Thermal tolerance and critical maxima examined in marine gastropods inhabiting around Karachi Nuclear Power Plant
- 1Centre of Excellence in Marine Biology, University of Karachi, Karachi, Pakistan
- 2Pakistan Isotope Application Division, PINSTECH, Islamabad, Pakistan
Bensthic communities along the coastal basins are an indication of ecosystem health but highly susceptible owing to manmade activities. This study envisages thermal tolerance in sea snails Monodonta canalifera, Nerita albicilla and Tylothais savignyi inhabiting around the outfall and intake structures of Karachi Nuclear Power Plant (KANUPP). To test the adaptability and vulnerability, a lethality test protocol under controlled temperature was applied in the laboratory where they were exposed at 25, 30, 35, and 40°C, which was raised to 45°C after an acclimation period of 1 week. The critical thermal maximum (CTmax) for the three species was found to be between 39 and 42°C, whereas the lethal temperature (LT50) tests revealed that at the utmost 45°C was lethal for M. canalifera. The correlation between LT50 and CTmaxima (R = 0.47, p = 0.00) and LT50 and body sizes reveals that the thermal adaptability in N. albicilla and T. savignyi (R = 0.65, p = 0.00) was relatively higher than that at 45°C given in the laboratory. In addition, microscopic changes due to temperature, which appeared in the foot (adhesive part) of each species, were deduced from the histological examination. The outcomes of this study would help to underline the ecosystem health around KANUPP and highlight precautionary measures required for the newly established K2/K3 power units to safeguard habitat.
Introduction
The Karachi Nuclear Power Plant (KANUPP) was established a semicentury back, with a capacity of 137 MWe energy generated using 140 tonnes of seawater per hour for the cooling system. A constant increase of energy demand in the Karachi metropolis was overwhelming and thus led to the establishment of K2/K3 power units with a capability of 2,200 KWe to satisfy the power needs. Therefore, seawater intake for cooling purposes and use in power generation has significantly soared. This mass-level water consumption is expected to bring some changes in water temperature and can harm the aquatic ecosystem. However, it would be better to provide energy to the public with the sustainable use of coastal resources. Here we provide data on how an increase in temperature can harm sessile organisms in natural environment and on using indoor experimental practices. It is commonly concurred that the world’s ocean and air temperatures have warmed by 0.6°C in the early 21st century and now reached 2.0–4.5°C (IPCC, 2007). The Arabian Sea deep water temperature rose by 0.65°C and that of coastal waters by 0.86°C; therefore, a low warming tendency persists (Rana et al., 2014). Tropical countries can contribute 6–10°C by means of cooling systems (Poornima et al., 2006), and this is 8–12°C in temperate areas (Hoffmeyer et al., 2005). It is estimated that high-capacity power plants of about 1,000 MWe would need more water, and this water, when released back into the sea, would surely create pollution and harm fish stocks. Thus, temperature is a significant abiotic factor in marine environments (Webb et al., 2008), driving other important changes at the community level by affecting organisms and their distributions, species composition as well as the entire ecosystem (Dallas, 2012). Fluctuations in temperature may alter its physiological functions such as growth rate (Vannote and Sweeney, 1980; Farooq et al., 2017), feeding rate (Kishi et al., 2005), metabolic rate (Eriksen, 1964; Brockington and Clarke, 2001; Ganser et al., 2015), fecundity (Byrne and Przeslawski, 2013), larval dispersal (O'Connor et al., 2007), reproduction (Tropea et al., 2015), immunity (Green et al., 2014), emergence (McKie et al., 2004), behavior (Mora and Ospina, 2001), and, ultimately, survival (Mairaj et al., 2021).
Intertidal ecological systems have been portrayed as “harbingers of fate” (Helmuth, 2002) or “early indicators” (Wethey and Woodin, 2008) for environmental impacts interceded by environmental changes. These types of systems are contrastingly not the same as most tropical conditions since they show massive thermal changes with time and area, with a large portion of the variety happening day by day and between tidal cycles (Helmuth et al., 2002). Thermal range fluctuates broadly among species. Many life forms can endure harsh temperatures in a short time by changing their physical systems under thermal pressure (Jiang et al., 2008). The thermal resistances of organisms are because of long-term natural selection and evolution under ecological systems, and organisms that adjust to elevated temperatures, which is referred to as thermal resilience, are better compared to the ones that desire decreased temperatures (Pörtner, 2001). The rocky intertidal zones are considered as physically complex and variable coastal environments on earth; therefore, thermal effluents are problematic primarily for benthic organisms (Warwick, 1993). Huge numbers of benthic species are sessile or stationary, and even numerous errant species move less throughout their lifetime—so they are defenseless against thermal discharge—since they have a restricted capacity to get away (Bamber and Spencer, 1984). Mollusks are the most important members of the benthic community in rocky intertidal zones. The capability of intertidal mollusk to endure high temperatures is identified with their location in the intertidal zone and their geological dispersal (Markel, 1971).
Upon exposure to heat, marine snails normally undergo a reversible condition of unconsciousness. This abstraction includes foot detachment from the substratum and becoming unsteady, with the foot expansion studied (Sandison, 1967; Fraenkel, 1968). Moreover, the response to thermal stress among various aquatic macroinvertebrates differs among taxa. Some common behavioral responses are loss of grip, which results in detachment from a substrate or rapid movement, ultimately proceeding to immobility and becoming irresponsive when stimulated with a jet of water (Dallas and Ketley, 2011). A microscopic study of snail foot can show the robust cellular deformation due to excessive temperatures (Pavlasek, 1950). In addition, the main part of the snail’s body is the muscular foot, which is well known for slime that secretes visco-elastic mucus gels and that functions to include feeding, protection, reproduction, locomotion, lubrication, defense, and adhesion (Pavlasek, 1950). Increasing the exposure temperature (48, 50, and 52°C) can increase the mucus secretion with enlargement and necrosis of mucocytes (Dittbrenner et al., 2009).
The basic ideas are as follows: (1) to determine the adaptability and vulnerability of sea snails Nerita albicella, Monodonta canalifera, and Tylothais savignyi dwelling around the intake and outfall structures of Karachi Nuclear Power Plant to demonstrate thermal acclimation and their tolerance to maximum temperatures, (2) to examine LT50 depending on size and age, and (3) to provide microscopic changes in the foot due to temperature under controlled conditions.
Materials and methods
Collection of test organisms
The test organisms M. canalifera (Lamarck, 1816), N. albicella (Linnaeus, 1758), and T. savignyi (Deshayes, 1844) were collected from the intake and outfall structures of Karachi Nuclear Power Plant near Paradise Point, Karachi, in October 2017. The specimens were hand-picked or dislodged from rock boulders by adopting random sampling method.
In situ temperature
Seawater temperature of each location was recorded by using hydro-lab (HL-4) USA. The in situ water temperature at the outfall was recorded as 24–31°C, with 28°C representing an intermediate value, whereas an average temperature (24 to 25°C) was recorded from the intake structures during the sampling period.
