Investigating food web structure and system function of an artificial reef ecosystem based on carbon and nitrogen stable isotope analysis: implications for reef management
- 1North China Sea Marine Forecasting Center of State Oceanic Administration, Qingdao, China
- 2Chinese Academy of Sciences (CAS) Key Laboratory of Marine Ecology and Environmental Sciences, Institute of Oceanology, Chinese Academy of Sciences, Qingdao, China
- 3Qingdao National Laboratory of Marine Science and Technology, Qingdao, China
- 4Center for Ocean Mega-Science, Chinese Academy of Sciences, Qingdao, China
- 5Chinese Academy of Sciences (CAS) Engineering Laboratory for Marine Ranching, Institute of Oceanology, Chinese Academy of Sciences, Qingdao, China
- 6Shandong Provincial Key Laboratory of Marine Ecological Environment and Disaster Prevention and Mitigation, Qingdao, China
- 7Laboratory of Marine Organism Taxonomy and Phylogeny, Qingdao Key Laboratory of Marine Biodiversity and Conservation, Institute of Oceanology, Chinese Academy of Sciences, Qingdao, China
- 8Fourth Institute of Oceanography, Ministry of Natural Resources, Beihai, China
- 9Shandong Fuhan Marine Technology Co., Ltd, Haiyang, China
Introduction: Food web is an important basis for identifying trophodynamic processes, and evaluating the structural and functional characteristics of ecosystems. The trophodynamics and system function of artificial reef (AR) ecosystems have rarely been examined.
Methods: Stable isotope analysis was used to investigate the food web structure and functions of an artificial reef (AR) ecosystem in this study.
Results and Discussion: The δ13C and δ15N values of particulate organic matter (POM) in AR showed noticeable seasonal changes, and the δ13C value of POM in autumn was significantly higher than that in other seasons (p<0.05). There were no significant seasonal variations in the δ13C values of solid organic matter (SOM), and no significant difference between SOM and POM was observed except in autumn. Moreover, macroalgae did not significantly affect the δ13C values of SOM. Phytoplankton may be the primary nutrient source in the AR ecosystem. The δ13C values of most crustaceans in the AR were approximately between (-17.03 ± 0.22) ‰ – (-17.74 ± 0.07) ‰, higher than those of most fish, indicating that they may have different basal nutrient sources. The trophic level (TL) of invertebrates was between 2.00 and 3.09, and that of fish was between 2.98 and 3.66. The distribution of δ13C and TLs of crustaceans and fish showed that, except for crustaceans, bivalve shellfish and zooplankton might also be important food sources for fish in the AR ecosystem.
Conclusion: The δ13C and δ15N values of most species in the AR showed good continuity, indicating that they may be mainly produced from the AR ecosystem. Migratory species such as Lateolabrax japonicus and Sepiella maindroni showed higher δ13C values, indicating that they may have migrated from other sea areas. To maintain the stability of the ecosystem structure and function of the AR ecosystem, fishing activities should be carried out following the maximum sustainable yield theory. Future research needs to identify the nutritional relationship between AR and its adjacent sea areas, to depict the food web structure of the AR with higher accuracy.
1 Introduction
Inshore areas are well known for their rich biodiversity and high productivity, the major habitats and fishing grounds of many commercial species (Jin et al., 2015). Chinese inshore fisheries account for more than 90% of the total marine catches and are an essential source of high-quality proteins for Chinese people (Jin et al., 2015). However, due to overfishing and other excessive human activities (e.g., pollution, coastal construction), marine biodiversity and inshore fishery resources have markedly declined, and the coastal environment has seriously deteriorated (Liu, 2013; Jin et al., 2015). Therefore, the fishery production mode urgently needs transformation and upgrading in China’s coastal areas, and the coastal environment requires protection expeditiously. As a new type of marine economy, marine ranching plays a vital role in enhancing fishery resources and restoring marine ecosystems, which is also of great importance for the sustainable development of marine fisheries in China (Yang et al., 2016).
Currently, the construction of marine ranching has developed rapidly in China owing to the strong social demand and government promotion (Yang et al., 2018). From 2015 to 2023, China has gradually established eight batches of 169 national marine ranching demonstration areas, and the total sea area for marine ranching has exceeded 850 km2. Artificial reef (AR) construction is one of the most important ways to build marine ranching (Lin et al., 2020). By the end of 2018, ARs constructed in China’s coastal waters reached 60.94 million cubic meters. AR influences the environment by changing the flow field, heterogeneity of spatial structure, and bottom sediment (Bohnsack et al., 1991; Harmelin and Bellan-Santini, 1997; Zalmon et al., 2014). The upwelling formed by the ARs brings nutrients from the deep seabed to the upper layers, providing nutrients for phytoplankton (Okano et al., 2011). In addition, the complex spatial structure of ARs provides shelter for low trophic-level organisms or species at a vulnerable life stage (Hixon and Beets, 1989; Charbonnel et al., 2002; Okumura et al., 2003; Ushiama et al., 2016). The deployment of artificial structures provides new hard substrata for marine fouling organisms to settle down, increasing food resources for other marine organisms (Boaventura et al., 2006; Walker et al., 2010). The environmental change caused by AR construction leads to a change in the biological environment, increasing the biomass of all the organisms in the reefs (Chen et al., 2002). Several studies have demonstrated that the increase in biological resources in AR ecosystems is due to increased biomass production (Polovina and Sakai, 1989; Bortone et al., 1994; Powers et al., 2003; Claisse et al., 2014; Cresson et al., 2014). However, protesters also insisted that the increased biomass is due to the attraction hypothesis, the ARs serve purely as an aggregation device without any increase in biomass production (Bohnsack et al., 1994; Grossman et al., 1997; Pitcher and Seaman, 2000).
