Trophic Ecology of Deep-Sea Megafauna in the Ultra-Oligotrophic Southeastern Mediterranean Sea
- National Institute of Oceanography, Israel Oceanographic and Limnological Research, Haifa, Israel
The trophic ecology of fourteen species of demersal fishes and six species of demersal decapod crustaceans from the continental slope and rise of the Southeastern Mediterranean Sea (SEMS) was examined using stable isotope analysis. Mean δ13C values among fish species varied by ca. 4.0‰, from -20.85‰ (Macroramphosus scolopax) to -16.57‰ and -16.89‰ (Conger conger and Centrophorus granulosus), showing an enrichment in 13C as a function of depth (200 – 1400 m). Mean δ13C values of the crustaceans showed smaller variation, between -18.54‰ (Aristeus antennatus) and -16.38‰ (Polycheles typhlops). This suggests a shift from pelagic to regenerated benthic carbon sources with depth. Benthic carbon regeneration is further supported by the low benthic-pelagic POM-δ13C values, averaging -24.7 ± 1.2‰, and the mixing model results, presenting relatively low contribution of epipelagic POM to the deep-sea fauna. Mean δ15N values of fish and crustacean species ranged 7.91 ± 0.36‰ to 11.36 ± 0.39‰ and 5.96 ± 0.24‰ to 7.73 ± 0.46‰, respectively, resulting in trophic position estimates, occupying the third and the fourth trophic levels. Thus, despite the proximity to the more productive areas of the shelf, low number of trophic levels (TL~1.0) and narrow isotopic niche breadths (SEAC<1) were observed for demersal crustaceans (TL = 2.94 ± 0.18) and fishes (TL = 3.62 ± 0.31) in the study area – probably due to the ultra-oligotrophic state of the SEMS resulting in limited carbon sources. Our results, which provide the first trophic description of deep-sea megafauna in the SEMS, offer insight into the carbon sources and food web structure of deep-sea ecosystems in oligotrophic marginal seas, and can be further used in ecological modeling and support the sustainable management of marine resources in the deep Levantine Sea.
Introduction
Deep-sea ecosystems cover much of the oceans seafloor and play a major role in large-scale biogeochemical cycles (Walsh, 1991; Drazen and Sutton, 2017). They provide ecosystem services that are important to humans, including carbon sequestration, nutrient recycling and burial, waste accumulation and fisheries production (Danovaro et al., 2008; Mengerink et al., 2014; Thurber et al., 2014). Recent studies have shown that an increasing number of stressors, including climate change (warming), deoxygenation, ocean acidification, as well as, overfishing, and natural resource extraction (e.g., Stramma et al., 2008; Yasuhara et al., 2008; Stramma et al., 2010; Helm et al., 2011; Tecchio et al., 2015) are expanding into deep environments, thus threatening the diversity and stability of deep-sea ecosystems. Consequently, studying the status of deep-sea communities and describing deep-sea ecosystem structures are currently gaining more and more attention.
Continental slopes account for ~11% of the total ocean floor (Ramirez-Llodra et al., 2010), connecting the shallow shelf productive areas with the abyssal plains along steep seabed gradients. Covering large bathymetric ranges (~ 200 – 2000 m), these dynamic habitats exhibit strong spatial differences in temperature, salinity, nutrient concentrations, sedimentological features, like organic carbon content, and consequently, in habitat suitability (Koslow, 1993; Gordon et al., 1995; Neat et al., 2008; Bergstad, 2013; Pajuelo et al., 2016). Bathyal habitats also support diverse deep-sea fauna (Gordon and Swan, 1997; Kelly et al., 1998; Menezes et al., 2006; Neat et al., 2008), even in ultra-oligotrophic basins, such as the easternmost Mediterranean Sea (Goren et al., 2008). In deep-sea benthic ecosystems, fish can play key ecological and biogeochemical roles (Drazen and Sutton, 2017) by regulating nutrient limitation and zooplankton populations (Hopkins and Gartner, 1992; Pakhomov et al., 1996).
Deep-sea benthic ecosystems largely rely on particulate organic matter (POM) that passively sinks from the surface waters or by lateral transport as a primary source of nutrients (Tecchio et al., 2013). Marine organisms that carry out vertical diel migrations through the water column (Trueman et al., 2014) and occasional sink of large animal carcasses is another important food source to deep-sea ecosystems (Smith and Baco, 2003). Each of these primary food sources may carry a distinct isotopic signature that reflects its origin, resulting from different chemo-physical processes. Thus, by knowing the isotopic composition of the food sources that fuels a specific food web, it is possible to reconstruct the trophic structure and dynamics of specific habitats (Post, 2002).
Stable isotope analysis (SIA) has been used successfully to study trophic level, important prey types, and trophic niche breadth in deep-sea ecosystems (e.g., Boyle et al., 2012; Shipley et al., 2017a). Nitrogen stable-isotope composition (δ15N) is used to determine the trophic position of an animal, as it preferentially fractionates as a function of its diet, where the heavy isotopes are retained in the consumers in respect to their prey by 2 – 4‰ (Post, 2002). Carbon stable isotopes (δ13C) fractionate much less with each trophic step (<1‰), but can be effectively used to infer basal sources of carbon. Moreover, SIA provides an integrated view of an organism’s diet over time-scales relevant to tissue turnover rates rather than digestion rates (Peterson and Fry, 1987; Post, 2002), thereby providing estimates of the trophic position of an organism within a specific food web.
Knowledge of food web structure and dynamics is key to our understanding of ecological communities and their functioning (Polis and Strong, 1996; Winemiller and Polis, 1996). This fundamental information is, however, lacking in many oceanographic regions, including the Southeastern Mediterranean Sea (hereafter, SEMS) (Parzanini et al., 2019) — one of the most oligotrophic, nutrient-impoverished marginal basin, worldwide (Kress et al., 2014). This basin exhibits extremely low open water primary production (~60 g C m−2 y−1) merely half of that measured in other oligotrophic areas of the ocean (Hazan et al., 2018), resulting in limited energy sources to its deep-sea habitats (Rahav et al., 2019). The greatest fraction of particulate flux to the SEMS deep-seafloor is transported laterally from the continental shelf at intermediate depths (Katz et al., 2020) and is considered to be highly refractory (Rubin-Blum et al., 2022). The SEMS, therefore, provides a miniature model of processes occurring in vast oligotrophic marginal seas, an ideal location to study food web structure and functioning under sever nutrient limitation. Furthermore, the SEMS is one of the regions where sea surface temperatures are rising at the fastest rates under recent climate changes (Sisma-Ventura et al., 2014; Ozer et al., 2017) and is one of most vulnerable marine regions to species invasions (Rilov and Galil, 2009), which have been also reported from deep-sea habitats (Galil et al., 2019). Understanding deep-sea community structure and functioning is of prime importance for developing better predictions regarding the ecological effects of future climate change. The main objective of this study was to elucidate the energy sources that sustain the deep-sea food-webs of the SEMS.
To date, much of the research describing the trophic ecology of the Eastern Mediterranean Sea has focused on zooplankton groups (Koppelmann et al., 2003; Koppelmann et al., 2009; Hannides et al., 2015; Protopapa et al., 2019), shallow rocky reefs (Fanelli et al., 2015), and on anthropogenically-influenced coastal environments (Grossowicz et al., 2019), while less attention has been paid to deep-sea fishes and crustaceans that occupy higher trophic levels. Here we used bulk carbon and nitrogen stable isotopes (δ13C and δ15N) of demersal and bathybenthic fishes and crustaceans from the southeast Mediterranean continental slope and rise. We explored potential factors that may explain the variability in stable isotope values across species. These data offer insights into the carbon sources and trophic complexity of deep-sea ecosystems in oligotrophic marginal seas.
Materials and Methods
Study Sites and Sampling Design
Sampling campaigns were conducted in the course of three oceanographic cruises during 2017 – 2019, as part of the national deep-water monitoring program of the Israeli Mediterranean Sea performed by Israel Oceanographic and Limnological Research (IOLR). Sampling sites were divided to three major benthic habitats: (1) the end of the continental shelf, with an average depth of 200 m; (2) the continental slope with depth range of 500 – 600 m; and (3) the deep bathyal plateau (continental rise) with depth range of 1000 – 1400 m (Figure 1). Specimens were collected onboard the R/V Bat-Galim, using a semi-balloon trawl net with an opening of eight meters and mesh size of 10 mm. Once the trawls were retrieved, specimens were sorted, enumerated, weighted and visually identified to species level. The total length (cm) of each specimen was recorded. Specimens for SIA were selected and frozen whole at –20°C until processed at the IOLR.
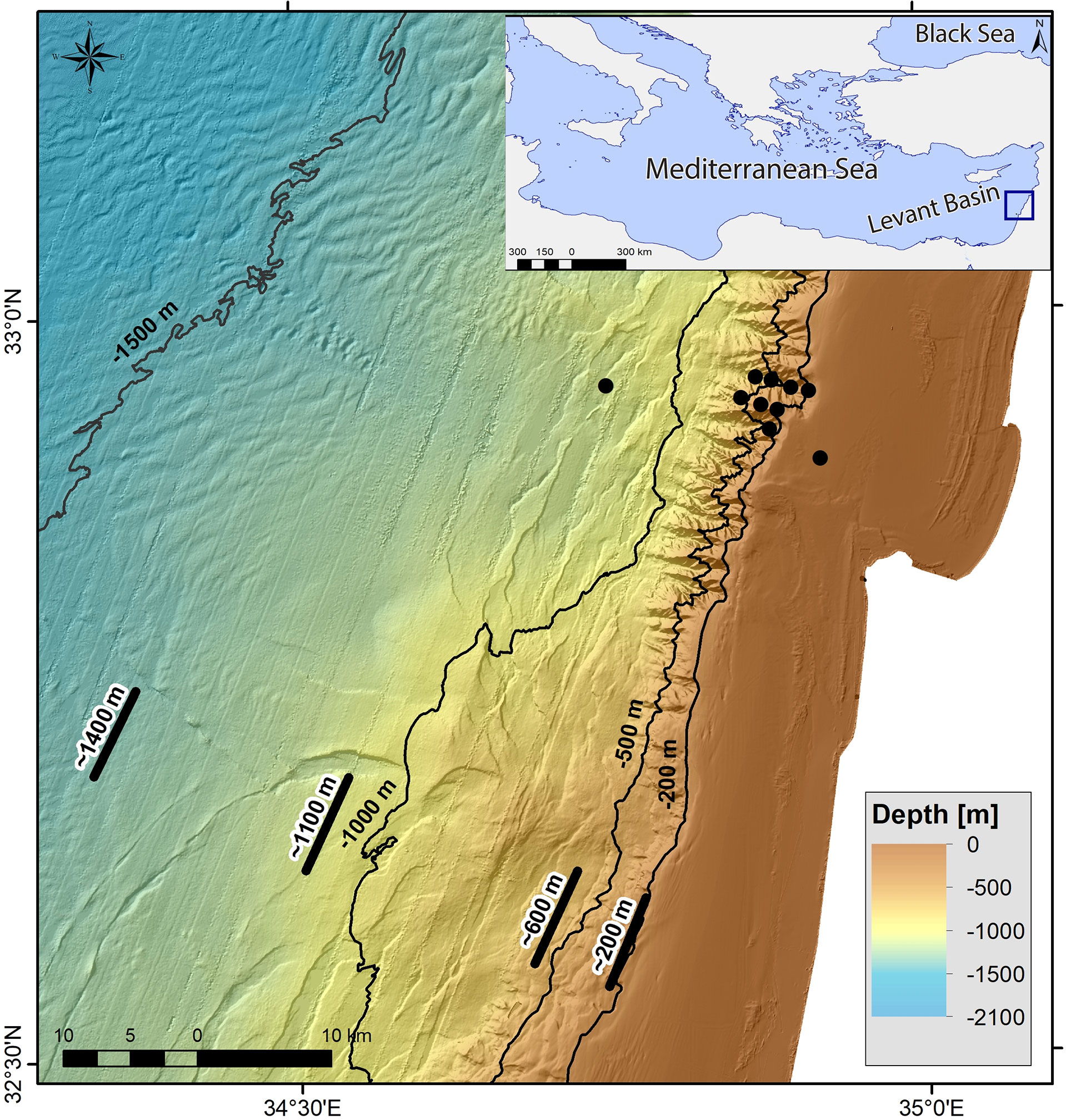
Figure 1 Map of sampling sites in the SEMS. The locations of POM samples are presented in black circles, and locations of fishes and crustaceans by trawl net are presented in black lines.
