Five golden principles to advance marine reef restoration by linking science and industry
- 1Department Hydraulic Engineering, Faculty of Civil Engineering and Geosciences, Delft University of Technology, Delft, Netherlands
- 2Department Engineering & Estimating, Van Oord Dredging and Marine Contractors, Rotterdam, Netherlands
- 3Department of Estuarine and Delta Systems, Royal Netherlands Institute for Sea Research (NIOZ), Yerseke, Netherlands
- 4Department of Physical Geography, Faculty of Geosciences, Utrecht University, Utrecht, Netherlands
- 5Delta Academy Applied Research Centre, HZ University of Applied Sciences, Vlissingen, Netherlands
Marine reef ecosystems have degraded massively worldwide, and restoration efforts have as yet not managed to realize the scale required to reverse continued degradation. To achieve effective scales, scientific insights in restoration methods should be paired with industry-based approaches used for infrastructural development. We illustrate by five principles how long-standing experience of marine contractors with executing large-scale projects, can support reef restoration: i) utilizing industrial techniques to achieve positive impact at scale, ii) landscaping infrastructure to optimize habitat for targeted species, iii) inducing life to overcome connectivity bottle-necks and steer community composition, iv) designing nature development efforts to be self-sustainable, and v) ensuring continuity beyond project boundaries by early stakeholder engagement. Consciously connecting scientific knowledge to industry-based activities increases the likelihood that marine infrastructure development and ecosystem rehabilitation can be aligned. We plead that synergizing practices by science and industry is needed to upscale restoration efforts and truly improve marine reef ecosystems.
Introduction: advancing reef restoration
Reef ecosystems such as oyster and coral reefs have declined rapidly worldwide (e.g., Beck et al., 2011; Eddy et al., 2018). Restoration efforts in terms of “assisting the recovery of an ecosystem that has been degraded, damaged or destroyed” (SER, 2004), are undertaken to protect biodiversity, secure food provision, and mitigate climate change through carbon storage (Sala et al., 2021). However, these don’t keep pace with the ongoing changes in our world caused by coastal urbanization, warming temperatures, and rising sea levels (Suding, 2011; Bellwood et al., 2019). Moreover, while restoration efforts have shown increased provision of biodiversity and ecosystem services, these are typically lower than in intact reference ecosystems (Rey Benayas et al., 2009). A key challenge identified to achieve more effective ecosystem restoration is the development of scalable restoration methods (Rinkevich, 2008; Abelson et al., 2020; Duarte et al., 2020). This is where industry can be of support, having the capability and experience of executing large-scale operations. For industry it is also of interest to invest in restoration practices, in order to build a track record that allows industry to be able to include nature-based solutions in future contracts, to improve reputation, and to offer employer attractiveness.
Marine construction works modify seascapes by replacing natural habitats and changing environmental conditions critical to habitat persistence (Bugnot et al., 2021). However, they can be designed to incorporate ecological principles that benefit marine life (Dafforn et al., 2015; Laboyrie et al., 2018). By no means this should be used as excuse to ignore or down-play the negative impact that infrastructural developments may have on a marine system (Firth et al., 2020) or as argument to restrict restoration efforts only to where infrastructural works take place. However, nature restoration goals and marine construction works can be synergized much better to not miss out on unique nature-enhancing opportunities. In this paper we present five golden principles on how marine contractors can support reef ecosystem restoration.
Principle I. Pursue upscaling – use industry-based techniques
Current practices for restoration are often too small in scope to combat the extent of anthropogenic threats driving habitat loss (Hughes et al., 2017; Bellwood et al., 2019). Hence, there is urgent need to move to cost-effective solutions that can be implemented at the kilometer-scale or above (Airoldi et al., 2021). Such innovative solutions might be borrowed from industries, as they have already discovered economy of scale (Price and Toonen, 2017) and can provide technological advances leading to efficiencies of scale (Abelson et al., 2020). Large scale and good connectivity of restoration sites is important for their sustainability, as it affects both biotic and abiotic interactions (Menz et al., 2013). For example, small and isolated restoration sites will have less genetic diversity, resulting in reduced resilience. Connectivity with remnant ecosystems, through proximity, stepping stones or corridors, allows for the exchange of species and genes, potentially resulting in enhanced biodiversity and resilience of the restored areas (Vaughn et al., 2010). For restoration practices to become both ecologically successful and cost effective, interventions should be executed at a large enough scale and include remediation of degraded ecosystems if necessary (Abelson et al., 2020).
Example upscaling assisted recruitment
Upscaling reef restoration is illustrated by the concept of using industry-based techniques for harvesting of coral larvae over vital reefs and releasing these on degraded ones along the Great Barrier Reef (Figure 1I). The concept entails large scale collection of coral spawn slicks with oil booms, pumping these slicks into storage tanks of commercial trailing suction hopper dredgers, culturing billions of larvae while being transported to degraded reefs, and once the larvae become settlement-competent, deploying them on these degraded reefs to initiate restoration (see Doropoulos et al., 2018). This restoration method is estimated to be much more cost-effective than restoring the same vast geographical area with garden-grown corals (Doropoulos et al., 2019). Also, the effect on the maintenance of natural populations is minimal, as it accesses an insignificant fraction of gametes released during a spawning event and refrains from any physical loss of the reef skeleton (Doropoulos et al., 2018). In potential, it provides the means for increasing coral settlement and assisting gene flow between isolated populations to increase coral recruitment at unprecedented scale on strategically important reefs.
