Strengthening the health surveillance of marine mammals in the waters of metropolitan France by monitoring strandings
- 1Pelagis, Unité d’Appui et de Recherche (UAR) 3462 Centre National de Recherche Scientifique (CNRS)/La Rochelle Université, La Rochelle, France
- 2French Office for Biodiversity (OFB), Brest, France
- 3Department of Pathology, Veterinary College, University of Liege, Liège, Belgium
- 4Laboratoire public conseil, expertise et analyse en Bretagne (LABOCEA), Ploufragan, France
- 5Centre d’Etudes Biologiques de Chizé (CEBC), Unité Mixte de Recherche (UMR) 7372 La Rochelle Université/Centre National de la Recherche Scientifique (CNRS), Villiers-en-Bois, France
Monitoring the health status of marine mammals is a priority theme that France aims to develop with the other European Union Member States in the next two years, in the context of the Marine Strategy Framework Directive. With approximately 5,000 km of coastline and for nearly ten years, France has been recording an average of 2,000 strandings per year, which are monitored by the National Stranding Network, managed by Pelagis, the observatory for the conservation of marine mammals from La Rochelle University and the French National Center for Scientific Research. Since 1972, this network has successively evolved from spatial and temporal faunistic description to, nowadays, the detection of major causes of mortality. It now aims to carry out epidemiological studies on a population scale. Thus, a strategy to strengthen the monitoring of marine mammals’ health status based on stranding data has been developed. This strategy will allow for a more accurate detection of anthropogenic cause of death as well as those of natural origin. It will allow the monitoring of time trends and geographical differences of diseases associated with conservation and public health issues while ensuring the early detection of emerging and/or zoonotic diseases of importance. It will also allow a better assessment of the consequences of human activities on these animal populations and on the environment. Thus, this strategy is fully in line with the “One Health” approach which implies an integrated vision of public, animal and environmental health. It is broken down into four surveillance modalities: (1) general event-based surveillance (GES); (2) programmed surveillance (PS); (3) specific event-based surveillance (SES); (4) and in the longer term, syndromic surveillance (SyS). This article describes the French strategy as well as these different surveillance modalities, the levels of examinations and the associated sampling protocols and finally, the method of standardisation of the data collected. The objective is to present the strategy developed at the French level in order to integrate it into a future strategy shared at the European level to standardise practices and especially complementary analysis, necessary for a better evaluation of the health status of these mobile marine species.
1 Introduction
Epidemiological surveillance of wildlife consists of monitoring the health status and risk factors in these animal populations (Toma et al., 2010). Improved knowledge allows measures to be taken to face major issues such as the preservation of biodiversity, the conservation of vulnerable species, the maintenance of the economy associated with domestic animals and the protection of public health. It is thus recognised that wildlife epidemiosurveillance needs to be better integrated into the One Health approach (Karesh and Cook, 2005; Buttke and Wild, 2014; Cunningham et al., 2017; Sleeman et al., 2017), which involves an integrated view of public, animal and environmental health. Many species of terrestrial wildlife are indeed responsible for zoonosis. For example, Eurasian badgers (Meles meles) in the United Kingdom and Ireland and wild boars (Sus scrofa) in Spain, are reservoirs of Mycobacterium bovis, which are involved in the transmission of Bovine tuberculosis to cattle (Réveillaud et al., 2018). Another example of pathogen’s transmission between wildlife and domestical animals is the canine distemper virus in the lion (Panthera leo) population of the Serengeti National Park in Tanzania. This virus is similar to the one circulating in domestic dogs (Canis lupus familiaris) and the contacts between these two species make it possible to transmit the virus between them (Cleaveland et al., 2000; Viana et al., 2015). In addition, terrestrial wildlife is very present in the cities and could also be responsible for the transmission of zoonosis. Rodents such as the Norway rat (Rattus norvegicus) are known to be intermediate hosts of alveolar echinococcosis and since 1996, the red fox (Vulpes vulpes) has been described as one of the definitive hosts of this parasite. These species present in our cities are therefore likely to transmit this parasitosis to humans through their faeces (Bresson-Hadni et al., 2004; Vuitton et al., 2010).
Unlike terrestrial wildlife, the interactions between the health of marine mammals and human or domestic animal health may seem less obvious, given the more limited contact with marine mammals (Crespo and Hall, 2001). Moreover, difficulties are met to conduct the monitoring on marine wildlife, as access to these animals is difficult because of their habitat and their behavior (i.e., cryptic species, highly mobile species, diving behavior). Nevertheless, marine mammals should be considered as sentinel species not only for the health of the oceans but also of humans’ health (Bossart, 2011). Thus, the monitoring of strandings represents a valuable source of data to inform conservation management (IJsseldijk et al., 2020b). In addition to the spatiotemporal mortality trends and the cause of death, necropsies and complementary analysis carried out on stranded animals allows to understand the circulation of pathogens (bacteria, viruses, parasites and fungi) in these animal populations (e.g. Stokholm et al., 2021) which can constitute a conservation or public health issue (Ossiboff et al., 2021). In addition to detecting pathogens that would cause the death of the animal, it is possible to identify pathogens carried by an animal without being responsible for the death, or to detect antibodies, which would provide evidence that the targeted agent is circulating in the population (Bodewes et al., 2015; Measures and Fouchier, 2021). Laboratory analysis can also detect phenomena such as antibiotic resistance in isolated bacteria which is of public health interest (e.g. Gross et al., 2022). As with terrestrial wildlife, determining the causes of mortality and monitoring the health status of marine mammals ultimately makes it possible to identify the main threats to these animals and to respond to a public health issue, particularly with the early detection of zoonotic agents (Kuiken et al., 2005).
Therefore, many coastal countries in Europe and around the world have set up systems to record strandings and carry out post-mortem examinations of carcasses, thus taking advantage of these strandings to improve scientific knowledge (Perrin and Geraci, 2009). In Europe, some of these networks are effective for health surveillance and carry out necropsies with additional analysis for diagnostic purposes, such as in Germany (Institute of Wildlife and Marine Research of the University of Hanover), in Netherlands (Faculty of Veterinary Medecine, Department of Biomolecular Health Sciences, Division of Pathology, Utrecht University), in Great Britain (UK Cetacean Stranding Investigation Programme and ZSL Institute of Zoology), in Belgium (Faculty of Veterinary Medicine in Liège), in Italy (National Reference Center for Diagnostic Activities on Stranded Marine Mammals) and in Spain (University of Valencia, University of Las Palmas de Gran Canaria). These efforts have led to numerous publications that make it possible to assess the health status of populations at least on a regional scale (e.g. Prenger-Berninghoff et al., 2008; Arbelo et al., 2013; Mahfouz et al., 2014; van de Velde et al., 2016; Kershaw et al., 2017; Pintore et al., 2018; Coombs et al., 2019; Kapetanou et al., 2020; Numberger et al., 2021; Audino et al., 2022). Routine analysis are also carried out, but apart from a few main pathogens known in marine mammals, they often differ from one country to another (Guillerit, 2017). This is why standardisation of analysis between countries would allow the results obtained to be inferred at the population level of these widely distributed mobile species. This is all the more important as there are other considerations to be taken into account when conducting health monitoring of marine mammals. They are protected species covered by numerous regional and international directives and conventions. At the international level, these are mainly the Convention on the Conservation of Migratory Species of Wild Animals (CMS, Bonn Convention) and the Convention on the Conservation of European Wildlife and Natural Habitats (Bern Convention). In the North East Atlantic, the OSPAR (Oslo-Paris) convention and ASCOBANS (Agreement on the Conservation of Small Cetaceans of the Baltic, North East Atlantic, Irish and North Seas) both cover the conservation of marine mammals. These species are also regulated in French law through the decree of the 1st of July 2011 in application of the CITES Convention (Washington Convention) and Directive 92/43/EEC (European Directive known as the Habitats-Fauna-Flora Directive). Furthermore, at European level, the 2008/56/EC Marine Strategy Framework Directive (MSFD) published on the 25th of June 2008 and transposed in France in a series of regulations concerning the marine environment, requires knowledge of the health status of marine mammal populations to be improved.
The French National Stranding Network (NSN) is coordinated by the Pelagis observatory in La Rochelle (Support and Research Unit, UAR 3462 La Rochelle University - French National Center for Scientific Research), appointed by the Ministry of Ecology to monitor the status of marine mammal populations and support the implementation of public conservation policies relating to these species. The monitoring of strandings makes it possible to meet the requirements linked to the regulated status of these species. For example, among the descriptors defined by MSFD and used to define Good Ecological Status (GES), marine mammals are involved in descriptor D1 “Biodiversity” and descriptor D8 “Contaminants” with the following expectations: (1) monitoring of coastal cetacean populations, (2) monitoring of seal populations, (3) monitoring of marine mammals at sea, (4) monitoring of marine mammal strandings, (5) monitoring of interactions with human activities, (6) monitoring of chemical contaminants in cetaceans. Thus, the monitoring of strandings is an integral part of this monitoring.
The NSN was created in 1972 as a natural science network. Since its beginnings, the network has relied on the participation of volunteers (correspondents), but over time it has become partially professional, with some correspondents such as field agents of the French Biodiversity Agency (Office Français de la Biodiversité - OFB) for whom this activity is part of their missions. The network has evolved from a descriptive activity of stranded animals to the detection of the main causes of mortality through the examination and necropsy of some selected carcasses and the study of the ecology of species through various samples and data analysis. From this point of view, this network is particularly functional and generates approximatively twenty publications per year in the field of ecology (e.g. Spitz et al., 2014; Peltier et al., 2021; Chouvelon et al., 2022; Méndez-Fernandez et al., 2022; Rouby et al., 2022). Certain zoonotic pathogens (Brucella sp. and Erysipelothrix rhusiopathiae) and epizootic agents (morbillivirus and avian influenza virus) are only investigated if macroscopic lesions are suggestive of infection by these pathogens. In this global context, Pelagis has developed a strategy to better monitor the health of marine mammals based on stranded marine mammals. To this end, it is necessary to develop the network and strengthen the system for monitoring the health of marine mammals in order (1) to harmonise the methods used to diagnose and identify the causes of mortality with greater accuracy (2) to improve the understanding of the main threats to marine mammals, particularly emblematic or critical species (because of their rarity or the associated conservation issue) as well as strandings associated with phenomena of interest (e.g. unusual mortality events); (3) to improve the ability to analyse data through epidemiological models (4) to allow the acquisition of knowledge on the circulation of pathogens in these populations and their impact on animal and public health in case of zoonosis; (5) to allow the early and easy detection of peak mortality and other monitored factors; and finally, (6) to allow the early detection of major (re-)emerging, exotic or zoonotic diseases. Thus, this strategy falls within the field of eco-epidemiology while integrating the “One Health” approach.
The French stranding network provides a case study: the conceptual framework will be confronted with reality, considering the objectives, structure, functioning and tools of the NSN, which will need to evolve further to better meet the requirements of the strategy. The limits and needs will be identified as well as the long-term prospects. The objective is to present the strategy developed at the French level (metropolitan and overseas) in order to integrate it into a future strategy shared at the European level to standardise practices and especially complementary analysis, necessary for a better evaluation of the health status of these mobile marine species.
2 Preliminary considerations for strategy development
2.1 Functioning of the NSN
The NSN is structured in a similar way to an epidemiological surveillance network, with the different levels of organisation being field, local, regional and central (Dufour and Hendrikx, 2011) (Figure 1).