POM was collected during three research expeditions in winter 2018, summer 2020, and winter 2021, across the shelf, slope and rise of the SEMS (Figure 1 and Table 1). POM samples were collected throughout the water column using 8-L Niskin bottles. Water samples were then filtered on pre-combusted 47-mm glass fiber filters (Whatman) in duplicates at low pressure and dried at 60°C for 24 h prior to isotope analysis.
Stable Isotopes Analysis
SIA was conducted on 86 fish and 46 crustacean specimens as well as 77 POM samples (Table 1). White muscle tissue for SIA was dissected from the dorsal musculature of fishes and from the abdominal segment of the crustaceans. Samples were rinsed with deionized water, frozen, and lyophilized for 48 h. Freeze-dried samples were homogenized using a mortar and pestle, weighed, and shipped to the Stable Isotope Facility at Cornell University (USA) for SIA analysis. The isotopic composition of organic carbon and nitrogen was determined by the analysis of CO2 and N2 continuous-flow produced by combustion on a Carlo Erba NC2500 connected on-line to a DeltaV isotope ratio mass spectrometer coupled with a ConFlo III interface.
Measured stable isotope ratios are reported in the δ-notation, i.e., as the deviation in per mill (‰) from the international standards:
where, R represents the 15N/14N or 13C/12C ratio. Stable isotope data are expressed relative to international standards of Vienna PeeDee belemnite and atmospheric N2 for carbon and nitrogen, respectively. The analytical precision for the in-house standard was ± 0.04‰ [1σ] for both δ13C and δ15N. The C/N ratios of fishes and crustaceans in this study were low (species mean C/N ranged between 2.33 – 4.48; where in 97% of individuals C/N< 4.0, see Supplementary Figures 1, 2), suggesting that lipids did not significantly affect the δ13C interpretation (Post et al., 2007). Therefore, all data analyses were performed on uncorrected δ13C values. To determine if the isotopic signatures of POM samples changed with depth, we used collection depth to classify POM samples as epipelagic (0 – 200 m), mesopelagic (200 – 800 m), or bathypelagic (>800 m).
Data Analysis
We estimated and compared modal trophic position (TP) and 95% credibility interval (i.e., 95% of modeled estimates of TP) for each species using the R package tRophicPosition (version 0.7.7; Quezada-Romegialli et al., 2018) according to the following equation:
Where δ15Nc corresponds to the nitrogen stable isotope value from the consumer that the TP is estimated, δ15Nb represents the nitrogen stable isotope value of the baseline consumer; ΔN corresponds to the trophic discrimination factor (TDF) for nitrogen and λ the TP from baseline consumer. We used the average δ15N in zooplankton (3.9 ± 1.8‰) measured by Koppelmann et al. (2009) in the Eastern Levantine Basin as the baseline ( λ=2) using the oneBaseline model option and applied the trophic discrimination factor △15N of 3.15 ± 1.28‰, which was previously used to calculate the trophic level of meso- and bathypelagic fish (Valls et al., 2014; Richards et al., 2018).
Least-squares linear regression analysis was conducted for each species to explore the relationship between fish length and the δ13C and δ15N values. Spatial variation in δ13C and δ15N of both fishes and crustaceans was investigated using least-squares linear regression between stable isotopic values and depth. All statistical analyses were performed in R v. 4.0.5 (R Core Team, 2020).The trophic breadth of each species (n ≥5) and trophic similarity among species were assessed by calculating Standard Ellipse Area (SEA) using the R package SIBER v. 2.1.6 (Jackson et al., 2011; Jackson and Parnell, 2021). Size-corrected SEAs (SEAc) and Bayesian estimate of SEAs (SEAB) were calculated for each species, which adjusts for underestimation of ellipse area at small sample sizes and allows for inter-study comparison of ellipse sizes (Jackson et al., 2011). Fish and crustacean community metrics were calculated based on Layman et al. (2007).
Bayesian mixing models were applied using R package MixSIAR v. 3.1.12 (Stock et al., 2018; Stock et al., 2021) to estimate the relative contribution of shelf, slope, and deep-basin surface vs. bottom POM to each species. These models are sensitive to variable discrimination factors (Bond and Diamond, 2011; Olin et al., 2013), which may be influenced by diet (Caut et al., 2009), tissue type (Malpica-Cruz et al., 2012), temperature (Britton and Busst, 2018), and species-specific metabolic rates (Pecquerie et al., 2010). Since the modeled species are predators and do not feed directly on POM, the total trophic fractionation per species was calculated as follows:
Where, TEFspecies is the total trophic fractionation in the consumer relative to its basal source, TEFTL is the mean enrichment per trophic level, TLspecies is the trophic position of the consumer, and TLsource is the trophic position of the source. To evaluate the contribution of the basal sources to the studied species, we have assigned to POM TLsource =1.0, since POM-δ13C values are compatible of the lowest size fraction (pico-phytoplankton) measured in the Western Mediterranean Sea (Hunt et al., 2017).
To calculate the enrichment factors from POM to the top consumers included in this study, we have used two discrimination factor levels — from POM to zooplankton, and from zooplankton to the studied species. Based on average Δ13C and Δ15N between POM to zooplankton measured in the Western Mediterranean Sea, we set the basal enrichment factors of 1.40 ± 1.15‰ for δ15N and 4.10 ± 1.63‰ for δ13C (Hunt et al., 2017). The enrichment from zooplankton to the studied species was done using discrimination factors of 3.15 ± 1.28‰ for δ15N and 0.97 ± 1.08‰ for δ13C (Sweeting et al., 2007), which have been previously used to study the trophic structure of meso- and bathypelagic fishes in the Gulf of Mexico (Richards et al., 2018) and in the Western Mediterranean Sea (Valls et al., 2014).
Each model was run with identical parameters (number of MCMC chains = 3; chain length = 300000; burn in = 200000; thin = 100), and model convergence was determined using Gelman-Rubin and Geweke diagnostic tests (Stock et al., 2018).
Results
Stable Isotopes
Species-specific mean δ13C values ranged from -20.85 to -16.89‰ for fish and from -18.54 to -16.38‰ for crustaceans. Fish mean δ13C values differed by 3.96‰, separating the most depleted (Macrorhamphosus scolopax: -20.85 ± 0.46‰, sampling depth of 200 m) and the most enriched species (Centrophorus granulosus and Conger conger: -16.89 and -16.57‰, respectively, sampling depth of ~1000m) (Table 1 and Figure 2). Crustaceans species-specific mean δ13C varied by 2.18‰, where the most depleted species was Aristeus antennatus (-18.54 ± 0.17‰, sampling depth of 600 m) and the most enriched species was Polycheles typhlops (-16.38 ± 0.21‰, sampling depth of ~1400 m). Species-specific differences in δ13C and δ15N were significant for both fish (MANOVA, F13,144 = 19.73, p< 0.001) and crustaceans (MANOVA, F5,78 = 14.62, p< 0.001).
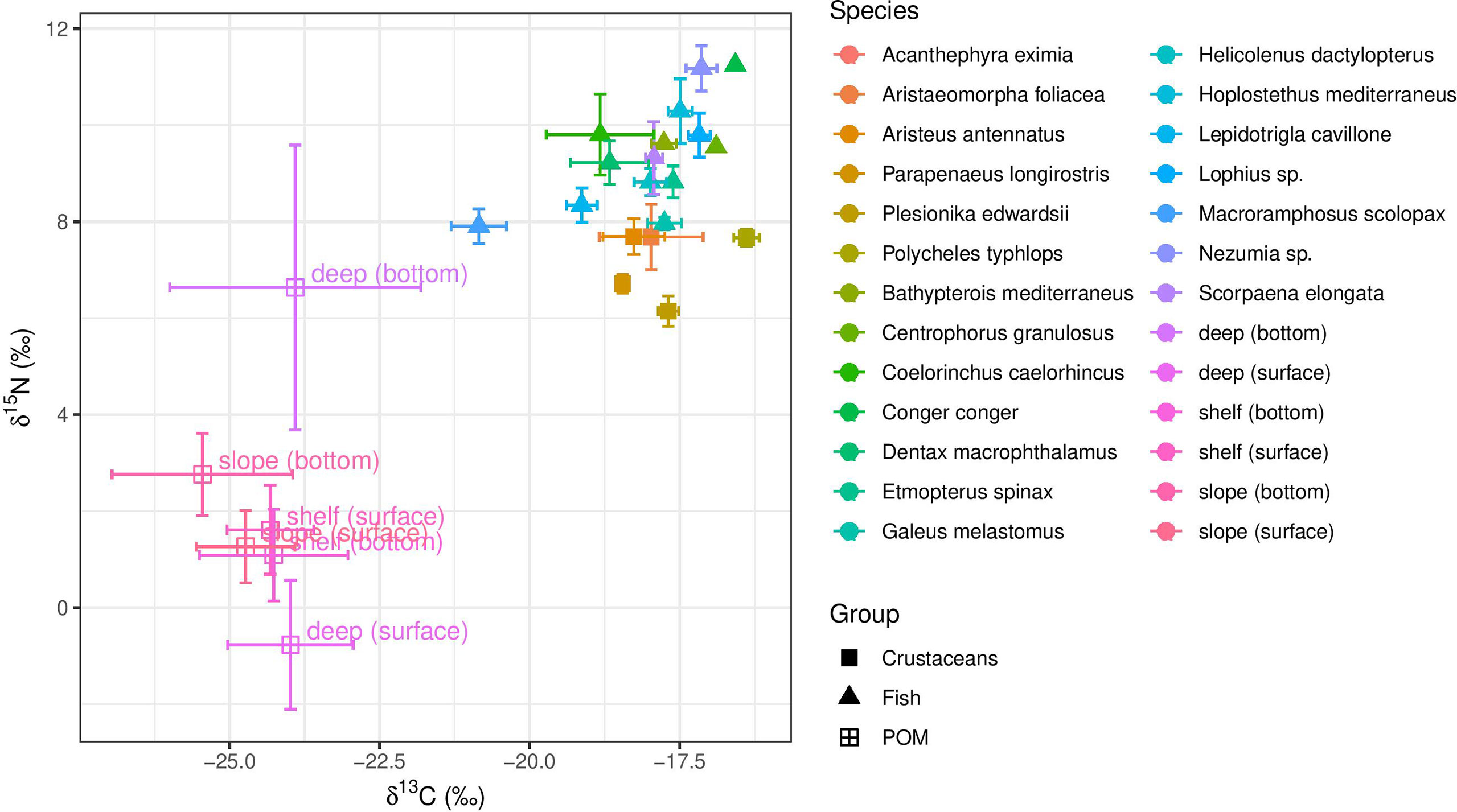
Figure 2 Isotope biplot of δ13C and δ15N values of decapod crustaceans (circles) and fishes (triangles), and POM (squares) from the shelf (200-250m), slope (400-800m) and deep-basin (1000-1400m) of the Southeastern Mediterranean Sea. Data points represent means and error bars represent ± SD.
Species-specific mean δ15N values varied from 7.91 ± 0.36‰ (M. scolopax, 200 m depth) to 11.36 ± 0.39‰ (Nezumia sp., 1100 m depth) in fish and from 5.96 ± 0.24‰ (Plesionika edwardsii; 200 m depth) to 7.73 ± 0.46‰ (Aristeus antennatus; 1100 – 1400 m depth) in crustaceans. Fish mean δ15N values positively correlated with the δ13C values (r2 = 0.6, p< 0.001, Figure 2 and Supplementary Figure 1) and varied among species (ANOVA, F13,72 = 24.22, p< 0.001). Crustaceans, however, did not show this correlation between δ15N and δ13C (r2 = 0.002, p > 0.05, Figure 2 and Supplementary Figure 2), observed in fish from similar depths. Due to limited spatial coverage within each species (the specimens of most species were sampled from the same depth), spatial variation could not be tested within each species, and therefore, spatial trends were tested by addressing all fish species together. Fish δ13C values positively varied with bottom depth (r2 = 0.42; P< 0.01, Figure 3), where the most enriched samples were found at the continental rise (> 1000 m) and the most depleted at the shallow slope (200 m) at the edge of the shelf. This pattern was less clear in the case of fish δ15N values (Figure 4), where species-specific mean values seem more variable in the continental rise (> 1000 m). Crustaceans mean δ15N values positively correlated with depth (r2 = 0.76, p = 0.053, Figure 4), while their mean δ13C values showed no such correlation (Figure 3).