Despite numerous studies of ARs, the attraction-production controversy persists as an unresolved research question (Smith et al., 2016). These two effects are closely associated with the fishery production of ARs (Bortone, 2008). The “attraction” effects made the ARs a more efficient fishing area by attracting fishery species with little or no production. Simultaneously, the “production” effects also gain a significant advantage of fishery production exports through the increased biomass production of fishery species in ARs. The settlement of this controversy will greatly help the scientific construction and management of ARs (Cresson et al., 2014).
Net production requires the availability of suitable food and recruitment of larvae (Bohnsack and Sutherland, 1985). The production of high trophic level (TL) species relies on food sources transferred from low TLs through food web structures (Tang, 1996), so the trophodynamics of ARs provide an essential solution to resolve the attraction-production controversy (Bohnsack and Sutherland, 1985). However, the trophodynamics of ARs have rarely been examined. In order to analyze the structure and function of the AR ecosystem, an investigation of AR’s food web structure and trophodynamic processes are desperately needed (Lin et al., 2020).
Stable isotope analysis (SIA) is a powerful tool for identifying food sources (Phillips et al., 2014; Grey, 2016), calculating trophic position (Sahm et al., 2021; Stewart et al., 2021), and constructing food web structures (Mao et al., 2014; Gusha et al., 2021). Compared to the traditional stomach content analysis, which only represents food composition in a short period, the SIA provides data reflecting the long-term accumulation of food sources. It provides a more dependable approach for investigating marine food web ecology and increasing the credibility of the food web structure (Parnell et al., 2010; Feng et al., 2018).
Several studies have already used C and N stable isotope methods to study the structure of food webs in AR ecosystems. Meanwhile, Cresson et al. (Cresson et al., 2012; Cresson et al., 2014; Cresson et al., 2019) used C and N SIA to investigate the food web structure and assess the trophic function (production or attraction) of ARs. Zhang et al. (Zhang et al., 2020a; Zhang et al., 2020b) used SIA to investigate the food web structure prior to and following the construction of ARs. Wei et al. (2013), Xu et al. (2017), Xie et al. (2017), Lin et al. (2018) and Zhang et al. (2019) used stable isotope analyses to determine the basal food sources and TL of organisms in ARs. However, none of these studies explored the production or attraction functions of ARs in China.
The Fuhan AR ecosystem is located in the outer waters of Rushankou in the northern Yellow Sea. Until now, no research associated with food web structure and system function has been conducted for this AR ecosystem, and most of the construction and management activities associated with AR have been conducted based on empirical activities. This study used the Fuhan AR as the target to investigate the trophic structure and the AR ecosystem pathway, to depict a basic food web structure and explore the “attraction” and “production” functions of the AR ecosystem, thereby providing a scientific basis for AR construction and management.
2 Materials and methods
2.1 Study area
The Fuhan AR is located in the northern Yellow Sea, southeast of the Shandong Peninsula. The coordinates of the central point were 36° 42′ 5.27″ N, 121° 27′ 31.16″ E. The specific locations of the AR are shown in Figure 1. The Fuhan marine ranching was built by AR construction and stock enhancement to restore fishery resources and degraded habitats. Since 2013, 0.25 million cubic meters of ARs have been deployed in a 6.67 km2 zone. The proliferation and release activities of sea cucumber Stichopus japonicus and Korean rockfish Sebastes schlegelii have also been conducted for several years. The average water depth of the Fuhan AR was approximately 12 m.
2.2 Sampling strategy
Samples of phytoplankton, zooplankton, particulate organic matter (POM), and solid organic matter (SOM) were collected in July, September, November 2017, and May 2018. Macroalgae were collected in July 2017. Samples of swimming creatures and zoobenthos were collected in September 2017. POM was obtained by filtration, and approximately 1 L of seawater was filtered through a screen with a mesh size of 63 μm to remove large particles and zooplankton and then onto precombusted (450°C, 6 h) and preweighed Whatman GF/F glass fiber filters under vacuum suction of less than one-third atmospheric pressure. The residue on the filter papers was rinsed with distilled double deionized water to remove the salt adsorbed on the particle surface. Zooplankton samples were obtained by horizontal towing using a 63 μm plankton net, which was then held in filtered seawater overnight to allow the evacuation of gut contents. After that, zooplankton samples were dried at 60°C for 48 h and processed into a fine powder using a mortar and pestle. Macroalgae were hand-collected and rinsed with distilled water immediately after sampling. Sediments were collected using a sediment sampler, large benthic invertebrates of Crassostrea gigas and A. japonicus were collected by SCUBA diving, and Perinereis aibuhitensis were collected using a clam bucket dredger. All other benthic invertebrates and fish species were collected using cage nets and gillnets.
The white muscles of individual fish were removed from the dorsal part for SIA, the abdominal muscles of shrimp, the adductor muscles of shellfish, and the claw muscles of crabs were taken for SIA. Small invertebrates (e.g., Caprella acanthogaster) were prepared by pooling a suitable number (10–20 individuals) of whole specimens.
