Characteristics and hazard risk of microplastics in Sinonovacula constricta: from farming to market
- 1Key Laboratory of Estuarine Ecological Security and Environmental Health, Education Department of Fujian, Tan Kah Kee College, Xiamen University, Zhangzhou, China
- 2Department of Environmental Science and Engineering, Tan Kah Kee College, Xiamen University, Zhangzhou, China
In this paper, I selected Sinonovacula constricta, a typical representative of commercial bivalve shellfish from Zhangzhou City, and found that microplastics were detected in all the samples with small sizes. The abundance in farm samples (3.65 n/g) was higher than in market samples (0.89 n/g), despite their smaller weight, which was mainly contributed by body fluid. Microplastics transported from the body fluid to the viscera and then metabolized into muscle were substantially reduced (69.3%), with only 0 or 1 microplastic observed in the muscles on the micron scale. The microplastics detected in the market samples were mainly concentrated in the viscera, accounting for 81%, while distributed in all the organs of farm samples, with body fluids accounting for 52%. A total of four shapes were detected, with the highest percentage of fibrous shape, while foam-like microplastics were not detected in the market samples. The abundance was not correlated with sample weight, but market samples showed a positive correlation. A total of seven polymers were detected, with the highest rate of polypropylene (PP) (27%). The polymer risk level reached level III. The percentage of polyvinyl chloride (PVC) in the market samples exceeded that of farms, which resulted in a higher hazard risk index despite their lower abundance. The cleaning process and excretory behavior, from farms to markets, greatly reduced microplastic contamination.
1 Introduction
Reports of plastic debris in the marine environment date back more than half a century (Carpenter & Smith, 1972). The concept of microplastics is defined as plastic debris less than 5 mm in size (Thompson et al., 2004), which is divided into primary and secondary microplastics. Primary microplastics are original particles that are manufactured by the plastic processing industry. Secondary microplastics are non-inheritable particles shaped by the physical, chemical, and biological effects within the surroundings during wear, fragmentation, and decomposition (Lehtiniemi et al., 2018). Microplastics are simply transported, unfold within the surroundings, and are inspected in water, soil, and air environments (Yu et al., 2020). Some plastics incorporate poisonous components, consisting of bisphenol A (BPA) and phthalates (GESAMP, 2019), which leach out from plastics, posing additional risks to marine organisms. In contrast, microplastics, due to their huge floor vicinity relative to their quantity, can adsorb numerous contaminants, along with heavy metals (Cole et al., 2011), persistent natural pollutants (POPs) (O’Donovan et al., 2020), and perfluorooctane sulfonate (PFOS) (Islam et al., 2021). Microplastics are known to affect the activity of marine organisms with those absorbed contaminants which could cause extra risks (Ziccardi et al., 2016). In a word, the pollution of microplastics is a global drawback; during a worldwide inspection, microplastics were found to be harmful to organisms and particularly have a potential impact on human health (Chen et al., 2020; Vo and Pham, 2021; Franceschini et al., 2021; Wang et al., 2022).
Previous studies have shown that microplastics can cause different levels of negative impacts on aquatic organisms. Chen et al. (2017) investigated the direct and indirect toxic effects of microplastics on the locomotor activity of zebrafish larvae and found that microplastics not only inhibited motility but also inhibited growth, development, and gene expression. In another experiment of microplastic exposure, Yang et al. (2020) found that microplastics accumulated in the digestive tract of juvenile crucian carp and damaged the intestine, such as the liver and gill tissues. Microplastics with small sizes could also cross the epidermis into muscle tissue, causing muscle tissue damage, thus reducing the activity and swimming speed of juvenile fish. The first study on microplastic extraction techniques from bivalve shellfish actually pointed it out (Claessens et al., 2013), and the first data on microplastic ingestion by bivalve mollusks were obtained in 2014 (Van Cauwenberghe & Janssen, 2014). Trends of the past literature indicate growing global concerns about microplastic contamination in bivalves and their associated risks of particular concern. Microplastics were detected in four popular bivalve species in Korea, with a mean concentration of 0.97 ± 0.74 n/a (number per individual) (Cho et al., 2019). Another study on microplastics in clams (Tegillarca granosa) was conducted in three completely different markets in Indonesia. The quantity of microplastics from the above markets was 9.87 ± 4.76, 20.93 ± 9.80, and 23.17 ± 13.02 n/a, severally (Suprayogi, 2018).
Since the characteristics of environmental microplastic pollution are variable, easily influenced by diverse factors such as wind, tides, and currents (Li et al., 2019; Chen, 2022), monitoring microplastics in bivalves is conducive to a more comprehensive assessment of the impact of microplastics on the environment and ecosystems (Cho et al., 2021). Bivalve shellfish have been identified as one of the groups most affected by microplastics due to their special characteristics, such as wide distribution, easy access, stationary life, and high tolerance to various environmental conditions (Ward et al., 2019). In addition to being used as indicator organisms for environmental pollution, bivalve shellfish are also important species for monitoring aquatic quality and safety (Qu et al., 2018). China is the main production and consumption region of bivalve shellfish worldwide because the annual production in China reached 153 million tons, accounting for almost 80% of the worldwide (FAO, 2020). According to the 2021 National Statistical Bulletin of Fishery Economy, aquaculture production was 15,456,700 tons in 2021, second only to fish. Bivalve shellfish, are the least mobile of the four major aquatic products fish, shrimp, crab, and shellfish; as filter feeders, they are among the species most likely to ingest microplastics (Wright et al., 2013; Germanov et al., 2018). They may be good bioindicators for microplastic monitoring and risk assessment of human exposure to microplastic ingestion through shellfish (Ward et al., 2019).
S. constricta belong to the Phylum Mollusca, Bivalvia. They are filter-feeding organisms and generally live in soft mud or sandy beaches, relying on their feet to dig burrows, which are vertically downward. When the burrows are flooded by seawater, the S. constricta rise to the mouth of the burrow to feed, taking in plankton through the inlet pipe and discharging metabolites through the outlet pipe (Chen et al., 2022). The study of microplastic characteristics of S. constricta can indirectly reflect the pollution level of their aquaculture environment and seafood security. Various plastic products used in fishery activities are left in the aquaculture environment by accident and abandonment. The total weight of plastic fishing gear entering the Pacific Ocean is more than 11,000 tons per year (Zhu et al., 2019). Nowadays, the four major commercial fish, shrimp, crab, and shellfish that people consume daily have been shown to have microplastic contamination. According to the latest report, a risk assessment study estimated that the global average intake of microplastics through shellfish consumption amounted to 751 particles/person/year (Ding et al., 2022). The potential hazards of microplastic contamination to aquatic products cannot be underestimated because of the large population in China, which has a strong consumption power. At present, there are limited reports on the microplastic characteristics of S. constricta, and there are few studies on the differences in the occurrence of microplastics between aquaculture farms and markets, especially comparing different organ distribution and hazard risk assessment between them. In this paper, I selected S. constricta, a typical representative of commercial bivalve shellfish in Zhangzhou, to investigate the microplastic contamination. Furthermore, I analyzed the microplastic fate and organ distribution characteristics in terms of size, abundance, shape, color, and polymer type to compare the microplastic fate characteristics between aquaculture farms and markets. Finally, I assessed the polymer hazard risk of microplastics in S. constricta from Zhangzhou. This paper can provide some data for the study of microplastic fugacity characteristics of bivalve shellfish and provide a basis for decision making on plastic control from aquaculture farms to markets, especially for seafood security.