Field observations, handling, and experiment procedures
Prior to placing the specimens in buckets, a few moments were spent to look into the behavior of sea snails in the natural ecosystem. In the laboratory prior temperature tests, the organisms were acclimatized at 25°C and maintained for 1 week before the experiments commenced. Later on, the snails were transferred into an already adjusted glass aquarium of dimension 44 × 30 × 31 cm (length × width × depth), each trial of both tests (CTmax and LT50) lost water through evaporation and was thus added with seawater to maintain the water level. A steady adjustment of temperature was made using a thermostat, keeping in mind the photoperiod time 16:08 LD. The circulating pump ensured that the percentage saturation of dissolved oxygen remained above 70% during the experiments so that the tanks were well aerated using compressed air passed through multiple airstones.
Critical thermal maxima (CTmax) test
Thirty individuals of each species were used to test CTmax following their random selection and transfer into aquariums for exposure. Two replicates of each exercise were adopted. During the test phase, the water temperature was allowed to stabilize at 25°C for 30 min after it was raised at 1 to 2 min manually with an aquarium heater/thermostat until the individuals were in a state of heat coma. Simultaneously, snail reaction to heating was observed continuously, where critical thermal endpoint was recorded for each species.
Lethal temperature (LT50) test
The lethality test was deduced from 10 individuals of each species which were allowed to adjust in separate experimental aquariums at constant temperatures, i.e., 25, 30, 35, 40, and 45°C, whereas 25°C was set for the control. Four treatments at 30, 35, 40, and 45°C with each of the replicates—thus a total of n = 300 individuals—were used. The test groups were heated for 4 days subsequently until the target temperature was reached. Constant behavioral adaptations for survival were recorded every 24 h.
Behavioral changes
Data on those organisms were included in the results, which were recovered from the critical thermal maxima test, whereas dead organisms at lethal temperature were separated immediately. The shell identification, photograph, lengths, and weights data were recorded for each individual. For the histological examination of the foot, each dead snail was fixed in Davidson fixative for 48 h. After that, the materials were dehydrated at increasing ethanol concentrations and then embedded in paraffin. The wax blocks were sectioned at 4 μm, using a microtome with tungsten knives, and stained with hematoxylin–eosin. The permanent slides were prepared and archived.
Statistical analysis
All statistical analyses were made using R software. Kruskal–Wallis one-way ANOVA and LSD (0.05) multiple comparisons were tested for CTmax. The calculations were made following the expression used (Diaz et al., 2011). The trimmed Spearman–Karber test (95% CI) was applied on the data of LT50 using 24-, 48-, 72-, and 96-h data. A linear model was applied on the snail body sizes against treatment temperature.
Results
Critical thermal maxima (CTmax)
Evaluation of thermal tolerance in M. canalifera (CTmax 40.50 ± 0.28 SE), N. albicilla (CTmax 39.33 ± 0.17 SE), and T. savignyi (CTmax 42.17 ± 0.17 SE) was undertaken in this study. It was revealed that each species displays a critical behavior against different temperatures (Figure 1). This was validated by Kruskal–Wallis one-way analysis of variance and the mean ranks of CTmax. These values were significantly different among the three test species and significant at p = 0.05. All species showed a common behavioral response to elevated temperature. These values were observed when the test species became unresponsive to touch and showed detachment from the wall and base of the tanks.
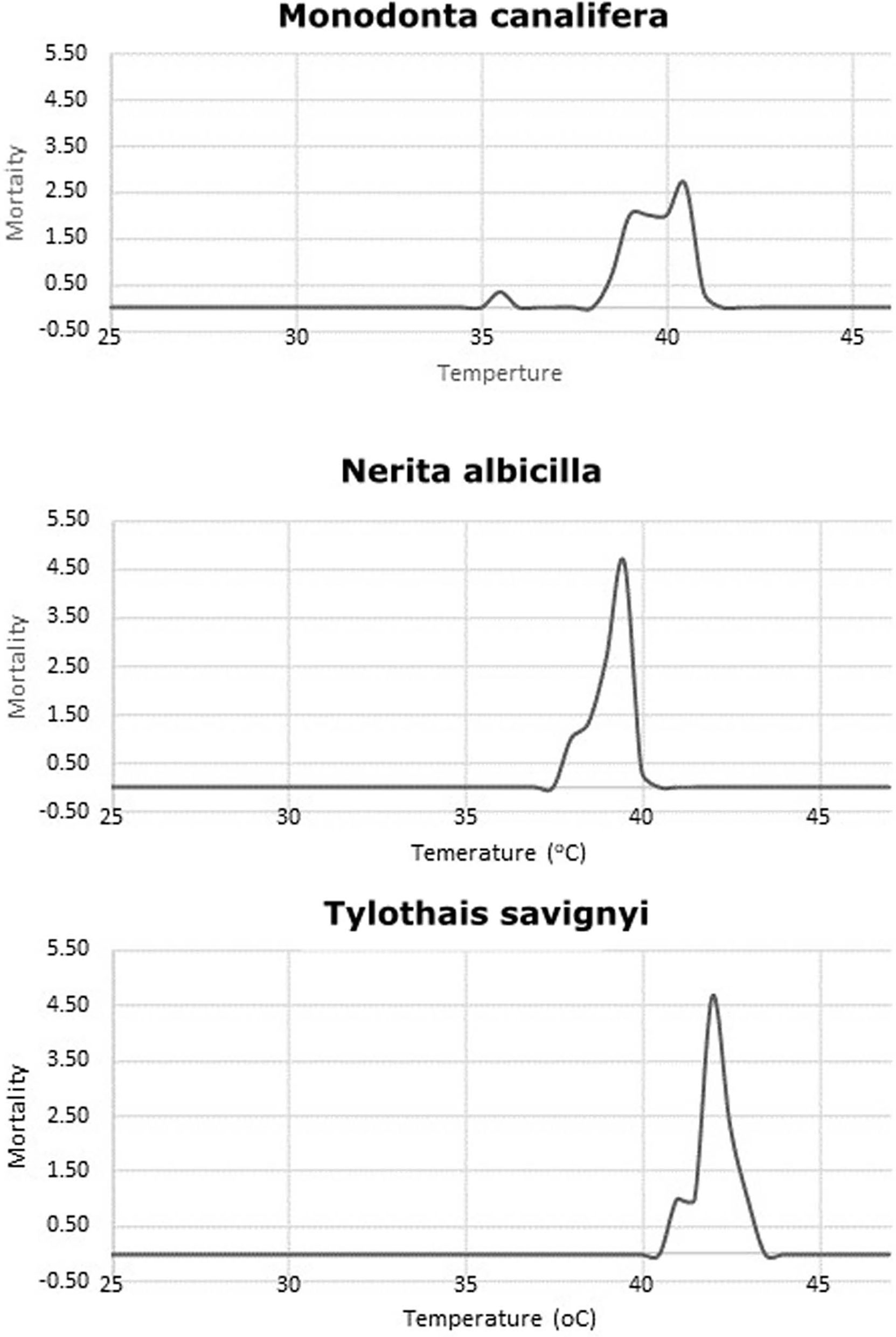
Figure 1 CTmax rise of temperature to determine the maximum critical temperature where the activities of organisms stop.