The national governance of the NSN is ensured by a Steering Committee (COPIL) made up of appointed members from different institutions (Pelagis, OFB, surveillance network for infectious diseases of birds and wild terrestrial mammals (SAGIR), National Museum of Natural History (NMNH), ministry in charge of Ecology), divers non-profit associations, members elected from among the NSN correspondents and invited experts. With the exception of the French Overseas Territories, coordination is carried out solely at national and not regional level, but depending on the region, data are sometimes centralised by a local representative correspondent. Technical and scientific support is provided by Pelagis. The network’s coordination unit, known as the “stranding team”, is located within Pelagis. To date, only two laboratories have signed agreements at regional level (LABOCEA in Ploufragan and Department of Morphology and Pathology of the Faculty of Veterinary Medicine in Liège).
Carcass data are collected in the field according to standard protocols by authorised NSN correspondents or in NSN associated laboratories. These authorisations (called “green card”) are allocated by the ministry in charge of Ecology, subsequent to the training delivered by Pelagis. The application of the various protocols (biometrics, standardised photographs, external examination, internal examination, necropsy and sampling) will depend on the state of decomposition of the animal, the level of training of the correspondent and the logistical means available (transport and storage of the carcass, storage of samples, etc.) (Table 1). The DCC, “Decomposition Code Categories”, is the indicator of the state of decomposition of the carcass used, which allows a score to be assigned from 1 to 5, from the freshest to the most putrefied respectively (Kuiken and García Hartmann, 1991; Jauniaux et al., 2002; Van Canneyt et al., 2015).
All the information is finally centralised by the stranding team and integrated into a database. The data, once standardised, are made available for studies by external entities. A tissue bank is also set up and available for research projects. Each year, approximatively thirty agreements for the use of biological samples are drawn up with external organisations. The data analysis and the studies’ results carried out by the observatory itself give rise to scientific publications, the annual stranding report, publications on the website and are also presented at an annual seminar which brings together the network’s correspondents and stakeholders.
Finally, the annual operating budget of the network managed by Pelagis can be divided into four main components: scientific and administrative coordination, human resources, interventions and sample analysis. The ministry in charge of ecological transition, as well as the supervisory institutions of Pelagis, which are the French National Center for Scientific Research and La Rochelle University, funds the coordination of the NSN. The stranding team and the interventions are mainly supported by the OFB and La Rochelle city. Depending on the year, the budget allocated to sample analysis varies and is supported by the OFB, the ministry and regional, national or European projects. The budget managed by Pelagis for stranding activities is estimated between €400,000 and €600,000 per year, depending on the projects integrating sample and data analysis. Finally, a large part of the NSN’s operation is ensured by the self-financing of the network’s stakeholders, which can be broken down as follows: valuation of the correspondents’ voluntary work, participation of non-profit organizations making use of their financial resources, provision of OFB agents, technical services of municipalities, prefectures and other structures, provision of premises or equipment by these same structures.
2.2 NSN’s stranding valuation tools: examinations and samples
There are different tools for valuing strandings that correspond to the levels of examination and sampling protocols carried out on the carcasses. Thus, depending on the operator and his training, four levels of examination and three standardised sampling protocols can be carried out (Table 1). Although it would be desirable to carry out the highest level of examination and sampling protocol on each carcass found, the very large number of annual strandings on the French coasts does not allow this at present, for logistical and financial reasons. The levels of examination and associations with sampling protocols have been revised and improved during 2022 to better meet the objectives pursued in the framework of the reinforcement of health surveillance.
The first examination level (L1) is an external examination associated to six standardised photographs (Figure 2). It is carried out on the majority of stranded animals and may provide initial information on the cause of death. From now on, the training of the correspondents implies that they are also able to describe precisely the external lesions observed with standard description criteria (location, shape, contours, distribution, size and extension, number, consistency and texture) while completing their examination with photos of the lesions described. Sections are systematically taken at the level of the observed alterations in order to examine and describe the underlying tissues (Figure 3). This makes it possible to determine whether these alterations occurred during the animal’s lifetime or after its death and facilitates interpretation.
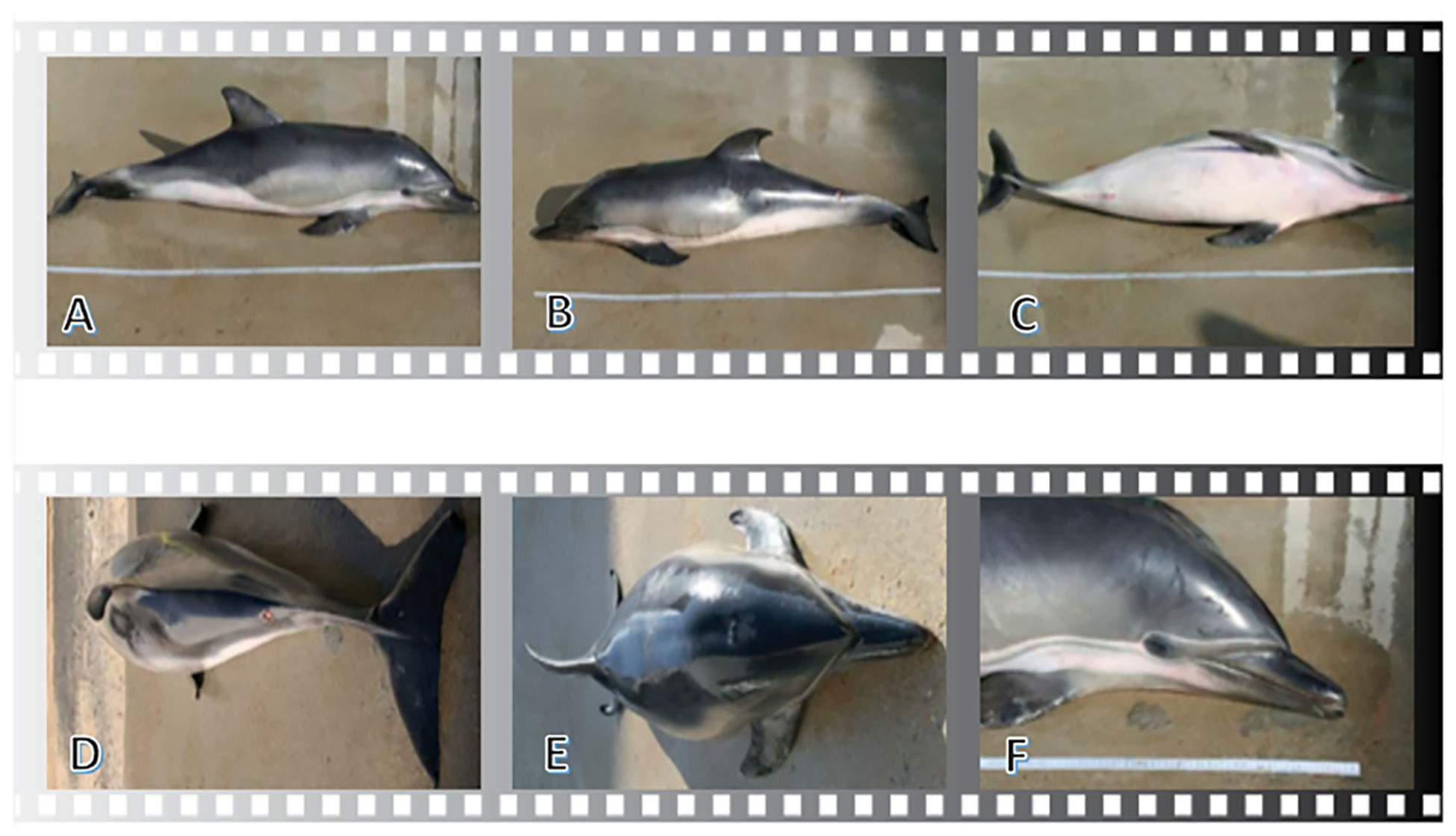
Figure 2 The six standardised photos, © PELAGIS. (A) Lateral right view; (B) Dorsal view; (C) Ventral view; (D) Rear view; (E) Front view; (F) Head focus.
The second level of examination (L2) used to consist of performing the L1 and opening cavities in a second stage, in order to perform the samples of the P2 protocol, intended mainly for ecological analysis. This level has been significantly modified and from now on correspondents will be trained to describe some main organs (descriptive criteria: volume, shape, colour, consistency, etc.). Thus, they must briefly describe the appearance of the lungs, the contents of the stomach, the liver, the spleen and finally, they must check the topography of all the thoracic and abdominal organs. These different elements are all photographed with a measurement and colour scale (Figure 4). These organs were chosen because they allow for a more accurate diagnosis of mortality linked to bycatch in fishing gear. Indeed, animals that die from bycatch usually show good body condition, large amounts of fresh or partially digested food remains in the stomach, congestive liver and spleen, lung edema, congestion and/or emphysema and finally, normal topography of the internal organs (Kuiken, 1996; de Quirós et al., 2018; Epple et al., 2020; IJsseldijk et al., 2021). This also helps to rule out other major pathology that would be macroscopically visible on the targeted organs.
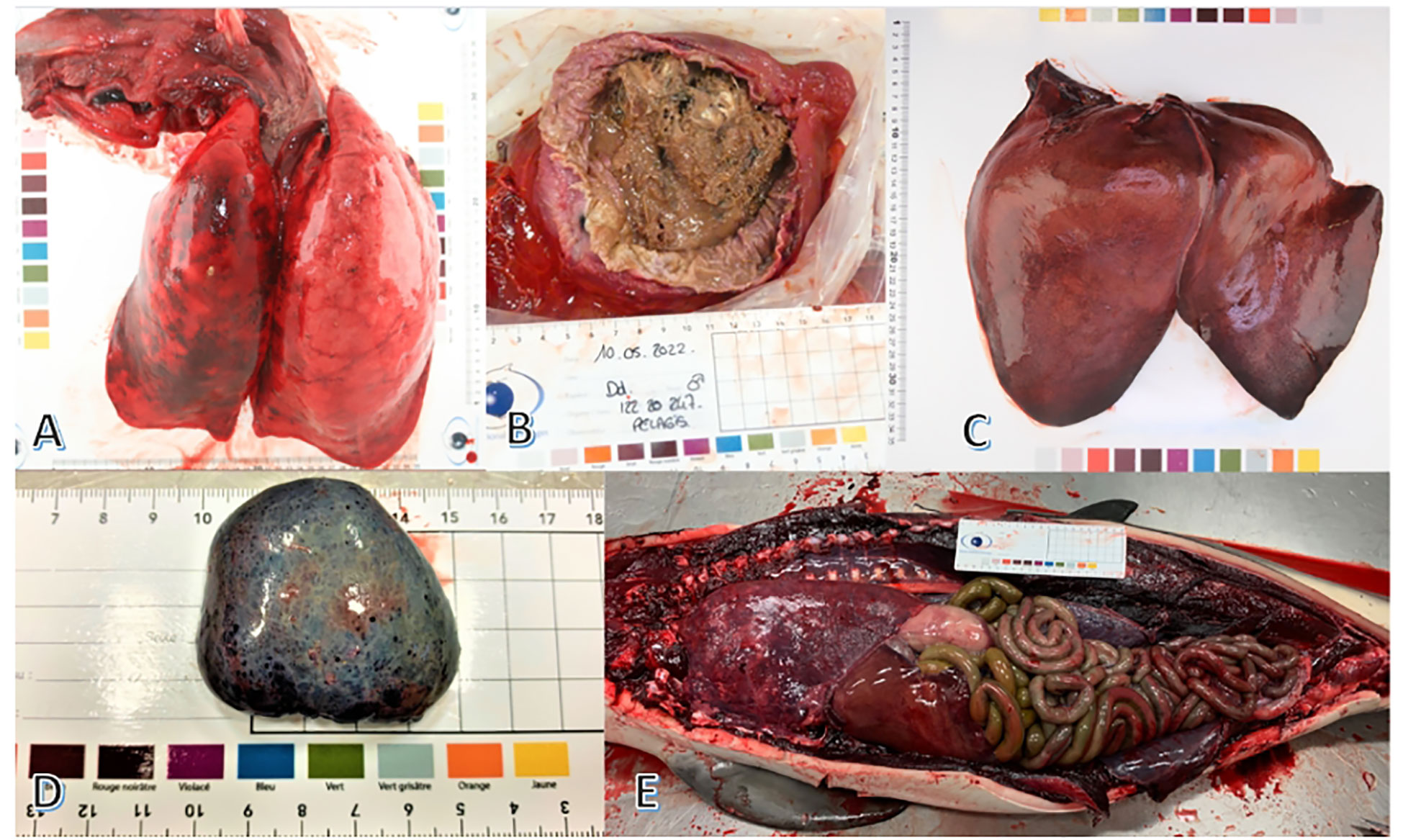
Figure 4 Organs described and photographed at L2 in a cetacean, (A) lungs, (B) gastric contents, (C) liver, (D) spleen, (E) thoracic and abdomen topography, © PELAGIS.