2 Materials and methods
2.1 Study area and sample collection
About the study area, the S. constricta samples used in this study were collected from Zhangzhou Gan Wen aquaculture farm (shortened to farm) and Xin Hua Du supermarket (shortened to market). Gan Wen aquaculture farm is located in Mangrove Reserve at the estuary of Jiulong River in Longhai District, Zhangzhou City, Fujian Province, and is one of the major S. constricta aquaculture farms in Fujian Province. Xin Hua Du market is a nationally known supermarket, and the selected store is located in downtown Zhangzhou City, which is one of the main aquatic product procurement channels for local residents.
The S. constricta collected from the farms were dug from the mudflats on site, while the commercially available S. constricta were collected from the market seafood pools, which were randomly selected from the seafood pools at three different collection points. For farm samples, three locations were also randomly selected for collection from each farm. One kilogram was collected from each collection site, covered with aluminum foil, and then stored in a laboratory refrigerator at −18°C for further analysis. All the samples were collected from July to September 2021. The samples were divided into two groups: the first are those collected on-site from Gan Wen aquaculture farms (labeled as S1 to S4), and the second are those purchased from Xin Hua Du markets (labeled as S5 to S8). Body length, body width, and body height are measured in mm as the unit, and body weight and mass are measured in g as the unit. The unit “n” indicates the number of microplastics, and “a” indicates an individual of S. constricta.
2.2 Experimental methods
The overall procedure of the experiment was measure, rinse, anatomy, digestion, vacuum filtration separation, microscope, and fourier transform infrared (FTIR) identification (Figure 1). For the first step, the body fluids were obtained by rinsing with pure water during pretreatment, after which the length, width, and height of the samples were measured using vernier calipers. Second, the soft tissues were stripped from the shells and weighed using an experimental balance; biological indices were obtained by dissecting the S. constricta according to different organ tissues such as muscles and internal organs and then digesting them in a constant temperature shaker at 65°C using a 10% KOH solution as the digestion solution. Third, the digestion solution was then filtered using vacuum filtration. Finally, the obtained membranes were then observed under a stereomicroscope (Li et al., 2015). If the digestion was not complete, digestion was performed again. If the background interference of the filter membrane was high, density separation with saturated NaCl solution was required. The final filter membranes need to be dried in an incubator at 60°C for 24 h pending microscopic identification. It is important to note in particular that S. constricta were dissected by rapid freezing after the halo before dissection. Filters were observed under a MOTIC SMZ-171-TED stereo microscopy. The image particles were captured using a digital camera attached to the stereo microscope. The particles were counted and classified by type (fiber, fragment, or film) and color. The maximum length of microplastic was measured with Moticam Pro282B software. FTIR (Thermo Fisher Scientific, Nicolet iN10, USA) was used to identify the polymer components of microplastics. Matching with the database polymer spectrogram, more than 75% of the coincidence was determined as the corresponding polymer (Xiang et al., 2022).
2.3 Data analysis
The number of microplastics per individual was expressed as n/a, and the number of microplastics per gram wet weight was expressed as n/g. The abundance units of microplastics were standardized to facilitate comparison with other studies. Data analysis was processed by Microsoft Office 2020 and Origin 2022. A risk model was used to evaluate the potential hazards of microplastics in S. constricta. According to a report from Lithner et al. (2011), hazard scores of plastic polymers were used as indicators to assess the risk of microplastics (Table 1).
The evaluation model was described as follows: 1) H is the calculated polymer risk index due to microplastics. 2) Pn is the percentage of each microplastic polymer type detected in S. constricta samples. 3) Sn is the hazard score from which the polymer compound the microplastics contain. 4) The microplastic risk index (H) was calculated as follows.
2.4 Quality control
In order to avoid contamination throughout sampling and analysis, the subsequent procedures were strictly followed. Metal-like chrome steel spatulas or non-plastic instrumentation like wood were employed in the sampling and analysis steps. Researchers wore laboratory coats created entirely of natural fibers. All filter membranes, scalpels, samples, glasswork, and chrome steel extractors were wrapped in tin foil before use throughout the analysis method. All glass containers were totally cleansed with Milli-Q water and then sealed with tin foil to stop air exposure. All instruments and glasswork were properly washed before use. Microplastic assays are highly susceptible to interference from the experimental environment, and the possible presence of fine microplastics in the air and on the operating platform of the experimental environment can cause errors in the experimental results. To circumvent the influence of the experimental environment on microplastic assay results, effective quality control means, such as cleaning the somatic microscope area before sample analysis, need to be used during the experimental procedure (Li et al., 2015). All experiments were carried out in a fume hood. Three laboratory blank replicates (deionized water only) were processed for each sample set to measure air and chemical contamination, if applicable. An average of one fiber particle was detected. The microplastic of the blank samples was determined from the sample.
3 Results
3.1 Abundance and size distribution of microplastics
The biological indicators of samples are shown in Table 2.
Microplastics were detected in all S. constricta samples of Zhangzhou irrespective if from the farm or market. According to the experimental results, a total of 115 microplastics were identified, 74 of the 115 microplastics observed passed through the filter membrane with a pore size of 30 µm, and only 41 microplastics were retained (Table 3). In other words, 35.7% of the observed microplastics in Zhangzhou S. constricta samples were bigger than 30 µm, while the remaining 64.3% were sized between 30 and 1 µm. It was possible that nanoscale microplastics have passed through the filter membrane with a pore size of 1 µm. Overall, the microplastics observed in S. constricta samples from Zhangzhou were predominantly small particles.
As shown in Table 3, the overall abundance of microplastics in farmed samples was higher (22 ± 3 n/a) than that in markets (7 ± 2 n/a). The highest abundance of microplastics in farmed samples reached 25 n/a, while the highest abundance in market samples was only 9 n/a. If the influence of different biological indicators was considered, as shown in Table 1, the average weight of market samples was greater than that collected from farms. In general, a larger size of the S. constricta is more likely to be harvested for sale. Combining Tables 1 and 3, the weights of samples collected in farms were smaller; however, more microplastics were detected than market samples. The total number of microplastics identified in farmed samples was 88, accounting for 76.5% of the total, while market samples were 27, accounting for 23.5%.
In terms of size distribution, small-sized microplastics accounted for most of the samples, whether collected from markets or farm sites, with an overall percentage of 64.3%. This is similar to the research of other scholars (Li et al., 2015; Cho et al., 2019). Microplastics with smaller sizes are more likely to be ingested by S. constricta, which may be related to the high proportion of smaller-size microplastics in the environment and also to their biological behavior, especially their feeding habits. Further comparing the size characteristics between markets and farms, the percentage of small and medium size was higher in the samples collected from markets, with 85.7% of S5 and 88.9% of S7, reaching an average of 85.2%, while the average percentage of samples from farms was 57.9%.
3.2 Microplastics in different organs
From the experimental results, as shown in Table 4, a total of 37 microplastics were observed in the visceral organs of the farm samples, accounting for 42% of the total. Only 0 or 1 microplastic was observed in the muscles of farmed S. constricta, which may be either not detected or the low level of microplastics in the muscles, which may indicate that microplastics on the micron scale are basically not present during the metabolic growth. There were 46 microplastics observed in the body fluids of S. constricta from farms, accounting for 52% of the total amount.