Lethal temperature (LT50 test)
In the laboratory, aquariums were set up (control = 24.9 ± 0.48, T1 = 30.6 ± 1.28, T2 = 35.3 ± 0.93,T3 = 40.3 ± 0.72, T4 = 44.5 ± 0.57), and all species were kept for 96 h, whereas LT50 and 95% confidence limits were estimated through trimmed Spearman–Karber analysis which showed that the thermal tolerance of N. albicella and T. savignyi increases significantly with increasing exposure duration. In the case of M. canalifera, the thermal tolerance inversely decreases with increasing exposure within 72 h (Figure 2). Linear regression was applied to test the relationship between body size and lethal temperature applied on three species of gastropods; it shows an inverse relationship between LT50 and body size (Figure 3). Linearity between lethal temperatures and median critical thermal maxima was calculated as R2 = 0.47, which defines a variation in CTmax to validate the efficacy of short-term estimates so as to predict long-term variation (Figure 4). The survival number of three gastropod species within 96 h of LT50 test indicates that the majority of individuals of N. albicella and T. savignyi endured various temperatures for up to 96 h, thus indicating their higher tolerance to elevated temperatures. However, individuals of M. canalifera survived with the lowest number at 96 h, indicating their sensitivity to warm water (Figure 5).
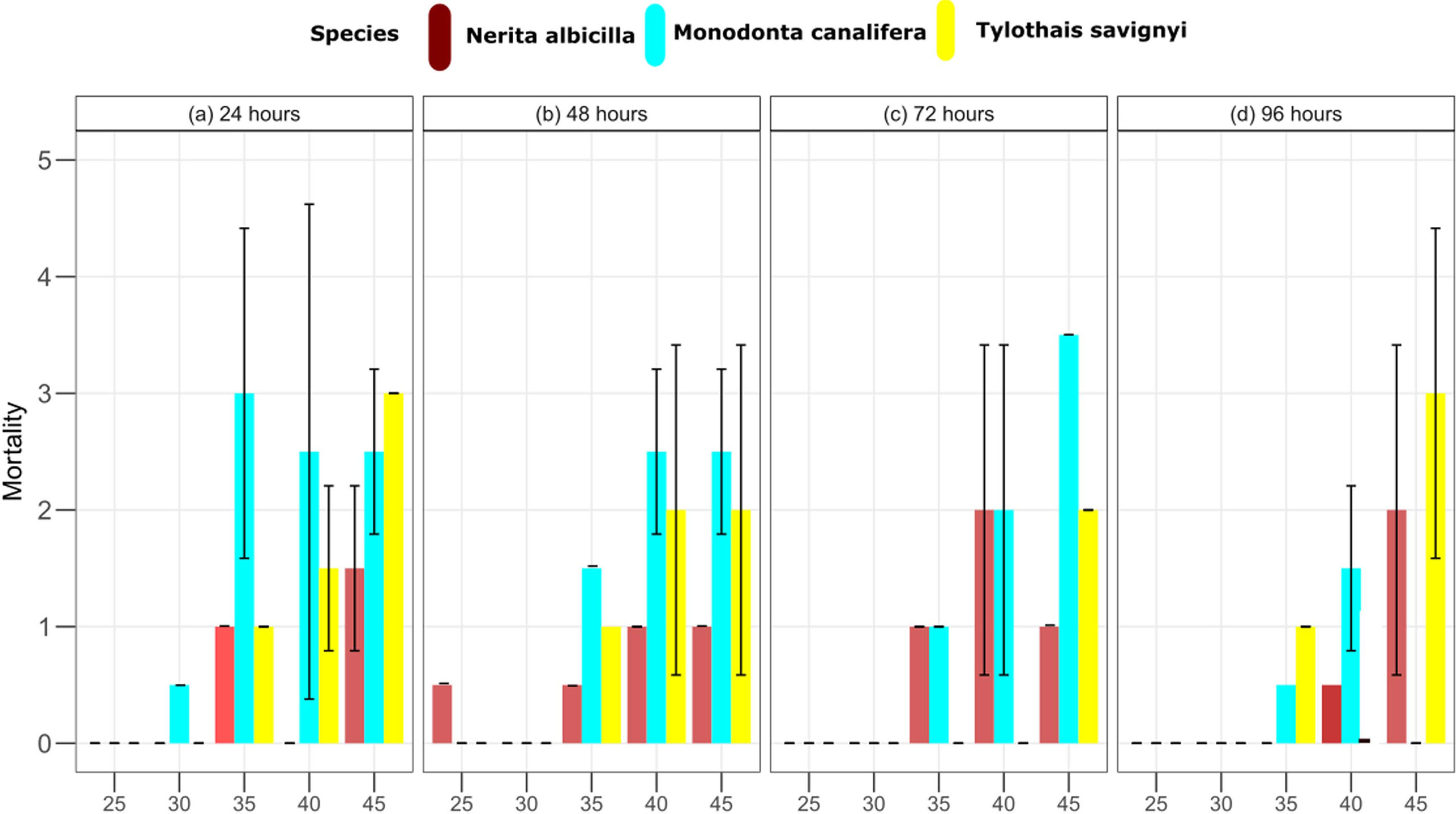
Figure 2 LT50 at constant temperatures of 25, 30, 35, 40, and 45°C at 24-, 48-, 72-, and 96-h duration.
