Fatal aspergillosis and evidence of unrelated hearing loss in a harbor porpoise (Phocoena phocoena) from the German Baltic Sea
- 1Institute for Terrestrial and Aquatic Wildlife Research, University of Veterinary Medicine Hannover, Foundation, Büsum, Germany
- 2Department of Pathology, University of Veterinary Medicine Hannover, Foundation, Hannover, Germany
- 3Department of Osteology and Biomechanics, University Medical Center Hamburg-Eppendorf, Hamburg, Germany
- 4Department of Ecological Chemistry, Helmholtz Centre for Environmental Research – UFZ Leipzig, Leipzig, Germany
- 5Institute for Environmental Research, RWTH Aachen University, Aachen, Germany
- 6Institute of Hygiene and Infectious Diseases of Animals, Justus Liebig University Giessen, Giessen, Germany
- 7National Reference Center for Invasive Fungal Infections (NRZMyk), Leibniz Institute of Natural Product Research and Infection Biology–Hans Knöll Institute, Jena, Germany
- 8University Medical Center Hamburg-Eppendorf Microscopy Imaging Facility, Hamburg, Germany
Detailed post-mortem investigations including the auditory pathway are needed to advance our understanding of how underwater noise and other stressors affect hearing in cetaceans. A 12-year-old female porpoise (Phocoena phocoena) stranded alive in June 2021 at the German Baltic Sea coast and died some hours later. The most significant pathological findings were lesions caused by a severe aspergillosis that spread from the lung and pulmonary lymph node to the cerebellum. Based on molecular sequencing, the fungus was identified as Aspergillus fumigatus. Severe pyogranulomatous and necrotizing inflammation was diagnosed in the lung and the associated lymph node. In the left part of the cerebellum, focal, severe purulent and necrotizing meningoencephalitis with intralesional fungal structures was confirmed histologically. In addition, multifocal, severe, chronic, granulomatous, and eosinophilic gastritis with intralesional parasite structures was found in the stomach. Parallel stripes (linear skin markings) were detected along the caudal part of both body sides, which have not been previously described for harbor porpoises. Inner ear analysis revealed evidence of focal loss of outer hair cells in several regions from 120 to 580 µm from the apex of the right cochlea using immunofluorescence. The evidence of low-frequency hearing impairment was compatible with noise-induced hearing loss. This is the first case of concurrent presumptive noise-induced hearing loss and unrelated aspergillosis in a free-ranging harbor porpoise.
Introduction
The harbor porpoise (Phocoena, Linneaus 1758) is the only resident cetacean species in German waters, including the Baltic Sea (Berggren, 1994; Christian Kinze et al., 2021). Previous research has shown the existence of three subpopulations inhabiting the Baltic (Huggenberger et al., 2002; Wiemann et al., 2010; Galatius et al., 2012; Benke et al., 2014). Despite potentially seasonally sharing habitat, it is assumed that these subpopulations are separated from each other (Sveegaard et al., 2011; Gallus et al., 2012; Sveegaard et al., 2015). Environmental conditions probably lead to migratory behavior in harbor porpoises, with peaks of recorded numbers along the Baltic shorelines during the summer months (Kröger, 1986; Koschinski, 2001). Overall, high porpoise densities in German waters occurred in the area of “Kiel Bight,” along the western part of the Baltic (Scheidat et al., 2008). Still, no reliable population trends are available for the Baltic porpoise populations due to limited survey data and non-uniform methodology (e.g., Hammond et al., 2002; Hammond et al., 2013; Viquerat et al., 2014; Unger et al., 2021).
Manifold conservation and protection efforts have been undertaken for the Baltic porpoise populations (Scheidat et al., 2006). Specifically, porpoises are listed under the European Habitats Directive, Annex II and IV (European Union, 1992). As migratory marine mammal species requiring cross-boundary management, the porpoise is also included in the European Marine Strategy Framework Directive (European Union, 2008). Conservation plans have been implemented by the members of the Agreement on the Conservation of Small Cetaceans of the Baltic, North East Atlantic, Irish and North Seas (ASCOBANS) (ASCOBANS, 2002; ASCOBANS, 2012) or the Baltic Marine Environment Protection Commission (HELCOM) (HELCOM, 2018).
Despite being strictly protected and the lack of population trend data, an overall decline among the Baltic porpoise populations is assumed, based among others on the numbers of porpoises found dead and accessible bycatch records (Kröger, 1986; Koschinski, 2001; Siebert et al., 2006; Benke et al., 2014; Meinig et al., 2020; Siebert et al., 2020; Unger et al., 2021). Similar to the North Sea (Nachtsheim et al., 2021), the Baltic Sea represents an area highly used by humans, leading to multiple anthropogenic stressors for wildlife (Koschinski, 2001; Dietz et al., 2021). In many studies, the effects of pollutants on harbor porpoises in the Baltic Sea have been investigated (Bruhn et al., 1999; Siebert et al., 1999; Beineke et al., 2005; Das et al., 2006; Sonne et al., 2020). PCBs were reported to cause an onset of physiological effects (Kannan et al., 2000b; Jepson et al., 2016) and potential reproductive failure (Murphy et al., 2015) to profound reproductive failure in mammals (Helle et al., 1976; Jepson et al., 2016), including harbor porpoises, when exceeding certain thresholds. Noise pollution from marine traffic, offshore windfarm construction (Tougaard et al., 2009; Teilmann and Carstensen, 2012; Schaffeld et al., 2020), military activities (Siebert et al., 2022), or seismic surveys (Lucke et al., 2009) additionally affect harbor porpoises (Koschinski, 2001; Wright et al., 2013).
There is an increasing concern on how anthropogenic underwater noise exposure affects cetaceans and their hearing capabilities (Simmonds et al., 2014; Erbe et al., 2018). Because hearing is fundamental to cetaceans, changes to their auditory capabilities may impact their ability to carry out vital activities, such as navigation, feeding, and communication. Previous studies have shown that the cochlea of the harbor porpoise, as in terrestrial mammals, contains two types of auditory sensory cells: the inner hair cells (IHCs) and the outer hair cells (OHCs) (Morell et al., 2015; Morell et al., 2020; Morell et al., 2021; Morell et al., 2022a). Within the organ of Corti (or hearing organ), the sensory cells are arranged in one single row of IHCs and three rows of OHCs. The disposition of supporting and sensory cells in the apex of the cochlea (the tip of the spiral, where the lowest frequencies are encoded) is variable in mammals. However, a recent study has described the arrangement of sensory cells in the apex of the harbor porpoise (Morell et al., 2022a), providing baseline information on the common pattern in this species. As a result of the high intensity and/or long duration of sound exposure, ultrastructural alterations can be detected in the sensory cells and type I afferent innervation (Bredberg et al., 1972; Hu et al., 2000). These alterations include changes in the stereocilia, the degeneration of sensory cells, and hair cell apoptosis. These alterations can be confused by post-mortem decomposition artifacts, except for the hair cell death and scar formation (Morell et al., 2015). When a mammalian cochlear hair cell dies, the neighboring supporting cells actively participate in hair cell elimination, resulting in a distinct scar (Raphael and Altschuler, 1991). The presence of scars within hair cell rows is an important criterion to assess for prior lifetime noise-induced cochlear lesions (Morell et al., 2015; Morell et al., 2017a).
The exploitation of natural resources may lead to a severe reduction or an actual loss of suitable prey species for harbor porpoises (Reijnders, 1992; Koschinski, 2001). Still, accidental bycatch in fisheries is probably the most serious threat to this species, which is ongoing for decades (Kinze, 1988; Christensen, 1991; Berggren, 1994; Kock and Benke, 1996; Koschinski, 2001; Siebert et al., 2001; Siebert et al., 2006; Scheidat et al., 2008; Siebert et al., 2020). Secondary bacterial infections of the respiratory tract after a parasitic coinfection are the main natural cause of death for porpoises in the Baltic (Siebert et al., 2001; Siebert et al., 2020). The endoparasitosis of various organs and related alterations are common in porpoises (Lehnert et al., 2005), whereas infections of the central nervous system remain exceptional (Siebert et al., 2001; Siebert et al., 2020). Although severe parasitic infections of low clinical relevance in the ears of porpoises are common, infections of the inner ear structures only occur sporadically (Morell et al., 2017b; Wohlsein et al., 2019). When compared to more pristine ecosystems like the Arctic, porpoises in the Baltic Sea suffer from overall higher infection rates, which might be related to the elevated levels of contaminants (Wünschmann et al., 2001) among other stressors. However, even in comparison to the North Sea, infection rates in the Baltic remain higher (Siebert et al., 2020). Kesselring et al. (2017) gave evidence that Baltic porpoises die at an increasingly young age, probably unable to reproduce, which adds to the already-compromised population health status.
Aspergillus spp. are saprophytic fungi, and the main route of infection among animals is the respiratory tract (Biberstein, 1999). Depending on the (compromised) immune response, primary granulomatous pneumonia is common. Secondary spread to other organs via blood vessels is typical in mammals such as cetaceans, which often involves the brain (Biberstein, 1999; St. Leger et al., 2018; Reidarson et al., 2018). As proposed by Geraci and Ridgway (1991), systemic aspergillosis is not contagious and mammals represent dead-end hosts. A. fumigatus is the most frequent pathogenic representative among mammals (Biberstein, 1999; Seyedmousavi et al., 2015). This also holds true for cetaceans, where further A. niger and A. terreus occur (St. Leger et al., 2018). Overall, clinical aspergillosis among this clade is believed to be underreported due to the lack of clear symptoms in marine mammals (Reidarson et al., 2018), as well as its hyphae can be difficult to detect in tissue (Dagleish et al., 2006).
It is of utmost concern to investigate the natural and anthropogenic causes of death of marine mammals, to further assess the health status of the porpoise populations and ecosystem (Bossart, 2011). Detailed post-mortem examinations of stranded cetaceans and the diagnosis of infectious diseases, such as potentially underreported aspergillosis, as well as anthropogenic threats, such as noise-induced hearing loss, will aid in determining the health status and supporting the conservation of harbor porpoise populations inhabiting the Baltic Sea. In this study, we describe the extensive investigation of a live-stranded harbor porpoise from the Baltic. Research included necropsy and gross morphological investigations, histopathological investigations, molecular screening for pathogens, and life history data, as well as pollutant analysis and inner ear analysis to determine the cause of death and ante-mortem health status.
Materials and methods
A harbor porpoise live-stranded on the 5th of June 2021 along the beach of Heidkate, Kiel Bight, Schleswig-Holstein, Germany. The animal reportedly swam upside down in the shallow water and died around 10:00 p.m. on that same day. It was recovered the following morning, 6th of June 2021, as part of the stranding network collecting all marine mammals found dead along the coastlines of the German federal state of Schleswig-Holstein and transported to the Institute for Terrestrial and Aquatic Wildlife Research (ITAW, University of Veterinary Medicine Hannover, Foundation) in Büsum, Germany, for necropsy.
Necropsy
Necropsy followed national and international guidelines for post-mortem investigations in cetaceans (Siebert et al., 2001; IJsseldijk et al., 2019; Siebert et al., 2020), including the assessment of biometrics, such as body weight and length. The age and sex of the porpoise were determined using habitus, length, weight, and primary and secondary sexual characteristics, among others (Siebert et al., 2001; Kesselring et al., 2017). Teeth from the lower jaw were taken for accurate cementum aging, following Lockyer (1995). Based on the blubber thickness, the development of certain parts of skeletal musculature, and the age of the animal, the nutritional status was ascertained (Siebert et al., 2001). Each organ was removed separately, weighed individually, and investigated macroscopically. Air-filled and auditory organs, such as the respiratory tract, the melon and acoustic fat around the lower jaw, the tympano-periotic complex, and peribullar and pterygoid sinuses were evaluated carefully during the dissection, as described in Raverty et al. (2018) and IJsseldijk et al. (2019) to assess potential gross lesions, as seen in cases of blast injury (Siebert et al., 2022).
Histopathology
Various organ samples were taken for histopathology (tongue, tonsils, retropharyngeal lymph node, esophagus, thyroid gland, diaphragm, lung, pulmonary lymph node, heart, aorta, rete mirabile, liver, pancreas, gastric compartments, spleen, kidneys, adrenal glands, intestines, mesenteric lymph node, urinary bladder, reproductive tract, mammary glands, skeletal muscle, skin, blubber, brain, pituitary gland, spinal cord, bone marrow, eye, acoustic fat around the lower jaw, melon, and nasal sacs). Formalin-fixed and paraffin-embedded slides were prepared and stained with hematoxylin and eosin, according to Siebert et al. (2001) and Wohlsein et al. (2019). For selected tissue sections, additional stainings including periodic acid–Schiff (PAS) reaction and Grocott´s methenamine silver impregnation were applied.
Microbiology
Swabs (swab set, sterile, Amies Medium; Covetrus DE GmbH, Hamburg, Germany) were taken from the uterus, cervix, and brain of the animal and stored at +4°C until analysis. The tissue samples of the liver, kidneys, spleen, lungs, pulmonary lymph node, intestines, mesenteric lymph node, brain, and reproductive tract were stored at -20°C until analysis. Cultivation of bacteria and fungi was carried out at the Justus-Liebig University, Giessen, Germany and followed the methods of Siebert et al. (2017). Only moderate to severe levels of bacterial and fungal growth were taken into account. The fungal isolate was identified morphologically, as well as molecularly based on partial sequence of beta-tubulin. Methods of DNA extraction and polymerase chain reaction (PCR) amplification have been described previously (Walther et al., 2021). The tissue samples of the kidneys, lung, intestines, and mesenteric lymph node were sampled and stored at -70°C until analysis. Investigations were performed at the University of Veterinary Medicine Hannover, Foundation, Germany. All organs were analyzed by PCR for the presence of influenza A virus, morbillivirus, herpesvirus, and pestivirus (van Devanter et al., 1996; Verna et al., 2017; Jo et al., 2019; Shin et al., 2019), as also described in Siebert et al. (2022).
Parasitology
Parasites were collected during necropsy, washed with water, and stored in 70% ethanol. The level of parasite infection was determined macroscopically and semiquantitatively as mild, moderate, or severe, as described in Siebert et al. (2001). After preparation with lactophenol, the parasites were identified following morphological characteristics according to scientific literature (Arnold and Gaskin, 1975).
