Two New Species and One New Genus of Glass Sponges (Hexactinellida: Euplectellidae and Euretidae), From a Transect on a Seamount in the Northwestern Pacific Ocean
- 1Key Laboratory of Marine Ecosystem Dynamics, Second Institute of Oceanography, Ministry of Natural Resources (MNR), Hangzhou, China
- 2Southern Marine Science and Engineering Guangdong Laboratory (Zhuhai), Zhuhai, China
- 3School of Oceanography, Shanghai Jiao Tong University, Shanghai, China
Hexactinellid sponges often form structural habitats for other organisms and thus support high biodiversity. Two representative morphotypes of hexactinellid sponges were sampled by a remotely operated vehicle along a transect (depths of 2377–2758 m) on the Ko-Hakucho Guyot in the northwestern Pacific Ocean, both new to science. One new species, Flavovirens hemiglobus gen. et sp. nov., which is pedunculate and bears the main choanosomal spicules of diactins, is clearly attributed to the euplectellid subfamily Bolosominae. Its set of microscleres present (namely, predominating oxyhexasters and discasters, and rare discohexasters and staurodiscs) characterizes it as a new genus. The other new species, Chonelasma tyloscopulatum sp. nov., which is funnel in form without dichotomous branching tubes or lateral oscula and has firm body walls supported by a three-layered dictyonal framework, is clearly attributed to the euretid genus Chonelasma (subfamily Chonelasmatinae). Its presence of surface pentactins, two types of scopules, and both oxy- and disco-tipped hexasters or hemihexasters as common microscleres, characterizes it as a new species. The placements are also supported by molecular phylogenetic evidence from nuclear 18S rDNA and 28S rDNA, and mitochondrial 16S rDNA and cytochrome c oxidase subunit I (COI) gene. More sampling efforts should be conducted to improve the understanding of the biodiversity of deep-sea seamount sponges.
Introduction
Sponges are important structure-forming taxa in the deep sea; they can provide biogenic microhabitats for other invertebrates to associate with, thus increasing local habitat heterogeneity and supporting high biodiversity (Bell, 2008; Hajdu et al., 2017). Compared to other invertebrates, hexactinellid sponges often have complex and exquisite frameworks; therefore, their functional significance has been highlighted (Shen et al., 2021). As one of the two subclasses of hexactinellid sponges, Hexasterophora is characterized by the presence of microhexasters as the main microscleres; however, there are significant differences in their frameworks, thus showing diverse and distinct appearances (Reiswig and Wheeler, 2002; Tabachnick, 2002). Therein, the family Euplectellidae (Gray, 1872) belongs to the order Lyssacinosida, which features frameworks formed by loose spicules (the texture is soft); the family Euretidae (Zittel, 1877) belongs to the order Sceptrulophora with dictyonal frameworks formed by fused spicules (the texture is hard and fragile). Both are widely distributed (Reiswig and Wheeler, 2002; Tabachnick, 2002) and the main groups of habitat-forming species on deep-water seamounts (Clark et al., 2011).
Sponges in the Euplectellidae family are generally tubular, cup-like or fungus-like and sometimes pedunculate (Tabachnick, 2002). According to the method of attachment to the substratum, the body form and the type of atrialia, this family is currently divided into three subfamilies: Euplectellinae (Gray, 1872); Corbitellinae (Gray, 1872); and Bolosominae Tabachnick, 2002; with a total of 31 genera (15 of which are monotypic) and 125 valid species to date (de Voogd et al., 2022). Previously, Euplectellidae was classified into three genera: Euplectella, Corbitella, and Heterotella (Gray, 1867). Schulze (1886; 1887) subdivided it into three subfamilies based on the dermal skeleton content: Euplectellinae, Holascinae, and Taegerinae (later synonymized with Corbitellinae), with six genera. Tabachnick (2002) then divided Euplectellidae into three subfamilies with a new subfamily named Bolosominae thus to form the basic taxa consisting of 27 genera in three subfamilies. Later, Dohrmann (2016) transferred the genus Symplectella (previously belonging to the subfamily Corbitellinae) to the family Rossellidae. Recently, five new monotypic genera have been added into Euplectellidae, of which four are bolosomines (Reiswig and Kelly, 2018; Shen et al., 2019; Castello-Branco et al., 2020). The definition of Bolosominae was emended by Shen et al. (2019) to include the rhizophytous attachment together with basiphytous attachment of pedunculate euplectellids. Thus, basiphytous (or rarely rhizophytous) and pedunculate euplectellids are allocated to the subfamily Bolosominae (Tabachnick, 2002; Shen et al., 2019), currently containing 13 genera (nine of which are monotypic) and 33 valid species (de Voogd et al., 2022). It should be noted that these three subfamilies are likely not monophyletic (Dohrmann et al., 2017; Dohrmann, 2019).
Sponges in the Euretidae family are predominately tubular, cup-funnel formed, or blade formed, with or without dichotomously branching secondary lateral tubes (Reiswig and Wheeler, 2002). According to the body form, this family is presently divided into two subfamilies: Euretinae (Zittel, 1877); and Chonelasmatinae (Schrammen, 1912); with a total of 15 genera (four of which are monotypic) and 58 valid species to date (de Voogd et al., 2022). Those with bodies without dichotomous branching are allocated to the subfamily Chonelasmatinae, including seven genera (two of which are monotypic) and 26 valid species to date (Reiswig and Wheeler, 2002; de Voogd et al., 2022). Therein, the genus Chonelasma Schulze, 1886 has the funnel-like body form without lateral oscula and currently accounts for 42% of the total species of Chonelasmatinae. The concept of family Euretidae and inclusion of taxa have varied widely; subdivision of the family into two subfamilies was proposed by Reid (1958) and modified slightly by Reiswig and Wheeler (2002) thus to form the basic classification. Previously, 16 genera were allocated into the family Euretidae by Reiswig and Wheeler (2002); later, Homoieurete Reiswig and Kelly, 2011 and Pinulasma Reiswig and Stone, 2013 were erected and added into the subfamily Chonelasmatinae, while the former was then removed based on the molecular evidence (Dohrmann et al., 2017); meanwhile, the two sceptrule- and uncinate-lacking genera, Heterorete (previously belonging to the subfamily Euretinae) and Myliusia (previously belonging to the subfamily Chonelasmatinae), were moved into Hexasterophora incertae sedis by Dohrmann et al. (2017) based on their phylogenetic placement. It should be noted that this family and its two subfamilies are not monophyletic groups; there currently seem to be two unrelated groups of euretid genera according to phylogenetic analyses and one of these two groups is closely related to the family Farreidae (Reiswig and Dohrmann, 2014; Dohrmann et al., 2017; Dohrmann, 2019).
In this study, two specimens of representative glass sponges have been sampled from a transect at depths ranging from 2377 m to 2758 m located in the northern Ko-Hakucho Guyot of the northwestern Pacific Ocean by a remotely operated vehicle (ROV); these two specimens are both described new to science, with one species attributed to a new genus of the family Euplectellidae and the other species to the genus Chonelasma of the family Euretidae, after both morphological and molecular assessments.
Materials and Methods
Sample Collection
Two new specimens were collected on Sep. 16, 2020 by ROV Hailong III from the Ko-Hakucho Guyot at one transect of dive ROV01a of cruise DY61/I onboard R/V Da Yang Yi Hao. The transect is located on the upper slope of the northern Ko-Hakucho Guyot at depths from 2377 m to 2758 m; this seamount is located on the northwestern Pacific Ocean adjacent to the Marcus-Wake Seamount chain to the east and the Magellan Seamount chain to the southeast. The holotype (catalog number: SIO-POR-292) of Flavovirens hemiglobus gen. et sp. nov. was collected by the ROV manipulator arms at the station at 23.5152°N, 148.5779°E, at a depth of 2716 m; the holotype (catalog number: SIO-POR-294) of Chonelasma tyloscopulatum sp. nov. was collected by the ROV suction samplers at the station at 23.5037°N, 148.5735°E, at a depth of 2425 m. The specimens were preserved in 95% ethanol and deposited in the Sample Repository of Second Institute of Oceanography (SRSIO), Hangzhou, China.
