A Roadmap for the Restoration of Mediterranean Macroalgal Forests
- 1Institut d’Ecologia Aquática, University of Girona, Girona, Spain
- 2Centre d’Estudis Avançats de Blanes, Girona, Spain
- 3Department of Biology and Evolution of Marine Organisms, Zoological Station Anton Dohrn, Naples, Italy
- 4Department of Biological and Environmental Sciences and Technologies, University of Salento, Lecce, Italy
- 5Department of Evolutionary Biology, Ecology and Environmental Sciences, Institut de Recerca de la Biodiversitat (IRBIO), University of Barcelona, Barcelona, Spain
- 6Instituto de Ciencias del Mar, Consejo Superior de Investigaciones Científicas (CSIC), Madrid, Spain
- 7Marche Polytechnic University, Ancona, Italy
- 8Stazione Zoologica Anton Dohrn Napoli, Naples, Italy
- 9Consorzio Nazionale Interuniversitario per le Scienze del Mare (CoNISMa), Rome, Italy
Canopy-forming macroalgae play a crucial role in coastal primary production and nutrient cycling, providing food, shelter, nurseries, and habitat for many vertebrate and invertebrate species. However, macroalgal forests are in decline in various places and natural recovery is almost impossible when populations become locally extinct. Hence, active restoration emerges as the most promising strategy to rebuild disappeared forests. In this regard, significant efforts have been made by several EU institutions to research new restoration tools for shallow and mesophotic reef habitats (e.g., MERCES EU project, AFRIMED, and ROCPOP-life) and effective techniques have subsequently been proposed to promote self-sustaining populations. Recent research indicates that macroalgal forest recovery requires a broad spectrum of measures, ranging from mitigating human impacts to restoring the most degraded populations and habitats, and that the viability of large restoration actions is compromised by ongoing human pressures (e.g., pollution, overgrazing, and climate change). We propose a roadmap for Mediterranean macroalgal restoration to assist researchers and stakeholders in decision-making, considering the most effective methods in terms of cost and cost-effectiveness, and taking background environmental conditions and potential threats into account. Last, the challenges currently faced by the restoration of rocky coastal ecosystems under changing climate conditions are also discussed.
Introduction
Macroalgal forests of canopy-forming fucoids are dominant on rocky reefs along all the Mediterranean coasts (Feldmann, 1937; Giaccone and Bruni, 1973; Verlaque, 1987; Ballesteros, 1992; Assis et al., 2020). They are recognized as hot spots of diversity and CO2 sink, providing food and shelter for diverse assemblages of understory species and enhancing coastal primary productivity (Molinier, 1960; Boudouresque, 1972; Ballesteros, 1992; Ballesteros et al., 2009; Sales et al., 2012; Cheminée et al., 2013; Thiriet et al., 2016). Macroalgal forests formed by fucalean brown algae (i.e., Cystoseira sensu latu, including the genera Cystoseira, Ericaria and Gongolaria; Molinari-Novoa and Guiry, 2020) can potentially thrive from the intertidal to the circalittoral, with different species replacing one another along the bathymetric gradient. Community structure (i.e., diversity and species richness) generally increases in complexity with depth, while population and community dynamics (i.e., productivity, turnover, and growth rates) slow down (e.g., Ballesteros, 1989, 1990; Ballesteros et al., 1998; Capdevila et al., 2015, 2016).
As a response to multiple stressors, including eutrophication, overgrazing, increasing coastal sediment loads, and impacts of urbanization, these habitats in both shallow and deep waters are being lost at alarming rates (Gros, 1978; Munda, 1982, 1993; Sala et al., 1998, 2011; Soltan et al., 2001; Thibaut et al., 2005; Vergés et al., 2014), and previously widespread canopy-forming algae have become extinct or have been reduced to remnant, fragmented, and isolated populations (e.g., Thibaut et al., 2005, 2016; Blanfuné et al., 2016; Mariani et al., 2019). Observational studies and manipulative experiments have demonstrated that these systems may switch toward degraded states (i.e., sea urchin barrens or algal turfs) if the canopy is removed or damaged (Cormaci and Furnari, 1999; Munda, 1993; Thibaut et al., 2005; Ling et al., 2015 and references therein). Very little evidence of natural recovery has been reported in macroalgal forests (Scheffer et al., 2001; Perkol-Finkel and Airoldi, 2010; Iveša et al., 2016), even when the area switches back to conditions before Cystoseira forest decline (Pinedo et al., 2013). Relict populations will ultimately disappear if effective restoration strategies are not attempted together with stressor identification, mitigation, or elimination, which should be an integral part of any restoration plan (Orth et al., 2006; seagrass).
Under these circumstances, and when the environmental conditions of the area to restore have been recovered to the levels prior to the regression or disappearance of the forest, human-induced recovery can be of critical importance. Coastal ecosystem restoration is still in the “innovation phase” (Waltham et al., 2020) and, despite increasing efforts toward macroalgal restoration (Gianni et al., 2013; Eger et al., 2020b, 2021), this ecosystem has received scarce attention compared to other coastal habitats (e.g., sea grasses and saltmarsh) (Goodsell and Chapman, 2009; Wahl et al., 2015). The criteria, targets, and methods applied until now have therefore been highly heterogeneous, making results scarcely comparable. Different transplantation methodologies have been implemented as part of various macroalgal restoration projects, aimed at introducing new individuals to the sea floor and/or a source of fertile material to obtain new individuals (Gianni et al., 2013 and references therein; Eger et al., 2021). In the Mediterranean Sea, the transplantation of individuals of Cystoseira was the first proposed methodology (Falace et al., 2006; Susini et al., 2007; Perkol-Finkel et al., 2012; Robvieux, 2013). However, since most Cystoseira s.l. species are considered threatened or endangered by the Barcelona Convention (Appendix II; UNEP/MAP, 2013), any technique that requires the harvesting of individuals from remaining populations is undesirable, leading to the exploration of less invasive restoration actions (see Gianni et al., 2013 for a review).
The framework is presented as several sections that support the process of planning a restoration effort. The objective priority is to provide researchers and managers with information that is important to consider when restoring macroalgae in the Mediterranean Sea. The planning process is broken down into five steps: (1) selection of the restoration sites; (2) selection of the target species; (3) selection of donor sites and specimens; (4) selection of restoration techniques; and (5) complementary actions (Figure 1). Last, we describe the cost/feasibility of broad-scale applications of the restoration techniques as a key element in planning activities. By developing more standardized approaches for macroalgal restoration, synergy can be achieved through cooperation among different stakeholders and NGOs and cost-effective measures.
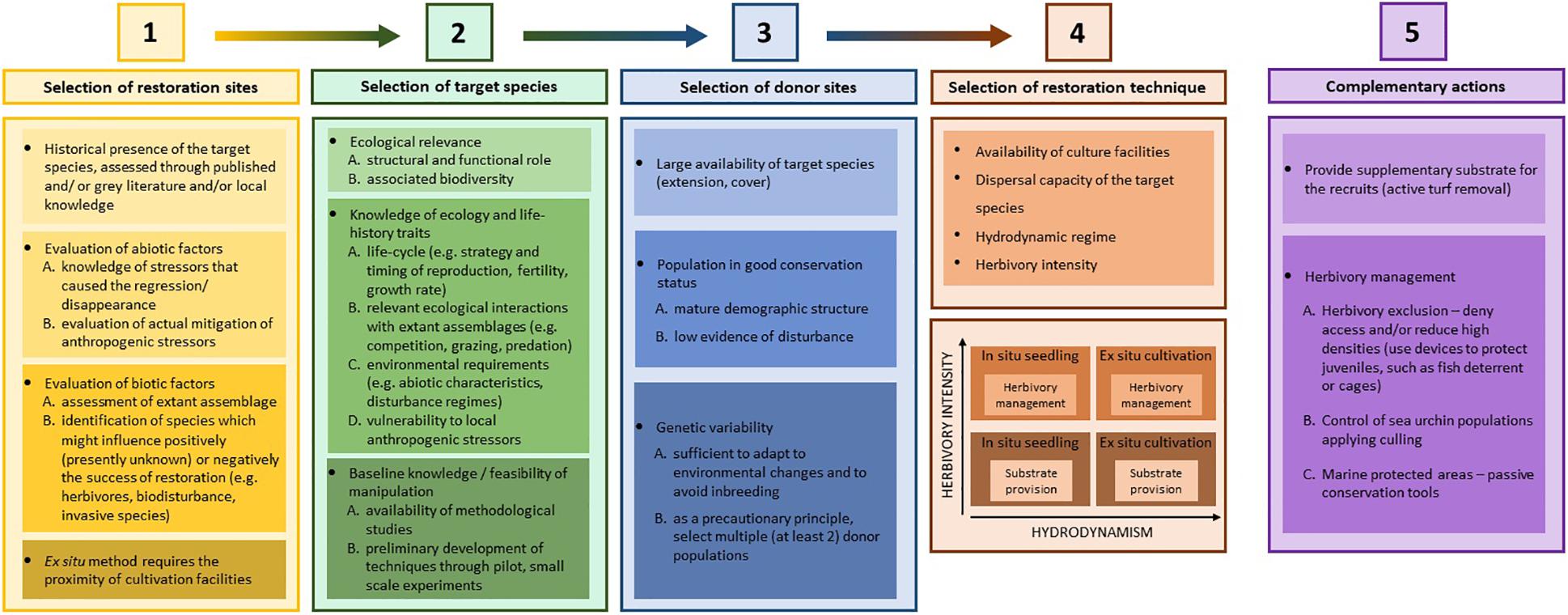
Figure 1. Conceptual model of the crucial steps to guide the restoration of macroalgae forests. The knowledge and main factors and processes that must be considered for an optimal selection of restoration sites, target species, donor sites, restoration techniques, and the use of complementary actions are detailed and explained below.
Selection of Restoration Sites
Several conceptual models to optimize site selection have been developed for seagrass communities (e.g., Calumpong and Fonseca, 2001; Campbell, 2002; Short et al., 2002), proposing that historic and literature-based information, reference data, and in situ environmental data are essential to identify and prioritize restoration sites. For macroalgae, site selection should similarly adopt a priori criteria with additional insights into habitat requirements and characteristics of eligible habitats on a local scale. In particular, the historical presence of the target species should be documented and considered a pre-requisite for identifying a site for restoration as eligible. Although this may seem obvious, the availability of scientific records to do so is generally scarce. Analyzing the gray literature or local ecological knowledge gathered from different approaches (e.g., photo and video reports, fishermen, expert opinions) may help to fill this knowledge gap.
Restoration planning also requires the identification of the factors that caused and maintained the loss of the target species in a putative restoration site. Restoration actions in locations where the stressors that have led to forest decline have not been mitigated or abolished are likely to be unsuccessful. If environmental conditions are not appropriate to ensure post-settlement survival, restoration is not viable, even if the propagule supply is increased. However, mitigation is only manageable for local stressors and cannot be achieved for global stressors. In this case, more resilient populations should be identified and used (see the future trends section). Nonetheless, the management of local stressors (i.e., their reduction or removal) may enhance the resilience of restored macroalgae to the ongoing pressures from global stressors (Morris et al., 2020). While we may be stating the obvious, the causal relationships between stressors and species loss are still often unknown across habitats and locations (Hillebrand et al., 2020). Therefore, the effects of anthropogenic stressors acting separately or in combination and their impact on different life-stages of target species must urgently be identified.