In contrast, from the results of samples from markets, more than 70% of the observed microplastics came from visceral organs, including important organs of the digestive system such as the mouth, stomach, and intestine. No microplastics were detected in body fluids, one or two microplastics with small particle sizes (1–30 µm) were detected in muscle, and no microplastics with large particle sizes were detected.
The highest detection rate of microplastics in market samples was found in the visceral organs, with the proportion of microplastics in the scale of 1–30 µm reaching 78%–86%. Almost all microplastics detected at the scale of 30 µm or more were in the viscera. Overall, the microplastics detected in the market samples were mainly concentrated in the viscera, accounting for 81%. Microplastics were detected in the muscle, viscera, and body fluids of samples collected from aquaculture farms, with body fluids accounting for 52% and the viscera for 42%.
3.3 Shape and color characteristics of microplastics in S. constricta
A total of four shapes were detected in all samples, which were fibrous, foamy, fragmented, and granular. A total of ten kinds of colors were detected, namely, brown, purple, gray, blue, transparent, yellow, white, green, red, and black, with a large variety of colors, which may indicate a complex and diverse origin. As Figure 2 shows, in the photographs of microplastics detected in S. constricta samples under a stereomicroscope, there are blue fibrous microplastics and gray fragmented microplastics on 1-µm filter membrane, which are purple fibrous microplastics and white foam microplastics on 30-µm filter membrane, respectively.
3.3.1 Shape characteristics
All four shapes were detected in the aquaculture farm samples, only three kinds of shapes were detected in the market samples, and only two kinds of shapes were detected in Samples S6 and S8, which were fibrous and fragmented. A total of nine granular shapes were detected, accounting for the smallest percentage (8%), with a larger percentage being fibrous (44%) and foamy (31%). The proportion of fibrous shapes was higher in the market samples, reaching 74%. Among the samples from farms, the shape of foams was the highest, followed by fibrous, fragmented, and granular (Figure 2).
Further comparison of the morphological distribution characteristics of microplastics in different organs of markets and farm samples shows that (Figure 3), except for the body fluid part of the farm samples, the fibrous shape was the dominant shape, accounting for 44%, and fibrous microplastics were detected in both market and farm samples, regardless of muscle, internal organs or body fluid. In contrast, foam-like microplastics were not detected in the samples of S. constricta from markets. Both fragmented and granular microplastics were detected in samples from farms, but no foamy microplastics were detected in the muscle tissue. Compared with visceral and muscle tissues, foam-like microplastics were found in a large number of body fluids of samples from farms, accounting for up to 55%. In contrast, granular microplastics were rarely detected in body fluids, only 3%. Contrary to the poor distribution characteristics of other microplastic shapes in different organs, fragmented microplastics were more stable and were detected in all three tissues.
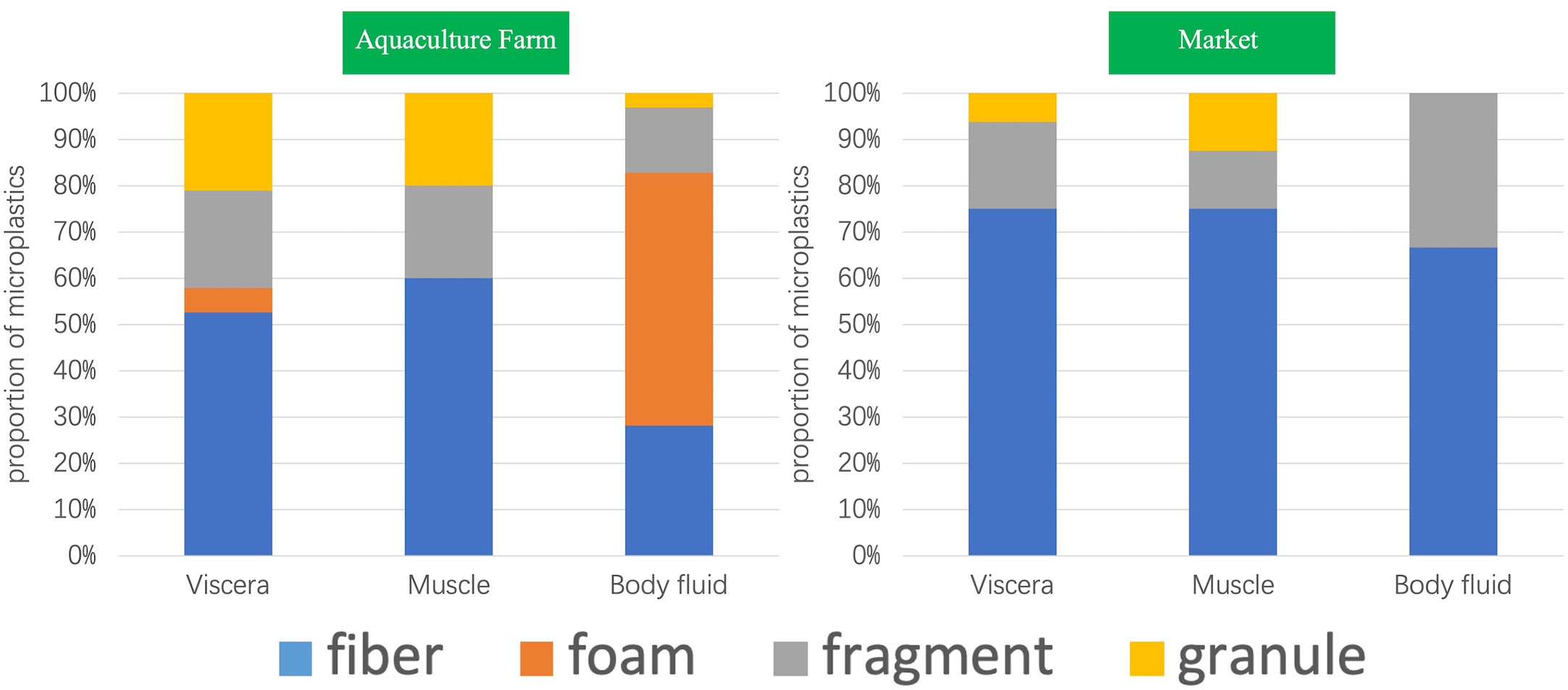
Figure 3 Distribution of microplastic shapes in different organs of Sinonovacula constricta between farm and market samples.
3.3.2 Color characteristics
Purple and gray microplastics accounted for a relatively large proportion of the S. constricta samples from Zhangzhou, accounting for 21% and 34%, respectively, more than half of all colors (Figure 4). Red and black microplastics accounted for the least amount (2%), which may be related to the color of the microplastics endowed in the environment and also to the feeding behavior of the S. constricta, which generally feed on microalgae such as diatoms and green algae, but there is no information that the S. constricta is selective in color intake. The color distribution of the S. constricta samples from the farms showed that the colors with a higher percentage were gray (44%), brown (15%), and purple (11%).
3.4 Polymer properties and distribution in different organs
FTIR identification results showed that numerous compound varieties were found within the samples (Figure 5), namely, polypropylene (PP), polystyrene (PS), polyethylene (PE), polyvinyl chloride (PVC), polyamide (PA), polycarbonate (PC), and polyethylene glycol terephthalate (PET). Overall, PP had the highest detection rate (27%), followed by PE (15%), PA and PET accounted for the same percentage (14%), and the lowest percentage was PC (8%).
