h at 25°C in LB medium and the L. fermentum strain was cultured for 24 h at 37°C in MRS medium. The medium used for the mass culture of the strains was the PMF medium. The mass culture was inoculated with a seed culture of 10% of the volume to be cultured. The culture was incubated at 25°C with shaking at 160 rpm and cultured for 72 h at 1 vvm of aeration.
Statistical Analysis
The experimental results of all groups were expressed as the mean ± standard deviation (mean ± SD), and the significance test of the control group and the experimental group was confirmed by a Student's t-test. When the significance probability was p < 0.05, it was determined that there was a significant difference between the control and experimental groups.
Results
Selection of Lactic Acid Bacteria for Co-Culture With Paracoccus haeundaensis
To increase the production of astaxanthin from P. haeundaensis, lactic acid bacteria were co-cultured with P. haeundaensis. The cell mass and astaxanthin concentrations of the co-cultures are shown in Figure 2. The co-culture of P. haeundaensis with L. fermentum, L. sakei, L. brevis, and L. casei significantly increased the cell mass and astaxanthin concentration compared to the monoculture of P. haeundaensis (p < 0.05). In particular, the cell mass of the co-culture of P. haeundaensis and L. fermentum was the highest at 2.33 ± 0.18 g/L, which was 1.28 times higher than that of 1.82 ± 0.12 g/L of P. haeundaensis alone.
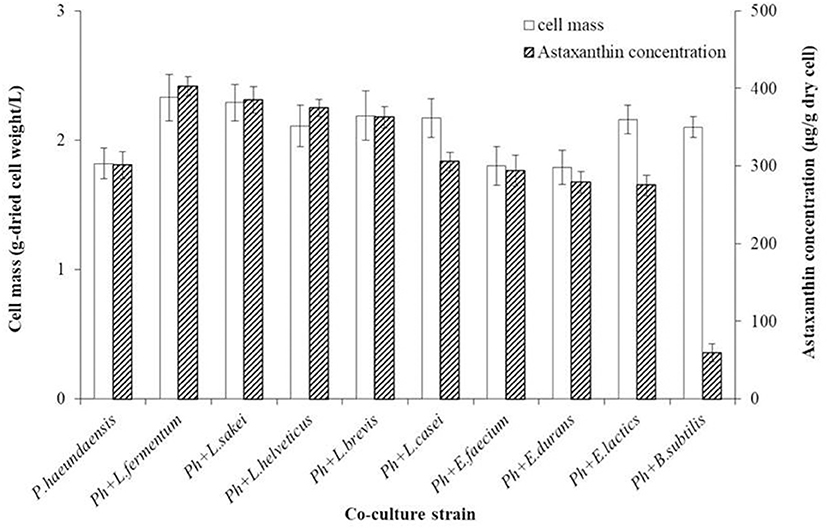
Figure 2. Comparison of cell mass and astaxanthin concentration by co-culture of Paracoccus haeundaensis and lactic acid bacteria.
The cell mass and astaxanthin concentrations from each co-culture using different lactic acid bacteria were compared. Astaxanthin concentrations were highest in the co-culture of P. haeundaensis and L. fermentum at 402.84 ± 12.60 μg/g-dried cell weight, which was 1.34 times higher than that of P. haeundaensis alone (301.14 ± 17.43 μg/g-dried cell weight). In addition, all co-cultures of P. haeundaensis and Lactobacillus spp. strains showed higher astaxanthin concentrations than P. haeundaensis monoculture, while the co-culture of P. haeundaensis with other genera, such as Enterococcus spp. and Bacillus subtilis, were even lower than those of P. haeundaensis.
When the amount of astaxanthin produced in 1 L of medium was calculated, the co-culture of P. haeundaensis and L. fermentum was highest at about 938.61 μg. This value is approximately 1.71 times higher than the 548.07 μg produced by P. haeundaensis, and 1.06 times higher than the 881.99 μg produced in the co-culture of P. haeundaensis and L. sakei. Therefore, L. fermentum was selected as the optimal strain for co-culture with P. haeundaensis.
Analysis of Astaxanthin Production According to Medium Composition
The LB medium is a basic nutrient medium widely used for bacterial cultures, and the PPES-II medium is a marine bacterial culture medium used for culturing P. haeundaensis in a previous study (Lee et al., 2004). Based on these two media, the optimum composition was prepared by modifying the media composition. Paracoccus haeundaensis and L. fermentum were co-cultured in LB, MLB, MLB50, PM, and PPES-II media in order to optimize the medium to increase the cell mass and astaxanthin concentration.
The highest yield of cell mass was 2.34 ± 0.08 g/L in the LB medium, while the cell mass in MLB and MLB50 was 2.29 ± 0.09 g/L and 1.66 ± 0.08 g/L, respectively. The lower the nutrient content of the medium, the less cell mass was produced. In the PPES-II medium, which contained lower nutrient contents than the modified LB medium, the cell mass was 0.36 ± 0.02 g/L. In contrast, the astaxanthin concentrations in the LB, MLB, and MLB50 were 401.46 ± 28.31 μg/g-dried cell weight, 420.63 ± 28.31 μg/g-dried cell weight, and 480.66 ± 23.65 μg/g-dried cell weight, respectively, and the astaxanthin concentration increased with decreasing nutrients in the LB medium. The astaxanthin concentration in the PPES-II medium was 490.12 ± 27.36 μg/g-dried cell weight. In order to supplement the astaxanthin production in the MLB50 and PPES-II media, the PM medium was prepared, which is a combination of the MLB50 and PPES-II media. The cell mass and astaxanthin concentration in PM were 1.82 ± 0.06 g/L and 560.31 ± 29.97 μg/g-dried cell weight, respectively, which was higher than that in the MLB50 and PPES-II media (Figure 3A).