Beyond examining abiotic environmental conditions, careful assessment of extant assemblages at the eligible restoration site is essential to identify species that could potentially limit the success of restoration, including fast growing species that may outcompete canopy-forming recruits, preventing settlement and post-settlement survival (Filbee-Dexter and Wernberg, 2018), or grazers (e.g., Tamburello et al., 2019). In general, the disappearance of habitat-forming species results in less complex and productive assemblages dominated by stress-tolerant taxa (Filbee-Dexter and Wernberg, 2018; Maggi et al., 2018). The establishment of new feedback mechanisms in degraded assemblages contributes to their self-sustainment and prevents the natural recovery of habitats (Filbee-Dexter and Wernberg, 2018). Even when habitat-forming species are reintroduced, ecological interactions with extant species may play a crucial role in determining the success of restoration action. It has recently been suggested that positive interactions of macroalgae with other species (at the same or different trophic level) might be just as frequent as the negative ones, thus the incorporation of facilitative effects into restoration practices is advocated to enhance their success (Eger et al., 2020a). Although specific applications of this theory are very scarce for macroalgae (Medrano et al., 2020), successful evidence has been presented for marine plants, wetlands, and mangroves (Eger et al., 2020a; Gagnon et al., 2020 and references therein).
Logistic considerations concerning accessibility and ease of manipulation of the target species are also important, although they should play a secondary role in the prioritization of restoration interventions. In the 2019 study conducted by Tamburello et al. (2019), abrupt variations in environmental conditions and mechanical damage during transportation or manipulation were possible factors that contributed to the mortality of germlings before and during settlement in the field, indicating that having nursery facilities close to restoration sites can significantly contribute to the success of the intervention. This is a critical matter considering the present and future need for restoration actions in areas of the world where facilities are still lacking, the impact of which could be minimized using the in situ technique (explained below).
Selection of Target Species
The criteria for the identification of target species should be based on their ecological relevance and status (Swan et al., 2016) and, if possible, their previous presence in the area. In fact, a successful restoration of habitat-forming species would provide structurally complex and highly productive habitats, hopefully supporting the recovery of the associated assemblages together with the ecosystem functions and services they provide.
In-depth knowledge of the ecology and life-history traits of target species is a key requisite for planning efficient restoration actions (Montero-Serra et al., 2018). This should include information on: (i) life cycle and life-history traits (e.g., strategy and timing of reproduction, fertility, growth rate, etc.); (ii) relevant ecological interactions with extant assemblages (e.g., competition, grazing, predation, susceptibility to pathogens, etc.); (iii) environmental requirements (i.e., physical-chemical characteristics of the substratum and water column, disturbance regimes, etc.); and (iv) vulnerability to local anthropogenic stressors (e.g., heavy metals, organic and inorganic pollutants, etc.). These requirements may vary at different life stages of the target species (de Caralt et al., 2020; Verdura et al., 2021). Since these could represent critical conditions or processes limiting the target species survival, strategies to mitigate specific stressors during the restoration actions can be implemented, thus optimizing restoration success. Unfortunately, complete information is lacking in most cases. Thus, it is important to fill the gaps and to identify what the most critical life-history traits, ecological interactions, environmental drivers, and anthropogenic stressors affecting the survival of the selected species are.
Last, if after all these considerations several species emerge as suitable candidates to be restored, species for which documented manipulation techniques are available should be selected first. If no information is available, pilot studies at a local scale are highly recommended to test implantation techniques.
Selection of Donor Sites and Specimens
The general criteria for the suitability of potential donor populations are based first on having well preserved conditions to support the extraction of individuals to foster new populations. Also of critical importance is being as close as possible to the area to restore to minimize manipulation of the specimens and to optimize action cost/effectiveness. However, the removal of large numbers of adult individuals is hardly sustainable for existing Cystoseira beds and, due to the scarce resilience of compromised canopies, would result in an irreversible disturbance of donor forests (Piazzi et al., 2017; Rindi et al., 2018). It has been suggested that the outplanting of Cystoseira germlings is more sustainable than destructive restoration methods and has no impact on donor populations since even a low number of scattered individuals thriving in a degraded area can be the source of hundreds or thousands of new recruits that can be used to re-enforce the donor population itself, or to re-introduce a population to a new area that displays better environmental conditions and is more likely to be successful (Falace et al., 2018; Verdura et al., 2018).
Donor populations should also display enough genetic variability to allow specimens selected for restoration actions to adapt to environmental changes and avoid inbreeding (Ingvarsson, 2001). While the importance of genetic variability of donor populations has been investigated for seagrasses, saltmarsh plants, and seaweeds (Procaccini and Piazzi, 2001; Oudot-Canaff et al., 2013; Evans et al., 2018; Wood et al., 2020), studies on Mediterranean macroalgal species are still lacking. As Cystoseira s.l. populations tend to be genetically differentiated at a small spatial scale (i.e., 10–100 m, Buonomo et al., 2017) due to their very limited dispersal capacity (generally < 10 m), it cannot be ruled out that donor populations can be locally adapted. Transplantation to other conditions can result in a reduced adaptive capacity of the restored population. Further investigations on this topic are advocated, preferably following the precautionary principle of selecting multiple donor populations.
Selection of Restoration Techniques
Several recruitment enhancement techniques (germlings outplanting) and complementary actions have proved successful to restore Cystoseira populations (e.g., Verdura et al., 2018; De La Fuente et al., 2019; Tamburello et al., 2019; Medrano et al., 2020; Figure 2). They consist in obtaining recruits from fertile branches of the donor populations, which are either placed directly in the area to be restored (in situ technique) or cultured on aquaria facilities and then transported to the field (ex situ technique). These techniques are especially suitable for Cystoseira s.l. species since they present a sexual reproduction from monoecious individuals, with male and female gametes housed within the same conceptacle, which are grouped in receptacles (Guern, 1962; Rodríguez-Prieto et al., 2013), thus ensuring male and female gametes for each fertile branch. Techniques can be selected according to different factors such as the availability of culture facilities, dispersal capacity of the target species, the hydrodynamic regime of the selected site to restore, and the grazing pressure. For the ex situ technique, production is hampered by the availability of facilities (e.g., the dimension and number of environmentally controlled rooms, the number of aquaria, labor; Savonitto et al., 2021) and the proximity of the restoration site, considering that transport to the field is a critical step for the success of the intervention (Tamburello et al., 2019). The in situ technique and the ex situ technique appear to be especially suitable for species with a higher and a lower dispersal capacity, respectively (Verdura et al., 2018; De La Fuente et al., 2019). Under low hydrodynamic conditions (e.g., at a depth below 10 m or inside lagoons) the in situ approach has proved to be the most effective (Verdura et al., 2018). Contrarily, the ex situ cultivation of zygotes should be prioritized (Verdura et al., 2018) in areas with high hydrodynamic conditions (e.g., on exposed intertidal and shallow subtidal rocky reefs), where zygotes can be swiped out to the water column. Other factors apart from hydrodynamism, such as herbivory, may lead to opting for the ex situ technique, thus avoiding the grazing of the recruits (Savonitto et al., 2021).
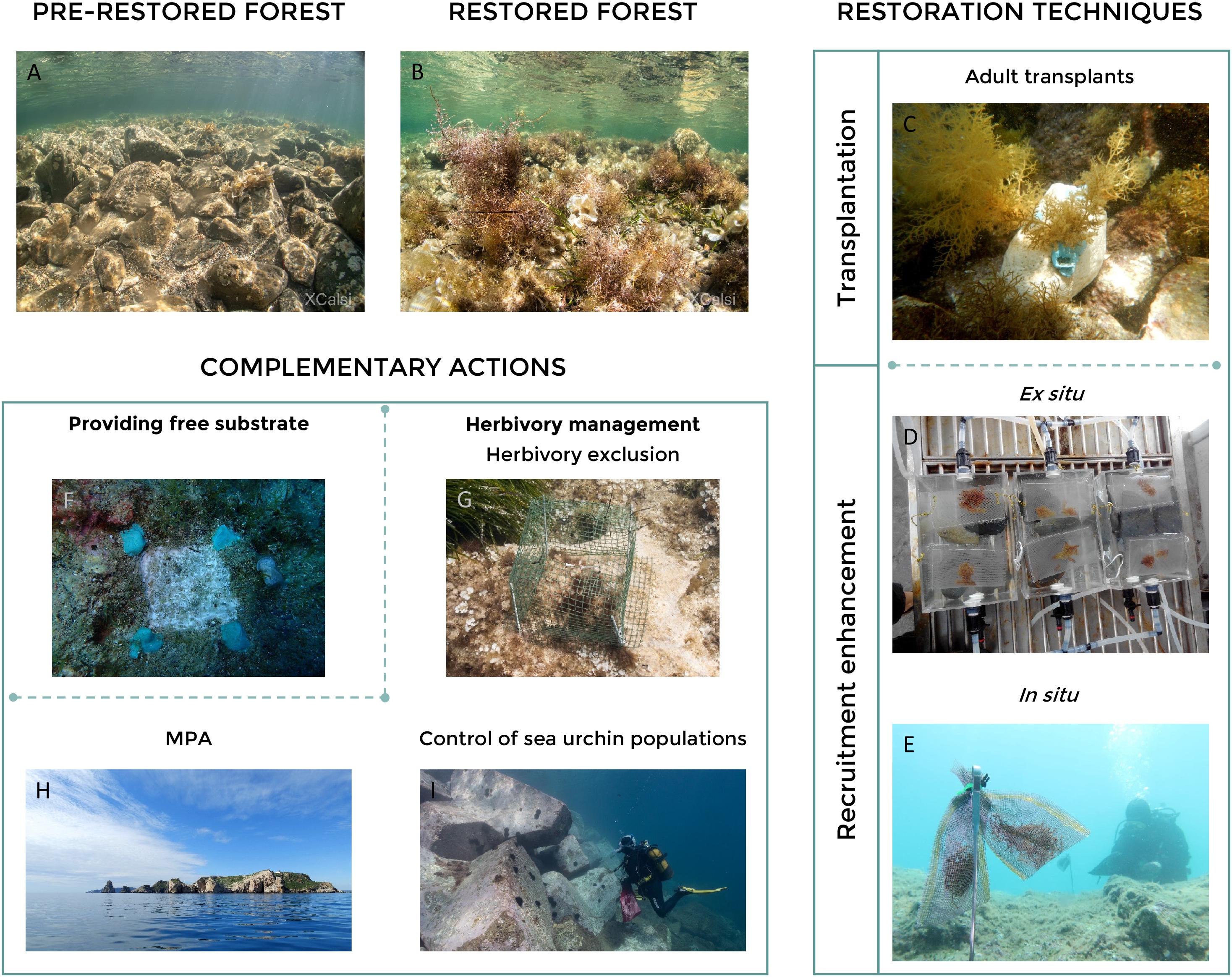
Figure 2. Image of the rocky reef before the restoration action (A) and after restoring the Cystoseira s.l. population (B). Restoration techniques: Cystoseira s.l. adult transplantation (C), ex situ approach (D) and in situ recruitment enhancement (E). Complementary actions: providing free substrate (F), herbivory exclusion (G), MPAs (H) and control of sea urchin populations (I). Photo credits: Xavi Calsina (@XCalsi; A,B,G), Margalida Monserrat (C), Jana Verdura (D), Simonetta Fraschetti (E), Alba Medrano (F), Josep Pascual (H) and Jordi Boada (I).