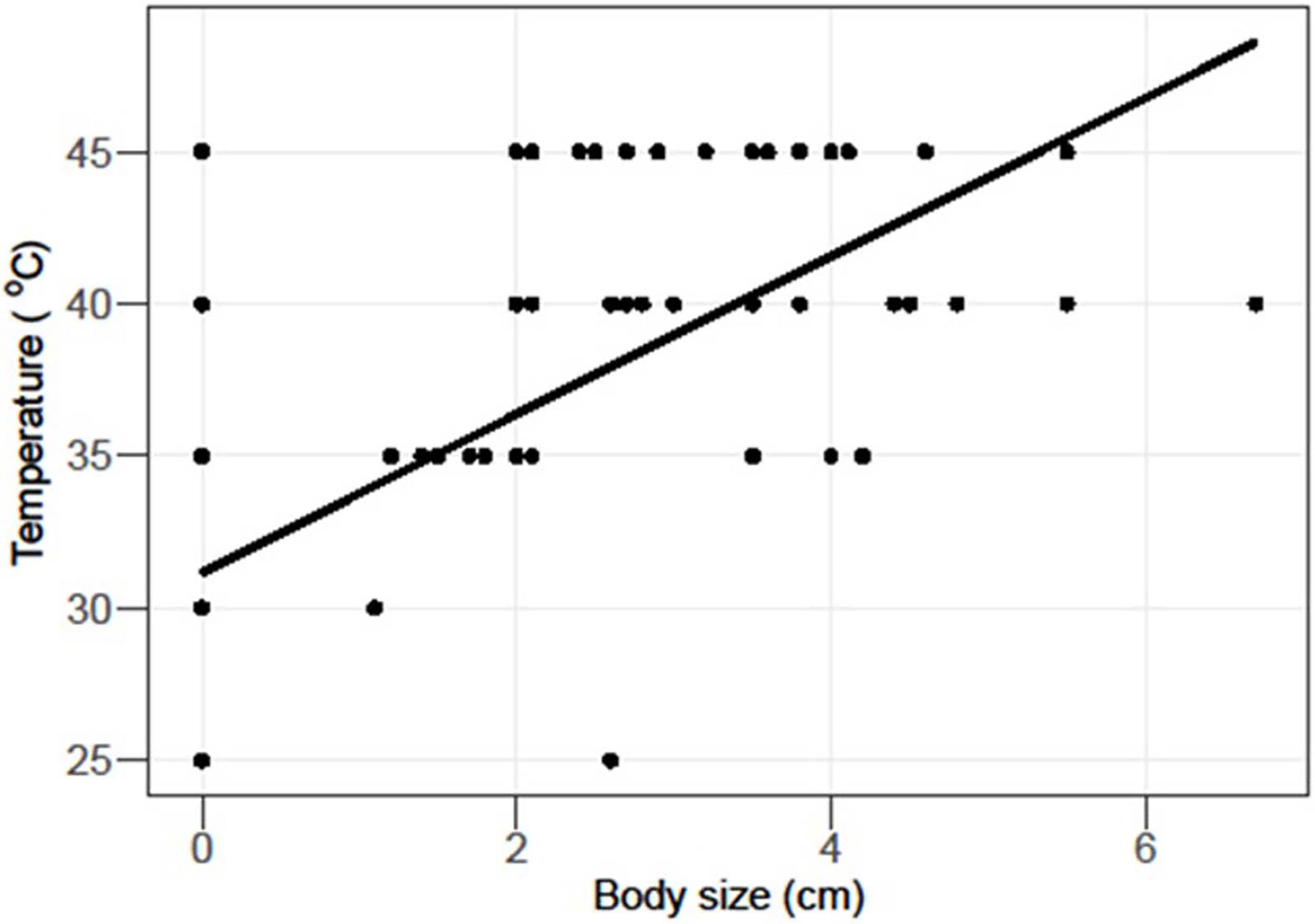
Figure 3 Linear regression applied to test LT50 and body sizes of Nerita albicilla, Tylothias savignyi and Monodonta canalifera. The coefficient of correlation (R) shows inverse relation between LT50 and body sizes.
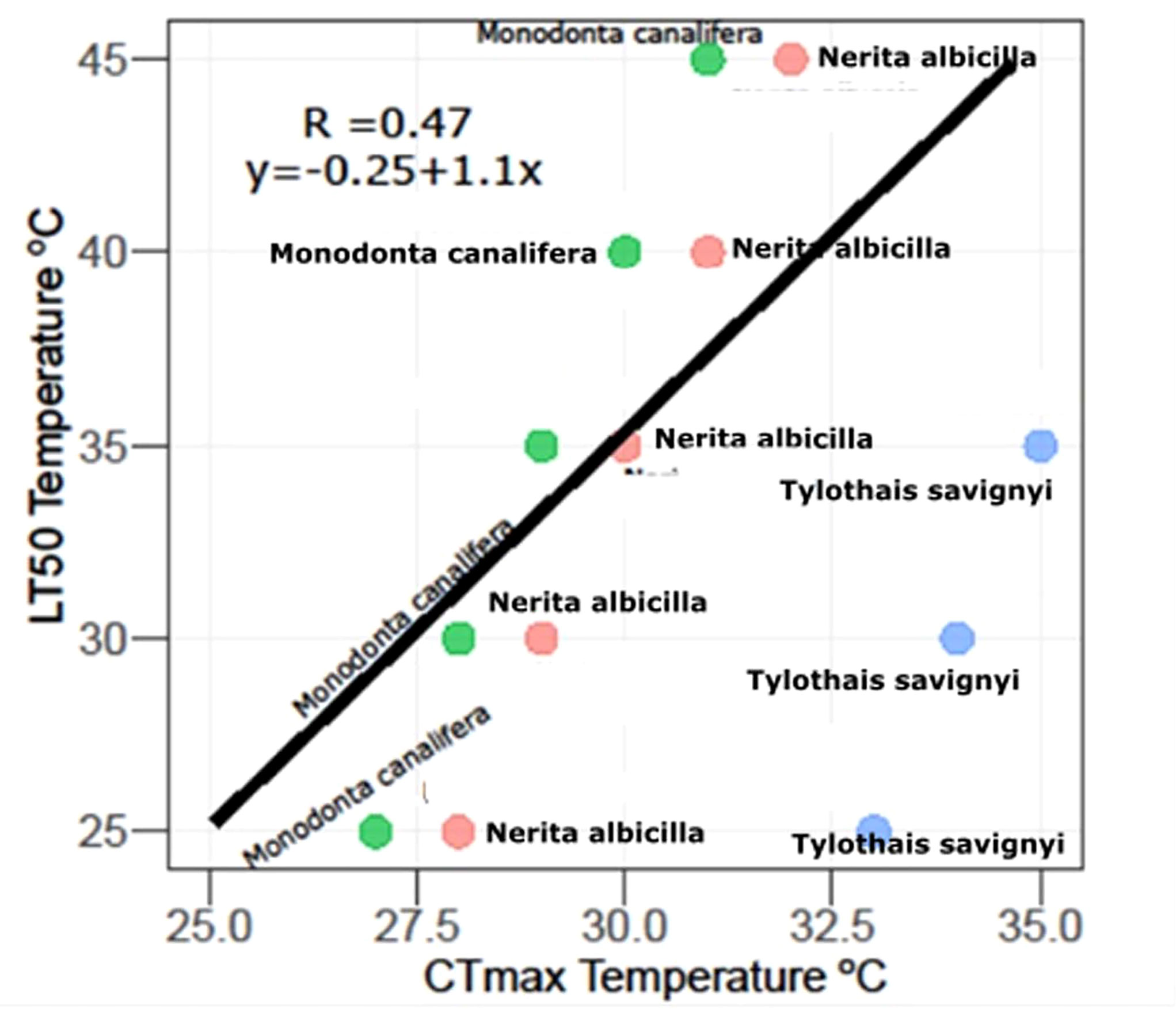
Figure 4 Relationship between L50 and CTmaxima statistically tested in three snails presented in this study.