Spicule and Skeleton Examinations
Spicules were isolated by digesting small pieces of tissue from several specific parts of the body, which were placed in different centrifuge tubes, in concentrated nitric acid using a metal bath maintained at about 80°C for one day until the tissues were completely dissolved. The spicules were then rinsed twice in distilled water and later suspended in alcohol for Light Microscope (LM) and Scanning Electron Microscope (SEM) observation. Frozen sections, 1.0–2.0 mm thick, were prepared by handing slicing vertically or horizontally to examine the dictyonal framework of the specimen belonging to euretids. The sections were placed on different grooved slides and digested in concentrated nitric acid by using a heating plate. The digested sections were then rinsed twice by pipetting distilled water and dried in the air for observation. Slides for LM were examined by ZEISS Imager A2. Spicules for SEM (TM-1000 Tabletop Microscope) were pipetted from the solution onto silicon wafers, and skeletons were moved from the grooved slides onto silicon wafers, all of which were subsequently coated with platinum. Photomicrographs were obtained by SEM, and measurements were made with the software ZEN pro 2012 1.1.2.0.
Molecular Work
DNA was extracted from pieces of sponge tissue by using a DNeasy Blood & Tissue Kit (Qiagen) according to the manufacturer’s instructions. The DNA quality and concentration were detected by Nanodrop 2000 and Qubit fluorometer. Then the genomic DNA was sequenced via Illumina PE150 strategy. One pair-end library was constructed with the insert size of approximately 300–400 bp and sequenced on HiSeq™ X-Ten platform (Illumina, CA, USA) at Zhejiang Tianke High Technology Development Co., Ltd. The total of fastq reads were qualified, trimmed and assembled by MEGAHIT assembler (Li et al., 2015; Li et al., 2016). The nuclear 18S ribosomal RNA gene (18S rDNA) and 28S ribosomal RNA gene (28S rDNA), and mitochondrial 16S ribosomal RNA gene (16S rDNA) and cytochrome c oxidase subunit I (COI) gene were recovered from the assembly contigs. The obtained four marker genes were used for phylogenetic analyses. The markers were checked by mapping the reported universal primer pairs (Folmer et al., 1994; Dohrmann et al., 2008) to the target sequences.
Phylogenetic Analyses
Detailed information of species and their marker sequences used for phylogenetic analyses in this study is shown in Supplementary Table 1. The reference alignments of four marker genes of 18S rDNA, 28S rDNA, 16S rDNA and COI sequences were provided by Dohrmann (2019) with secondary structure information of 18S and 28S rDNA in alignments (available online: https://doi.org/10.6084/m9.figshare.5951965). The newly added four marker-gene sequences of the two new species and Tretopleura weijica were manually aligned with those alignment files and edited in the Geneious prime software (Biomatters Ltd., New Zealand). The single-gene alignments were aligned separately, whose unalignable regions were trimmed manually, and then concatenated into one supermatrix file with partition information for exporting (available online: https://doi.org/10.6084/m9.figshare.19447883). Semperella schultzei QM G318562 belonging to the family Pheronematidae was selected as the outgroup. The phylogenetic tree was reconstructed with partitioned maximum likelihood (ML) analysis using RAxML GUI v.1.5 (Silvestro and Michalak, 2012; Stamatakis, 2014), with rapid bootstrapping (1000 replicates) and the GTR+G model (Lanave et al., 1984; Yang, 1994). The ML tree was visualized in Figtree (http://tree.bio.ed.ac.uk/software/figtree/).
Results
Systematics of New Species of Euplectellidae
Phylum Porifera (Grant, 1836)
Class Hexactinellida (Schmidt, 1870)
Subclass Hexasterophora (Schulze, 1886)
Order Lyssacinosida (Zittel, 1877)
Family Euplectellidae (Gray, 1867)
Subfamily Bolosominae (Tabachnick, 2002)
Genus Flavovirens gen. nov.
Type species. Flavovirens hemiglobus gen. et sp. nov. (described below).
Diagnosis
Body is hemiglobular, pedunculate, basiphytous with a wide, upright opening. No apparent extended margin or marginalia. Dermal and atrial surfaces are cratered, without apparent lattice covers. Choanosomal spicules are mainly diactins, plus uncommon hexactins, pentactins, stauractins, tetractins and triactins. Dermalia and atrialia are pinular hexactins. Microscleres predominate as oxyhexasters and discasters and rare discohexasters and staurodiscs.
Etymology
The new genus Flavovirens refers to the in situ color of the peduncle of holotype which is yellowish green (described below; Figure 1A). The new name is formed from flavus (Latin word for yellow) and virens (Latin word for green); the gender is neuter.
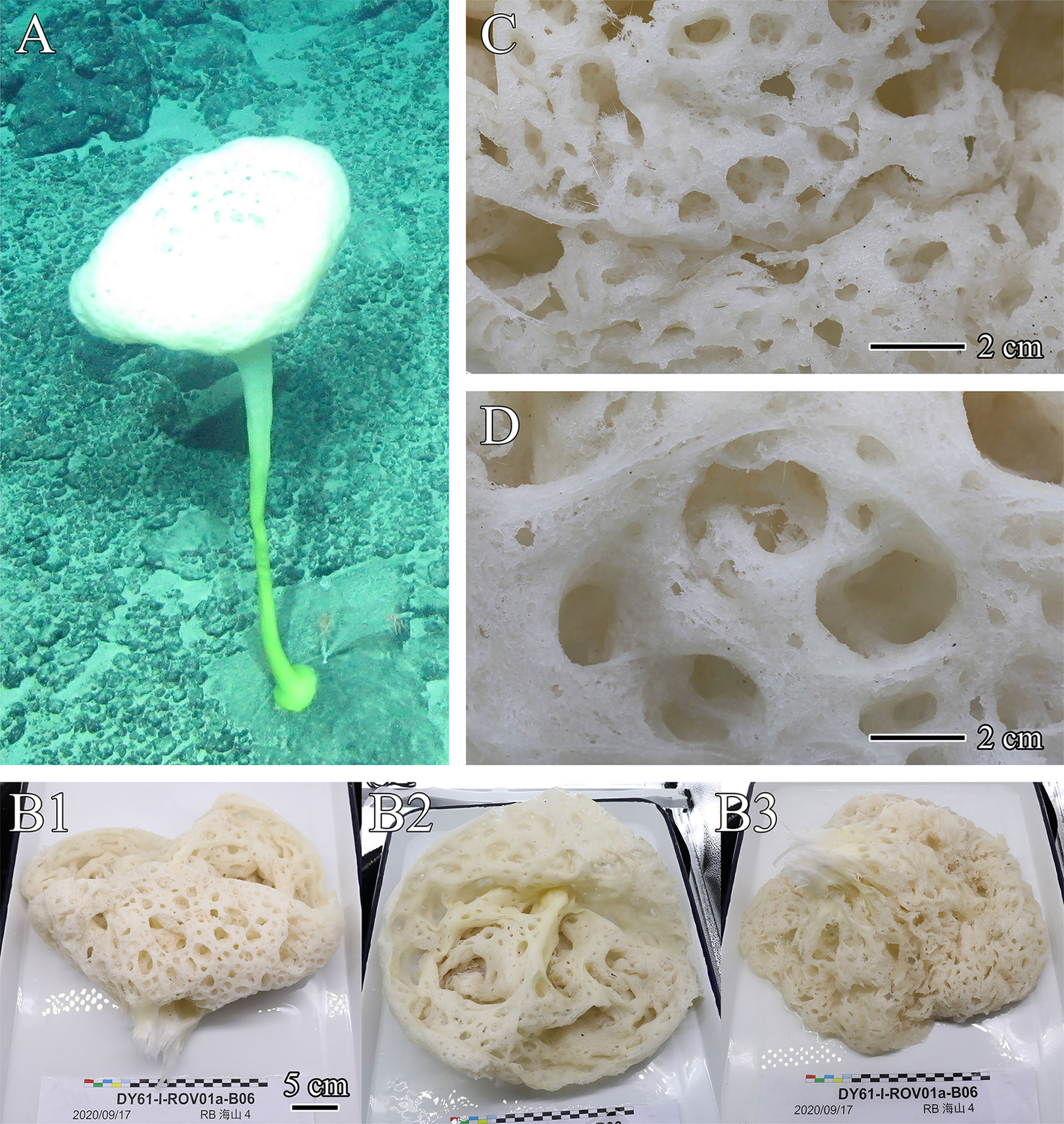
Figure 1 Flavovirens hemiglobus gen. et sp. nov., specimen of holotype. (A) In situ image. (B) Whole collected specimen, lateral view of body head (B1), top view of opening (B2), and bottom view of body head (B3). (C) Close-up view of dermal surface. (D) Close-up view of atrial surface.
Remarks
Due to the main choanosomal spicules of diactins and the presence of a long peduncle, in addition to the presence of discasters and oxyhexasters as the main microscleres, the new specimen is clearly attributed to the subfamily Bolosominae of the family Euplectellidae. It is distinguished from other bolosomines by the set of microscleres present, namely, predominating oxyhexasters and discasters and rare discohexasters and staurodiscs.