In situ Approach
The in situ approach has been successfully tested with Ericaria barbata and Gongolaria elegans (Verdura et al., 2018; Medrano et al., 2020). When the receptacles are mature, fertile apical branches are collected from donor populations using scissors and transported to the restoration site in plastic bags without any water and in cold and dark conditions. Approximately ten fertile receptacles should be placed in each dispersal bag (8 × 10 cm) made of 36% fiberglass and 64% PVC, with a mesh size of 1.20 × 1.28 mm. Dispersal bags are tied to a pick and fixed to the substratum using a hammer and/or epoxy putty at a vertical distance of 25 cm from the bottom. The distance between dispersal bags should be approximately 2–3 m (Verdura et al., 2018). Within this area, free substratum to promote the settlement of zygotes should be provided, especially if the area to restore is dominated by turf algae that can outcompete the new recruits. This can be done by placing flat stones deprived of any organisms in the area, or by pre-empting the rocky substrate with a metal brush. The dispersal bags can be removed after 4 days.
Ex situ Approach
The ex situ technique has been tested for Ericaria amentacea, E. crinita, E. zosteroides, and Gongolaria barbata. Ex situ seeding provides a large number of healthy individuals to be re-introduced in the environment without impacting the natural populations (Falace et al., 2006, 2018; Verdura et al., 2018). During harvesting, up to 3 fertile apexes should be collected from each individual to ensure a minimum degree of genetic variability and to avoid compromising the reproductive capability of exploited individuals. During transportation to the laboratory, fertile branches should be preserved in plastic bags without water and in cold and dark conditions. Transport should be completed within 48 h of collection. Once in the laboratory, fertile branches are stored in fridges (4°C) in dark conditions for 12–24 h to promote zygote liberation. Cultivation is carried out in aquaria with a close-water circuit of filtered natural seawater continuously aerated by air pumps. The photoperiod should be selected to reflect the seasonal conditions of the donor site. Temperature and irradiance conditions are set according to the specific requirements of the cultivated species (Verdura et al., 2015).
The substratum used for cultivation can be natural stones previously cleaned with a hard brush. Clay tiles can be used if stones are not available in the environment, after carefully considering their rugosity (Tamburello et al., 2019). Falace et al. (2018) used two different substrata (pebbles and tiles) to test settlement and recruitment in laboratory culture conditions, finding a significantly higher settlement of eggs in tiles than in pebbles, which presented more roughness, although successive germling growth and survival were not affected by the substrata. Fertile branches should be placed uniformly in mesh bags, ensuring they float on the surface of the tanks while releasing the gametes and the zygotes. Water movement inside the tanks should be kept as low and stable as possible for the first 4 days to facilitate zygote settlement. Adult branches can then be removed to eliminate possible sources of contamination such as epiphytes and particulate organic matter. The water of the aquaria must be partially renewed once a week. Epiphytes on the stones can eventually be eliminated by gently cleaning their surface with a paint brush. Cultured germlings should grow for at least 3 weeks (when they became visible with the naked eye) and up to 3 months, when early stage individuals reach 1–1.5 mm and can be transported to the field. The transport of germlings from the laboratory to the field should be carried out in dark and in situ temperature conditions. Once at the destination, the displacement or attachment of colonized stones with epoxy putty should take place rapidly (within a few hours) to ensure that the germlings do not undergo thermal stress.
Phenology Knowledge: An Essential Step
In all cases, a priori knowledge of the phenology and recruitment periods of the species to be restored is needed because the proposed techniques are based on obtaining fertile branches and the survival of recruits. Regarding obtaining fertile branches, donor populations characterized by dense Cystoseira s.l. cover should be identified and monitored monthly to detect the reproductive time of the year when receptacles of the target species become available. When Cystoseira s.l. fronds exhibit mature receptacles, fertile branches need to be collected for fertilization. Personal observations report that nearly 100 mature branches with receptacles (from 40 individuals) are required to generate about 400 adults, which has proved to be enough to restore approximately 50 square meters of a rocky shore.
Since the reproductive capability of a species depends on several environmental conditions, zygote availability can be extremely compromised. As demonstrated by Marion and Orth (2010), seagrass seed production in donor forests can vary dramatically from year to year, also due to climate change (Bevilacqua et al., 2019). Likewise, abnormal winter temperatures and exceptional wind periods (Bevilacqua et al., 2019; Orlando-Bonaca et al., 2021; Savonitto et al., 2021) lead to serious biological anomalies and the loss of the reproductive potential of Gongolaria barbata. Therefore, it is crucial to act as fast as possible during the reproductive season of the selected species to collect an appropriate number of mature apexes. Their availability represents an intrinsic limit of any restoration technique since interventions cannot be repeated until the following reproductive period of the target species. Although fertility and recruitment may vary geographically, here we provide a table with the approximate fertility periods of the most common species, using data gathered from personal observations and published sporadic and local studies (Supplementary Table 1). However, data scarcity on algal demography limits the generalization of these results, so more data and in-depth studies are needed to infer general life-history patterns and how they are affected by climate change (Capdevila et al., 2016; Verdura et al., 2021).
Complementary Actions
Restoration success can also be compromised by the ecological interactions between the canopy-forming algae and the multiple species thriving in the area to restore. Among them, herbivory represents one of the major threats to the survival of Cystoseira juveniles once reintroduced at restoration sites because it can drastically limit the survival of reintroduced individuals, with estimated losses of up to 90% in just a few days (Hereu, 2006; Gianni et al., 2013; Agnetta et al., 2015). Another major threat is competition with opportunistic species such as turf-forming algae (Airoldi, 2000; Filbee-Dexter and Wernberg, 2018). Therefore, in many areas there is a need to remove the extant algal assemblages potentially limiting restoration success (Verdura et al., 2018), and herbivores (Piazzi and Ceccherelli, 2019; Tamburello et al., 2019; Medrano et al., 2020) should complement the restoration action.
Providing Supplementary Substrate
On many coastal reefs, macroalgal forests face competition and overgrowth by turf, whose abundance may increase due to disturbance events such as chronic nutrient enrichment, sediment load and climate change (Filbee-Dexter and Wernberg, 2018 and references therein). Mediterranean turf algae are simple populations dominated by small fast-growing species (e.g., Cladostephus hirsutus, Dasycladus vermicularis, Dictyotales, Sphacelaria spp., Halopteris scoparia, Laurencia spp., Padina spp.; Thibaut et al., 2017 and references therein). Turf can potentially compromise restoration success by quickly overgrowing and monopolizing primary substrate, limiting the availability of the suitable hard substratum required for canopy forming macroalgae settlement (Airoldi, 1998, 2003; Gorgula and Connell, 2004; Connell and Russell, 2010), and because of its ability to accumulate sediment (e.g., Isaeus et al., 2004; Filbee-Dexter et al., 2016), reducing the survival rates of canopy-forming macroalgae recruits (Reed, 1990; Isaeus et al., 2004; Gorman and Connell, 2009). Likewise, it has been suggested that turf-forming algae affect early life-history stages of Mediterranean canopy algae (Gros, 1978; Benedetti-Cecchi and Cinelli, 1992; Ballesteros et al., 2009). Therefore, active turf removal should be carried out as a management tool to reduce this competitive effect and provide space for new macroalgal recruitment. This action represents a supplementary method to support recruitment enhancement (Capdevila et al., 2015; Medrano et al., 2020). Providing available substrate by itself does not translate into a restored macroalgal forest if no neighboring Cystoseira s. l. populations are available, but it does facilitate recruitment of new individuals when restored individuals become fertile. Scraping some plots to provide a newly available substrate for new recruits is preferably required in areas colonized by turf or other less complex macroalgal habitats (Verdura et al., 2018; Medrano et al., 2020), although it is probably not needed in barren areas. Cleaning periods are species-dependent and they should match recruitment periods from the first year of fertility (Supplementary Table 1). They should be maintained annually or biannually.
Herbivory Management
One of the main factors limiting the success of macroalgal restoration is the presence of herbivores at high densities (mainly the sea urchins Paracentrotus lividus and Arbacia lixula and, especially in the Eastern Mediterranean, the fish Sarpa salpa and Siganus spp.). A preliminary assessment of the density of herbivores and grazing pressure at restoration sites should be carried out and, where possible, intensively grazed sites should be discarded. Alternatively, devices to prevent access by grazers (fish and sea urchins) and herbivore removal/culling should be considered. Notably, knowledge on the critical threshold density of herbivores affecting recruitment success in macroalgae is still to be ascertained and will vary depending on environmental conditions, including nutrient regimes (Boada et al., 2017).
Herbivory Exclusion
Devices to prevent access by grazers can be used to protect the juveniles at least for the initial months of growth after settlement or transplantation from the aquaria. For instance, fish deterrent devices have shown to successfully limit the access of salema fish (Sarpa salpa) in intertidal forests of Ericaria amentacea (Gianni et al., 2020), while cages fixed to the substratum can protect Cystoseira s.l. juveniles (Tamburello et al., 2019). Cage structures made with double metal mesh and wire can be screwed to the substratum using an underwater drill and sealed with epoxy putty. If cages are provided with a top side, they can eventually also protect from fish grazing. Cages should be periodically cleaned with a metal brush to prevent shading due to epiphytes. Cage size will depend on the hydrodynamic conditions, usually measuring 20 × 20 cm in shallow, high hydrodynamic areas and 50 × 50 cm in deeper areas or where hydrodynamism is negligible. However, setting up exclusion devices requires intense maintenance since epiphytism may strongly modify irradiance and water exchange conditions, and hydrodynamism can deteriorate fixed structures.
Control of Sea Urchin Population Density
Recent studies have shown a positive effect of sea urchin removal (harvesting and culling) on the recovery of overexploited macroalgal beds in subtidal rocky habitats (Piazzi and Ceccherelli, 2019). To date, the practice of extensive sea urchin culling has been applied in some areas around the world, principally for kelp restoration (Tracey et al., 2014), and for the first time in the Mediterranean Sea within the MERCES1 project (Guarnieri et al., 2020; Medrano et al., 2020). Culling actions can be opportunely carried out either to complement recruitment enhancement or to protect adult Cystoseira s.l. individuals seriously threatened by overgrazing. In this case, sea urchin culling can be complemented by providing free substrate for recruitment when simple turf algae dominate the seascape. Recent studies (Guarnieri et al., 2020; Medrano et al., 2020) have confirmed that where top-down regulatory processes have been seriously compromised, a single severe large-scale action might affect grazer density over mid-long periods due to low recolonization of sea urchins driven by a complex suite of ecological processes, ruling out the need for maintenance over time. However, in other areas, more frequent interventions have been needed to obtain long-lasting results (Watanuki et al., 2010; Wood et al., 2019).
In the Mediterranean Sea it is widely accepted that P. lividus harvesting may be a potential effective method to mitigate overgrazing in areas of severe overfishing (Guidetti et al., 2004; Piazzi and Ceccherelli, 2019; Farina et al., 2020). Direct extraction of P. lividus can result in community level effects triggering Cystoseira s.l. recruitment and recovery (Piazzi and Ceccherelli, 2019). Hence, an integrated management of P. lividus harvesting could be considered a useful complementary tool in areas where the density of sea urchins may compromise the viability of restoration actions. However, it should be mentioned that sea urchin fisheries are only focused on P. lividus, so indirect facilitation effects of its counterpart A. lixula on algal beds cannot be disregarded (Bulleri et al., 1999).