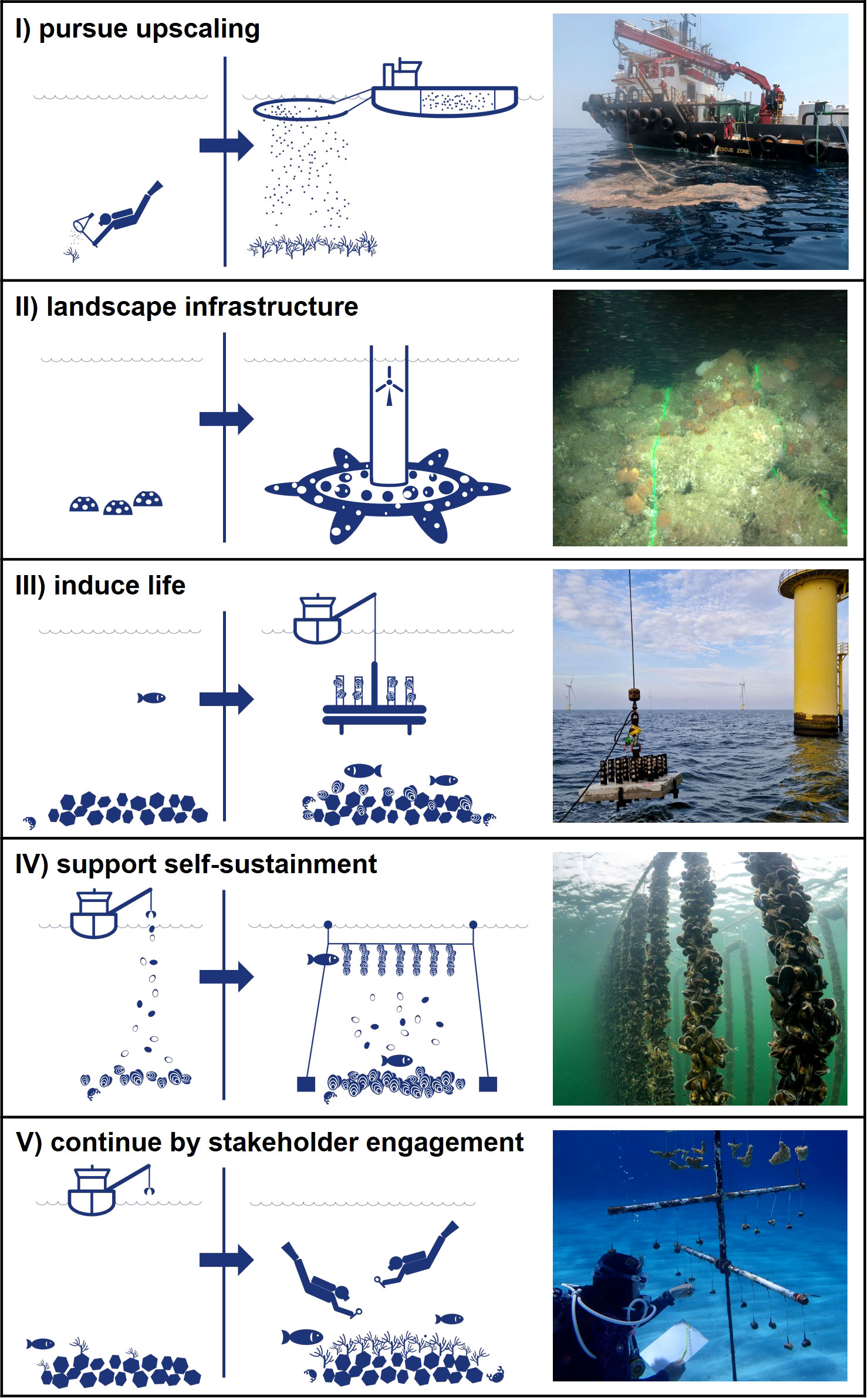
Figure 1 The five golden principles to advance marine reef restoration by linking science and industry, illustrated by examples from practice: (I) pursue upscaling: Changing from manually collecting coral gametes to harvesting using industry-based techniques to achieve positive impact at scale. (II) landscape infrastructure: Changing from using artificial reef structures to nature-friendly designs of marine infrastructure to establish habitat complexity. (III) induce life: Changing from lack of recruitment to installation of broodstock to overcome connectivity bottle-necks and kickstart reef development. (IV) support self-sustainment: Changing from ad hoc human interventions to continuous nature-steered supply of materials to create suitable conditions for reef restoration. (V) continue by stakeholder engagement: Changing from short-term restoration efforts during construction projects to long-term gains through stakeholder engagement beyond project boundaries. Photo credit: I, II, III, V Van Oord; IV Oscar Bos.