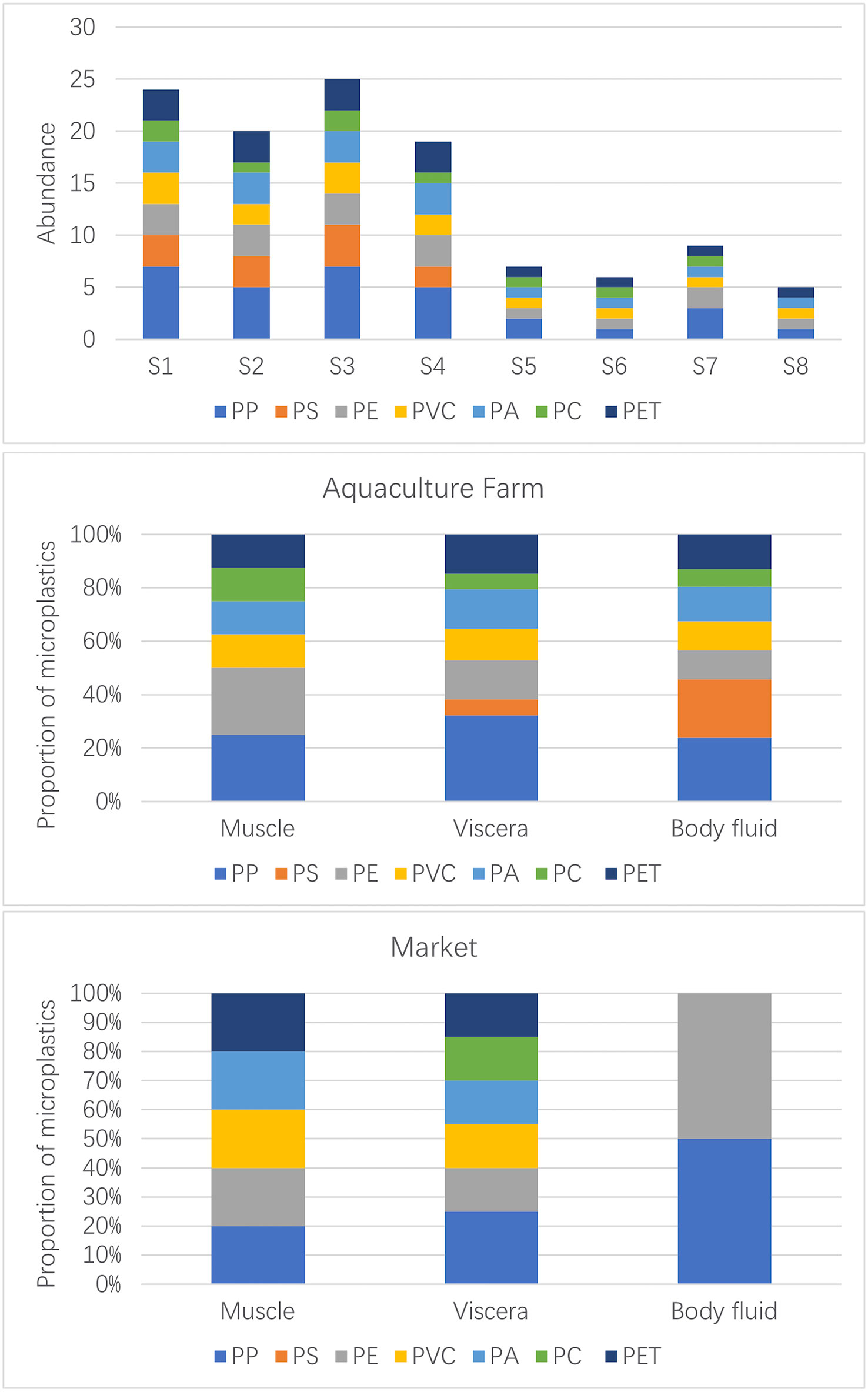
Figure 5 Distribution of different polymers in Sinonovacula constricta between farm and market samples.
From the distribution characteristics in different organs (Figure 5), PP was found in the viscera and body fluids of the S. constricta, while PS was apparently mainly distributed in the body fluids, with very few detected in the viscera and none in the muscles, which seems to make the S. constricta naturally “immune” to PS in a cultural environment where PS is widely present in the external environment. The other aggregation types were detected in one or two traces in the muscle, and the main distribution was concentrated in the viscera and body fluids, suggesting that microplastic contamination in the external environment can be ingested by S. constricta and enter the visceral tissues, including the digestive tract. Since the study did not further subdivide the visceral tissues into specific organs such as the intestine and liver, it is not possible to infer whether microplastic contamination from the digestive system would enter other systems. In contrast, in the market samples, PP was mainly found in the visceral organs, with trace amounts detected in body fluids and muscles. PS was not detected in any of the samples, and in contrast to the farmed S. constricta, it can be inferred that PS was in fact objectively present in the farmed environment and therefore was detected in the farmed viscera. It is possible that the PS was excreted when the S. constricta spit out sediment in the seafood pool. PS was detected in both farmed and market S. constricta samples. In the market samples, other polymer types were detected in the viscera, only one PE was detected in the body fluids, and no PC was detected in the muscle of the market samples.
4 Discussion
4.1 Abundance and size of microplastics
The number of microplastics in farm samples was over three times that in market samples. The reason may be that S. constricta from markets is washed and cleaned before being sold and placed in the seafood ponds. S. constricta may excrete some of the microplastics from their bodies when they breathe and feed in clear water. Further investigation found that the S. constricta sold in the Zhangzhou Xin Hua Du market were generally from local aquaculture farms. It is possible that some procedures from the farm to the market seafood pools have changed the microplastic content. For example, after harvesting from the farm, a large amount of attached soil needs to be cleaned off, and before being placed on market shelves, further cleaning is required before the seafood pool. Logistics such as transportation and storage may also affect microplastic abundance before and after. Van Cauwenberghe and Janssen (2014) observed that microplastic concentrations in mussels and oysters were reduced by approximately 30% after 3 days of decontamination. In contrast, microplastic contamination levels in bivalve shellfish mirror microplastic pollution levels in sediment and water around cultivation farms (Qu et al., 2018). In particular, farmed organisms like S. constricta, which live in mudflats for a long time, can reflect the level of microplastic contamination in their habitat. Overall, from the perspective of aquatic product quality and safety, it can be seen that the abundance of microplastics in S. constricta of Zhangzhou decreased significantly (69.3%) from farms to markets.
In terms of size distribution, smaller microplastics were more likely to be ingested into the digestive tract and other internal organs. The samples collected at the farm site had a higher detection rate because microplastics from the environment were more likely to be attached to the outside. Several stages of cleaning from the farm to the market seafood pools may have removed the larger-size microplastics attached to the outside, resulting in a higher relative proportion of smaller-size microplastics. Overall, the high proportion of smaller microplastics predicts a higher proportion of small-sized microplastics in the S. constricta aquaculture environment. In addition to primary microplastics such as cosmetics and other sources of smaller-size microplastic particles, the longer the plastic is present in the environment, the more likely it is to break down into smaller-size microplastics. The detection of smaller-size microplastics in all samples may indicate the long-term occurrence of microplastics in the farming environment.