Synergistic Interaction Between Restoration and Marine Protected Areas
The effectiveness of Marine Protected Areas (MPAs) and their direct and indirect effects on populations is extremely variable depending on multiple factors such as the level of protection, size, history, and ecological traits of the protected species and human activities inside and outside the MPA (Claudet et al., 2011), among others. Furthermore, the potential effects of protection on the effectiveness of macroalgae restoration is still controversial, according to the available data. In this line, while examples of macroalgae restoration can be found in both MPAs and non-protected areas (e.g., Verdura et al., 2018; Medrano et al., 2020), studies commonly point to herbivory as the major threat to restoration processes (Carney et al., 2005; Campbell et al., 2014; Tamburello et al., 2019; Savonitto et al., 2021). Some MPAs have worked to restore kelp populations, especially where cascading effects have facilitated grazer control and where no other stressors are present (e.g., increases in the populations of urchin predators such as sea otters, fish, or lobsters) (e.g., Shears and Babcock, 2003; Watson and Estes, 2011; Caselle et al., 2018; Eger and Baum, 2020). However, to successfully revegetate barren grounds, a synergy between passive (MPAs) and active restoration strategies (intentional activity to help macroalgae recovery) might be key, indicating that restoration actions should be encouraged in already protected areas to enhance and speed up the recovery of degraded macroalgal forest (Gianni et al., 2013; Filbee-Dexter and Scheibling, 2014; Medrano et al., 2019).
Cost and Cost-Effectiveness
Ecosystem restoration is cost and labor intensive, with median costs in marine ecosystems (Bayraktarov et al., 2016) reaching hundreds of thousands of US dollars. Examples of restoration projects reporting cost estimates are limited (but see Carney et al., 2005; Campbell et al., 2014; Tracey et al., 2014; Fredriksen et al., 2020; Layton et al., 2020), with cost-benefit analyses even more lacking (however, see Bayraktarov et al., 2016 for a review of factors affecting cost-effectiveness). Uncertainties about restoration costs and feasibility can impede decisions on whether, what, how, where, and how much to restore (Bayraktarov et al., 2016). For macroalgae, a recent review reported global average cost numbers of restoration activities, finding high variation between methodologies and attempts (Eger et al., 2021). For example, among the reviewed methods, sea urchin control was found to be the one that requires the least investment (quickliming and manual removal, approximately 1,300$/ha and 43,800$/ha, respectively). This method is followed by seeding with propagules (approximately 441,300$/ha), the direct transplant of individuals (approximately 582,100$/ha), and the creation of artificial reefs (approximately 593,400$/ha). Given the few examples of Cystoseira s.l. restoration to date and the considerable variability in costs (Table 1), it is currently very difficult to accurately estimate the costs and cost-benefit of macroalgae Mediterranean forest restoration. Cost will ultimately depend on the species, its ecology, the specific characteristics of the habitat to be restored, and the availability of infrastructures.
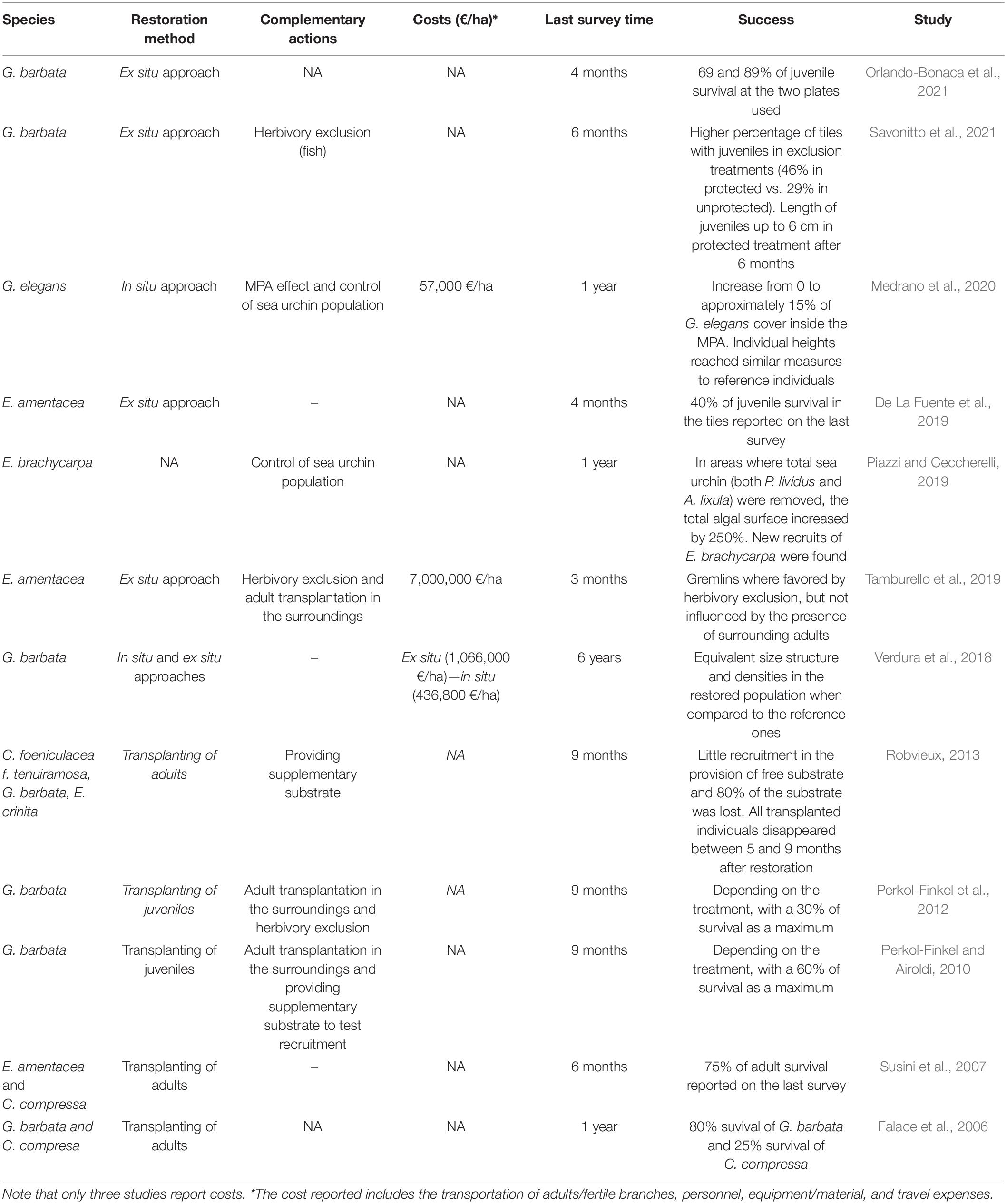
Table 1. Available studies reporting restoration methods for Cystoseira s.l. species in the Mediterranean (using at least one method described in this study).
By way of example, the cost of restoring a shallow species of Cystoseira s.l. in an area very close to facilities (few meters) and with optimal environmental conditions (limited hydrodynamism and herbivory) has been estimated at approximately 1 million €/ha for ex situ methods and 450,000 €/ha for in situ methods (Verdura et al., 2018). This estimate includes a 2-person experienced team, basic equipment, and consumables. Meanwhile, the cost of in situ revegetation techniques together with sea urchin eradications in a nearby area with all the suitable equipment (Medrano et al., 2020) has been estimated at 57,000 €/ha. The cost increases to almost 7 million €/ha when the restored area is far from facilities, the ex situ method requires laboratory culturing for 40 days, and complementary grazing exclusion devices are needed (Tamburello et al., 2019). These data are in line with those reported for other areas of the world. For example, the cost of the Operation Crayweed project has been estimated at 5.7 million $/ha (Layton et al., 2020), and an estimate of 70,000 $/ha has been reported by Fredriksen et al. (2020) for the green gravel method. A potential source of variation is the reference area considered for cost estimation since costs can refer to the initial restored area or to the final restored area, with the first normally leading to a lower cost estimate given that the final area is usually larger. Note that these cost exercises do not include the science and the specific background needed to underpin decisions such as choice of donor and restoration sites, size of the area to be restored, taxonomic skills, and selection of the optimal restoration method and of eventual complementary approaches (Layton et al., 2020). In addition, cost estimates do not include the non-consumable laboratory instrumentation needed for the ex situ approach to assess fertility (e.g., stereomicroscope, fridge, sensors to measure light irradiation, temperature, and salinity), the laboratory facilities provided with running seawater, or the diving materials. The distance among facilities and sites involved in the restoration also strongly affects the cost and feasibility of interventions, with transport conditions and durations also directly related to a critical bottleneck for germling survival. The transport must be carried out rapidly and in optimal conditions to minimize mortality, making proximity of nursery structures to restoration sites crucial. As a critical stage for recruit survival, long transport distances may affect the final cost of the restoration action, substantially increasing the cost-effectiveness ratio. Moreover, if conducted in the subtidal, the additional costs of sea transport, the logistic constraints of scuba diving (e.g., limited working hours per day), and related equipment must be added. A list of the activities and materials required is reported in Figure 3. To help decision makers to prioritize conservation and restoration actions, a standardization of cost-effectiveness of interventions will help to allocate financial resources more effectively. To this effect, a solution could be to analyse costs based on item-based metrics (i.e., number of surviving individuals, growth of seedlings) or the probability of meeting specific targets (Ahtikoski et al., 2010; Grose, 2013), or by dividing the resulting native cover post-restoration by cost per hectare (Kimball et al., 2015). However, bearing in mind the limited natural extension of some species due to the small size of the remaining populations, cost-effectiveness evaluations should also consider the increase in percentage resulting from the restoration action per se relative to the original cover of the native remnant population (Maron et al., 2013).