The third examination level (L3) is divided into two phases. The first step consists in the production of a dissection report by a trained NSN’s correspondent (biologist or veterinarian), which includes the external examination and a detailed description of all internal organs, supported by photographs. The second phase is the interpretation and validation of this report by an experienced veterinarian who can then conclude, when possible, on the cause of death. This level has been developed by Pelagis in 2019 as part of a veterinary thesis (Laporte et al., 2021) in a context of judicialization of the problem of bycatch in fishing gear. As the systematic intervention of an experienced veterinarian on all the carcasses of bycaught animals is difficult to consider, it was a priority to find alternative solutions to respond more accurately for the diagnosis of mortality linked to bycatch. This level involves to train operators in the detailed macroscopic description of all the organs and in taking photographs in a standardised way in order to produce an interpretable report. The operators trained to the L3 are non-veterinarian marine biologists, specialized in the monitoring of stranded marine mammals, and volunteered veterinarians with less experience. The inclusion of veterinarians in the L3 allows for systematic cross-checking of their interpretations and conclusions by more experienced veterinarians, which contributes to the harmonization of diagnosis.
The fourth examination level (L4) corresponds to a necropsy performed by an experienced veterinarian with consolidated knowledge of marine mammal pathology and who has performed numerous necropsies on these species. The necropsy is performed according to an adapted standard protocol (IJsseldijk et al., 2020a). L4 is the level with the best diagnostic value and generally allows the determination of the cause of death.
In addition to the post-mortem examinations carried out, there are different sampling protocols for various analysis that can be carried out depending on the level of examination conducted (Table 1). The P1 sampling protocol consists of taking the teeth to determine the age of the animal. This sampling protocol may be carried out on all animals regardless the DCC. The P2 sampling protocol consists of taking different samples in addition to P1 in order to acquire biological, ecological, demographic and contaminant data. It can be carried out on animals of up to DCC 3. A new standard sampling protocol recently defined, the P3, which is added to P1 and P2 protocols, is intended for epidemiological surveillance and can only be carried out on animals of DCC 1 or 2. It was defined according to several criteria: improvement of knowledge, conservation and public health interests, financial cost, technical and logistical feasibility. The number of organs taken for standard formalin samples for histopathological analysis is 23 (or more if the animal is pregnant). Of these, 11 only are analysed as part of research projects in order to limit the financial cost. For example, the thyroid and adrenal glands are systematically sampled but not analysed, but could be analysed at a later time in the context of projects looking at the impact of contaminants on the endocrine system. The organs whose samples are systematically analysed are some main vital organs (heart, brain, liver, spleen, kidneys, pancreas, intestine and lungs) and the lymph nodes (with the exception of the marginal lymph node in cetaceans, which is taken in addition to the tracheobronchial nodes for the respiratory system and which is only analysed on a project basis or at the specific request of the veterinarian). Moreover, six standard refrigerated samples are taken for bacteriological analysis (spleen, liver, heart, lungs, kidneys and brain). The samples are inoculated on various culture media in different atmospheres to test for aerobic and anaerobic bacteria. Specific culture media may be used when the veterinarian suspects the presence of bacteria requiring it, such as Salmonella spp. or Erysipelothrix rhusiopathiae. Antibiotic susceptibility testing are performed on bacteria on the list of antibiotic-resistant “priority pathogens” (Tacconelli and Magrini, 2017; Tacconelli et al., 2018). Standard samples are frozen for routine molecular analysis. Ten organs are systematically sampled (lymph nodes, spleen, intestinal segment, liver, kidney, lung and brain) to test for morbilliviruses, influenza viruses, herpesviruses, Brucella sp. and finally mycotic agents by (RT-)PCR. If the signal is positive, sequencing is performed to confirm the signal and determine the pathogen involved. Faeces and urine samples are also frozen for future test for algal biotoxins. Samples of parasites found are systematically preserved in ethanol for identification. Finally, upon receipt of the histology results, additional tests for pathogens other than those routinely sought may be requested by the veterinarian (PCR, immunohistochemistry, etc.).
In addition to the standardised protocols, P1, P2 and P3, other samples for diagnostic purposes (P0) may be taken at the request of Pelagis or by the veterinarian in charge of the necropsy, who will then determine the analysis to be performed.
2.3 NSN work focus: strandings and causes of mortality
Reported stranding numbers are the result of four parameters: species abundance at sea, mortality, drift conditions and reporting. The first two are biological variables, while the next two depend on weather conditions and carcass buoyancy, and the last one on the probability of sighting and the observer’s ability or willingness to report the stranding (Van Canneyt et al., 2015). To date, the reporting rate in metropolitan France is very high (events are regularly reported several times by different people) and it is considered that almost all strandings are detected (Authier et al., 2014).
The first factors recorded are the specie and sex identification, the biometric data and spatial and temporal distributions of the stranded marine mammals, allowing to follow the trends of the stranding rates and to highlight unusual mortality events. Indeed, changes in these parameters can be indicative of modifications in abundance, mortality, distribution or of pressures affecting marine mammal populations. Diversity within the marine mammal community can also be assessed (Chan et al., 2017; Dars et al., 2021). The external examination of carcasses allows in some cases to suspect the causes of mortality. This is the case, for example, of an animal showing morphological alterations caused by interactions with fishing gear (net imprints, linear lesions encircling the rostrum, mandibular fractures, etc.) or even amputations of appendages or perforations carried out during untangling operations (Figures 5, 6).
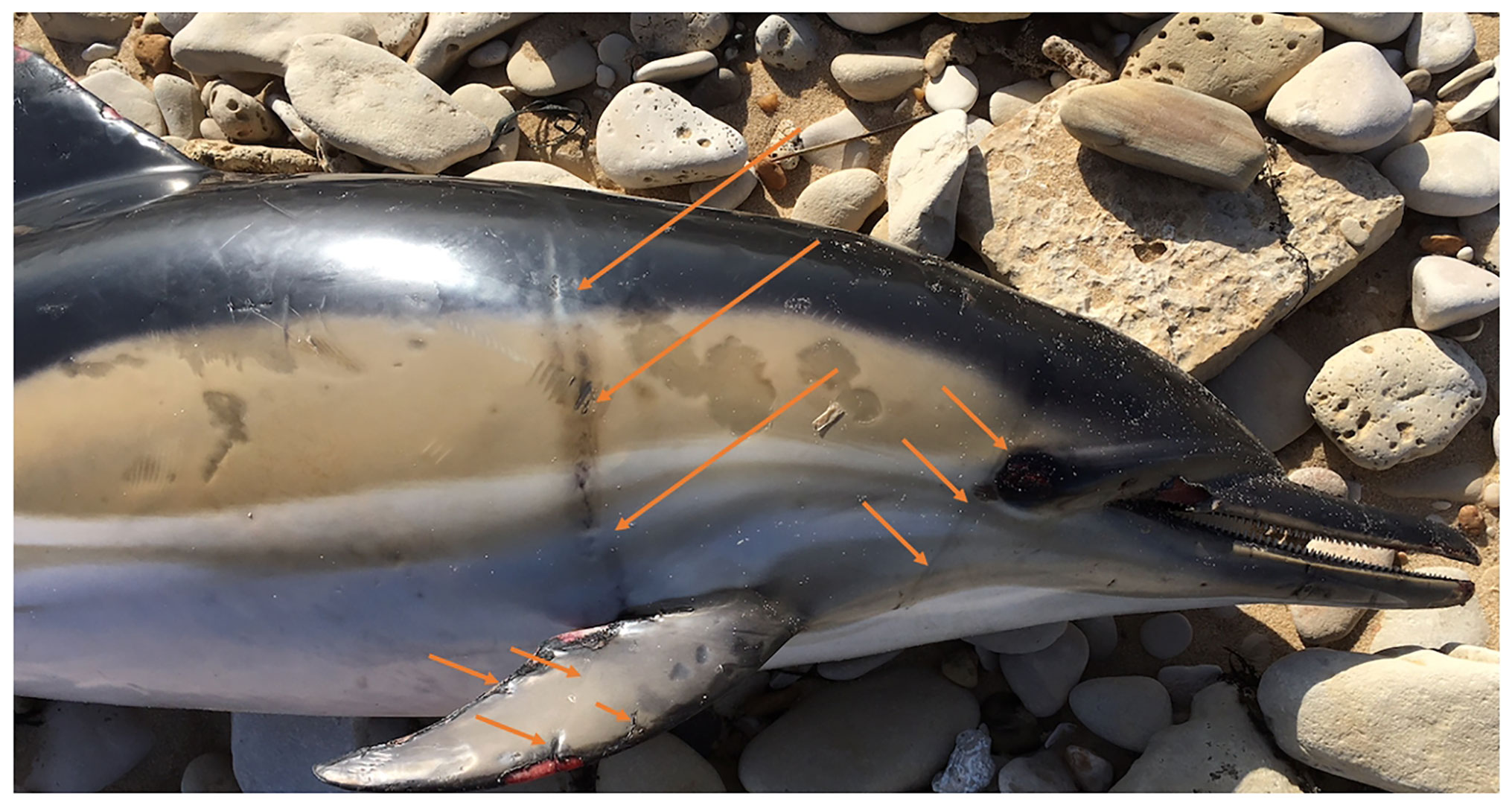
Figure 5 Morphological alterations caused by interactions with fishing gear (net imprints) – Common dolphin, February 2019, Rivedoux (17), © PELAGIS.
