Stable Isotope Analysis Revealed Ontogenetic Changes in Trophic Ecology and Migration Patterns of Sepia esculenta in the Northern Coastal Waters of China
- 1The Key Laboratory of Mariculture, Ministry of Education, Ocean University of China, Qingdao, China
- 2Fisheries College, Zhejiang Ocean University, Zhoushan, China
- 3Laboratory for Marine Fisheries Science and Food Production Processes, Qingdao National Laboratory for Marine Science and Technology, Qingdao, China
Golden cuttlefish (Sepia esculenta), the most economically important cephalopod in the northern coastal seas of China, had experienced greatly reduced population biomass due to continuous fishing pressure in recent decades. Understanding the trophic ecology and clarifying the spatial distribution of wintering ground can help develop management plans for this species. In this study, the ontogenetic changes in the trophic ecology of S. esculenta in the Qingdao coastal water were determined, and the migration patterns were studied using stable isotope analysis. Cluster analysis based on isotopic values divided S. esculenta with different lengths into groups: 11–20, 21–100, and 121–200 mm. A significant difference in the δ13C values between the groups 11–20 mm (−17.10‰) and 21–100 mm (−15.89‰) illustrates an ontogenetic change in the feeding habits. Due to the migratory habits of S. esculenta, the δ13C value of the group 121–200 mm (−16.39‰) was lower than that of the group 21–100 mm. The δ15N values of S. esculenta were found to increase in length, and there was a clear linear relationship between different S. esculenta groups, suggesting that the wintering ground may locate in the same latitude as the spawning ground (i.e., the middle Yellow Sea). Furthermore, the trophic relationship between S. esculenta and coexisting species was assessed, revealing that the group 11–20 mm of S. esculenta has some overlap of carbon isotope space with other species, suggesting that these species may feed on it as prey. Thus, slightly increasing the length to more than 20 mm may reduce the pressure of being the prey of post-release juveniles of S. esculenta and improve the effect of release.
Introduction
Sepia esculenta (Hoyle, 1885), also known as golden cuttlefish, is distributed in Chinese, South Korean, and Japanese coastal waters and has fixed migratory habits of pelagic wintering and inshore spawning (Zheng et al., 2004). In China, S. esculenta was the most economically important cephalopod in the northern coastal seas and was listed as one of the four major fisheries in the Bohai Sea and the Yellow Sea until the 1970s (Dong, 1991). Since then, the abundance of this species has been greatly reduced due to continuous fishing pressures and dwindling spawning fields (Hao et al., 2007). Due to this, the northern region of China has adopted a variety of resource conservation measures to restore the stock situation of S. esculenta. The release of artificially hatched juveniles has been considered a chief measure to compensate for this decline in S. esculenta, and the number of releases is increasing year by year (Zhang et al., 2019). In addition, S. esculenta lays sinking eggs, so they wrap their fertilized eggs around the egg-attaching matrix for hatching. Therefore, it is also an important means to protect its resources by placing artificial spawning attachments into the sea during the breeding period of S. esculenta (Niu et al., 2017). At present, the catches and resource status of S. esculenta are slowly recovering under the joint action of multiple resource conservation measures (Zhang et al., 2019).
Understanding the diets and trophic ecology of species can help to promote ecological knowledge and develop management plans for commercial species (Zhang et al., 2019); however, there have been no such investigations of S. esculenta. Unlike most fishes, which swallow prey whole, cephalopods tear food into small pieces, making it more difficult to identify the prey species and hindering the applicability of the traditional, intuitive method of stomach contents (Cherel and Hobson, 2005). This may be the main hindrance to conducting relevant research of S. esculenta. In addition, spawning grounds and wintering grounds are indispensable and important areas in the life cycle of migratory species, so it is of great significance to clarify their spatial distribution and strengthen management to maintain the structure and quantity of fishery stocks (Li et al., 2018). The S. esculenta spawning off the coast of China can be divided into two geographical populations: one spawn in Zhoushan-Yangtze River estuary and the other spawn in the southeastern coastal waters of Shandong Province (Wang et al., 2019), but the wintering grounds of both populations are not yet clear.
The stable isotopes of carbon (δ13C) and nitrogen (δ15N) can reflect the long-term dietary sources, and trophic levels of predators provide powerful tools for researching the trophic ecology of species (Peterson and Fry, 1987; Layman et al., 2012). The carbon isotopic signatures of predators are slightly enriched at a small level compared with those of their prey and are thus used to provide references to the feeding habits and dietary compositions of consumers (McCutchan et al., 2003). In contrast, the nitrogen isotopic signatures of organisms are normally used to estimate trophic levels because there is a greater enrichment in consumers over their prey (Fry, 1988; Zanden and Rasmussen, 2001). Through isotopic analysis, the trophic ecology has been investigated for many cephalopods including Ommastrephes bartramii and Sthenoteuthis oualaniensis (Parry, 2008), Architeuthis dux (Guerra et al., 2010), Sepia officinalis (Hobson and Cherel, 2006), Spirula spirula (Ohkouchi et al., 2013), and Doryteuthis gahi (Rosas-Luis et al., 2017). In addition, stable isotope analysis has also been used to study the migratory patterns of cephalopods due to the difference in stable isotopic signatures in different marine ecosystems (e.g., the relative abundance of 13C in coastal food webs is typically higher than that in oceanic food webs), such as Dosidicus gigas (Argüelles et al., 2012; Trasviña-Carrillo et al., 2018), S. officinalis (Rooker et al., 2020), and Illex argentinus (Rosas-Luis et al., 2017; Queirós et al., 2019).
Qingdao is one of the main spawning grounds of the S. esculenta population along the southeastern coastal waters of Shandong Province and is also one of the main areas of the S. esculenta proliferation and conservation in northern China (Zhang et al., 2019). In this study, the ontogenetic changes in the trophic ecology of S. esculenta in the coastal waters of Qingdao were determined using carbon and nitrogen stable isotope analysis, and the trophic relationships between S. esculenta and other species were further clarified. In addition, the migration pattern of the S. esculenta population in this area was studied, and the spatial distribution of its wintering ground was speculated. The purpose of this study is to enrich the basic biological knowledge of S. esculenta and to provide references for resource management.