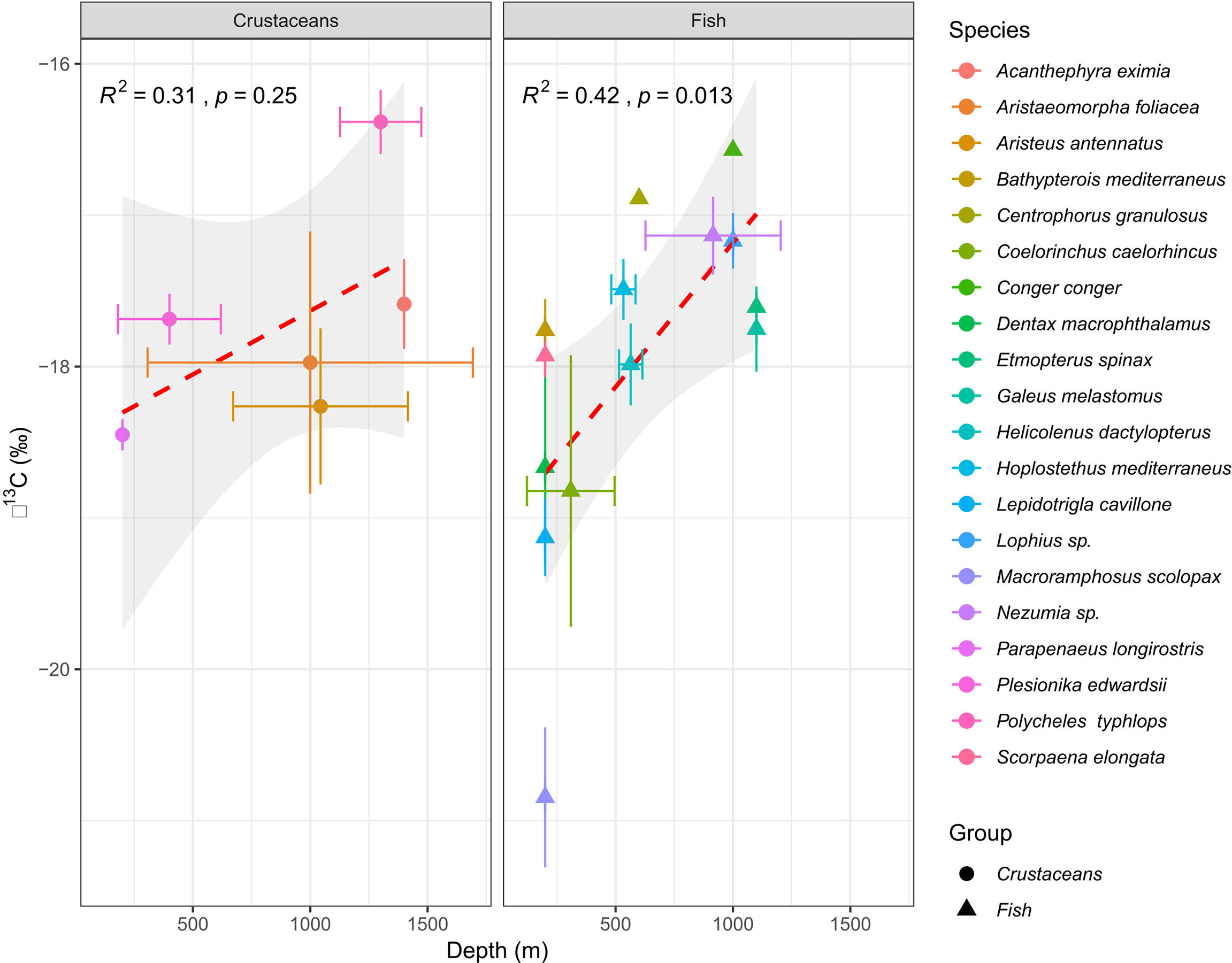
Figure 3 Correlations between mean δ13C (‰) and depth (m) in Levantine deep-sea decapod crustaceans (left) and fishes (right). Dash lines represent Least-squares regression line.
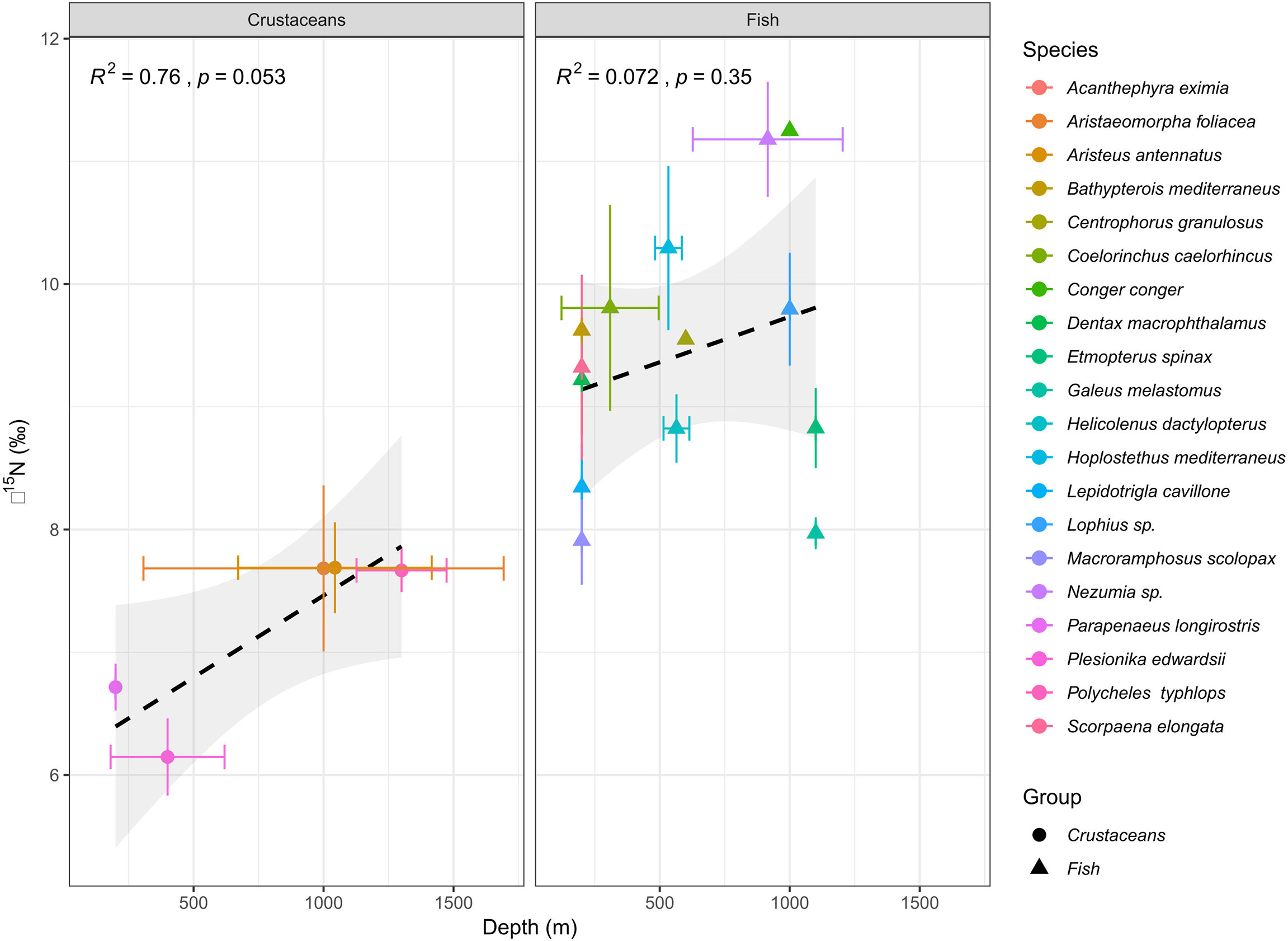
Figure 4 Correlations between mean δ15N (‰) and depth (m) in Levantine deep-sea decapod crustaceans (left) and fishes (right). Dash lines represent Least-squares regression line.
POM collected from depths ranging from 0 to 1135 m over the continental shelf, slope and rise exhibited a wide δ13C range (-27.72 to -21.36‰) and δ15N range (-3.25 to 12.76‰), with POM samples generally becoming more enriched in 15N and more depleted in 13C at bottom depths (Figure 5). Significant differences in POM δ13C and δ15N among the zones and depths were observed (MANOVA, F5,98 = 7.15, p< 0.001). POM-δ13C and C/N ratio exhibited a significant negative correlation (Pearson correlation, r = -0.566, p< 0.001, Supplementary Figure 3), which was not observed in POM-δ15N and C/N ratio.
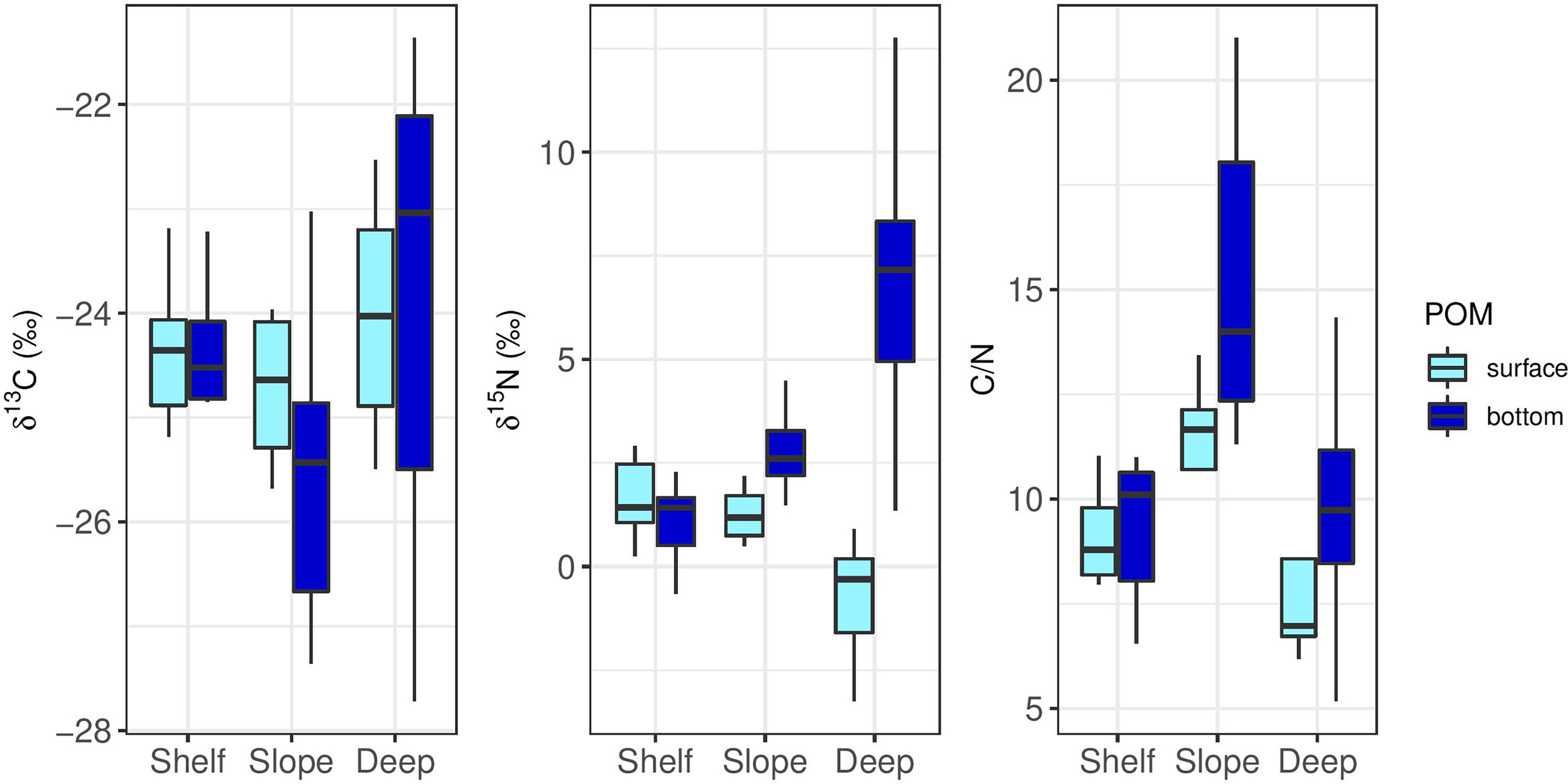
Figure 5 Boxplots showing POM-δ15N (‰), POM-δ13C (‰) and POM-C/N ratios collected from the surface and bottom of the continental shelf (200-250m), slope (400-800m) and deep-basin (1100-1400m) in the Southeastern Mediterranean Sea during 2018-2021.