All samples were dried to a constant weight in an oven at 60°C. The dried tissue and sediments were pulverized into a fine powder using a mortar and pestle to ensure homogeneity. Samples for carbon SIA were acidized for 4 h with 1N HCl to remove carbonate and then dried at 60°C for 24 h. Samples for nitrogen stable isotope analysis were directly measured without the treatment of acidification (Cui et al., 2012). All samples were then stored at −20 °C prior to subsequent SIA.
2.3 Water quality determination
The water quality of marine ranching was measured in July, September, November 2017, and May 2018, respectively. The temperature, salinity, pH, and dissolved oxygen were measured by using a EXO2 multiparameter water quality detector (YSI co., USA). The mixed water samples (2 L) for determining inorganic nutrients (PO43-, NH4+, NO3-, NO2-, and SiO32-) and Chlorophyll (Chl) a were taken from the surface, middle, and bottom layers of the marine ranching. The water samples for determining inorganic nutrients were first filtered through pre-combusted (450°C, 2 h) Whatman GF/F filters, and then measured according to the method of Lei (2006). The samples for determining Chl a were filtered through a 0.45 μm glass fiber filter, then the Chl a on the filter papers was extracted for 30 h with acetone (90%) under cold conditions (4 °C) and centrifuged. The absorbance values of the solutions were measured at 665 and 750 nm using a spectrophotometer. The concentration of Chl a was calculated following the method described by Lorenzen and Jeffrey (1980).
2.4 Measurement of stable isotope
The carbon and nitrogen isotope ratios of the prepared samples were measured using an elemental analyzer coupled with an isotope ratio mass spectrometer (EA-IRMS, Thermo Finnigan MAT Delta-plus) (Vander Zanden and Rasmussen, 2001). Stable isotope ratios are expressed in standard δ-unit notation, which is defined as follows:
where X represents 13C or 15N, and R represents the 13C:12C ratio for carbon or the 15N: 14N ratio for nitrogen. The results were reported relative to the Vienna Pee Dee Belemnite standard (PDB) for carbon and air N2 for nitrogen. A laboratory working standard (glycine) was run for every ten samples. The analytical precision for the carbon and nitrogen measurements was ± 0.01‰.
2.5 Data analysis
Carbon and nitrogen stable isotopes are generally delivered along food chains with predictable stepwise enrichment between prey and consumers (Hobson and Welch, 1992). Therefore, TL was determined relative to the filter-feeding bivalves (Crassostrea gigas) as primary consumers, assumed to occupy the 2nd TL (Vander Zanden and Rasmussen, 2001). Using 3.4‰ enrichment of δ15N per TL averaged over multiple TLs (Minagawa and Wada, 1984; Peterson and Fry, 1987; Post, 2002).
where TL is the trophic level, δ15Nconsumer is the nitrogen isotopic ratio of consumers, δ15Nreference is the nitrogen isotopic ratio of marine primary consumers (Crassostrea gigas), and δ15NTEF is the trophic enrichment factor. Because the primary consumers were at the bottom of the trophic ladder, they were assumed to occupy the 2nd TL in this study.
Data are presented as means and standard deviations (mean ± SD). In addition, the values of δ13C and δ15N of the measured samples were compared using one-way ANOVA followed by Duncan’s multiple comparison test. The analyses were performed using SPSS software (version 17.0; SPSS Inc., Richmond, CA, USA), and values of p< 0.05 were considered significant.
3 Results
3.1 Water quality of the AR environment
The water quality data are listed in Tables 1, 2. During the sampling period, salinity varied between 30.29‰ and 32.54‰, temperature varied between 6.51–27.66 °C, pH varied between 8.21–8.31, and dissolved oxygen varied between 6.26–8.92 mg/L. The concentration of varied between 0.01–0.03 mg/L, the concentration of varied between 0.03–0.08 mg/L, the concentration of varied between 0.08–0.18 mg/L, the concentration of varied between 0.004–0.021 mg/L, and the concentration of varied between 0.04–0.17 mg/L. Seasonal fluctuations in Chl a concentration in the AR ecosystem were observed, as shown in Figure 2. The highest Chl a concentration was observed in autumn and lowest in winter.
3.2 Isotopic compositions of organic matters, and primary producers
The carbon and nitrogen stable isotope ratios of POM, SOM, and macroalgae are presented in Table 3 and Figure 3. The δ13C and δ15N values of POM ranged from -21.40‰ – -18.20‰ and 7.19‰–8.68‰ during the four seasons, respectively. Significant differences among POM pools were observed for δ13C and δ15N values (p<0.05). The highest δ13C value of POM was observed in autumn and lowest in winter. The highest δ13N value of POM was observed in summer and lowest in autumn. No significant differences among the SOM pools were observed (p<0.05). Among all the primary food sources, Ulva pertusa had the highest δ13C and δ15N values. No significant differences were observed for the organic pools of POM and SOM, except for POM and SOM in autumn (p<0.05), indicating transparent pelagic-benthic coupling processes.