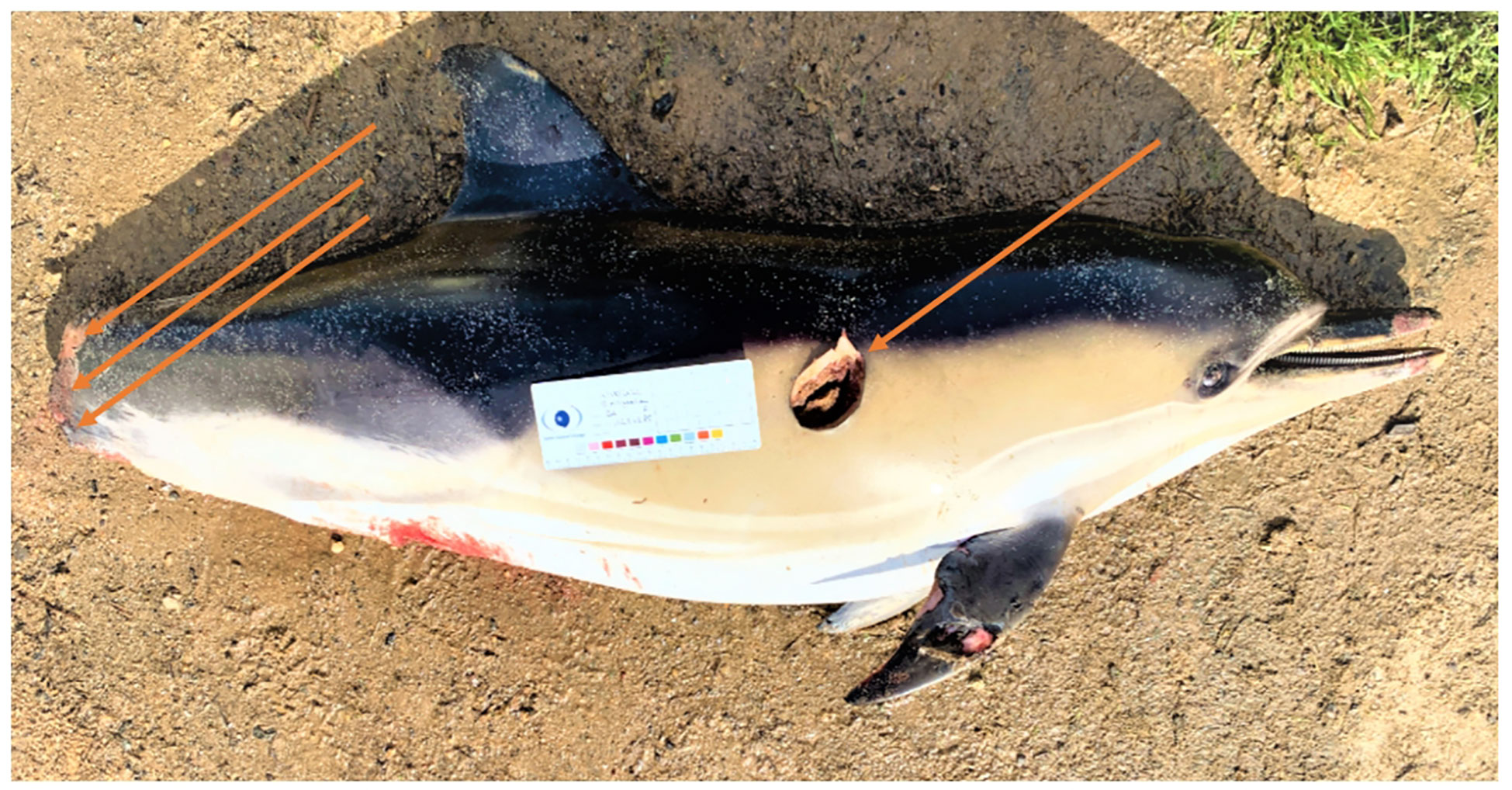
Figure 6 Amputations of appendages and perforations carried out during stripping operations – Common dolphin, March 2022, Bretignolles-Sur-Mer (85), © PELAGIS.
Nevertheless, only a necropsy (post-mortem examination by a trained veterinarian) accompanied by additional analysis (e.g. histology, microbiology, virology, parasitology) can lead to a definitive veterinary diagnosis. Necropsy protocols recommand the best practices for cetacean post mortem investigation and tissue sampling for additional analysis (Jauniaux et al., 2019; IJsseldijk et al., 2020a). This allows the cause of death to be determined with higher degrees of certainty than an external examination alone.
According to the stranding database and the annual reports produced by Pelagis, more than 2,000 strandings have taken place each year since 2016. More than 60% of strandings have occurred on the Atlantic coast, around 25% on the Channel and North Sea coast and less than 5% on the Mediterranean coast. More than thirty species of marine mammals have already stranded in metropolitan France. However, the vast majority of strandings (percentage 2016-2021) are represented by only eight species: common dolphin (Delphinus delphis, 57%), striped dolphin (Stenella coeruleoalba, 6%), bottlenose dolphin (Tursiops truncatus, 3%), harbour porpoise (Phocoena phocoena, 17%), long-finned pilot whale (Globicephala melas, 1%), Risso’s dolphin (Grampus griseus, <0,5%), grey seal (Halichoerus grypus, 9%) and harbour seal (Phoca vitulina, 6%). Nevertheless, for the last five years, due to the increase of the numbers of common dolphin strandings, the relative proportion of the long-finned pilot whale and Risso’s dolphin represented less than 0.5% of annual strandings each (Van Canneyt et al., 2015). In 2021, 2046 strandings were reported to the NSN. Of these reports, nearly 83% of the carcasses were examined by a NSN correspondent, while less than 5% were necropsied, demonstrating the need to increase the number of necropsies (Dars et al., 2021).
The causes of mortality identified through post-mortem examinations of stranded marine mammals are numerous. Among them, are the traumatic causes. One cause widely encountered in small cetaceans is bycatch in fishing gear (Read and Murray, 2000; Jauniaux et al., 2002; Peltier et al., 2021). In 2021, it was the main cause of mortality for common dolphins (87% of the external examinations) and harbour porpoises (52%) in France. Bycatch mortality has also been reported for bottlenose dolphins (16%), striped dolphins (13%) and, to a lesser extent, grey (12%) and harbour seals (8%) (Dars et al., 2021). Collisions with ships are a frequent cause of mortality, especially for large cetaceans. In the Mediterranean Sea, where maritime traffic is intense, more than 50 collisions were reported between 1972 and 2017, representing the main cause of mortality identified in large cetaceans (Peltier et al., 2019). Some deaths are also attributed to entanglement with debris or ingestion of macroplastics (Simmonds, 2012; Moore and Barco, 2013) with the recent example of a fatal entanglement of a young harbour seal in Normandy in 2021 (Dars et al., 2021). It is also possible to observe fatal injuries inflicted by other individuals of the same or other species associated with competitive or predatory behaviour. For example, bottlenose dolphins appear to be particularly aggressive with each other and with other small cetacean species (Ross and Wilson, 1996; Dunn et al., 2002; Nery and Simão, 2009; Gross et al., 2020). Thereby five cases of striped dolphins stranded in the Mediterranean in 2021 showed lesions corroborating this hypothesis (Dars et al., 2021).
Among the causes of natural death are those of infectious origin. In recent decades, numerous pathogens have been isolated from marine mammals worldwide (Gulland et al., 2018). Among the causes of natural death are also those of non-infectious origin such as starvation (which is very often encountered in young animals separated from their mothers), congenital anomalies (Herr et al., 2020; Morell et al., 2022), neoplasms (Newman and Smith, 2006) or dystocia. In 2021, depending on the type of examination carried out on stranded individuals in France (full or external examination only), natural death resulting from a pathological condition concerned between 18% and 34% of cases in cetaceans and between 6% and 25% in seals (Dars et al., 2021). Pathological condition was considered the cause of death when the animals showed advanced emaciation or major macroscopic lesions on postmortem examination, after eliminating lesions suggestive of traumatic death. External examinations of these animals revealed mainly inflammatory skin lesions scattered over the body and internal examinations revealed that the respiratory system was most often affected (bacterial and/or parasitic pneumonia).
Finally, in recent years, live strandings of cetaceans have represented around 5% of strandings in metropolitan France (Van Canneyt et al., 2015). For live stranded cetaceans, the priority action, whatever the origin, is refloating. Refloating animals in good body condition is often successful. For seals, when their survival depends on it, they are firstly taken care of by a rehabilitation center (mainly first-year individuals, recently weaned or unweaned and separated from the mother).
In 2022, the French news highlighted several cases of out of habitat marine mammals, notably a killer whale (Orcinus orca) observed in the Seine River in May followed by a beluga whale (Delphinapterus leucas) in August. These cases of out-of-habitats animals often remain unexplained, and there may be multiple reasons for them, such as pathological condition, age (sub-adults disperse more easily), social isolation or environmental conditions (Hennessy et al., 2001; Pryce et al., 2002; Stephens et al., 2005; Clutton-Brock and Huchard, 2013; Vetulani, 2013; Massen et al., 2015; Thompson et al., 2017).
3 Strategy developed and data standardization
3.1 Strategy developed: four health surveillance modalities
In order to meet the objectives of Pelagis and to optimise the use of data obtained from strandings, the strengthening of health monitoring will be based on four methods (Table 2), which may occasionally be supplemented by ad hoc surveys. These four methods are: (1) general event-based surveillance (GES), which will apply to all stranded individuals; (2) programmed surveillance (PS), also called active surveillance and defined by the World Health Organization as the collection of case study information as a continuous pre-organized process (World Health Organization, 2002) – the methodology must allow the results found to be inferred to the monitored population and will apply to a sample of 100 individuals representative of the stranded marine mammal population; (3) specific event-based surveillance (SES), which will apply to situations requiring in-depth investigations; and finally, (4) syndromic surveillance (SyS), which will also apply to all stranded individuals.
3.1.1 General event-based surveillance
General event-based surveillance is the monitoring modality that will focus on stranding as an event. It will be based on spontaneous reporting of strandings by the general public. This monitoring has been carried out by the NSN for nearly 40 years and must be maintained as part of the health monitoring strategy developed, as it may help to identify the causes of mortality. The main parameter measured by this modality will be the spatio-temporal distribution of strandings. For example, using reverse drift models, the presumed area of mortality at sea can be defined, which will make it possible to identify possible threats to these animals in this area and thus obtain hypotheses on the cause of death (Peltier et al., 2020). Continuous monitoring of strandings will help to ensure epidemiological vigilance by highlighting unusual mortality events and sometimes identifying the cause, as well as allowing the detection of anomalies on the carcasses. Epidemiological vigilance will be of priority interest as it will ensure the early detection of emerging and/or zoonotic diseases of major importance. In addition, whenever possible, a carcass examination should be carried out by a NSN correspondent, favouring the highest level of examination. Thus, GES is a surveillance modality that will apply to all reported strandings, regardless of the level of examination and sampling protocol carried out.
3.1.2 Programmed surveillance
Programmed surveillance (PS) is a surveillance modality that will involve individuals being subjected to necropsy (L4) and to the P3 sampling protocol which is specific to this modality.
Logistical and financial constraints limit the application of the PS to 100 fresh carcasses per year (DCC 1 or DCC 2 without freezing). Therefore, a sampling plan is proposed to represent the stranded marine mammal population. This plan describes the number of animals expected for each species, by seaboard and by quarter, proportionally reduced for a total of 100 individuals per year based on the last five years of consolidated data, for which all the data are available (from 2016 to 2020 for the 2023 plan). Any stranded animal that falls within this sampling will have to be handled by the PS modality until the quotas are reached. If the expected number of animals for a species, seaboard and quarter is not reached, the animals will be analysed/investigated in the next quarter.
Systematic necropsies accompanied by standardised analysis will allow descriptive epidemiology to be carried out by revealing the circulation of pathogens in these animals and measuring it in time and space. The prevalence of some agents (morbillivirus, Brucella sp., avian influenza, herpesvirus and mycotic agents) in the species of marine mammals most frequently stranded in metropolitan France will be calculated. The same will be done for the prevalence of bacterias identified from the culture media used routinely, as well as for parasites systematically sampled. In addition to infer the results obtained to a reference population, the additional diagnostic analysis included in P3 will make possible to investigate the etiology of the various lesions encountered and thus to better understand their impact on the health of individuals and therefore of populations.
Moreover, obtaining complete lesion tables and numerous laboratory results makes it possible to carry out analytical epidemiology, in particular by conducting case-control studies to measure the effect of exposure to factors on the incidence of diseases or other events. Through this type of study already conducted abroad, it has been possible for example to demonstrate the existence of a positive correlation between exposure to polychlorinated biphenyls (PCBs) and the incidence of infectious diseases in harbour porpoises in Great Britain (Hall et al., 2006). Such studies could easily be carried out given the important work carried out for several years by Pelagis on contaminants and essential trace elements (Cariou et al., 2021; Chouvelon et al., 2022; Méndez-Fernandez et al., 2022). Finally, systematic sampling allows samples to be made available for future research programmes.