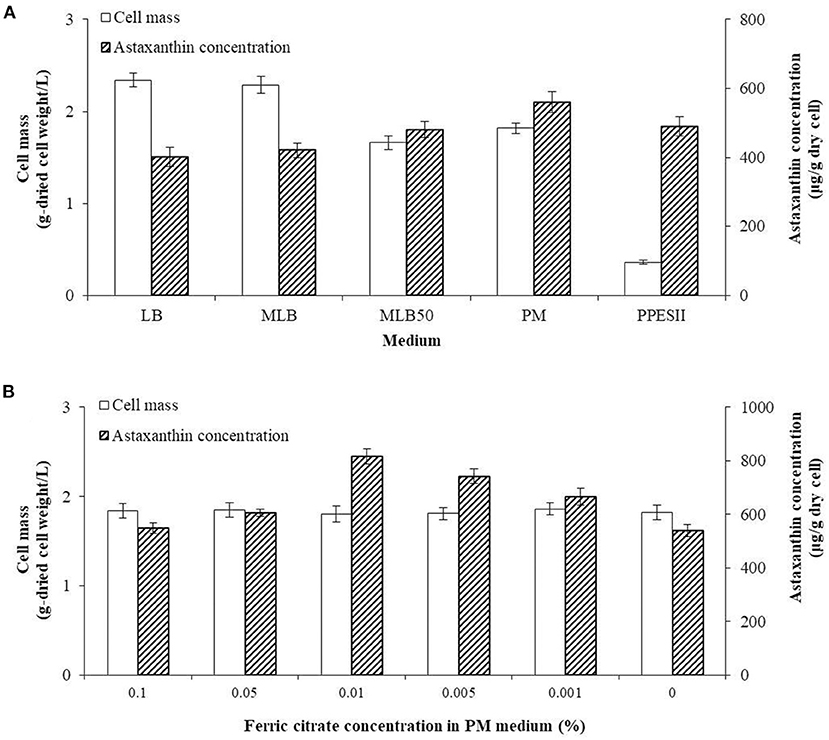
Figure 3. Comparison of cell mass and astaxanthin concentration by medium composition. (A) Optimizing the concentration of the dried cell mass and astaxanthin by varying the type of medium. (B) The concentration of the cell mass and astaxanthin produced after varying the concentration of ferric citrate in the PM medium.
To optimize the production of the co-culture cells and astaxanthin, the co-culture was performed in PM with different ferric citrate concentrations. The production of the cells did not significantly differ among the different concentrations of ferric citrate. As the concentration of ferric citrate in the PM medium changed, the astaxanthin concentration gradually increased from 548.16 ± 18.97 μg/g -dried cell weight at 0.1% ferric citrate to 817.49 ± 28.73 μg/g-dried cell weight at 0.01%. However, when the concentration of ferric citrate was lower than 0.01%, the astaxanthin concentration decreased (Figure 3B).
As a result of this experiment, we determined that the optimal medium for producing astaxanthin in the co-culture was a PM medium containing 0.01% ferric citrate, which was named as the PMF medium.
Analysis of Astaxanthin Production According to Inoculation Ratio
In order to confirm the optimal inoculation amount in the co-culture, the inoculation amount of P. haeundaensis was fixed at 10%, and L. fermentum was inoculated at different concentrations in the PMF medium. The cell mass and astaxanthin concentrations increased gradually up to 10% of the inoculation amount of P. haeundaensis, and the cell mass and astaxanthin concentrations were highest at 1.85 ± 0.07 g/L and 817.49 ± 21.84 μg/g-dried cell weight, respectively, when the inoculation amount of L. fermentum was 10%. However, when the inoculum of L. fermentum was 15%, the cell mass and astaxanthin concentration decreased to 1.31 ± 0.09 g/L and 294.36 ± 22.07 μg/g-dried cell weight, respectively (Figure 4A).