The absence of graphiocomes prevents its assignment to Trachycaulus, Vityaziella, Trychella, Neocaledoniella or Advhena. In addition, Trachycaulus lacks disco-tipped microscleres which are present in the new specimen; Vityaziella and Trychella present amphidiscs which are absent in the new specimen; Neocaledoniella and Advhena have discohexasters in the form of calycocomes, while the new specimen has discohexasters mainly in the form of discasters but not calycocomes. The absence of plumicomes prevents its assignment to Caulocalyx (bearing only plumicomes and discohexasters as microscleres) or Saccocalyx (various microscleres including other codonhexasters, drepanocomes, and spirodiscohexasters). The absence of floricomes prevents its assignment to Amphoreus, Hyalostylus or Rhabdopectella. In addition, Amphoreus features an amphora shape, while the new specimen has a hemiglobular body with a stalk; Hyalostylus and Rhabdopectella both have sigmatocomes. The absence of amphidiscs prevents its assignment to Amphidiscella. The presence of abundant oxy-tipped microscleres prevents its assignment to Rhizophyta or Bolosoma since they have only disco-tipped microscleres. Therefore, the set of microscleres renders the new specimen unique among bolosomines and thus justifies the proposal of a new genus.
Flavovirens hemiglobus gen. et sp. nov. (Figures 1, 2; Table 1).
Material Examined
Holotype (Figure 1): SIO-POR-292 (original number: DY61-ROV01a-B06), SRSIO, Hailong III ROV DY61/I, dive ROV01a, collected by Chengcheng Shen, Sep. 16, 2020, on the Ko-Hakucho Guyot in the northwestern Pacific Ocean (23.5152°N, 148.5779°E), depth of 2716 m, stored in 95% ethanol.
Description
The new species presents a large, soft, hemispherical body borne on a moderately long, robust, slightly curved peduncle with a disc-like base of attachment (Figure 1A). The atrium is approximately 14.4 cm in depth, and the osculum is upright and wide, approximately 33.5 cm in diameter, without an extended margin or marginalia (Figure 1B). The inhalant and exhalant systems are composed of wide and open compound apertures without lattice covers, cratering the dermal and atrial surfaces (Figures 1C, D). The inhalant and exhalant apertures are 5.1 cm×3.6 cm in maximum size. In situ color is white for the body and yellowish green for the below half of the peduncle (Figure 1A); on the desk and preserved, the body is light beige (Figure 1B).
Megascleres
Surface megascleres of the body are dermal, atrial and marginal pinular hexactins; choanosomal megascleres are common diactins (two types, together >56.0%), a number of hexactins (two types, together <22.4%) and pentactins (<11.2%), and rare stauractins (<3.7%), tetractins (<1.9%) and triactins (<4.7%; Figures 2A–I; Table 1).
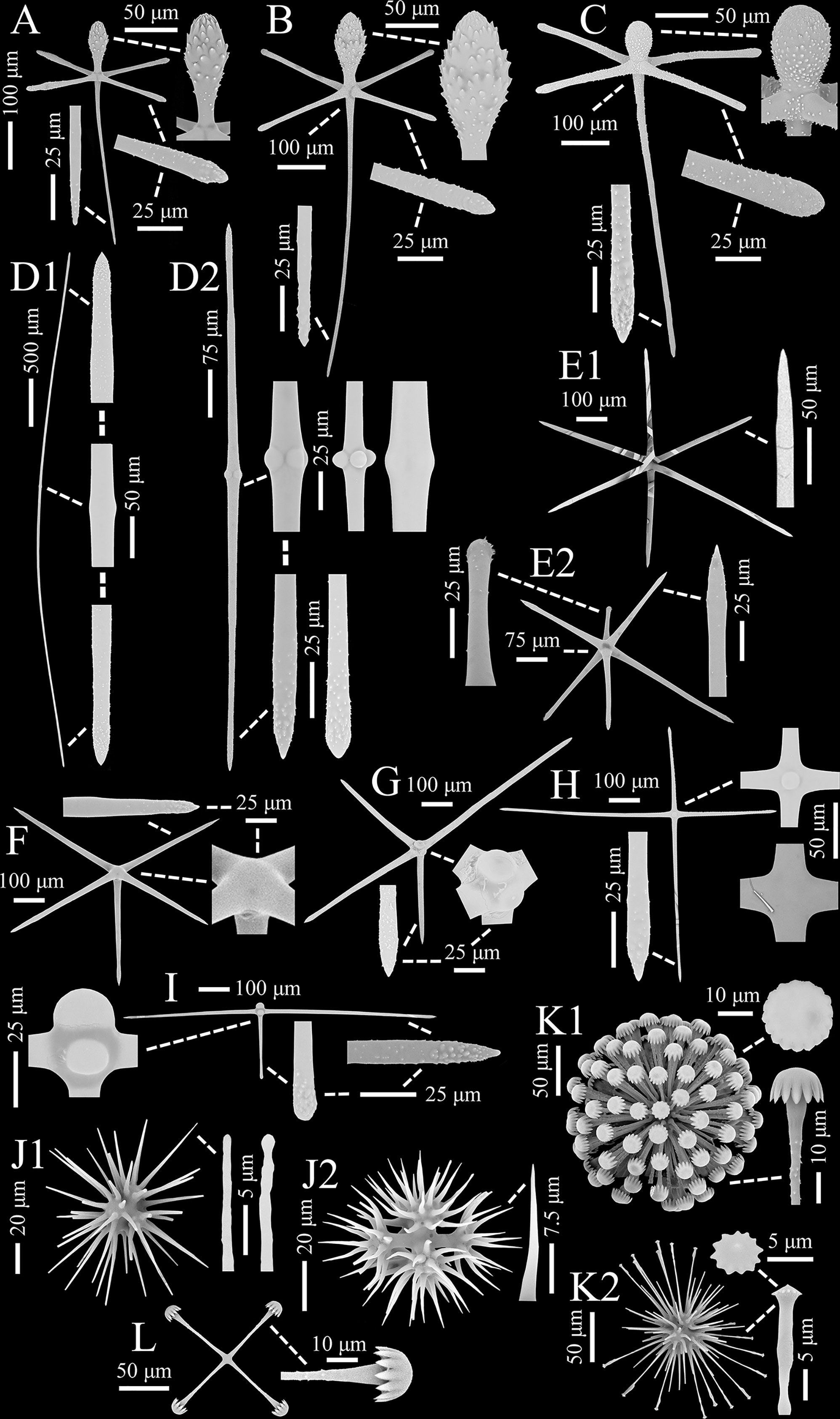
Figure 2 Flavovirens hemiglobus gen. et sp. nov., spicules of holotype (SEM). (A) Pinular dermal hexactin, whole and enlarged ray ends. (B) Pinular atrial hexactin, whole and enlarged ray ends. (C) Pinular marginal hexactin, whole and enlarged ray ends. (D) Two types of choanosomal diactins, whole and enlarged tips and center. (E) Two types of choanosomal hexactins, whole and enlarged ray ends. (F) Choanosomal pentacin, whole and enlarged tip and cross center. (G) Choanosomal tetractin, whole and enlarged tip and cross center. (H) Choanosomal stauractin, whole and enlarged tip and cross centers. (I) Choanosomal triactin, whole and enlarged tips and cross center. (J) Two examples of oxyhexasters, whole and enlarged secondary ray tips. (K) Two types of discohexasters, whole and enlarged secondary ray tip and terminal disc. (L) Staurodisc, whole and enlarged ray tip.
Pinular dermal hexactins (Figure 2A) are spined in the ray ends but smooth in the cross center and most of the tangential and proximal rays. The pinular ray is oval or clavate, 28–(98)–148 µm long, 1.3–(2.6)–5.2 times larger than its maximum width; the tangential rays have bluntly pointed, clavate or spindle-like ray tips, usually inflated near the tips, 153–(203)–285 µm long; the proximal ray is usually long and tapers to a bluntly conical ray rip, up to three times longer than the tangential rays, while sometimes short with a clavate ray tip, less than half of the tangential rays; the proximal ray is 6–(9)–15 µm thick, slightly thinner than the tangential rays. In addition, there may be more than one pinular ray in a single pinular hexactin or one pinular ray and one rough clavate ray. Pinular atrial hexactins (Figure 2B) have the same form and ray ornamentation as the dermal hexactins but are slightly larger in dimension. Furthermore, the pinular hexactins in the margin of the dermal and atrial surfaces are quite different from the dermal and atrial ones. Pinular marginal hexactins (Figure 2C) are microspined over the whole body and may be sparsely spined or smooth in the middle of the proximal ray; the pinular ray is club-like with very short thorns; they are larger in dimension with more robust tangential and proximal rays, approximately 1.5 times thicker than the dermal and atrial rays.