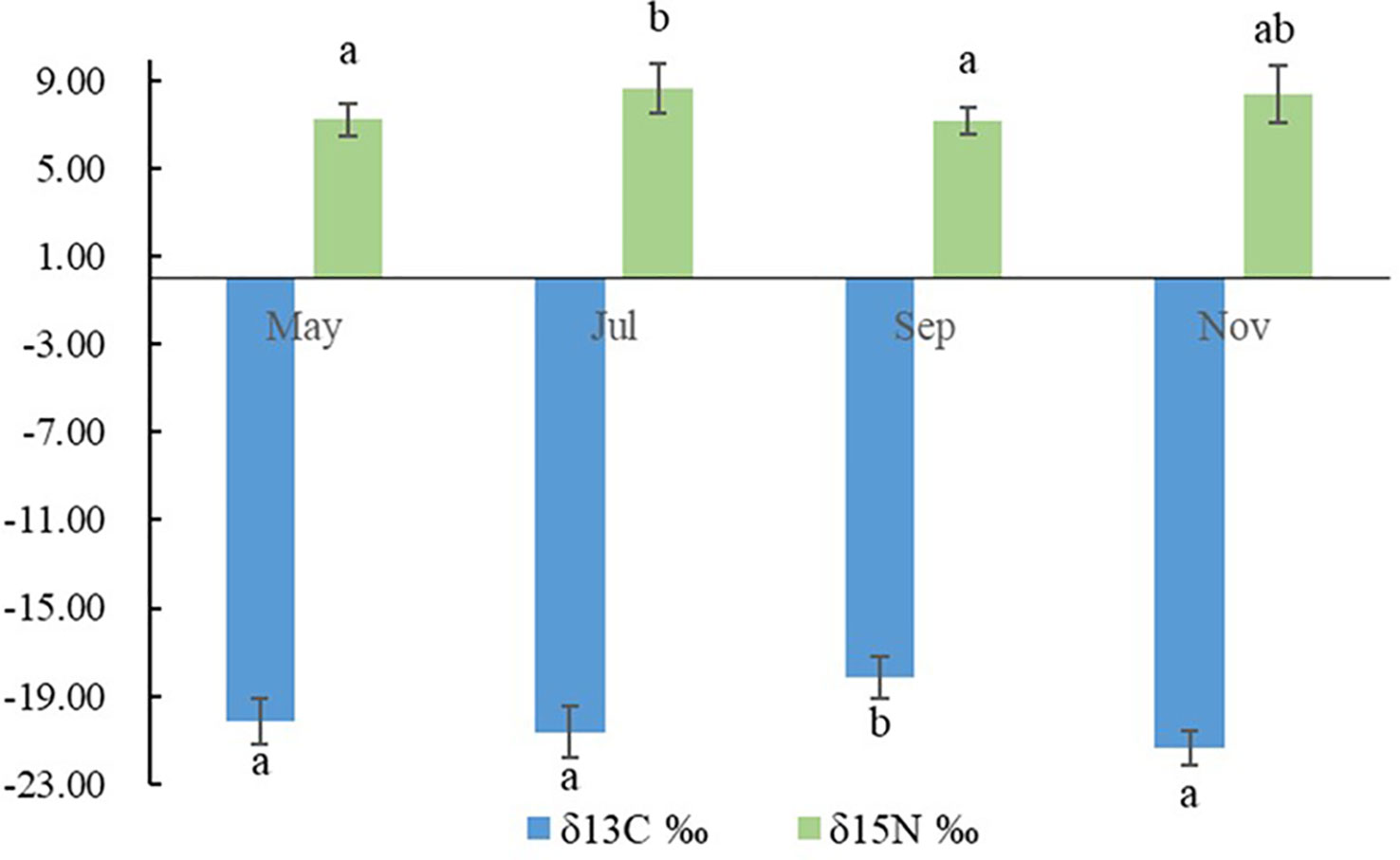
Figure 3 Seasonal variations in the δ13C and δ15N values of particulate organic matter (POM). Columns with different letters indicate significant differences at p< 0.05.
3.3 Isotopic composition of consumers
The δ13C and δ15N values of consumers in this AR ecosystem are presented in Table 4 and Figure 4. Comparison of mean δ13C and δ15N revealed substantial isotopic differences (p<0.05) among all invertebrate species, encompassing isotopic values of 5.09‰ for δ13C, and 3.70‰ for δ15N. The mean δ13C values of the benthic invertebrates ranged from the most δ13C depleted value of -20.43‰ for C. gigas bivalves, to the most enriched -15.34‰ for crab Eucrate crenata, with δ15N values ranging from 7.97‰ for C. gigas to 11.67‰ for shrimp Oratosquilla oratoria. Moreover, crabs of Charybdis japonica and cephalopoda Octopus vulgaris showed higher δ15N values than the other invertebrates, with mean values of 11.50. The TL of invertebrate species ranged from 2.0–3.09, and O. oratoria displayed the highest TL (Figure 5).