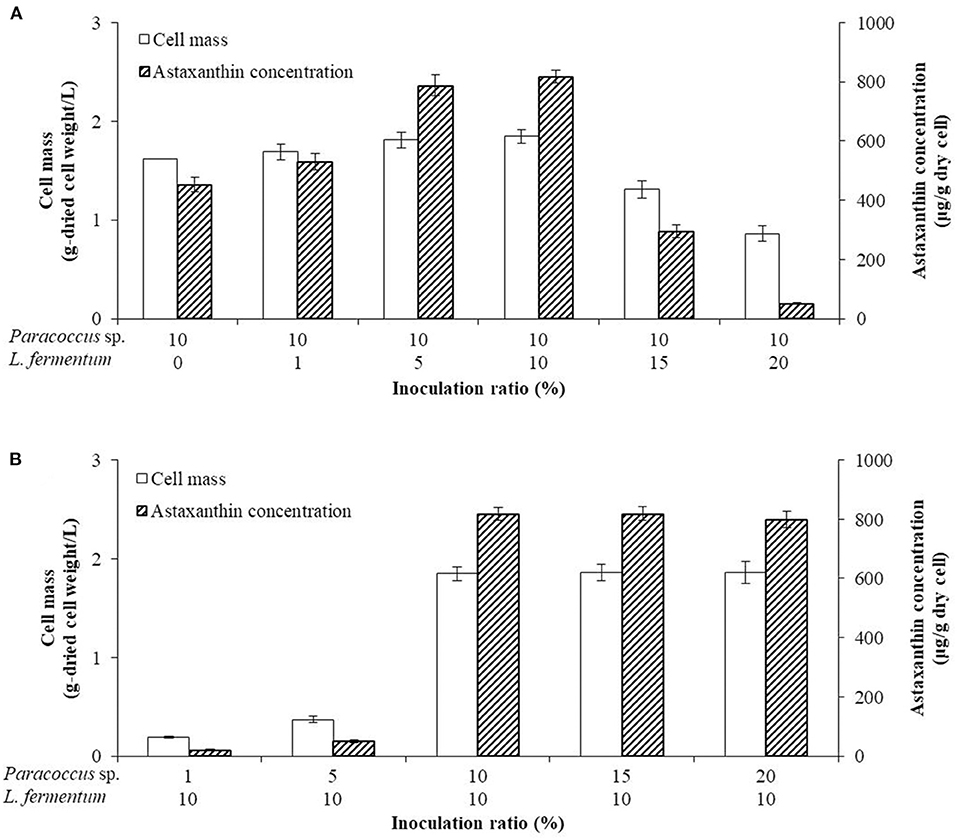
Figure 4. Comparison of cells and astaxanthin concentrations by the ratio of inoculation. The optimization of the inoculation ratio of the two strain cultures was confirmed by (A) fixing the inoculation of Paracoccus haeundaensis at 10% and varying the inoculation volume of Lactobacillus fermentum; and (B) fixing the inoculation of L. fermentum at 10% and varying the inoculation volume of P. haeundaensis.
The inoculation amount of L. fermentum was fixed at 10%, and P. haeundaensis was inoculated at different concentrations. The cell mass and astaxanthin concentration at 1% of P. haeundaensis were 0.19 ± 0.01 g/L and 21.23 ± 1.99 μg/g-dried cell weight, respectively, and at 5% were 0.37 ± 0.03 g/L and 49.93 ± 3.39 μg/g-dried cell weight, respectively. In addition, the cell mass and concentration of astaxanthin at 15% of P. haeundaensis were 1.86 ± 0.08 g/L and 818.63 ± 22.69 μg/g-dried cell weight, respectively. Compared with the results of the 10% inoculation of P. haeundaensis, the cell mass and astaxanthin concentration in the 1 and 5% P. haeundaensis inoculations showed very low productivity, and the production at more than 15% P. haeundaensis was not significantly different compared to the production at 10% (Figure 4B). From these experiments, it was confirmed that the productivity was the highest when the inoculation amounts of P. haeundaensis and L. fermentum were both at 10%. In order to confirm the production of cell mass over time, the growth curve was confirmed while incubating for 72 h. Cell mass was measured at 3 h intervals. At the end of the experiment, the concentration of dried cells in co-culture was 1.82 ± 0.05 g/L, which was slightly increased compared to 1.64 ± 0.03 g/L in a single culture of P. haeundaensis. In contrast, the dried cell mass of L. fermentum single culture was 0.23 ± 0.02 g/L, and it was confirmed that the growth rate was slow at 25°C incubation temperature (Figure 5).
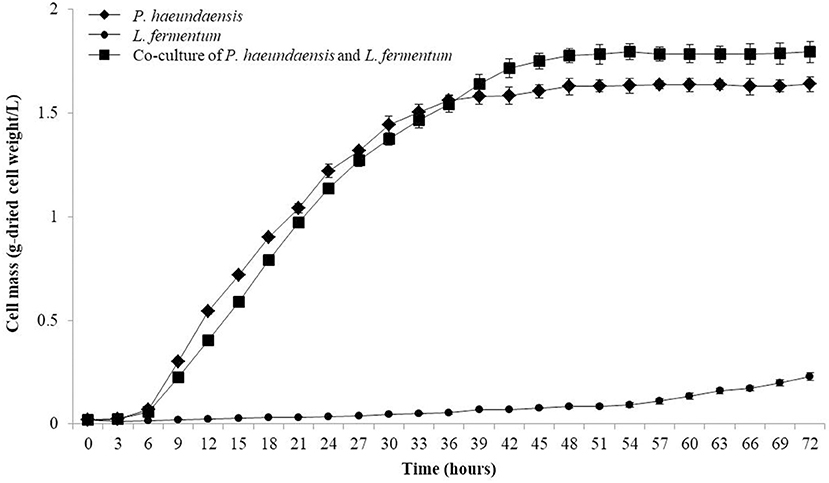
Figure 5. Growth curve of P. haeundaensis single culture, L. fermentum single culture and co-culture of P. haeundaensis, and L. fermentum.
Total Carotene in the Co-Culture of Paracoccus haeundaensis and Lactobacillus fermentum
To confirm the effect of media optimization on carotenoid synthesis, the carotenoids were analyzed by culturing P. haeundaensis in the LB and PMF media, respectively. In addition, to confirm the carotenoid change in the co-culture, carotenoids were analyzed from the co-culture of P. haeundaensis and L. fermentum in the PMF medium and compared with the results of P. haeundaensis single culture. The quantitative analysis of the carotenoids produced from P. haeundaensis in the LB and PMF medium and the co-culture in the PMF medium used zeaxanthin, canthaxanthin, β-carotene, and lycopene standards in HPLC analysis and are shown in Figure 6.