Choanosomal diactins (Figure 2D), slightly curved or straight, have rough terminal ends and a smooth center. They occur as two types in different forms and sizes. The larger one (diactin I; Figure 2D1), 1750–(3770)–7070 µm long and 14–(25)–45 µm thick, has conically pointed terminal ends and inconspicuous central swellings. The smaller one (diactin II; Figure 2D2), one-third as long as and half the width of the larger one, has conically pointed, rounded or seldomly clavated terminal ends, and the shapes of their centers vary with inconspicuous or four swellings. Choanosomal hexactins (Figure 2E) are smooth in the cross center but slightly rough in the ray tips. Rays of choanosomal hexactins usually taper to sharply pointed tips, forming a regular form of hexactins (hexactin I; Figure 2E1), 250–(346)–436 µm in ray length and 11–(18)–26 µm in ray width. However, one ray therein may occur with a clavate tip (hexactin II; Figure 2E2), which is 64–(114)–235 µm in ray length, approximately one-third as long as the other rays with sharply pointed tips. Choanosomal pentactins (Figure 2F) have the same ray shape and ornamentation and similar dimensions as regular choanosomal hexactins. They are smooth or rarely microspined and flat in the cross center without swelling. Choanosomal tetractins (Figure 2G) have hemispherical swellings in the smooth cross center and sparsely spined ray ends tapering to sharply pointed tips. Their dimensions are similar to those of choanosomal stauractins. Choanosomal stauractins (Figure 2H) are smooth in the cross center, sometimes with hemispherical swelling, while microspined in ray ends with sharply pointed tips. Each ray in a single stauractin is unequally long, with an average of 406–(498)–620 µm in length and 13–(19)–22 µm in width. Choanosomal triactins (Figure 2I) have the same form and ornamentation as tetractins, but sometimes the proximal ray may be short and end in a rounded tip. Their rays are approximately 15 µm in average width, slightly thinner than tetractins, and almost the same length as tetractins.
Microscleres
Microscleres are common oxyhexasters (>51.9%) and discohexasters (two types, together >44.2%) and rare staurodiscs (<3.9%; Figures 2J–L; Table 1).
Oxyhexasters (Figure 2J) are entirely smooth and vary greatly in size, of which the whole diameter ranges from 52 to 193 µm with an average of 116 µm. The short primary rays support 8–12 secondary rays that diverge and are straight or slightly curved. Secondary rays vary greatly in length, ranging from 18 to 74 µm with an average of 43 µm. The longer secondary rays taper gently and usually end in bluntly pointed tips or rarely spherical knobs (Figure 2J1), while the shorter secondary rays quickly taper to sharply pointed tips (Figure 2J2). The secondary rays are thin, 1–(2)–3 µm in width.
Discohexasters (Figure 2K) are spherical with very short, smooth primary rays and sparsely spined or smooth, straight secondary rays; they occur in two types. The more common type (discohexaster I; Figure 2K1), 160–(195)–223 µm in diameter, is in the general high-density form of discasters; the primary rays are smooth and bear approximately 25 secondary rays per primary ray; the secondary rays are sparsely microspined and 3–(3)–4 µm in width; the terminal discs are 15–(18)–20 µm in diameter, with 11–17 well-developed marginal teeth. The uncommon type (discohexaster II; Figure 2K2) features thinner secondary rays and smaller terminal discs; the primary rays bear approximately 20 secondary rays on each primary ray; the secondary rays below the discs are usually swollen, while most of the secondary rays are thin, approximately 2 µm in width; the terminal discs are very small, 3–(4)–5 µm in diameter, with 12–13 undeveloped marginal teeth. The two types of discohexasters are similar in their diameters, which are larger than those of oxyhexasters.
Staurodiscs (Figure 2L) are large with a maximum diameter of 160 µm or small with a minimum diameter of 45 µm; their terminal discs are in the same form as those of common discohexasters with well-developed marginal teeth.
Ecology
Flavovirens hemiglobus gen. et sp. nov. is distributed on the Ko-Hakucho Guyot in the northwestern Pacific seamounts (the holotype was collected at a depth of 2716 m). The bottom disc of the long peduncle was attached to hard substrates.
Etymology
The new species name hemiglobus refers to the hemiglobular body.
Remarks
The new specimen described here has a hemiglobular body with a wide, upright opening and a moderately long peduncle (approximately three times longer than the body head) with a plate-like base attached to hard substrates. It bears diactins as the main choanosomal spicules, plus uncommon hexactins, pentactins, stauractins, tetractins and triactins. Therefore, it is clearly attributed to the subfamily Bolosominae of the family Euplectellidae. However, the new specimen cannot be accommodated in any of the currently accepted genera in this subfamily. It is distinguished from other bolosomines by the set of microscleres present, namely, predominating oxyhexasters and discasters, rare discohexasters and staurodiscs (described above). Thus, we propose a new monospecific genus within Bolosominae to include Flavovirens hemiglobus gen. et sp. nov.
Systematics of New Species of Euretidae
Phylum Porifera (Grant, 1836)
Class Hexactinellida (Schmidt, 1870)
Subclass Hexasterophora (Schulze, 1886)
Order Sceptrulophora (Mehl, 1992)
Family Euretidae (Zittel, 1877)
Subfamily Chonelasmatinae (Schrammen, 1912)
Genus Chonelasma (Schulze, 1886)
Type species. Chonelasma lamella (Schulze, 1886)
Chonelasma tyloscopulatum sp. nov. (Figures 3, 4; Table 2).
Material Examined
Holotype (Figure 3): SIO-POR-294 (original number: DY61-ROV01a-B09), SRSIO, Hailong III ROV DY61/I, dive ROV01a, collected by Chengcheng Shen, Sep. 16, 2020, on the Ko-Hakucho Guyot in the northwestern Pacific Ocean (23.5037°N, 148.5735°E), depth of 2425 m, stored in 95% ethanol.
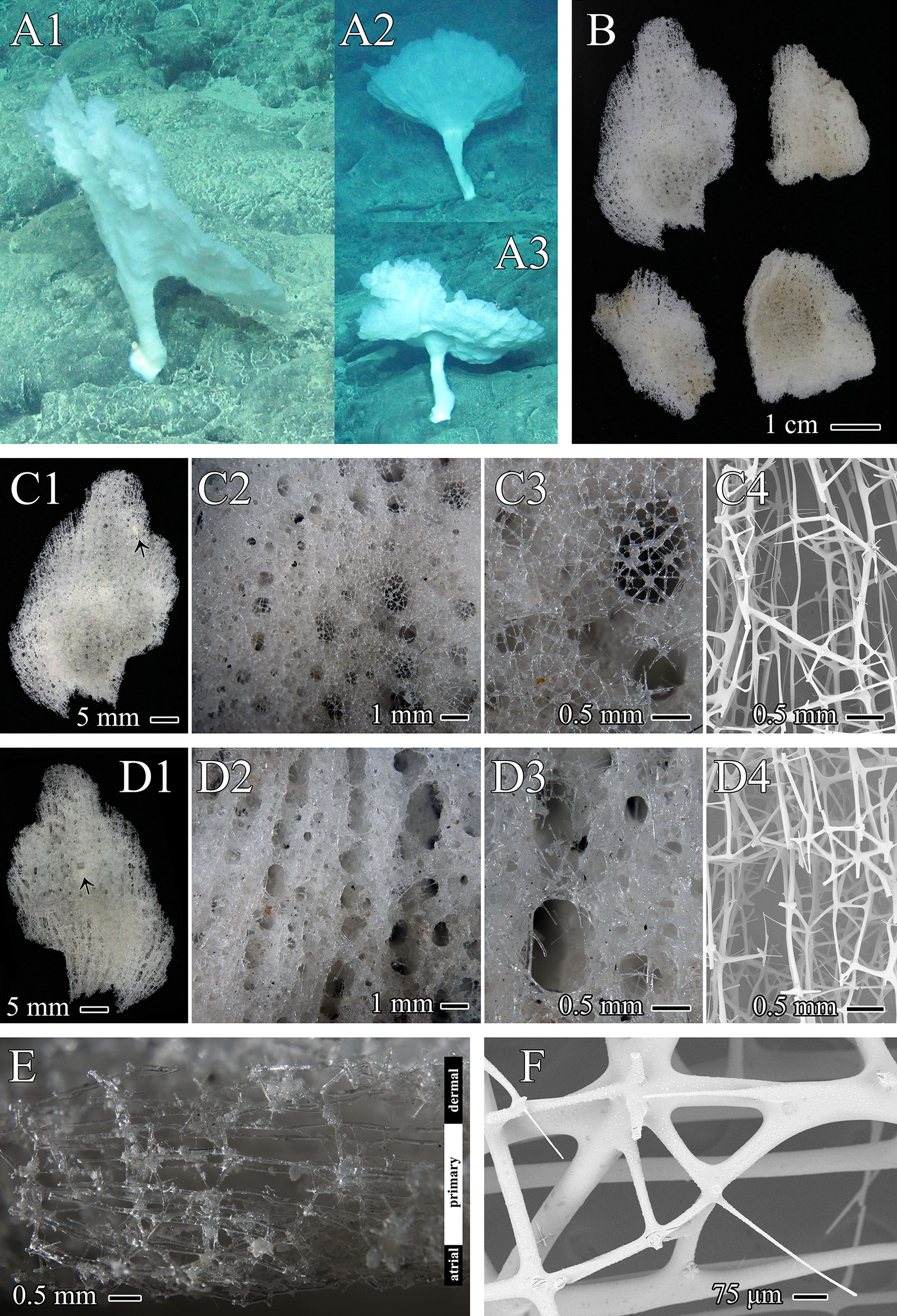
Figure 3 Chonelasma tyloscopulatum sp. nov., specimen, surfaces and skeletons of holotype. (A) In situ images of holotype (A1) and two other same morphotypes (A2, A3). (B) Whole collected specimen of holotype including four segments (dermal surface upward). (C) Dermal surface, close-up images in different scales with epirhyses in surface view (C1–C3), symbiotic anthozoan indicated by an arrowhead (C1) and SEM of cleaned skeleton (C4), growth margin upward. (D) Atrial surface, close-up images in different scales with aporhyses in surface view (D1–D3), symbiotic anthozoan indicated by an arrowhead (D1) and SEM of cleaned skeleton (D4), growth margin upward. (E) Vertical-longitudinal section of the dictyonal framework, consisting of dermal cortex, primary middle layer and atrial cortex, dermal side upward. (F) Close-up view of dermal cortex with digitate spurs (SEM).