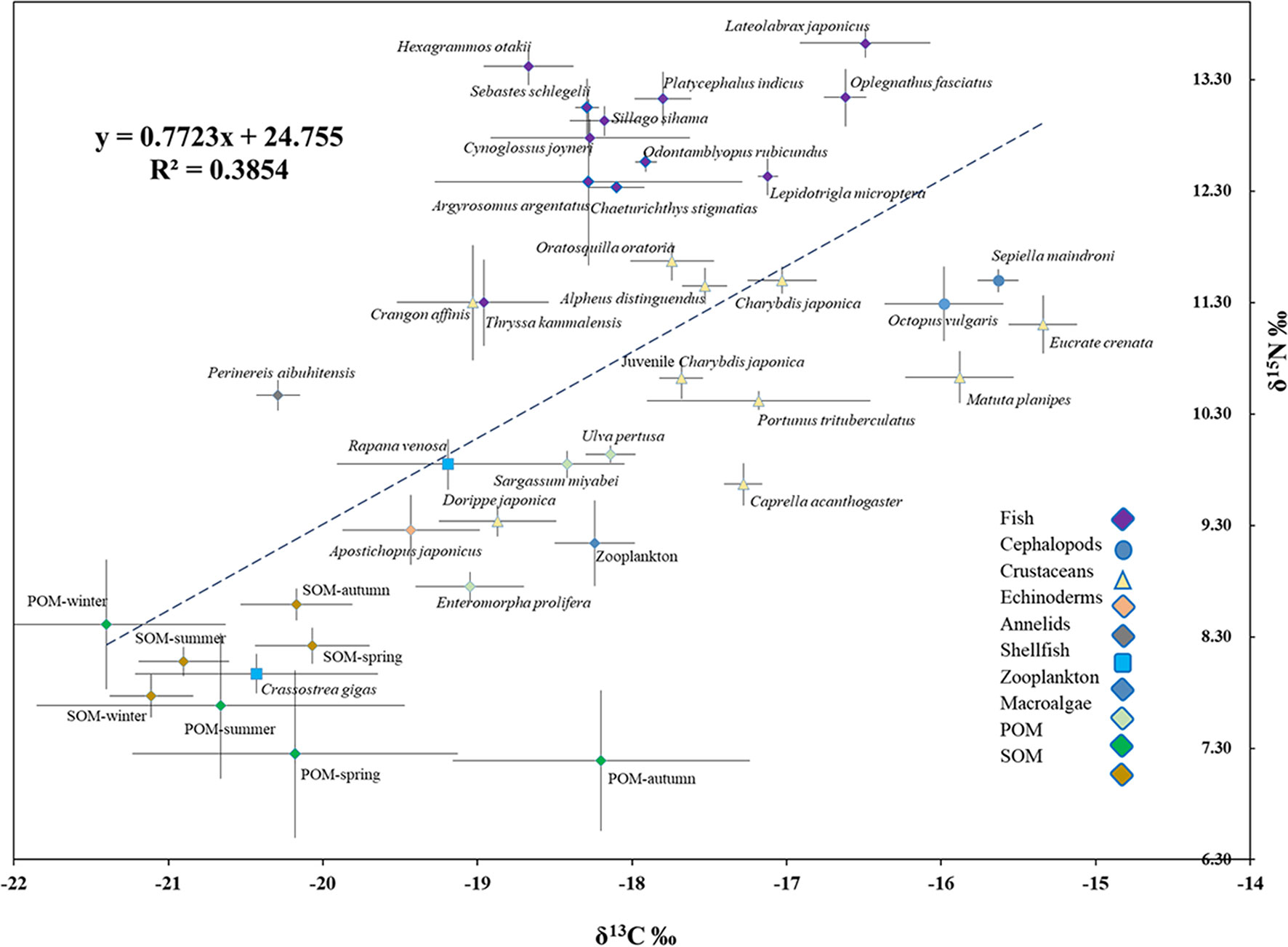
Figure 4 Bi-plots of δ13C against δ15N (mean ± SD) of SOM, POM, zooplankton, and different species of fish, cephalopods, crustaceans, echinoderms, annelids, shellfish, and macroalgae in the artificial reef ecosystem.
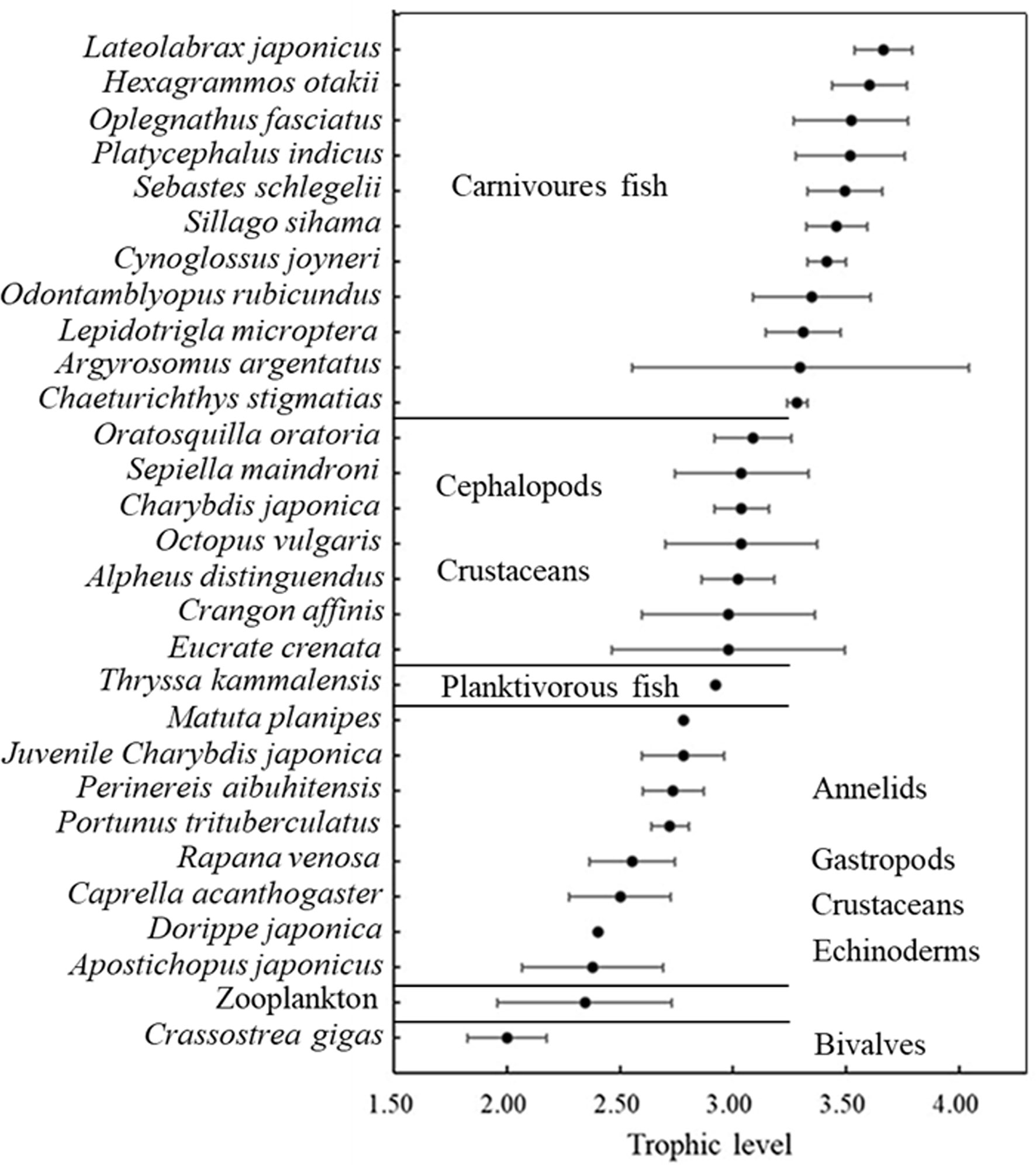
Figure 5 Trophic positions of the animal species based on the nitrogen stable isotope analysis. Trophic levels were calculated using the enrichment factor of 3.4‰ per trophic level.
A steady enrichment of δ13C with an increasing trend from the filter feeders (bivalves) to deposit feeders of P. aibuhitensis and A. japonicus, then the omnivorous crustaceans and cephalopods. The crustaceans C. japonica, Portunus trituberculatus, Crangon affinis, C. acanthogaster, Alpheus distinguendus, and O. oratoria, were considered to have similar primary food sources, as most of them had similar δ13C values.
The deposit-feeding species that graze organic content from the sediment on the seabed surface, including P. aibuhitensis and A. japonicus, showed higher δ15N values relative to those of filter-feeding bivalves and zooplankton. In contrast to the stable nitrogen isotope, the zooplankton group showed higher δ13C values than the main species of bivalves, annelids, and echinodermata. Dorippe japonica and C. acanthogaster have low δ15N values among crustaceans and cephalopods, indicating lower TL among these species.
For fish species, the range of isotopic ratios measured in fishes was lower for δ13C (2.54‰ only), but higher for δ15N (5.19‰) than invertebrates. Thryssa kammalensis was the main pelagic fish in this ecosystem, which expressed the lowest δ13C and δ15N values among all the investigated fish, with mean values of -19.03‰ and 11.30‰, respectively. The highest δ13C and δ15N values were observed for Lateolabrax japonicus, with mean values of -16.49‰ and 13.63‰, respectively. Oplegnathus fasciatus and Hexagrammos otakii had the second-highest δ13C and δ15N values, respectively. The TL of fish ranged from 2.98–3.66, T. kammalensis displayed the lowest TL, and L. japonicus displayed the highest TL (Figure 5).