4.2 Microplastics in organs and the biometric correlation
S. constricta samples in markets were more mature with bigger sizes, and the detection rate of the viscera was higher. The absence of microplastics in body fluid observation may be related to the cleaning in logistics or the selling process. S. constricta for sale in the market seafood ponds will generally spit out some sediments and may also release some microplastics. Farmed S. constricta samples were collected in the loose mudflats at the junction of salt and fresh water in the Jiulong River estuary. The S. constricta dig burrows, and when the burrows are flooded with water, they will feed, mainly on diatoms and other algae. When feeding, water enters the cavity of the S. constricta through the inlet pipe, and then the water is respired and filtered by the gills before being discharged from the body through the outlet pipe. During this process, the gills filter the food out of the water, which is then passed into the stomach for digestion. Microplastics with small sizes in aquaculture farms may be accidentally ingested during the above process, and they may become difficult to digest and remain temporarily in the digestive tract. The above experimental results also suggest that the feeding behavior of S. constricta may be related to the high percentage of microplastics in the gut. It has been suggested that microplastic contamination in organisms may be influenced by their own feeding behavior in addition to the surrounding environment, which may affect the distribution characteristics in vivo (Thammatorn and Palić, 2022; Deng et al., 2023). In contrast, microplastics in cultured S. constricta samples were mainly distributed in the internal organs and body fluids. In terms of size distribution characteristics, larger sizes (over 30 µm) accounted for a higher percentage of body fluids. Microplastics with smaller particle sizes were more easily ingested and accounted for a higher percentage compared to larger sizes. The microplastics found in body fluids should be from the environment attached to the body surface and muscle surface, while microplastics in the muscle may be staining the inner wall of the coated membrane or embedded in the muscle. Microplastics detected in muscle were only found in samples with small particle sizes (1 µm), and only one microplastic with a large particle size was found in Sample S3. More microplastics were detected in body fluids of cultured samples than muscle because the muscle is wrapped by the shell; usually, only a part of the muscle will be exposed while the shell is fully exposed to the environment; the exposed area is larger than the muscle, and there may be more microplastics attached.
There were significant differences in body fluid samples between farms and markets. It should be noted that the proportion of microplastics is a relative value. Almost half of the microplastics were basically removed after cleaning from the farms, and the S. constricta sold in markets also came from farms. Regardless of the farm or market samples, the microplastics detected in the muscle were minimal, and the percentage of visceral microplastics was still relatively high. Unlike fish, whose main eating part is the muscle, S. constricta can be eaten whole without removing the internal organs, including the digestive system, and microplastics in the internal tissues may be a major potential health risk factor.
One study reported the microplastic contamination and characteristics of bivalve shellfish collected from coastal areas of Yantai, China, demonstrating a strong correlation between microplastic abundance and mollusk biometric parameters (shell length, shell height, and soft tissue weight). It was concluded that buried bivalves with long growth periods and high weights contained large amounts of microplastics (Cho et al., 2019). However, the experimental results in this paper showed that there was no correlation between individual microplastic abundance (n/a) and sample weight (g), regardless if based on the individual abundance of microplastics or abundance per unit mass. It is speculated that this may be due to the fact that the sample contained two collection sources, the farm site and the market seafood pond, where the presence of microplastics on the body surface from different environments had a greater effect on the overall abundance content. It may also be because of the small number of samples, which is insufficient to support correlation analysis. However, when viewed separately, it can be seen that microplastic abundance in the farm (within the orange dashed circle in Figure 6) samples did not correlate with weight, while market samples showed a positive correlation between microplastic abundance and weight (shown by the dashed green straight line in Figure 6). Future studies can increase the number of samples and subdivide different organ weights as microplastic abundance correlation factors to improve the accuracy and objectivity of the overall study.
4.3 Interfering sources of microplastic shape and color
The large proportion of fibrous microplastics may be related to its source of contamination in the environment of aquaculture farms. Studies have shown that fiber shedding occurs in the washing process of various fibrous textiles (Stark, 2022). Browne et al. (2011) made a statistical result of the dropped fibers produced by PET fabric after washing: 1,900 dropped fibers are produced by each garment. Another source of fibrous microplastics may also be fishing gears used in fishery and aquaculture activities, such as fishing nets, fishing lines, and ropes. These fishing gears can be discarded or lost for various reasons, and statistics indicate that discarded fishing nets can account for at least 46% of the total weight of Pacific Ocean trash and that more than 11,000 tons of nets may be discarded each year (Zhu et al., 2019). At the same time, the fishing gear may also shed fibers due to friction, wear, and pulling during use. In summary, the overall shape characteristics of the S. constricta samples predicted that their microplastic sources may be mainly secondary microplastics because their shapes are not regular microplastic particles or small round balls. It is speculated that the microplastics may come from the aquaculture environment, plastic products used in aquaculture activities, and surrounding sewage discharge.
The results of the highest proportion of fibers in S. constricta samples from Zhangzhou are consistent with the research results of some scholars. It was found that fibrous microplastics accounted for 95.4% of the total microplastics in more than 10 species collected from Xiangshan Bay, including large yellow crocea, short-spined silver perch, goby, and oyster (Yu et al., 2022). The reason for the large proportion of fibrous microplastics in organisms may be that fibrous microplastics, due to their slender, elongated shape, are more easily broken down and torn into smaller fibers by physical and chemical effects in the environment when compared to fragmented and granular microplastics. The fine fibers are difficult to be detected when suspended in the water environment, and they are very easy to be eaten by aquatic organisms by accident during feeding. Such fine fibers are easily attached to the gills and digestive tract of aquatic organisms and are difficult to be discharged from the body, resulting in their accumulation in the organism.
Microplastics need to pass from the environmental medium to the body from outside to inside through body fluids and internal tissues such as the digestive tract and finally may be metabolized into the muscle. The high percentage of foamy microplastics in body fluids traceable in the viscera, but not in the muscle, may indicate that the S. constricta have a natural feeding screening or excretion function for foamy microplastics. In contrast, market samples had a more uniform distribution of fibrous microplastics in different organ tissues, which may indicate that fibrous forms are more likely to enter different organs in S. constricta from the environment. This is the same as the conclusion from the study of microplastics in oysters from Georgia estuaries that microfibrils and microfragments were considered more difficult to excrete in filter feeders (Keisling et al., 2020; Ding et al., 2022).
At the same time, the widespread and high proportion of fibrous forms of microplastics detected in different organs may also indicate that the washing in the distribution and sales chain does not thoroughly remove microplastics of this shape, or it may be that fibrous microplastics endowed in S. constricta are not easily excreted in the seafood pool. In contrast, the granular form was higher in the muscles of market S. constricta samples than in the guts, probably because granular microplastics are more easily excreted from the digestive organs. Foamy microplastics were not detected in any of the three organ tissues of the market samples, with reference to the samples collected at the farm. In addition to the above reasons, foamy microplastics are lighter and more likely to float on the surface of the seafood pool, easily removed in the process of distribution and sales chain cleaning.
S. constricta samples collected directly from the farm basically reflect the environmental conditions of the culture more realistically. Two typical patterns of S. constricta culture can be observed at the farm in Gan Wen Farm, Longhai District, Zhangzhou, one of which is the deployment of purple plastic nets at the bottom of the mudflats to facilitate harvesting on a large scale during the harvesting season, which may account for the prevalence of purple microplastics detected. The high percentage of gray microplastics in S. constricta samples from farms may be due to the color of the plastic itself, a large amount of discarded foamy gray plastic from farms may be its source, or the white or transparent microplastics may have been affected by environmental factors such as UV light, changing the original color. In contrast, the color distribution characteristics of market constrictor samples are somewhat different from those of the farms, with the market samples accounting for a higher percentage of the color purple (52%). As mentioned above, the heavy use of purple ground nets during the harvest season may be their main source. Red microplastics were only detected in market samples, presumably not from farms but probably introduced during the distribution or sales process. Market samples were dominated by purple microplastics, while farms were dominated by gray. In addition, the color of the farm and market samples was relatively consistent.