Trophic Position Estimates
We used the average δ15N value of Eastern Levantine zooplankton (3.9 ± 1.8‰) obtained from Koppelmann et al. (2009) as a baseline (λ=2.0) for estimating species-specific TL. Based on the low δ15N values of zooplankton in the eastern Mediterranean, it was assumed that the primary food source, namely smaller zooplankton, phytoplankton and particles have a δ15N value around zero (Koppelmann et al., 2009). Large mesozooplankton (333-µm mesh size, upper water column) δ15N values in the EMS showed an enrichment trend across a west-east transect (SE Crete mean δ15N value ~2.0‰ and SE Cyprus mean δ15N value ~4.0‰, Koppelmann et al., 2009). Using these data to set the baseline, fish modal TL ranged from 3.17 (M. scolopax; 200 m depth) to 4.19 (C. conger; 1000 m depth), while the average modal TL of all fish species was 3.62 ± 0.31 (Figure 6). Crustaceans- δ15N values yielded modal TLs between 2.66 (P. edwardsii; 200-600 m depth) and 3.11 (A. antennatus; 600 – 1400 m), with an average of 2.94 ± 0.18 (Figure 6).
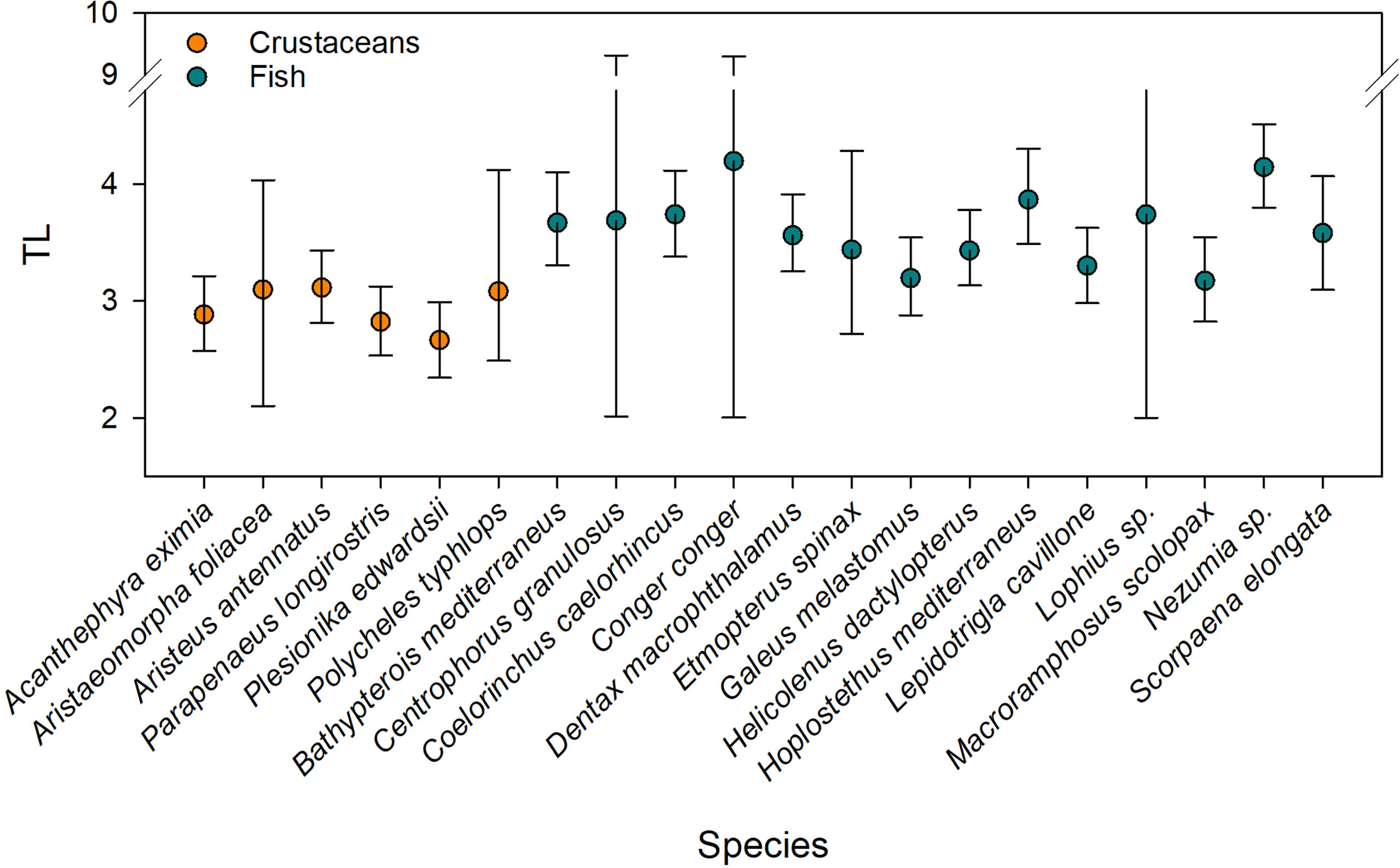
Figure 6 Trophic level (TL) Bayesian estimates based on δ15N data of deep-sea Levantine decapod crustacean (orange, n=6) and fish (blue, n=14) species using zooplankton of the Eastern Levantine Basin (Koppelmann et al., 2009) as an isotopic baseline. Error bars denote 95% confidence intervals.
Of the species examined, only few enabled an estimation of ontogenetic effect (Figure 7). This is due to the low range of body size within individual species that were sampled in this study. Nevertheless, the crustaceans A. eximia (r2 = 0.82; p< 0.001) and P. edwardsii (r2 = 0.72; p< 0.05) exhibited a positive relationship between length and δ15N values. Size and δ13C values did not yield significant correlations. Positive relationship between size and δ15N values was also observed for the fish D. macrophthalmus (r2 = 0.59; p< 0.05).
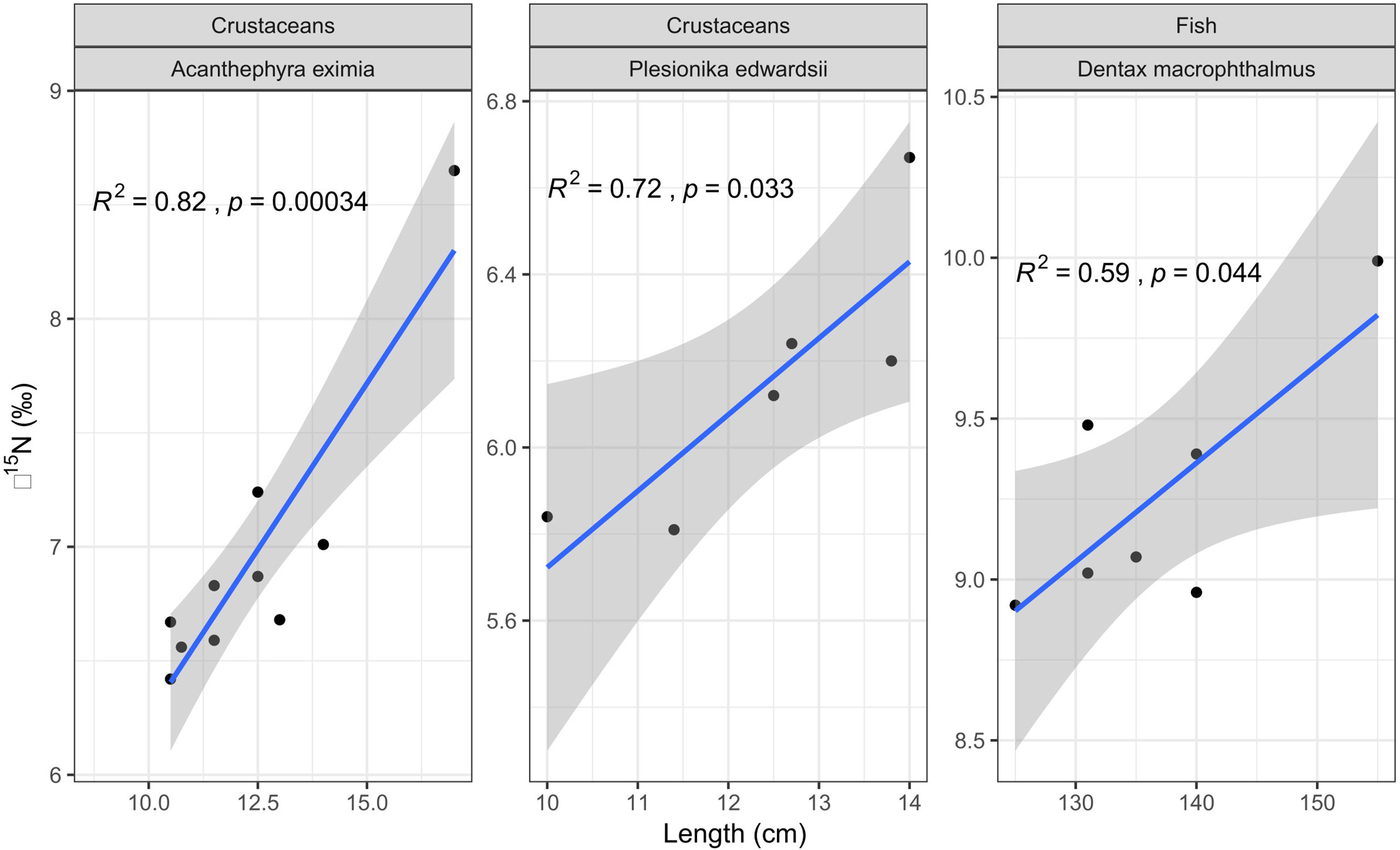
Figure 7 Least-squares regression analysis between total length (cm) and δ15N values in the decapod crustaceans A. eximia (n=10) and P. edwardsii (n=6), and the fish D. macrophthalmus (n=7).
Trophic Niche Breadth
Isotopic niche breadth, calculated using size-corrected standardized ellipse area SEAC (Supplementary Table 1 and Figure 8), was largest for the fish collected from the shallow continental slope C. caelorhincus (SEAC = 2.04), D. macrophthalmus (SEAC = 0.94) and M. scolopax (SEAC = 0.66), and for the shrimps A. antennatus (SEAC = 0.63) and A. eximia (SEAC = 0.56), both opportunistic carnivores. The smallest isotopic niche breadth belonged to the deep-water rose shrimp P. longirostris (SEAC = 0.07). Fish and crustacean assemblage metrics (Table 2A) showed a contrasting trend of isotopic niche size with depth, where fish isotopic niche size was largest in the shelf assemblage (SEAC = 1.81) and crustaceans isotopic niche size was largest in the deep assemblage (SEAC = 1.43). As a trophic guild (Table 2B), the fish showed higher convex hull area (TA = 7.37) than the crustaceans (TA = 0.81), indicating a larger trophic community width (Layman et al., 2007).