Pollutant analysis
Blubber and liver samples were homogenized by cryogenic grinding with an oscillating mixer mill (MM 400, Retsch, Haan, Germany). The homogenized tissues were extracted according to the modified method II by Jensen et al. (2003), as described by Wernicke et al. (2022). Briefly, 10 mg of blubber and 215 mg of liver were extracted in three steps with different mixtures of 2-propanol, diethyl ether, and hexane. The collected and combined solvent extracts were blown down to a constant weight, and the extracted lipid mass was determined. The lipid was redissolved in acetonitrile and submitted to a non-destructive cleanup on Captiva EMR-Lipid cartridges [Agilent Technologies, USA, Muz et al. (2021)], in combination with a primary secondary amine (PSA) sorbent (Agilent Technologies, Santa Clara, CA, USA) and dried magnesium sulfate. The extracts were then evaporated to dryness, and the residue was redissolved in ethyl acetate, containing 100 ng ml-1 of the following stable isotope-labeled internal standards: 13C12-PCBs 28, 52, 101, 118, 153, 138, 180 (Wellington Laboratories, Guelph, Ontario, Canada). The extracts were analyzed for PCB congeners 28/31, 52, 101, 118, 153, 138 and 180 by gas chromatography-high-resolution Orbitrap mass spectrometry [GC-HRMS QExactive, Thermo Fisher Scientific, Waltham, MA, United States, Muz et al. (2021)], based on method-matched calibrations. The obtained data were processed, and the peak areas of the above-mentioned PCBs were integrated using the software TraceFinder V 5.1. The results of the pollutant analysis are given as the sum of seven PCB congeners: ∑PCB7, i.e., summed-up lipid-normalized concentrations of the seven so-called ‘indicator’ PCBs 28, 52, 101, 118, 138, 153, and 180.
Ear analysis
At necropsy, the left tympano-periotic complex was fixed by immersion with 10% neutral-buffered formalin while the right inner ear was perfused with 4% paraformaldehyde in phosphate-buffered saline (PBS, pH 7.3) around 16 h post-mortem, following a previously optimized protocol for cetaceans (Morell and André, 2009; Raverty et al., 2018).
CT scan
The left tympano-periotic complex was examined by high-resolution peripheral quantitative computed tomography (HR-pQCT, XtremeCT II®, Scanco Medical, Brüttisellen, Switzerland, voxel size 42 μm) at the Department of Osteology and Biomechanics (University Medical Centre Hamburg-Eppendorf, UKE, Germany). This technique allows detailed imaging and quantification of bone microarchitecture.
Histopathology
The left tympano-periotic complex was completely decalcified using 14% EDTA (ethylenediaminetetraacetic acid) tetrasodium salt (pH 7.4) for 147 days at room temperature, changing the media every 7–10 days. The endpoint of the decalcification process was confirmed by digital contact radiography using a Faxitron X-ray cabinet (Faxitron X-ray Corp., Wheeling, IL, USA) at the UKE Hamburg.
The ears were then sectioned transversely on six levels while immersed in PBS (Figure 1). The tissue sections were placed in cassettes, rinsed in 50% ethanol, and routinely embedded in paraffin. The 3µm-thick sections were prepared from the paraffin blocks, stained routinely with hematoxylin and eosin, and evaluated microscopically at the Department of Pathology (University of Veterinary Medicine Hannover, Foundation, Germany).
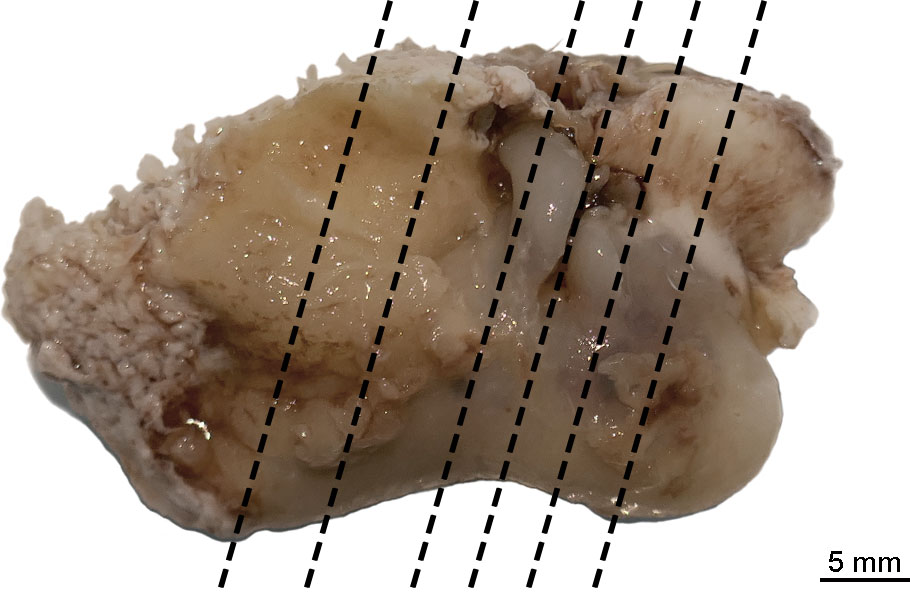
Figure 1 Sectioning planes of the left tympano-periotic complex. The background was manually removed from the image.
Immunofluorescence
The right periotic bone was decalcified with 14% EDTA (see above) for 45 days at room temperature. Then, the bone was removed, and the cochlea was dissected using the whole-mount technique, following the method described in Morell et al. (2020). The organ of Corti was labeled with an anti-prestin antibody (courtesy of Dr. Zheng, ref. NW958, 1:1,000 overnight) that is specific for OHC basolateral membrane labeling (Matsuda et al., 2004) and with Alexa Fluor™ 488 Phalloidin (Invitrogen A12379, 1:100 for 2 h) that labels F-actin. Type I afferent innervation was labeled with anti-neurofilament H antibody (Millipore ref. AB5539, 1:5,000). Nuclei were counterstained with DAPI (40, 6-diamidino-20-phenylindole, dihydrochloride; Thermo Scientific™, 62247, 1:1,000). As secondary antibodies we used Alexa Fluor® Plus 555 donkey anti-rabbit IgG (A32794, 1:400), and Alexa Fluor® 633 donkey anti-chicken IgY (Sigma-Aldrich ref. SAB4600127, 1:400). The whole mounts were blocked for 1 h with 5% normal donkey serum, incubated overnight with anti-prestin and anti-neurofilament H primary antibodies at 4°C, rinsed with PBT (0.1% triton-X 100 with 2 mg/l bovine serum albumin in PBS, three times, 10 min each), incubated with secondary antibodies, DAPI and phalloidin 2 h in the dark, rinsed with PBS (three times, 10 min each), and mounted individually on a glass slide with 0.1% N-propyl gallate in 90% glycerol. Two small subsegments were processed as controls: 1) control for the non-specific binding of the secondary antibodies (the subsegment was incubated without the primary antibodies but with the same concentrations of the secondary antibody and DAPI as used on experimental segments) and 2) control for autofluorescence (no primary and no secondary antibodies were used).
The right cochlea was observed in an optic fluorescence microscope (Kern OBN-14) at the facilities of ITAW and a confocal microscope Leica SP8 at UKE Hamburg Microscopy Imaging Facility. The micrographs of the two controls were taken using the same settings as their respective treatments (i.e., same magnification and same intensity of the four lasers).
Image processing
Maximum projections from confocal images were prepared with ImageJ software and three-dimensional reconstructions via segmentation of the lesions with Bitplane Imaris software. The brightness and contrast of images were adjusted in Adobe Photoshop 2021 (San Jose, CA, USA) and ImageJ software. Identical treatments were conducted for control and experimental segments.
Results
Life history
The porpoise measured 144 cm in total length, but the body weight of 43.8 kg was not accurate, as the carcass was incomplete. By the time it was recovered from the beach, parallel stripes were already recorded bilaterally on the flanks of the animal. It was identified as a 12-year-old female, based on cementum aging. The porpoise was lactating and showed prominent teats as signs of reproductive activity. The left uterine horn was enlarged and a corpus luteum was present on the left ovary, indicating that the porpoise had recently given birth. Stranding occurred within the known breeding season for porpoises in German Baltic waters (Kesselring et al., 2017), but no calf was observed nearby neither directly nor in the following days. According to the location of the stranding, the individual most likely belonged to the Belt Sea subpopulation (Huggenberger et al., 2002; Wiemann et al., 2010; Teilmann et al., 2011; Galatius et al., 2012; Benke et al., 2014). However, no genetic analysis was yet performed to confirm the subpopulation that this individual belonged to.
Pathological findings
The porpoise was classified as decomposition condition code 1 (i.e., freshly dead). A larger area along the left cranial part of the body was scavenged, tissue was missing, and the left eye was damaged. Based on the blubber thickness of 1.2–2.5 cm, the absence of visceral fat, and the well-developed musculature along the spine, the animal was in moderate nutritional condition. Along the caudal part of the body, bilateral, vertical, linear marks of grayish color in parallel order were present on the skin and not elevated above the skin level (Figure 2). All marks were of 5 mm width and 5–16 cm long. On the left side of the body, seven marks from 7 to 16 cm long were present. On the right side, five marks from 5 to 10 cm long were detected. Histologically, the undulating surface of the epidermis without cellular reaction was observed in those skin marks.
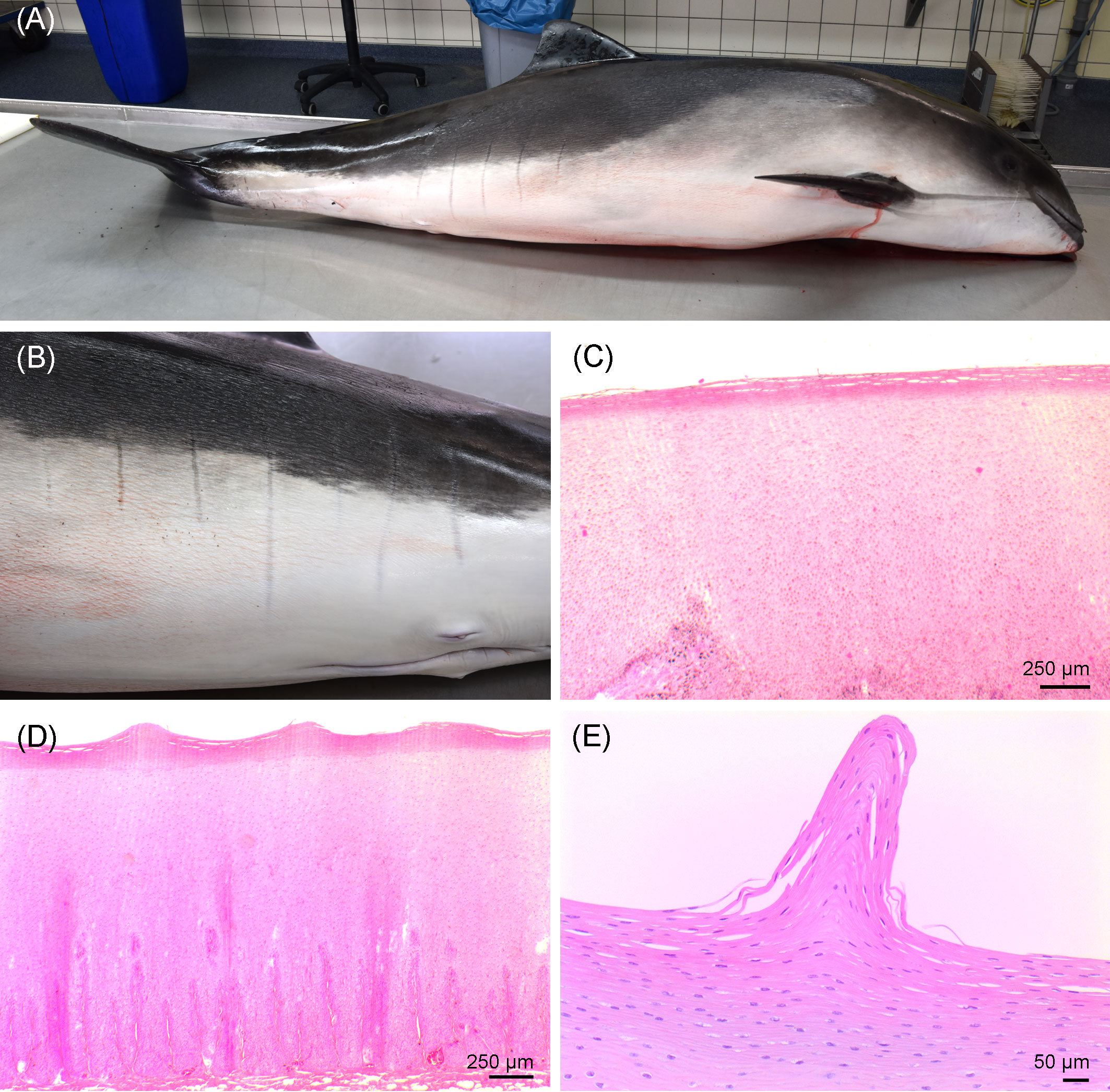
Figure 2 (A) Right lateral view of the investigated porpoise. (B) Vertical, parallel stripes on the left caudal part of the body of the porpoise. (C) Histological image of the unchanged skin. (D, E) Linear skin markings histologically, showing an undulating pattern in the stratum corneum.
Severe pyogranulomatous and necrotizing pneumonia was diagnosed in the lung, which presented a dark-red color and granulomas up to 10 cm in diameter, with necrotic content (Figures 3A, B). Similarly, severe pyogranulomatous and necrotizing lymphadenitis was diagnosed in the pulmonary lymph node. Intralesional faintly stained, light-brownish fungal spherules were detected, mostly in the cytoplasm of macrophages and multinucleated giant cells. They stained intensely positive using the PAS reaction and Grocott’s methenamine silver impregnation. The fungal structures consisted of round-to-oval or lemon-shaped often-empty spherules with thick, refractile walls with a diameter of 5–20 µm, occasionally with some basophilic finely granular content. The fungal elements were often arranged in short rows. Rarely septated swollen hyphal structures with parallel walls or hyphae with an arborizing growth pattern and dichotomous branching at acute angles were observed (Figures 3C–E). In the left part of the cerebellum, an area of approximately 1.5 cm in diameter, caseous texture, and brownish-to-yellowish color was found in the neuroparenchyma (Figure 4A), which was histologically diagnosed as granulomatous meningoencephalitis with necrotizing arteritis and intralesional fungal structures similar to those in the lung and pulmonary lymph node (Figures 4B, C). In addition, multifocal, mild-to-moderate perivascular bleedings were seen in the brain and spinal cord. In the proventricular part of the stomach, diffuse, elongated erosions of the gastric mucosa of up to 1 cm in length were seen. A poorly demarcated nodule of approximately 3 cm in diameter was located in the proventricular part (Figures 5A, B). The nodule was round shaped, elevated above the mucosal surface and with a central porus, surrounded by the hyperplastic epithelium and presented a creamy yellowish and fluid blackish content when cut. Histologically, a multifocal-to-coalescing, severe, chronic, granulomatous and eosinophilic, mural gastritis with intralesional parasite structures and severe, perifocal fibrosis was found (Figure 5C). In the melon, a focal, moderate acute hemorrhage was found histologically (Figure 6).