Materials and Methods
Sample Collection
The study area (water depth range: 25–30 m) is located in the coastal waters of Qingdao, in the western Yellow Sea (Figure 1). Juvenile and immature individuals (mantle length: 11--100 mm) of S. esculenta were collected using a trawl net of a commercial fishing vessel in September 2017. Other coexisting species were also extensively caught by the trawl net and were identified to the lowest taxonomic level. These species contain crustaceans, cephalopods, and teleosteans. Considering the diversity of teleosteans species, they were further subdivided into mesodemersal and demersal fishes based on their ecological habits such as the distribution of the water layer (excerpted from Fishbase1). Zooplankton was collected by oblique towing with the net mesh size of 300 μm at the same station to represent baseline species in this study. Adult S. esculenta (mantle length: 120–200 mm) were collected in June 2018, which fell into the closed fishing period in the Yellow Sea. Therefore, S. esculenta adults were captured using cages. The mantle length of the S. esculenta specimens ranged from 11 to 200 mm, which essentially covered the size range observed throughout its life cycle, with the maximum mantle length of about 200 mm (Natsukari and Tashiro, 1991). Due to the mesh size limitations of the sample nets, we did not capture S. esculenta larvae less than 10 mm in mantle length. The mantle length of newborn S. esculenta was 4–5 mm, with egg yolk carried in the mouth, which was the main nutritional source before starting prey (Wang et al., 2018). In the earliest stages of a species, they initially carry stable isotopic traits from their mothers for some time, especially those that feed on the yolk after hatching (Rudolf, 2020). Therefore, the introduction of S. esculenta larvae less than 10 mm will not help to increase the integrity of this study. All the samples were carefully stored at −20°C until laboratory processing.
Sample Treatment
Details on sample treatment and analysis were provided in a study by Newsome et al. (2004). The length and weight of all the specimens were measured to the nearest 0.1 mm and 0.1 g, respectively, and the S. esculenta samples were classified at mantle length intervals of 10 mm. For the stable isotope analyses, chitinous beaks and soft tissues, such as dorsal muscle and wrist, have typically been applied as research materials in trophic ecology studies of cephalopods (Parry, 2008; Guerra et al., 2010; Ohkouchi et al., 2013; Guerreiro et al., 2015; Rosas-Luis et al., 2017; Trasviña-Carrillo et al., 2018; Golikov et al., 2019; Urrutia-Olvera et al., 2021), but there were significant differences in isotopic ratios among various tissues (Cherel et al., 2009a). These tiny differentiae could bias the results of experiments (McCutchan et al., 2003), especially in dietary research of consumers based on the δ13C values. Consequently, pairwise comparisons of the stable isotopic values between tissues (muscle tissue of the wrists and wings of the later beaks) from the same S. esculenta individuals were performed before the formal experiment in this study. Then, a small section of muscle tissue was sampled from the wrist of the S. esculenta and other cephalopods, together with dorsal muscles, abdominal muscles, and claw muscles for fishes, shrimps, and crabs, respectively. When available, six individuals from each species were randomly selected for isotopic determination. Before the analyses, the samples were fully dried to a constant weight in a drying oven at 65°C and then ground to a homogeneous powder with a quartz mortar, after which lipids were extracted from the powdered samples with a mixture of 2:1 chloroform:methanol (Folch et al., 1957). The processed samples were subsequently dried and ground again and preserved in a low temperature and dry environment for stable isotope determination.
Stable Isotope Analysis
The stable isotopic ratios of aquatic species were analyzed using a Flash EA 1112 Elemental analyzer coupled with a Thermo Finnigan MAT 253 stable isotope ratio mass Spectrometers (EA-IRMS) (Thermo Finnigan Scientific, Inc., United States) at the Qingdao Institute of Marine Geology, and the obtained results were presented in the conventional δ notation relative to international standard materials (Vienna Pee Dee Belemnite for carbon and atmospheric N2 for nitrogen), which is calculated as follows: X(%) = (Rsample/Rstandard−1)×103, where X is 13C or 15N and R is 13C/12C or 15N/14N. The quality control for the samples was performed after measuring each ten samples by interspacing the standard reference materials with known elemental composition and isotope ratios (UREA, δ13C: −8.02 ± 0.05%, δ15N: 20.17 ± 0.06%; B2151, δ13C: −26.27 ± 0.15%, δ15N: 4.42 ± 0.29%; EDTA, δ13C: −40.23 ± 0.01%, δ15N: −0.73 ± 0.09%). All the samples were analyzed in a continuous flow mode.
The trophic levels of S. esculenta were estimated using the method described by Post (2002): TL = (δ15Nsample−δ15Nbaseline)/Δδ15N + TLbaseline, where δ15N is the stable isotopic ratio (15N/14N) of the specimens and baseline organisms, which were measured directly using the mass spectrometer, and Δ15N is the enrichment in δ15N per trophic level, which is obtained from published data (3.4‰, DeNiro and Epstein, 1981; Post, 2002). TLbaseline is the trophic level of the baseline organism (zooplankton in this study) and was given a value of 2 in this study.
Statistical Analysis
The S. esculenta samples with different measurements were grouped using cluster analysis. Their δ13C and δ15N values were used to distinguish the feeding habits of different developmental stages. Since all the S. esculenta samples were presented in the form of δ13C − δ15N biplots based on the cluster analysis results, the size-corrected Bayesian standardized ellipse areas (SEAc) were plotted using SIBER (Jackson et al., 2011) to represent the isotopic niche and trophic breadth of different S. esculenta groups. The isotopic niche size of S. esculenta groups was calculated using the metrics of total areas encompassing all data points (TA), Bayesian standardized ellipse areas (SEA), and SEAc (Jackson et al., 2011). The overlap proportion between two SEAc was used as a proxy for trophic similarity and was calculated by estimating the extent of niche overlap between different S. esculenta groups. The percentage of overlap between S. esculenta groups was calculated by dividing the area of overlap (‰2) by the total combined SEAc (‰2) of the two groups being compared using SIBER (Jackson et al., 2011). After the normality and homogeneity of variance were examined using the Shapiro–Wilk test and Levene’s test, the assumption of correlations between δ13C and δ15N and S. esculenta mantle length were checked using a linear regression model. All the data were analyzed using R 4.1.1 software (R Development Core Team, 2020).