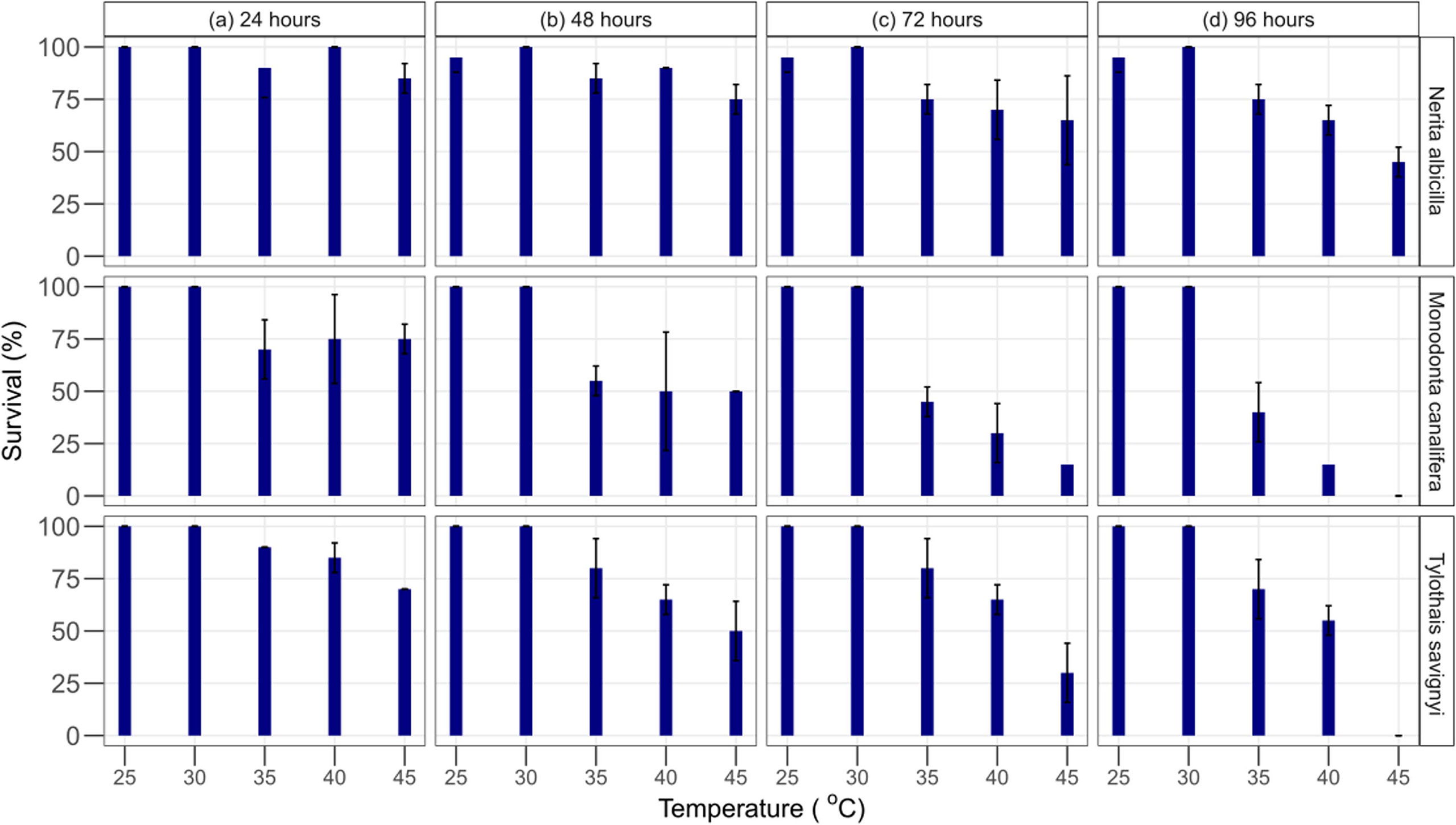
Figure 5 Survival % of Monodonta canalifera, Nerita albicilla, and Tylothias savignyi at constant temperatures of 25, 30, 35, 40, and 45°C at duration (A) 24 hours, (B) 48 hours, (C) 72 hours, (D) 96 hours.
Histopathological alterations in the foot
The foot tissue of control snails mainly contained columnar muscle cells, mucocytes, and normal epithelium. No histological alteration in the foot of three gastropod species was observed at 25°C (control) and 30°C (treatment I) (Figures 6A, B). These changes occurred at an exposure temperature of 35°C (treatment II) and became more adverse at exposure temperatures of 40°C (treatment III) and 45°C (treatment IV). The most conspicuous effects were observed in treatments II, III, and IV as deduced from a microscopic examination of the foot. When the temperature reached 40°C, the epithelium (outer covering) started rupturing, and the microvilli started to be denatured with deranged cilia. A further increase in temperature at 45°C resulted in eroded epithelium and scattered and deranged cilia with deformed microvilli. The eroded epithelium, disruption of columnar muscle fibers with gap between the cells, and empty and shrunken mucocytes can be seen (Figures 6C, D). The eroded epithelium and cilia (ci) formed spaces and gaps between the muscle fibers and deformed microvilli (Figure 6E). Comparison and validation of the cellular structure of the normal foot of organisms sampled from the intake and those taken from the treatments were conducted in the laboratory (Figure 6F).
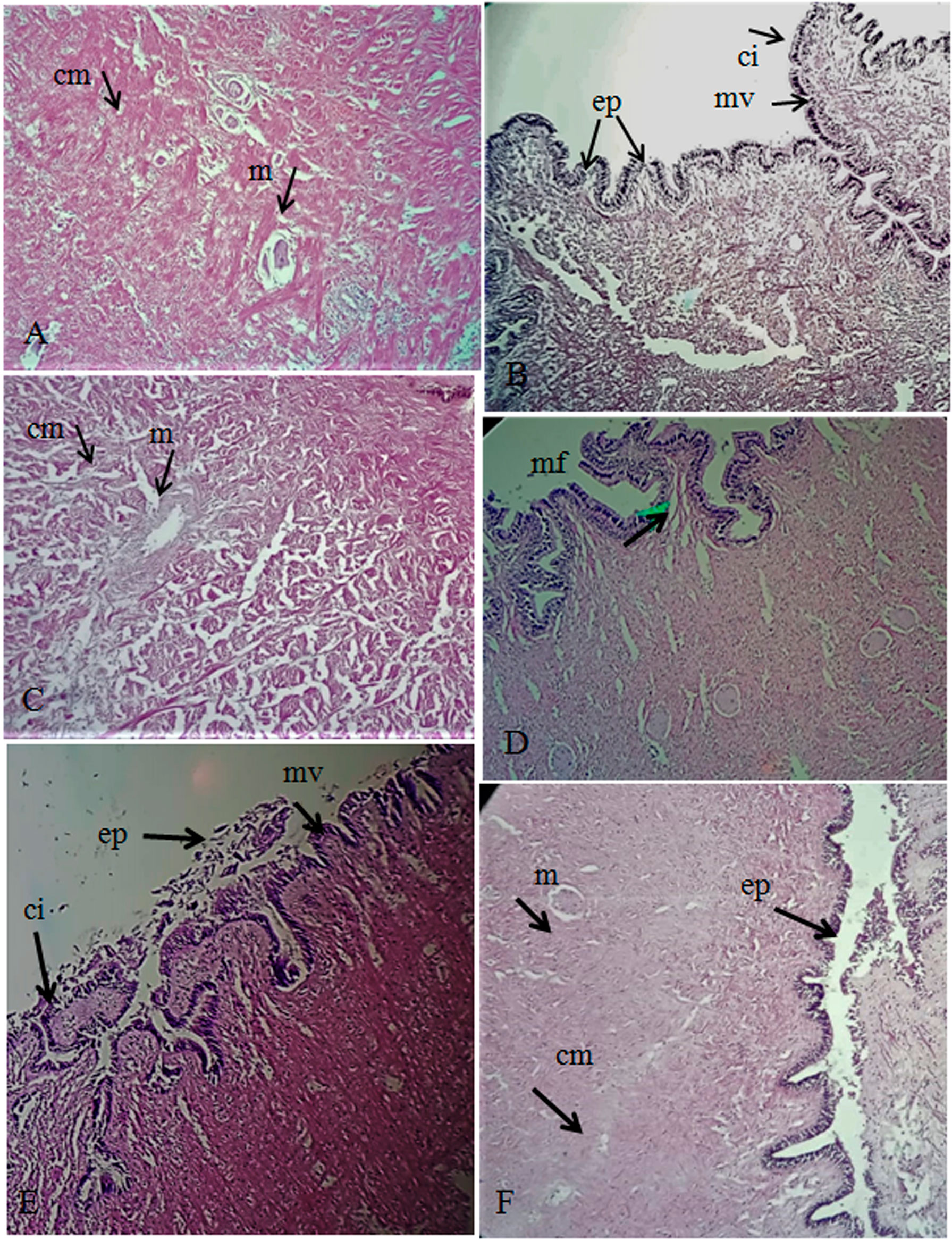
Figure 6 (A) Foot tissue (TS) of control snail showing normal columnar muscle cells (cm) and mucocytes (m). (B) Foot TS of control snail showing normal epithelium. (C) Foot TS of exposed snails showing emptied and shrinkage mucocytes (m) and deranged columnar muscle fibers (cm) with increased spaces in between them (arrow). (D) The epithelium (ep) was partially damaged, and the microvilli (mv) started to denature with deranged cilia. (E) The ep was eroded and the cilia (ci) scattered and deranged with deforming microvilli (mv). (F) Normal foot TS of snail from the intake structure of KANUPP, showing normal epithelium (ep), microvilli (mv), and columnar muscle fibers (cm).
Discussion
This study unveils temperature tolerance in sea snails inhabiting around Karachi Nuclear Power Units and the utmost level where they can survive, are vulnerable, or die in natural and captive environments. Three commonly occurring species showed a variable level of tolerance—for example, N. albicella and T. savignyi had a higher range of temperature, whereas M. canalifera was relatively much sensitive to the temperature. Moreover, it is obvious that coastal degradation can worsen with the increase in temperature by power units or other industrial units on the sea basin, which can cause damage to coastal ecosystems and make sessile organisms vulnerable around the outfall of the power plant. It is assumed that temperature is one of the major ecological factors since it influences the living region (Pörtner, 2001). Increased seawater temperature and its effects on marine biota have already gained a lot of attention and have become a notable issue when developing or planning a coastal power plant unit. Therefore, experiments on the capability of aquatic organisms, especially benthic species, are necessary to check their tolerance to elevated temperature in relation to their long-term management. CTM has proven to be a simple approach for assessing aquatic macro-invertebrates to the upper thermal limits because it requires minimum collection and experimental effort. The LT50 trials, on the other hand, took longer and required a larger number of test organisms to get consistent and reliable results. Its calculation also gives a longer-term understanding of the impact of increased water temperatures on the survival of aquatic macro-invertebrates (Dallas and Ketley, 2011). Therefore, in this study, we examined three different benthic species inhabiting near the intake and outfall of KNPC with both test CTmax and LT50 in order to check whether the occasionally released warm water from the outfall have some impacts on benthic organisms upon shorter and longer thermal exposure. Hence, the experiment revealed clear intraspecific differences