Principle II. Landscaping – optimizing marine infrastructure as habitat
Restoring reef ecosystems is often done through the installation of artificial reef structures, to provide hard substrate in varying three-dimensional shapes to promote biodiversity (Baine, 2001; Vivier et al., 2021). These structures are tailor-made for a local system, targeting specific species groups, such as corals (Higgins et al., 2022) or fish (Paxton et al., 2020). Often concrete is used as their main construction material, allowing variation in both micro- and meso-habitat complexity (ter Hofstede et al., 2023). Downsides of using concrete include its toxicity, as the cement mortars often leach trace metals over time (Hillier et al., 1999; Wilding and Sayer, 2002), and emissions of carbon dioxide during its fabrication process (Fennell et al., 2021). Moreover, for artificial reef structures to achieve impact at scale, they need to be deployed in huge quantities, as biomass and species richness of the associated marine life is proportional to their extent (Bohnsack and Sutherland, 1985).
Besides restoring habitat by consciously adding artificial reef structures, it should also be considered to achieve the desired impacts by optimizing existing or novel marine infrastructure. This landscaping could serve similar restoration goals, be it at a much larger scale, even by using the same or only marginal additional materials. Marine construction works such as coastal breakwaters, quay walls in ports, and scour protection in offshore wind farms, already inherently provide artificial habitat. Their long-term presence allows nature development, and designs can be optimized to target desired species. For example, if concrete is used as construction material, its texture can be roughened to mimic natural rock which promotes colonization by pioneering species (Moschella et al., 2005; Potet et al., 2021), and its toxicity can be reduced by using nature-friendly adhesives (Perkol-Finkel and Sella, 2014). Improved reef habitat can be achieved at a far larger scale, more cost-efficient and with a lower carbon-footprint when optimizing the marine infrastructure than by just adding artificial reef structures.
It is recognized that a location for infrastructural development is typically selected for human needs, not for nature goals. Marine reef restoration is required at scales far beyond these locations. Therefore, optimizing marine infrastructure for reef development will never be able to fully replace targeted restoration, but it does provide a valuable extra opportunity to restore marine reefs at scale.
Example ecological enhanced marine infrastructure
Landscaping infrastructure to serve as habitat for marine species can be illustrated by designing nature inclusive scour protections at the base of wind turbines in offshore wind farms. These scour protections are layers of rock material, to prevent the seabed from scouring due to monopile induced turbulence and flow acceleration (Guan et al., 2022). They generally resemble a flat pancake, composed of a filter base layer consisting of small-sized quarried rock, such as granite, topped with an armor layer of larger rocks (ter Hofstede et al., 2022). The rocky material acts as an artificial reef, hosting a broad range of marine species (Coolen et al., 2018; ter Hofstede et al., 2022). Conventional scour protection can be adjusted to increase the habitat complexity by bringing in more variety in use of materials, shapes and dimensions (ter Hofstede et al., 2023; Figure 1II), and is expected to result in a higher biodiversity (Lapointe and Bourget, 1999; Firth et al., 2014). The use of calcareous rock such as limestone or marble will trigger increased settlement by shellfish (Hidu et al., 1975; Soniat et al., 1991). Irregular extensions in both vertical and horizontal directions, making heaps and berms, will increase surface area and provide leesides for shelter. Narrowing down the rock grading will result in more crevices, and variation in rock size at different locations will increase habitat diversity, serving a wide range of rock-dwelling species. If considered early in the design process such changes can easily be incorporated to ecologically enhance marine infrastructure, often at marginal additional cost.
Principle III. Induce life – kickstart and steer community composition
Any new-built marine structure provides hard substrate habitat and is prone to be colonized by marine organisms (e.g. Komyakova et al., 2022). The development of the benthic community at a new structure can be guided into a desired direction, not only by optimizing the habitat conditions, but also by pro-actively bringing in targeted species. This so-called priming is essential when there is no connectivity between the new structure and a natural system hosting the preferred species (ter Hofstede et al., 2023), and is advisable when one desires to influence competition in favor of targeted species (McCook et al., 2001). Bringing in oyster or coral broodstock, for example, is a means to provide a local source of larvae to mitigate connectivity issues and increase the probability of settlement at the infrastructure. The installation of broodstock requires careful design and timing, taking into account species-specific life-history traits, to increase the likelihood of survival and long-term reproduction success.
Example using broodstock to kickstart and steer community composition
Introducing reef-building species can kickstart reef-development at remote locations that lack connectivity with natural reef systems. In the North Sea this is practiced via active introduction of flat oyster broodstock in offshore wind farms, with the aim to produce larvae that can settle locally and develop into thriving reefs (e.g. Didderen et al., 2019; Schutter et al., 2021; Figure 1III). The broodstock is fixed on tailor-made structures that increase survival rates under governing local environmental conditions, such as providing stability, offering access to nutrients and oxygen, and avoiding burial by sedimentation. Two types of structures can be used: liftable ones installed with a crane (Van Rie, 2020), and droppable ones side-casted (Siderius, 2022), both having their pro’s and con’s. Liftable broodstock structures are large and stable, provide maximal security for the oysters, and can be replaced to other locations after the reef development has been kickstarted. However, installation of liftable broodstock structures is an expensive operation due to the required equipment. Droppable broodstock structures can also designed to be stable and robust during deployment and operation, but are smaller in size, allowing more cost-effective manual installation. However, being smaller in size, they may provide less security against sedimentation and predation, and they cannot be easily re-used at other locations. Despite the con’s, the use of either broodstock structure is preferred over the distribution of lose mature oysters, as it protects the oysters from sedimentation and wash-out, resulting in a local high density of broodstock needed to ensure reproductive success.