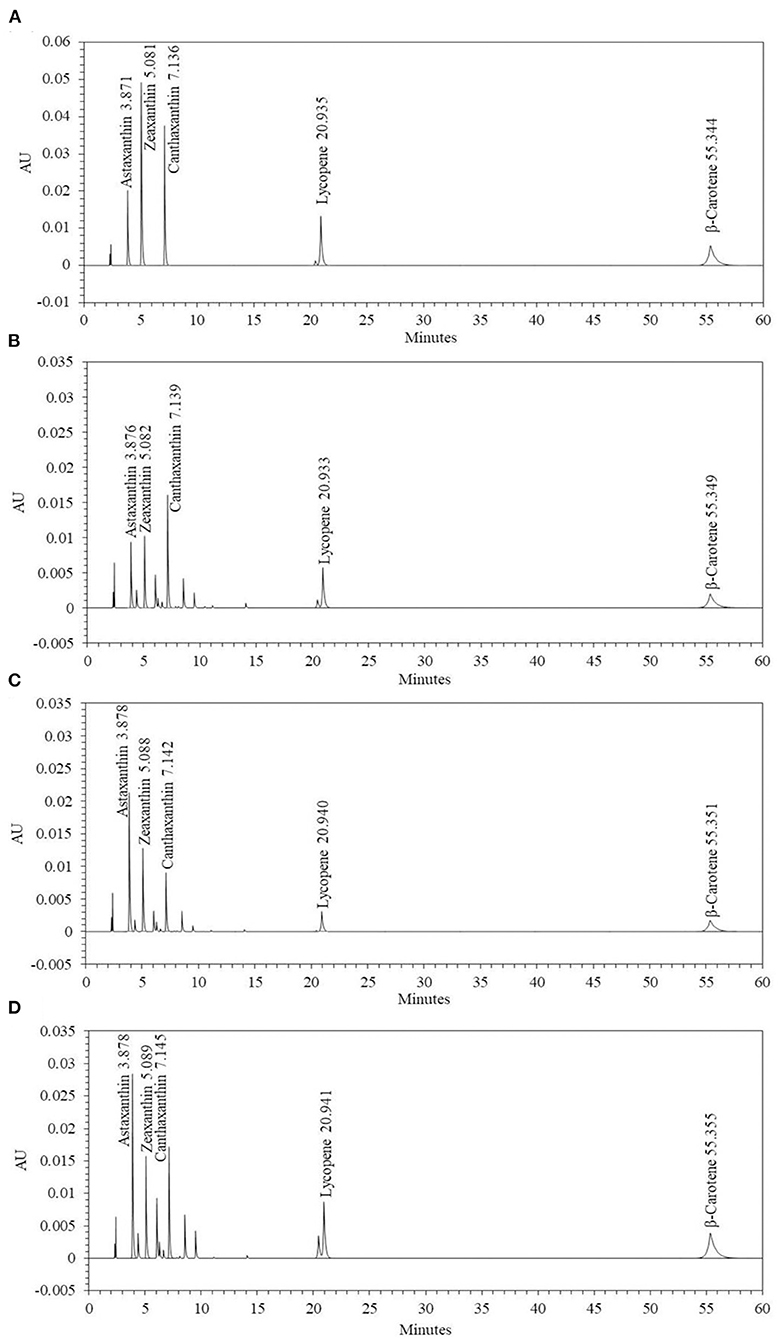
Figure 6. High performance liquid chromatography chromatograms of the carotenoids extracted from Paracoccus haeundaensis and the co-culture with Lactobacillus fermentum. (A) Standard carotenoids, (B) P. haeundaensis cultured in the LB medium, (C) P. haeundaensis cultured in the PMF medium, (D) co-culture of P. haeundaensis and L. fermentum in the PMF medium.
The amount of carotenoids under each condition are shown in Table 2. When the concentrations of the carotenoids in the LB and PMF media were compared, the astaxanthin concentration increased significantly in PMF, while β-carotene and lycopene decreased significantly. The zeaxanthin and canthaxanthin tended to increase and decrease, respectively, but there was no significant difference in the concentrations. These results were related to the optimization of the composition of the medium, and in particular, it is assumed that the activity of CrtZ increased due to the Fe2+ ions contained in the PMF medium. As the activity of CrtZ increased, the biosynthesis of astaxanthin and zeaxanthin increased, and β-carotene and lycopene were shown to decrease due to their consumption as substrates in the carotenoid synthesis pathway.

Table 2. Carotenoid concentrations according to culture medium and the co-culture of Paracoccus haeundaensis and Lactobacillus fermentum.
When P. haeundaensis and the co-culture carotenoid concentrations were compared in the PMF medium, the astaxanthin production increased 1.77 times, and the other carotenoids, except canthaxanthin, also increased significantly. It is assumed that the increase in the concentration of most carotenoids in the co-culture is related to the activity of enzymes involved in the carotenoid synthesis pathway.