3.1.3 Specific event-based surveillance
Specific event-based surveillance is a monitoring modality in which some stranding events will be investigated in depth to meet conservation, public health or knowledge acquisition objectives. These events are called “cases”. They will be revised each year or more regularly depending on the epidemiological context. This surveillance method will be based on spontaneous reporting of cases at the time of reporting by the general public or by the network’s correspondents when they intervene on the stranding field. The SES has thus been established to allow for enhanced monitoring of the following three main categories of cases, each with specific objectives: (1) enhanced monitoring of the health status of critical species and emblematic species for knowledge or conservation purposes; (2) enhanced monitoring of phenomena of interest for knowledge, conservation and public health purposes; (3) enhanced monitoring of diseases of interest for conservation and public health issues and to guarantee early warning (epidemiological vigilance).
All species that have never or rarely been observed stranded on a coastline and for which in-depth investigations are required are considered critical. Emblematic species are the animals already included in the strategy for monitoring contaminants in cetaceans on the French coast established by Pelagis within the framework of the MSFD (Méndez-Fernandez et al., 2019).
Phenomena of particular interest are considered to be situations corresponding to unusual mortality events and/or revealing the consequences of anthropic activities on marine mammal populations. The phenomena selected are: (1) mass strandings, (2) multiple unexplained strandings, (3) strandings suspected of being associated with noise pollution, (4) strandings suspected of being associated with acute chemical pollution, (5) lesions suspected of being of intentional anthropogenic origin and, finally, (6) strandings suspected of being related with marine biotoxins.
For diseases of priority interest, it was agreed to focus on those with known conservation or public health issues that have already had an impact in regional waters, such as avian influenza and morbilliviruses.
Each case is defined according to criteria such as the species concerned, the geographical area, the time window or the warning signs. The definition of cases must be sensitive enough to guarantee detection but also specific enough to avoid having to treat too many animals, which would not be logistically and financially feasible. For each of these cases, a procedure is established. It defines the level of examination to be carried out and a specific sampling protocol (P0) which is different for each case. Technical notes including the definition of the case, the interest of reinforcing surveillance and the procedure to follow will be made available to all the network’s correspondents.
3.1.4 Syndromic surveillance
Syndromic surveillance will consist of the continuous collection, analysis and interpretation of health indicators to ensure a rapid assessment of the impact of health risks on the health of the population monitored. The continuous signal will allow the production of a time series, i.e. curves, and the implementation of algorithms that detect unusual peaks in the indicators monitored, that have to be preliminary defined. This modality will achieve three objectives: (1) the anticipated detection of expected phenomena, such as an increase in bycatch mortality during the winter season or an increase in strandings in an area where a source of noise pollution is identified (e.g. during military exercises at sea); (2) the detection of unexpected phenomena, such as increased strandings at a time and in an area where this has never been described before; and finally, (3) the assessment of the impact (or lack thereof) of a phenomenon, such as the correlation between increased strandings in an area and the building of an offshore wind farm.
Only the “mortality” indicator will be systematically surveyed at short term. This will make it easier to detect mortality peaks or mortality events associated with identified phenomena. In the longer term, other indicators could be monitored continuously, such as the presence of pathogens or specific lesions as neoplasms. SyS will be carried out on all reported stranded animals. However, it will not always be possible to obtain data on all indicators for each animal as this will depend on the level of examination and analysis carried out. This monitoring modality will require a consolidated database with rapid and systematic integration of the indicators monitored and standardisation of the lexicon used to describe them. It will also require the development of adequate algorithms and a good interpretation of the results obtained.
3.2 Data standardisation
Large-scale standardised data integration has been developed to facilitate data analysis and comparison between the different information obtained from strandings. This standardisation will also facilitate the exchange of data with stranding networks in neighbouring countries. Thus, a major effort has been made to harmonise data on causes of death, lesions identified during necropsies and the results of additional analysis.
3.2.1 Standardisation of individual cause-of-death data
Individual cause of death data should be systematically included as follows: (1) initial cause of death (mandatory); (2) immediate cause of death (optional); (3) degree of certainty associated with the diagnosis of the initial cause of death (mandatory); (4) contributory causes (optional); (5) anthropogenic origin of the initial cause of death (mandatory). In addition, a commentary may accompany these data to provide details of the cause of death such as a pathogen involved.
The initial cause of death must be completed with one of the death cause categories defined (Table 3). These categories and subcategories of causes of death have been defined, similarly as it is usually done in human forensic medicine, while trying to maintain continuity with what was done previously in the network. When two or more causes of death are recorded, the veterinarian (or the biologist performing the examination) will be asked to indicate the initial cause and the immediate cause of death. For example, an animal may have suffered a predatory bite that resulted in fatal septicemia. In this case, the initial cause is indeed the bite while the immediate cause is the septicemia. The veterinarian may also indicate conditions not directly related to the cause of death but which contributed to it (contributing causes). The initial cause of death will be used for statistical analysis at the population level. Each initial cause will come with a degree of certainty on a scale of 1 to 5. The scale is the same as the one used in the Epifaune database, developed by the OFB in partnership with ADILVA (French association of directors and managers of public veterinary analysis laboratories) and Faunapath (an anatomo-pathology laboratory specialising in non-captive wildlife in Lyon). In order to facilitate the standardisation of degrees of certainty between the different levels of examination (generally stronger when the level of examination is higher), adapted definitions have been developed (Table 4). Guidelines specifying the elements that should lead to the attribution of the different degrees of certainty will be presented during the training sessions delivered to the correspondents in order to limit the operators biases.
Finally, it will be stated whether the initial cause of death has an anthropogenic origin or not. A commentary may be added to clarify the cause of death. For example, in the case of a natural death of infectious origin, it could be stated that it was a pneumonia compatible with a bacterial origin according to the macroscopic lesions.
3.2.2 Standardisation of necropsy data and additional analysis results
In order to facilitate analysis of the data obtained during necropsies on a population scale, the terms used to define organs and lesions, as well as the results of the complementary analysis, were standardised.
Thus, the list of organs is broken down into three levels as done in the SAGIR vademecum (Gauthier et al., 2016): matrix category, generic matrix and specific matrix. Among the matrix categories are the major categories or systems examined during marine mammal necropsies, which are as follows: whole cadaver, fetal appendages, cardiovascular system, digestive system, haemato-lymphopoietic system, respiratory system, urogenital system, oral cavity, cavities/serous membranes, muscles and tendons (active locomotor system), sense organ, skeleton (passive locomotor system), endocrine system, nervous system, integumentary system, and finally, adipose connective tissue. The generic and specific matrices make it possible to specify the organ and its possible location. For example, for the cardiovascular system it could be the heart and the myocardium for the generic and specific matrices respectively.
Similarly, lesions detected on macroscopic examination and histological analysis are broken down into three levels which are analyte category, analyte and sub-analyte. The analyte categories are: morphological abnormality, content, inflammatory, mechanical and traumatic, metabolic, proliferative and finally vascular. The analyte and sub-analyte help to specify the nature of the lesion. For example, for a vascular lesion it could be hemorrhage and petechiae for the analyte and sub-analyte respectively. The severity of the injury will be specified (mild to severe) as well as the duration of the condition (acute to chronic). Measurements may be added and the time of onset specified (ante or post mortem). Lesions may have been diagnosed by macroscopic examination or by histological analysis. Thus, the method of diagnosis will be specified.
Finally, all data from additional analysis (excluding histology) will also be integrated in a standardised way. The nature of the analysis, the method used, the presence of a positive or negative signal will be specified. The result will be broken down into three levels which are again the analyte category, the analyte and the sub-analyte. The analyte categories are numerous and include for example bacteria, viruses, parasites, fungi, toxins or contaminants. The analyte and sub-analyte will help to specify the agent of interest. In the case of biological agents, these are the genus and species respectively. Finally, the veterinarian should specify whether the agent in question is considered to have caused disorders in the animal’s lifetime.
4 Discussion
The health monitoring of marine mammals as presented here is an ambitious project requiring as many stranding data as possible. Pelagis’ first ambition was to improve its means of expertise in order to conclude with more precision and certainty on the causes of mortality. The development of these means was the opportunity to reinforce the global health monitoring of marine mammal populations. This strategy will allow to improve the knowledge of the health status of these species subjected to numerous pressures by following the evolution in time and space of diseases associated with conservation and public health issues while ensuring the early detection of emerging and/or zoonotic diseases of importance. It will also allow a better assessment of the consequences of anthropic activities on these animal populations and on the environment.
The high number of strandings in metropolitan France, combined with logistical, financial and human resources, imply that the highest level of examinations cannot be implemented on every stranded marine mammal. Thus, a strategy with different surveillance modalities has been established, allowing us to choose the individuals on which the most in-depth investigations will be carried out, while representing at best the stranded marine mammal population. The strategy, based on four surveillance modalities which are general event-based surveillance, programmed surveillance, specific event-based surveillance and syndromic surveillance should imply many evolutions. Among these, the main ones are the dissemination of expertise means and their appropriation by the network actors, the ability to meet the sampling plan and the appropriation of the P3 sampling protocol within the framework of the programmed surveillance and the integration of standardised data (standardized samples and analysis), which imply a significant reorganisation of the database structure.
The systematic performance of standardized analyses within the framework of the PS will make it possible to carry out descriptive epidemiology by identifying the health problems of populations and by measuring them in time and space. In addition to being able to infer the results obtained to a reference population, the laboratory analyses that complete the necropsy allow the cause of death to be investigated with greater precision.
Various criteria were considered in order to define the reference population for the sampling plan to conduct the PS. Emblematic Species already included in the strategy for monitoring contaminants in cetaceans (bottlenose dolphin for the 3 metropolitan seaboards, common dolphin for the Atlantic coast, harbour porpoise for the English Channel coast and stripped dolphin for the Mediterranean coast) on the French coast established by Pelagis within the framework of the MSFD were considered as a priority (Méndez-Fernandez et al., 2019). Moreover, to date, very few studies have been conducted on the pathogens circulating in marine mammal populations in France, not allowing species to be targeted according to their health vulnerability, with the exception of the striped dolphin, which has been the victim of mass mortalities caused by morbillivirus on several occasions in regional waters (Dhermain et al., 1995; Keck et al., 2010; Van Bressem et al., 2014) and harbour seals which have been victims of Avian Influenza in the North Sea (Bodewes et al., 2015). These two species were therefore also considered as a priority. The lack of general data at this stage has therefore led to the selection of the most frequently encountered stranded marine mammal species as reference populations in the first instance, while ensuring that the previously mentioned priority species are included. This will allow us to obtain initial data on the circulation of pathogens in these populations as well as on their health impact, and to subsequently make more specific choices concerning the marine mammal species and pathogens monitored. It is expected that after a period of one or two years, other strategic choices will be made. For example, instead of conducting the PS on a representative sample of the entire stranded population, the priority could be shifted to emblematic species. However, the distribution of logistical means and expertise on the territory did not allow to make this choice at this stage: for example, to be interested in the striped dolphins in the same way as the common dolphins would imply making a very important logistical effort in the Mediterranean coast. Thus, according to the current sampling plan, some species-seaboard pairs are under-represented, or even unrepresented when they constitute less than 0.5%. It will therefore be difficult or impossible to obtain valid data for these species, such as the long-finned pilot whale, which will be only marginally represented in the plan. The same applies to the maritime frontage. With less than ten animals in the sampling plan for the Mediterranean the information obtained will be very limited. It is also important to remember that current knowledge does not allow us to estimate the representativeness of the living marine mammal population based on strandings, which also implies a major limitation. Furthermore, the sampling plan is confronted with major sampling biases that must be considered when analyzing the various data obtained. Indeed, a series of non-standardised filters related to detectability, reporting, access to carcasses, DCC, human and logistical response capacities make these biases unavoidable and must therefore be considered when analyzing and interpreting the data.