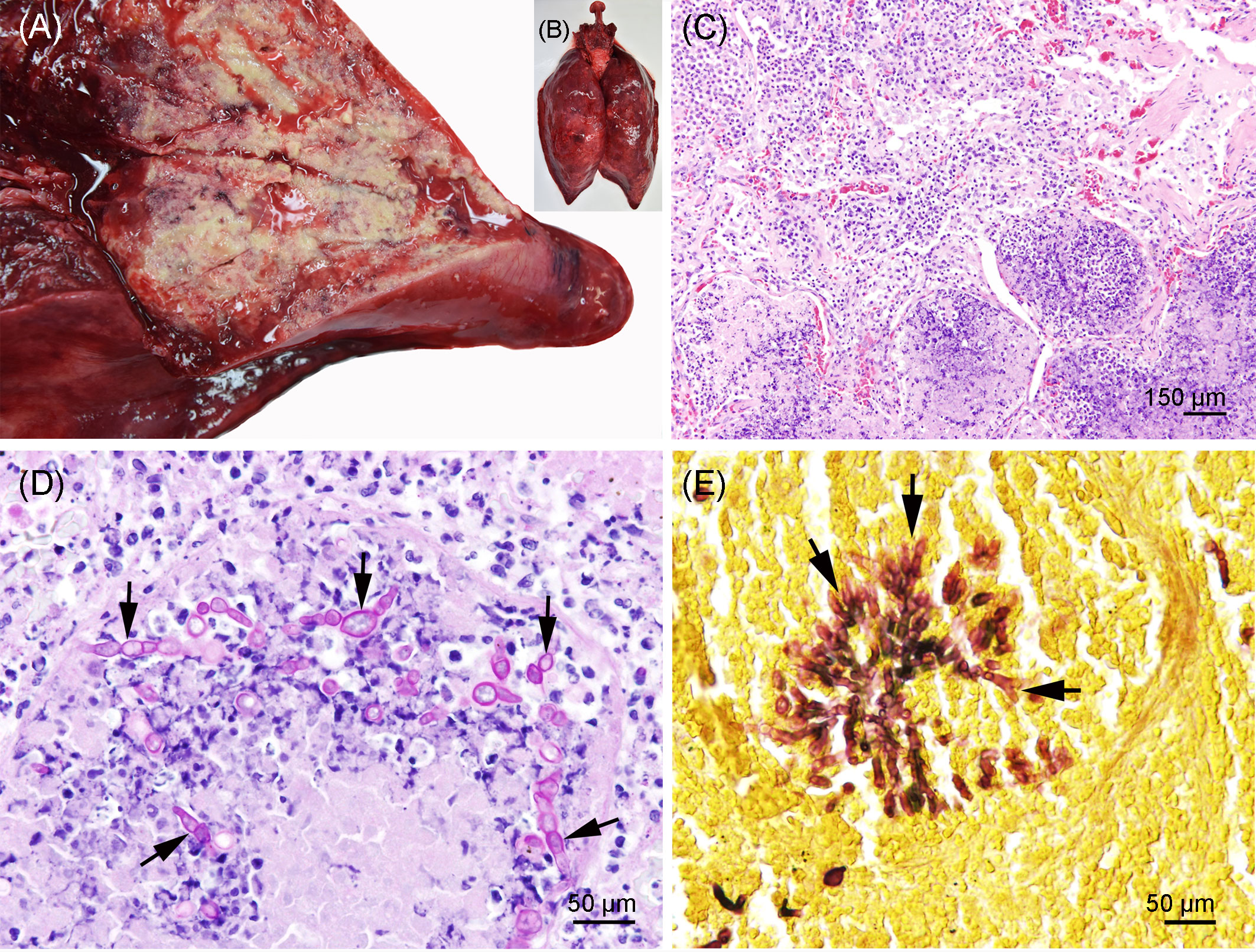
Figure 3 (A, B) Pyogranulomatous and necrotizing pneumonia in the lung of the porpoise. The background was manually removed from the image. (C) Lung with severe necrosuppurative (bottom) and pyogranulomatous (lop left) inflammation; hematoxylin and eosin stain. (D) Lung with severe granulomatous and necrotizing pneumonia and numerous intralesional germinating fungal spherules (arrows); periodic acid–Schiff (PAS) reaction. (E) Lung with focal arborizing fungal growth and dichotomous branching at acute angles (arrows); Grocott’s methenamine silver stain.
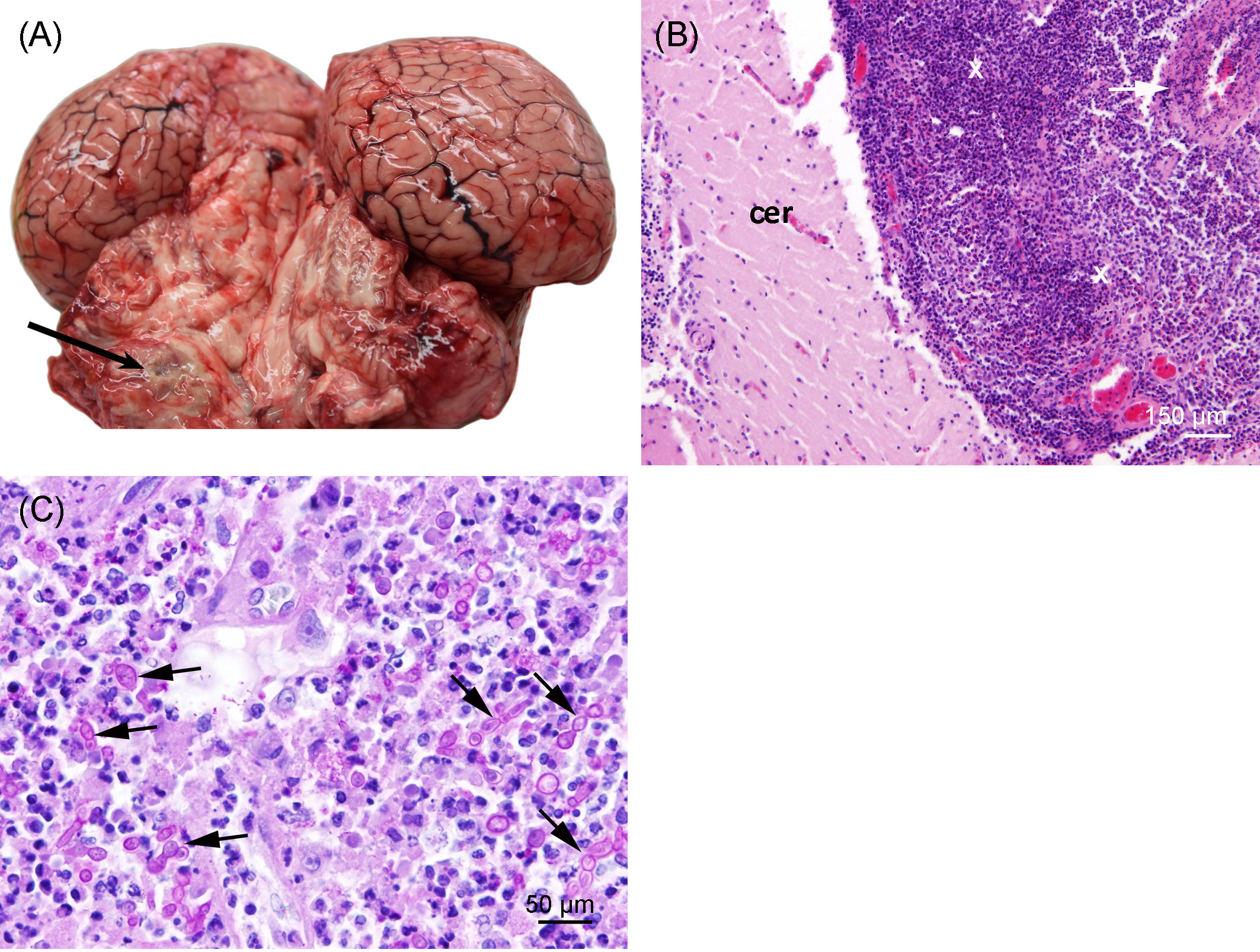
Figure 4 (A) Granuloma (arrow) in the left cerebellum of the porpoise. The background was manually removed from the image. (B) Cerebellum (cer) with severe chronic granulomatous leptomeningitis (X) and necrotizing arteritis (arrow); hematoxylin and eosin stain. (C) Severe granulomatous and necrotizing leptomeningitis with numerous, often-germinating fungal spherules (arrows); PAS reaction.
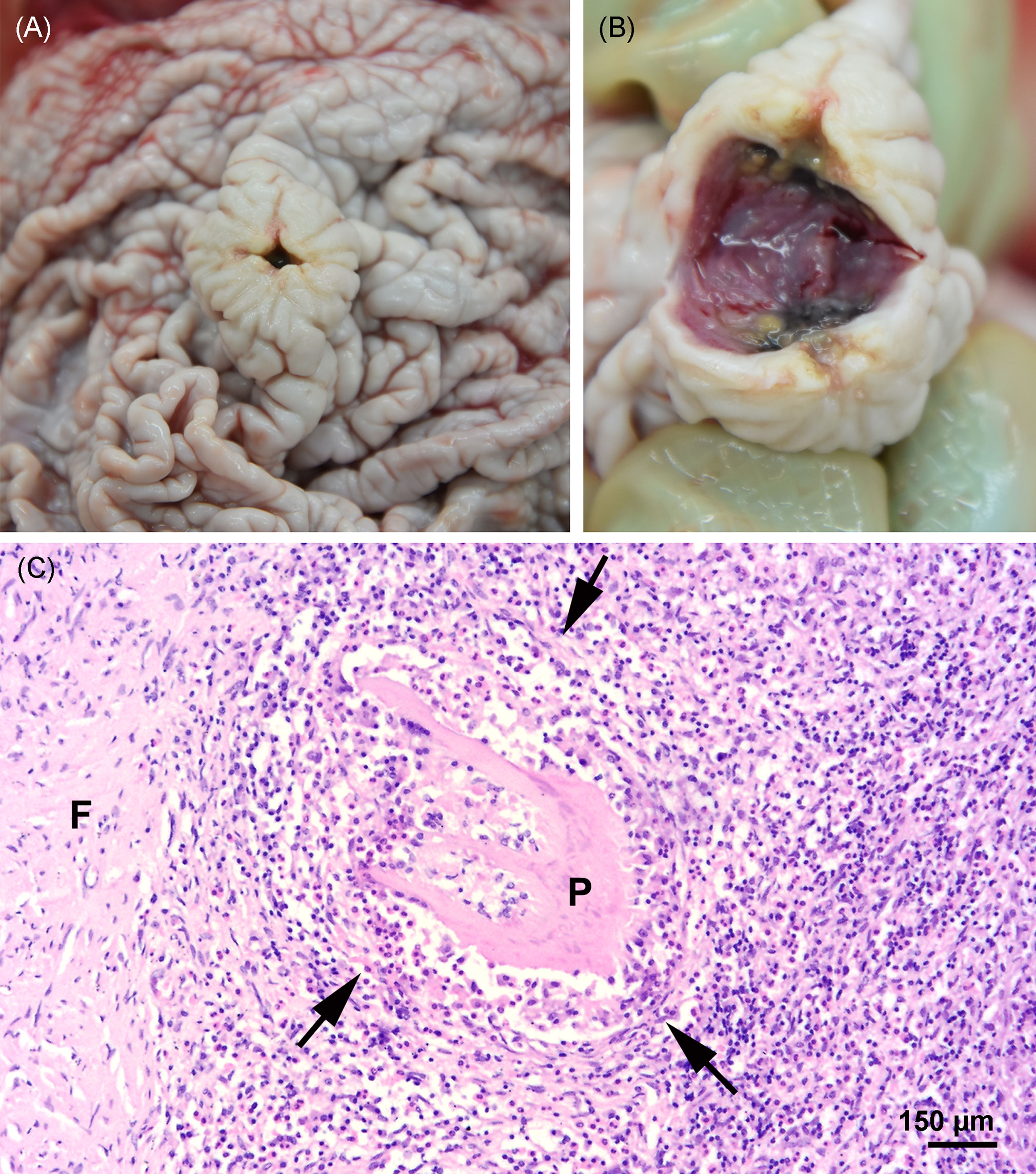
Figure 5 (A, B) Nodule in the stomach at necropsy. (C) Focal severe granulomatous, mural gastritis (arrows), intralesional parasitic structure (P), and perifocal fibrosis (F) in the stomach; hematoxylin and eosin stain.
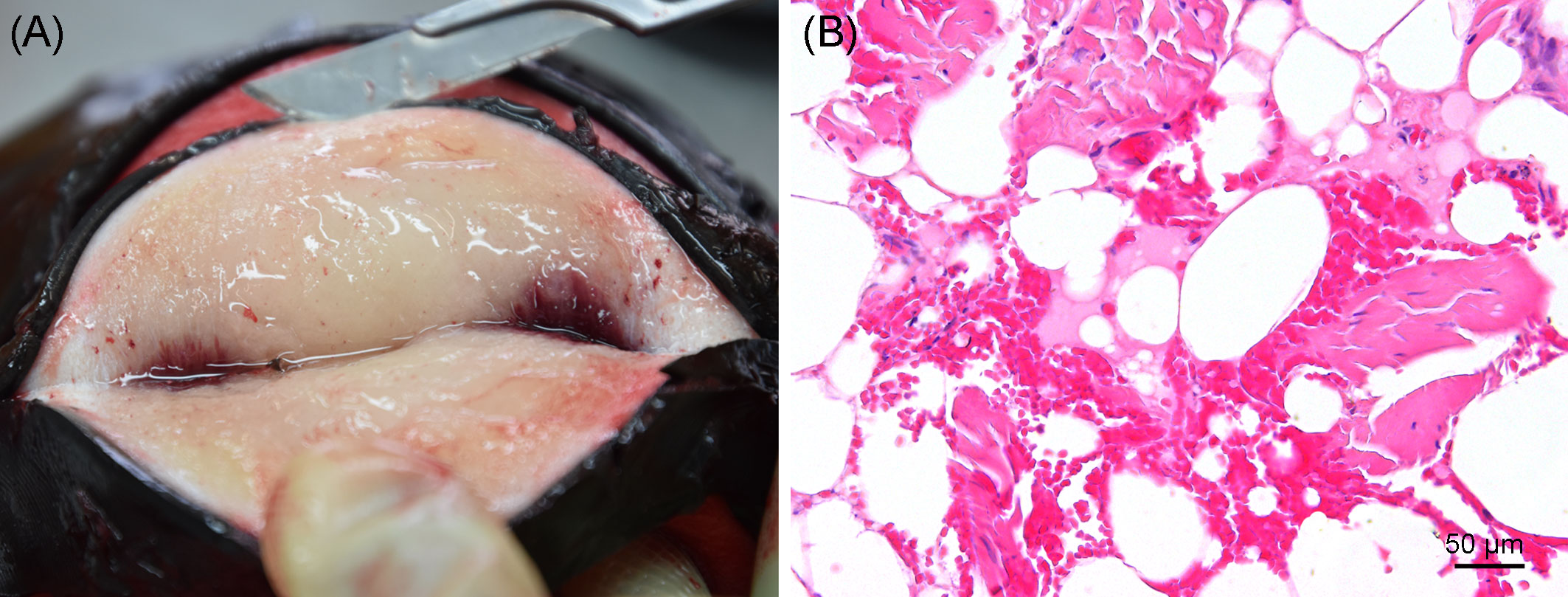
Figure 6 (A) Gross image of the melon at necropsy. (B) Focal acute hemorrhage in the fatty tissue of the melon; hematoxylin and eosin stain.