RNA Levels of Carotenoid Biosynthesis Genes
In order to confirm the transcription level of the carotenoid biosynthesis genes of P. haeundaensis in the co-culture, cDNA was synthesized using reverse primers for each gene, and real-time qPCR was performed. The RNA levels of each gene were analyzed with Ct values and normalized to the rpoB gene transcript level (Figure 7). Among the six carotenoid biosynthesis genes, crtE, crtB, and crtW did not show a significant difference in relative RNA levels. The relative RNA level of the crtI gene was significantly increased by 3.2 times when co-cultured (0.879 ± 0.023) compared to that of P. haeundaensis alone (0.272 ± 0.010). In the co-culture, the relative RNA level of the crtY gene was 0.596 ± 0.029, which was 1.9 times higher than 0.312 ± 0.012 of P. haeundaensis. In addition, the crtZ gene was 8.748 ± 0.265 in the co-culture and increased by 1.9 times compared to 4.683 ± 0.172 in P. haeundaensis.
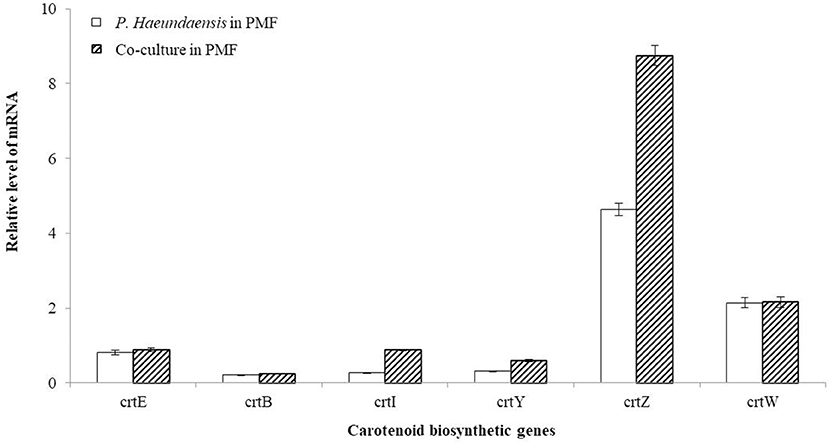
Figure 7. Analysis of the RNA level of the co-culture. The RNA levels of the carotenoid biosynthesis genes crtE, crtB, crtI, crtY, crtZ, crtW, and housekeeping gene rpoB determined by real-time qPCR analysis. The RNA level of the carotenoid biosynthesis genes was analyzed with the Ct value, and the Ct value of rpoB was used as a control. The RNA level indicates mean ± standard deviation.
Cell Growth and Astaxanthin Production in the 5 L Co-Culture System
The co-culture of P. haeundaensis and L. fermentum was performed in a 5 L jar fermenter. As a medium for the 5 L fermentation, the PMF medium was used, and P. haeundaensis and L. fermentum strains were simultaneously inoculated and incubated for 72 h. The concentration of cells and astaxanthin were measured at 6-h intervals. The growth rate of the cells and the production rate of astaxanthin remained constant and there was no difference between the laboratory scale production and the 5 L jar fermenter. Regarding the cell concentration rate in the co-culture, the stationary phase was reached in 42 h in both the laboratory scale and the 5 L jar fermenter. At the end of the experiment, the dried cell concentration of the 5 L jar fermenter was 1.81 ± 0.04 g/L, which was about 99.6% compared to 1.81 ± 0.07 g/L on the laboratory scale cultured in flask. In addition, the maximum astaxanthin production in the co-culture culture was reached in 54 h in both samples. The astaxanthin concentration of the 5 L jar fermenter sample at 72 h was 808.72 ± 15.58 μg/g-dried cell weight, which was about 99.3% compared to 814.20 ± 35.03 μg/g-dried cell weight on the laboratory scale (Figure 8).
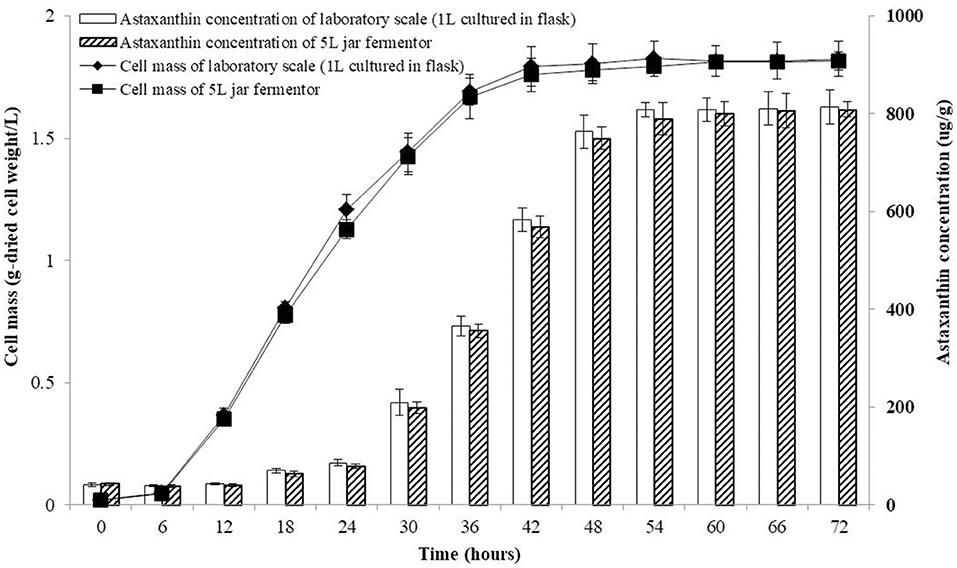
Figure 8. Growth and astaxanthin production curve of the co-culture of Paracoccus haeundaensis and Lactobacillus fermentum at the laboratory scale and in a 5 L jar fermenter.