among all three species: M. canalifera was the most thermally sensitive because its LT50 values progressively increased with time. Its mean thermal tolerance declined with time, in contrast to N. albicilla and Tylothias savignyi. Their LT50 values progressively decreased with time. These species are not sensitive to slowly change relative to the water temperature and are able to adopt to temperature changes with time. Our behavioral observation revealed that M. canalifera showed an adverse reaction upon continuous heating by losing its operculum. However, N. albicella and T. savignyi also showed an instantaneous reaction to heating by detachment from the base and walls of the aquarium and also displayed foot expansion. The CTmax results show the lowest thermal tolerance of Nerita albicella in comparison to M. canalifera and T. savignyi. In contrast to the 96-h temperature exposure, we can say that N. albicella has a lower ability to recover from temporary thermal induction, as its behavior in rapid temperature change showed unconsciousness, although soon after the end of the experiment, it was back to its normal state and started to attach to the wall of the aquarium. Hence, it is assumed that the difference of the results in both tests is due to exposure period. Further studies are still needed on the time limitation of thermal limits for different gastropods if we wish to assess the impact of extreme events (e.g., heat exposure) on different populations. The correlation of the LT50 data with the CTmax in Figure 4 indicates that temperature stress may be important in determining the distributions of several of the species investigated (Figure 2). In particular, the maximum recorded temperatures exceeded the 96-h LT50 values of M. canalifera. High temperature is more likely to be an important factor limiting lotic invertebrates in the water body near KANUPP. The behavioral observation during the overall experiment depicted snails as susceptible to drying up during action rather than pulling back into their shell and staying idle at high and possibly distressing surrounding temperatures. Subsequently, they can endure a critical range of temperatures when inactive (Marshall and McQuaid, 2011; Marshall et al., 2011), but it may lead to predation and limiting their distribution in the wild, ultimately threatening their life. The analysis of histopathological alteration offers information about the general health status of this species because it reflects cellular and subcellular morphological changes. There is evidence that thermal induction causes histological alteration in the foot of the tested snails. The atrophic tubules were rarely observed in lower temperature exposures, i.e., 25–30°C; however, atrophy gradually increased, even showing necrosis after higher thermal exposures at 35, 40, and 45°C. These alterations affect the mucus-secreting cells, leading to loss of mobility. On further exposure, a final consequence of this impairment is leading the snail to death. Generally, both the release of mucus and the hypertrophy of mucus cells seem to be involved in the adaptation of snails to extreme temperatures.
Various examinations on temperature influence due to coastal power plants have been recorded, such as Branett in 1972 who carried out studies on the outfall area of Hunterston Power Plant and suggested that the gastropods’ growth is disturbed and that spawning time was advanced by 3 months as the water temperature is raised. Other investigations found that the majority of benthic organisms, including gastropods, were affected due to the high temperature near the outfall region of Brazil Nuclear Power Plant (Teixeira et al., 2012). Among gastropods, we found that most studies about thermal tolerance have been carried out with Nerita spp. The lethal temperature of N. albicilla is described to be at 50°C, with severe effects on enzymatic activity that ultimately results in loss of activity (Dong et al., 2018). Another research about the episodic exposure to extreme temperature for N. albicilla is described at 39.6°C (Waltham and Sheaves, 2020). The thermoregulation of Nerita atramentosa was also tested, and it describes the endpoint as 45°C (Caddy-Retalic et al., 2011). Hence, the mortality or disappearance of gastropods may occur if the temperature increments were beyond their adaptive ranges.