4.4 Polymers and their risk evaluation
The excessive percent of PE and PP is common because those polymers are usually found in aquaculture environments, which may be a probable purpose for the abundance of those compound varieties in S. constricta as they will be filter-feeding species (Cole et al., 2011; Lambert and Wagner, 2016). Plastic materials of PE, PP, PET, and PA are broadly used in fisheries and aquaculture, including plastic ropes, nets, and tubes (Laskar & Kumar, 2019). These plastic merchandises are crucial for bivalve aquaculture in nearshore waters through rafting or backside seeding. Plastic products utilized in aquaculture regions produce huge quantities of microplastics after prolonged exposure to sunlight radiation and mechanical wear (Song et al., 2021). PET is a vital fibrous chemical compound element within the textile business and is often found in microplastic samples from marine accumulation. The supply of PET is expected to come from the decomposition of clothing and carpets that square measure discharged into the marine setting with sewerage (Dalla Fontana et al., 2020). The above results suggest that environmental pollution and polymer composition may influence the microplastic contamination of bivalves. More types of polymers were detected in farm samples, and no PS was detected in market samples, which may be due to the fact that PS floats easily in water and is easily removed by washing during distribution and sales. The highest percentage of farm samples was PP, and the lowest was PC, which is the same as the results detected in market samples. Overall, the microplastics in the farmed S. constricta samples were more diverse and numerous. The cleaning and natural sedimentation behavior of S. constricta in all stages of distribution and sale, from the farm to market seafood ponds, greatly reduces the level of microplastic contamination.
Analysis of the organ distribution characteristics shows that the viscera and body surface are the main microplastic-bearing tissues; in the muscle, microplastics are rarely present; in the body surface, microplastic can be removed by washing; the viscera is the main part that ensures the quality and safety of fish products. Unlike most fish, S. constricta is generally consumed without removing the internal organs, which may pose a potential human health risk (Figure 7). Microplastics are shown to possess harmful effects on the growth, feeding, development, and longevity of various organisms (Mazurais et al., 2015; Chae and An, 2020). A study in which European perch were placed in cultures containing polyvinyl chloride particles found that polyvinyl chloride caused inflammation in the intestine of European perch, especially after exposure experiments lasting 90 days. European perch showed severe degradation of intestinal function, with some intestinal functions completely impaired (Pedà et al., 2016). In addition, the intake of microplastics will cause abrasions and ulcers in the organism, blocking the digestive tract and leading to early satiety, hunger, and degeneration (Wright et al., 2013; Koraltan et al., 2022; Kalčíková, 2023). Wang et al. (2021) found that microplastics caused metabolic disorders in bivalves such as oysters, philippine clams, and ctenophore scallops after microplastic exposure experiments, which damaged the stomach and digestive glands of the shellfish, reducing feeding and respiration rates. Therefore, this might result in reduced feeding ability and procreative diversifications, which can lead to reduced rejection of predators and ultimately mortality (Haegerbaeumer et al., 2019). For mussels, enhanced concentrations of microplastics (PE, PS, and PVC) will cut back respiration rates, filtration, and attachment strength or increase mortality (Browne et al., 2008; Hoellein et al., 2021).
Some studies have calculated the annual dietary intake of microplastics by humans through the consumption of bivalve shellfish (Van Cauwenberghe and Janssen, 2014; Barboza et al., 2018; Cho et al., 2019). However, the danger displayed by microplastics in seafood to human health continues to be mostly unknown (Barboza et al., 2018). Of all the polymers detected in seafood, PU, PAN, and PVC are the top three polymers with the highest hazard scores, mainly because the chemical monomers contained in these polymers are thought to cause serious health effects and even cancer (Lithner et al., 2011). Consistent with the analysis of scholars, the chemical compound polymer hazard index (PHI) and risk level criteria of microplastic pollution square measure are shown in Table 5 (Xu et al., 2018).
In this paper, the potential risk of microplastics in Zhangzhou S. constricta was assessed based on the polymer type and its hazard score (Lithner et al., 2011) as a microplastic risk indicator. The results showed that the hazard risk level of microplastics in Zhangzhou S. constricta all reached level III and did not reach level IV (Figure 8). Overall hazard risk H value is 669, of which market hazard risk value H is 819, exceeding the H value of 623 for farmed S. constricta. The potential risk level of microplastics to human health in Zhangzhou S. constricta is higher than the maximum value of hazard class II (100) but lower than the minimum value of hazard class IV (1000). However, the direct connection between the real risk and the risk index figures remains subject to additional research projects and discussion. Given the broad consistency of the microplastic fugacity characteristics and effluent levels within the Zhangzhou S. constricta according here with the global shellfish microplastic contamination characteristics, it is necessary to discuss the danger to human health exhibited by microplastics. Although there is proof that the potential human health risk is going to be reduced once the variety of meat is removed, the consumption habits of S. constricta might increase the potential risk.
It is noteworthy that market samples had the lowest abundance of microplastics, with an average of 0.89 n/g, while farmed samples had an average abundance of 3.65 n/g; yet the polymer hazard risk index of the market samples exceeded that of farmed S. constricta (819 > 623). The reason for this may be related to the difference in the type and percentage of polymers in markets and cultured S. constricta. PVC had the highest hazard index for the polymers found in the Zhangzhou S. constricta samples, while the percentage of PVC in the market samples exceeded that of the cultured, which resulted in an overall higher hazard index for markets. Since this study solely provided a really limited potential hazard index for a restricted variety of polymers, and therefore the potential hazard index was calculated supported monomers, the potential human health risks measurable during this study are also biased to some extent. Although the danger of ingesting microplastics is nonetheless great, it is necessary to notice that our analysis does not target actual knowledge but rather provides a preliminary discussion for any risk assessment of all bivalve shellfish. Improved assessment models and extra chemical compound hazard index knowledge are required to value the potential risk to human health from microplastics in seafood more accurately.
5 Conclusion
Microplastics were detected in all Zhangzhou S. constricta samples, which may be the main pathway of microplastic ingestion by aquatic products. The abundance of microplastics in farmed S. constricta (3.65 n/g) was higher than in market samples (0.89 n/g), and microplastics detected in farmed S. constricta were more than four times higher than in market samples despite their smaller weight, which was mainly influenced by the body fluid fugacity. In terms of physical properties, the microplastics observed in Zhangzhou S. constricta samples were mainly small in size, which may be related to the high proportion of small- and medium-size microplastics in the farming environment. Although S. constricta can ingest microplastics, their transport from the body surface to the viscera and then metabolism into muscle tissue is substantially reduced (69.3%). The high variety of colors detected may indicate a complex and diverse origin. A total of four shapes were detected, with the highest percentage of fibrous shape. In contrast, foam-like microplastics were not detected in the market samples. This may be due to the fact that foam is easily removed during the logistics and sales cleaning process. The overall shape characteristics of the S. constricta samples suggest that the source of microplastics may be mainly secondary microplastics. The detection of smaller-size microplastics in all samples may indicate the long-term fugacity of microplastics in the culture environment.