Microbiology
A severe growth of Aspergillus sp. was detected in both the lung and the pulmonary lymph node. The isolate was identified as A. fumigatus because its partial beta-tubulin sequence was 100% identical with the reference strain of this species CBS 133.61 (GenBank accession number AY685150). It is deposited in the Jena Microbial Resource Collection with strain number JMRC:NRZ:3861. No other bacteria or fungi were cultured from the other investigated swabs and tissue samples including the brain. PCRs for influenza A virus, morbillivirus, herpesvirus, and pestivirus were negative.
Parasitology
Parasites were detected in both cranial sinuses (pterygoid and peribullar), lung, heart, esophagus, stomach, and liver of the harbor porpoise. The pterygoid and peribullar sinuses showed a moderate infestation on the right and a severe infestation on the left side with nematodes consistent with Stenurus minor. Both the bronchi and blood vessels of both sides of the lung were severely infected with Torynurus convolutus. In the heart, a mild amount of nematodes were detected, which could be identified as lungworms of the species T. convolutus. Both the esophagus and the first compartment of the stomach were mildly infected with nematodes of the family Anisakidae. In the liver, a mild amount of trematodes was detected, which were morphologically consistent with Campula oblonga.
Pollutant analysis
The ∑PCB7 was 1.640 mg/kg lipid in the blubber and 0.659 mg/kg lipid in the liver. The highest concentrations were observed for the hydrophobic hexachlorinated PCB 153 (800 vs. 470 µg/kg lipid) and the lowest for the less bioaccumulative tri- and tetrachlorinated PCBs 28 (4.63 vs. 1.53 µg/kg lipid) and 52, which showed 3.49 µg/kg lipid in blubber and was below the method detection limit in the liver. Additional PCB congeners were observed, in particular in the blubber tissue (PCBs 18, 44, 149, and 170) but partly also in the liver tissue (PCB 149).
Ear analysis
Left ear: High-resolution peripheral quantitative computed tomography scan and histopathology
The HR-pQCT scan of the tympano-periotic complex did not show apparent abnormalities (Figures 7A–C).
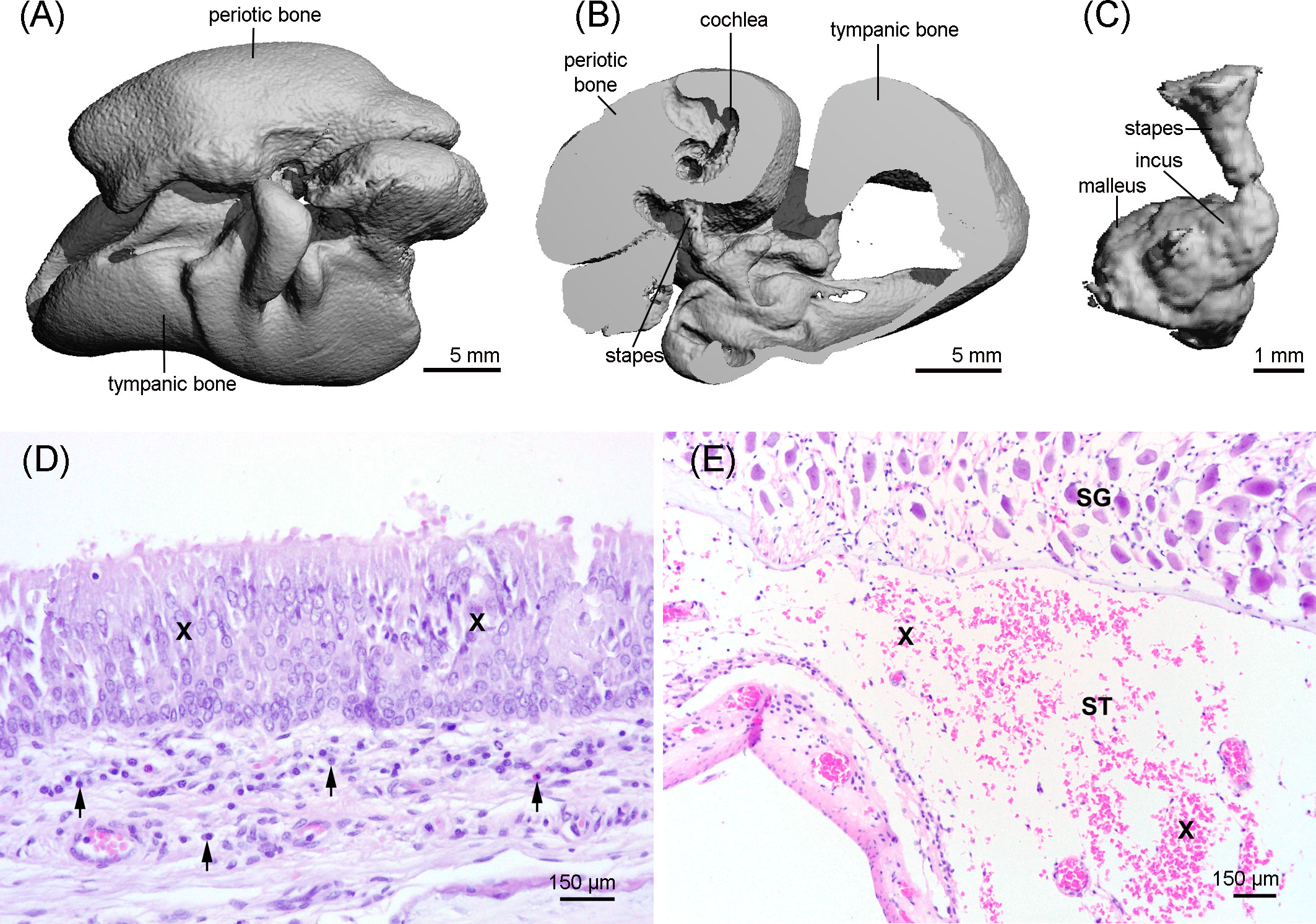
Figure 7 3D reconstruction of the tympano-periotic complex (A, B) and the middle ear ossicles (C) showing an apparent normal morphology. (D) Middle ear with moderate-to-marked epithelial hyperplasia (X) and diffuse mild subepithelial inflammation (arrows), hematoxylin and eosin stain. (E) Moderate acute hemorrhage (X) in the scala tympani (ST); SG: spiral ganglion cells; hematoxylin and eosin stain.
The histopathological evaluation of the tympano-periotic complex revealed a moderate endoparasitosis in the tympanic cavity with intraluminal adult and larval nematodes admixed with few eosinophils and macrophages. In the subepithelial connective tissue, a mild diffuse lymphohistiocytic and plasmacytic inflammation with single eosinophilic granulocytes was present. The epithelium showed partially a moderate to marked epithelial hyperplasia (Figure 7D). In the peribullar sinus, a moderate endoparasitosis with nematodes, mild eosinophilic and lymphoplasmacytic inflammation, and segmental pseudosquamous metaplasia of the respiratory epithelium was found. In the inner ear, there were moderate acute hemorrhages in the scala tympani (Figure 7E), but there was no evidence of the presence of parasites.
Right ear: Immunofluorescence
During the dissection of the cochlea, the round window niche and the vein toward the cochlear aqueduct were subsampled for histopathology. There was a moderate amount of fibrous connective tissue arranged in two tissue strands, in between a loosely arranged fibrovascular tissue web with few capillaries in the round window niche. In addition, there was a moderate amount of fibrous connective tissue with severely hyperemic blood vessels attached to bone in the vein toward the cochlear aqueduct. However, there was no evidence of degenerative or inflammatory changes in any of the two structures.
The immunofluorescence analysis of the right cochlea of this individual revealed that there was a region in the apex of the cochlea (region that encodes for low-frequency sounds) where there was focal loss of OHCs (white arrows in Figure 8). There was a first region of approximately 120 µm with one-to-two rows of OHCs, considered as the normal morphological variability of the apex. Following this first region, there were several regions with the loss of OHCs from 120 to 150 µm, 170 to 200 µm, 250 to 290 µm, 435 to 465 µm, 495 to 545 µm, and 565 to 580 μm from the begining of the apex. The three latter regions showed a total loss of OHCs (Figures 8C–E). After 580 µm from the apex, there was a transition zone of approximately 285 µm with a few missing OHCs, and, from 865 µm from the beginning of the apex and onwards, the typical pattern of three rows of OHCs was observed. Thus, the OHCs of the organ of Corti of the rest of the spiral did not show evidences of lesions (Figure 9). The IHCs showed post-mortem decomposition artifacts and were in reasonably good condition for diagnosis in several regions of the apical and middle turn. In those locations where the IHCs were present, there were no evidences of lesions (Figures 8, 9). In addition, there was no apparent degeneration of the spiral ganglion neurons in any region of the cochlear spiral. The controls were negative (Figure 9B).
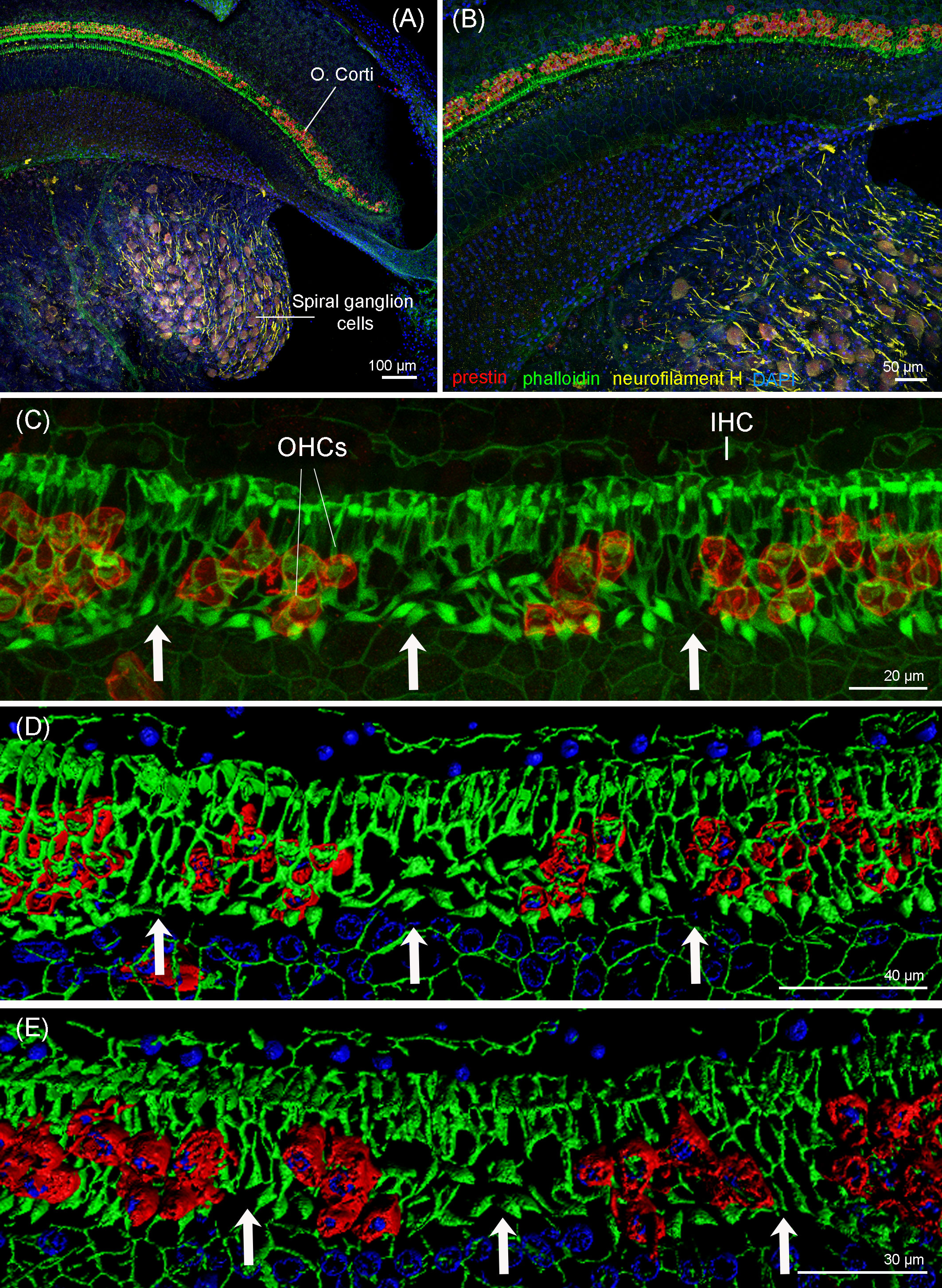
Figure 8 Maximum projections (up to 289 planes each 0.3–3 μm, depending on the magnification) from confocal microscope images of the beginning of the apex of the right cochlea of the harbor porpoise at 10× (A), 20× (B), and 63× (C) magnification. The white arrows highlight the regions with evidence of outer hair cell loss. (D, E) 3D reconstruction of the region of the cochlea in (C) from the vestibular (D) and tympanic (E) scala. The apical turn of the cochlea was labeled with anti-prestin (red) and anti-neurofilament H (yellow) antibodies; phalloidin (green) and nuclei were counterstained with DAPI (blue).