Description
The specimen was collected as four slightly undulating, plate-like fragments from a funnel-like body form (Figures 3A, B). The funnel-shaped body protrudes from a cylindrical peduncle (Figure 3A). The preserved fragments are 4.4 cm×2.8 cm in maximum size and 2.0–3.8 mm in thickness. The texture is hard and fragile. Dermal and atrial surfaces are smooth (Figures 3C, D). Channels (epirhyses and aporhyses) are observable on both surfaces. Epirhyses, approximately 1.9 mm×1.1 mm in maximum size, are covered by a porous tissue membrane (Figures 3C2, C3). Aporhyses, approximately 2.6 mm×1.4 mm in maximum size, are arranged longitudinally, most of which lack lattice-like covers (Figures 3D2, D3). The color in situ is white (Figure 3A); on the desk and preserved, it is light beige (Figure 3B).
Skeleton
The choanosomal skeleton is a three-layered dictyonal framework consisting of a primary middle layer with elongated rectangular meshes and two irregularly meshed cortical layers on both the dermal and atrial sides (Figures 3C4, D4, E). Dictyonal skeletons are often sparsely spined and attached by rough, digitate spurs (Figure 3F). The ectosomal skeleton is composed of a lattice of loose pentactins overlying all choanosomal framework surfaces and entrances to epirhyses but not most of the openings of aporhyses (Figures 3C3, D3).
Megascleres
Megascleres of the body are surface pentactins, scopules (two types) and uncinates (Figures 4A–C; Table 2).
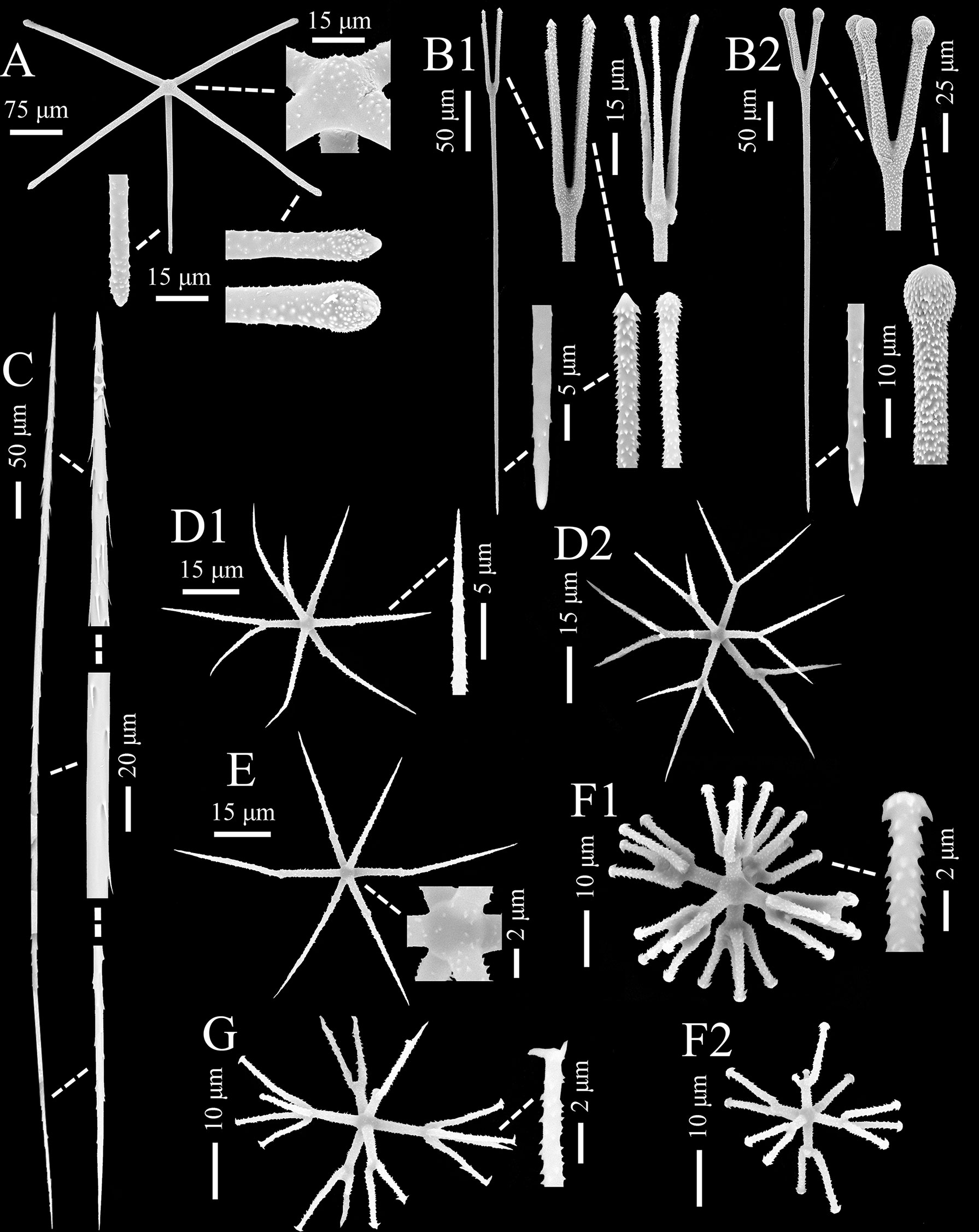
Figure 4 Chonelasma tyloscopulatum sp. nov., spicules of holotype (SEM). (A) Surface pentactin, whole and enlarged ray ends and cross center. (B) Two types of scopules, whole and enlarged heads and tips. (C) Uncinate, whole and enlarged tips and center. (D) Hemioxyhexaster (D1) and oxyhexaster (D2), whole and enlarged ray end. (E) Oxyhexactin, whole and enlarged cross center. (F) Discohexaster (F1) and hemidiscohexaster (F2), whole and enlarged secondary ray end. (G) Onycho-tipped hexaster, whole and enlarged secondary ray end.
The surface pentactins (Figure 4A) are in regular form with slight or rare hemispherical swelling in the cross center. They are uniformly microspined over the whole body and similar on both dermal and atrial surfaces. The tangential rays taper to bluntly conical or clavate ray tips, 166–(272)–404 µm in length, 6–(12)–21 µm in width; the proximal ray is slightly shorter and thinner than the tangential rays, 93–(228)–350 µm in length and 6–(11)–18 µm in width, with a tapering and bluntly conical tip.