Conclusion
This study increases our understanding on how sessile organisms can recover from sudden heat shocks and that they already have the ability to cope with shorter thermal exposures but cannot survive longer thermal induction even for a few days. Besides this, thermal exposure is independent of the size of the organism that is vulnerable either to shorter or larger or to constant thermal exposure which may lead to death. Hence, it is clear that constant increases in water temperature caused by the outfall structure of the power plant can be lethal to slow-moving organisms. The LT50 and CTmax test demonstrate that the temperature tolerance in two species remained at around 45°C for N. albicilla and T. savignyi, whereas M. canalifera can endure >45°C on constant exposure that may lead to an assumption of whether populations can possibly increase or become extinct. Moreover, the histological study of the foot demonstrates cellular atrophy and necrosis in the three species of sea snail.
Data availability statement
The raw data supporting the conclusions of this article will be made available by the authors without undue reservation.
Author contributions
WS conducted the field and laboratory exercises. SKP designed and supervised research, and finalized the manuscript. NY helped in improving the quality of the manuscript. All authors contributed to the article and approved the submitted version.
Acknowledgments
This research work was financially supported by PAEC/PMDC-West projects and a part of the PhD dissertation of WS. This part of research is dedicated to late Dr. Azhar Mashiatullah, Ex-Head, Isotope Division, PINSTECH-Islamabad, for his encouragements, affection, and care that will always be kept in our hearts: “love you, Sir”.
Conflict of interest
The authors declare that the research was conducted in the absence of any commercial or financial relationships that could be construed as a potential conflict of interest.
Publisher’s note
All claims expressed in this article are solely those of the authors and do not necessarily represent those of their affiliated organizations, or those of the publisher, the editors and the reviewers. Any product that may be evaluated in this article, or claim that may be made by its manufacturer, is not guaranteed or endorsed by the publisher.
References
Bamber R. N., Spencer J. F. (1984). The benthos of a coastal power station thermal discharge canal. J. Mar. Biol. Assoc. United Kingdom. 64 (3), 603–623. doi: 10.1017/S0025315400030290
Brockington S., Clarke A. (2001). The relative influuence of temperature and food on the metabolism of a marine invertebrates. J. Exp. Mar. Biol. Ecol. 258, 87–99. doi: 10.1016/S0022-0981(00)00347-6
Byrne M., Przeslawski R. (2013). Multistressor impacts of warming and acidification of the ocean on marine invertebrates. Life histories Integr. Comp. Biol. 53 (3), 582–596. doi: 10.1093/icb/ict049
Caddy-Retalic S., Benkendorff1 K., Fairweather P. G. (2011). Visualizing hotspots: applying thermal imaging to monitor internal temperatures in intertidal gastropods. Molluscan Res. 31 (2), 106–113. ISSN 1323-5818.
Dallas H. F., Ketley Z. A. (2011). Upper thermal limits of aquatic macroinvertebrates: Comparing critical thermal maxima with 96-LT50 values. J. Thermal Biol. 36 (6), 322–327. doi: 10.1007/s10750-011-0856-4
Dallas H. F., Rivers Moore N. A. (2012). Critical thermal maxima of aquatic macroinvertebrates: towards identifying bioindicators of thermal alteration. Hydrobiologia 679 (1), 61–76. doi: 10.1007/s10750-011-0856-4
Deshayes G. P., Milne-Edwards H. (1844). Histoire naturelle des animaux sans vertèbres présentant les caractères généraux et particuliers de ces animaux leur distribution leurs classes leurs familles leurs genres et la citation des principales espèces qui s'y rapportent, par J. B. P. A. de Lamarck. Deuxième édition, Tome dixième. Histoire des Mollusques. Baillière J. B.: Paris. 638 pp. Available at: http://www.biodiversitylibrary.org/item/64020
Diaz F., Salas A., Re A. D., Gonzalez M., Reyes I. (2011). Thermal preference and tolerance of Megastrea (Lithopoma) undosa (Wood 1828; Gastropoda: turbinidae). J. Thermal Biol. 36 (1), 34–37. doi: 10.1016/j.jtherbio.2010.10.004
Dittbrenner N., Lazzara R., Köhler H. R., Mazzia C., Capowiez Y., Triebskorn R. (2009). Heat tolerance in Mediterranean land snails: histopathology after exposure to different temperature regimes. J. Molluscan Stud. 75 (1), 9–18. doi: 10.1093/mollus/eyn033
Dong Y. W., Liao M. L., Meng X. L., Somero G. N. (2018). Structural flexibility and protein adaptation to temperature: Molecular dynamics analysis of malate dehydrogenases of marine molluscs. Proc. Natl. Acad. Sci. 115 (6), 1274–1279. doi: 10.1073/pnas.1718910115
Eriksen C. (1964). Evidence of a spring rise in metabolic rate in the burrowing mayfluy ephemera simulans walker. Hydrobiologia 23, 506–510. doi: 10.1073/pnas.1718910115
Farooq N., Qamar N., Panhwar S. K. (2017). Characterization of feeding habits, prey diversity and diet overlap of two sympatric species: Bronze catfish, Netuma bilineata (Valenciennes 1840) and blacktip sea catfish, Plicofollis dussumieri (Valenciennes 1840) in the northern Arabian Sea. J. Appl. Ichthyology. 33, 709–719. doi: 10.1111/jai.13377
Fraenkel G. S. (1968). The heat resistance of intertidal snails at bimini, bahamas; ocean springs, mississippi; and woods hole, Massachusetts. Physiol. Zoology. 41, 1–13. doi: 10.1086/physzool.41.1.30158481
Ganser A. M., Newton T. J., Haro R. J. (2015). Efects of elevated water temperature on physiological responses in adult freshwater mussels. Freshw. Biol. 60, 1705–1716. doi: 10.1111/fwb.12603
Green T. J., Montagnani C., Benkendor K., Robinson N. (2014). Ontogeny and water temperature influuences the antiviral response of the pacific oyster, crassostrea gigas. Fish Shellfish Immunol. 36 (1), 151–157. doi: 10.1016/j.fsi.2013.10.026
Helmuth B. (2002). How do we measure the environment? linking intertidal thermal physiology and ecology through biophysics. Integr. Comp. Biol. 42 (4), 837–845. doi: 10.1093/icb/42.4.837
Helmuth B., Harley C. D., Halpin P. M., O'Donnell M., Hofmann G. E., Blanchette C. A. (2002). Climate change and latitudinal patterns of intertidal thermal stress. Science 298 (5595), 1015–1017. doi: 10.1126/science.1076814
Hoffmeyer M. S., Biancalana F., Berasategui A. (2005). Impact of a power plant cooling system on copepod and meroplankton survival (Bahía blanca estuary, Argentina). Iheringia Série Zoologia. 95 (3), 311–318. doi: 10.1590/S0073-47212005000300011
IPCC (2007). “Climate change: the physical science basis. contribution of working group I to the fourth assessment,” in Report of the intergovernmental panel on climate change. Eds. Solomon S., Qin D., Manning M., Chen Z., Marquis M., Averyt K. B., Tignor M., Miller H. I. (Cambridge, United Kingdom and New York, NY, USA: Cambridge University Press), 996.