Principle IV. Support self-sustainment – create the conditions
Natural recovery of an ecosystem is preferred over active restoration interventions (Abelson et al., 2015). If interventions are needed to initiate the recovery, one would ideally achieve a self-regulating and self-sustaining ecosystem without the need for future human intervention to further steer restoration outcomes (Palmer and Stewart, 2020). This aim for this so-called rewilding of a system (Perino et al., 2019) has both economic and ecological benefits. If the targeted system becomes self-sustaining, costly interventions are no longer needed. Self-sustainment also indicates good health, the ecosystem being able to maintain its structure and function over time in the face of external stressors (Costanza and Mageau, 1999; Tett et al., 2013). However, restoration efforts often remain far below reference conditions in terms of ecological metrics such as biodiversity, even after decades of maturing (Palmer and Stewart, 2020). To become self-sustainable, the natural interactions between biota and abiotic physical features should be restored within the system (Suding et al., 2015), and connectivity with remnant healthy ecosystems should be established (Mokany et al., 2020). Only then are the restoration efforts likely to result in truly self-sustaining ecosystems in which human interventions are no longer required.
Example creating self-sustaining reefs
An example of an intervention to establish self-sustainment, is the concept of installing vertical bivalve reefs in the water column, from which living and dead bivalves will drop, to stimulate biogenic reef formation at a soft sediment seafloor. That is, an agglomeration of shells at the seabed forms a complex matrix for settling juveniles and associated fauna, ensuring reef persistence over time (Mann and Powell, 2007; Schulte et al., 2009). This self-sustaining production concept has been designed for offshore wind farms in the North Sea, in which a vertical reef consisting of strings of blue mussels (Mytilus edulis) hanging in the water column produces a continuous supply of shell material to the seabed (Figure 1IV). It is based on structures used for commercial farming purposes, and comprises a longline anchored to the seabed, provided with vertical culture ropes at a depth suitable for mussel growth. Blue mussel larvae are abundant in the North Sea and known to attach rapidly to suitable substrates when offered (Coolen et al., 2018; Coolen et al., 2020). After installation of the structure, these larvae are expected to naturally settle on the culture ropes and grow into thriving mussel reefs with a rate of at least 5 cm shell length in the first 5 years (Bayne and Worrall, 1980). Once the mussels die or fall off, their shells are expected to sink and accumulate at the seabed, providing substrate suitable for reef development at the seafloor. By utilizing proven approaches from the mussel-aquaculture industry this design concept has a high likelihood of success at a large scale, and even a partial commercial setup seems conceivable.
Principle V. Ensure continuity – active stakeholder involvement beyond initiation
Timescales for ecological restoration rarely match the timescales of both marine construction and active restoration projects. Large marine infrastructure projects generally last for a couple of years, from design to completion. Activities and resources, such as equipment and people on site, peak during the construction phase. Once the construction works come to an end, the activities on site will fade. The same will typically hold for imposing active restoration measures, for which activities are also concentrated in a limited time frame. However, reaching long-term overarching restoration objectives may require more time than foreseen for the initiated measures, and the need for continuation of activities is probable (see Figure 1V). Also, restoration efforts should be monitored for a fair number of years (15-20), to allow for a solid evaluation whether recovery of the ecosystem and its associated functions and services has been reached (Bayraktarov et al., 2016; Abelson et al., 2020). Ensuring such continuation of restoration activities and their evaluation requires the involvement of local partners who are willing to take responsibility beyond the initiation phase. Ideally partners that have an intrinsic interest in the success of the restoration efforts should be already involved during the design phase, thus way before starting marine construction or active restoration. Early involvement is important to ensure that partners take ownership of the activities, and to ensure that sufficient resources to continue monitoring and maintenance after the work have been put in place. Involving industry partners in ecological restoration practices will generate momentum and scale that would otherwise be inconceivable, while close involvement of local stakeholders will ensure the long-term continuation of the activities.
Example stakeholder involvement beyond project boundaries
Involving local parties to ensure long-term continuity of restoration efforts initiated as part of a marine construction project, took place at the island New Providence, Bahamas in the years 2015-2017. Along with port upgrade works, a so-called ‘Coral Engine’ was developed to promote local reef rehabilitation (ter Hofstede et al., 2019). Alignment with the construction works allowed the restoration works to make use of essential logistical capacity on site. The Coral Engine comprised an in-situ coral nursery that was filled with hundreds of fragments of opportunity obtained from the field, and tens of thousands of sexual recruits reared in a mobile breeding facility (Van Koningsveld et al., 2017). Having these corals ‘in stock’ in the nursery, a continuous supply of genetically diverse corals for quick reef restoration is ensured, e.g. following hurricane disasters. Local NGO’s, government, and a recreational diving center, were involved at an early stage for the long-term operation of the Coral Engine, using its benefits for tourism, research, education, and local employment. Following the project development until today, the Coral Engine is continued by the local stakeholders and demonstrates that initiating reef restoration activities coupled to an infrastructural development project can provide a long-term contribution to a local natural and socio-economic system well beyond the traditional time-scale of construction projects.