Scopules (Figure 4B) occur as two types in different forms and sizes. The smaller one (scopule I; Figure 4B1), 352–(422)–545 µm in total length and 3–(5)–6 µm in shaft width, is in the form of strongyloscopules; its narrow or wide neck carries four or rarely three straight or slightly curved tines ending in triangular or rounded tips; the shaft is sparsely spined ending in a bluntly pointed tip. The larger one (scopule II; Figure 4B2) is in the form of tyloscopules, 469–(550)–644 µm in total length, and almost twice as wide as the smaller one in shaft width. The narrow neck bears four or rarely five straight or slightly curved tines ending in spherical knobs; the tines are profusely covered with fine recurved spines, but the distal surfaces of the knobs are bare; most of the shaft is sparsely spined ending in an abrupt sharp tip.
Uncinates (Figure 4C) are generally straight and sometimes slightly curved, 610–(1000)–1250 µm long and 3–(6)–10 µm thick, with barbs curving out from the spicule surface. One end is full of well-developed barbs, which are oriented toward the other end; the barbs on the other end are undeveloped and similar to forward-curved spines. Both tips are sharply pointed.
Microscleres
Microscleres are common hemioxyhexasters, rare oxyhexasters, a number of oxyhexactins, common discohexasters, rare hemidiscohexasters and onychohexasters (Figures 4D–G; Table 2).
Hemioxyhexasters and oxyhexasters (Figure 4D) are 59–(77)–100 µm in diameter; the cross center is spherical and spined; the primary and secondary rays are entirely covered by short, relined hooks; hemioxyhexasters have 1–5 rays branched, and they bear two or rarely three thin, straight or out-curved secondary rays tapering to sharply pointed tips; primary rays are 9–(13)–19 µm long, and approximately half of the secondary rays (primary to secondary ray length ratio ranging from 0.3 to 0.7). Oxyhexactins (Figure 4E) have the same dimensions, ray shape and ornamentation as hemioxyhexasters.
Discohexasters and hemidiscohexasters (Figure 4F) are stellate in form. They have the same spherical cross center and ornamentation as those of the hemioxyhexasters, but they are smaller in dimensions, 28–(39)–50 µm in diameter, approximately half the diameter of the oxy-tipped one; hemidiscohexasters usually have five rays branched, and they bear 2–7 straight or slightly sigmoid secondary rays ending in small discs with about eight marginal teeth; primary rays are 4–(6)–12 µm long, approximately half of the secondary rays (primary to secondary ray length ratio ranging from 0.3 to 0.8); primary rays are relatively robust, with 2–(2)–3 µm in width.
In addition, only one onycho-tipped hexaster (Figure 4G) was observed, which has the same dimension, form and ray ornamentation as those of the discohexasters. Its terminal ray tips are onychoid or pointed, and the onychoid tips are distinct with 1–3 claws.
Ecology
Chonelasma tyloscopulatum sp. nov. is distributed on the Ko-Hakucho Guyot in the northwestern Pacific Ocean (the holotype was collected at a depth of 2425 m). The bottom disc of the short peduncle is attached to hard substrates. Small anthozoans were found both in the dermal and arial cavities, which are probably symbionts (Figures 3C1, D1), and this scenario has also been found in other euretid specimens (Reiswig and Kelly, 2011).
Etymology
The new species name tyloscopulatum refers to the large scopules (scopule II), which are in the form of tyloscopules and have not been observed in other valid species of this genus to date.
Remarks
The new specimen is plate-like in fragments with body form of a single-wall funnel; its firm body wall is supported by a three-layered dictyonal framework; dermalia and atrialia are pentactins; scopules and uncinates present; microscleres are predominantly oxy- and disco-tipped; therefore, this new specimen is attributed to the family Euretidae. Therein, due to its funnel-like body form without dichotomous branching tubes or lateral oscula, the new specimen belongs to the subfamily Chonelasmatinae. Furthermore, its body walls consisting of a middle primary layer with elongated rectangular meshes and two irregular-meshed cortical layers with epirhyses on dermal side or aporhyses on atrial side, its spiny pentactins on both surfaces, and its microscleres including hemioxyhexasters/oxyhexasters, discohexasters/hemidiscohexasters and rare onychohexasters support its assignment to the genus Chonelasma. It differs from each of the 11 previously recognized valid species by significant features of spiculation.
The pentactine surface spicules prevent its assignment to C. hamatum (Schulze, 1886); C. doederleini (Schulze, 1886); C. chathamense (Reiswig and Kelly, 2011); or C. oreia (Reiswig, 2014), each of which has hexactine surface spicules. The new specimen bears both oxy- and disco-tipped hexasters or hemihexasters as common microscleres. However, disco-tipped microscleres are absent or scarce in C. lamella (Schulze, 1886); C. choanoides (Schulze and Kirkpatrick, 1910); or C. glaciale (Reiswig and Kelly, 2011); oxy-tipped microscleres are absent or scarce in C. ijimai (Topsent, 1901); C. australe (Reiswig and Kelly, 2011); or C. biscopulatum (Reiswig and Kelly, 2011). The new specimen cannot be assigned to C. bispinula (Kersken et al., 2019), since C. bispinula has only hemihexasters as microscleres with two rudimentary primary rays lying in one axis, and this feature is more various in the new specimen; in addition, the pentactins of the new specimen are finely spined, but C. bispinula is covered by large spines; the new specimen has two types of scopules, while C. bispinula has only one type; the body size of C. bispinula, less than 6 cm in height, is much smaller than those of the other valid species as well as the new specimen.
It should be noted that the tyloscopules have not been observed in other valid species of the genus Chonelasma to date. The presence of tyloscopules combined with oxyhexasters and discohexasters has been reported in the genera Periphragella (belonging to the subfamily Chonelasmatinae) and Lefroyella (belonging to the subfamily Euretinae). Despite of their similarity in funnel-like body form, the new species clearly cannot be assigned to these two genera due to its absence of dichotomous branching tubes or lateral oscula. Also, it should be noted that the new species does not group with C. bispinula or Lefroyella decora in the phylogenetic tree (described below; Figure 5).
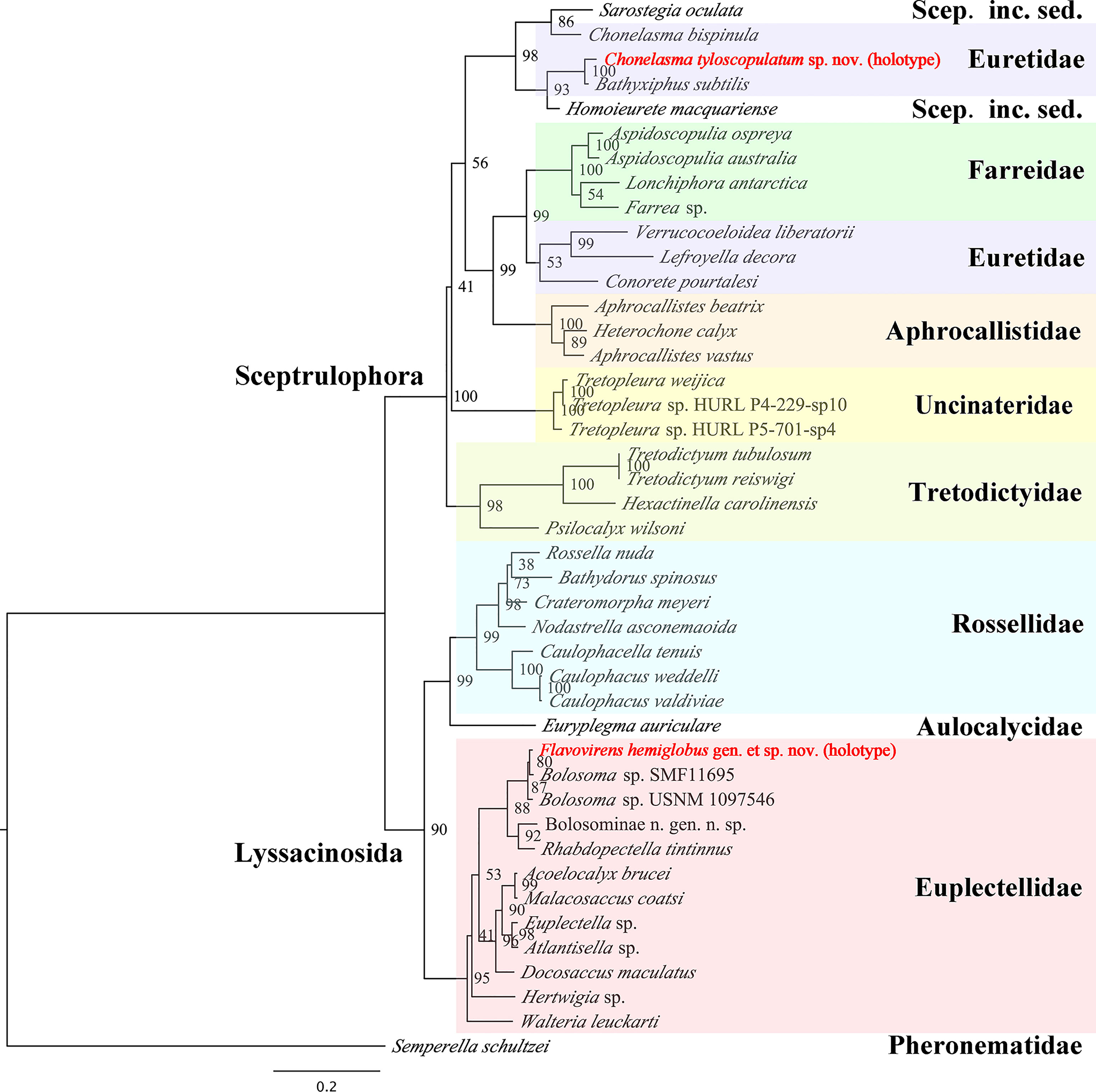
Figure 5 Maximum-likelihood phylogenetic tree of 43 species of hexactinellid sponges inferred from the concatenated alignment of four molecular markers (18S rDNA, 28S rDNA, 16S rDNA sequences and COI gene) with RAxML. Scep. inc. sed. refers to Sceptrulophora incertae sedis. Bootstrap values are shown next to nodes (based on 1000 replicates).