Jiang Z., Zeng J., Chen Q., Huang Y., Xu X., Liao Y., et al. (2008). Tolerance of copepods to short-term thermal stress caused by coastal power stations. J. Thermal Biol. 33 (7), 419–423. doi: 10.1016/j.jtherbio.2008.06.008
Kishi D., Murakami M., Nakano S., Maekawa K. (2005). Water temperature determines strength of top down control in a stream food web. Freshw. Biol. 50 (8), 1315–1322. doi: 10.1111/j.1365-2427.2005.01404
Lamarck J. B. (1816). Liste des objets représentés dans les planches de cette livraison. In: Tableau encyclopédique et méthodique des trois règnes de la Nature. Mollusques et Polypes divers. Agasse, Paris.16 pp.
Linnaeus C. (1758). Systema Naturae per regna tria naturae, secundum classes, ordines, genera, species, cum characteribus, differentiis, synonymis, locis. Editio decima, reformata [10th revised edition], vol. 1:824 pp. Laurentius Salvius: Holmiae. Available at: https://biodiversitylibrary.org/page/726886
Mairaj M., Panhwar S. K., Qamar N., Rashid S. (2021). Indus river estuary: an assessment of potential risk of contaminants and ecosystem susceptibility. SN Appl. Science. 3, 730. doi: 10.1007/s42452-021-04721-2
Markel R. P. (1971). Temperature relations in two species of tropical western American littorines. Ecology 52 (6), 1126–1130. doi: 10.2307/1933823
Marshall D. J., Dong Y. W., McQuaid C. D., Williams G. A. (2011). Thermal adaptation in intertidal snail echinolittorina malaccana contradicts current theory by revealing the crucial roles of resting metabolism. J. Exp. Biol. 214 (27), 3649–3657. doi: 10.1242/jeb.059899
Mckie B.,. G., Cranston P. S., Pearson R. G. (2004). Gondwanan mesotherms and cosmopolitan eurytherms: Effects of temperature on the development and survival of Australian chironomidae (Diptera) from tropical and temperate populations. Mar. Freshw. Res. 55 (8), 759–767. doi: 10.1071/MF04023
Mora C., Ospina A. (2001). Tolerance to high temperatures and potential impact of sea warming on reef fishes of gorgona island (tropical eastern pacific). Mar. Biol. 139 (4), 765–769. doi: 10.1007/s002270100626
O'Connor M. I., Bruno J. F., Gaines S. D., Halpern B. S., Lester S. E., Kinlan B. P. (2007). Temperature control of larval dispersal and the implications for marine ecology, evolution, and conservation. Proc. Natl. Acad. Sci. U.S.A. 104 (4), 1266–1271. doi: 10.1073/pnas.0603422104
Pavlasek J. (1950). Morfologie a biologie hlemyzde zahradniho (Helix pomatia L.) vzhledem k potravinarstvi. Disertacni prace, VSV Brno, 120s.
Poornima E. H., Rajaduraia M., Rao V. N. R., Narasimhan S. V., Venugopalan V. P. (2006). Use of coastal waters as condenser coolant in electric power plants: Impaction on phytoplankton and primary productivity. J. Thermal Biol. 31 (7), 556–564. doi: 10.1016/j.jtherbio.2006.05.009
Pörtner H. (2001). Climate change and temperature-dependent biogeography: oxygen limitation of thermal tolerance in animals. Naturwissenschaften 88 (4), 137–146. doi: 10.1007/s001140100216
Rana A. S., Zaman Q., Afzal M., Haroon M. A. (2014). Characteristics of sea surface temperature of the Arabian Sea coast of Pakistan and impact of tropical cyclones on SST. Pakistan J. Meteorology. 11 (21), 61–70.
Sandison E. E. (1967). Respiratory response to temperature and temperature tolerance of some intertidal gastropods. J. Exp. Mar. Biol. Ecology. 1 (2), 271–281. doi: 10.1016/0022-0981(67)90019-6
Teixeira T. P., Neves L. M., Araújo F. G. (2012). Thermal impact of a nuclear power plant in a coastal area in Southeastern Brazil: Effects of heating and physical structure on benthic cover and fish communities. Hydrobiologia. 684 (1), 161–175. doi: 10.1007/s10750-011-0980-1
Tropea C., Stumpf L., Lopez Greco L. S. (2015). Effect of temperature on biochemical composition, growth and reproduction of the ornamental red cherry shrimp neocaridina heteropoda (Decapoda, caridea). Public Library Sci. One 10 (3), e0119468. doi: 10.1371/journal.pone.0119468
Vannote R., Sweeney B. (1980). Geographic analysis of thermal equilibria: A conceptual model for evaluating the effect of natural and modified thermal regimes on aquatic insect communities. Am. Naturalist. 115, 667–695. doi: 10.1086/283591
Waltham N. J., Sheaves M. (2020). Thermal exposure risks to mobile tropical marine snails: Are eco-engineered rock pools on seawalls scale-specific enough for comprehensive biodiversity outcome? Mar. pollut. Bulletin. 156, 111237. doi: 10.1016/j.marpolbul.2020.111237
Warwick R. M. (1993). Environmental impact studies on marine communities: pragmatical considerations. Aust. J. Ecology. 18, 63–80. doi: 10.1111/j.1442-9993.1993.tb00435
Webb B. W., Hannah D. M., Moore R. D., Brown L. E., Nobilis F. (2008). Recent advances in stream and river temperature research. Hydrological Processes: Int. J. 22 (7), 902–918. doi: 10.1007/s10750-008-93
Keywords: sea snails, thermal tolerance, foot histology, outfall structure, nuclear power units Karachi
Citation: Shaikh W, Panhwar SK and Yaqoob N (2022) Thermal tolerance and critical maxima examined in marine gastropods inhabiting around Karachi Nuclear Power Plant. Front. Mar. Sci. 9:1006551. doi: 10.3389/fmars.2022.1006551
Received: 29 July 2022; Accepted: 16 September 2022;
Published: 07 October 2022.
Edited by:
Kaizhi Li, South China Sea Institute of Oceanology (CAS), ChinaReviewed by:
Zeng Jiangning, Ministry of Natural Resources, ChinaYang-Guang Gu, South China Sea Fisheries Research Institute (CAFS), China
Copyright © 2022 Shaikh, Panhwar and Yaqoob. This is an open-access article distributed under the terms of the Creative Commons Attribution License (CC BY). The use, distribution or reproduction in other forums is permitted, provided the original author(s) and the copyright owner(s) are credited and that the original publication in this journal is cited, in accordance with accepted academic practice. No use, distribution or reproduction is permitted which does not comply with these terms.
*Correspondence: Sher Khan Panhwar, sk.panhwar@uok.edu.pk