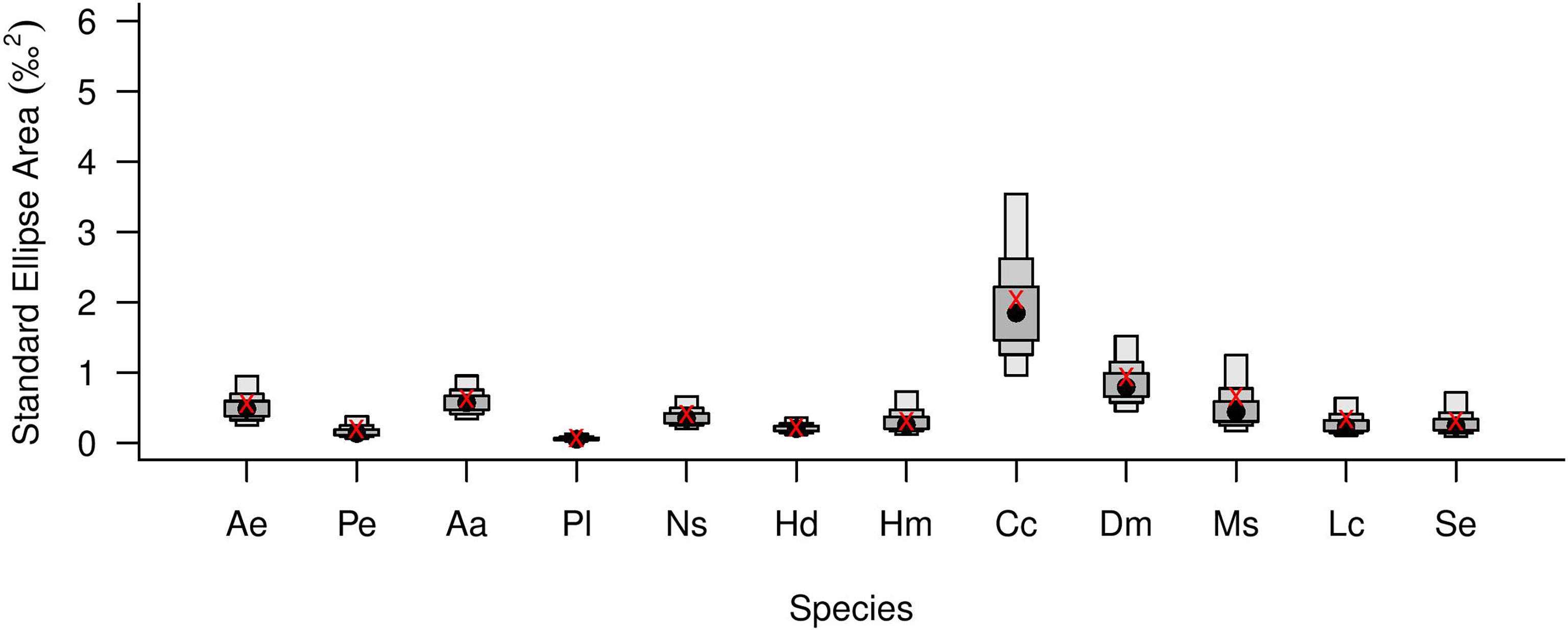
Figure 8 Trophic niche breadth of Levantine fishes and decapod crustaceans (n≥5) estimated by size-corrected standard ellipse area (SEAC) boxplots (showing 95, 75 and 50% credibility intervals). Black circles represent means; red x symbols represent the maximum likelihood estimates of SEA. Ae , Acanthephyra eximia; Pe , Plesionika edwardsii; Aa , Aristeus antennatus; Pl , Parapenaeus longirostris; Ns , Nezumia sp.; Hd , Helicolenus dactylopterus; Hm , Hoplostethus mediterraneus; Cc , Coelorinchus caelorhincus; Dm , Dentex macrophthalmus; Ms , Macroramphosus scolopax; Lc , Lepidotrigla cavillone; Se , Scorpaena elongata.
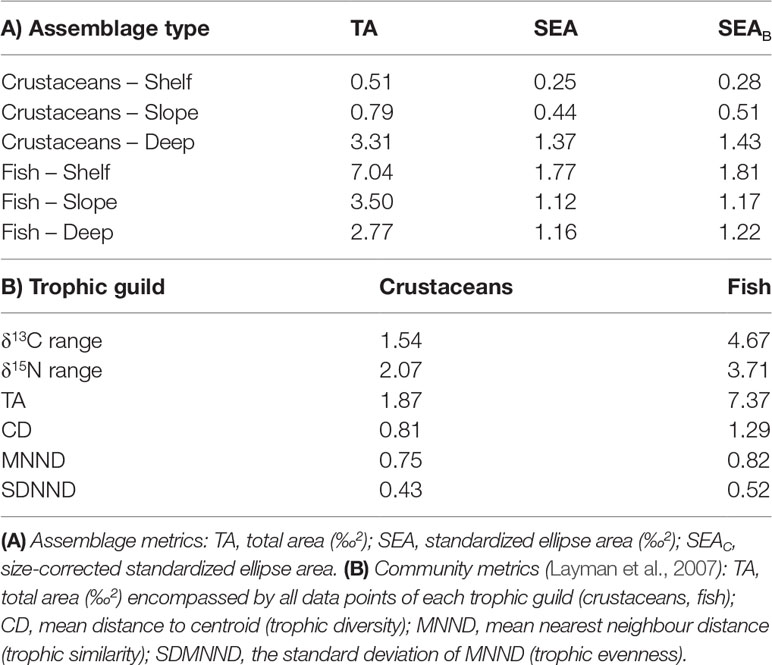
Table 2 Metrics for estimating assemblage and community (trophic guild) isotopic niche size in fish and decapod crustaceans from the Levantine deep-sea.
Bayesian Mixing Models
The results of the mixing models indicated variable sources sustaining the deep-sea fish and crustacean consumers included in this study (Figure 9). The contribution of deep bathypelagic POM was highest in the decapod crustaceans A. eximia (74.8 ± 10.8%), A. antennatus (63.3 ± 9.5%) and P. typhlops (43.1 ± 1.7%), and in the rattail fish Nezumia sp. (66.3 ± 8.0%). The contribution of pelagic POM to the deep bathypelagic fauna spanned 26 ± 4%, increasing in the slope, varying between 24 and 61% (including both the shelf and slope surface POM). Whereas, the models showed an increased contribution of benthic POM to the deep-sea fauna (Figures 9G–O). For example, the benthic contribution to the red shrimp A. antennatus was 20 ± 18.9% in the slope (Figure 9A) versus 63.3 ± 9.5% in the rise (Figure 9I). Therefore, the model results support a shift from pelagic to benthic source with increasing depth, i.e. with distance from shore.
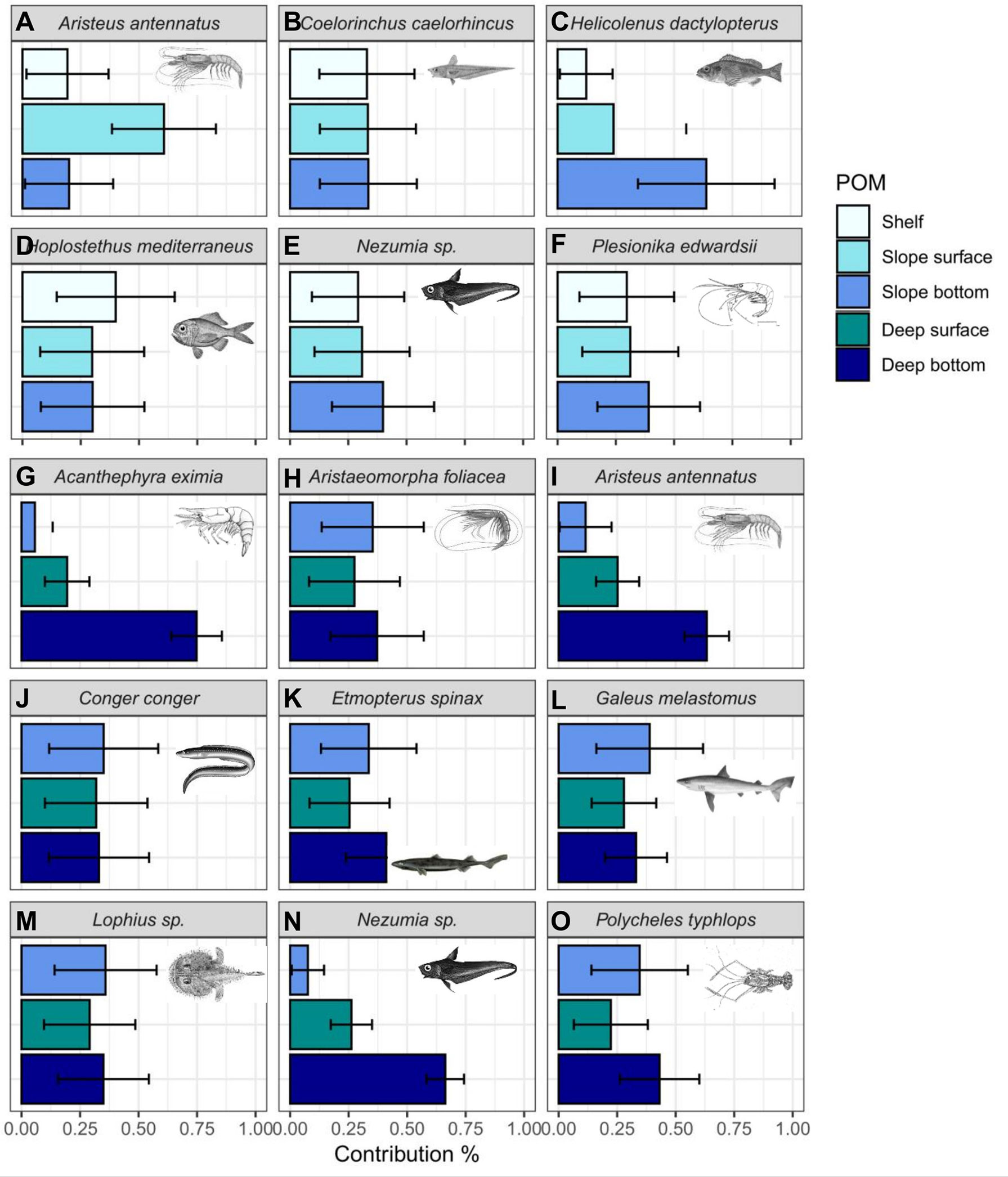
Figure 9 Estimated relative contributions (%) of POM collected from the surface and bottom depths of the continental slope and rise (400-800, 1100-1400 m, respectively) to Levantine fish and decapod crustaceans, based on Bayesian mixing models. (A–F). Species collected from the continental slope (400-600m). (G–O). Species collected from the continental rise (1000-1400m). Bars represent mean contributions and error bars represent ± SD.
Discussion
This is the first attempt to elucidate the trophic ecology of deep-sea fish and crustacean species in the SEMS. The knowledge gained in this study provides insights into the main energy sources sustaining deep-sea food webs in one of the most oligotrophic, nutrient-improvised marine basins, worldwide. However, insights gained in this study are not limited to the SEMS alone, and can be relevant to many oligotrophic basins with limited carbon and nutrient sources.
Our δ13C and δ15N values varied across fish species and as a function of depth, suggesting that depth and diet are controlling the trophic positions inferred from our stable isotope data. As expected, top predators such as the European conger eel C. conger, occupied the highest trophic position. The rattail Nezumia sp., a small macrourid fish that was collected from similar depths of >1000 m, yielded similar high δ15N values. Both species occupied a maximum trophic position of 4.14 – 4.19 (modal TL). Polunin et al. (2001) found similar trophic position of 4.4 for both the shark Centroscymnus coelolepsis and Nezumia aequalis in the continental slope of the Balearic Islands. High δ15N values of Nezumia (11.09 ± 0.58‰ and 11.31‰) were also recorded by Fanelli and Cartes (2010) in the Archipelago of Cabrera (Algerian Basin) and by Papiol et al. (2013) in the Balearic Islands (Catalan Sea, West Mediterranean), respectively, and were attributed to the suprabenthic crustaceans and polychaetes that constitute the diet of this macrourid. Our TL data also agree well with that of benthic carnivorous fish from Bay of Banyuls-sur-Mer (northwest Mediterranean, France; Carlier et al., 2007). Among the fish, the lowest trophic position (3.17) was found in the snipefish M. scolopax, which feeds on hyperbenthic demersal zooplankton during daytime (Carpentieri et al., 2016). This was also inferred from the results of the mixing models, indicating a relatively high contribution of mesopelagic POM to the diet of M. scolopax. Relatively to the fish, the bathybenthic crustaceans measured in this study occupied lower trophic positions — between 2.66 and 3.11, in agreement with the TL of deep benthic invertebrates of the Western Mediterranean (Carlier et al., 2007; Zorica et al., 2021).