In terms of chemical properties and risk of harm, a total of seven polymers were detected, with the highest detection rate of PP and PS mainly in body fluids, very little in internal organs, and not detected in muscle, and it seems that S. constricta is naturally “immune” to PS in a farming environment where PS is widely present in the external environment. The overall microplastic abundance of S. constricta in Zhangzhou was not correlated with sample weight, while market samples showed a positive correlation between abundance and weight. In terms of polymer hazard, the risk level reached level III. PVC had the highest hazard index among the polymers found in the Zhangzhou S. constricta samples, while the percentage of PVC in the market samples exceeded that of the cultured S. constricta, which resulted in a higher hazard risk index in the market samples despite their lower abundance. Although the danger of ingesting microplastics is not well-known, our analysis does not target precise knowledge but rather provides a preliminary discussion for additional risk assessment of S. constricta and all bivalve shellfish. S. constricta are popular seafood, and unlike fish whose primary eating site is the muscle, S. constricta can be consumed whole without the removal of internal organs, including the digestive system, and microplastics in the internal tissues may be a major potential health risk impact factor.
Data availability statement
The original contributions presented in the study are included in the article/supplementary material. Further inquiries can be directed to the corresponding author.
Ethics statement
Ethical review and approval was not required for the study on animals in accordance with the local legislation and institutional requirements.
Author contributions
The author confirms being the sole contributor of this work and has approved it for publication.
Funding
This research was supported by the Natural Science Foundation of Zhangzhou City of China (ZZ2021J34), the Natural Science Foundation of Xiamen City of China (3502Z20227322), and the Education Research Project for Youth and Middle-aged Teachers of Fujian Province (JAT210632).
Conflict of interest
The authors declares that the research was conducted in the absence of any commercial or financial relationships that could be construed as a potential conflict of interest.
Publisher’s note
All claims expressed in this article are solely those of the authors and do not necessarily represent those of their affiliated organizations, or those of the publisher, the editors and the reviewers. Any product that may be evaluated in this article, or claim that may be made by its manufacturer, is not guaranteed or endorsed by the publisher.
References
Barboza L. G. A., Dick Vethaak A., Lavorante B. R. B. O., Lundebye A.-K., Guilhermino L. (2018). Marine microplastic debris: An emerging issue for food security, food safety and human health. Mar. Pollut. Bull. 133, 336–348. doi: 10.1016/j.marpolbul.2018.05.047
Browne M. A., Crump P., Niven S. J., Teuten E., Tonkin A., Galloway T., et al. (2011). Accumulation of microplastic on shorelines woldwide: sources and sinks. Environ. Sci. Technology. 45 (21), 9175–9179.
Browne M. A., Dissanayake A., Galloway T. S., Lowe D. M., Thompson R. C. (2008). Ingested microscopic plastic translocates to the circulatory system of the mussel, mytilus edulis (L.). Environ. Sci. Technology. 42 (13), 5026–5031.
Carpenter E. J., Smith K. L. Jr. (1972). Plastics on the Sargasso Sea surface. Science. 175 (4027), 1240–1241.
Chae Y., An Y. J. (2020). Effects of food presence on microplastic ingestion and egestion in mytilus galloprovincialis. Chemosphere. 240, 124855.
Chen B. (2022). Current status and trends of research on microplastic fugacity characteristics and pollution levels in mangrove wetlands. Front. Environ. Sci. 10.
Chen Y., Du X., Dong Z., Li Y., Niu D. (2022). Heritability estimation of high salt tolerance in razor clam (Sinonovacula constricta). Aquaculture. 559, 738423.
Chen B., Fan Y., Huang W., Rayhan A. B. M. S., Chen K., Cai M. (2020). Observation of microplastics in mariculture water of longjiao bay, southeast China: influence by human activities. Mar. pollut. Bulletin. 160, 111655.
Chen Q., Yin D., Jia Y., Schiwy S., Legradi J., Yang S., et al. (2017). Enhanced uptake of BPA in the presence of nanoplastics can lead to neurotoxic effects in adult zebrafish. Sci. Total Environment. 609, 1312–1321.
Cho Y., Shim W. J., Jang M., Han G. M., Hong S. H. (2019). Abundance and characteristics of microplastics in market bivalves from south Korea. Environ. Pollution. 245, 1107–1116.
Cho Y., Shim W. J., Jang M., Han G. M., Hong S. H. (2021). Nationwide monitoring of microplastics in bivalves from the coastal environment of Korea. Environ. Pollution. 270, 116175.
Claessens M., Van Cauwenberghe L., Vandegehuchte M. B., Janssen C. R. (2013). New techniques for the detection of microplastics in sediments and field collected organisms. Mar. pollut. Bulletin. 70 (1–2), 227–233.
Cole M., Lindeque P., Halsband C., Galloway T. S. (2011). Microplastics as contaminants in the marine environment: a review. Mar. pollut. Bulletin. 62 (12), 2588–2597.
Dalla Fontana G., Mossotti R., Montarsolo A. (2020). Assessment of microplastics release from polyester fabrics: the impact of different washing conditions. Environ. Pollution. 264, 113960.
Deng Y., Wu J., Chen J., Kang K. (2023). Overview of microplastic pollution and its influence on the health of organisms. J. Environ. Sci. Health Part A 58 (4), 412–422.
Ding J., Sun Y., He C., Li J., Li F. (2022). Towards risk assessments of microplastics in bivalve mollusks globally. J. Mar. Sci. Engineering. 10 (2), 288.
FAO (2020). FAOHome. Available at: https://www.fao.org/ (Accessed May 2, 2023).
Franceschini S., Cau A., D’Andrea L., Follesa M. C., Russo T. (2021). Eating near the dump: identification of nearby plastic hotspot as a proxy for potential microplastic contamination in the Norwegian lobster (Nephrops norvegicus). Front. Mar. Sci. 8.
Germanov E. S., Marshall A. D., Bejder L., Fossi M. C., Loneragan N. R. (2018). Microplastics: no small problem for filter-feeding megafauna. Trends Ecol. Evolution. 33 (4), 227–232.
GESAMP (2019). GESAMP. Available at: http://www.gesamp.org/ (Accessed May 2, 2023).
Haegerbaeumer A., Mueller M.-T., Fueser H., Traunspurger W. (2019). Impacts of micro- and nano-sized plastic particles on benthic invertebrates: A literature review and gap analysis. Front. Environ. Sci. 7. doi: 10.3389/fenvs.2019.00017
Hoellein T., Rovegno C., Uhrin A. V., Johnson E., Herring C. (2021). Microplastics in invasive freshwater mussels (Dreissena sp.): spatiotemporal variation and occurrence with chemical contaminants. Front. Mar. Sci. 8.
Islam N., Garcia da Fonseca T., Vilke J., Gonçalves J. M., Pedro P., Keiter S., et al. (2021). Perfluorooctane sulfonic acid (PFOS) adsorbed to polyethylene microplastics: accumulation and ecotoxicological effects in the clam scrobicularia plana. Mar. Environ. Res. 164, 105249.
Kalčíková G. (2023). Beyond ingestion: adhesion of microplastics to aquatic organisms. Aquat. Toxicology. 258, 106480.