Discussion: collaboration over conflict
The rapid decline of reef ecosystems requires a change of current restoration practices, and the development of novel approaches (Svejcar et al., 2022). Common key players to catalyze restoration actions are funding organizations, governmental bodies, scientists and citizens (Gann et al., 2019; Danovaro et al., 2021). The engagement of private companies, however, has recently been identified as critical in the implementation phase (Danovaro et al., 2021). Taking an interdisciplinary approach has been identified as a key feature in successful ecosystem restoration (Gann et al., 2019; Saunders et al., 2020). The five golden principles presented in this paper show how including the expertise of industry partners can promote effective marine reef restoration. It complements the science-based knowledge of the functioning of targeted species and their associated habitats, which is fundamental for restoration efforts to last long-term (Bayraktarov et al., 2016; Fraschetti et al., 2021). The synergy between science and industry requires a new way of thinking, acting and interacting (De Vriend and Van Koningsveld, 2012), as the incentives of both parties are fundamentally different. Exaggeratedly said, while ‘classic restoration ecologists’ aim for highest nature values within the margins of the foreseen ecosystem, ‘conventional civil engineers’ seek for solutions that minimize risks and costs. Also, negative past experiences have led to mutual mistrust, as green science has halted infrastructural development (Grorud-Colvert et al., 2021) and grey industry irreversible harmed pristine seascapes (Bugnot et al., 2021).
Both parties should set aside their differences and take a cooperative approach to find common ground in marine reef restoration. Win-win solutions should be embedded in the early phases of both restoration and infrastructural projects, to allow for upscaling restoration practices with maximum benefits against minimal costs, and to incorporate nature-benefitting features in the design of marine infrastructure (Pioch et al., 2018). Over the past decades, our general perception about what is acceptable for the marine environment has normalized towards it being degraded and in an artificial state (Strain et al., 2019). But we should refuse to adhere to this and join forces to turn the tide. If we now start by synergizing scientific insights and industry-based approaches, we can still reverse the degradation at the scale needed to regain healthy marine reef ecosystems for future generations.
Data availability statement
The original contributions presented in the study are included in the article. Further inquiries can be directed to the corresponding author.
Ethics statement
Written informed consent was obtained from the individual(s) for the publication of any identifiable images or data included in this article.
Author contributions
RH lead the writing of the manuscript. All authors contributed to the writing and the editing of the manuscript and approved its submission.
Funding
This work was supported by the Dutch Research Council NWO [grant number 17671 (North Sea ReVIFES)].
Conflict of interest
The authors declare that the research was conducted in the absence of any commercial or financial relationships that could be construed as a potential conflict of interest.
Publisher’s note
All claims expressed in this article are solely those of the authors and do not necessarily represent those of their affiliated organizations, or those of the publisher, the editors and the reviewers. Any product that may be evaluated in this article, or claim that may be made by its manufacturer, is not guaranteed or endorsed by the publisher.
References
Abelson A., Halpern B. S., Reed D. C., Orth R. J., Kendrick G. A., Beck M. W., et al. (2015). Upgrading marine ecosystem restoration using ecological-social concepts. Bioscience 66, 156–163. doi: 10.1093/biosci/biv171
Abelson A., Reed D. C., Edgar G. J., Smith C. S., Kendrick G. A., Orth R. J., et al. (2020). Challenges for restoration of coastal marine ecosystems in the anthropocene. Front. Mar. Sci. 7, 544105. doi: 10.3389/fmars.2020.544105
Airoldi L., Beck M. W., Firth L. B., Bugnot A. B., Steinberg P. D., Dafforn K. A. (2021). Emerging solutions to return nature to the urban ocean. Ann. Rev. Mar. Sci. 13, 445–477. doi: 10.1146/annurev-marine-032020-020015
Baine M. (2001). Artificial reefs: a review of their design, application, management and performance. Ocean Coast. Manage. 44, 241–259. doi: 10.1016/S0964-5691(01)00048-5
Bayne B., Worrall C. (1980). Growth and production of mussels mytilus edulis from two populations. Mar. Ecol. Prog. Ser. 3, 317–328. doi: 10.3354/meps003317
Bayraktarov E., Saunders M. I., Abdullah S., Mills M., Beher J., Possingham H. P., et al. (2016). The cost and feasibility of marine coastal restoration. Ecol. Appl. 26, 1055–1074. doi: 10.1890/15-1077
Beck M. W., Brumbaugh R. D., Airoldi L., Carranza A., Coen L. D., Crawford C., et al. (2011). Oyster reefs at risk and recommendations for conservation, restoration, and management. Bioscience 61, 107–116. doi: 10.1525/bio.2011.61.2.5
Bellwood D. R., Pratchett M. S., Morrison T. H., Gurney G. G., Hughes T. P., Álvarez-Romero J. G., et al. (2019). Coral reef conservation in the anthropocene: confronting spatial mismatches and prioritizing functions. Biol. Conserv. 236, 604–615. doi: 10.1016/j.biocon.2019.05.056
Bohnsack J. A., Sutherland D. L. (1985). Artificial reef research: a review with recommendations for future priorities. Bull. Mar. Sci. 37, 11–39.