Morevover, as these are mobile species that should be considered on a basin or regional scale, collaborations with neighbouring countries where strandings of animals from the same populations as those occurring on our coasts will be necessary. Although necropsy and common sampling protocols have already been proposed on a European scale, the situation is different for routine analysis (IJsseldijk et al., 2020a). Thus, a minimum of common routine analysis should be considered in order to obtain more global and representative data of these animal populations and to ensure large-scale health surveillance in a One Health context. The agreements of ASCOBANS and ACCOBAMS as well as the ICES and OSPAR working groups and the European Cetacean Society should be the framework for discussions and exchanges on practices with other European countries in order to harmonise not only necropsies and tissue sampling (Jauniaux et al., 2019; IJsseldijk et al., 2020a) but also the complementary analysis carried out by the laboratories as initiated at the pathology workshop of the European Cetacean Society in 1991 (Kuiken and García Hartmann, 1991).
The choices concerning standardised routine analysis within the framework of P3 and therefore of programmed surveillance were made according to several criteria: the improvement of knowledge and the interests for conservation and public health in relation to the financial cost and technical and logistical feasibility (easy access to laboratory techniques).The post mortem investigation and tissue sampling protocol already established under the auspices of ASCOBANS (Agreement on the Conservation of Small Cetaceans of the Baltic, North East Atlantic, Irish and North Seas) and ACCOBAMS (Accord sur la Conservation des Cétacés de la Mer Noire, de la Méditerranée et de la zone Atlantique adjacente) (IJsseldijk et al., 2020a), has guided our choices as well as the most frequently analysis performed in foreign European networks (Prenger-Berninghoff et al., 2008; van de Velde et al., 2016; Kershaw et al., 2017; Sonne et al., 2020; Stokholm et al., 2023). Investigations for morbilliviruses, influenza viruses and Brucella sp. will be sought for their known impact on marine mammal populations for the first two and for their zoonotic character for the last two. Morbilliviruses have indeed been the cause of mass mortalities on several occasions in regional waters, in the Mediterranean and in the North and Baltic Seas (Dhermain et al., 1995; Rijks et al., 2008; Keck et al., 2010; Duignan et al., 2014; Van Bressem et al., 2014). For influenza, a mass mortality event has also occurred in the North Sea (Bodewes et al., 2015). Furthermore, the risk for public health is not negligible as the high reassortment potential of this virus means that it could become more virulent and pathogenic, both for marine mammals and humans (Anthony et al., 2012; Fereidouni et al., 2016; Van den Brand et al., 2016). Similarly, brucellosis of marine origin, in addition to affecting reproduction, has repeatedly caused infections in humans and is most likely under-diagnosed (Brew et al., 1999; Jouffroy, 2020). Moreover, herpesviruses will be investigated as various studies have shown that these viruses circulate widely in marine mammal populations and that the clinical pictures, although variable, can lead to death (Duignan et al., 2018). Finally, mycotic agents will be looked for in their entirety as they are considered a major emerging cause of mortality in both humans and marine mammals (Reidarson et al., 2018). Other pathogens for which the risks are less known or for which massive mortality events have occurred but in distant waters have not been retained at this stage. They will nevertheless be the subject of reflection by a working group on the prioritisation of health hazards in marine mammals and may at any time be included in the P3 protocol or in the case of SES. In addition, future collaboration with neighbouring countries may identify other risks to be monitored.
The results of analysis carried out on carcasses, although they are of DCC 1 or 2, should be interpreted with caution, particularly for bacteriological analysis, due to possible post-mortem contamination (Palmiere et al., 2016). Also, it should always be considered that pathogen testing on carcasses allows us to know if the animal was infected at the time of death but not if the animal was infected earlier and then cured. Therefore, it does not give an overall picture of the circulation in the population. For this, it would be desirable to obtain seroprevalences as it has been carried out for avian influenza in seals (Bodewes et al., 2015; Measures and Fouchier, 2021).
Epidemiological vigilance will be ensured by the different modalities, in particular for cases managed with specific event-based surveillance. The example of the major avian influenza outbreak in Europe in 2022 (Adlhoch et al., 2022) reminds us of the importance of detecting potential outbreaks in marine mammal populations. Epidemiological vigilance will be constrained by certain limitations such as the DCC of the carcass, which will have a strong impact on the ability to obtain quality information. The identification of cases subject to specific event-based surveillance based on spontaneous reporting by correspondents implies that they are well informed and that case definitions are simple, sensitive and specific enough. In addition, case management under SES requires the availability of network correspondents trained in the level of examination and sampling protocol required for the case but also the involvement of laboratories performing the defined analysis. Although the volume of animals covered by this surveillance modality can be estimated, it is important to note that it is impossible to know precisely the number, nature and location of data that will be collected.
Beyond the defined strategy, ad hoc surveys may be carried out to answer specific questions from research projects or to respond to questions related to the epidemiological context, as could have been done by looking at the susceptibility of marine mammals to SARS-CoV-2 in marine mammals during the epidemic that Europe experienced, as it has been done in Italian waters for example (Audino et al., 2021).
Many aspects of the strategy are based on data obtained over the last five years for which all the information is already available (2016 to 2020). This arbitrary choice of a relatively short period was made to consider population shifts and changes in the environment (environmental parameters, fishing practices, construction of offshore parks…) and to try to be as close as possible to what might happen in the coming year. The time windows of the MSFD could possibly be used as early as 2025 (beginning of the third MSFD cycle) in order to harmonise the strategies.
Generally speaking, the strategy for strengthening health surveillance developed by Pelagis provides a framework that allows all the actors in the NSN to be informed of the approach followed and to understand its ins and outs. Thus, the effort of training (a 3 days initial training is required to incorporate the NSN, and retraining every five years at least, concurrently with the evolution of protocols) and informing correspondents is essential to guarantee their support and investment in the project and to limit operator bias harmonising practices. It is also important to continue to train new correspondents at the various levels, as the more numerous they are, the greater the number of animals evaluated.
The overall cost of this strategy, implemented in January 2023, is significant mainly due to the laboratory analysis carried out within the framework of the PS, which are added to the logistical costs of managing strandings. The discussions that will be conducted by the working group on the prioritisation of health hazards should make it possible to reach a compromise between the benefits of these analysis and the associated cost in the next two years.
Although there are many constraints to strengthening health monitoring, the structure of the NSN is very similar to that of an epidemiological surveillance network, which makes it a major strength. Moreover, the network has already proven its capacity to detect almost all strandings and to collect data on a large scale in space and time.
Similarly, health monitoring should focus on live marine mammals too. This mainly concerns pinnipeds, including those entering care centres before being released, but also live cetacean strandings. Finally, marine mammal populations in French overseas waters that face different problems to those in metropolitan France should also be subject to health monitoring.
Data availability statement
The original contributions presented in the study are included in the article/supplementary material. Further inquiries can be directed to the corresponding author.
Author contributions
The main work was carried out by SW under the guidance of FC, and with the main support of EM. All authors contributed to the article and approved the submitted version.
Acknowledgments
We would like to thank the veterinarians implicated in this work for their valuable help and their enthusiasm. We are grateful to all the members of the French stranding scheme for their continuous effort in collecting data on stranded cetaceans. The Observatoire PELAGIS is funded by the Ministry in charge of the Environment, the French Office for Biodiversity (Office Français pour la Biodiversité—AFB) and the Communauté d’Agglomération de la Ville de La Rochelle.
Conflict of interest
The authors declare that the research was conducted in the absence of any commercial or financial relationships that could be construed as a potential conflict of interest.
Publisher’s note
All claims expressed in this article are solely those of the authors and do not necessarily represent those of their affiliated organizations, or those of the publisher, the editors and the reviewers. Any product that may be evaluated in this article, or claim that may be made by its manufacturer, is not guaranteed or endorsed by the publisher.
References
Adlhoch C., Fusaro A., Gonzales J. L., Kuiken T., Marangon S., Niqueux É., et al. (2022). Avian influenza overview march – June 2022. EFSA J. 20 (8), e07415. doi: 10.2903/j.efsa.2022.7415
Anthony S. J., St. Leger J. A., Pugliares K., Ip H. S., Chan J. M., Carpenter Z. W., et al. (2012). Emergence of fatal avian influenza in new England harbor seals. mBio 3 (4), e00166–e00112. doi: 10.1128/mBio.00166-12
Arbelo M., Espinosa de los Monteros A., Herráez P., Andrada M., Sierra E., Rodríguez F., et al. (2013). Pathology and causes of death of stranded cetaceans in the canary islands, (1999-2005). Dis. Aquat. Organisms 103 (2), 87–99. doi: 10.3354/dao02558
Audino T., Grattarola C., Centelleghe C., Peletto S., Giorda F., Florio C. L., et al. (2021). SARS-CoV-2, a threat to marine mammals? a study from Italian seawaters. Animals: an Open Access J. MDPI 11 (6), 1663. doi: 10.3390/ani11061663
Audino T., Berrone E., Grattarola C., Giorda F., Mattioda V., Martelli W., et al. (2022). Potential SARS-CoV-2 susceptibility of cetaceans stranded along the Italian coastline. Pathogens 11 (10), 1096. doi: 10.3390/pathogens11101096
Authier M., Peltier H., Dorémus G., Dabin W., Canneyt O., Ridoux V. (2014). How much are stranding records affected by variation in reporting rates? a case study of small delphinids in the bay of Biscay. Biodiversity Conserv. 23, 2591–2612. doi: 10.1007/s10531-014-0741-3
Bodewes R., Bestebroer T. M., van der Vries E., Verhagen J. H., Herfst S., Koopmans M. P., et al. (2015). Avian influenza A(H10N7) virus–associated mass deaths among harbor seals. Emerging Infect. Dis. 21 (4), 720–722. doi: 10.3201/eid2104.141675
Bossart G. D. (2011). Marine mammals as sentinel species for oceans and human health. Veterinary Pathol. 48 (3), 676–690. doi: 10.1177/0300985810388525
Bresson-Hadni S., Mantion G.-A., Vuitton D.-A., Delabrousse E., Bartholomot B., Miguet J.-P. (2004). Échinococcose alvéolaire en région parisienne. Gastroentérologie Clinique Biologique 28 (11), 1147–1151. doi: 10.1016/S0399-8320(04)95194-9
Brew S. D., Perrett L. L., Stack J. A., MacMillan A. P., Staunton N. J. (1999). Human exposure to brucella recovered from a sea mammal. Veterinary Rec. 144 (17), 483.