The position of fish groups on the δ13C versus δ15N biplot (Figure 4) indicated that all fish species fit well into the continuity of the position occupied by invertebrates in the trophic network of AR. This result was also consistent with the significant correlation observed between δ15N and δ13C of all consumers, invertebrates, and fish combined (δ15N = 0.7952 δ13C + 24.539; R² = 0.3718, p< 0.05), suggesting that most consumers belonged to a similar trophic pathway, with fish at its top.
4 Discussion
4.1 Basal nutrient sources of the AR ecosystem
POM (mainly composed of phytoplankton and other water detritus), SOM (mainly composed of microphytobenthos and organic detritus), macroalgae, and benthic microalgae are the primary food sources of AR ecosystems (Cresson et al., 2014; Xu et al., 2017; Zhang et al., 2021). The δ13C of POM in this study ranged from -21.40‰ ± 0.77‰ – -18.20‰ ± 0.96‰, which was similar to the δ13C value of POM usually ranged from -18‰ – -24‰ in temperate waters (Gearing et al., 1984; Fry and Wainright, 1991; Harmelin-Vivien et al., 2008). The δ13C of POM in autumn was significantly higher than that in the other three seasons, which may be associated with a Noctiluca scientillans bloom outbreak in the AR in autumn. N. scientillans usually have a larger particle size than most other phytoplankton cells (Uhlig and Sahling, 1990; Sriwoon et al., 2010), its δ13C value may also be higher, as phytoplankton with larger sizes were observed to have higher δ13C values than smaller phytoplankton (Rau et al., 1990; Fry and Wainright, 1991; Grégori et al., 2001; Cresson et al., 2012).