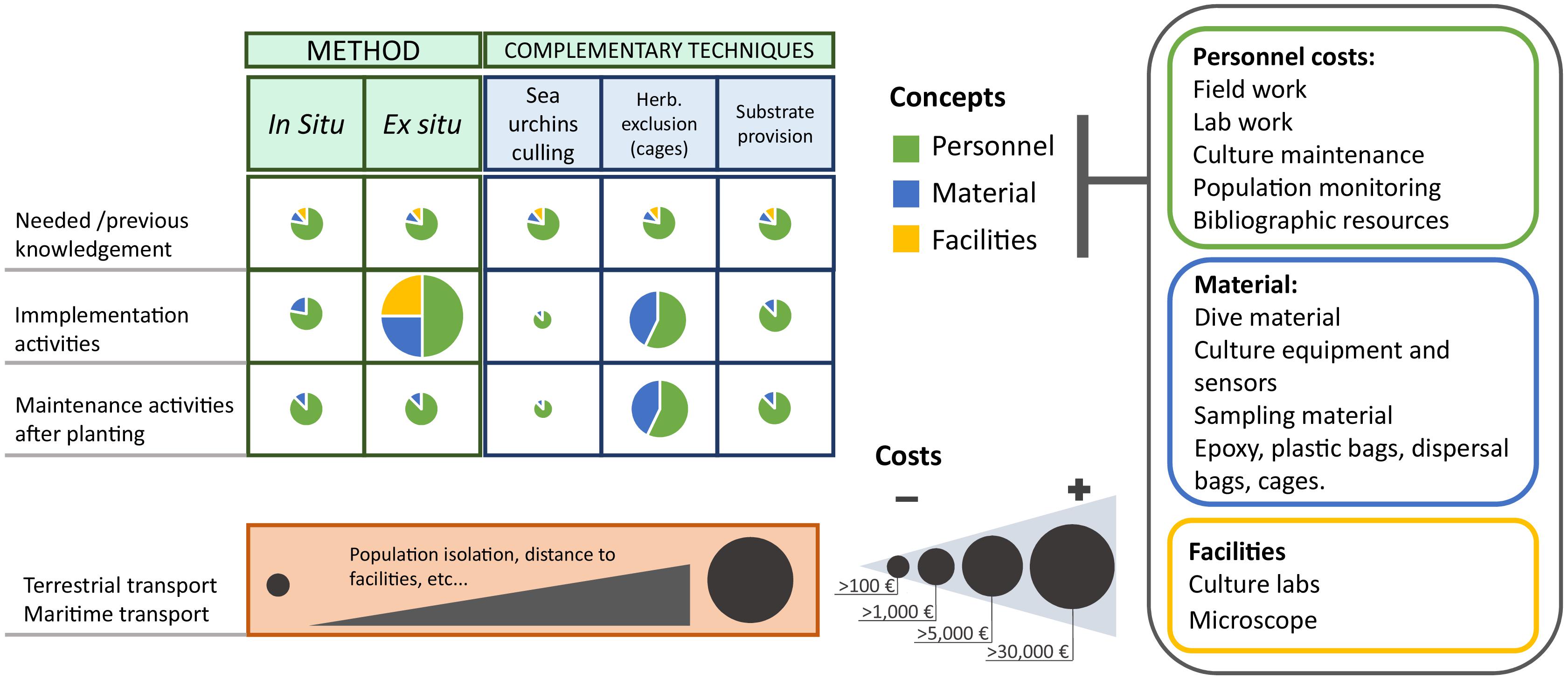
Figure 3. Composition of restoration costs (€) for fucalean macroalgal forests, according to the restoration method and the complementary techniques. The size of the circle represents the cost amount, and the color and size of the different sectors represent the contribution of the different concepts (e.g., personnel, material, and facilities) to the total cost for previous knowledge acquisition, the implementation activities, and the maintenance activities to be performed after planting. Maritime and terrestrial transport are also considered.
Future Perspectives
The interventions aimed at the active restoration of macroalgal forests generally cover a temporal interval of a few months to 1 year. Very few studies cover longer time scales (however, see Verdura et al., 2018; Eger et al., 2020b references therein), generally due to insufficient and intermittent financial investments derived from short-term and discontinuous projects (Eger et al., 2020b). This impairs the assessment of the recovery of ecosystem functioning and thus the actual outcome of restoration in terms of success or failure. According to the guidelines provided by the Society of Ecological Restoration, successful restoration implies that ecosystem functioning and habitat resilience capacity are reverted to the state preceding the degradation (Peterson and Lipcius, 2003; Shackelford et al., 2013). To this effect, indicators of success for macroalgal forest restoration are mostly based on variables such as survival of recruits (Falace et al., 2018; Tamburello et al., 2019; Fredriksen et al., 2020) or transplants (Campbell et al., 2014), the density of juveniles and size structure comparison with donor populations (Verdura et al., 2018), or the percentage of algal cover (Medrano et al., 2020). However, such indicators are far from being capable of estimating the real recovery of the functionality of the system. Conversely, in terrestrial environments, assessment techniques are predominantly based on variables such as biodiversity, vegetation structure, or ecological functions that can provide reliable information on the functionality and ecosystem services recovery (Ruiz-Jaen and Aide, 2005; but see Marzinelli et al., 2016).
In other marine habitats, such as seagrass meadows, it has been demonstrated that restoration scale and feasibility are positively correlated (van Katwijk et al., 2016) due to mechanisms that are likely also relevant for macroalgal forests. For example, the settlement of more specimens would provide a critical mass for stress amelioration by the starting founders, thus enhancing self-sustaining feedbacks which, in turn, would further increase population growth (Eckman et al., 1989; Steneck et al., 2002). However, further studies are required to identify the minimum spatial extension of intervention over which these mechanisms may become relevant to recover macroalgal forests. Notably, however, although increasing the restoration scale can be positive it also has its disadvantages such as the arguably lower feasibility of getting funding as costs increase. Similarly, further studies are needed to assess the outcome of restoration in relation to species diversity. Experimental studies on seagrass restoration show that transplanting different species simultaneously may improve their overall survival and growth and thus the trajectory toward successful restoration (Valdez et al., 2020). Incorporating species diversity into restoration heralds a shift in practice from establishing a single founder species and recognizes the widely documented positive effects that biodiversity has on ecosystem function and services (Williams et al., 2017). Restoration actions should also embrace habitat variability and support adaptation to local conditions. Therefore, to gain more habitat-specific knowledge we need to know which environmental drivers favor the targeted habitat, which species need to be manipulated, and what the functional difference due to the absence of some species will be. Among the biological and ecological features, life-history traits, population connectivity, spatial distribution, structural complexity, and the potential for transition from one state to another rank as the main factors contributing to the successful accomplishment of habitat restoration (Bekkby et al., 2020). However, most of these aspects remain unknown for many assemblages and species. Basic research and monitoring programs are therefore essential to fill this gap. Long-term data on key processes and habitat features will provide a robust basis for upscale restoration actions that will have a significant ecological impact at the seascape level. Moreover, the combination of active restoration strategies, such as sea urchin removal together with algal recruitment enhancement, and passive conservation tools, such as MPAs enabling the increase of sea urchin predators, could have synergistic effects and reduce the maintenance costs of restoration activities by means of re-establishing ecological functions (Gianni et al., 2013; Caselle et al., 2018).
The vulnerability of canopy-forming macroalgae to climate change (Verdura et al., 2021) should also undoubtedly be considered to reduce the uncertainty of investing in a long-term restoration plan (Wood et al., 2019). The viability of ecological restoration could be strongly compromised by accelerated environmental modifications associated with climate change. An important limitation of most current studies is the lack of information on how the current conservation status of canopy-forming populations determines future responses to climate change. To this effect, basal knowledge about the conservation of Cystoseira populations is key to setting a common baseline and further determining how they will respond to future climate warming. Multiple stressors have historically been eroding the conservation status of many Cystoseira forests (Thibaut et al., 2005, 2015; Blanfuné et al., 2016; Mariani et al., 2019), not only impairing important biological and demographic population parameters but also having unknown further consequences on population genetics, thus constraining their resistance and resilience to future climate stressors. A promising but as yet untapped opportunity to counteract likely undesired effects related to climate change is the exploitation of the natural genetic variability of key species (Prober et al., 2015). While the capacity of plants to adapt to environmental change through plasticity, selection, or gene flow has been intensively explored (Prober et al., 2015), the available knowledge for macroalgal habitats and species is still scarce. In this regard, experiments exploring physiological and biological responses to future climate change, considering the actual conservation status and genetic characteristics of different populations and species, will provide new insights into the persistence of Cystoseira populations in the face of future cumulative impacts scenarios.
The impacts of climate change are highly variable geographically, so modeling approaches that consider the intrinsic adaptation of habitats and species, together with predictions of threats related to climate change, are essential to properly assess the fate of species, habitats, and sites following restoration. All this information on the conservation status of forests, resistance to climate change, and intra-species variability to thermal tolerance, in addition to accurate models of the future suitability of habitats, must be considered to design restoration strategies adapted to cope with the inevitable changing future climate conditions.
Although the last decade has seen a growing interest in the restoration of macroalgal forests (Abelson et al., 2020) there is still a lack of knowledge, which needs to be expanded for the successful scaling up of restoration interventions. The inherent uncertainty linked to the above-described outcomes of the steps in active restoration and the difficulty in involving the private sector further impair restoration efforts. We recommend that all restoration steps must be planned carefully before implementation. Although the protocols provided in this study are user-friendly, volunteers and other potential stakeholders should never launch initiatives without expert scientific supervision.
Author Contributions
EC, SF, JV, and LT: conceptualization, writing—review, editing, and overall supervision of the work. All authors contributed to the article and approved the submitted version.
Funding
This work was supported by the CSIC Library will cover 25% of the APC. AFRIMED. European Maritime and Fisheries Fund EMFF (Grant/Award No. EASME/EMFF/2017/1.2.1.12/S4/01/SI2.789059), MERCES, Horizon 2020 Framework Programme (Grant/Award No. 689518), FoRestA, Spanish Ministry of Science and Innovation (Grant/Award No. PID2020-112985GB-I00), Generalitat de Catalunya (Grant/Award No. 2017 SGR 1521), Universitat de Girona, Ph.D. Grant to JV (Award No. IFUdG-2016), and the Fundación tatiana Pérez de Guzman el Bueno Ph.D. Grant to CG.
Conflict of Interest
The authors declare that the research was conducted in the absence of any commercial or financial relationships that could be construed as a potential conflict of interest.
Publisher’s Note
All claims expressed in this article are solely those of the authors and do not necessarily represent those of their affiliated organizations, or those of the publisher, the editors and the reviewers. Any product that may be evaluated in this article, or claim that may be made by its manufacturer, is not guaranteed or endorsed by the publisher.
Supplementary Material
The Supplementary Material for this article can be found online at: https://www.frontiersin.org/articles/10.3389/fmars.2021.709219/full#supplementary-material
Footnotes
References
Abelson, A., Reed, D. C., Edgar, G. J., Smith, C. S., Kendrick, G. A., Orth, R. J., et al. (2020). Challenges for restoration of coastal marine ecosystems in the Anthropocene. Front. Mar. Sci. 7:544105. doi: 10.3389/fmars.2020.544105
Agnetta, D., Badalamenti, F., Ceccherelli, G., Di Trapani, F., Bonaviri, C., and Gianguzza, P. (2015). Role of two co-occurring Mediterranean sea urchins in the formation of barren from Cystoseira canopy. Estuar. Coast. Shelf Sci. 152, 73–77. doi: 10.1016/j.ecss.2014.11.023
Ahtikoski, A., Alenius, V., and Mäkitalo, K. (2010). Scots pine stand establishment with special emphasis on uncertainty and cost-effectiveness, the case of northern Finland. New For. 40, 69–84. doi: 10.1007/s11056-009-9183-2
Airoldi, L. (1998). Roles of disturbance, sediment stress, and substratum retention on spatial dominance in algal turf. Ecology 79, 2759–2770.
Airoldi, L. (2000). Responses of algae with different life histories to temporal and spatial variability of disturbance in subtidal reefs. Mar. Ecol. Prog. Ser. 195, 81–92. doi: 10.3354/meps195081
Airoldi, L. (2003). The effects of sedimentation on rocky coast assemblages. Oceanogr. Mar. Biol. 41, 161–236. doi: 10.1201/9780203180570.ch4
Assis, J., Fragkopoulou, E., Neiva, J., Abecasis, D., Faugeron, S., and Serrão, E. A. (2020). A fine-tuned global distribution dataset of marine forests. Sci. Data 7, 1–9. doi: 10.1038/s41597-020-0459-x
Ballesteros, E. (1989). “Els fons de l’illa de Menorca: bionomia, estat general de conservaciò, interès i zones a protegir,” in Jornades sobre Conservació i Desenvolupament A Menorca, eds J. Vidal and J. Riga (MAB, Unesco: Maò), 137–141.
Ballesteros, E. (1990). Structure and dynamics of the community of Cystoseira zosteroides (Turner) C. Agardh (Fucales, Phaeophyceae) in the Northwestern Mediterranean. Sci. Mar. 54, 217–229.