Molecular Phylogenetic Analysis of New Sponge Species
The lengths of 18S rDNA, 28S rDNA, 16S rDNA sequences and COI gene were confirmed by universal PCR primers as noted in the Methods section. The sequences of the PCR primers were located in the relevant base position of the marker genes correctly. The dataset of 18S rDNA, 28S rDNA, 16S rDNA partial sequence and COI complete sequence of Flavovirens hemiglobus gen. et sp. nov. (holotype) contained 1954 bp, 1233 bp, 494 bp and 1274 bp, respectively (deposited in GenBank with accession numbers OK245429, OK253063, OK245431 and OK255492, respectively); the same four marker genes of Chonelasma tyloscopulatum sp. nov. (holotype) contained 735 bp, 1239 bp, 540 bp and 709 bp, respectively (deposited in GenBank with accession numbers OK245428, OK253062, OK245430 and OK254902, respectively). The ML tree was reconstructed based on the concatenated alignment file of the two new species and 41 other reference species of hexactinellid sponges (Figure 5).
The phylogenetic relationship was largely congruent to that of glass sponges in a previous study (Dohrmann, 2019). Flavovirens hemiglobus gen. et sp. nov. grouped well with the other Bolosominae. It should be noted that this new genus grouped with the other two specimens which were assigned to undetermined species of genus Bolosoma but no morphological evidence present. More molecular and morphological data should be collected to further study the relationship between the new genus and the genus Bolosoma. For the euretid genera, it is confirmed that there are currently two separate branches (Dohrmann, 2019); Chonelasma tyloscopulatum sp. nov. grouped with the branch consisting of Bathyxiphus and Chonelasma and mixing with Homoieurete and Sarostegia from Sceptrulophora incertae sedis. Moreover, Chonelasma tyloscopulatum sp. nov. grouped with another genus Bathyxiphus rather than the congeneric species (C. bispinula SMF 12084), which showed that this genus currently is not monophyletic. In addition to the difference in spiculation, especially for the presence of tyloscopules in the new species, it indicated that either Chonelasma is not a valid genus, or the new species might have to be classified in a new genus in future revisions.
Conclusions
According to both morphological and molecular assessments, these two specimens described here are both clearly new to science, with one species attributed to a new genus of the family Euplectellidae (named Flavovirens hemiglobus gen. et sp. nov.) and the other species to the genus Chonelasma of the family Euretidae (named Chonelasma tyloscopulatum sp. nov.). It should be noted that the new genus Flavovirens grouped with two undescribed specimens (currently assigned to the genus Bolosoma); the relationship of the two genera should be further studied with more molecular and morphological data. Also, the new Chonelasma species, with the presence of tyloscopules, did not group with the congeneric species (C. bispinula SMF 12084), implying that Chonelasma may be not a valid genus. Otherwise, the new species might have to be classified in a new genus in future revisions. More Chonelasma spp. should be sampled for molecular systematics to clarify this issue.
As important components of deep-sea ecosystems, hexactinellid sponges have still not been sufficiently collected, described, or identified. The two specimens collected in this study as the representative morphotypes of sponges were both identified new to science, which further indicated the shortage of sampling efforts in the deep sea. Limited sampling efforts should be conducted to consider both the morphological typicality of samples and their diversity, so as to assist in improving the understanding of the biodiversity of seamount sponges and to provide a reference status for the conservation and management of vulnerable marine ecosystems on seamounts.
Data Availability Statement
The datasets presented in this study can be found in online repositories. The names of the repository/repositories and accession number(s) can be found below:
NCBI (accession: OK245429, OK253063, OK245431, OK255492, OK245428, OK253062, OK245430 and OK254902).
Author Contributions
CS and CW conceptualized the study. CS, HC, and DZ (chief scientist) participated in the research cruise and collected specimens. CS performed the sponge identification. HC performed the molecular phylogenetic analysis. CS and CW wrote the manuscript with contributions from HC and DZ. All authors contributed to the article and approved the submitted version.
Funding
This study was funded by the National Natural Science Foundation of China (Nos. 41706149 and 42076135), the Scientific Research Fund of the Second Institute of Oceanography, MNR (No. JG1716), the Foundation of China Ocean Mineral Resources Research and Development Association (No. DY135-E2-2-03).
Conflict of Interest
The authors declare that the research was conducted in the absence of any commercial or financial relationships that could be construed as a potential conflict of interest.
Publisher’s Note
All claims expressed in this article are solely those of the authors and do not necessarily represent those of their affiliated organizations, or those of the publisher, the editors and the reviewers. Any product that may be evaluated in this article, or claim that may be made by its manufacturer, is not guaranteed or endorsed by the publisher.
Acknowledgments
We thank all the scientists and crew on the R/V “Da Yang Yi Hao” and the ROV Hailong III team, for their dedicated research work and collection of deep-sea specimens. We thank the three reviewers for their constructive suggestions.
Supplementary Material
The Supplementary Material for this article can be found online at: https://www.frontiersin.org/articles/10.3389/fmars.2022.852498/full#supplementary-material
References
Bell J. J. (2008). The Functional Roles of Marine Sponges. Estuar. Coast. Shelf Sci. 79 (3), 341–353. doi: 10.1016/j.ecss.2008.05.002
Castello-Branco C., Collins A. G., Hajdu E. (2020). A Collection of Hexactinellids (Porifera) From the Deep South Atlantic and North Pacific: New Genus, New Species and New Records. PeerJ 8, e9431. doi: 10.7717/peerj.9431
Clark M. R., Kelley C., Baco A., Rowden A. A. (2011). “Fauna of Cobalt-Rich Ferromanganese Crust Seamounts,” in International Seabed Authority Technical Study No. 8 (Kingston, Jamaica: International Seabed Authority).
de Voogd N. J., Alvarez B., Boury-Esnault N., Carballo J. L., Cárdenas P., Díaz M.-C., et al. (2022) World Porifera Database. Available at: https://www.marinespecies.org/porifera
Dohrmann M., Janussen D., Reitner J., Collins A. G., Wörheide G. (2008). Phylogeny and Evolution of Glass Sponges (Porifera, Hexactinellida). Syst. Biol. 57 (3), 388–405. doi: 10.1080/10635150802161088
Dohrmann M. (2016). Symplectella Rowi (Porifera: Hexactinellida: Lyssacinosida) Is a Rossellid, Not a Euplectellid. J. Mar. Biol. Assoc. United Kingdom 96 (2), 291–295. doi: 10.1017/S0025315414001805
Dohrmann M. (2019). Progress in Glass Sponge Phylogenetics: A Comment on Kersken et al. (2018). Hydrobiologia 843, 51–59. doi: 10.1007/s10750-018-3708-7
Dohrmann M., Kelley C., Kelly M., Pisera A., Hooper J. N. A., Reiswig H. M. (2017). An Integrative Systematic Framework Helps to Reconstruct Skeletal Evolution of Glass Sponges (Porifera, Hexactinellida). Front. Zool. 14, 18. doi: 10.1186/s12983-017-0191-3
Folmer O., Black M., Hoeh W., Lutz R., Vrijenhoek R. (1994). DNA Primers for Amplification of Mitochondrial Cytochrome C Oxidase Subunit I From Diverse Metazoan Invertebrates. Mol. Mar. Biol. Biotechnol. 3 (5), 294–299.