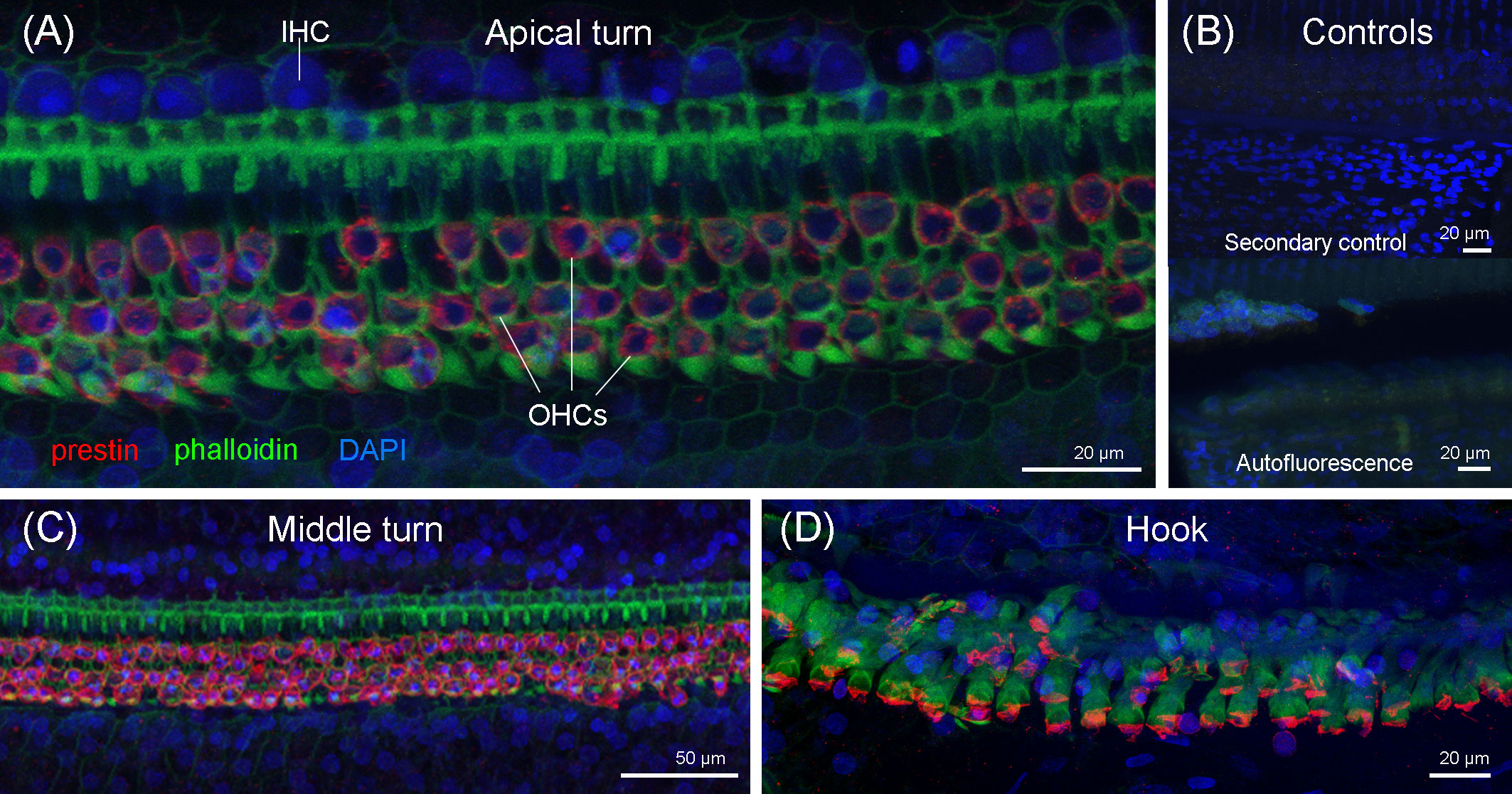
Figure 9 Maximum projections (up to 424 planes each 0.3–1 μm, depending on the magnification) from the confocal microscope images of the apical turn (A), middle turn (C), and extreme basal turn or hook (D) of the right cochlea of the harbor porpoise. (B) Secondary (control for the non-specific binding of the secondary antibodies) and autofluorescence controls were taken with the same settings as the apical region (Figure 8C).
Discussion
Summary
In this study, a live stranded female harbor porpoise that recently had given birth was investigated. It died due to infection with A. fumigatus. The evidence of non-related hearing loss was present in the inner ears, which was compatible with noise exposure. Linear skin markings represented unusual yet additional findings with no clinical relevance for the animal.
Fatal aspergillosis
Detailed post-mortem investigations determined that the porpoise in this study died due to severe aspergillosis. Aspergillosis is considered a sporadic infection in cetaceans (St. Leger et al., 2018). Despite occurring as coinfection together with various morbilliviruses in immunocompromised individuals (Domingo et al., 1992a; Cassle et al., 2016; St. Leger et al., 2018), infections with morbilliviruses and thus coinfections are rare in harbor porpoises (Kennedy et al., 1991; Jepson et al., 2000). So far, only a few cases of aspergillosis have been detected in harbor porpoise stranding schemes: Kapetanou et al. (2020) found 11 out of 754 porpoises (i.e., 1.5%) from the Dutch North Sea to be infected. In contrast, a higher frequency of aspergillosis (9/61, 14.7%) was detected by van Elk et al. (2019) in porpoises from Dutch, Belgian, and German coastlines, whereas all those animals died during attempted rehabilitation, which might have favored infection (Kapetanou et al., 2020). There were 14 additional cases of mycotic infections in harbor porpoises recorded by the stranding network covering the coastline of Northern Germany between 1995 and 2019. This includes Aspergillus sp. (n = 2, mild growth), A. niger (n = 2, single colonies), Mucor sp. (n = 2, single colonies) and Rhizopus sp. (n = 8, single colonies, moderate growth). All other documented cases of aspergillosis (n = 4) known to the authors originated from the UK (Dagleish et al., 2006; Prahl et al., 2011; St. Leger et al., 2018) and Denmark (Seibel et al., 2010).
Primary viral or bacterial infections and an impaired immune status may facilitate the spread of otherwise commensal fungi in cetaceans, which can then lead to secondary infection (Geraci and Ridgway, 1991; Domingo et al., 1992b; Jepson et al., 2000; Cassle et al., 2016; Reidarson et al., 2018; St. Leger et al., 2018). In the herein-presented case, the investigated porpoise had a moderate nutritional condition and an empty stomach, indicating that the animal had not eaten recently before death [for example, Wisniewska et al. (2016), but see also Hoekendijk et al. (2018)]. Even though this is not indicative for reduced immunity itself, an empty stomach might be a sign of general poor health condition, as porpoises usually constantly feed to meet high energetic demands (Gaskin, 1978; Kastelein et al., 1997; Wisniewska et al., 2016; Hoekendijk et al., 2018).
Pregnancy can lead to a changed immune response and therefore facilitate the spread of pathogens (Bridwell et al., 2019; Rohner et al., 2020). Together with the severe parasitosis of the lung and evidence of low-frequency hearing loss, an overall reduction of the immune system functioning therefore seems possible. In addition, nematode infections of harbor porpoise lungs are positively correlated with bronchopneumonia (Lehnert et al., 2005), which may facilitate the entrance and maintenance of secondary infections, like aspergillosis. The suspected route of infection for Aspergillus spp. is usually via the respiratory tract, and, later on, transmission via the blood vessel system is common (Prahl et al., 2011; St. Leger et al., 2018; van Elk et al., 2019). This was most likely also the case in the study animal, which suffered from severe pyogranulomatous and necrotizing pneumonia and, likewise, lymphadenitis of the associated lymph node, caused by A. fumigatus. Whereas van Elk et al. (2019) also proposed the ears as a possible port of entry in cases of aspergillosis in porpoises, Prahl et al. (2011) endorsed the respiratory tract as primary location of infection in their investigated case of A. terreus infection in the ear of a harbor porpoise. Since we did not find evidences of fungal elements in the ears of the porpoise studied here, a spread from the lungs and pulmonary lymph nodes to the cerebellum was most likely the pathway of infection.
The observed granulomatous and necrotizing meningoencephalitis with arteritis and intralesional fungal structures in the study animal most likely represented hematogenous metastatic spread of A. fumigatus. Similar pathology was suspected in a previous case of a porpoise from the UK with an intracranial granuloma due to A. fumigatus infection, even though no infection of the lung could be observed (Dagleish et al., 2006). Noteworthy, Dagleish et al. (2006) further hypothesized that asporogenic Aspergillus spp. might be overlooked during sampling as they are located deep in the respective tissue, which could explain the lack of corresponding pneumonia in their case. In the Netherlands, the respiratory tract was the most affected organ system in a study on mycotic infections in porpoises, whereas only one individual with pulmonal aspergillosis also showed encephalitis (Kapetanou et al., 2020). Another study with animals from Dutch, Belgian, and German coastlines found a similar pattern, with also only one porpoise showing both pneumonia and dissemination to the brain (van Elk et al., 2019). Fatal mycotic encephalitis due to A. fumigatus infection originated in the respiratory tract of a Northern bottlenose whale (Hyperoodon ampullatus) (Dagleish et al., 2008), closely resembling the observed pathology in this study animal. Interestingly, Reidarson et al. (2018) considered the A. fumigatus mycoses of the central nervous system as more characteristic among cetaceans, including aberrant neurological signs and acute death. This perception coincides with the observed behavioral disorder in the study animal, which reportedly swam upside down before it died shortly after its initial live-stranding. Mostly A. fumigatus but also A. terreus and A. niger are isolated from marine mammals, such as cetaceans (St. Leger et al., 2018). To date, there is only one other reported case of mycotic infection of the central nervous system in a harbor porpoise from the Baltic Sea, but, contrarily to this study, the phenomenon was caused by Rhizopus sp. (Wünschmann et al., 1999).
Low-frequency hearing loss
Inner ear analysis by immunofluorescence revealed evidence of focal OHC loss in several regions from 120 to 580 µm from the apex of the right cochlea. This pattern of OHC loss differs from previous descriptions of the normal anatomy of the organ of Corti in harbor porpoises using several imaging techniques (Morell et al., 2015; Morell et al., 2020; Morell et al., 2022a). The lack of OHCs (especially OHCs from the third row) can be considered part of the normal apical variability in harbor porpoise and other mammals (Morell et al., 2022a). However, because the ratio of hair cells and supporting Deiters cells is 1:1 (Morell et al., 2022a), there was strong evidence of scars as a result of OHC death by apoptosis in our case, rather than artefact or normal anatomic variation, as observed in the first 120 µm from the apex. OHC death in mammals results in permanent hearing loss for the associated frequencies (Saunders et al., 1991; Kujawa and Liberman, 2019; Burton et al., 2020). The selection of antibodies used for this sample was optimal for discriminating between newly formed lesions and old ones (Morell et al., 2020). Phalloidin was selected as it is able to identify lesions up to 9 h postexposure and the anti-prestin antibody up to 9–10 days postexposure to noise or ototoxic drugs, according to the rate of scar formation in terrestrial mammals (Raphael and Altschuler, 1991; Abrashkin et al., 2006). In the cases of recent noise-induced hearing loss in guinea pigs, clumps of the protein prestin were found in the cytoplasm of the supporting cells up to 9–10 days postexposure (Abrashkin et al., 2006). Presuming a similar rate of scar formation occurs in harbor porpoises, the loss of hair cells from the right ear of this individual was not associated with recent injury.
Permanent hearing loss can be caused by several factors, including exposure to noise, ototoxic drugs, pollutants such as PCBs, age, barotrauma, blast injury, congenital or immunological disorders, and other infections (Johnsson and Hawkins, 1972; Black et al., 1976; Lim and Dunn, 1979; Sun, 1987; Crofton et al., 2000; Cho et al., 2013).
Mycotic infections of the inner ear have only rarely been reported in humans (Meyerhoff et al., 1979; Lyos et al., 1993) and are found especially in immunocompetent patients (Cho et al., 2007). While the main routes of fungal infection in the inner ear are assumed to be through the middle ear, meningogenic and hematogenic (Meyerhoff et al., 1979), little is known on the specific characteristics of the pathophysiology of fungal infections in the cochlea. Prahl et al. (2011) described the lesions in the middle and inner ear of a porpoise with a severe infection of A. terreus. The sensory and supporting cells of the organ of Corti were replaced by (or dedifferentiated by) normal epithelial cells, forming a cuboidal epithelium. Only a few inner and outer pillar cells were left towards the apical region. The types of lesions described after Aspergillus spp. infection are different from the lesions observed in the porpoise of our study, where there were no apparent degenerated spiral ganglion neurons, nor OHC loss at the base of the cochlea. Pathogenic impacts of sensorineural hearing loss have been described to affect the entire hearing range uniformly in humans and terrestrial mammals, or result in hearing loss predominantly in the high frequencies, which are encoded at the cochlear base (Sheridan, 1964; Chandrasekhar et al., 2000; Cureoglu et al., 2004; Cohen et al., 2014). At present, there are no recognized pathogens exclusively and focally affecting the apex of the cochlea, to the best of our knowledge. Therefore, based on the location and pattern of OHC loss in this case, an infectious etiology is unlikely.
Presbycusis (age-related hearing loss), barotrauma, and ototoxic drug exposure primarily affect high frequencies (Johnsson and Hawkins, 1972; Sun, 1987; Houser and Finneran, 2006; Houser et al., 2008; Wang and Puel, 2018). However, there were no apparent lesions in the sensorineural epithelium at the base of the cochlea that were consistent with presbycusis, ototoxic drug exposure, or barotrauma.
Exposure to PCBs during developmental stages can result in severe hearing loss with a corresponding mild-to-moderate loss of OHCs in the apical and upper-middle turns in rats (Goldey et al., 1995; Herr et al., 1996; Crofton et al., 2000). The animal of our study had a total lipid-normalized ∑PCB7-concentration of 1.640 mg/kg in the blubber and 0.659 mg/kg in the liver. Levels in the blubber and liver were below the currently known thresholds considered to cause physiological effects in marine mammals (Kannan et al., 2000a; Jepson et al., 2005; Murphy et al., 2015). It is hence unlikely that overexposure to PCBs alone was a plausible cause for the OHC death, although more studies are needed on PCB levels in different types of tissues and fluids and their effects on hearing. In addition, there are large numbers of other pollutants present in the environment that accumulate to different degrees in biota tissues, possibly eliciting mixture effects of both legacy and new, emerging pollutants that cannot be fully assessed with the present methodologies.
Other causes in humans that are rarely reported, such as developmental defects or immunological disorders, were deemed unlikely for their extremely low prevalence [less than 1% of all cases of hearing impairment in humans (Mancini et al., 2018)]. Morell et al. (2022b) recently described a case of congenital hearing loss in a harbor seal, with selective IHC loss while OHCs appeared normal, which is different from the pathophysiology observed in the porpoise presented here.
The type of lesions seen in the cochlea of the individual in our study are compatible with noise-induced hearing loss. The location of noise-induced damage within the cochlea depends on the frequency of the source. Previous research on marine and terrestrial mammals exposed to high-intensity noise levels showed that the frequency of maximum hearing loss was half an octave, up to one octave, above the exposing tone (Davis et al., 1950; Kastelein et al., 2014; Finneran, 2015; Reichmuth et al., 2019). Future research on predicting the cochlear frequency maps (i.e., frequency distribution along the cochlear spiral) on harbor porpoises based on morphological features is needed. These frequency maps are important for determining the frequency range that is impaired if lesions are found. In addition, in the cases of noise-induced hearing loss, frequency maps can ultimately provide key information on the frequency characteristics of the causal sources of the damage in the cochlea. Although, at this moment, we do not know the exact frequency range that is impaired in this individual, it is likely that the low-frequency hearing loss is outside the frequency band that porpoises use in their acoustic repertoire, with a peak and centroid frequencies ranging from 129 to 145 kHz (Villadsgaard et al., 2007). It is challenging to assess the consequences of having a hearing loss in the low frequencies for harbor porpoises. In part, this is due to the difficulty to provide a large number of individuals whose ears can be fixed rapidly after their death. To date, 3 out of 21 (14.3%) harbor porpoise individuals that had the beginning of the apex in a good preservation status for diagnosis, showed evidences of low-frequency hearing loss (Morell et al., 2015; Morell et al., 2021; Morell et al., 2022a; Ijsseldijk et al., 2022). However, this prevalence may be underestimated since not all 21 individuals had a complete information of the apex. Morell et al. (2021) described a case of low-frequency hearing loss with unrelated severe cerebral toxoplasmosis in a harbor porpoise. Together with the individual of this study, evidence of hearing loss in porpoises with unrelated severe infections may suggest that damage in the auditory apparatus could ultimately reduce the immune system functioning in affected animals. However, further research combining ear analysis with pathological findings of other vital organs in porpoises should be conducted to establish a potential correlation between hearing impairment and vulnerability due to the exposure of pathogens.