The δ15N values of the deep-sea decapods A. eximia and P. edwardsii increased significantly with length indicating an ontogenetic effect. Such trend was found in A. eximia from the Catalan Sea (Western Mediterranean), where gut content analysis indicated a dietary shift from scavenging and detritivory in small individuals to active predation in larger individuals (Cartes, 1993). Smaller sized A. eximia were suggested to be better adapted to regions where resources are scarce (Thiel, 1983; Pérès, 1985). Similar dietary preferences were observed in P. edwardsii (Cartes, 1993). The lower δ15N that we measured in the smaller individuals of these decapods may indicate a detritivorous mode of feeding (Polunin et al., 2001). We found a similar ontogenetic effect in the demersal fish D. macrophthalmus. A gut analysis of this sparid fish off Angola and Namibia (South Atlantic Ocean) supports our finding, as the smaller fish tended to feed almost exclusively on polychaetes and euphausiids, but the larger fish preferred fish prey (Kilongo et al., 2007).
In the fish species examined here, mean δ15N values spanned 3.45‰, about 1.1 TL, while in the crustacean species mean δ15N values spanned 1.77‰, about 0.6 TL (assuming trophic enrichment factor of 3.15‰). Our observed ranges of estimated trophic levels are in line with other studies examining Mediterranean (1.1 TL, Valls et al., 2014), Pacific (1.6 TL, Choy et al., 2015), and the Gulf of Mexico (0.62 TL, Richards et al., 2018). Different feeding strategies as well as different migration habits may explain wider range of δ15N (Shipley et al., 2017a; Richards et al., 2020). Despite of the reliance on similar basal production, mesopelagic fishes from the Western Mediterranean were segregated by trophic position, between 2.9 for the small bristlemouth Cyclothone braueri to 4.0 for the lanternfish Lobianchia dofleini (Valls et al., 2014), and bathyal fishes off the Balearic Islands appeared to be foraging over two to three full trophic levels (Polunin et al., 2001). Our results support a much narrower trophic range for bathyal fish and bathybenthic decapod crustaceans in the SEMS. We attribute this narrow range to the ultra-oligotrophic state of the SEMS, resulting in limited carbon sources to sustain the deep-sea food webs, reflected by a general increase of δ13C in fish as function of bottom depth. This pattern could be driven by a number of factors including shifting production sources, or shifts in community composition and feeding strategies, and or switching from benthic to pelagic prey (Fanelli et al., 2011; Trueman et al., 2014). For example, 13C became more depleted in individuals captured at greater depths in the deep-sea island slope system of the Exuma Sound, the Bahamas (Shipley et al., 2017b). Inshore-to-offshore depletion in 13C values were also apparent in epipelagic fishes in the northern California Current, where copepods, gelatinous zooplankton, and nekton showed a significant linear decrease in δ13C with distance offshore (Miller et al., 2008). Our results show a different trend of increase in fish δ13C as function of bottom depth, i.e., distance offshore.
The major carbon sources supporting deep-sea food webs are poorly defined, aside from oligotrophic open-ocean gyres, where sinking phytoplanktonic-POM is considered the main energy source (Shipley et al., 2017b). This was observed by a narrow range of δ13C in meso- and bathypelagic predatory fishes in the Gulf of Mexico, indicating an exclusive epipelagic carbon source (Richards et al., 2018). Differently, the results of our mixing-model show increased contribution of bathypelagic POM and a reduction in the epipelagic source with increasing depth, i.e. distance offshore. This pattern agrees well with the extremely low primary production and carbon flux from the surface open water of the SEMS to the deep-seafloor (Katz et al., 2020). While most of the flux to the deep seabed is transported laterally.
Indeed, the majority of carbon supporting the species examined in this study is not derived from epipelagic sources. An alternative hypothesis is that the source of carbon in the deep-sea originates from the shelf. A significant proportion of neritic-derived primary production may be transported into deep-sea systems by currents (Suchanek et al., 1985; Sanchez-Vidal et al., 2012; Efrati et al., 2013), or via lateral transport (Fahl and Nöthig, 2007), and once assimilated into the food web, more enriched 13C values are to be expected (Polunin et al., 2001; Fanelli et al., 2011). Katz et al. (2020) used deep-sea sediment traps in the Israeli Southeastern Mediterranean Sea and showed that lateral transport from the continental margin contributes the greatest fraction of particulate flux to the seafloor. Therefore, we suggest that lateral transport constitutes the main source of carbon to the deep-sea food web in the Southeastern Mediterranean Sea. Our mixing model results and the relative decrease of C:N ratio in fish with increasing depth, indicating lower lipid content in deep-sea fish, further support a shift from pelagic to regenerated benthic carbon sources with depth. Given that the δ13C of POM slightly vary between the different foraging habitats, it is likely that this shift in δ13C occurs in the sediments water interface, by the enhanced bacterial regeneration of organic matter. This is likely driven by the high temperatures of the SEMS deep water near the seafloor (~13-14°C), compared to much lower temperatures of ~4°C at similar depths in most oceanic basins and despite the relatively low organic content of the SEMS sediments <1% (Ogrinc et al., 2007).
Since the carbon signature of primary producers can significantly vary between macroalgae and different phytoplankton groups (Fanelli et al., 2011; Grossowicz et al., 2019), food webs that show a linear relationship between δ15N and δ13C values are suggestive of a single food source (Polunin et al., 2001; Carlier et al., 2007). Generally weak δ13C–δ15N correlations were found in deep-sea macrozooplankton and micronekton off the Catalan slope likely due to the consumption of different kinds of sinking particles (e.g. marine snow, phytodetritus). Multiple recycling of POM constituted an enrichment effect on the δ13C and δ15N values of deep-sea macrozooplankton and micronekton (Fanelli et al., 2011). Our results yielded significant positive correlation between fish δ15N and δ13C values, further supporting a single food source.
Previous studies have shown that δ13C fractionates less than 1.0‰ for each trophic position. The Δ13C between the mean water column POM-δ13C (-24.13 ± 1.56‰) and fish/crustaceans δ13C (-18.10 ± 0.93‰) of the SEMS amounted to 6.04‰ (equal to at least six trophic positions), and therefore, cannot be attributed to trophic enrichment alone, but could be partly linked to the regeneration of benthic carbon sources, which may be relevant for detritus feeding animals, including all of the crustacean and some of the fish studied here Moreover, our δ13C-C/N data support the potential effect of microbially degraded phyto-detritus resulting in higher isotopic values of nitrogen and carbon in deep benthic food webs compared with pelagic food webs (Papiol et al., 2013; Romero-Romero et al., 2021).
Deep-sea ecosystems are subjected to exacerbating anthropogenic stressors, including overfishing, chemical pollution, mining, dumping, litter, plastics, and climate change (Davies et al., 2007). In oligotrophic environments such as the ultra-oligotrophic SEMS, deep-sea ecosystems are further vulnerable to reduced food availability (Kröncke et al., 2003). Regeneration of benthic carbon sources, supported by this study, provides oligotrophic deep-sea food webs with a greater ability to endure carbon limitation. Benthic carbon sources originating in lateral transport from the shallow shelf to the deep-sea, as indicated here, may hold important implications for marine spatial planning and the establishment of Marine Protected Areas (MPAs) in the SEMS exclusive economic zones (EEZ), promoting the extension of protected areas from the shelf to the deep-sea in a continuum rather than the establishment of MPAs that are disconnected from the continental shelf and slope. Furthermore, lateral transport from the shelf to the deep sea may carry detrimental implications to the ecosystem via pollutant accumulation and biomagnification (Liu et al., 2020). This is particularly important in marginal seas that are prone to anthropogenic pollution (Kim et al., 2019; Shoham-Frider et al., 2020). Continuous studies should be undertaken to further unveil the implications of lateral transport and benthic carbon regeneration to deep-sea food webs.
Data Availability Statement
The datasets presented in this study can be found in: Guy-Haim, Tamar; Stern, Nir; Sisma-Ventura, Guy (2022): Bulk stable isotopes of deep sea fish and crustaceans from the Southeastern Mediterranean Sea. PANGAEA, https://doi.org/10.1594/PANGAEA.945321.
Ethics Statement
The animal study was reviewed and approved by Israel National Institute of Oceanography Ethics Committee.
Author Contributions
GS-V and TG-H conceived this study. GS-V, NS, and TG-H collected the data. NS provided species identification and measurements. GS-V and TG-H analyzed and modeled the stable isotope data. All co-authors contributed substantially to drafting the manuscript, and approved the final submitted manuscript.
Conflict of Interest
The authors declare that the research was conducted in the absence of any commercial or financial relationships that could be construed as a potential conflict of interest.
Publisher’s Note
All claims expressed in this article are solely those of the authors and do not necessarily represent those of their affiliated organizations, or those of the publisher, the editors and the reviewers. Any product that may be evaluated in this article, or claim that may be made by its manufacturer, is not guaranteed or endorsed by the publisher.
Acknowledgments
We wish to thank Mor Kanari for map preparation, and the captain and crew of R/V Bat-Galim, including onboard technical and scientific personnel for assistance in sampling. We also thank the two reviewers for critically reading the manuscript and suggesting substantial improvements. This work was supported by the National Monitoring Program of the Israeli Mediterranean waters.