Bugnot A. B., Mayer-Pinto M., Airoldi L., Heery E. C., Johnston E. L., Critchley L. P., et al. (2021). Current and projected global extent of marine built structures. Nat. Sustain. 4, 33–41. doi: 10.1038/s41893-020-00595-1
Coolen J. W. P., Boon A. R., Crooijmans R., van Pelt H., Kleissen F., Gerla D., et al. (2020). Marine stepping-stones: connectivity of mytilus edulis populations between offshore energy installations. Mol. Ecol. 29, 686–703. doi: 10.1111/mec.15364
Coolen J. W. P., van der Weide B. E., Cuperus J., Blomberg M., van Moorsel G., Faasse M., et al. (2018). Benthic biodiversity on old platforms, young wind farms and rocky reefs. ICES J. Mar. Sci. 77, 1250–1265. doi: 10.1093/icesjms/fsy092
Costanza R., Mageau M. (1999). What is a healthy ecosystem? Aquat. Ecol. 33, 105–115. doi: 10.1023/A:1009930313242
Dafforn K. A., Glasby T. M., Airoldi L., Rivero N. K., Mayer-Pinto M., Johnston E. L. (2015). Marine urbanisation: an ecological framework for designing multifunctional artificial structures. Front. Ecol. Environ. 13, 82–90. doi: 10.1890/140050
Danovaro R., Aronson J., Cimino R., Gambi C., Snelgrove P. V. R., Van Dover C. (2021). Marine ecosystem restoration in a changing ocean. Restor. Ecol. 29, e13432. doi: 10.1111/rec.13432
De Vriend H. J., Van Koningsveld M. (2012). Building with nature: thinking, acting and interacting differently (Dordrecht, The Netherlands: EcoShape).
Didderen K., Bergsma J. H., Kamermans P. (2019). Offshore flat oyster pilot luchterduinen wind farm. results campaign 2 and lessons learned (Culemborg, The Netherlands: Bureau Waardenburg), 19–184.
Doropoulos C., Elzinga J., Ter Hofstede R., Van Koningsveld M., Babcock R. C. (2018). Optimizing industrial-scale coral reef restoration: comparing harvesting wild coral spawn slicks and transplanting gravid adult colonies. Restor. Ecol. 27, 758–767. doi: 10.1111/rec.12918
Doropoulos C., Vons F., Elzinga J., Ter Hofstede R., Salee K., Van Koningsveld M., et al. (2019). Testing industrial-scale coral restoration techniques: harvesting and culturing wild coral-spawn slicks. Front. Mar. Sci. 6, 658. doi: 10.3389/fmars.2019.00658
Duarte C. M., Agusti S., Barbier E., Britten G. L., Castilla J. C., Gattuso J. P., et al. (2020). Rebuilding marine life. Nature 580, 39–51. doi: 10.1038/s41586-020-2146-7
Eddy T., Lam V. W. Y., Reygondeau G., Cisneros-Montemayor A. M., Greer K., Palomares M. L. D., et al. (2018). Global decline in capacity of coral reefs to provide ecosystem services. One Earth 4, 1278–1285. doi: 10.1016/j.oneear.2021.08.016
Fennell P. S., Davis S. J., Mohammed A. (2021). Decarbonizing cement production. Joule 5, 1301–1311. doi: 10.1016/j.joule.2021.04.011
Firth L. B., Airoldi L., Bulleri F., Challinor S., Chee S. Y., Evans A. J., et al. (2020). Greening of grey infrastructure should not be used as a Trojan horse to facilitate coastal development. J. Appl. Ecol. 57, 1762–1768. doi: 10.1111/1365-2664.13683
Firth L. B., Thompson R. C., Bohna K., Abbiati M., Airoldi L., Bouma T. J., et al. (2014). Between a rock and a hard place: environmental and engineering considerations when designing coastal defence structures. Coast. Eng. 87, 122–135. doi: 10.1016/j.coastaleng.2013.10.015
Fraschetti S., McOwen C., Papa L., Papadopoulou N., Bilan M., Boström C., et al. (2021). Where is more important than how in coastal and marine ecosystems restoration. Front. Mar. Sci. 8, 626843. doi: 10.3389/fmars.2021.626843
Gann G. D., McDonald T., Walder B., Aronson J., Nelson C. R., Johnson J., et al. (2019). International principles and standards for the practice of ecological restoration. Second edition. Restor. Ecol. 27, S1–S46. doi: 10.1111/rec.13035
Grorud-Colvert K., Sullivan-Stack J., Roberts C., Constant V., Horta e Costa B., Pike E., et al. (2021). The MPA guide: a framework to achieve global goals for the ocean. Science 373, 1–10. doi: 10.1126/science.abf0861
Guan D. W., Xie Y., Yao Z., Chiew Y. M., Zhang J., Zheng J. (2022). Local scour at offshore windfarm monopile foundations: a review. Water Sci. Eng. 15, 29–39. doi: 10.1016/j.wse.2021.12.006
Hidu H., Chapman S., Soule P. W. (1975). Cultchless setting of European oysters, ostrea edulis, using polished marble. Proc. Natl. Shellfish. Ass. 65, 13–14.