Discussion
In this study, we confirmed the astaxanthin production according to the co-culture of P. haeundaensis and L. fermentum. First, in order to identify the optimal medium, various mediums were prepared to confirm astaxanthin production in co-culture. When the composition of the marine bacterial medium PPES-II medium was added with modified LB medium, the production of astaxanthin and cell mass were increased. The medium to be used for co-culture was optimized by adjusting the concentration of ferric citrate in the medium composition. In some studies, there were reported that Fe2+ ions enhance the production of astaxanthin (Zhou et al., 2017). In the composition of the PM medium, 0.1% ferric citrate is present, and it has been shown that ferric citrate increases the astaxanthin production of the PPES-II medium and PM medium. To optimize the production of the co-culture cells and astaxanthin, the co-culture was performed in PM with different ferric citrate concentrations. The production of the cells did not significantly differ among the different concentrations of ferric citrate. As the concentration of ferric citrate in the PM medium changed, the astaxanthin concentration highly increased at 0.01%. As a result of this experiment, we determined that the optimal medium for producing astaxanthin in the co-culture was a PMF medium which is PM medium containing 0.01% ferric citrate, and Fe2+ ions contained in the medium were shown to increase the production of astaxanthin. Second, as a result of confirming the astaxanthin production by the inoculation amount, it was confirmed that the initial inoculation amount affects astaxanthin production. When the inoculation amount of L. fermentum is higher than the optimal inoculation amount, it seems to inhibit the growth of P. haeundaensis. In addition, L. fermentum grows slowly at 25°C. For this reason, both strains cannot grow during co-culture, and as a result, the production of cell mass seems to decrease. These results indicate that the optimization of the medium and the inoculation amount are important for the production of and astaxanthin.
Compared with the single culture of P. haeundaensis, the cell mass was increased in co-culture. It was thought that the growth of L. fermentum strain was inhibited and the cell mass of P. haeundaensis increased at a culture temperature of 25°C. However, as a result of single culture of L. fermentum at 25°C, an increase in cell mass was confirmed, although the growth was slow. Therefore, it is assumed that the increase in cell mass in co-culture was due to the growth of the L. fermentum strain, not the growth of the P. haeundaensis strain.
An increase in the transcription level of the crtI gene plays an important role in carotenoid biosynthesis, as CrtI converts phytoene to lycopene by removing eight hydrogen atoms and inducing four double bonds. In plants, this step is involved in several enzymes, such as phytoene desaturase, ζ-carotene desaturase, ζ-carotene cis-trans isomerase, and carotene cis-trans isomerase, but only one enzyme is involved in CrtI in bacteria. This step is the main regulatory step of the carotenoid biosynthesis pathway, and thus, CrtI plays a key enzyme role in the pathway (Schaub et al., 2012; Choi et al., 2018). Therefore, the increased transcription of the crtI gene from P. haeundaensis in the co-culture increases not only lycopene synthesis but also most of the carotenoids synthesized after this step.
The crtZ is a gene that converts β-carotene into zeaxanthin and synthesizes astaxanthin together with the crtW gene, and thus plays an important role in the synthesis of astaxanthin. When P. haeundaensis and L. fermentum were co-cultured, the RNA level of crtW did not significantly change, but crtZ increased approximately 1.9 times compared to that in the P. haeundaensis single culture. This result is closely related to the slight decrease in canthaxanthin and the increased content of zeaxanthin and astaxanthin in the co-culture. Increasing the RNA levels of the crtI and crtY genes enhances the induction of β-carotene biosynthesis, which increases the concentration of β-carotene, and the high concentration of the β-carotene, a substrate for CrtZ and CrtW enzymes, would enhance the synthesis of zeaxanthin and canthaxanthin with the CrtZ and CrtW enzymes, respectively. However, the increase in the expression of CrtZ induces more conversion of β-carotene to zeaxanthin, and it is presumed that the production of canthaxanthin from β-carotene by CrtW is relatively small. In addition, it is presumed that a slight decrease in the concentration of canthaxanthin in the co-culture is competitively limited to the use of CrtW β-carotene by the increase of the CrtZ enzyme, and is caused by the competitive inhibitor of increased zeaxanthin, another substrate of CrtW.
In several studies, L. fermentum has been reported to produce useful substances such as organic acids and vitamins (LeBlanc et al., 2011; Khalil et al., 2018), which are thought to increase the RNA level of astaxanthin biosynthetic enzyme from P. haeundaensis. However, it was not known exactly how the substance or interaction stimulates P. haeundaensis to promote the production of astaxanthin. The co-culture conditions of the 5 L jar fermenter were established to conditions for astaxanthin concentration on a larger scale. However, compared to the laboratory-scale flask culture, astaxanthin production has not improved at the fermenter scale, despite better control of process parameters such as temperature, pH, DO, etc. in 5 L jar fermenter. It appears that further research is needed to search for substances produced by L. fermentum that stimulate astaxanthin production from P. haeundaensis, and to find the optimal conditions for increasing astaxanthin production in a 5 L jar fermenter.
In this study, the co-culture of P. haeundaensis and L. fermentum increased astaxanthin concentration. This indicates that L. fermentum stimulates the astaxanthin production in the co-culture. These studies may be useful as reference for co-culture and astaxanthin production from carotenoid biosynthesis microorganisms.
Data Availability Statement
The raw data supporting the conclusions of this article will be made available by the authors, without undue reservation.