Results
Isotopic Values in Wrists and Beaks of Sepia esculenta
No significant variations were observed in the mean δ13C values (Mann–Whitney U-test, n = 7, P > 0.05) between muscle (−16.41 ± 0.29) and beaks (−16.26 ± 0.46), but the δ15N values of the muscle (9.64 ± 0.17) were significantly higher than those of beaks (7.11 ± 0.33) (Mann–Whitney U-test, P < 0.05).
Ontogenetic Changes in the Trophic Ecology of Sepia esculenta
The isotope values of the juvenile and immature S. esculenta (11–100 mm) ranged from −17.30 to −15.27‰ for δ13C and from 8.34 to 11.43‰ for δ15N. For adults (121–200 mm), the ranges of δ13C and δ15N were from −16.95 to −15.63 and 11.24 to 12.74‰, respectively (Supplementary Table 1). Cluster analysis was used to measure the square Euclidean distance of the δ13C and δ15N values of S. esculenta and to distinguish the feeding habits of individuals with different measurements, and the results illustrated that the S. esculenta samples were sorted into three distinct groups: group 11–20 mm, group 21–100 mm, and group 121–200 mm (Figure 2A). Isotopic niche size, represented by SEAc, was largest for group 21–100 mm (0.690), followed by group 121–200 mm (0.455) and group 11–20 mm (0.117) (Table 1). There was no niche overlap between group 11–20 mm and the other two groups, while a little niche overlap (7.3%) was found between the groups 21–100 and 121–200 mm (Figure 2B).
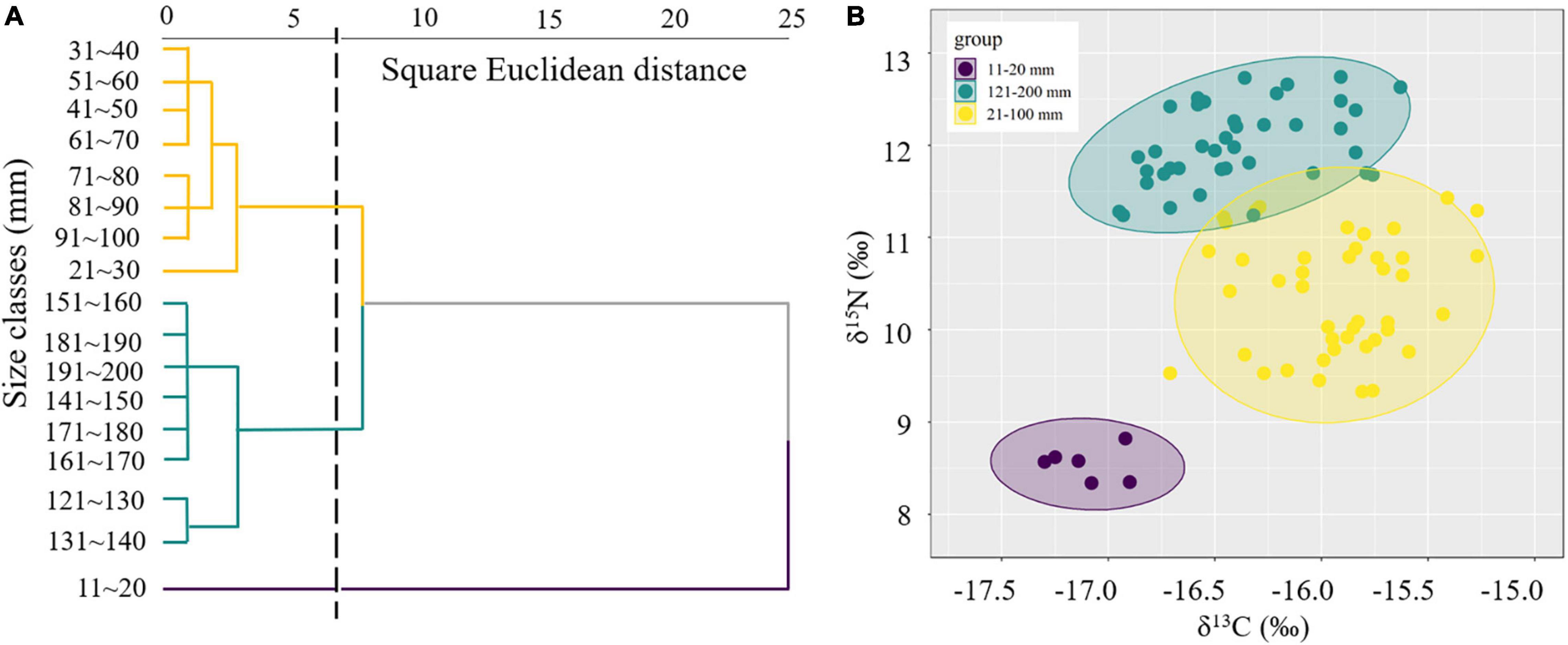
Figure 2. Cluster analysis of the isotopic values with different measurements (A) and size-corrected Bayesian standardized ellipse areas (SEAc) for each group (B) of S. esculenta.
Correlations Between the Isotopic Values and Mantle Length of Sepia esculenta
The changes in the δ13C and δ15N values associated with increasing S. esculenta mantle length were examined using regression analysis and are illustrated in Figure 3. There was no trend for the δ13C values with mantle length (P > 0.05). Thus, we fit the linear regression within the groups, respectively (Figure 3A). In the groups 11–20 and 121–200 mm, the δ13C values were increased with mantle length, while for group 21–100 mm, the δ13C values hardly changed with mantle length. In contrast, there was an increasing tendency in the δ15N values related to mantle length (P < 0.001, R2 = 0.87, Figure 3B).
Isotopic Information of Coexisting Species
In terms of the coexisting species, the δ13C values ranged from −19.24 ± 0.78‰ [red tongue sole (Cynoglossus joyneri) (Günther, 1878)] to −16.83 ± 0.15‰ [small yellow croaker (Larimichthys polyactis) (Bleeker, 1877)]. Forceps snapping shrimp (Alpheus distinguendus) (De Haan, 1844) had the lowest δ15N (9.75 ± 0.34‰) values, while Japanese sillago (Sillago japonica) (Temminck and Schlegel, 1843) had the highest (13.23‰) (Supplementary Table 2). The coexisting species were divided into four logical groups (mesodemersal fishes, demersal fishes, crustaceans, and cephalopods) based on their habitat relevance. Overall, most mesodemersal fishes had the δ13C values of approximately −17.5‰, most demersal fishes had the δ13C values of approximately −19.0‰, and most crustaceans had intermediate δ13C values, falling between those of the demersal and mesodemersal fishes, at approximately −18.5‰ (Figure 4).