Except in autumn, no significant differences were found between the δ13C of SOM and POM in this AR ecosystem. These results differed from those of Cresson et al. (2012) and Xu et al. (2017), who found that the δ13C value of SOM was significantly lower than that of POM in AR ecosystems. Microphytobenthos, POM, and macroalgae are considered the main contributors to SOM in neritic ecosystems (Cahoon, 1999; Letourneur et al., 2013; Briand et al., 2015; Zhang et al., 2021). Benthic microalgae are usually δ13C enriched compared to planktonic microalgae because of differences in light temperature and photosynthesis mechanisms (Wiencke and Fisher, 1990; Fry and Wainright, 1991; France, 1995). In this study, the average Chl a of the benthic microalgae was only 3.5 mg C m-2 (unpublished data), which was very low compared to the other neritic ecosystems (Cahoon, 1999), indicating that benthic microalgae may not be an important energy source of SOM in this ecosystem. U. pertusa and E. prolifera were the main macroalgal species in the AR ecosystem, most imported from adjacent sea areas in summer. The outbreak of E. prolifera was considered a natural disaster in the Yellow sea (Chen et al., 2018; Xu, 2018), and a large part of E. prolifera was floated to Fuhan marine ranching in June and July (the covered area was approximately 20000 m2). The significantly higher δ13C value of the two macroalgal species than that of the SOM indicated that the macroalgae were also not a critical energy source for SOM. The similar δ13C values of POM and SOM indicate that POM may be the main contributor of SOM, and strong coupling of benthic-pelagic mechanism existed in the AR ecosystem.
4.2 Food sources of consumers in the AR ecosystem
The sessile filter feeder, oyster C. gigas, is an ecosystem engineer that creates and maintains the oyster reef structure and provides resources for other organisms (Padilla, 2010), dominated in the fauna of this AR ecosystem, which showed a δ13C value lower than that of the POM in autumn. This seems contradictory, as POM is usually considered the primary food source for oysters. The lower δ13C value of oysters may be ascribed to its qualitative selection for microalgae, especially for diatoms (Riisgard and Larsen, 2000; Decottignies et al., 2007; Marín Leal et al., 2008; Kang et al., 2009), because most diatoms have a smaller particle size than N. scientillans, and the diatoms may have lower δ13C values that that of the POM in this AR ecosystem (Sato et al., 2006; Sugie and Suzuki, 2015).
Snails of Rapana venosa are usually considered carnivorous predators, such as bivalves (Byon et al., 2015; Song et al., 2016). The oyster was inferred as its main food source in the artificial oyster reef (Xu, personal communication); however, the δ15N value of R. venosa was only 1.66‰ higher than that of the oyster, and the TL of R. venosa was 2.55 in this study, indicating that almost half of its food originated from TL I. This seems contradictory to previous results: Qi (2017) discovered that adult R. venosa had a low amylase activity, which reflected that this species could only ingest a low level of plant food, and Yang et al. (2020) further discovered that R. venosa did not feed on phytoplankton based on fatty acid analysis. Moreover, it seems that only oyster production can support the high biomass of R. venosa in this AR (the biomass of R. venosa and oyster in this AR were approximately 270 g m-2 and 1500 g m-2, respectively) (unpublished data). The relatively low δ15N value of this species may be because R. venosa has a very low nitrogen fractionation. DeNiro and Epstein (1981) and Vanderklift and Ponsard (2003) demonstrated that the discrimination factor of δ15N may vary among species. The δ15N discrimination factors of Assiminea japonica and Angustassiminea castanea snails were lower than one (Kurata et al., 2001). The actual fractionation factor of this species needs to be estimated to provide a firm explanation for this phenomenon.
The deposit feeder, A. japonicus, is an important economic species and has a high biomass in the AR ecosystem. It had a δ13C value similar to that for SOM. Sun et al. (2012) discovered that the δ13C and δ15N discrimination factors of A. japonicus were 1.2‰ and 1.9‰, respectively. Based on these data, it can be speculated that the δ13C and δ15N values of sea cucumbers were consistent with the POM. Therefore, POM may be the main food source for sea cucumbers in this AR. This result is similar to Yokoyama (2013), who found that phytoplankton was the primary food source for A. japonicus in a sea cucumber-Pacific oyster integrated multi-trophic aquaculture ecosystem. Oysters may play an important role in supporting food sources for sea cucumbers. The filtering activity of oysters can transfer a large part of POM to the bottom of the AR (Cheung et al., 2010; Cresson et al., 2014), and the pseudofeces and feces from bivalves are an important food source for sea cucumbers (Yuan et al., 2008; Slater et al., 2009; Zamora et al., 2014).