Buttke D., Wild M. (2014). The role of one health in wildlife conservation: a challenge and opportunity. J. wildlife Dis. 51 (1), 1–8. doi: 10.7589/2014-01-004
Cariou R., Méndez-Fernandez P., Hutinet S., Guitton Y., Caurant F., Le Bizec B., et al. (2021). Nontargeted LC/ESI-HRMS detection of polyhalogenated compounds in marine mammals stranded on French Atlantic coasts. ACS ES&T Water 1 (2), 309–318. doi: 10.1021/acsestwater.0c00091
Chan D. K. P., Tsui H. C. L., Kot B. C. W. (2017). Database documentation of marine mammal stranding and mortality: current status review and future prospects. Dis. Aquat. Organisms 126 (3), 247–256. doi: 10.3354/dao03179
Chouvelon T., Gilbert L., Caurant F., Méndez‐Fernandez P., Bustamante P., Brault‐Favrou M., et al. (2022). Nutritional grouping of marine forage species reveals contrasted exposure of high trophic levels to essential micro-nutrients. Oikos 2022 (7), e08844. doi: 10.1111/oik.08844
Cleaveland S., Appel M. G., Chalmers W. S., Chillingworth C., Kaare M., Dye C. (2000). Serological and demographic evidence for domestic dogs as a source of canine distemper virus infection for Serengeti wildlife. Veterinary Microbiol. 72 (3–4), 217–227. doi: 10.1016/s0378-1135(99)00207-2
Clutton-Brock T., Huchard E. (2013). Social competition and its consequences in female mammals. J. Zoology 289 (3), 151–171. doi: 10.1111/jzo.12023
Coombs E. J., Deaville R., Sabin R. C., Allan L., O’Connell M., Berrow S., et al. (2019). What can cetacean stranding records tell us? a study of UK and Irish cetacean diversity over the past 100 years. Mar. Mammal Sci. 35 (4), 1527–1555. doi: 10.1111/mms.12610
Crespo E. A., Hall M. A. (2001). “Interactions between aquatic mammals and humans in the context of ecosystem management,” in Marine mammals: biology and conservation. Eds. Evans P. G. H., Raga J. A. (Boston: MA: Springer US), 463–490. doi: 10.1007/978-1-4615-0529-7_13
Cunningham A. A., Daszak P., Wood J. L. N. (2017). One health, emerging infectious diseases and wildlife: two decades of progress? Philos. Trans. R. Soc. London. Ser. B Biol. Sci. 372 (1725), 20160167. doi: 10.1098/rstb.2016.0167
Dars C., Meheust E., Genu M., Méndez-Fernandez P., Peltier H., Wund S., et al. (2021). Rapport Annuel: Les échouages de mammifères marins sur le littoral français en 2021 (Université de La Rochelle et CNRS: Observatoire Pelagis).
de Quirós Y. B., de Hartwick M., Rotstein D. S., Garner M. M., Bogomolni A., Greer W., et al. (2018). Discrimination between bycatch and other causes of cetacean and pinniped stranding. Dis. Aquat. Organisms 127 (2), 83–95. doi: 10.3354/dao03189
Dhermain F., Bompar J. M., Chappuis G., Folacci M., Poitevin F.. (1995). The morbillivirus epidemic of the strip dolphin stenella coeruleoalba in the Mediterranean sea [1994]. Recueil Medecine Veterinaire (France) 170, 85–92.
Duignan P. J., Bressem M.-F. V., Cortéz-Hinojosa G., Kennedy-Stoskopf S.. (2014). Phocine distemper virus: current knowledge and future directions. Viruses 6 (12), 5093–5134. doi: 10.3390/v6125093
Duignan P. J., Van Bressem M.-F., Baker J. D., Barbieri M., Colegrove K. M., De Guise S., et al. (2018). “Viruses,” in CRC Handbook of marine mammal medicine, 3rd edn (Boca Raton, Florida:CRC Press).
Dunn D. G., Barco S. G., Pabst D. A., McLellan W. A.. (2002). Evidence for infanticide in bottlenose dolphins of the western north atlantic. J. Wildlife Dis. 38 (3), 505–510. doi: 10.7589/0090-3558-38.3.505
Epple A. L., Daniel J. T., Barco S. G., Rotstein D. S., Costidis A. M.. (2020). Novel necropsy findings linked to peracute underwater entrapment in bottlenose dolphins (Tursiops truncatus). Front. Mar. Sci. 7. doi: 10.3389/fmars.2020.00503
Fereidouni S., Munoz O., Von Dobschuetz S., De Nardi M.. (2016). Influenza virus infection of marine mammals. EcoHealth 13 (1), 161–170. doi: 10.1007/s10393-014-0968-1
Gauthier D., Lamberger K., Decors A. (2016). Vade-mecum des laboratoires départementaux d’analyses vétérinaires -le diagnostic des maladies de la faune sauvage libre. Office Natl. la Chasse la Faune Sauvage. 80.
Gross S., Claus P., Wohlsein P., Kesselring T., Lakemeyer J., Reckendorf A., et al. (2020). Indication of lethal interactions between a solitary bottlenose dolphin (Tursiops truncatus) and harbor porpoises (Phocoena phocoena) in the German Baltic Sea. BMC Zoology 5 (1), 12. doi: 10.1186/s40850-020-00061-7
Gross S., Müller A., Seinige D., Wohlsein P., Oliveira M., Steinhagen D., et al. (2022). Occurrence of antimicrobial-resistant escherichia coli in marine mammals of the north and Baltic seas: sentinels for human health. Antibiotics 11 (9), 1248. doi: 10.3390/antibiotics11091248
Guillerit F. (2017). Vers un réseau de surveillance épidémiologique des mammifères marins: valorisation du réseau national Échouages. thèse d’exercice (Ecole Nationale Vétérinaire d’ Alfort: École nationale vétérinaire d’Alfort).
Gulland F. M. D., Dierauf L. A., Whitman K. L. (2018). CRC Handbook of marine mammal medicine. 3rd edn (Boca Raton, Florida: CRC Press).
Hall A. J., Hugunin K., Deaville R., Law R. J., Allchin C. R., Jepson P. D.. (2006). The risk of infection from polychlorinated biphenyl exposure in the harbor porpoise (Phocoena phocoena): a case–control approach. Environ. Health Perspect. 114 (5), 704–711. doi: 10.1289/ehp.8222
Hennessy M. B., Deak T., Schiml-Webb P. A. (2001). Stress-induced sickness behaviors: an alternative hypothesis for responses during maternal separation. Dev. Psychobiology 39 (2), 76–83. doi: 10.1002/dev.1031
Herr H., Burkhardt-Holm P., Heyer K., Siebert U., Selling J. (2020). Injuries, malformations, and epidermal conditions in cetaceans of the strait of Gibraltar. Aquat. Mammals 46, 215–235. doi: 10.1578/AM.46.2.2020.215
IJsseldijk L.L., Brownlow A.C., Mazzariol S. eds. (2020a) Best Practice on Cetacean Post-mortem Investigation and Tissue Sampling | ASCOBANS-ACCOBAMS. Available at: https://www.ascobans.org/en/document/best-practice-cetacean-post-mortem-investigation-and-tissue-sampling-0 (Accessed 27 April 2022).
IJsseldijk L. L., ten Doeschate M. T. I., Brownlow A., Davison N. J., Deaville R., Galatius A., et al. (2020b). Spatiotemporal mortality and demographic trends in a small cetacean: strandings to inform conservation management. Biol. Conserv. 249, 108733. doi: 10.1016/j.biocon.2020.108733
IJsseldijk L. L., Scheidat M., Siemensma M. L., Couperus B., Leopold M. F., Morell M., et al. (2021). Challenges in the assessment of bycatch: postmortem findings in harbor porpoises (Phocoena phocoena) retrieved from gillnets. Veterinary Pathol. 58 (2), 405–415. doi: 10.1177/0300985820972454
Jauniaux T., García Hartmann M., Haelters J., J T., F C. (2002). Echouage de mammiféres marins : guide d’intervention et procédures d’autopsie. = Stranding of marine mammals: operations guide and necropsy procedures. Ann. Médecine Vét 146, 261–276. ISSN 0003-4118.
Jauniaux T., André M., Dabin W., Vreese de Gillet S. A., Morell M. (2019)Marine mammals stranding: guidelines for post-mortem investigations of cetaceans & pinnipeds. In: 13rd cetacean necropsy workshop (Liège). Available at: https://upcommons.upc.edu/handle/2117/335529 (Accessed 4 February 2022).
Jouffroy K. (2020). Incidence de brucella spp. chez les mammifères marins échoués sur les côtes belge et française. travail de fin d’Etudes vétérinaires (Liège, Belgique: Université de Liège).
Kapetanou A., IJsseldijk L. L., Willems D. S., Broens E. M., Everaarts E., Buil J. B., et al. (2020). Mycotic infections in free-ranging harbor porpoises (Phocoena phocoena). Front. Mar. Sci. 7, 344. doi: 10.3389/fmars.2020.00344
Karesh W. B., Cook R. A. (2005). The human-animal link. Foreign Affairs 84 (4), 38–50. doi: 10.2307/20034419
Keck N., Kwiatek O., Dhermain F., Dupraz F., Boulet H., Danes C., et al. (2010). Resurgence of Morbillivirus infection in Mediterranean dolphins off the French coast. Veterinary Rec. 166 (21), 654–655. doi: 10.1136/vr.b4837
Kershaw J., Stubberfield E., Foster G., Brownlow A., Hall A., and Perrett L.. (2017). Exposure of harbour seals phoca vitulina to brucella in declining populations across Scotland. Dis. Aquat. Organisms 126 (1), 13–23. doi: 10.3354/dao03163
Kuiken T. (1996). ‘Diagnosis of By-catch in Cetaceans.” In Proceedings of the Second European Cetacean Society Workshop on Cetacean Pathology , Montpellier, France, 2 March 1994. in (The Society), 56.
Kuiken T., Leighton F. A., Fouchier R. M., LeDuc J. W., Peiris J. S.M., Schudel A., et al. (2005). Public health. pathogen surveillance in animals. Sci. (New York N.Y.) 309 (5741), 1680–1681. doi: 10.1126/science.1113310
Kuiken T., García Hartmann M. (1991). Proceedings of the first ECS workshop on cetacean pathology: dissection techniques and tissue sampling: Leiden, the Netherlands, 13-14 September 1991 (Stralsund, Germany: European Cetacean Society (ECS newsletter (European Cetacean Society).
Laporte R., Dabin W., Dars C., Caurant F. (2021). “Guide de reconnaissance et de description des états pathologiques des organes internes de mammifères marins / biosécurité et protocole de dissection,” in Cahier technique de l’Observatoire PELAGIS sur le suivi de la mégafaune marine (Université de La Rochelle et CNRS: La Rochelle Université et CNRS), 56 pages.
Mahfouz C., Henry F., Courcot L., Pezeril S., Bouveroux T., Dabin W., et al. (2014). Harbour porpoises (Phocoena phocoena) stranded along the southern north Sea: an assessment through metallic contamination. Environ. Res. 133, 266–273. doi: 10.1016/j.envres.2014.06.006
Massen J. J. M., Ritter C., Bugnyar T. (2015). Tolerance and reward equity predict cooperation in ravens (Corvus corax). Sci. Rep. 5 (1), 15021. doi: 10.1038/srep15021
Measures L. N., Fouchier R. A. M. (2021). Antibodies against influenza virus types a and b in canadian seals. J. Wildlife Dis. 57 (4), 808–819. doi: 10.7589/JWD-D-20-00175
Méndez-Fernandez P., Caurant F., Spitz J. (2019). Stratégie de suivi des contaminants chez les cétacés des côtes françaises. Observatoire Pelagis, 24.
Méndez-Fernandez P., Spitz J., Dars C., Dabin W., Mahfouz C., André J.-M., et al. (2022). Two cetacean species reveal different long-term trends for toxic trace elements in European Atlantic French waters. Chemosphere 294, 133676. doi: 10.1016/j.chemosphere.2022.133676
Moore K. T., Barco S. G. (2013). Handbook for recognizing, evaluating, and documenting human interaction in stranded cetaceans and pinnipeds (Southwest Fisheries Science Center (U.S). Available at: https://repository.library.noaa.gov/view/noaa/4429.