Trophic Levels Estimates
Zooplankton was used as primary consumers to set the baseline, and their mean δ15N values (6.26 ± 1.28‰) were used to estimate the trophic levels of each species (Figure 5). The trophic levels of the main species in the Qingdao coastal food web could reach up to fourth Trophic level (TL), and most fishes, crustaceans, and cephalopods fell within the third and fourth TLs. In fishes, the TL was largest for S. japonica and lowest for red barracuda (Sphyraena pinguis) (Günther, 1874); Japanese squillid mantis shrimp (Oratosquilla oratoria) (De Haan, 1844) exhibited the highest TL in crustaceans and followed by Shiba shrimp (Metapenaeus joyneri) (Miers, 1880); The highest TL of cephalopod was found in group 121–200 mm of S. esculenta, with the highest TL of individuals within the group reaching 3.97 (Supplementary Table 2). Group 11–20 mm of S. esculenta was the lowest TL consumer other than zooplankton, with the trophic levels between the second and third TLs. With the ontogeny of S. esculenta, approximately 1.3 trophic levels experienced the difference between small and large individuals (Figure 5).
Discussion
The Use of Muscle in Isotopic Analysis for Sepia esculenta
In this study, the δ15N values of the muscle were significantly higher than those of beaks in S. esculenta, which is in accordance with the nitrogen signatures of the tissues in the Antarctic flying squid (Todarodes filippovae) (Adam, 1975), caused by the depletion of 15N compared with protein during chitin formation (Cherel and Hobson, 2005; Cherel et al., 2009a). Hence, the wrist muscle was used for the isotopic analyses in the subsequent process.
The Characteristics of Isotopic Changes With Ontogeny
For a more intuitive view of the feeding conversion process, cluster analysis based on isotopic signatures was used to interpret ontogenetic transition patterns in trophic niches of S. esculenta and divided the individuals into three groups (11–20, 21–100, and 121–200 mm) (Figure 2A). There was a significant difference (Supplementary Table 1) in the δ13C values between the groups 11–20 mm (δ13C: −17.10 ± 0.15‰) and 21–100 mm (δ13C: −15.89 ± 0.31‰), illustrating an ontogenetic change in resource use and feeding habits. This is consistent with other research assessing the ontogenetic shift in the diet of cephalopods and fishes. For example, stable isotope analysis revealed a positive relationship between isotope ratios and body size of D. gigas, which reflect the changes in the diet of the species (Trasviña-Carrillo et al., 2018). Also, based on stable isotope and stomach content analyses, the walleye pollock (Theragra chalcogramma) (Pallas, 1814) was found to exhibit a dietary shift with growth from consuming mesozooplankton to cephalopods and shrimps as grew (Park et al., 2018). This may be explained by the improvement in predation capacity and a change in the relative abundance of prey. The dietary shift in fish species is almost exclusive due to their improvement in predation ability, gape size, locomotor skills, and speed (Grey, 2001; Borrell et al., 2011). Similarly, for S. esculenta, the predatory ability also increases rapidly with body growth in early life (Lei et al., 2016).
Within group 21–100 mm, the δ13C values hardly changed with mantle length, suggesting a relatively stable feeding orientation or feeding habit, which may indicate that the predation ability of S. esculenta has been equipped at this stage. However, as the mantle length increased, the points representing the δ13C values became more dispersed (Figure 3A). For this, this S. esculenta group was divided into two parts according to the length interval including part 21–60 and part 61–100 mm, and the SEAc of the two parts were calculated, respectively. It was found that part 61–100 mm (SEAc = 0.521) had a larger trophic niche/isotopic niche breadth than part 21–60 mm (SEAc = 0.352), indicating that the S. esculenta within this group expanded its food source range with an increase in mantle length. The ontogenetic increase in the niche width was also noted for other cephalopods. For example, with the increase in the number and abundance of prey species, the SEAc of two generalist feeders I. argentinus and D. gahi also increased with ontogeny (Rosas-Luis et al., 2017). Similar results were also found for the greater hooked squid (Onykia ingens) (Smith, 1881; Rosas-Luis et al., 2014). In this study, more food sources provide more options for S. esculenta and help meet their energy storage needs for overwintering and migration.
For group 121–200 mm, the δ13C values (−16.39 ± 0.35‰) were characterized by a depletion compared with that in group 21–100 mm (−15.89 ± 0.31‰) (Figure 3A), which was mainly related to the migratory habits of S. esculenta. According to previous surveys in the coastal waters of Qingdao, the population abundance of S. esculenta was gradually decreased in September–October and almost completely left the area by November (Wang et al., 2019; Zhang et al., 2019). Next spring, adult S. esculenta returned from wintering ground to spawning ground, so that we were able to collect individuals with a size of more than 120 mm during this period. Compared with oceanic waters, the nutrient flow through relatively long and complex energy pathways in coastal food webs may lead to more enrichment of 13C in species and lead to larger δ13C values of the ecosystem (McCutchan et al., 2003; Cherel et al., 2008; Loc’H et al., 2008). Stable isotopes in food usually take several weeks to reflect in the muscle tissue of the predator (Taylor and Mazumder, 2010), so the lower δ13C value of the S. esculenta 121–200 mm group may partly reflect the stable isotopic characteristics of the wintering ground ecosystems. This is consistent with the isotopic signatures of D. gigas in Peruvian waters, whose δ13C values showed a decreasing trend with increasing distance to the shelf breaks (Argüelles et al., 2012). Similar conditions were also found in fishes (Wu et al., 2019) and seabirds (Hobson et al., 1994; Cherel et al., 2000). The δ13C values within group 121–200 mm increased with mantle length, suggesting that S. esculenta were more inclined to capture prey with higher δ13C values and trophic levels to meet higher energy requirements during the reproductive period. This was different from the way S. esculenta (group 21–100 mm) stored energy by expanding its food source range before overwintering, which may be due to the stronger locomotion and predation ability of the adult S. esculenta (group 121–200 mm), making them more active and selective in predation.