Previous cases reported harbor porpoises from the North and Baltic Seas with sublethal lesions in the middle or inner ears (Morell et al., 2017b; Wohlsein et al., 2019; Morell et al., 2021; Siebert et al., 2022), which highlighted the importance of including the ear analysis in full post-mortem examinations. Evidence of hearing loss was also reported in by-caught porpoises, e.g., for one individual with a severe hemorrhage in the inner ear, most likely caused by a parasitic infection (Morell et al., 2017b), as well as otitis media (2 out of 16 individuals), and a healed fracture of the tympanic bone in 1 out of 16 individuals (Seibel et al., 2010; Wohlsein et al., 2019). Wohlsein et al. (2019) concluded that damage to hearing structures may explain starvation due to the impaired ability to catch prey or unusual behavior such as entanglement in nets or stranding. There was neither evidence of the dislocations of the middle ear ossicles and hemorrhages, nor degeneration or death of sensory cells and type I afferent innervation detected in the base of the cochlea in this animal, which are indications of blast trauma (Cho et al., 2013; Siebert et al., 2022). There were no hemorrhages, ruptures of alveolar walls, or lacerations of larger parts of the lung. In addition, myocardial, meningeal, and intracerebral hemorrhages were described in the cases of blast injury in terrestrial mammals (Clemedson, 1956). The hemorrhages into the tympanic scala at the base of the cochlea and the perivascular hemorrhages in the central nervous system were acute without the evidence of resorptive reactions. They possibly represent agonal changes. The moderate acute hemorrhage found in the melon in the porpoise could be related to scavenging, rather than related with blast injury.
Linear skin markings
The detected parallel stripes bilaterally on flanks of the study animal resembled observations made in common bottlenose dolphins (Tursiops truncatus) in Florida (Titcomb et al., 2020) and, to a lesser extent, in Hawaii (Noren et al., 2011). The so-called linear skin markings (LSMs) were thought to represent stretch marks (Striae distensae) in humans (Schuck et al., 2020). Notably, 85.1% of the affected study animals in Florida (N = 96) showing LSMs were females that all had given birth at least once before (Titcomb et al., 2020). However, the histological investigations of the studied porpoise only found an undulating surface without cellular reaction in the LSMs, while scarred tissue is to be expected in human stretch marks (Salter and Kimball, 2006). Despite the etiology remaining unclear in this case, such LSMs have not been described for harbor porpoises before, nor have they been documented in cetaceans from the Baltic Sea, to the knowledge of the authors.
The rapid change in the marine environment and growing anthropogenic influence may foster the occurrence of mycotic infections, as well as infections of other etiologies, in marine mammals (Kapetanou et al., 2020) in the future, in addition to increase the underwater noise levels. Full comprehensive post-mortem examinations with extended sampling protocols, including sound reception structures, are needed to understand the effects of natural and anthropogenic stressors and the combination of both on marine mammals.
Data availability statement
The original contributions presented in the study are included in the article. Further inquiries can be directed to the corresponding author.
Ethics statement
Ethical review and approval was not required for the animal study because the investigated animal was collected once it was dead within the German stranding network, which conducts work (collect and hold carcasses and samples from European protected species) on German strandings following appropriate licenses from the relevant authorities (Ministry of Energy, Agriculture, the Environment, Nature and Digitalization, Ministry of Agriculture, Environment and Rural areas, Permit Nr. DE 03 201 0046 21).
Author contributions
SR conducted the necropsy. MM performed the ear collection, inner ear dissection, imaging, and image interpretation. PW was in charge of the histopathological evaluation of all organs. JS did the X-ray and CT scanning and image interpretation. AVF supervised the confocal imaging and prepared the 3D reconstructions of the organ of Corti. LCS performed the parasitological identification. EP-B and CW did microbiological analysis, GW the fungal identification, and EBR and AJ pollutant analysis. SR, MM, PW, JS, EBR, AJ, EP-B, CE, GW, LCS, AVF, and US helped with writing and editing the manuscript. US and PW supervised the work and its publication. US acquired the funding. All authors contributed to the article and approved the submitted version.
Funding
The study was funded by the Ministry for Energy Transition, Agriculture, Environment, Nature, and Digitalization of Schleswig-Holstein (MELUND, reference ZB-50-21-1051000-4121.5) and the Schleswig-Holstein Agency for Coastal Defence, National Park and Marine Conservation (LKN.SH). The analytical instrument used in this study is part of the major infrastructure initiative CITEPro (Chemicals in the Environment Profiler) funded by the Helmholtz Association, and analysis was supported by the Helmholtz EXPOSO-METER project (W2/W3-126). This Open Access publication was funded by the Deutsche Forschungsgemeinschaft (DFG, German Research Foundation) - 491094227 "Open Access Publication Funding" and the University of Veterinary Medicine Hannover, Foundation.
Acknowledgments
We thank Eckhard Kasten for recovering the porpoise. Furthermore, special thanks are for Miriam Hillmann and Kornelia Wolff-Schmidt for their contribution in the cementum aging. The authors are grateful to Jana Klink who assisted in the necropsy and the Institute of Virology who performed virology analysis. The authors thank Jörg Watzke for help with extraction and sample preparation for PCB analysis (funded by the ERC Starting Grant CHEMO-RISK, grant agreement no. 715173). In addition, we would like to thank the team at UKE Hamburg Microscopy Imaging Facility for technical assistance and Dr. Jing Zheng (Northwestern University) who kindly provided the anti-prestin antibody for this study (ref. NW958).
Conflict of interest
The authors declare that the research was conducted in the absence of any commercial or financial relationships that could be construed as a potential conflict of interest.
The reviewer LI declared a past collaboration with several of the authors MM, PW, US to the handling Editor.
Publisher’s note
All claims expressed in this article are solely those of the authors and do not necessarily represent those of their affiliated organizations, or those of the publisher, the editors and the reviewers. Any product that may be evaluated in this article, or claim that may be made by its manufacturer, is not guaranteed or endorsed by the publisher.
References
Abrashkin K. A., Izumikawa M., Miyazawa T., Wang C. H., Crumling M. A., Swiderski D. L., et al. (2006). The fate of outer hair cells after acoustic or ototoxic insults. Hear. Res. 218, 20–29. doi: 10.1016/j.heares.2006.04.001
Arnold P. W., Gaskin D. E. (1975). Lungworms (Metastrongyloidea: Pseudaliidae) of harbor porpoise Phocoena phocoena (L. 1758). Can. J. Zool. 53, 713–735. doi: 10.1139/z75-087
ASCOBANS (2002). Recovery plan for Baltic harbour porpoises (Jastarnia plan). ASCOBANS Rep. 7, 1–39. Available at: https://www.ascobans.org/en/document/draft-recovery-plan-baltic-harbour-porpoisesjastarnia-plan.
ASCOBANS (2012). Conservation plan for the harbour porpoise population in the Western Baltic, the belt Sea and the kattegat. ASCOBANS Rep., 1–38. Available at: https://www.ascobans.org/en/documents/action%20plans/Western-Baltic-Conservation-Plan.
Beineke A., Siebert U., McLachlan M., Bruhn R., Thron K., Failing K., et al. (2005). Investigations of the potential influence of environmental contaminants on the thymus and spleen of harbor porpoises (Phocoena phocoena). Environ. Sci. Technol. 39, 3933–3938. doi: 10.1021/es048709j
Benke H., Bräger S., Dähne M., Gallus A., Hansen S., Honnef C. G., et al. (2014). Baltic Sea Harbour porpoise populations: status and conservation needs derived from recent survey results. Mar. Ecol. Prog. Ser. 495, 275–290. doi: 10.3354/meps10538
Berggren P. (1994). Bycatches of the harbour porpoise (Phocoena phocoena) in the Swedish skagerrak, kattegat and Baltic seas 1973-1993. Rep. Int. Whal. Commun. 15, 211–215.
Biberstein E. L. (1999). “Agents of systemic mycoses,” in Veterinary microbiology. Eds. Hirsh D. C., Chung Zee Y. (Malden, MA, USA: Blackwell Science), 256–273.
Black R. E., Lau W. K., Weinstein R. J., Young L. S., Hewitt W. L. (1976). Ototoxicity of amikacin. Antimicrob. Agents Chemother. 9, 956–961. doi: 10.1128/AAC.9.6.956
Bossart G. D. (2011). Marine mammals as sentinel species for oceans and human health. Vet. Pathol. 48, 676–690. doi: 10.1177/0300985810388525
Bredberg G., Ades H. W., Engström H. (1972). Scanning electron microscopy of the normal and pathologically altered organ of corti. Acta Otolaryngol. 73, 3–48. doi: 10.3109/00016487209122688
Bridwell R. E., Carius B. M., Long B., Oliver J. J., Schmitz G. (2019). Sepsis in pregnancy: Recognition and resuscitation. West. J. Emerg. Med. 20, 822–832. doi: 10.5811/westjem.2019.6.43369
Bruhn R., Kannan N., Petrick G., Schulz-Bull D. E., Duinker J. C. (1999). Persistent chlorinated organic contaminants in harbour porpoises from the north Sea, the Baltic Sea and Arctic waters. Sci. Total Environ. 237–238, 351–361. doi: 10.1016/S0048-9697(99)00148-5
Burton J. A., Mackey C. A., MacDonald K. S., Hackett T. A., Ramachandran R. (2020). Changes in audiometric threshold and frequency selectivity correlate with cochlear histopathology in macaque monkeys with permanent noise-induced hearing loss. Hear. Res. 398, 1–13. doi: 10.1016/j.heares.2020.108082
Cassle S. E., Landrau-Giovannetti N., Farina L. L., Leone A., Wellehan J. F. X., Stacy N. I., et al. (2016). Coinfection by cetacean morbillivirus and Aspergillus fumigatus in a juvenile bottlenose dolphin (Tursiops truncatus) in the gulf of Mexico. J. Vet. Diagn. Investig. 28, 729–734. doi: 10.1177/1040638716664761
Chandrasekhar S. S., Connelly P. E., Brahmbhatt S. S., Shah C. S., Kloser P. C., Baredes S. (2000). Otologic and audiologic evaluation of human immunodeficiency virus- infected patients. Am. J. Otolaryngol. - Head Neck Med. Surg. 21, 1–9. doi: 10.1016/S0196-0709(00)80117-9
Cho S., Gao S. S., Xia A., Wang R., Salles F. T., Raphael P. D., et al. (2013). Mechanisms of hearing loss after blast injury to the ear. PloS One 8, e67618. doi: 10.1371/journal.pone.0067618
Cho Y. S., Lee D. K., Hong S. D., Oh W. S. (2007). Intracranial aspergillosis involving the internal auditory canal and inner ear in an immunocompetent patient. Am. J. Neuroradiol. 28, 138–140.
Christensen O. (1991) Bycatches in the salmon drift net fishery in the Baltic Sea. Available at: http://scholar.google.com/scholar?hl=en&btnG=Search&q=intitle:BYCATCHES+IN+THE+SALMON+DRIFT+NET+FISHERY+IN+THE+BALTIC+SEA.
Christian Kinze C., Czeck R., Herr H., Siebert U. (2021). Cetacean strandings along the German north Sea coastline 1604–2017. J. Mar. Biol. Assoc. United Kingdom 101, 483–502. doi: 10.1017/S0025315421000503
Cohen B. E., Durstenfeld A., Roehm P. C. (2014). Viral causes of hearing loss: A review for hearing health professionals. Trends Hear. 18, 1–17. doi: 10.1177/2331216514541361
Crofton K. M., Ding D. L., Padich R., Taylor M., Henderson D. (2000). Hearing loss following exposure during development to polychlorinated biphenyls: A cochlear site of action. Hear. Res. 144, 196–204. doi: 10.1016/S0378-5955(00)00062-9
Cureoglu S., Schachern P. A., Paparella M. M., Lindgren B. R. (2004). Cochlear changes in chronic otitis media. Laryngoscope 114, 622–626. doi: 10.1097/00005537-200404000-00006
Dagleish M. P., Buxton D., Patterson I. A. P., Foster G., Reid R. J., Linton C. (2006). Intracranial granuloma caused by asporogenic Aspergillus fumigatus in a harbour porpoise (Phocoena phocoena). Vet. Rec. 159, 458–460. doi: 10.1136/vr.159.14.458
Dagleish M. P., Foster G., Howie F. E., Reid R. J., Barley J. (2008). Fatal mycotic encephalitis caused by Aspergillus fumigatus in a northern bottlenose whale (Hyperoodon ampullatus). Vet. Rec. 163, 602–604. doi: 10.1136/vr.163.20.602
Das K., Vossen A., Tolley K., Víkingsson G., Thron K., Müller G., et al. (2006). Interfollicular fibrosis in the thyroid of the harbour porpoise: An endocrine disruption? Arch. Environ. Contam. Toxicol. 51, 720–729. doi: 10.1007/s00244-005-0098-4
Davis H., Morgan C. T., Hawkins J. E., Galambos R., Smith F. W. (1950). Temporary deafness following exposure to loud tones and noise. Acta Otolaryngol. 88, 1–56.
Dietz R., Sonne C., Jenssen B. M., Das K., de Wit C. A., Harding K. C., et al. (2021). The Baltic Sea: An ecosystem with multiple stressors. Environ. Int. 147, 106324. doi: 10.1016/j.envint.2020.106324
Domingo M., Visa J., Pumarola M., Marco A. J., Ferrer L., Rabanal R., et al. (1992a). Pathologic and immunocytochemical studies of morbillivirus infection in striped dolphins (Stenella coeruleoalba). Vet. Pathol. 29, 1–10. doi: 10.1177/030098589202900101
Domingo M., Visa J., Pumarola M., Marco A. J., Ferrer L., Rabanal R., et al. (1992b). Pathological and immunocytochemical studies of morbillivirus infection in striped dolphins (Stenella coeruleoalba). Vet. Pathol. 29, 1–10. doi: 10.1177/030098589202900101
Erbe C., Dunlop R., Dolman S. (2018). “Effects of noise on marine mammals,” in Effects of anthropogenic noise on animals. Eds. Slabbekoorn H., Popper A. N., Dooling R. J., Fay R. R. (New York: Springer), 277–309. doi: 10.1007/978-1-4939-8574-6
European Union (2008). Directive 2008/56/EC of the European parliament and of the council of 17 June 2008, establishing a framework for community action in the field of marine environmental policy (Marine strategy framework directive). Off. J. Eur. Union, 19–40.