Ballesteros, E. (1992). Els vegetals i la zonació litoral: espècies, comunitats i factors que influeixen en la seva distribució. Arx. Secció Ciències Inst. d’Estudis Catalans 101, 1–616. doi: 10.1017/CBO9781107415324.004
Ballesteros, E., Garrabou, J., Hereu, B., Zabala, M., Cebrian, E., and Sala, E. (2009). Deep-water stands of Cystoseira zosteroides C. Agardh (Fucales, Ochrophyta) in the Northwestern Mediterranean: insights into assemblage structure and population dynamics. Estuar. Coast. Shelf Sci. 82, 477–484. doi: 10.1016/j.ecss.2009.02.013
Ballesteros, E., Sala, E., Garrabou, J., and Zabala, M. (1998). Community structure and frond size distribution of a deep water stand of Cystoseira spinosa (Phaeophyta) in the Northwestern Mediterranean. Eur. J. Phycol. 33, 121–128.
Bayraktarov, E., Saunders, M. I., Abdullah, S., Mills, M., Beher, J., Possingham, H. P., et al. (2016). The cost and feasibility of marine coastal restoration. Ecol. Appl. 26, 1055–1074. doi: 10.5061/dryad.rc0jn
Bekkby, T., Papadopoulou, N., Fiorentino, D., McOwen, C. J., Rinde, E., Boström, C., et al. (2020). Habitat features and their influence on the restoration potential of marine habitats in Europe. Front. Mar. Sci. 7:184. doi: 10.3389/fmars.2020.00184
Benedetti-Cecchi, L., and Cinelli, F. (1992). Effects of canopy cover, herbivores and substratum type on patterns of Cystoseira spp. settlement and recruitment in littoral rockpools. Mar. Ecol. Prog. Ser. 90, 183–191. doi: 10.3354/meps090183
Bevilacqua, S., Savonitto, G., Lipizer, M., Mancuso, P., Ciriaco, S., Srijemsi, M., et al. (2019). Climatic anomalies may create a long-lasting ecological phase shift by altering the reproduction of a foundation species. Ecology 100:e02838. doi: 10.1002/ecy.2838
Blanfuné, A., Boudouresque, C. F., Verlaque, M., and Thibaut, T. (2016). The fate of Cystoseira crinita, a forest-forming Fucale (Phaeophyceae. Stramenopiles), in France (North Western Mediterranean Sea). Estuar. Coast. Shelf Sci. 181, 196–208. doi: 10.1016/j.ecss.2016.08.049
Boada, J., Arthur, R., Alonso, D., Pagès, J. F., Pessarrodona, A., Oliva, S., et al. (2017). Immanent conditions determine imminent collapses: nutrient regimes define the resilience of macroalgal communities. Proc. R. Soc. B Biol. Sci 284:20162814. doi: 10.1098/rspb.2016.2814
Boudouresque, C. F. (1972). Recherches de bionomie analytique, structurale et expérimentale sur les peuplements benthiques sciaphiles de Méditerranée Occidentale: le sous-strate sciaphile d’un peuplement photophile de mode calme, le peuplement à Cystoseira crinita. Bull. du Muséum d’Histoire Nat. Marseille 32, 253–263.
Bulleri, F., Benedetti-Cecchi, L., and Cinelli, F. (1999). Grazing by the sea urchins Arbacia lixula L. and Paracentrotus lividus Lam. in the Northwest Mediterranean. J. Exp. Mar. Bio. Ecol. 241, 81–95. doi: 10.1016/S0022-0981(99)00073-8
Buonomo, R., Assis, J., Fernandes, F., Engelen, A. H., Airoldi, L., and Serrão, E. A. (2017). Habitat continuity and stepping-stone oceanographic distances explain population genetic connectivity of the brown alga Cystoseira amentacea. Mol. Ecol. 26, 766–780. doi: 10.1111/mec.13960
Calumpong, H., and Fonseca, M. (2001). “Seagrass transplantation and other seagrass restoration methods,” in Global Seagrass Research Methods, eds F. Short, C. Short, and R. Coles (Amsterdam: Elservier), 425–443.
Campbell, A. H., Marzinelli, E. M., Vergés, A., Coleman, M. A., and Steinberg, P. D. (2014). Towards restoration of missing underwater forests. PLoS One 9:e84106. doi: 10.1371/journal.pone.0084106
Campbell, M. L. (2002). Getting the foundation right: a scientifically based management framework to aid in the planning and implementation of seagrass transplant efforts. Bull. Mar. Sci. 71, 1405–1414.
Capdevila, P., Hereu, B., Riera, J. L., and Linares, C. (2016). Unravelling the natural dynamics and resilience patterns of underwater Mediterranean forests: insights from the demography of the brown alga Cystoseira zosteroides. J. Ecol. 140, 1799–1808. doi: 10.1111/1365-2745.12625
Capdevila, P., Linares, C., Aspillaga, E., Navarro, L., Kersting, D., and Hereu, B. (2015). Recruitment patterns in the Mediterranean deep-water alga Cystoseira zosteroides. Mar. Biol. 162, 1165–1174. doi: 10.1007/s00227-015-2658-0
Carney, L. T., Waaland, J. R., Klinger, T., and Ewing, K. (2005). Restoration of the bull kelp Nereocystis luetkeana in nearshore rocky habitats. Mar. Ecol. Prog. Ser. 302, 49–61. doi: 10.3354/meps302049
Caselle, J. E., Davis, K., and Marks, L. M. (2018). Marine management affects the invasion success of a non-native species in a temperate reef system in California. U.S.A. Ecol. Lett. 21, 43–53. doi: 10.1111/ele.12869
Cheminée, A., Sala, E., Pastor, J., Bodilis, P., Thiriet, P., Mangialajo, L., et al. (2013). Nursery value of Cystoseira forests for Mediterranean rocky reef fishes. J. Exp. Mar. Bio. Ecol. 442, 70–79. doi: 10.1016/j.jembe.2013.02.003
Claudet, J., Guidetti, P., Mouillot, D., Shears, N., and Micheli, F. (2011). “Effects of marine protected areas. Ecology: ecological effects of marine protected areas: conservation, restoration, and functioning,” in Marine Protected Areas. A Multidisciplinary Approach, ed. J. Claudet (Cambridge: Cambridge University Press), 37–71.
Connell, S. D., and Russell, B. D. (2010). The direct effects of increasing CO2 and temperature on non-calcifying organisms: increasing the potential for phase shifts in kelp forests. Proc. R. Soc. B Biol. Sci. 277, 1409–1415. doi: 10.1098/rspb.2009.2069
Cormaci, M., and Furnari, G. (1999). “Changes of the benthic algal flora of the Tremiti Islands (southern Adriatic) Italy,”in Sixtheen International Seaweed Symposium, Developments in Hydrobiology, Vol 39, eds M.T. Brown and M. Lahaye (New York, NY: Springer), 75–79. doi: 10.1007/978-94-011-4449-0_9
de Caralt, S., Verdura, J., Vergés, A., Ballesteros, E., and Cebrian, E. (2020). Differential effects of pollution on adult and recruits of a canopy-forming alga: implications for population viability under low pollutant levels. Sci. Rep. 10:17825. doi: 10.1038/s41598-020-73990-5
De La Fuente, G., Chiantore, M., Asnaghi, V., Kaleb, S., and Falace, A. (2019). First ex situ outplanting of the habitat- forming seaweed Cystoseira amentacea var. stricta from a restoration perspective. PeerJ 7:e7290. doi: 10.7717/peerj.7290
Eckman, J. E., Duggins, D. O., and Sewell, A. T. (1989). Ecology of under story kelp environments. I. Effects of kelps on flow and particle transport near the bottom. J. Exp. Mar. Biol. Ecol. 129, 173–187. doi: 10.1016/0022-0981(89)90055-5
Eger, A. M., and Baum, J. (2020). Trophic cascades and connectivity in coastal benthic marine ecosystems: a meta-analysis of experimental and observational research. Mar. Ecol. Prog. Ser. 656, 139–152. doi: 10.3354/meps13430
Eger, A. M., Marzinelli, E., Christie, H., Fujita, D., Hong, S., Kim, J. H., et al. (2021). Global kelp forest restoration: past lessons, status, and future goals. EcoEvoRxiv [Preprint] doi: 10.32942/osf.io/emaz2
Eger, A. M., Vergés, A., Choi, C. G., Christie, H., Coleman, M. A., Fagerli, C. W., et al. (2020b). Financial and institutional support are important for large-scale kelp forest restoration. Front. Mar. Sci. 7:535277. doi: 10.3389/fmars.2020.535277
Eger, A. M., Marzinelli, E., Gribben, P., Johnson, C. R., Layton, C., Steinberg, P. D., et al. (2020a). Playing to the positives: using synergies to enhance kelp forest restoration. Front. Mar. Sci. 7:544. doi: 10.3389/fmars.2020.00544
Evans, S. M., Sinclair, E. A., Poore, A. G. B., Bain, K. F., and Vergés, A. (2018). Assessing the effect of genetic diversity on the early establishment of the threatened seagrass Posidonia australis using a reciprocal-transplant experiment. Restor. Ecol. 26, 570–580. doi: 10.1111/rec.12595
Falace, A., Kaleb, S., De La Fuente, G., Asnaghi, V., and Chiantore, M. (2018). Ex situ cultivation protocol for Cystoseira amentacea var. stricta (Fucales, Phaeophyceae) from a restoration perspective. PLoS One 13:e0193011. doi: 10.1371/journal.pone.0193011
Falace, A., Zanelli, E., and Bressan, G. (2006). Algal transplantation as a potential tool for artificial reef management and environmental mitigation. Bull. Mar. Sci. 78, 161–166.
Farina, S., Baroli, M., Brundu, R., Conforti, A., Cucco, A., De Falco, G., et al. (2020). The challenge of managing the commercial harvesting of the sea urchin Paracentrotus lividus: advanced approaches are required. PeerJ 8, 1–27. doi: 10.7717/peerj.10093
Feldmann, J. (1937). Recherches sur la végétation marine de la Méditerranée: la côte des Albères. Revue Algologique 10, 1–340.
Filbee-Dexter, K., and Scheibling, R. E. (2014). Sea urchin barrens as alternative stable states of collapsed kelp ecosystems. Mar. Ecol. Prog. Ser. 495, 1–25. doi: 10.3354/meps10573
Filbee-Dexter, K., and Wernberg, T. (2018). Rise of turfs: a new battlefront for globally declining kelp forests. BioScience 68, 64–76. doi: 10.1093/biosci/bix147
Filbee-Dexter, K., Feehan, C. J., and Scheibling, R. E. (2016). Large-scale degradation of a kelp ecosystem in an ocean warming hotspot. Mar. Ecol. Prog. Ser. 543, 141–152. doi: 10.3354/meps11554
Fredriksen, S., Filbee-Dexter, K., Norderhaug, K. M., Steen, H., Bodvin, T., Coleman, M. A., et al. (2020). Green gravel: a novel restoration tool to combat kelp forest decline. Sci. Rep. 10:3983. doi: 10.1038/s41598-020-60553-x
Gagnon, K., Rinde, E., Bengil, E. G. T., Carugati, L., Christianen, M. J. A., Danovaro, R., et al. (2020). Facilitating foundation species: the potential for plant–bivalve interactions to improve habitat restoration success. J. Appl. Ecol. 57, 1161–1179. doi: 10.1111/1365-2664.13605
Giaccone, G., and Bruni, A. (1973). Le Cystoseire e la vegetazione sommersa del Mediterraneo. Atti Istituto Veneto Scienze, Lettere ed Arti 131, 59–103.