Morell M., Rojas L., Haulena M., Busse B., Siebert U., Shadwick R. E., et al. (2022). Selective inner hair cell loss in a neonate harbor seal (Phoca vitulina). Animals 12 (2), 180. doi: 10.3390/ani12020180
Nery M. F., Simão S. M. (2009). Sexual coercion and aggression towards a newborn calf of marine tucuxi dolphins (Sotalia guianensis). Mar. Mammal Sci. 25 (2), 450–454. doi: 10.1111/j.1748-7692.2008.00275.x
Newman S. J., Smith S. A. (2006). Marine mammal neoplasia: a review. Veterinary Pathol. 43 (6), 865–880. doi: 10.1354/vp.43-6-865
Numberger D., Siebert U., Fulde M., Valentin-Weigand P.. (2021). Streptococcal infections in marine mammals. Microorganisms 9 (2), 350. doi: 10.3390/microorganisms9020350
Ossiboff R. J., Stacy N. I., Origgi F. C. (2021). Health and disease in free-ranging and captive wildlife. Front. Media SA 7. doi: 10.3389/fvets.2020.620685
Palmiere C., Egger C., Prod’Hom G., Greub G. (2016). Bacterial translocation and sample contamination in postmortem microbiological analyses. J. Forensic Sci. 61 (2), 367–374. doi: 10.1111/1556-4029.12991
Peltier H., Beaufils A., Cesarini C., Dabin W., Dars C., Demaret F., et al. (2019). Monitoring of marine mammal strandings along French coasts reveals the importance of ship strikes on Large cetaceans: a challenge for the European marine strategy framework directive. Front. Mar. Sci. 6. doi: 10.3389/fmars.2019.00486
Peltier H., Authier M., Dabin W., Dars C., Demaret F., Doremus G., et al. (2020). Can modelling the drift of bycaught dolphin stranded carcasses help identify involved fisheries? an exploratory study. Global Ecol. Conserv. 21, e00843. doi: 10.1016/j.gecco.2019.e00843
Peltier H., Authier M., Caurant F., Dabin W., Daniel P., Dars C., et al. (2021). In the wrong place at the wrong time: identifying spatiotemporal Co-occurrence of bycaught common dolphins and fisheries in the bay of Biscay (NE Atlantic) from 2010 to 2019. Front. Mar. Sci. 8. doi: 10.3389/fmars.2021.617342
Perrin W. F., Geraci J. R. (2009). “Stranding,” in Encyclopedia of marine mammals (Second edition). Eds. Perrin W. F., Würsig B., Thewissen J. G. M. (London: Academic Press), 1118–1123. doi: 10.1016/B978-0-12-373553-9.00256-X
Pintore M. D., Mignone W., Di Guardo G., Mazzariol S., Ballardini M., Florio C. L., et al. (2018). Neuropathologic findings in cetaceans stranded in italy, (2002–14). J. Wildlife Dis. 54 (2), 295–303. doi: 10.7589/2017-02-035
Prenger-Berninghoff E., Siebert U., Stede M., König A., Weiß R., Baljer G. (2008). Incidence of brucella species in marine mammals of the German north Sea. Dis. Aquat. Organisms 81, 65–71. doi: 10.3354/dao01920
Pryce C. R., Rüedi-Bettschen D., Dettling A. C., Feldon J. (2002). Early life stress: long-term physiological impact in rodents and primates. News Physiol. Sciences: Int. J. Physiol. Produced Jointly by Int. Union Physiol. Sci. Am. Physiol. Soc. 17, 150–155. doi: 10.1152/nips.01367.2001
Read A. J., Murray K. T. (2000). Gross evidence of human-induced mortality in small cetaceans. Ed. Duke M. L. (United States: University and N.M.F.S). Available at: https://repository.library.noaa.gov/view/noaa/3679.
Reidarson T. H., Garcia-Parraga D., Wiederhold N. (2018). “Marine mammal mycoses,” in Gulland et al. CRC handbook of marine mammal medicine, 3rd edn (Boca Raton, Florida: CRC Press), 389–424.
Réveillaud É., Desvaux S., Boschiroli M.-L., Hars J., Faure É., Fediaevsky A., et al. (2018). Infection of wildlife by mycobacterium bovis in France assessment through a national surveillance system, sylvatub. Front. Veterinary Sci. 5. doi: 10.3389/fvets.2018.00262 (Accessed: 1 March 2023).
Rijks J. M., Read F. L., van de Bildt M. W. G., van Bolhuis H. G., Martina B. E. E., Wagenaar J. A., et al. (2008). Quantitative analysis of the 2002 phocine distemper epidemic in the Netherlands. Veterinary Pathol. 45 (4), 516–530. doi: 10.1354/vp.45-4-516
Ross H. M., Wilson B. (1996). Violent interactions between bottlenose dolphins and harbour porpoises. Proc. R. Soc. London. Ser. B: Biol. Sci. 263 (1368), 283–286. doi: 10.1098/rspb.1996.0043
Rouby E., Dubroca L., Cloâtre T., Demanèche S., Genu M., Macleod K., et al. (2022). Estimating bycatch from non-representative samples (II): a case study of pair trawlers and common dolphins in the bay of Biscay. Front. Mar. Sci. 8. doi: 10.3389/fmars.2021.795942
Simmonds M. P. (2012). Cetaceans and marine debris: the great unknown. J. Mar. Biol. 2012, e684279. doi: 10.1155/2012/684279
Sleeman J. M., DeLiberto T., Nguyen N. (2017). Optimization of human, animal, and environmental health by using the one health approach. J. Veterinary Sci. 18 (S1), 263–268. doi: 10.4142/jvs.2017.18.S1.263
Sonne C., Lakemeyer J., Desforges J.-P., Eulaers I., Persson S., Stokholm I., et al. (2020). A review of pathogens in selected Baltic Sea indicator species. Environ. Int. 137, 105565. doi: 10.1016/j.envint.2020.105565
Spitz J., Ridoux V., Brind’Amour A. (2014). Let’s go beyond taxonomy in diet description: testing a trait-based approach to prey–predator relationships. J. Anim. Ecol. 83 (5), 1137–1148. doi: 10.1111/1365-2656.12218
Stephens P. A., Russell A. F., Young A. J., Sutherland W. J., Clutton‐Brock T. H. (2005). Dispersal, eviction, and conflict in meerkats (Suricata suricatta): an evolutionarily stable strategy model. Am. Nat. 165 (1), 120–135. doi: 10.1086/426597
Stokholm I., Puryear W., Sawatzki K., Knudsen S. W., Terkelsen T., Becher P., et al. (2021). ‘Emergence and radiation of distemper viruses in terrestrial and marine mammals’, proceedings. Biol. Sciences 288(1961) p, 20211969. doi: 10.1098/rspb.2021.1969
Stokholm I., Baechlein C., Persson S., Roos A., Galatius A., Kyhn L. A., et al. (2023). Screening for influenza and morbillivirus in seals and porpoises in the Baltic and north Sea. Pathogens 12 (3), 357. doi: 10.3390/pathogens12030357
Tacconelli E., Carrara E., Savoldi A., Harbarth S., Mendelson M., Monnet D. L., et al. (2018). Discovery, research, and development of new antibiotics: the WHO priority list of antibiotic-resistant bacteria and tuberculosis. Lancet Infect. Dis. 18 (3), 318–327. doi: 10.1016/S1473-3099(17)30753-3
Tacconelli E., Magrini N. (2017). Global priority list of antibiotic-resistant bacteria to guide research, discovery, and development of new antibiotics. South Africa: Infection Control Africa Network. Available at: https://policycommons.net/artifacts/1818147/global-priority-list-of-antibiotic-resistant-bacteria-to-guide-research-discovery-and-development/2555608/.
Thompson F. J., Cant M. A., Marshall H. H., Vitikainen E. I. K., Sanderson J. L., Nichols H. J., et al. (2017). Explaining negative kin discrimination in a cooperative mammal society. Proc. Natl. Acad. Sci. USA 114 (20), 5207–5212. doi: 10.1073/pnas.1612235114
Toma B., Shaw A., Rivière J., Moutou F., Dufour B., Benet J.-J. (2010). Epidémiologie appliquée à la lutte collective contre les maladies animales transmissibles majeures. 4th edn (AEEMA).
Van Bressem M.-F., Duignan P. J., Banyard A., Barbieri M., Colegrove K. M., De Guise S., et al. (2014). Cetacean morbillivirus: current knowledge and future directions. Viruses 6 (12), 5145–5181. doi: 10.3390/v6125145
Van Canneyt O., Dabin W., Cécile D., Dorémus G., Laurence G., Ridoux V., et al. (2015). Guide des échouages de mammifères marins. Université de La Rochelle et CNRS. doi: 10.13140/RG.2.1.1495.6002
Van den Brand J., Wohlsein P., Herfst S., Bodewes R., Pfankuche V. M., van de Bildt M., et al. (2016). Influenza a (H10N7) virus causes respiratory tract disease in harbor seals and ferrets. PloS One 11 (7), e0159625. doi: 10.1371/journal.pone.0159625
van de Velde N., Devleesschauwer B., Leopold M., Begeman L., IJsseldijk L., Hiemstra S., et al. (2016). Toxoplasma gondii in stranded marine mammals from the north Sea and Eastern Atlantic ocean: findings and diagnostic difficulties. Veterinary Parasitol. 230, 25–32. doi: 10.1016/j.vetpar.2016.10.021
Vetulani J. (2013). Early maternal separation: a rodent model of depression and a prevailing human condition. Pharmacol. reports: PR 65 (6), 1451–1461. doi: 10.1016/s1734-1140(13)71505-6
Viana M., Cleaveland S., Matthiopoulos J., Halliday J., Packer C., Craft M. E., et al. (2015). Dynamics of a morbillivirus at the domestic-wildlife interface: canine distemper virus in domestic dogs and lions. Proc. Natl. Acad. Sci. USA 112 (5), 1464–1469. doi: 10.1073/pnas.1411623112
Vuitton D. A., Bresson-Hadni S., Giraudoux P., Bartholomot B., Laplante J.-J., Delabrousse E., et al. (2010). Échinococcose alvéolaire : d’une maladie rurale incurable à une infection urbaine sous contrôle? La Presse Médicale 39 (2), 216–230. doi: 10.1016/j.lpm.2008.10.028
World Health Organization (2002) The importance of pharmacovigilance (World Health Organization). Available at: https://apps.who.int/iris/handle/10665/42493 (Accessed 3 March 2023).
Keywords: marine mammals, health surveillance, eco-epidemiology, One Health-strandings, epidemiosurveillance network, conservation, wildlife, metropolitan France
Citation: Wund S, Méheust E, Dars C, Dabin W, Demaret F, Guichard B, Jauniaux T, Labrut S, Spitz J, Van Canneyt O and Caurant F (2023) Strengthening the health surveillance of marine mammals in the waters of metropolitan France by monitoring strandings. Front. Mar. Sci. 10:1116819. doi: 10.3389/fmars.2023.1116819
Received: 05 December 2022; Accepted: 10 April 2023;
Published: 01 May 2023.
Edited by:
Stephanie Plön, Stellenbosch University, South AfricaReviewed by:
Kristina Lehnert, University of Veterinary Medicine Hannover, GermanyVanessa Estrade, GLOBICE REUNION, Réunion
Copyright © 2023 Wund, Méheust, Dars, Dabin, Demaret, Guichard, Jauniaux, Labrut, Spitz, Van Canneyt and Caurant. This is an open-access article distributed under the terms of the Creative Commons Attribution License (CC BY). The use, distribution or reproduction in other forums is permitted, provided the original author(s) and the copyright owner(s) are credited and that the original publication in this journal is cited, in accordance with accepted academic practice. No use, distribution or reproduction is permitted which does not comply with these terms.
*Correspondence: S. Wund, sarah.wund@univ-lr.fr