Supplementary Material
The Supplementary Material for this article can be found online at: https://www.frontiersin.org/articles/10.3389/fmars.2022.857179/full#supplementary-material
References
Bergstad O. (2013). North Atlantic Demersal Deep-Water Fish Distribution and Biology: Present Knowledge and Challenges for the Future. J. Fish. Biol. 83, 1489–1507. doi: 10.1111/jfb.12208
Bond A. L., Diamond A. W. (2011). Recent Bayesian Stable-Isotope Mixing Models are Highly Sensitive to Variation in Discrimination Factors. Ecol. Appl. 21, 1017–1023. doi: 10.1890/09-2409.1
Boyle M., Ebert D., Cailliet G. (2012). Stable-Isotope Analysis of a Deep-sea Benthic-Fish Assemblage: Evidence of an Enriched Benthic Food Web. J. Fish. Biol. 80, 1485–1507. doi: 10.1111/j.1095-8649.2012.03243.x
Britton J. R., Busst G. M. (2018). Stable Isotope Discrimination Factors of Omnivorous Fishes: Influence of Tissue Type, Temperature, Diet Composition and Formulated Feeds. Hydrobiologia 808, 219–234. doi: 10.1007/s10750-017-3423-9
Carlier A., Riera P., Amouroux J.-M., Bodiou J.-Y., Grémare A. (2007). Benthic Trophic Network in the Bay of Banyuls-sur-Mer (Northwest Mediterranean, France): An Assessment Based on Stable Carbon and Nitrogen Isotopes Analysis. Estuarine. Coast. Shelf. Sci. 72, 1–15. doi: 10.1016/j.ecss.2006.10.001
Carpentieri P., Serpetti N., Colloca F., Criscoli A., Ardizzone G. (2016). Food Preferences and Rhythms of Feeding Activity of Two Co-Existing Demersal Fish, the Longspine Snipefish, Macroramphosus Scolopax (Linnaeus 1758), and the Boarfish Capros Aper (Linnaeus 1758), on the Mediterranean Deep Shelf. Mar. Ecol. 37, 106–118. doi: 10.1111/maec.12265
Cartes J. (1993). Feeding Habits of Oplophorid Shrimps in the Deep Western Mediterranean. J. Mar. Biol. Assoc. United. Kingdom. 73, 193–206. doi: 10.1017/S0025315400032720
Caut S., Angulo E., Courchamp F. (2009). Variation in Discrimination Factors (Δ15N and Δ13C): The Effect of Diet Isotopic Values and Applications for Diet Reconstruction. J. Appl. Ecol. 46, 443–453. doi: 10.1111/j.1365-2664.2009.01620.x
Choy C. A., Popp B. N., Hannides C. C., Drazen J. C. (2015). Trophic Structure and Food Resources of Epipelagic and Mesopelagic Fishes in the North Pacific Subtropical Gyre Ecosystem Inferred from Nitrogen Isotopic Compositions. Limnology. Oceanogr. 60, 1156–1171. doi: 10.1002/lno.10085
Danovaro R., Dell’anno A., Corinaldesi C., Magagnini M., Noble R., Tamburini C., et al. (2008). Major Viral Impact on the Functioning of Benthic Deep-Sea Ecosystems. Nature 454, 1084–1087. doi: 10.1038/nature07268
Davies A. J., Roberts J. M., Hall-Spencer J. (2007). Preserving Deep-Sea Natural Heritage: Emerging Issues in Offshore Conservation and Management. Biol. Conserv. 138, 299–312. doi: 10.1016/j.biocon.2007.05.011
Drazen J. C., Sutton T. T. (2017). Dining in the Deep: The Feeding Ecology of Deep-Sea Fishes. Annu. Rev. Mar. Sci. 9, 337–366. doi: 10.1146/annurev-marine-010816-060543
Efrati S., Lehahn Y., Rahav E., Kress N., Herut B., Gertman I., et al. (2013). Intrusion of Coastal Waters Into the Pelagic Eastern Mediterranean: In Situ and Satellite-Based Characterization. Biogeosciences 10, 3349–3357. doi: 10.5194/bg-10-3349-2013
Fahl K., Nöthig E.-M. (2007). Lithogenic and Biogenic Particle Fluxes on the Lomonosov Ridge (central Arctic Ocean) and their Relevance for Sediment Accumulation: Vertical vs. Lateral Transport. Deep. Sea. Res. Part I.: Oceanogr. Res. Papers. 54, 1256–1272. doi: 10.1016/j.dsr.2007.04.014
Fanelli E., Azzurro E., Bariche M., Cartes J. E., Maynou F. (2015). Depicting the Novel Eastern Mediterranean Food Web: a Stable Isotopes Study Following Lessepsian Fish Invasion. Biol. Invasions. 17, 2163–2178. doi: 10.1007/s10530-015-0868-5
Fanelli E., Cartes J. E. (2010). Temporal Variations in the Feeding Habits and Trophic Levels of Three Deep-Sea Demersal Fishes from the Western Mediterranean Sea, Based on Stomach Contents and Stable Isotope Analyses. Mar. Ecol. Prog. Ser. 402, 213–232. doi: 10.3354/meps08421
Fanelli E., Cartes J. E., Papiol V. (2011). Food Web Structure of Deep-Sea Macrozooplankton and Micronekton Off the Catalan Slope: Insight From Stable Isotopes. J. Mar. Syst. 87, 79–89. doi: 10.1016/j.jmarsys.2011.03.003
Galil B. S., Danovaro R., Rothman S., Gevili R., Goren M. (2019). Invasive Biota in the Deep-Sea Mediterranean: an Emerging Issue in Marine Conservation and Management. Biol. Invasions. 21, 281–288. doi: 10.1007/s10530-018-1826-9
Gordon J. D., Merrett N. R., Haedrich R. L. (1995). “Environmental and Biological Aspects of Slope-Dwelling Fishes of the North Atlantic,” in Deep-Water Fisheries of the North Atlantic Oceanic Slope ( Dordrecht, Springer), 1–26.
Gordon J., Swan S. (1997). “Deep-Water Demersal Fishes: Data for Assessment and Biological Analysis. Final Report of European Commission”. EUROPEAN COMMISSION.
Goren M., Mienis H., Galil B. (2008). Not so Poor—More Deep-Sea Records From the Levant Sea, Eastern Mediterranean. Mar. Biodiversity Records (Cambridge University Press; Cambridge) 1, 1–4. doi: 10.1017/S1755267206005203
Grossowicz M., Sisma-Ventura G., Gal G. (2019). Using Stable Carbon and Nitrogen Isotopes to Investigate the Impact of Desalination Brine Discharge on Marine Food Webs. Front. Mar. Sci. 6, 142. doi: 10.3389/fmars.2019.00142
Hannides C. C., Zervoudaki S., Frangoulis C., Lange M. A. (2015). Mesozooplankton Stable Isotope Composition in Cyprus Coastal Waters and Comparison with the Aegean Sea (Eastern Mediterranean). Estuarine. Coast. Shelf. Sci. 154, 12–18. doi: 10.1016/j.ecss.2014.12.009
Hazan O., Silverman J., Sisma-Ventura G., Ozer T., Gertman I., Shoham-Frider E., et al. (2018). Mesopelagic Prokaryotes Alter Surface Phytoplankton Production During Simulated Deep Mixing Experiments in Eastern Mediterranean Sea Waters. Front. Mar. Sci. 5, 1. doi: 10.3389/fmars.2018.00001
Helm K. P., Bindoff N. L., Church J. A. (2011). Observed Decreases in Oxygen Content of the Global Ocean. Geophys. Res. Lett. 38, 1–6.doi: 10.1029/2011GL049513
Hopkins T., Gartner J. (1992). Resource-Partitioning and Predation Impact of a Low-Latitude Myctophid Community. Mar. Biol. 114, 185–197. doi: 10.1007/BF00349518
Hunt B. P., Carlotti F., Donoso K., Pagano M., D’ortenzio F., Taillandier V., et al. (2017). Trophic Pathways of Phytoplankton Size Classes Through the Zooplankton Food Web Over the Spring Transition Period in the North-West M Editerranean Sea. J. Geophys. Research.: Oceans. 122, 6309–6324. doi: 10.1002/2016JC012658
Jackson A. L., Inger R., Parnell A. C., Bearhop S. (2011). Comparing Isotopic Niche Widths Among and Within Communities: SIBER–Stable Isotope Bayesian Ellipses in R. J. Anim. Ecol. 80, 595–602. doi: 10.1111/j.1365-2656.2011.01806.x
Katz T., Weinstein Y., Alkalay R., Biton E., Toledo Y., Lazar A., et al. (2020). The First Deep-Sea Mooring Station in the Eastern Levantine Basin (DeepLev), Outline and Insights Into Regional Sedimentological Processes. Deep. Sea. Res. Part II.: Topical. Stud. Oceanogr. 171, 104663. doi: 10.1016/j.dsr2.2019.10466
Kelly C., Connolly P., Clarke M. (1998). The Deep Water Fisheries of the Rockall Trough: Some Insights Gleaned From Irish Survey Data. CES CM. O:40, 22 pp.
Kilongo K., Barros P., Diehdiou M. (2007). Diet of Large-Eye Dentex Macrophthalmus (Pisces: Sparidae) Off Angola and Namibia. Afr. J. Mar. Sci. 29, 49–54. doi: 10.2989/AJMS.2007.29.1.4.69
Kim H., Lee K., Lim D.-I., Nam S.-I., Hee Han S., Kim J., et al. (2019). Increase in Anthropogenic Mercury in Marginal Sea Sediments of the Northwest Pacific Ocean. Sci. Total. Environ. 654, 801–810. doi: 10.1016/j.scitotenv.2018.11.076
Koppelmann R., Böttger-Schnack R., Möbius J., Weikert H. (2009). Trophic Relationships of Zooplankton in the Eastern Mediterranean Based on Stable Isotope Measurements. J. Plankton. Res. 31, 669–686. doi: 10.1093/plankt/fbp013
Koppelmann R., Weikert H., Lahajnar N. (2003). Vertical Distribution of Mesozooplankton and its δ15N Signature at a Deep-sea Site in the Levantine Sea (Eastern Mediterranean) in April 1999. J. Geophys. Research.: Oceans. 108, 1–11. doi: 10.1029/2002JC001351
Koslow J. A. (1993). Community structure in North Atlantic Deep-Sea Fishes. Prog. Oceanogr. 31, 321–338. doi: 10.1016/0079-6611(93)90005-X
Kress N., Gertman I., Herut B. (2014). Temporal Evolution of Physical and Chemical Characteristics of the Water Column in the Easternmost Levantine Basin (Eastern Mediterranean Sea) from 2002 to 2010. J. Mar. Syst. 135, 6–13. doi: 10.1016/j.jmarsys.2013.11.016
Kröncke I., Türkay M., Fiege D. (2003). Macrofauna Communities in the Eastern Mediterranean Deep Sea. Mar. Ecol. 24, 193–216. doi: 10.1046/j.0173-9565.2003.00825.x
Layman C. A., Arrington D. A., Montaña C. G., Post D. M. (2007). Can Stable Isotope Ratios Provide for Community-Ide Measures of Trophic Structure? Ecology 88, 42–48. doi: 10.1890/0012-9658(2007)88[42:CSIRPF]2.0.CO;2
Liu M., Xiao W., Zhang Q., Shi L., Wang X., Xu Y. (2020). Methylmercury Bioaccumulation In Deepest Ocean Fauna: Implications For Ocean Mercury Biotransport Through Food Webs. Environ. Sci. Technol. Lett. 7, 469–476. doi: 10.1021/acs.estlett.0c00299
Malpica-Cruz L., Herzka S. Z., Sosa-Nishizaki O., Lazo J. P. (2012). Tissue-Specific Isotope Trophic Discrimination Factors And Turnover Rates In A Marine Elasmobranch: Empirical And Modeling Results. Can. J. Fisheries. Aquat. Sci. 69, 551–564. doi: 10.1139/f2011-172
Menezes G. M., Sigler M. F., Silva H. M., Pinho M. R. (2006). Structure And Zonation Of Demersal Fish Assemblages Off The Azores Archipelago (Mid-Atlantic). Mar. Ecol. Prog. Ser. 324, 241–260. doi: 10.3354/meps324241
Mengerink K. J., Van Dover C. L., Ardron J., Baker M., Escobar-Briones E., Gjerde K., et al. (2014). A Call For Deep-Ocean Stewardship. Science 344, 696–698. doi: 10.1126/science.1251458
Miller T. W., Brodeur R. D., Rau G. H. (2008). Carbon Stable Isotopes Reveal Relative Contribution Of Shelf-Slope Production To The Northern California Current Pelagic Community. Limnology. Oceanogr. 53, 1493–1503. doi: 10.4319/lo.2008.53.4.1493
Neat F., Burns F., Drewery J. (2008). The Deepwater Ecosystem Of The Continental Shelf Slope And Seamounts Of The Rockall Trough: A Report On The Ecology And Biodiversity Based On Frs Scientific Surveys. Fisheries. Res. Serv. Internal Rep. 1–30.
Ogrinc N., Monperrus M., Kotnik J., Fajon V., Vidimova K., Amouroux D., et al. (2007). Distribution Of Mercury And Methylmercury In Deep-Sea Surficial Sediments Of The Mediterranean Sea. Mar. Chem. 107, 31–48. doi: 10.1016/j.marchem.2007.01.019
Olin J. A., Hussey N. E., Grgicak-Mannion A., Fritts M. W., Wintner S. P., Fisk A. T. (2013). Variable Δ15n Diet-Tissue Discrimination Factors Among Sharks: Implications For Trophic Position, Diet And Food Web Models. PloS One 8, e77567. doi: 10.1371/journal.pone.0077567
Ozer T., Gertman I., Kress N., Silverman J., Herut B. (2017). Interannual Thermohaline, (1979–2014) And Nutrient, (2002–2014) Dynamics In The Levantine Surface And Intermediate Water Masses, Se Mediterranean Sea. Global Planetary. Change 151, 60–67. doi: 10.1016/j.gloplacha.2016.04.001
Pajuelo J. G., Seoane J., Biscoito M., Freitas M., González J. A. (2016). Assemblages Of Deep-Sea Fishes On The Middle Slope Off Northwest Africa (26–33 N, Eastern Atlantic). Deep. Sea. Res. Part I.: Oceanogr. Res. Papers. 118, 66–83. doi: 10.1016/j.dsr.2016.10.011
Pakhomov E., Perissinotto R., Mcquaid C. (1996). Prey Composition And Daily Rations Of Myctophid Fishes In The Southern Ocean. Mar. Ecol. Prog. Ser. 134, 1–14. doi: 10.3354/meps134001
Papiol V., Cartes J. E., Fanelli E., Rumolo P. (2013). Food Web Structure And Seasonality Of Slope Megafauna In The Nw Mediterranean Elucidated By Stable Isotopes: Relationship With Available Food Sources. J. Sea. Res. 77, 53–69. doi: 10.1016/j.seares.2012.10.002
Parzanini C., Parrish C. C., Hamel J.-F., Mercier A. (2019). Reviews And Syntheses: Insights Into Deep-Sea Food Webs And Global Environmental Gradients Revealed By Stable Isotope (Δ 15 N, Δ 13 C) And Fatty Acid Trophic Biomarkers. Biogeosciences 16, 2837–2856. doi: 10.5194/bg-16-2837-2019
Pecquerie L., Nisbet R. M., Fablet R., Lorrain A., Kooijman S. A. (2010). The Impact Of Metabolism On Stable Isotope Dynamics: A Theoretical Framework. Philos. Trans. R. Soc. B.: Biol. Sci. 365, 3455–3468. doi: 10.1098/rstb.2010.0097
Pérès J. (1985). History Of The Mediterranean Biota And The Colonization Of The Depths. Western. Mediterr. 1, 198–232.