The δ15N values of S. esculenta were found to increase with mantle length, suggesting that the larger an S. esculenta is, the higher its trophic level, indicating a preferred change in dietary items from lower δ15N values to higher δ15N values (Figure 3B). There was a clear and significant linear relationship (P < 0.001, R2 = 0.87) between different S. esculenta groups, with no difference in slope, suggesting that their spawning ground and wintering ground may have similar nitrogen stable isotope characteristics. The stable isotope values (especially for δ15N) at the base (baseline species) of the food webs showed a latitudinal (north–south) gradient change under the influence of CO2 concentration, sea surface temperature, and specific growth rates (Argüelles et al., 2012; Troina et al., 2020), and these latitudinal differences also have been observed in the northern Pacific (Ruiz-Cooley et al., 2010). Previous studies have reported that the wintering grounds of S. esculenta in the coastal waters of China are in the middle and southern Yellow Sea and the northern and middle East China Sea, with large and ambiguous latitudinal spans (Zheng et al., 2003; Wang et al., 2019). Given the variation of δ15N with latitude, we speculate that the wintering ground of the S. esculenta population in Qingdao may be located in the middle of the Yellow Sea, at the same latitude as the spawning ground.
Trophic Relationship and Trophic Levels of Sepia esculenta and Other Organisms
Except for cephalopods, most species in each logical group have similar δ13C values, which mainly reflects the feeding habits of different logical groups. There was one outlier in each logical group (mesodemersal fishes, demersal fishes, and crustaceans), which may be because the species we collected were still too small to form a fixed life habit. For example, the maximum total length of whitespotted conger (Conger myriaster) (Brevoort, 1856) in demersal fishes can reach 1,000 mm (Fishbase), while the sample lengths in this study were only about 220 mm.
Larimichthys polyactis, silver croaker (Pennahia argentata) (Houttuyn, 1782), Johnius grypotus (Richardson, 1846) of mesodemersal fishes, M. joyneri of crustaceans, and Japanese squid (Loligo japonica) (Hoyle, 1885) of cephalopods had some overlaps with the group 11–20 mm of S. esculenta in δ13C values, and higher δ15N values (2–4‰) than that of S. esculenta, which suggests that these species may feed on group 11–20 mm of S. esculenta as one of their food sources. Other studies based on stomach content analysis have also shown that some fishes, crustaceans, and cephalopods in the Yellow Sea feed on S. esculenta (Cheng and Zhu, 1997; Zhang et al., 2011). The stable isotope space (area bounded in the stable isotope biplot) of S. esculenta in the groups 21–100 and 121–200 mm did not overlap with other species after correcting for trophic enrichment. It has been reported that S. esculenta feed on small fishes and crustaceans (Natsukari and Tashiro, 1991), but this relationship was not observed in this study, which may be due to the other species collected did not include their larva/juvenile stage.
There is quite a large range in the δ15N values (4.4‰, Supplementary Table 1), corresponding to 1.3 trophic level (Figure 5) throughout the 1-year life cycle of S. esculenta. This is a shared feature of cephalopods. For example, isotope analysis revealed that T. filippovae with the length of 250–450 mm had δ15N values between 10 and 13‰, corresponding to one trophic grade level (Cherel et al., 2009a). The δ15N value range of S. oualaniensis with a length of 0–300 mm was also about 3%, and O. bartramii with a length of 0–600 mm had a larger range of δ15N value of about 7% (Parry, 2008). The δ15N range of the S. esculenta is relatively large when the size of these cephalopods is taken into account. Such large ranges were also found in both I. argentinus and D. gahi (Rosas-Luis et al., 2017), which reflects the characteristics of trophic plasticity and fast growth of cephalopods (Rodhouse and Nigmatullin, 1996; Parry, 2008; Cherel et al., 2009b). The increase in trophic position indicates the importance of S. esculenta as consumers at various trophic levels throughout their life cycle (Cherel et al., 2009b).
Implications for Resource Proliferation and Management
In recent years, the release intensity of S. esculenta juveniles in Shandong Province has increased year by year, with the release number of more than 1.3 million per year, which has become the most important measure for resource conservation (Oceanic Administration of Shandong Province, 2016). Of these, 0.6 million were released into the coastal waters of Qingdao, accounting for 46% (People, 2018). The low survival rate of juveniles was an important factor affecting the effect of releasing (Hervas et al., 2010). S. esculenta has a 1-year life cycle (Yamada et al., 1986); however, the phenomenon of growth dispersion within the population in natural water is very prominent. According to our previous resource survey (in October 2017) in coastal Qingdao, the mantle length of different individuals captured at the same period could vary by up to six times (16–100 mm). Based on the trophic shifts (the difference in feeding habits between the groups 11–20 and 21–100 mm), we speculate that this may be a life history strategy adopted by S. esculenta, through food conversion and resources partitioning, to reduce the intraspecies predatory competition (Mablouké et al., 2013) and increase the survival rates of juveniles. Therefore, juvenile releases in different periods should be considered to improve the survival rate of the larvae during large-scale juvenile release events.
It is suggested that the survival rates of post-release juveniles were significantly affected by the length (Hervas et al., 2010). At present, the mantle length of S. esculenta releasing juveniles is mostly between 12 and 15 mm (Oceanic Administration of Shandong Province, 2016). S. esculenta in this period may be in the prey range of fishes and crustaceans, such as L. polyactis and M. joyneri, as one of their prey organisms (Figure 4). However, the δ13C and δ15N values of S. esculenta increased significantly (Supplementary Table 1) when they grew to 21–30 mm (Supplementary Table 1), with the overlap of carbon isotope space with other species decreased obviously, indicating that they had been greatly reduced the proportion in the diet composition of other species. S. esculenta grows fast (Zhang et al., 2019), so if the artificial culture time of prerelease can be slightly extended to increase the length to more than 20 mm, the pressure of being prey may be reduced significantly and the effect of release can be improved.
Conclusion
In this study, the ontogenetic trophic ecology and migration patterns of S. esculenta in Chinese northern coastal areas were investigated using the isotope analysis. S. esculenta were divided into three groups (11–20, 21–100, and 121–200 mm) based on isotopic values, with a significant difference in the δ13C values between the groups 11–20 and 21–100 mm, illustrating an ontogenetic change in resource use and feeding habits. The δ13C value of group 121–200 mm was lower than that of group 21–100 mm, which was mainly related to the migratory habits of S. esculenta and partly reflected the stable isotopic characteristics of the wintering ground ecosystems. The δ15N values of S. esculenta were found to increase in mantle length, and there was a clear and significant linear relationship between different groups. It is suggested that the wintering ground of S. esculenta in Qingdao coastal waters may have similar nitrogen stable isotope characteristics with spawning ground. In addition, it suggested that some species may feed on group 11–20 mm of S. esculenta as one of their food sources. Thus, slightly increasing the length to more than 20 mm may significantly reduce the pressure of being the prey of post-release juveniles and improve the effect of release.