Higgins E., Metaxas A., Scheibling R. E. (2022). A systematic review of artificial reefs as platforms for coral reef research and conservation. PloS One 17, e0261964. doi: 10.1371/journal.pone.0261964
Hillier S. R., Sangha C. M., Plunkett B. A., Walden P. J. (1999). Long-term leaching of toxic trace metals from Portland cement concrete. Cem. Concr. Res. 29, 515–521. doi: 10.1016/S0008-8846(98)00200-2
Hughes T. P., Barnes M. L., Bellwood D. R., Cinner J. E., Cumming G. S., Jackson J. B. C., et al. (2017). Coral reefs in the anthropocene. Nature 546, 82–90. doi: 10.1038/nature22901
Komyakova V., Jaffrés J. B. D., Strain E. M. A., Cullen-Knox C., Fudge M., Langhamer O., et al. (2022). Conceptualisation of multiple impacts interacting in the marine environment using marine infrastructure as an example. Sci. Total Environ. 830, 154748. doi: 10.1016/j.scitotenv.2022.154748
Laboyrie P., Van Koningsveld M., Aarninkhof S., Van Parys M., Lee M., Jensen A. A. C., et al. (Eds.) (2018). Dredging for sustainable infrastructure (The Netherlands: CEDA).
Lapointe L., Bourget E. (1999). Influence of substratum heterogeneity scales and complexity on a temperate epibenthic marine community. Mar. Ecol. Prog. Ser. 189, 159–170. doi: 10.3354/meps189159
Mann R., Powell E. N. (2007). Why oyster restoration goals in the Chesapeake bay are not and probably cannot be achieved. J. Sea Res. 26, 905–917. doi: 10.2983/0730-8000(2007)26[905:WORGIT]2.0.CO;2
McCook L. J., Jompa J., Diaz-Pulido G. (2001). Competition between corals and algae on coral reefs: a review of evidence and mechanisms. Coral Reefs 19, 400–417. doi: 10.1007/s003380000129
Menz M. H., Dixon K. W., Hobbs R. J. (2013). Hurdles and opportunities for landscape-scale restoration. Science 339, 526–527. doi: 10.1126/science.1228334
Mokany K., Ferrier S., Harwood T. D., Ware C., Di Marco M., Grantham H. S., et al. (2020). Reconciling global priorities for conserving biodiversity habitat. Proc. Natl. Acad. Sci. U.S.A. 117, 9906–9911. doi: 10.1073/pnas.1918373117
Moschella P. S., Abbiati M., Åberg P., Airoldi L., Anderson J. M., Bacchiocchi F., et al. (2005). Low-crested coastal defence structures as artificial habitats for marine life: using ecological criteria in design. Coast. Eng. 52, 1053–1071. doi: 10.1016/j.coastaleng.2005.09.014
Palmer M. A., Stewart G. A. (2020). Ecosystem restoration is risky… but we can change that. One Earth 18, 661–664. doi: 10.1016/j.oneear.2020.11.019
Paxton A. B., Shertzer K. W., Bacheler N. M., Kellison G. T., Riley K. L., Taylor J. C. (2020). Meta-analysis reveals artificial reefs can be effective tools for fish community enhancement but are not one-Size-Fits-All. Front. Mar. Sci. 7, 282. doi: 10.3389/fmars.2020.00282
Perino A., Pereira H. M., Navarro L. M., Fernández N., Bullock J. M., Ceausu S., et al. (2019). Rewilding complex ecosystems. Science 364, eaav5570. doi: 10.1126/science.aav5570
Perkol-Finkel S., Sella I. (2014). “Ecologically active concrete for coastal and marine infrastructure: innovative matrices and designs,” in From Sea to shore – meeting the challenges of the Sea. Eds. Allops W., Burgess K. (London, United Kingdom: ICE Publishing), 1139–1149.
Pioch S., Relini G., Souche J. C., Stive M. J. F., De Monbrison D., Nassif S., et al. (2018). Enhancing eco-engineering of coastal infrastructure with eco-design: moving from mitigation to integration. Ecol. Eng. 120, 574–584. doi: 10.1016/j.ecoleng.2018.05.034
Potet M., Fabien A., Chaudemanche S., Sebaibi N., Guillet T., Gachelin S., et al. (2021). Which concrete substrate suits you? ostrea edulis larval preferences and implications for shellfish restoration in Europe. Ecol. Eng. 162, 106159. doi: 10.1016/j.ecoleng.2021.106159
Price M. R., Toonen R. J. (2017). Scaling up restoration efforts in the pacific islands: a call for clear management objectives, targeted research to minimize uncertainty, and innovative solutions to a wicked problem. Pac. Sci. 71, 391–399. doi: 10.2984/71.4.1
Rey Benayas J. M., Newton A. C., Diaz A., Bullock J. M. (2009). Enhancement of biodiversity and ecosystem services by ecological restoration: a meta-analysis. Science 325, 1121–1124. doi: 10.1126/science.1172460
Rinkevich B. (2008). Management of coral reefs: we have gone wrong when neglecting active reef restoration. Mar. pollut. Bull. 56, 1821–1824. doi: 10.1016/j.marpolbul.2008.08.014
Sala E., Mayorga J., Bradley D., Cabral R. B., Atwood T. B., Auber A., et al. (2021). Protecting the global ocean for biodiversity, food and climate. Nature 592, 397–402. doi: 10.1038/s41586-021-03371-z
Saunders M. I., Doropoulos C., Bayraktarov E., Babcock R. C., Gorman D., Eger A. M., et al. (2020). Bright spots in coastal marine ecosystem restoration. Curr. Biol. 30, 1500–1510. doi: 10.1016/j.cub.2020.10.056
Schulte D. M., Burk R. P., Lipcius R. N. (2009). Unprecedented restoration of a native oyster metapopulation. Science 325, 1124–1128. doi: 10.1126/science.1176516
Schutter M., Tonk L., Kamermans P., Kardinaal E., Ter Hofstede R. (2021). EcoScour project borssele – outplacement methods of European flat oysters (Culemborg, The Netherlands: Bureau Waardenburg), 21–328.