Author Contributions
SC conducted an overall experiment and wrote this paper. YS provided ideas for the initial experiment, and gave interpretation and advice on the experimental results. S-WN gave advice on conducting the experiment. G-DK was the corresponding author. All authors contributed to the article and approved the submitted version.
Funding
This work was supported by a National Research Foundation of Korea (NRF) grant funded by the Korean government (MSIT) (No. NRF-2020R1F1A1076700).
Conflict of Interest
YS was employed by the company CBS Bioscience Co., Ltd.
The remaining authors declare that the research was conducted in the absence of any commercial or financial relationships that could be construed as a potential conflict of interest.
References
An, G. H., Bielich, J., Auerbach, R., and Johnson, E. A. (1991). Isolation and characterization of carotenoid hyperproducing mutants of yeast by flow cytometry and cell sorting. Bio/Technology 9, 70–73. doi: 10.1038/nbt0191-70
Bohutskyia, P., Kuceka, L. A., Hill, E., Pinchuk, G. E., Mundree, S. G., and Beliaev, A. S. (2018). Conversion of stranded waste-stream carbon and nutrients into value-added products via metabolically coupled binary heterotroph-photoautotroph system. Bioresour. Technol. 260, 68–75. doi: 10.1016/j.biortech.2018.02.080
Bubrick, P. (1991). Production of astaxanthin from Haematococcus. Bioresour. Technol. 38, 237–239. doi: 10.1016/0960-8524(91)90161-C
Buzzini, P. (2001). Batch and fed-batch carotenoid production by Rhodotorula glutinis-Debaryomyces castellii co-cultures in corn syrup. J. Appl. Microbiol. 90, 843–847. doi: 10.1046/j.1365-2672.2001.01319.x
Castelblanco-Matiz, L. M., Barbachano-Torres, A., Ponce-Noyola, T., Ramos-Valdivia, A. C., Cerda García-Rojas, C. M., Flores-Ortiz, C. M., et al. (2015). Carotenoid production and gene expression in an astaxanthin-overproducing Xanthophyllomyces dendrorhous mutant strain. Arch. Microbiol. 197, 1129–1139. doi: 10.1007/s00203-015-1153-9
Choi, S. S., Seo, Y. B., Lim, H. K., Nam, S. W., and Kim, G. D. (2018). Molecular cloning and overexpression of phytoene desaturase (CrtI) from Paracoccus haeundaensis. Microbiol. Biotechnol. Lett. 46, 145–153. doi: 10.4014/mbl.1802.02013
Dahllöf, I., Baillie, H., and Kjelleberg, S. (2000). rpoB-Based microbial community analysis avoids limitations inherent in 16S rRNA gene intraspecies heterogeneity. Appl. Environ. Microbiol. 66, 3376–3380. doi: 10.1128/AEM.66.8.3376-3380.2000
Domonkos, I., Kis, M., Gombos, Z., and Ughy, B. (2013). Carotenoids, versatile components of oxygenic photosynthesis. Prog Lipid Res. 52, 539–561. doi: 10.1016/j.plipres.2013.07.001
Emmerstorfer-Augustin, A., Moser, S., and Pichler, H. (2016). Screening for improved isoprenoid biosynthesis in microorganisms. J. Biotechnol. 235, 112–120. doi: 10.1016/j.jbiotec.2016.03.051
Fassett, R. G., and Coombes, J. S. (2009). Astaxanthin, oxidative stress, inflammation and cardiovascular disease. Future Cardiol. 5, 333–342. doi: 10.2217/fca.09.19
Fassett, R. G., and Coombes, J. S. (2011). Astaxanthin: a potential therapeutic agent in cardiovascular disease. Mar. Drugs. 9, 447–465. doi: 10.3390/md9030447
Fraser, P. D., Miura, Y., and Misawa, N. (1997). In vitro characterization of astaxanthin biosynthetic enzymes. J. Biol. Chem. 272, 6128–6135 doi: 10.1074/jbc.272.10.6128
Ide, T., Hoya, M., Tanaka, T., and Harayama, S. (2012). Enhanced production of astaxanthin in Paracoccus sp. strain N-81106 by using random mutagenesis and genetic engineering. Biochem. Eng. J. 65, 37–43. doi: 10.1016/j.bej.2012.03.015
Jhang, C., Chen, X., and Too, H. P. (2020). Microbial astaxanthin biosynthesis: recent achievements, challenges, and commercialization outlook. Appl. Microbiol. Biotechnol. 67, 571–578. doi: 10.1007/s00253-020-10648-2
Jones, J. A., and Wang, X. (2018). Use of bacterial co-cultures for the efficient production of chemicals. Curr. Opin. Biotechnol. 53, 33–38. doi: 10.1016/j.copbio.2017.11.012
Khalil, E. S., Abd Manap, M. Y., Mustafa, S., Alhelli, A. M., and Shokryazdan, P. (2018). Probiotic properties of exopolysaccharide-producing Lactobacillus strains isolated from tempoyak. Molecules 23:E398. doi: 10.3390/molecules23020398
Kobayashi, M., Kakizono, T., and Nagai, S. (1993). Enhanced carotenoid biosynthesis by oxidative stress in acetate-induced cyst cells of a green unicellular alga, Haematococcus pluvialis. Appl. Environ. Microbiol. 59, 867–873. doi: 10.1128/AEM.59.3.867-873.1993