Data Availability Statement
The datasets presented in this study can be found in online repositories. The names of the repository/repositories and accession number(s) can be found in the article/Supplementary Material.
Ethics Statement
The animal study was reviewed and approved by the Animal Care and Ethics Committee of the Ocean University of China.
Author Contributions
LW contributed to conceptualization, methodology, investigation, resources, writing-original draft, and writing-review and editing. YL contributed to data curation and investigation. XZ contributed to supervision, project administration, conceptualization, methodology, resources, and writing-review and editing. All authors contributed to the article and approved the submitted version.
Funding
This study was supported financially by funds from the National Key R&D Program of China (2019YFD0901303) and the National Natural Science Foundation of China (41676153 and 31172447).
Conflict of Interest
The authors declare that the research was conducted in the absence of any commercial or financial relationships that could be construed as a potential conflict of interest.
Publisher’s Note
All claims expressed in this article are solely those of the authors and do not necessarily represent those of their affiliated organizations, or those of the publisher, the editors and the reviewers. Any product that may be evaluated in this article, or claim that may be made by its manufacturer, is not guaranteed or endorsed by the publisher.
Acknowledgments
The authors express their gratitude to the staff of Marine Stock Enhancement Ecology Laboratory of Ocean University of China for the help with the experiment.
Supplementary Material
The Supplementary Material for this article can be found online at: https://www.frontiersin.org/articles/10.3389/fmars.2022.818088/full#supplementary-material
Footnotes
References
Argüelles, J., Lorrain, A., Cherel, Y., Graco, M., Tafur, R., Alegre, A., et al. (2012). Tracking habitat and resource use for the jumbo squid Dosidicus gigas: a stable isotope analysis in the Northern Humboldt Current System. Mar. Biol. 159, 2105–2116. doi: 10.1007/s00227-012-1998-2
Borrell, A., Cardona, L., Kumarran, R., and Aguilar, A. (2011). Trophic ecology of elasmobranchs caught off Gujarat, India, as inferred from stable isotopes. ICES J. Mar. Sci. 68, 547–554. doi: 10.1093/icesjms/fsq170
Cheng, J., and Zhu, J. (1997). Feeding characteristics and trophic levels of main economic invertebrates in the Yellow Sea. Acta. Oceanol. Sin. 19, 102–108 (in Chinese).
Cherel, Y., Ducatez, S., Fontaine, C., Richard, P., and Guinet, C. (2008). Stable isotopes reveal the trophic position and mesopelagic fish diet of female southern elephant seals breeding on the Kerguelen Islands. Mar. Ecol. Prog. Ser. 370, 239–247. doi: 10.3354/meps07673
Cherel, Y., Fontaine, C., Jackson, G., Jackson, C., and Richard, P. (2009a). Tissue, ontogenic and sex-related differences in δ13C and δ15N values of the oceanic squid Todarodes filippovae (Cephalopoda: Ommastrephidae). Mar. Biol. 156, 699–708. doi: 10.1007/s00227-008-1121-x
Cherel, Y., Hobson, K., and Weimerskirch, H. (2000). Using stable-isotope analysis of feathers to distinguish moulting and breeding origins of seabirds. Oecologia 122, 155–162. doi: 10.1007/PL00008843
Cherel, Y., Ridoux, V., Spitz, J., and Richard, P. (2009b). Stable isotopes document the trophic structure of a deep-sea cephalopod assemblage including giant octopod and giant squid. Biol. Lett. 5, 364–367. doi: 10.1098/rsbl.2009.0024
Cherel, Y., and Hobson, K. (2005). Stable isotopes, beaks and predators: a new tool to study the trophic ecology of cephalopods, including giant and colossal squids. Proc. R. Soc. B: Biol. Sci. 272, 1601–1607. doi: 10.1098/rspb.2005.3115
DeNiro, M., and Epstein, S. (1981). Influence of diet on the distribution of nitrogen isotopes in animals. Geochim. Cosmochim. Ac. 42, 495–506. doi: 10.1016/0016-7037(81)90244-1
Dong, Z. (1991). Biology of the Economic Species of Cephalopods in the World Oceans. Jinan. China: Shandong Science and Technology Press.
Folch, J., Lees, M., and Sloane Stanley, G. H. (1957). A simple method for the isolation and purification of total lipids from animal tissues. J. Biol. Chem. 226, 497–509. doi: 10.1016/S0021-9258(18)64849-5
Fry, B. (1988). Food web structure on Georges Bank from stable C, N, and S isotopic compositions. Limnol. Oceanogr. 33, 1182–1190. doi: 10.4319/lo.1988.33.5.1182
Golikov, A. V., Ceia, F. R., Sabirov, R. M., Ablett, J. D., Gleadall, L. G., Guamundsson, G., et al. (2019). The first global deep-sea stable isotope assessment reveals the unique trophic ecology of Vampire Squid Vampyroteuthis infernalis (Cephalopoda). Sci. Rep. 9:19099. doi: 10.1038/s41598-019-55719-1
Grey, J. (2001). Ontogeny and dietary specialization in brown trout (Salmo trutta L.) from Loch Ness, Scotland, examined using stable isotopes of carbon and nitrogen. Ecol. Freshw. Fish 10, 168–176. doi: 10.1034/j.1600-0633.2001.100306.x
Guerra, A., Rodriguez-Navarro, A., González, Á, Romanek, C., Alvarez-Lloret, P., and Pierce, G. (2010). Life-history traits of the giant squid Architeuthis dux revealed from stable isotope signatures recorded in beaks. ICES J. Mar. Sci. 67, 1425–1431. doi: 10.1093/icesjms/fsq091
Guerreiro, M., Phillips, R., Cherel, Y., Ceia, F., Alvito, P., Rosa, R., et al. (2015). Habitat and trophic ecology of Southern Ocean cephalopods from stable isotope analyses. Mar. Ecol. Prog. Ser. 530, 119–134. doi: 10.3354/meps11266
Hao, Z., Zhang, X., and Zhang, P. (2007). Biological characteristics and multiplication techniques of Sepia esculenta. Chin. J. Ecol. 26, 601–606.