SER (2004). The SER international primer on ecological restoration (Tucson: Society for Ecological Restoration International). Available at: www.ser.org.
Siderius E. J. S. (2022). Droppable oyster broodstock structure – experimental study on optimal design for flat oyster broodstock structure, that can be installed at the scour protection of Dutch offshore wind farms, via dropping from a vessel (Delft, The Netherlands: Delft University of Technology).
Soniat T. M., Broadhurst R. C., Haywood E. L. (1991). Alternatives to clamshell as cultch for oysters, and the use of gypsum for the production of cultchless oysters. J. Shellfish Res. 10, 405–410. doi: 10.2983/0730-8000(2005)24[483:ACOTEO]2.0.CO;2
Strain E. M. A., Alexander K. A., Kienker S., Morris R., Jarvis R., Coleman R., et al. (2019). Urban blue: a global analysis of the factors shaping people’s perceptions of the marine environment and ecological engineering in harbours. Sci. Total Environ. 658, 1293–1305. doi: 10.1016/j.scitotenv.2018.12.285
Suding K. N. (2011). Toward an era of restoration in ecology: successes, failures, and opportunities ahead. Annu. Rev. Ecol. Evol. Syst. 42, 465–487. doi: 10.1146/annurev-ecolsys-102710-145115
Suding K. N., Higgs E., Palmer M. A., Callicott J. B., Anderson C. B., Baker M., et al. (2015). Committing to ecological restoration. Science 348, 638–640. doi: 10.1126/science.aaa4216
Svejcar L. N., Brown V. S., Ritchie A. L., Davies K. W., Svejcar T. J. (2022). A new perspective and approach to ecosystem restoration: a seed enhancement technology guide and case study. Restor. Ecol. 30, e13615. doi: 10.1111/rec.13615
ter Hofstede R., Driessen F. M. F., Elzinga P. J., Van Koningsveld M., Schutter M. (2022). Offshore wind farms contribute to epibenthic biodiversity in the north Sea. J. Sea Res. 185, 102229. doi: 10.1016/j.seares.2022.102229
ter Hofstede R., Elzinga J., Carr H., Van Koningsveld M. (2019). “The coral engine: the way for local communities to sustainably produce corals for reef rehabilitation at a large scale,” in Proceedings of 22nd World Dredging Congress and Exposition, WODCON 2019, Shanghai, China. 772–780.
ter Hofstede R., Williams G., Van Koningsveld M. (2023). The potential impact of human interventions at different scales in offshore wind farms to promote flat oyster (Ostrea edulis) reef development in the southern north Sea. Aquat. Living Resour. 36, 4. doi: 10.1051/alr/2023001
Tett P., Gowen R. J., Painting S. J., Elliott M., Forster R., Mills D. K., et al. (2013). Framework for understanding marine ecosystem health. Mar. Ecol. Prog. Ser. 494, 1–27. doi: 10.3354/meps10539
Van Koningsveld M., Ter Hofstede R., Elzinga J., Smolders T., Schutter M., Osinga R. (2017). ReefGuard: a scientific approach to active reef rehabilitation. Terra Aqua 147, 5–16.
Van Rie V. (2020). Oyster broodstock stuctures in offshore wind farms (Delft, The Netherlands: MSc-thesis report Delft University of Technology).
Vaughn K. J., Porensky L. M., Wilkerson M. L., Balachowski J., Peffer E., Riginos C., et al. (2010). Restoration ecology. Nat. Educ. Knowledge 3, 66.
Vivier B., Dauvin J. C., Navon M., Rusig A. M., Mussio I., Orvain F., et al. (2021). Marine artificial reefs, a meta-analysis of their design, objectives and effectiveness. Glob. Ecol. Conserv. 27, e01538. doi: 10.1016/j.gecco.2021.e01538
Keywords: reefs, upscaling, restoration, industry, infrastructure
Citation: ter Hofstede R, Bouma TJ and van Koningsveld M (2023) Five golden principles to advance marine reef restoration by linking science and industry. Front. Mar. Sci. 10:1143242. doi: 10.3389/fmars.2023.1143242
Received: 12 January 2023; Accepted: 02 May 2023;
Published: 17 May 2023.
Edited by:
Ronald Osinga, Wageningen University and Research, NetherlandsReviewed by:
Martina Milanese, Studio Associato Gaia snc, ItalyCarlo Cerrano, Marche Polytechnic University, Italy
Copyright © 2023 ter Hofstede, Bouma and van Koningsveld. This is an open-access article distributed under the terms of the Creative Commons Attribution License (CC BY). The use, distribution or reproduction in other forums is permitted, provided the original author(s) and the copyright owner(s) are credited and that the original publication in this journal is cited, in accordance with accepted academic practice. No use, distribution or reproduction is permitted which does not comply with these terms.
*Correspondence: Remment ter Hofstede, r.terhofstede@tudelft.nl