Finneran J. J. (2015). Noise-induced hearing loss in marine mammals : A review of temporary threshold shift studies from 1996 to 2015. J. Acoust. Soc Am. 138, 1702–1726. doi: 10.1121/1.4927418
Galatius A., Kinze C. C., Teilmann J. (2012). Population structure of harbour porpoises in the Baltic region: evidence of separation based on geometric morphometric comparisons. J. Mar. Biol. Assoc. UK 92, 1669–1676. doi: 10.1017/S0025315412000513
Gallus A., Dähne M., Verfuß U. K., Bräger S., Adler S., Siebert U., et al. (2012). Use of static passive acoustic monitoring to assess the status of the ‘Critically endangered’ Baltic harbour porpoise in German waters. Endanger Species Res. 18, 265–278. doi: 10.3354/esr00448
Gaskin D. E. (1978). Form and function in the digestive tract and associated organs in cetacea, with consideration of metabolic rates and specific energy budgets. Oceanogr. Mar. Biol. an Annu. Rev. 16, 313–345.
Geraci J. R., Ridgway S. H. (1991). On disease transmission between cetaceans and humans. Mar. Mammal Sci. 7, 191–194. doi: 10.1111/j.1748-7692.1991.tb00565.x
Goldey E. S., Kehn L. S., Lau C., Rehnberg G. L., Crofton K. M. (1995). Developmental exposure to polychlorinated biphenyls (Aroclor 1254) reduces circulating thyroid hormone concentrations and causes hearing deficits in rats. Toxicol. Appl. Pharmacol. 135, 77–88. doi: 10.1006/taap.1995.1210
Hammond P. S., Berggren P., Benke H., Borchers D. L., Collet A., Heide-Jørgensen M. P., et al. (2002). Abundance of harbour porpoise and other cetaceans in the north Sea and adjacent waters. J. Appl. Ecol. 39, 361–376. doi: 10.1046/j.1365-2664.2002.00713.x
Hammond P. S., Macleod K., Berggren P., Borchers D. L., Burt L., Cañadas A., et al. (2013). Cetacean abundance and distribution in European Atlantic shelf waters to inform conservation and management. Biol. Conserv. 164, 107–122. doi: 10.1016/j.biocon.2013.04.010
HELCOM (2018) Implementation of the Baltic Sea action plan 2018. Available at: https://helcom.fi/wp-content/uploads/2019/06/Implementation-of-the-BSAP-2018.pdf.
Helle E., Olsson M., Jensen S. (1976). PCB Levels correlated with pathological changes in seal uteri. Ambio 5, 261–263. doi: 10.2307/4312230
Herr D. W., Goldey E. S., Crofton K. M. (1996). Developmental exposure to aroclor 1254 produces low-frequency alterations in adult rat brainstem auditory evoked responses. Fundam. Appl. Toxicol. 33, 120–128. doi: 10.1006/faat.1996.0149
Hoekendijk J. P. A., Spitz J., Read A. J., Leopold M. F., Fontaine M. C. (2018). Resilience of harbor porpoises to anthropogenic disturbance: Must they really feed continuously? Mar. Mammal Sci. 34, 258–264. doi: 10.1111/mms.12446
Houser D. S., Finneran J. J. (2006). Variation in the hearing sensitivity of a dolphin population determined through the use of evoked potential audiometry. J. Acoust. Soc Am. 120, 4090–4099. doi: 10.1121/1.2357993
Houser D. S., Gomez-Rubio A., Finneran J. J. (2008). Evoked potential audiometry of 13 pacific bottlenose dolphins (Tursiops truncatus gilli). Mar. Mammal Sci. 24, 28–41. doi: 10.1111/j.1748-7692.2007.00148.x
Huggenberger S., Benke H., Kinze C. C. (2002). Geographical variation in harbour porpoise (Phocoena phocoena) skulls: Support for a separate non-migratory population in the Baltic proper. Ophelia 56, 1–12. doi: 10.1080/00785236.2002.10409484
Hu B. H., Guo W., Wang P. Y., Henderson D., Jiang S. C. (2000). Intense noise-induced apoptosis in hair cells of Guinea pig cochleae. Acta Otolaryngol. 120, 19–24. doi: 10.1080/000164800750044443
IJsseldijk L. L., Brownlow A. C., Mazzariol S. (2019). Best practice on cetacean post mortem investigation and tissue sampling. doi: 10.31219/osf.io/zh4ra
Ijsseldijk L. L., Leopold M. F., Begeman L., Kik M. J. L., Wiersma L., Morell M., et al. (2022). Pathological fi ndings in stranded harbor porpoises (Phocoena phocoena) with special focus on anthropogenic causes. Front. Mar. Sci. 9. doi: 10.3389/fmars.2022.997388
Jensen S., Häggberg L., Jörundsdóttir H., Odham G. (2003). A quantitative lipid extraction method for residue analysis of fish involving nonhalogenated solvents. J. Agric. Food Chem. 51, 5607–5611. doi: 10.1021/jf0301201
Jepson P. D., Bennett P. M., Deaville R., Allchin C. R., Baker J. R., Law R. J. (2005). Relationships between polychlorinated biphenyls and health status in harbor porpoises (Phocoena phocoena) stranded in the united kingdom. Environ. Toxicol. Chem. 24, 238–248. doi: 10.1897/03-663.1
Jepson P. D., Deaville R., Barber J. L., Aguilar À., Borrell A., Murphy S., et al. (2016). PCB Pollution continues to impact populations of orcas and other dolphins in European waters. Sci. Rep. 6, 18573. doi: 10.1038/srep18573
Jepson P. D., Kuiken T., Bennett P. M., Baker J. R., Simpson V. R., Kennedy S. (2000). Pulmonary pathology of harbour porpoises (Phocoena phocoena) stranded in England and Wales between 1990 and 1996. Vet. Rec. 146, 721–728. doi: 10.1136/vr.146.25.721
Johnsson L. G., Hawkins J. E. (1972). Sensory and neural degeneration with aging, as seen in microdissections of human inner ear. Ann. Otol. Rhinol. Laryngol. 81, 179–193. doi: 10.1177/000348947208100203
Jo W. K., van Elk C., van de Bildt M., van Run P., Petry M., Jesse S. T., et al. (2019). An evolutionary divergent pestivirus lacking the npro gene systemically infects a whale species. Emerg. Microbes Infect. 8, 1383–1392. doi: 10.1080/22221751.2019.1664940
Kannan K., Blankenship A. L., Jones P. D., Giesy J. P. (2000a). Toxicity reference values for the toxic effects of polychlorinated biphenyls to aquatic mammals. Hum. Ecol. Risk Assess. 6, 181–201. doi: 10.1080/10807030091124491
Kannan K., Blankenship A. L., Jones P. D., Giesy J. P. (2000b). Toxicity reference values for the toxic effects of polychlorinated biphenyls to aquatic mammals. Hum. Ecol. Risk Assess. Int. J. 6, 181–201. doi: 10.1080/10807030091124491
Kapetanou A., IJsseldijk L. L., Willems D. S., Broens E. M., Everaarts E., Buil J. B., et al. (2020). Mycotic infections in free-ranging harbor porpoises (Phocoena phocoena). Front. Mar. Sci. 7. doi: 10.3389/fmars.2020.00344
Kastelein R. A., Nieuwstraten S. H., Verstegen M. W. A. (1997). “Passage time of carmine red dye through the digestive tract of harbour porpoises (Phocoena phocoena),” in The biology of the harbour porpoise. Eds. Read A. J., Wiepkema P. R., Nachtigall P. E. (Woerden, NL: De Spil Publishers), 265–275.
Kastelein R., Schop J., Gransier R., Hoek L. (2014). Frequency of greatest temporary hearing threshold shift in harbor porpoises (Phocoena phocoena) depends on the noise level. J. Acoust. Soc Am. 136, 1410–1418. doi: 10.1121/1.4892794
Kennedy S., Smyth J., Cush P. F., McAliskey M., McCullough S. J., Rima B. K. (1991). Histopathologic and immunocytochemical studies of distemper in harbor porpoises. Vet. Pathol. 28, 1–7. doi: 10.1177/030098589102800101
Kesselring T., Viquerat S., Brehm R., Siebert U. (2017). Coming of age: - do female harbour porpoises (Phocoena phocoena) from the north Sea and Baltic Sea have sufficient time to reproduce in a human influenced environment? PloS One 12, e0186951. doi: 10.1371/journal.pone.0186951
Kinze C. C. (1988). Incidental catches of harbour porpoises (Phocoena phocoena) in Danish waters 1986-89: Recent data and behavioural implications. Rep. Int. Whal. Commun. 1–14
Kock K.-H., Benke H. (1996). On the by-catch of harbour porpoise (Phocoena phocoena) in German fisheries in the Baltic and the north Sea. Arch. Fish. Mar. Res. 44, 95–114.
Koschinski S. (2001). Current knowledge on harbour porpoises (Phocoena phocoena) in the Baltic Sea. Ophelia 55, 167–197. doi: 10.1080/00785326.2001.10409483
Kröger R. H. H. (1986). The decrease of harbour porpoise populations in the Baltic and north Sea (Germany: Bonn).
Kujawa S. G., Liberman M. C. (2019). Translating animal models to human therapeutics in noise-induced and age-related hearing loss. Hear. Res. 377, 44–52. doi: 10.1016/j.heares.2019.03.003
Lehnert K., Raga J., Siebert U. (2005). Macroparasites in stranded and bycaught harbour porpoises from German and Norwegian waters. Dis. Aquat. Organ. 64, 265–269. doi: 10.3354/dao064265
Lim D. J., Dunn D. E. (1979). Anatomic correlates of noise induced hearing loss. Otolaryngol. Clin. North Am. 12, 493–513. doi: 10.1016/S0030-6665(20)32439-7
Lockyer C. (1995). “A review of factors involved in zonation in odontocete teeth, and an investigation of the likely impact of environmental factors and major life events on harbour porpoise tooth structure,” in Report of the international whaling commission. Eds. Bjørge G. P., Donovan A. 16, 511–529.
Lucke K., Siebert U., Lepper P. A., Blanchet M.-A. (2009). Temporary shift in masked hearing thresholds in a harbor porpoise (Phocoena phocoena) after exposure to seismic airgun stimuli. J. Acoust. Soc Am. 125, 4060–4070. doi: 10.1121/1.3117443
Lyos A. T., Malpica A., Estrada R., Katz C. D., Jenkins H. A. (1993). Invasive aspergillosis of the temporal bone: An unusual manifestation of acquired immunodeficiency syndrome. Am. J. Otolaryngol. Neck Med. Surg. 14, 444–448. doi: 10.1016/0196-0709(93)90121-M
Mancini P., Atturo F., Di Mario A., Portanova G., Ralli M., De Virgilio A., et al. (2018). Hearing loss in autoimmune disorders: Prevalence and therapeutic options. Autoimmun. Rev. 17, 644–652. doi: 10.1016/j.autrev.2018.01.014
Matsuda K., Zheng J., Du G. G., Klöcker N., Madison L. D., Dallos P. (2004). N-linked glycosylation sites of the motor protein prestin: Effects on membrane targeting and electrophysiological function. J. Neurochem. 89, 928–938. doi: 10.1111/j.1471-4159.2004.02377.x
Meinig H., Boye P., Dähne M., Hutterer R., Lang J. (2020). Rote liste und gesamtartenliste der säugetiere (Mammalia) deutschlands. Naturschutz und Biol. Vielfalt 170, 73. doi: 10.19213/972172/
Meyerhoff W. L., Paparella M. M., Makoto O., Shea D. (1979). Mycotic infections of the inner ear. Laryngoscope 89, 1725–1734. doi: 10.1288/00005537-197911000-00003
Morell M., André M. (2009). Cetacean ear extraction and fixation protocol. 2–7. Available at: http://www.zoology.ubc.ca/files/Ear_extraction_and_fixation_protocol_UBC.pdf.
Morell M., Brownlow A., McGovern B., Raverty S. A., Shadwick R. E., André M. (2017a). Implementation of a method to visualize noise-induced hearing loss in mass stranded cetaceans. Sci. Rep. 7, 41848. doi: 10.1038/srep41848
Morell M., Ijsseldijk L. L., Berends A. J., Gröne A., Siebert U., Raverty S. A., et al. (2021). Evidence of hearing loss and unrelated toxoplasmosis in a free - ranging harbour porpoise (Phocoena phocoena). Animals 11, 3085. doi: 10.3390/ani11113058
Morell M., IJsseldijk L. L., Piscitelli-Doshkov M., Ostertag S., Estrade V., Haulena M., et al. (2022a). Cochlear apical morphology in toothed whales: Using the pairing hair cell–deiters’ cell as a marker to detect lesions. Anat. Rec. 305, 622–642. doi: 10.1002/ar.24680
Morell M., Lehnert K., IJsseldijk L., Raverty S., Wohlsein P., Gröne A., et al. (2017b). Parasites in the inner ear of harbour porpoise: cases from the north and Baltic seas. Dis. Aquat. Organ. 127, 57–63. doi: 10.3354/dao03178
Morell M., Lenoir M., Shadwick R. E., Jauniaux T., Dabin W., Begeman L., et al. (2015). Ultrastructure of the odontocete organ of corti: Scanning and transmission electron microscopy. J. Comp. Neurol. 523, 431–448. doi: 10.1002/cne.23688
Morell M., Rojas L., Haulena M., Busse B., Siebert U., Shadwick R. E., et al. (2022b). Selective inner hair cell loss in a neonate harbor seal (Phoca vitulina). Animals 12, 180. doi: 10.3390/ani12020180
Morell M., Vogl A. W., IJsseldijk L. L., Piscitelli-Doshkov M., Tong L., Ostertag S., et al. (2020). Echolocating whales and bats express the motor protein prestin in the inner ear: A potential marker for hearing loss. Front. Vet. Sci. 7. doi: 10.3389/fvets.2020.00429
Murphy S., Barber J. L., Learmonth J. A., Read F. L., Deaville R., Perkins M. W., et al. (2015). Reproductive failure in UK harbour porpoises Phocoena phocoena: Legacy of pollutant exposure? PloS One 10, e0131085. doi: 10.1371/journal.pone.0131085
Muz M., Rojo-Nieto E., Jahnke A. (2021). Removing disturbing matrix constituents from biota extracts from total extraction and silicone-based passive sampling. Environ. Toxicol. Chem. 40, 2693–2704. doi: 10.1002/etc.5153
Nachtsheim D. A., Viquerat S., Ramírez-Martínez N. C., Unger B., Siebert U., Gilles A. (2021). Small cetacean in a human high-use area: Trends in harbor porpoise abundance in the north Sea over two decades. Front. Mar. Sci. 7. doi: 10.3389/fmars.2020.606609
Noren S. R., Redfern J. V., Edwards E. F. (2011). Pregnancy is a drag: Hydrodynamics, kinematics and performance in pre-and postparturition bottlenose dolphins (Tursiops truncatus). J. Exp. Biol. 214, 4151–4159. doi: 10.1242/jeb.059121
Prahl S., Jepson P. D., Sanchez-Hanke M., Deaville R., Siebert U. (2011). Aspergillosis in the middle ear of a harbour porpoise (Phocoena phocoena): A case report. Mycoses 54, 260–264. doi: 10.1111/j.1439-0507.2010.01863.x
Raphael Y., Altschuler R. A. (1991). Reorganization of cytoskeletal and junctional proteins during cochlear hair cell degeneration. Cell Motil. Cytoskeleton 18, 215–227. doi: 10.1002/cm.970180307
Raverty S. A., Duignan P. J., Jepson P. D., Morell M. (2018). “Gross necropsy and specimen collection protocols,” in CRC Handbook of marine mammal MedicineMarine mammal medicine. Eds. Gulland F., Dierauf L. A. (CRC Press/Taylor & Francis Group), 249–266.