Hervas, S., Lorenzen, K., Shane, M. A., and Drawbridge, M. A. (2010). Quantitative assessment of a white seabass (Atractoscion nobilis) stock enhancement program in California: post-release dispersal, growth and survival. Fish. Res. 105, 237–243. doi: 10.1016/j.fishres.2010.06.001
Hobson, K., and Cherel, Y. (2006). Isotopic reconstruction of marine food webs using cephalopod beaks: new insight from captively raised Sepia officinalis. Can. J. Zool. 84, 766–770. doi: 10.1139/z06-049
Hobson, K., Piatt, J., and Pitocchelli, J. (1994). Using stable isotopes to determine seabird trophic relationships. J. Anim. Ecol. 68, 786–798. doi: 10.2307/5256
Jackson, A. L., Inger, R., Parnell, A. C., and Bearhop, S. (2011). Comparing isotopic niche widths among and within communities: SIBER-Stable Isotope Bayesian Ellipses in R. J. Anim. Ecol. 80, 595–602. doi: 10.1111/j.1365-2656.2011.01806.x
Layman, C., Araujo, M., Boucek, R., Hammerschlag-Peyer, C., Harrison, E., Jud, Z., et al. (2012). Applying stable isotopes to examine food-web structure: an overview of analytical tools. Biol. Rev. 87, 545–562. doi: 10.1111/j.1469-185X.2011.00208.x
Lei, S., Zhang, X., Zhang, P., and Qin, L. (2016). Studies on the feeding behavior and ability of juveniles Sepia esculenta (Cephalopoda: Sepiida). Period. Ocean Univ. China 46, 37–42.
Li, X., Zhao, J., Liu, H., Zhang, H., and Hou, X. (2018). Status, problems and optimized management of spawning, feeding, overwintering grounds and migration route of marine fishery resources in Bohai Sea and Yellow Sea. Trans. Ocean. Limnol. 5, 147–157. doi: 10.13984/j.cnki.cn37-1141.2018.05.019
Loc’H, F., Hily, C., and Grall, J. (2008). Benthic community and food web structure on the continental shelf of the Bay of Biscay (North Eastern Atlantic) revealed by stable isotopes analysis. J. Mar. Syst. 72, 17–34. doi: 10.1016/j.jmarsys.2007.05.011
Mablouké, C., Kolasinski, J., Potier, M., Cuvillier, A., Potin, G., Bigot, L., et al. (2013). Feeding habits and food partitioning between three commercial fish associated with artificial reefs in a tropical coastal environment. Afr. J. Mar. Sci. 35, 323–334. doi: 10.2989/1814232X.2013.829790
McCutchan, J., Lewis, W., Kendall, C., and Mcgrath, C. (2003). Variation in trophic shift for stable isotope ratios of carbon, nitrogen, and sulfur. Oikos 102, 378–390. doi: 10.1034/j.1600-0706.2003.12098.x
Natsukari, Y., and Tashiro, M. (1991). Neritic squid resources and cuttlefish resources in Japan. Mar. Freshw. Behav. Physiol. 18, 149–226. doi: 10.1080/10236249109378785
Newsome, S., Phillips, D., Culleton, B., Guilderson, T., and Koch, P. (2004). Dietary reconstruction of an early to middle Holocene human population from the central California coast: insights from advanced stable isotope mixing models. J. Archaeol. Sci. 31, 1101–1115. doi: 10.1016/j.jas.2004.02.001
Niu, C., Yang, C., Huang, Y., and Zhang, X. (2017). The efficacy of new spawning substrates for Sepia esculenta oosperm adhesion. J. Fish. Sci. China 24, 1234–1244. doi: 10.3724/SP.J.1118.2017.16368
Oceanic Administration of Shandong Province. (2016). E. coli. http://hyj.shandong.gov.cn/xwzx/sjdt/201607/t20160719_552183.html (accessed October 15, 2021).
Ohkouchi, N., Tsuda, R., Chikaraishi, Y., and Tanabe, K. (2013). A preliminary estimate of the trophic position of the deep-water ram’s horn squid Spirula spirula based on the nitrogen isotopic composition of amino acids. Mar. Biol. 160, 773–779. doi: 10.1007/s00227-012-2132-1
Park, H., Park, T., Lee, C., and Kang, C. (2018). Ontogenetic shifts in diet and trophic position of walleye pollock, Theragra chalcogramma, in the western East Sea (Japan Sea) revealed by stable isotope and stomach content analyses. Fish. Res. 204, 297–304. doi: 10.1016/j.fishres.2018.03.006
Parry, M. (2008). Trophic variation with length in two ommastrephid squids, Ommastrephes bartramii and Sthenoteuthis oualaniensis. Mar. Biol. 153, 249–256. doi: 10.1007/s00227-007-0800-3
People, C. N. (2018). E. coli. http://pic.people.com.cn/n1/2018/0727/c1016-30172809.html (accessed October 15, 2021).
Peterson, B., and Fry, B. (1987). Stable isotopes in ecosystem studies. Annu. Rev. Ecol. Evol. S. 18, 293–320. doi: 10.1146/annurev.es.18.110187.001453
Post, D. (2002). Using stable isotopes to estimate trophic position: models, methods, and assumptions. Ecology 83, 703–718. doi: 10.1111/j.1095-8649.2012.03251.x
Queirós, J. P., Phillips, R. A., Baeta, A., Abreu, J., and Xavier, J. C. (2019). Habitat, trophic levels and migration patterns of the short-finned squid Illex argentinus from stable isotope analysis of beak regions. Polar Biol. 42, 2299–2304. doi: 10.1007/s00300-019-02598-x
R Development Core Team. (2020). R: a language and environment for statistical computing. Vienna, Austria: R Foundation for Statistical Computing.