Gianni, F., Bartolini, F., Airoldi, L., Ballesteros, E., Francour, P., Guidetti, P., et al. (2013). Conservation and restoration of marine forests in the Mediterranean Sea and the potential role of Marine Protected Areas. Adv. Oceanogr. Limnol. 4, 83–101. doi: 10.1080/19475721.2013.845604
Gianni, F., Mačić, V., Bartolini, F., Pey, A., Laurent, M., and Mangialajo, L. (2020). Optimizing canopy-forming algae conservation and restoration with a new herbivorous fish deterrent device. Restor. Ecol. 28, 750–756. doi: 10.1111/rec.13143
Goodsell, P. J., and Chapman, M. G. (2009). “Rehabilitation of habitat and the value of artificial reefs,” in Marine Hard Bottom Communities: Patterns, Dynamics, Diversity, and Change, ed. M. Wahl (New York, NY: Springer), 333–344. doi: 10.1007/978-3-540-92704-4
Gorgula, S. K., and Connell, S. D. (2004). Expansive covers of turf-forming algae on human-dominated coast: the relative effects of increasing nutrient and sediment loads. Mar. Biol. 145, 613–619. doi: 10.1007/s00227-004-1335-5
Gorman, D., and Connell, S. D. (2009). Recovering subtidal forests in human-dominated landscapes. J. Appl. Ecol. 46, 1258–1265. doi: 10.1111/j.1365-2664.2009.01711.x
Gros, C. (1978). Le genre Cystoseira sur la côte des Albères. Répartition, écologie, morphogénèse. [dissertation thesis]. Univ. Pierre et Marie Curic, Paris.
Grose, P. J. (2013). Cost-effectiveness of different revegetation techniques for Slender Banksia. Ecol. Restor. 31, 237–240. doi: 10.3368/er.31.3.237
Guarnieri, G., Bevilacqua, S., Figueras, N., Tamburello, L., and Fraschetti, S. (2020). Large-scale sea urchin culling drives the reduction of subtidal barren grounds in the Mediterranean Sea. Front. Mar. Sci. 7:519. doi: 10.3389/fmars.2020.00519
Guern, M. (1962). Embryologie de quelques espèces du genre Cystoseira Agardh 1821 (Fucales). Vie et Milieu 13, 649–679.
Guidetti, P., Terlizzi, A., and Boero, F. (2004). Effects of the edible sea urchin. Paracentrotus lividus, fishery along the Apulian rocky coast (SE Italy, Mediterranean Sea). Fish. Res. 66, 287–297. doi: 10.1016/S0165-7836(03)00206-6
Hereu, B. (2006). Depletion of palatable algae by sea urchins and fishes in a Mediterranean subtidal community. Mar. Ecol. Prog. Ser. 313, 95–103. doi: 10.3354/meps313095
Hillebrand, H., Jacob, U., and Leslie, H. M. (2020). Integrative research perspectives on marine conservation. Phil. Trans. R. Soc. B 375:20190444. doi: 10.1098/rstb.2019.0444rstb20190444
Ingvarsson, P. K. (2001). Restoration of genetic variation lost-the genetic rescue hypothesis. Trends Ecol. Evol. 16, 62–63. doi: 10.1016/S0169-5347(00)02065-6
Isaeus, M., Malm, T., Persson, S., and Svensson, A. (2004). Effects of filamentous algae and sediment on recruitment and survival of Fucus serratus (Phaeophyceae) juveniles in the eutrophic Baltic Sea. Eur. J. Phycol. 39, 301–307. doi: 10.1080/09670260410001714732
Iveša, L., Djakovac, T., and Devescovi, M. (2016). Long-term fluctuations in Cystoseira populations along the west Istrian Coast (Croatia) related to eutrophication patterns in the northern Adriatic Sea. Mar. Pollut. Bull. 106, 162–173. doi: 10.1016/j.marpolbul.2016.03.010
Kimball, S., Lulow, M., Sorenson, Q., Balazs, K., Fang, Y. C., Davis, S. J., et al. (2015). Cost-effective ecological restoration. Restor. Ecol. 23, 800–810. doi: 10.1111/rec.12261
Layton, C., Coleman, M. A., Marzinelli, E. M., Steinberg, P. D., Swearer, S. E., Vergés, A., et al. (2020). Kelp forest restoration in Australia. Front. Mar. Sci. 7:74. doi: 10.3389/fmars.2020.00074
Ling, S. D., Scheibling, R. E., Rassweiler, A., Johnson, C. R., Shears, N., Connell, S. D., et al. (2015). Global regime shift dynamics of catastrophic sea urchin overgrazing. Phil. Trans. R. Soc. B 370:20130269. doi: 10.1098/rstb.2013.0269
Maggi, E., Puccinelli, E., and Benedetti-Cecchi, L. (2018). Ecological feedback mechanisms and variable disturbance regimes: the uncertain future of Mediterranean macroalgal forests. Mar. Environ. Res. 140, 342–357. doi: 10.1016/j.marenvres.2018.07.002
Mariani, S., Cefalì, M. E., Chappuis, E., Terradas, M., Pinedo, S., Torras, X., et al. (2019). Past and present of Fucales from shallow and sheltered shores in Catalonia. Reg. Stud. Mar. Sci. 32:100824. doi: 10.1016/j.rsma.2019.100824
Marion, S. R., and Orth, R. J. (2010). Innovative techniques for large-scale seagrass restoration using Zostera marina (eelgrass) seeds. Restor. Ecol. 18, 514–526. doi: 10.1111/j.1526-100X.2010.00692.x
Maron, M., Rhodes, J. R., and Gibbons, P. (2013). Calculating the benefit of conservation actions. Conserv. Lett. 6, 359–367. doi: 10.1111/conl.12007
Marzinelli, E. M., Leong, M. R., Campbell, A. H., Steinberg, P. D., and Verges, A. (2016). Does restoration of a habitat-forming seaweed restore associated faunal diversity? Restor. Ecol. 24, 81–90. doi: 10.1111/rec.12292
Medrano, A., Hereu, B., Cleminson, M., Pagès-Escolà, M., Rovira, G., Solà, J., et al. (2020). From marine deserts to algal beds: Treptacantha elegans revegetation to reverse stable degraded ecosystems inside and outside a No-Take marine reserve. Restor. Ecol. 28, 632–644. doi: 10.1111/rec.13123
Medrano, A., Linares, C., Aspillaga, E., Capdevila, P., Montero-Serra, I., Pagès-Escolà, M., et al. (2019). No-take marine reserves control the recovery of sea urchin populations after mass mortality events. Mar. Environ. Res. 145, 147–154. doi: 10.1016/j.marenvres.2019.02.013
Molinari-Novoa, E., and Guiry, M. (2020). Reinstatement of the genera Gongolaria Boehmer and Ericaria Stackhouse (Sargassaceae. Phaeophyceae). Not. Algarum 172, 1–10.
Molinier, R. (1960). Étude des biocénoses marines du Cap Corse. Vegetatio 9, 212–312. doi: 10.1007/BF02917032
Montero-Serra, I., Garrabou, J., Doak, D. F., Figuerola, L., Hereu, B., Ledoux, J. B., et al. (2018). Accounting for life-history strategies and timescales in marine restoration. Conser. Lett. 11, 1–9. doi: 10.1111/conl.12341
Morris, R. L., Hale, R., Strain, E. M. A., Reeves, S. E., Vergés, A., Marzinelli, E. M., et al. (2020). Key principles for managing recovery of kelp forests through restoration. Bioscience 70, 688–698. doi: 10.1093/biosci/biaa058
Munda, I. M. (1982). The effects of organic pollution on the distribution of fucoid algae from the Istrian coast (vicinity of Rovinj). Acta Adriatica 23, 329–337.
Munda, I. M. (1993). Changes and degradation of seaweed stands in the Northern Adriatic. Hydrobiologia 260, 239–253. doi: 10.1007/BF00049025
Orlando-Bonaca, M., Pitacco, V., Slavinec, P., Šiško, M., Makovec, T., and Falace, A. (2021). First restoration experiment for Gongolaria barbata in Slovenian coastal waters. What can go wrong? Plants 10:239.
Orth, R. J., Carruthers, T. J. B., Dennison, W. C., Duarte, C. M., Fourqurean, J. W., Heck, K. L. Jr., et al. (2006). A global crisis for seagrass ecosystems. Bioscience 56, 987–996.
Oudot-Canaff, J., Bornette, G., Viricel, M. R., Piola, F., Mousset, S., and Martel, E. (2013). The short-term impact of wetland restoration on the genetic diversity of a predominantly clonal plant species. Aquat. Bot. 110, 16–23. doi: 10.1016/j.aquabot.2013.04.006
Perkol-Finkel, S., and Airoldi, L. (2010). Loss and recovery potential of marine habitats: an experimental study of factors maintaining resilience in subtidal algal forests at the Adriatic Sea. PLoS One 5:e10791. doi: 10.1371/journal.pone.0010791
Perkol-Finkel, S., Ferrario, F., Nicotera, V., and Airoldi, L. (2012). Conservation challenges in urban seascapes: promoting the growth of threatened species on coastal infrastructures. J. Appl. Ecol. 49, 1457–1466. doi: 10.1111/j.1365-2664.2012.02204.x
Peterson, C. H., and Lipcius, R. N. (2003). Conceptual progress towards predicting quantitative ecosystem benefits of ecological restorations. Mar. Ecol. Prog. Ser. 264, 297–307. doi: 10.3354/meps264297
Piazzi, L., and Ceccherelli, G. (2019). Effect of sea urchin human harvest in promoting canopy forming algae restoration. Estuar. Coast. Shelf Sci. 219, 273–277. doi: 10.1016/j.ecss.2019.02.028
Piazzi, L., Balata, D., and Ceccherelli, G. (2017). Resilience of Cystoseira beds: lack of canopy effects on recruitment. Bot. Mar. 60, 49–53. doi: 10.1515/bot-2016-0088
Pinedo, S., Zabala, M., and Ballesteros, E. (2013). Long-term changes in sublittoral macroalgal assemblages related to water quality improvement. Bot. Mar. 56, 461–469. doi: 10.1515/bot-2013-0018
Prober, S. M., Byrne, M., McLean, E. H., Steane, D. A., Potts, B. M., Vaillancourt, R. E., et al. (2015). Climate-adjusted provenancing: a strategy for climate-resilient ecological restoration. Front. Ecol. Evol. 3:65. doi: 10.3389/fevo.2015.00065
Procaccini, G., and Piazzi, L. (2001). Genetic polymorphism and transplantation success in the mediterranean seagrass Posidonia oceanica. Restor. Ecol. 9, 332–338. doi: 10.1046/j.1526-100X.2001.009003332.x
Reed, D. C. (1990). The effects of variable settlement and early competition on patterns of kelp recruitment. Ecology 71, 776–787.
Rindi, L., Dal Bello, M., and Benedetti-Cecchi, L. (2018). Experimental evidence of spatial signatures of approaching regime shifts in macroalgal canopies. Ecology 99, 1709–1715. doi: 10.1002/ecy.2391
Robvieux, P. (2013). Conservation des populations de Cystoseira en régions Provence-Alpes-Côte-d’Azur et Corse. [dissertation thesis], Université Nice Sophia Antipolis.