Keisling C., Harris R. D., Blaze J., Coffin J., Byers J. E. (2020). Low concentrations and low spatial variability of marine microplastics in oysters (Crassostrea virginica) in a rural Georgia estuary. Mar. pollut. Bulletin. 150, 110672.
Koraltan İ, Mavruk S., Güven O. (2022). Effect of biological and environmental factors on microplastic ingestion of commercial fish species. Chemosphere. 303, 135101.
Lambert S., Wagner M. (2016). Exploring the effects of microplastics in freshwater environments. Integrated Environ. Assess. Management. 12 (2), 404–405.
Laskar N., Kumar U. (2019). Plastics and microplastics: a threat to environment. Environ. Technol. Innovation. 14, 100352.
Lehtiniemi M., Hartikainen S., Näkki P., Engström-Öst J., Koistinen A., Setälä O. (2018). Size matters more than shape: ingestion of primary and secondary microplastics by small predators. Food Webs. 17, e00097.
Li J., Lusher A. L., Rotchell J. M., Deudero S., Turra A., Bråte I. L. N., et al. (2019). Using mussel as a global bioindicator of coastal microplastic pollution. Environ. Pollution. 244, 522–533.
Li J., Yang D., Li L., Jabeen K., Shi H. (2015). Microplastics in commercial bivalves from China. Environ. Pollution. 207, 190–195.
Lithner D., Larsson Å, Dave G. (2011). Environmental and health hazard ranking and assessment of plastic polymers based on chemical composition. Sci. Total Environment. 409 (18), 3309–3324.
Mazurais D., Ernande B., Quazuguel P., Severe A., Huelvan C., Madec L., et al. (2015). Evaluation of the impact of polyethylene microbeads ingestion in European sea bass (Dicentrarchus labrax) larvae. Mar. Environ. Res. 112, 78–85.
O’Donovan S., Mestre N. C., Abel S., Fonseca T. G., Carteny C. C., Willems T., et al. (2020). Effects of the UV filter, oxybenzone, adsorbed to microplastics in the clam scrobicularia plana. Sci. Total Environment. 733, 139102.
Pedà C., Caccamo L., Fossi M. C., Gai F., Andaloro F., Genovese L., et al. (2016). Intestinal alterations in European sea bass dicentrarchus labrax (Linnaeus 1758) exposed to microplastics: preliminary results. Environ. Pollution. 212, 251–256.
Qu X., Su L., Li H., Liang M., Shi H. (2018). Assessing the relationship between the abundance and properties of microplastics in water and in mussels. Sci. Total Environment. 621 (2018), 679–686.
Song X., Wu X., Song X., Shi C., Zhang Z. (2021). Sorption and desorption of petroleum hydrocarbons on biodegradable and nondegradable microplastics. Chemosphere. 273, 128553.
Stark M. (2022). Comment on “Characterization of nanoplastics, fibrils, and microplastics released during washing and abrasion of polyester textiles.” Environ. Sci. Technol. 56 (14), 10543–10544.
Suprayogi (2018). Case study of microplastics on blood cockle from three traditional markets in semarang (Indonesia: Soegijapranata Catholic University).
Thammatorn W., Palić D. (2022). Potential risks of microplastic fomites to aquatic organisms with special emphasis on polyethylene-Microplastic-Glyphosate exposure case in aquacultured shrimp. Appl. Sci. 12 (10), 5135.
Thompson R. C., Olsen Y., Mitchell R. P., Davis A., Rowland S. J., John A. W. G., et al. (2004). Lost at Sea: where is all the plastic? Science 304 (5672), 838–838.
Van Cauwenberghe L., Janssen C. R. (2014). Microplastics in bivalves cultured for human consumption. Environ. Pollution. 193, 65–70.
Vo H. C., Pham M. H. (2021). Ecotoxicological effects of microplastics on aquatic organisms: a review. Environ. Sci. pollut. Res. 28 (33), 44716–44725.
Wang T., Li B., Wang D. (2021). The abundance and characteristics of microplastics in commonly consumed shellfish in the jiangsu coastal region of China. Environ. Sci. pollut. Res. 28 (43), 60753–60764.
Wang D., Zhen Y., Wei L., Dai Y., Wang X., Tong S., et al. (2022). Microplastic pollution in finless porpoises and their habitats along the fujian coast of the East China Sea. Front. Mar. Sci. 9.
Ward J., Zhao S., Holohan B., Mladinich K. M., Griffin T. (2019). Selective ingestion and egestion of plastic particles by the blue mussel (Mytilus edulis) and Eastern oyster (Crassostrea virginica): implications for using bivalves as bioindicators of microplastic pollution. Environ. Sci. Technology. 53 (15), 8776–84.
Wright S. L., Thompson R. C., Galloway T. S. (2013). The physical impacts of microplastics on marine organisms: a review. Environ. Pollution. 178, 483–492.
Xiang S., Xie Y., Sun X., Du H., Wang J. (2022). Identification and quantification of microplastics in aquaculture environment. Front. Mar. Sci. 8.
Xu P., Peng G., Su L., Gao Y., Gao L., Li D. (2018). Microplastic risk assessment in surface waters: a case study in the changjiang estuary, China. Mar. pollut. Bulletin. 133, 647–654.
Yang H., Xiong H., Mi K., Xue W., Wei W., Zhang Y. (2020). Toxicity comparison of nano-sized and micron-sized microplastics to goldfish carassius auratus larvae. J. Hazardous Materials. 388, 122058.
Yu Q., Hu X., Yang B., Zhang G., Wang J., Ling W. (2020). Distribution, abundance and risks of microplastics in the environment. Chemosphere. 249, 126059.
Yu X., Huang W., Wang Y., Wang Y., Cao L., Yang Z., et al. (2022). Microplastic pollution in the environment and organisms of Xiangshan Bay, East China Sea: An area of intensive mariculture. Water Research 212, 118117. doi: 10.1016/j.watres.2022.118117
Zhu L., Wang H., Chen B., Sun X., Qu K., Xia B. (2019). Microplastic ingestion in deep-sea fish from the south China Sea. Sci. Total Environ. 677 (AUG.10), 493–501.
Zhu J., Zhang Q., Li Y., Tan S., Kang Z., Yu X., et al. (2019). Microplastic pollution in the maowei Sea, a typical mariculture bay of China. Sci. Total Environment. 658, 62–68.
Keywords: microplastics, hazard risk assessment, seafood security, organ distribution, characteristics, Sinonovacula constricta
Citation: Chen B (2023) Characteristics and hazard risk of microplastics in Sinonovacula constricta: from farming to market. Front. Mar. Sci. 10:1151523. doi: 10.3389/fmars.2023.1151523
Received: 26 January 2023; Accepted: 24 April 2023;
Published: 12 May 2023.
Edited by:
Seyyed Morteza Hoseini, Iranian Fisheries Science Research Institute (IFSRI), IranReviewed by:
Levent Bat, Sinop University, TürkiyeAlessio Gomiero, Norwegian Research Institute (NORCE), Norway
Copyright © 2023 Chen. This is an open-access article distributed under the terms of the Creative Commons Attribution License (CC BY). The use, distribution or reproduction in other forums is permitted, provided the original author(s) and the copyright owner(s) are credited and that the original publication in this journal is cited, in accordance with accepted academic practice. No use, distribution or reproduction is permitted which does not comply with these terms.
*Correspondence: Bin Chen, chenbin@xujc.com