Grant R. E. (1836). “Animal Kingdom,” in The Cyclopaedia of Anatomy and Physiology, vol. 1 . Ed. Todd R. B. (London: Sherwood, Gilbert, and Piper), 107–118.
Gray J. E. (1867). Notes on the Arrangement of Sponges, With the Descriptions of Some New Genera. Proc. Zool. Soc. Lond. 1867 (2), 492–558, pls XXVII-XXVIII.
Gray J. E. (1872). On a New Genus of Hexaradiate and Other Sponges Discovered in the Philippine Islands by Dr. A.B. Meyer. Ann. Mag. Nat. Hist. 10 (56), 134–139. doi: 10.1080/00222937208696659
Hajdu E., Castello-Branco C., Lopes D. A., Sumida P. Y. G., Perez J. A. A. (2017). Deep-Sea Dives Reveal an Unexpected Hexactinellid Sponge Garden on the Rio Grande Rise (SW Atlantic). A Mimicking Habitat? Deep Sea Res. Part II: Topical Stud. Oceanogr. 146, 93–100. doi: 10.1016/j.dsr2.2017.11.009
Kersken D., Janussen D., Martínez A. P. (2019). Deep-Sea Glass Sponges (Hexactinellida) From Polymetallic Nodule Fields in the Clarion-Clipperton Fracture Zone (CCFZ), Northeastern Pacific: Part II—Hexasterophora. Mar. Biodivers. 49, 947–987. doi: 10.1007/s12526-018-0880-y
Lanave C., Preparata G., Saccone C., Serio G. (1984). A New Method for Calculating Evolutionary Substitution Rates. J. Mol. Evol. 20, 86–93. doi: 10.1007/BF02101990
Li D., Liu C., Luo R., Sadakane K., Lam T. (2015). MEGAHIT: An Ultra-Fast Single-Node Solution for Large and Complex Metagenomics Assembly via Succinct De Bruijn Graph. Bioinformatics 31 (10), 1674–1676. doi: 10.1093/bioinformatics/btv033
Li D., Luo R., Liu C., Leung C., Ting H., Sadakane K., et al. (2016). MEGAHIT V1.0: A Fast and Scalable Metagenome Assembler Driven by Advanced Methodologies and Community Practices. Methods 102 (1), 3–11. doi: 10.1016/j.ymeth.2016.02.020
Mehl D. (1992). Die Entwicklung Der Hexactinellida Seit Dem Mesozoikum. Paläobiol. Phylogenie Evolutionsök. Berl. Geowissenschaftliche Abh. Reihe E (Paläobiol.) 2, 1–164.
Reid R. E. H. (1958). A Monograph of the Upper Cretaceous Hexactinellida of Great Britain and Northern Ireland. Part I. Paleontogr. Soc. (Monogr.) 111, i–xlvi.
Reiswig H. M. (2014). Six New Species of Glass Sponges (Porifera: Hexactinellida) From the North-Eastern Pacific Ocean. J. Mar. Biol. Assoc. United Kingdom 94 (2), 267–284. doi: 10.1017/s0025315413000210
Reiswig H. M., Dohrmann M. (2014). Three New Species of Glass Sponges (Porifera: Hexactinellida) From the West Indies, and Molecular Phylogenetics of Euretidae and Auloplacidae (Sceptrulophora). Zool. J. Linn. Soc. 171 (2), 233–253. doi: 10.1111/zoj.12138
Reiswig H. M., Kelly M. (2011). The Marine Fauna of New Zealand: Hexasterophoran Glass Sponges of New Zealand (Porifera: Hexactinellida: Hexasterophora): Orders Hexactinosida, Aulocalycoida and Lychniscosida. NIWA Biodivers. Memoirs 124, 1–176.
Reiswig H. M., Kelly M. (2018). The Marine Fauna of New Zealand. Euplectellid Glass Sponges (Hexactinellida, Lyssacinosida, Euplectellidae). NIWA Biodivers. Memoirs 130, 1–170.
Reiswig H. M., Stone R. P. (2013). New Glass Sponges (Porifera: Hexactinellida) From Deep Waters of the Central Aleutian Islands, Alaska. Zootaxa 3628, 1–64. doi: 10.11646/zootaxa.3628.1.1
Reiswig H. M., Wheeler B. (2002). “Family Euretidae Zittel 1877,” in Systema Porifera: A Guide to the Classification of Sponges. Eds. Hooper J. N. A., Van Soest R. W. M. (New York: Kluwer Academic/Plenum Publishers), 1301–1331.
Schmidt O. (1870). Grundzüge Einer Spongien-Fauna Des Atlantischen Gebietes (Leipzig: Wilhelm Engelmann), iii–iv 1–88, pls I-VI.
Schrammen A. (1912). Die Kieselspongien Der Oberen Kreide Von Nordwestdeutschland. II. Teil Triaxonia (Hexactinellida). Palaeontogr. 5 (supplement), 177–385, pls XXV-XLV.
Schulze F. E. (1886). Über Den Bau Und Das System Der Hexactinelliden. Abh. Königlichen Akad. Wiss. Berlin (Phys. Math. Classe), 1–97.
Schulze F. E. (1887). Report on the Hexactinellida Collected by H.M.S. ‘Challenger’ During the Years 1873–1876. Report on the Scientific Results of the Voyage of H.M.S. ‘Challenger’, 1873–1876. Zoology 21, 1–514.
Schulze F. E., Kirkpatrick R. (1910). Preliminary Notice on Hexactinellida of the Gauss-Expedition. Zool. Anz. 35 (9–10), 293–302.
Shen C., Cheng H., Zhang D., Lu B., Wang C. (2021). A New Species of the Glass Sponge Genus Walteria (Hexactinellida: Lyssacinosida: Euplectellidae) From Northwestern Pacific Seamounts, Providing a Biogenic Microhabitat in the Deep Sea. Acta Oceanol. Sin. 40 (12), 1–11. doi: 10.1007/s13131-021-1939-3
Shen C., Dohrmann M., Zhang D., Wang C. (2019). A New Glass Sponge Genus (Hexactinellida: Euplectellidae) From Abyssal Depth of the Yap Trench, Northwestern Pacific Ocean. Zootaxa 4567, 367–378. doi: 10.11646/zootaxa.4567.2.9
Silvestro D., Michalak I. (2012). RaxmlGUI: A Graphical Front-End for RAxML. Org. Diversity Evol. 12, 335–337. doi: 10.1007/s13127-011-0056-0
Stamatakis A. (2014). RAxML Version 8: A Tool for Phylogenetic Analysis and Post-Analysis of Large Phylogenies. Bioinformatics 30, 1312–1313. doi: 10.1093/bioinformatics/btu033
Tabachnick K. R. (2002). “Family Euplectellidae Gray 1867,” in Systema Porifera: A Guide to the Classification of Sponges. Eds. Hooper J. N. A., Van Soest R. W. M. (New York: Kluwer Academic/Plenum Publishers), 1388–1434.
Yang Z. (1994). Maximum Likelihood Phylogenetic Estimation From DNA Sequences With Variable Rates Over Sites: Approximate Methods. J. Mol. Evol. 39, 306–314. doi: 10.1007/BF00160154
Keywords: Euretidae, Euplectellidae, integrative taxonomy, Northwestern Pacific Ocean, seamount, Porifera
Citation: Shen C, Cheng H, Zhang D and Wang C (2022) Two New Species and One New Genus of Glass Sponges (Hexactinellida: Euplectellidae and Euretidae), From a Transect on a Seamount in the Northwestern Pacific Ocean. Front. Mar. Sci. 9:852498. doi: 10.3389/fmars.2022.852498
Received: 11 January 2022; Accepted: 07 April 2022;
Published: 19 May 2022.
Edited by:
Jose Angel Alvarez Perez, Universidade do Vale do Itajaí, BrazilReviewed by:
Konstantin Tabachnick, P.P. Shirshov Institute of Oceanology (RAS), RussiaMartin Dohrmann, Independent Scholar, Munich, Germany
Lin Gong, Institute of Oceanology (CAS), China
Copyright © 2022 Shen, Cheng, Zhang and Wang. This is an open-access article distributed under the terms of the Creative Commons Attribution License (CC BY). The use, distribution or reproduction in other forums is permitted, provided the original author(s) and the copyright owner(s) are credited and that the original publication in this journal is cited, in accordance with accepted academic practice. No use, distribution or reproduction is permitted which does not comply with these terms.
*Correspondence: Chunsheng Wang, wangsio@sio.org.cn