Rodríguez-Prieto, C., Ballesteros, E., Boisset, F., and Afonso-Carrillo, J. (2013). Guía De Las Macroalgas Y Fanerógamas Marinas Del Mediterráneo Occidental. Barcelona: Omega.
Ruiz-Jaen, M. C., and Aide, T. M. (2005). Restoration success: how is it being measured? Restor. Ecol. 13, 569–577. doi: 10.1111/j.1526-100X.2005.00072.x
Sala, E., Boudouresque, C. F., and Harmelin, M. (1998). Fishing, trophic cascades, and the structure of algal assemblages: evaluation of and old but untested paradigm. Oikos 82, 425–439. doi: 10.2307/3546364
Sala, E., Kizilkaya, Z., Yildirim, D., and Ballesteros, E. (2011). Alien marine fishes deplete algal biomass in the Eastern Mediterranean. PLoS One 6:e17356. doi: 10.1371/journal.pone.0017356
Sales, M., Ballesteros, E., Anderson, M. J., Iveša, L., and Cardona, E. (2012). Biogeographical patterns of algal communities in the Mediterranean Sea: Cystoseira crinita-dominated assemblages as a case study. J. Biogeogr. 39, 140–152. doi: 10.1111/j.1365-2699.2011.02564.x
Savonitto, G., De La Fuente, G., Tordoni, E., Ciriaco, S., Srijemsi, M., Bacaro, G., et al. (2021). Addressing reproductive stochasticity and grazing impacts in the restoration of a canopy-forming brown alga by implementing mitigation solutions. Aquat. Conserv. Mar. Freshw. Ecosyst. 31, 1611–1623. doi: 10.1002/aqc.3555
Scheffer, M., Carpenter, S., Foley, A. F., Folke, C., and Walker, B. (2001). Catastrophic shifts in ecosystems. Nature 413, 591–596. doi: 10.1038/35098000
Shackelford, N., Hobbs, R. J., Burgar, J. M., Erickson, T. E., Fontaine, J. B., Laliberté, E., et al. (2013). Primed for change: developing ecological restoration for the 21st century. Restor. Ecol. 21, 297–304. doi: 10.1111/rec.12012
Shears, N. T., and Babcock, R. C. (2003). Continuing trophic cascade effects after 25 years of no-take marine reserve protection. Mar. Ecol. Prog. Ser. 246, 1–16. doi: 10.3354/meps246001
Short, F. T., Davis, R. C., Kopp, B. S., Short, C. A., and Burdick, D. M. (2002). Site-selection model for optimal transplantation of eelgrass Zostera marina in the northeastern US. Mar. Ecol. Prog. Ser. 227, 253–267.
Soltan, D., Verlaque, M., Boudouresque, C. F., and Francour, P. (2001). Changes in macroalgal communities in the vicinity of a Mediterranean sewage outfall after the setting up of a treatment plant. Mar. Pollut. Bull. 42, 59–70. doi: 10.1016/S0025-326X(00)00116-8
Steneck, R. S., Graham, M. H., Bourque, B. J., Corbett, D., Erlandson, J. M., Estes, J. A., et al. (2002). Kelp forest ecosystems: biodiversity, stability, resilience and future. Environ. Conserv. 29, 436–459. doi: 10.1017/S0376892902000322
Susini, M. L., Mangialajo, L., Thibaut, T., and Meinesz, A. (2007). Development of a transplantation technique of Cystoseira amentacea var. stricta and Cystoseira compressa. Hydrobiologia 580, 241–244. doi: 10.1007/s10750-006-0449-9
Swan, K. D., McPherson, J. M., Seddon, P. J., and Moehrenschlager, A. (2016). Managing marine biodiversity: the rising diversity and prevalence of marine conservation translocations. Conserv. Lett. 9, 239–251. doi: 10.1111/conl.12217
Tamburello, L., Papa, L., Guarnieri, G., Basconi, L., Zampardi, S., Scipione, M. B., et al. (2019). Are we ready for scaling up restoration actions? An insight from Mediterranean macroalgal canopies. PLoS One 14:e0224477. doi: 10.1371/journal.pone.0224477
Thibaut, T., Blanfuné, A., Boudouresque, C. F., and Verlaque, M. (2015). Decline and local extinction of fucales in french riviera: the harbinger of future extinctions? Mediterr. Mar. Sci. 16, 206–224. doi: 10.12681/mms.1032
Thibaut, T., Blanfuné, A., Boudouresque, C. F., Personnic, S., Ruitton, S., Ballesteros, E., et al. (2017). An ecosystem-based approach to assess the status of Mediterranean algae-dominated shallow rocky reefs. Mar. Pollut. Bull. 117, 311–329. doi: 10.1016/j.marpolbul.2017.01.029
Thibaut, T., Bottin, L., Aurelle, D., Boudouresque, C.-F., Blanfuné, A., Verlaque, M., et al. (2016). Connectivity of populations of the seaweed Cystoseira amentacea within the Bay of Marseille (Mediterranean Sea): genetic structure and hydrodynamic connections. Cryptogam. Algol. 37, 233–255. doi: 10.7872/crya/v37.iss4.2016.233
Thibaut, T., Pinedo, S., Torras, X., and Ballesteros, E. (2005). Long-term decline of the populations of Fucales (Cystoseira spp. and Sargassum spp.) in the Albères coast (France, North-western Mediterranean). Mar. Pollut. Bull. 50, 1472–1489. doi: 10.1016/j.marpolbul.2005.06.014
Thiriet, P. D., Di Franco, A., Cheminée, A., Guidetti, P., Bianchimani, O., Basthard-Bogain, S., et al. (2016). Abundance and diversity of crypto- and necto-benthiccoastal fish are higher in marine forests than in structurally less complex macroalgal assemblages. PLoS One 11:e0164121. doi: 10.1371/journal.pone.0164121
Tracey, S. R., Baulch, T., Hartmann, K., Ling, S. D., Lucieer, V., Marzloff, M. P., et al. (2014). Systematic culling controls a climate driven, habitat modifying invader. Biol. Invasions 17, 1885–1896. doi: 10.1007/s10530-015-0845-z
UNEP/MAP. (2013). Protocol Concerning Specially Protected Areas And Biological Diversity in the Mediterranean. List of Endangered Species. Athina: UNEP/MAP.
Valdez, S. R., Zhang, Y. S., van der Heide, T., Vanderklift, M. A., Tarquinio, F., Orth, R. J., et al. (2020). Positive ecological interactions and the success of seagrass restoration. Front. Mar. Sci. 7:91. doi: 10.3389/fmars.2020.00091
van Katwijk, M. M., Thorhaug, A., Marbà, N., Orth, R. J., Duarte, C. M., Kendrick, G. A., et al. (2016). Global analysis of seagrass restoration: the importance of large-scale planting. J. Appl. Ecol. 53, 567–578. doi: 10.1111/1365-2664.12562
Verdura, J., Cefalì, M., Orfila, E., Verges, A., Sanchez, M., and Cebrian, E. (2015). Optimal environmental conditions in Cystoseira sp. early life stages. Eur. J. Phycol. 50:214.
Verdura, J., Sales, M., Ballesteros, E., Cefalì, M. E., and Cebrian, E. (2018). Restoration of a canopy-forming alga based on recruitment enhancement: methods and long-term success assessment. Front. Plant Sci. 9:1832. doi: 10.3389/fpls.2018.01832
Verdura, J., Santamaría, J., Ballesteros, E., Smale, D. A., Cefalì, M. E., Golo, R., et al. (2021). Local-scale climatic refugia offer sanctuary for a habitat-forming species during a marine heatwave. J. Ecol. 109, 1758–1773. doi: 10.1111/1365-2745.13599
Vergés, A., Tomas, F., Cebrian, E., Ballesteros, E., Kizilkaya, Z., Verg, A., et al. (2014). Tropical rabbit fish and the deforestation of a warming temperate sea. J. Ecol. 102, 1518–1527. doi: 10.1111/1365-2745.12324
Verlaque, M. (1987). Contributions à l’étude du phytobenthos d’un écosystème photophile thermophile marin en Méditerranée occidentale?: étude structurale et dynamique du phytobenthos et analyse des relations faune - flore. [dissertation thesis], Aix-Marseille.
Wahl, M., Molis, M., Hobday, A. J., Dudgeon, S., Neumann, R., Steinberg, P., et al. (2015). The responses of brown macroalgae to environmental change from local to global scales: direct versus ecologically mediated effects. Perspect. Phycol. 2, 11–29. doi: 10.1127/pip/2015/0019
Waltham, N. J., Elliott, M., Lee, S. Y., Lovelock, C., Duarte, C. M., Buelow, C., et al. (2020). UN Decade on ecosystem restoration 2021-2030-what chance for success in restoring coastal ecosystems? Front. Mar. Sci. 7:1–5. doi: 10.3389/fmars.2020.00071
Watanuki, A., Aota, T., Otsuka, E., Kawai, T., Iwahashi, Y., Kuwahara, H., et al. (2010). Restoration of kelp beds on an urchin barren: removal of sea urchins by citizen divers in southwestern Hokkaido. Bull. Fish Res. Agency 32, 83–87.
Watson, J., and Estes, J. A. (2011). Stability, resilience, and phase shifts in rocky subtidal communities along the west coast of Vancouver Island, Canada. Ecol. Monogr. 81, 215–239. doi: 10.1890/10-0262.1
Williams, S. L., Ambo-Rappe, R., Sur, C., Abbott, J. M., and Limbong, S. R. (2017). Species richness accelerates marine ecosystem restoration in the Coral Triangle. Proc. Natl. Acad. Sci. U.S.A. 114, 11986–11991. doi: 10.1073/pnas.1707962114
Wood, G., Marzinelli, E. M., Coleman, M. A., Campbell, A. H., Santini, N. S., Kajlich, L., et al. (2019). Restoring subtidal marine macrophytes in the Anthropocene: trajectories and future-proofing. Mar. Freshw. Res 70, 936–951. doi: 10.1071/MF18226
Keywords: marine restoration, macroalgae, canopy forming seaweed, macroalgae culture, marine conservation, Cystoseira, fucalean algae, Mediterranean conservation
Citation: Cebrian E, Tamburello L, Verdura J, Guarnieri G, Medrano A, Linares C, Hereu B, Garrabou J, Cerrano C, Galobart C and Fraschetti S (2021) A Roadmap for the Restoration of Mediterranean Macroalgal Forests. Front. Mar. Sci. 8:709219. doi: 10.3389/fmars.2021.709219
Received: 13 May 2021; Accepted: 16 September 2021;
Published: 15 October 2021.
Edited by:
Thomas Wernberg, University of Western Australia, AustraliaReviewed by:
Megan Irene Saunders, Oceans and Atmosphere (CSIRO), AustraliaDaniel Gorman, Commonwealth Scientific and Industrial Research Organisation (CSIRO), Australia
Leigh W. Tait, National Institute of Water and Atmospheric Research (NIWA), New Zealand
Copyright © 2021 Cebrian, Tamburello, Verdura, Guarnieri, Medrano, Linares, Hereu, Garrabou, Cerrano, Galobart and Fraschetti. This is an open-access article distributed under the terms of the Creative Commons Attribution License (CC BY). The use, distribution or reproduction in other forums is permitted, provided the original author(s) and the copyright owner(s) are credited and that the original publication in this journal is cited, in accordance with accepted academic practice. No use, distribution or reproduction is permitted which does not comply with these terms.
*Correspondence: Emma Cebrian, emma@ceab.csic.es