Peterson B. J., Fry B. (1987). Stable Isotopes In Ecosystem Studies. Annu. Rev. Ecol. systematics. 18, 293–320. doi: 10.1146/annurev.es.18.110187.001453
Polis G. A., Strong D. R. (1996). Food Web Complexity And Community Dynamics. Am. Nat. 147, 813–846. doi: 10.1086/285880
Polunin N., Morales-Nin B., Pawsey W., Cartes J. E., Pinnegar J. K., Moranta J. (2001). Feeding Relationships In Mediterranean Bathyal Assemblages Elucidated By Stable Nitrogen And Carbon Isotope Data. Mar. Ecol. Prog. Ser. 220, 13–23. doi: 10.3354/meps220013
Post D. M. (2002). Using Stable Isotopes To Estimate Trophic Position: Models, Methods, And Assumptions. Ecology 83, 703–718. doi: 10.1890/0012-9658(2002)083[0703:USITET]2.0.CO;2
Post D. M., Layman C. A., Arrington D. A., Takimoto G., Quattrochi J., Montana C. G. (2007). Getting To The Fat Of The Matter: Models, Methods And Assumptions For Dealing With Lipids In Stable Isotope Analyses. Oecologia 152, 179–189. doi: 10.1007/s00442-006-0630-x
Protopapa M., Koppelmann R., Zervoudaki S., Wunsch C., Peters J., Parinos C., et al. (2019). Trophic Positioning Of Prominent Copepods In The Epi-And Mesopelagic Zone Of The Ultra-Oligotrophic Eastern Mediterranean Sea. Deep. Sea. Res. Part II.: Topical. Stud. Oceanogr. 164, 144–155. doi: 10.1016/j.dsr2.2019.04.011
Quezada-Romegialli C., Jackson A. L., Hayden B., Kahilainen K. K., Lopes C., Harrod C. (2018). Trophicposition, An R Package For The Bayesian Estimation Of Trophic Position From Consumer Stable Isotope Ratios. Methods Ecol. Evol. 9, 1592–1599. doi: 10.1111/2041-210X.13009
Rahav E., Silverman J., Raveh O., Hazan O., Rubin-Blum M., Zeri C., et al. (2019). The Deep Water Of Eastern Mediterranean Sea Is A Hotspot For Bacterial Activity. Deep. Sea. Res. Part II.: Topical. Stud. Oceanogr. 164, 135–143. doi: 10.1016/j.dsr2.2019.03.004
Ramirez-Llodra E., Brandt A., Danovaro R., Mol B. D., Escobar E., German C. R., et al. (2010). Deep, Diverse And Definitely Different: Unique Attributes Of The World’s Largest Ecosystem. Biogeosciences 7, 2851–2899. doi: 10.5194/bg-7-2851-2010
R Core Team (2020). “R: A Language and Environment for Statistical Computing” (Vienna, Austria: R Foundation for Statistical Computing).
Richards T. M., Gipson E. E., Cook A., Sutton T. T., Wells R. D. (2018). Trophic Ecology Of Meso-And Bathypelagic Predatory Fishes In The Gulf Of Mexico. ICES. J. Mar. Sci. 76, 662–672. doi: 10.1093/icesjms/fsy074
Richards T. M., Sutton T. T., Wells R. (2020). Trophic Structure And Sources Of Variation Influencing The Stable Isotope Signatures Of Meso-And Bathypelagic Micronekton Fishes. Front. Mar. Sci. 7, 876. doi: 10.3389/fmars.2020.507992
Rilov G., Galil B. (2009). “Marine Bioinvasions In The Mediterranean Sea–History, Distribution And Ecology,” In Biological Invasions In Marine Ecosystems (Berlin Heidelberg, Springer-Verlag), 549–575.
Romero-Romero S., Miller E. C., Black J. A., Popp B. N., Drazen J. C. (2021). Abyssal Deposit Feeders Are Secondary Consumers Of Detritus And Rely On Nutrition Derived From Microbial Communities In Their Guts. Sci. Rep. 11, 1–11. doi: 10.1038/s41598-021-91927-4
Rubin-Blum M., Sisma-Ventura G., Yudkovski Y., Belkin N., Kanari M., Herut B., et al. (2022). Diversity, Activity, And Abundance Of Benthic Microbes In The Southeastern Mediterranean Sea. FEMS Microbiol. Ecol. 98, fiac009. doi: 10.1093/femsec/fiac009
Sanchez-Vidal A., Canals M., Calafat A. M., Lastras G., Pedrosa-Pàmies R., Menéndez M., et al. (2012). Impacts On The Deep-Sea Ecosystem By A Severe Coastal Storm. PloS One 7, e30395. doi: 10.1371/journal.pone.0030395
Shipley O. N., Brooks E. J., Madigan D. J., Sweeting C. J., Grubbs R. D. (2017a). Stable Isotope Analysis In Deep-Sea Chondrichthyans: Recent Challenges, Ecological Insights, And Future Directions. Rev. Fish. Biol. Fisheries. 27, 481–497. doi: 10.1007/s11160-017-9466-1
Shipley O. N., Polunin N. V., Newman S. P., Sweeting C. J., Barker S., Witt M. J., et al. (2017b). Stable Isotopes Reveal Food Web Dynamics Of A Data-Poor Deep-Sea Island Slope Community. Food Webs. 10, 22–25. doi: 10.1016/j.fooweb.2017.02.004
Shoham-Frider E., Gertner Y., Guy-Haim T., Herut B., Kress N., Shefer E., et al. (2020). Legacy Groundwater Pollution As A Source Of Mercury Enrichment In Marine Food Web, Haifa Bay, Israel. Sci. Total. Environ. 714, 136711. doi: 10.1016/j.scitotenv.2020.136711
Sisma-Ventura G., Yam R., Shemesh A. (2014). Recent Unprecedented Warming And Oligotrophy Of The Eastern Mediterranean Sea Within The Last Millennium. Geophys. Res. Lett. 41, 5158–5166. doi: 10.1002/2014GL060393
Smith C. R., Baco A. R. (2003). “Ecology Of Whale Falls At The Deep-Sea Floor,” in Oceanography and Marine Biology, An Annual Review, Volume 41 (Florida, CRC Press), 319–333.
Stock B. C., Jackson A. L., Ward E. J., Parnell A. C., Phillips D. L., Semmens B. X. (2018). Analyzing Mixing Systems Using A New Generation Of Bayesian Tracer Mixing Models. PeerJ 6, e5096. doi: 10.7717/peerj.5096
Stock B., Semmens B., Ward E., Parnell A., Jackson A., Phillips D. (2021). “Package ‘MixSIAR’,” in Bayesian Mixing Model s in R, Version 3, 1.12.
Stramma L., Brandt P., Schafstall J., Schott F., Fischer J., Körtzinger A. (2008). Oxygen Minimum Zone In The North Atlantic South And East Of The Cape Verde Islands. J. Geophys. Research.: Oceans. 113, 1–15. doi: 10.1029/2007JC004369
Stramma L., Schmidtko S., Levin L. A., Johnson G. C. (2010). Ocean Oxygen Minima Expansions And Their Biological Impacts. Deep. Sea. Res. Part I.: Oceanogr. Res. Papers. 57, 587–595. doi: 10.1016/j.dsr.2010.01.005
Suchanek T. H., Williams S. L., Ogden J. C., Hubbard D. K., Gill I. P. (1985). Utilization Of Shallow-Water Seagrass Detritus By Carribbean Deep-Sea Macrofauna: δ13C evidence. Deep. Sea. Res. Part A. Oceanogr. Res. Papers. 32, 201–214. doi: 10.1016/0198-0149(85)90028-7
Sweeting C., Barry J., Barnes C., Polunin N., Jennings S. (2007). Effects Of Body Size And Environment On Diet-Tissue Δ15n Fractionation In Fishes. J. Exp. Mar. Biol. Ecol. 340, 1–10. doi: 10.1016/j.jembe.2006.07.023
Tecchio S., Coll M., Sardà F. (2015). Structure, Functioning, And Cumulative Stressors Of Mediterranean Deep-Sea Ecosystems. Prog. Oceanogr. 135, 156–167. doi: 10.1016/j.pocean.2015.05.018
Tecchio S., Van Oevelen D., Soetaert K., Navarro J., Ramírez-Llodra E. (2013). Trophic Dynamics Of Deep-Sea Megabenthos Are Mediated By Surface Productivity. PloS One 8, e63796. doi: 10.1371/journal.pone.0063796
Thiel H. (1983). Meiobenthos and nanobenthos of the deep sea. in: G. rowe (ed.), Deep-Sea Biololgy. Wiley, New York. pp. 167–230
Thurber A. R., Sweetman A. K., Narayanaswamy B. E., Jones D. O., Ingels J., Hansman R. (2014). Ecosystem Function And Services Provided By The Deep Sea. Biogeosciences 11, 3941–3963. doi: 10.5194/bg-11-3941-2014
Trueman C., Johnston G., O’hea B., Mackenzie K. (2014). Trophic Interactions Of Fish Communities At Midwater Depths Enhance Long-Term Carbon Storage And Benthic Production On Continental Slopes. Proc. R. Soc. B.: Biol. Sci. 281, 20140669. doi: 10.1098/rspb.2014.0669
Valls M., Olivar M. P., De Puelles M. F., Molí B., Bernal A., Sweeting C. (2014). Trophic Structure Of Mesopelagic Fishes In The Western Mediterranean Based On Stable Isotopes Of Carbon And Nitrogen. J. Mar. Syst. 138, 160–170. doi: 10.1016/j.jmarsys.2014.04.007
Walsh J. J. (1991). Importance Of Continental Margins In The Marine Biogeochemical Cycling Of Carbon And Nitrogen. Nature 350, 53–55. doi: 10.1038/350053a0
Winemiller K. O., Polis G. A. (1996). “Food Webs: What Can They Tell Us About The World?” in Food Webs (New York, NY: Springer), 1–22.
Yasuhara M., Cronin T. M., Demenocal P. B., Okahashi H., Linsley B. K. (2008). Abrupt Climate Change And Collapse Of Deep-Sea Ecosystems. Proc. Natl. Acad. Sci. 105, 1556–1560. doi: 10.1073/pnas.0705486105
Keywords: stable isotope analysis, trophic level, vertical vs. lateral transport, Bayesian mixing models, benthic carbon regeneration, carbon limitation, continental slope and rise
Citation: Guy-Haim T, Stern N and Sisma-Ventura G (2022) Trophic Ecology of Deep-Sea Megafauna in the Ultra-Oligotrophic Southeastern Mediterranean Sea. Front. Mar. Sci. 9:857179. doi: 10.3389/fmars.2022.857179
Received: 18 January 2022; Accepted: 03 June 2022;
Published: 27 July 2022.
Edited by:
Sylvie Marylène Gaudron, Sorbonne Universités, FranceReviewed by:
Gilles Lepoint, Fonds National de la Recherche Scientifique (FNRS), BelgiumJeffrey C. Drazen, University of Hawaii at Manoa, United States
Copyright © 2022 Guy-Haim, Stern and Sisma-Ventura. This is an open-access article distributed under the terms of the Creative Commons Attribution License (CC BY). The use, distribution or reproduction in other forums is permitted, provided the original author(s) and the copyright owner(s) are credited and that the original publication in this journal is cited, in accordance with accepted academic practice. No use, distribution or reproduction is permitted which does not comply with these terms.
*Correspondence: Tamar Guy-Haim, tamar.guy-haim@ocean.org.il; Guy Sisma-Ventura, guy.siv@ocean.org.il