Reichmuth C., Sills J. M., Mulsow J., Ghoul A. (2019). Long-term evidence of noise-induced permanent threshold shift in a harbor seal (Phoca vitulina). J. Acoust. Soc Am. 146, 2552–2561. doi: 10.1121/1.5129379
Reidarson T. H., Garcia-Parraga D., Wiederhold N. P. (2018). “Marine mammal mycoses,” in Marine mammal medicine. Eds. Gulland F. M. D., Dierauf L. A., Whitman K. L. (Boca Raton, Fl, USA: CRC Press), 389–423. doi: 10.1201/9781315144931
Reijnders P. J. H. (1992). Harbour porpoises Phocoena phocoena in the north Sea: Numerical responses to changes in environmental conditions. Netherlands J. Aquat. Ecol. 26, 75–85. doi: 10.1007/BF02298029
Rohner S., Hülskötter K., Gross S., Wohlsein P., Abdulmawjood A., Plötz M., et al. (2020). Male Grey seal commits fatal sexual interaction with adult female harbour seals in the German wadden Sea. Sci. Rep. 10, 13679. doi: 10.1038/s41598-020-69986-w
Salter S. A., Kimball A. B. (2006). Striae gravidarum. Clin. Dermatol. 24, 97–100. doi: 10.1016/j.clindermatol.2005.10.008
Saunders J. C., Cohen Y. E., Szymko Y. M. (1991). The structural and functional consequences of acoustic injury in the cochlea and peripheral auditory system: A five year update ontrol. J. Acoust. Soc Am. 90, 136–146. doi: 10.1121/1.401307
Schaffeld T., Schnitzler J. G., Ruser A., Woelfing B., Baltzer J., Siebert U. (2020). Effects of multiple exposures to pile driving noise on harbor porpoise hearing during simulated flights–an evaluation tool. J. Acoust. Soc Am. 147, 685–697. doi: 10.1121/10.0000595
Scheidat M., Gilles A., Kock K., Siebert U. (2008). Harbour porpoise Phocoena phocoena abundance in the southwestern Baltic Sea. Endanger Species Res. 5, 215–223. doi: 10.3354/esr00161
Scheidat M., Gilles A., Siebert U. (2006). “Evaluating the distribution and density of harbour porpoises (Phocoena phocoena) in selected areas in German waters,” in Progress in marine conservation in Europe part IV. Eds. von Nordhein H., Boedecker D., Krause J. C. (Springer Berlin), 189–208.
Schuck D. C., de Carvalho C. M., Sousa M. P. J., Fávero P. P., Martin A. A., Lorencini M., et al. (2020). Unraveling the molecular and cellular mechanisms of stretch marks. J. Cosmet. Dermatol. 19, 190–198. doi: 10.1111/jocd.12974
Seibel H., Beineke A., Siebert U. (2010). Mycotic otitis media in a harbour porpoise (Phocoena phocoena). J. Comp. Pathol. 143, 294–296. doi: 10.1016/j.jcpa.2010.03.002
Seyedmousavi S., Guillot J., Arné P., De Hoog G. S., Mouton J. W., Melchers W. J. G., et al. (2015). Aspergillus and aspergilloses in wild and domestic animals: A global health concern with parallels to human disease. Med. Mycol. 53, 765–797. doi: 10.1093/mmy/myv067
Sheridan M. D. (1964). Final report of a prospective study of children whose mothers had rubella in early pregnancy. Br. Med. J. 2, 536–539. doi: 10.1136/bmj.2.5408.536
Shin D. L., Siebert U., Lakemeyer J., Grilo M., Pawliczka I., Wu N. H., et al. (2019). Highly pathogenic avian influenza A(H5N8) virus in gray seals, Baltic Sea. Emerg. Infect. Dis. 25, 2295–2298. doi: 10.3201/eid2512.181472
Siebert U., Gilles A., Lucke K., Ludwig M., Benke H., Kock K.-H., et al. (2006). A decade of harbour porpoise occurrence in German waters - analyses of aerial surveys, incidental sightings and strandings. J. Sea Res. 56, 65–80. doi: 10.1016/j.seares.2006.01.003
Siebert U., Joiris C., Holsbeek L., Benke H., Failing K., Frese K., et al. (1999). Potential relation between mercury concentrations and necropsy findings in cetaceans from German waters of the north and Baltic seas. Mar. pollut. Bull. 38, 285–295. doi: 10.1016/S0025-326X(98)00147-7
Siebert U., Pawliczka I., Benke H., von Vietinghoff V., Wolf P., Pilāts V., et al. (2020). Health assessment of harbour porpoises (PHOCOENA PHOCOENA) from Baltic area of Denmark, Germany, Poland and Latvia. Environ. Int. 143, 105904. doi: 10.1016/j.envint.2020.105904
Siebert U., Rademaker M., Ulrich S. A., Wohlsein P., Ronnenberg K., Prenger-Berninghoff E. (2017). Bacterial microbiota in harbor seals (Phoca vitulina) from the north Sea of schleswig-Holstein, Germany, around the time of morbillivirus and influenza epidemics. J. Wildl. Dis. 53, 201–214. doi: 10.7589/2015-11-320
Siebert U., Stürznickel J., Schaffeld T., Oheim R., Rolvien T., Prenger-Berninghoff E., et al. (2022). Blast injury on harbour porpoises (Phocoena phocoena) from the Baltic Sea after explosions of deposits of world war II ammunition. Environ. Int. 159, 107014. doi: 10.1016/j.envint.2021.107014
Siebert U., Wünschmann A., Weiss R., Frank H., Benke H., Frese K. (2001). Post-mortem findings in harbour porpoises (Phocoena phocoena) from the German north and Baltic seas. J. Comp. Pathol. 124, 102–114. doi: 10.1053/jcpa.2000.0436
Simmonds M., Dolman S., Jasny M., Parsons E., Weilgart L., Wright A., et al. (2014). Marine noise pollution - increasing recognition but need for more practical action. J. Ocean Technol. 9, 71–90.
Sonne C., Siebert U., Gonnsen K., Desforges J.-P., Eulaers I., Persson S., et al. (2020). Health effects from contaminant exposure in Baltic Sea birds and marine mammals: A review. Environ. Int. 139, 105725. doi: 10.1016/j.envint.2020.105725
St. Leger J., Raverty S., Mena A. (2018). “Cetacea,” in Pathology of wildlife and zoo animals. Eds. Terio K. A., McAloose D., St. Leger J. (London, Cambridge, Oxford, UK; San Diego, CA, USA: Elsevier Academic Press), 533–568. doi: 10.1016/C2015-0-01586-6
Sun J. (1987). Histopathological observation on the inner ear barotrauma in guinea pigs. J. Tongji Med. Univ. 7, 136–142. doi: 10.1007/BF02888206
Sveegaard S., Galatius A., Dietz R., Kyhn L., Koblitz J. C., Amundin M., et al. (2015). Defining management units for cetaceans by combining genetics, morphology, acoustics and satellite tracking. Glob. Ecol. Conserv. 3, 839–850. doi: 10.1016/j.gecco.2015.04.002
Sveegaard S., Teilmann J., Tougaard J., Dietz R., Mouritsen K. N., Desportes G., et al. (2011). High-density areas for harbor porpoises (Phocoena phocoena) identified by satellite tracking. Mar. Mammal Sci. 27, 230–246. doi: 10.1111/j.1748-7692.2010.00379.x
Teilmann J., Carstensen J. (2012). Negative long term effects on harbour porpoises from a large scale offshore wind farm in the Baltic–evidence of slow recovery. Environ. Res. Lett. 7, 45101. doi: 10.1088/1748-9326/7/4/045101
Teilmann J., Sveegaard S., Dietz R. (2011). Status of a harbour porpoise population - evidence for population separation and declining abundance.
Titcomb E. M., Stevens J., Sleeman A., Nelson B., Yrastorza L., Schaefer A. M., et al. (2020). Linear skin markings in common bottlenose dolphins (Tursiops truncatus) from the Indian river lagoon, Florida. Mar. Mammal Sci. 36, 858–870. doi: 10.1111/mms.12685
Tougaard J., Carstensen J., Teilmann J., Skov H., Rasmussen P. (2009). Pile driving zone of responsiveness extends beyond 20 km for harbor porpoises (Phocoena phocoena (L.)). J. Acoust. Soc Am. 126, 11–14. doi: 10.1121/1.3132523
Unger B., Nachtsheim D., Ramírez Martínez N., Siebert U., Sveegaard S., Kyhn L. A., et al. (2021) MiniSCANS-II: Aerial survey for harbour porpoises in the western Baltic Sea, belt Sea, the sound and kattegat in 2020. joint survey by Denmark, Germany and Sweden. Available at: https://www.tiho-hannover.de/fileadmin/57_79_terr_aqua_Wildtierforschung/79_Buesum/downloads/Berichte/20210913_Report_MiniSCANSII_2020_revised.pdf.
van Devanter D. R., Warrener P., Bennett L., Schultz E. R., Coulter S., Garber R. L., et al. (1996). Detection and analysis of diverse herpesviral species by consensus primer PCR. J. Clin. Microbiol. 34, 1666–1671. doi: 10.1128/jcm.34.7.1666-1671.1996
van Elk C. E., van de Bildt M. W. G., van Run P. R. W. A., Bunskoek P., Meerbeek J., Foster G., et al. (2019). Clinical, pathological, and laboratory diagnoses of diseases of harbour porpoises (Phocoena phocoena), live stranded on the Dutch and adjacent coasts from 2003 to 2016. Vet. Res. 50, 88. doi: 10.1186/s13567-019-0706-3
Verna F., Giorda F., Miceli I., Rizzo G., Pautasso A., Romano A., et al. (2017). Detection of morbillivirus infection by RT-PCR RFLP analysis in cetaceans and carnivores. J. Virol. Methods 247, 22–27. doi: 10.1016/j.jviromet.2017.05.009
Villadsgaard A., Wahlberg M., Tougaard J. (2007). Echolocation signals of wild harbour porpoises, Phocoena phocoena. J. Exp. Biol. 210, 56–64. doi: 10.1242/jeb.02618
Viquerat S., Herr H., Gilles A., Peschko V., Siebert U., Sveegaard S., et al. (2014). Abundance of harbour porpoises (Phocoena phocoena) in the western Baltic, belt seas and kattegat. Mar. Biol. 161, 745–754. doi: 10.1007/s00227-013-2374-6
Walther G., Zimmermann A., Theuersbacher J., Kaerger K., von Lilienfeld-Toal M., Roth M., et al. (2021). Eye infections caused by filamentous fungi: Spectrum and antifungal susceptibility of the prevailing agents in Germany. J. Fungi 7, 1–14. doi: 10.3390/jof7070511
Wang J., Puel J. L. (2018). Toward cochlear therapies. Physiol. Rev. 98, 2477–2522. doi: 10.1152/physrev.00053.2017
Wernicke T., Abel S., Escher B. I., Koschorreck J., Rüdel H., Jahnke A. (2022). Equilibrium sampling of suspended particulate matter as a universal proxy for fish and mussel monitoring. Ecotoxicol. Environ. Saf. 232, 1–7. doi: 10.1016/j.ecoenv.2022.113285
Wiemann A., Andersen L. W., Berggren P., Siebert U., Benke H., Teilmann J., et al. (2010). Mitochondrial control region and microsatellite analyses on harbour porpoise (Phocoena phocoena) unravel population differentiation in the Baltic Sea and adjacent waters. Conserv. Genet. 11, 195–211. doi: 10.1007/s10592-009-0023-x
Wisniewska D. M., Johnson M., Teilmann J., Rojano-Doñate L., Shearer J., Sveegaard S., et al. (2016). Ultra-high foraging rates of harbor porpoises make them vulnerable to anthropogenic disturbance. Curr. Biol. 26, 1441–1446. doi: 10.1016/j.cub.2016.03.069
Wohlsein P., Seibel H., Beineke A., Baumgärtner W., Siebert U. (2019). Morphological and pathological findings in the middle and inner ears of harbour porpoises (Phocoena phocoena). J. Comp. Pathol. 172, 93–106. doi: 10.1016/j.jcpa.2019.09.005
Wright A. J., Maar M., Mohn C., Nabe-Nielsen J., Siebert U., Jensen L. F., et al. (2013). Possible causes of a harbour porpoise mass stranding in Danish waters in 2005. PloS One 8, e55553. doi: 10.1371/journal.pone.0055553
Wünschmann A., Siebert U., Frese K., Weiss R., Lockyer C., Heide-Jorgensen M. P., et al. (2001). Evidence of infectious diseases in harbour porpoises (Phocoena phocoena) hunted in the waters of Greenland and by-caught in the German north Sea and Baltic Sea. Vet. Rec. 148, 715–720. doi: 10.1136/vr.148.23.715
Keywords: Aspergillus, Baltic Sea, noise-induced hearing loss, inner ear, hair cell, linear skin markings (LSM)
Citation: Rohner S, Morell M, Wohlsein P, Stürznickel J, Reiter EB, Jahnke A, Prenger-Berninghoff E, Ewers C, Walther G, Striewe LC, Failla AV and Siebert U (2022) Fatal aspergillosis and evidence of unrelated hearing loss in a harbor porpoise (Phocoena phocoena) from the German Baltic Sea. Front. Mar. Sci. 9:958019. doi: 10.3389/fmars.2022.958019
Received: 31 May 2022; Accepted: 18 November 2022;
Published: 21 December 2022.
Edited by:
Alexander Supin, Russian Academy of Sciences (RAS), RussiaReviewed by:
Karina Vishnyakova, University of Padua, ItalyZhongchang Song, Xiamen University, China
Lonneke Liza IJsseldijk, Utrecht University, Netherlands
Copyright © 2022 Rohner, Morell, Wohlsein, Stürznickel, Reiter, Jahnke, Prenger-Berninghoff, Ewers, Walther, Striewe, Failla and Siebert. This is an open-access article distributed under the terms of the Creative Commons Attribution License (CC BY). The use, distribution or reproduction in other forums is permitted, provided the original author(s) and the copyright owner(s) are credited and that the original publication in this journal is cited, in accordance with accepted academic practice. No use, distribution or reproduction is permitted which does not comply with these terms.
*Correspondence: Maria Morell, maria.morell@tiho-hannover.de
†These authors have contributed equally to this work and share first authorship