Rodhouse, P., and Nigmatullin, C. (1996). Role as consumers. Philos. Trans. R. Soc. Lond. Ser. B-Biol. Sci. 351, 1003–1022. doi: 10.1098/rstb.1996.0090
Rosas-Luis, R., Navarro, J., Martínez-Baena, F., and Sánchez, P. (2017). Differences in the trophic niche along the gladius of the squids Illex argentinus and Doryteuthis gahi based on their isotopic values. Reg. Stud. Mar. Sci. 11, 17–22. doi: 10.1016/j.rsma.2017.02.003
Rooker, J. R., David Wells, R. J., Addis, P., Arrizabalaga, H., Baptista, M., Bearzi, G., et al. (2020). Natural geochemical markers reveal environmental history and population connectivity of common cuttlefish in the Atlantic Ocean and Mediterranean Sea. J. R. Soc. Interface 17:20200309. doi: 10.6084/m9.figshare
Rosas-Luis, R., Sáncheza, P., Portelab, J. M., and Rio, J. L. (2014). Feeding habits and trophic interactions of Doryteuthis gahi, Illex argentinus and Onykia ingens in the marine ecosystem off the Patagonian Shelf. Fish. Res. 152, 37–44. doi: 10.1016/j.fishres.2013.11.004
Rudolf, V. H. W. (2020). A multivariate approach reveals diversity of ontogenetic niche shifts across taxonomic and functional groups. Freshw. Biol. 65, 745–756. doi: 10.1111/fwb.13463
Ruiz-Cooley, R. I., Villa, E. C., and Gould, W. R. (2010). Ontogenetic variation of updelta13C and updelta15N recorded in the gladius of the jumbo squid Dosidicus gigas: geographic differences. Mar. Ecol. Prog. Ser. 399, 187–198. doi: 10.3354/meps08383
Taylor, M., and Mazumder, D. (2010). Stable isotopes reveal post-release trophodynamic and ontogenetic changes in a released finfish, mulloway (Argyrosomus japonicus). Mar. Freshw. Res. 61, 302–308. doi: 10.1071/MF09014
Trasviña-Carrillo, L. D., Hernández-Herrera, A., Torres-Rojas, Y. E., Galván-Magaña, F., Sánchez-González, A., and Aguíñiga-García, S. (2018). Spatial and trophic preferences of jumbo squid Dosidicus gigas (D’Orbigny, 1835) in the central Gulf of California: ecological inferences using stable isotopes. Rapid Commun. Mass Spectrom. 32, 1225–1236. doi: 10.1002/rcm.8147
Troina, G. C., Dehairs, F., Botta, S., Tullio, J. C. D., Elskens, M., and Secchi, E. R. (2020). Zooplankton-based δ13C and δ15N isoscapes from the outer continental shelf and slope in the subtropical western South Atlantic. Deep Sea Res. I Oceanogr. Res. Pap. 159:103235. doi: 10.1016/j.dsr.2020.103235
Urrutia-Olvera, A., Jordán-Garza, A. G., Villegas-Sánchez, C. A., Arizmendi-Rodríguez, D. I., and Rosas-Luis, R. (2021). Prey contribution to the diet of Octopus insularis (Leite and Haimovici, 2008) using stable isotopes and stomach content analysis in the Western Gulf of Mexico. Aquat. Ecol. 55, 765–777. doi: 10.1007/s10452-021-09859-0
Wang, L., Wang, Z., Zhang, X., and Zhang, X. (2018). Effects of different substrate and temperature on hunger tolerance in Sepia esculenta juveniles. J. Fish. Sci. China 25, 1071–1081. doi: 10.3724/SP.J.1118.2018.17375
Wang, L., Zhang, X., Wang, Z., Song, N., and Gao, T. (2019). Morphological characteristics and genetic differentiation of a breedingpopulation of Sepia esculenta in Qingdao. J. Fish. Sci. China 26, 342–352. doi: 10.3724/SP.J.1118.2019.18169
Wu, Z., Zhang, X., Dromard, C., Tweedley, J., and Loneragan, N. (2019). Partitioning of food resources among three sympatric scorpionfish (Scorpaeniformes) in coastal waters of the northern Yellow Sea. Hydrobiologia 826, 331–351. doi: 10.1007/s10750-018-3747-0
Yamada, U., Tagawa, M., Kishita, S., and Hisi, H. (1986). Fishes of the East China Sea and the Yellow Sea. Nagasaki: Seikai: National Fisheries Research Laboratory.
Zanden, J., and Rasmussen, J. (2001). Variation in δ15N and δ13C trophic fractionation: Implications for aquatic food web studies. Limnol. Oceanogr. 46, 2061–2066. doi: 10.4319/lo.2001.46.8.2061
Zhang, X., Wang, L., Zhang, Y., Luo, G., and Gao, H. (2019). Strategy optimization of stock enhancement of golden cuttlefish, (Sepia esculenta) based on structural characteristics of reproductive and recruitment populations. J. Fish. China 43, 1890–1899. doi: 10.11964/jfc.20190711877
Zhang, Y., Xu, K., and Jin, H. (2011). Feeding habits and ontogenetic variation of Lophius litulon in the East China Sea and Yellow Sea. Prog. Fish. Sci. 33, 9–18.
Zheng, X., Zhao, J., Xiao, S., Wang, R., Wang, S., and Zhou, W. (2004). Isozymes analysis of the golden Cuttlefish Sepia esculenta (Cephalopoda: Sepiidae). J. Ocean Univ. China 3, 48–52.
Keywords: Sepia esculenta, ontogeny, dietary shift, trophic ecology, isotopic analysis, resource management
Citation: Wang L, Liu Y and Zhang X (2022) Stable Isotope Analysis Revealed Ontogenetic Changes in Trophic Ecology and Migration Patterns of Sepia esculenta in the Northern Coastal Waters of China. Front. Mar. Sci. 9:818088. doi: 10.3389/fmars.2022.818088
Received: 19 November 2021; Accepted: 13 January 2022;
Published: 28 February 2022.
Edited by:
Çetin Keskin, Istanbul University, TurkeyReviewed by:
Rigoberto Rosas-Luis, Technological Institute of Chetumal, MexicoAlberto Sánchez-González, Instituto Politécnico Nacional (IPN), Mexico
Copyright © 2022 Wang, Liu and Zhang. This is an open-access article distributed under the terms of the Creative Commons Attribution License (CC BY). The use, distribution or reproduction in other forums is permitted, provided the original author(s) and the copyright owner(s) are credited and that the original publication in this journal is cited, in accordance with accepted academic practice. No use, distribution or reproduction is permitted which does not comply with these terms.
*Correspondence: Xiumei Zhang, xiumei1227@163.com