LeBlanc, J. G., Laiño, J. E., del Valle, M. J., Vannini, V., van Sinderen, D., Taranto, M. P., et al. (2011). B-group vitamin production by lactic acid bacteria - current knowledge and potential applications. J. Appl. Microbiol. 111, 1297–1309. doi: 10.1111/j.1365-2672.2011.05157.x
Lee, J. H., Kim, Y. S., Choi, T. J., Lee, W. J., and Kim, Y. T. (2004). Paracoccus haeundaensis sp. nov., a Gram-negative, halophilic, astaxanthin-producing bacterium. Int J Syst Evol Microbiol. 54, 1699–1702 doi: 10.1099/ijs.0.63146-0
Li, C., Li, B., Zhang, N., Wei, N., Wang, Q., Wang, W., et al. (2019). Salt stress increases carotenoid production of Sporidiobolus pararoseus NGR via torulene biosynthetic pathway. J. Gen. Appl. Microbiol. 65, 111–120. doi: 10.2323/jgam.2018.07.001
Miller, M. W., Yoneyama, M., and Soneda, M. (1976). Phaffia, a new yeast genus in the Deuteromycotina (Blastomycetes). Int. J. Syst. Bacteriol. 26, 286–291. doi: 10.1099/00207713-26-2-286
Rodrigues, D. F., and Tiedje, J. M. (2007). Multi-locus real-time PCR for quantitation of bacteria in the environment reveals Exiguobacterium to be prevalent in permafrost. FEMS Microbiol Ecol. 59, 489–499. doi: 10.1111/j.1574-6941.2006.00233.x
Schaub, P., Yu, Q., Gemmecker, S., Poussin-Courmontagne, P., Mailliot, J., McEwen, A. G., et al. (2012). On the structure and function of the phytoene desaturase CRTI from Pantoea ananatis, a membrane-peripheral and FAD-dependent oxidase/isomerase. PLoS ONE. 7:e39550. doi: 10.1371/journal.pone.0039550
Seo, Y. B., Jeong, T. H., Choi, S. S., Lim, H. K., and Kim, G. D. (2017). Enhanced production of astaxanthin in Paracoccus haeundaensis strain by physical and chemical mutagenesis. J. Life Sci. 3, 339–345. doi: 10.5352/JLS.2017.27.3.339
Simova, E. D., Frengova, G., and Beshkova, D. M. (2003). Effect of aeration on the production of carotenoid pigments by Rhodotorula rubra-Lactobacillus casei subsp. casei co-cultures in whey ultrafiltrate. Z. Naturforsch C. J. Biosci. 58, 225–229. doi: 10.1515/znc-2003-3-415
Simova, E. D., Frengova, G. I., and Beshkova, D. M. (2004). Synthesis of carotenoids by Rhodotorula rubra GED8 co-cultured with yogurt starter cultures in whey ultrafiltrate. J. Ind. Microbiol. Biotechnol. 31, 115–121 doi: 10.1007/s10295-004-0122-0
Watanabe, K., Arafiles, K. H. V., Higashi, R., Okamura, Y., Tajima, T., Matsumura, Y., et al. (2018). Isolation of high carotenoid-producing Aurantiochytrium sp. mutants and improvement of astaxanthin productivity using metabolic information. J. Oleo Sci. 675, 571–578. doi: 10.5650/jos.ess17230
Yabuzaki, J. (2017). Carotenoids Database: structures, chemical fingerprints and distribution among organisms. Database (Oxford). 2017. doi: 10.1093/database/bax004
Zhang, Z., Pang, Z., Xu, S., Wei, T., Song, L., Wang, G., et al. (2019). Improved carotenoid productivity and COD removal efficiency by co-culture of Rhodotorula glutinis and Chlorella vulgaris using starch wastewaters as raw material. Appl. Biochem. Biotechnol. 189, 193–205. doi: 10.1007/s12010-019-03016-y
Zhou, P., Xie, W., Li, A., Wang, F., Yao, Z., Bian, Q., et al. (2017). Alleviation of metabolic bottleneck by combinatorial engineering enhanced astaxanthin synthesis in Saccharomyces cerevisiae. Enzyme Microb. Technol. 100, 28–36. doi: 10.1016/j.enzmictec.2017.02.006
Keywords: astaxanthin, co-culture, ferric citrate, Lactobacillus fermentum, Paracoccus haeundaensis
Citation: Choi SS, Seo YB, Nam S-W and Kim G-D (2021) Enhanced Production of Astaxanthin by Co-culture of Paracoccus haeundaensis and Lactic Acid Bacteria. Front. Mar. Sci. 7:597553. doi: 10.3389/fmars.2020.597553
Received: 21 August 2020; Accepted: 22 December 2020;
Published: 02 February 2021.
Edited by:
Ana Rotter, National Institute of Biology (NIB), SloveniaReviewed by:
Antje Labes, Fachhochschule Flensburg, GermanyHaoran Zhang, Rutgers, The State University of New Jersey, United States
Copyright © 2021 Choi, Seo, Nam and Kim. This is an open-access article distributed under the terms of the Creative Commons Attribution License (CC BY). The use, distribution or reproduction in other forums is permitted, provided the original author(s) and the copyright owner(s) are credited and that the original publication in this journal is cited, in accordance with accepted academic practice. No use, distribution or reproduction is permitted which does not comply with these terms.
*Correspondence: Gun-Do Kim, gundokim@pknu.ac.kr