Most crustaceans are omnivorous in marine ecosystems, usually positioned at intermediate levels, and play important roles in transferring energy from lower to higher TLs (Wilson et al., 2001). The crustaceans showed a narrow range of δ13C values, except for D. japonica and Matuta planipes, indicating that most crustaceans may have similar primary food sources. The δ13C of the crustaceans was close to that of POM in autumn, U. pertusa, and S. muticum. Considering the integration time of crustaceans (Fry and Arnold, 1982), δ13C may reflect the diets of these crustacea–1–2 months ago, when a lot of macroalgae were floated into the AR. Therefore, the macroalgae U. pertusa and S. muticum were probably important food sources for these crustaceans. These results conformed to Feng et al. (2014), who found that macroalgae were an important contributor to the nutritional support of crustaceans.
Cephalopods O. vulgaris and Sepiella maindroni showed the lowest δ13C values in the AR ecosystem, significantly lower than those of POM and macroalgae. The stable isotope signatures in animal tissues reflect the local food source where the animal resides (Peterson and Fry, 1987; Michener and Schell, 1994). The particularly low δ13C values of these two species indicate that they may consume their food from entirely different surroundings. These results were consistent with our observation that these two species usually moved into the AR from other sea areas in autumn. Zhang et al. (1997) reported that S. maindroni usually stayed in coastal areas for spawning from May to November, suggesting that S. maindroni may have migrated into the AR ecosystem from another coastal sea area. Stomach content analysis indicated that S. maindroni and O. vulgaris mainly feed on fish and crustaceans (Yang, 2001; Yang et al., 2018), whose TLs were 4.2 and 4.5, respectively, in the Bohai Sea, which was significantly higher than those (3.04) in this study. However, the isotopic analysis conducted by Zhang et al. (2020) indicated that the TL of O. variabilis in AR was only 2.72. This may be ascribed to the differences between stomach content analysis and SIA, as the stomach content analysis only reflects the organism’s instantaneous food information, while the SIA provides integrated food information over a long period (Hobson and Welch, 1992; Qu et al., 2016).
The δ13C and δ15N values of fish in this AR ecosystem ranged from -19.03‰ –16.62‰ and 12.33‰–13.63‰, respectively. The average δ13C values of the fish species were quite similar, except for O. fasciatus, L. japonicus, and T. kammalensis. T. kammalensis is a pelagic predator that mainly feeds on plankton (Guo and Tang, 2000), so its δ13C values were relatively lower and close to the phytoplankton. L. japonicus is a seasonal migratory predator whose food sources may come from a significantly larger area than the AR, thereby showing a different δ13C value. S. schlegelii and Hexagrammos otakii are typical reef fishes that usually remain in the AR area (Zhang et al., 2015), which were discovered in the AR with high biomass (unpublished data). Similar δ13C values of these fishes indicate that they may have similar primary food sources. Assuming a δ13C discrimination factor of 0.75‰ or 1‰, phytoplankton should be their main primary food source. However, similar δ13C values did not indicate similar food compositions. According to Zhang et al. (2018) and Zhang et al. (2021), no significant niche overlap was observed between H. otakii and S. schlegelii in ARs based on stomach content analysis and SIA. H. otakii tends to eat decapods and amphipods, whereas S. schlegelii tends to eat fish and shrimp (Wei and Jiang, 1992; Park et al., 2007; Ji, 2014; Zhang et al., 2014; Zhang et al., 2018; Zhang et al., 2021). The δ15N values of these species also indicated that they had relatively different ecological niches. Cresson et al. (2014) highlighted that crustaceans are the most commonly ingested prey type in the AR ecosystem. However, most fishes in the Fuhan AR ecosystem showed lower δ13C values than the crustaceans, and the TL of these fish mostly ranged from 3.00–3.66; the crustaceans mostly ranged from 2.40–3.04, these indicated that food sources such as zooplankton and bivalves with higher δ13C values and lower TLs were also important food sources for fish. These results differed from Zhang et al. (2018) and Deng et al. (1997), who found that S. schlegelii, P. argentata, and H. otakii consumed very few bivalves, annelidas, and zooplankton as their food sources.
4.3 The TL and food web structure of the AR ecosystem
In this study, TLs of C. gigas, zooplankton, and A. japonicus were close to TL II. TLs of most crustaceans were close to but lower than TL III, and TLs of most fish ranged from 3.28–3.66. Fish were the top predators of this ecosystem, however, even the fish with the highest TL did not reach four. The reason for the short food chain length of this ecosystem may be due to the fact that the most fish individuals are relatively small, even the largest fish sampled in the reef was not heavier than 500 g (unpublished data). These fishes were unable to consume large invertebrates or fish at high TL. The TL of S. schlegelii, P. argentata, and H. otakii was lower than that in the Bohai Sea, as reported by Yang (2001). These results were supported by Zhang et al. (2020), who found that TLs of fish in AR were lower than those in the natural ecosystem.
Based on the SIA, a simplified diagram of the food web in the AR ecosystem was obtained, as shown in Figure 6. Four energy flow pathways were observed in the simplified food web: (1) POM→ zooplankton→ planktivorous fish; (2) POM→ bivalves→ carnivorous snails/crustaceans → carnivorous fish; (3) SOM→ detritivorous echinoderm; (4) SOM/macroalgae → detritivorous annelids and crustaceans→ omnivorous crustaceans→ carnivorous fish. As most species were benthic predators, the food web was mainly dominated by the benthic energy flow pathway in the AR. The bivalve C. gigas seemed to play a key role in the benthic food web structure, as C. gigas transported large amounts of phytoplankton to the bottom environment, which provided sufficient energy for benthic food webs.
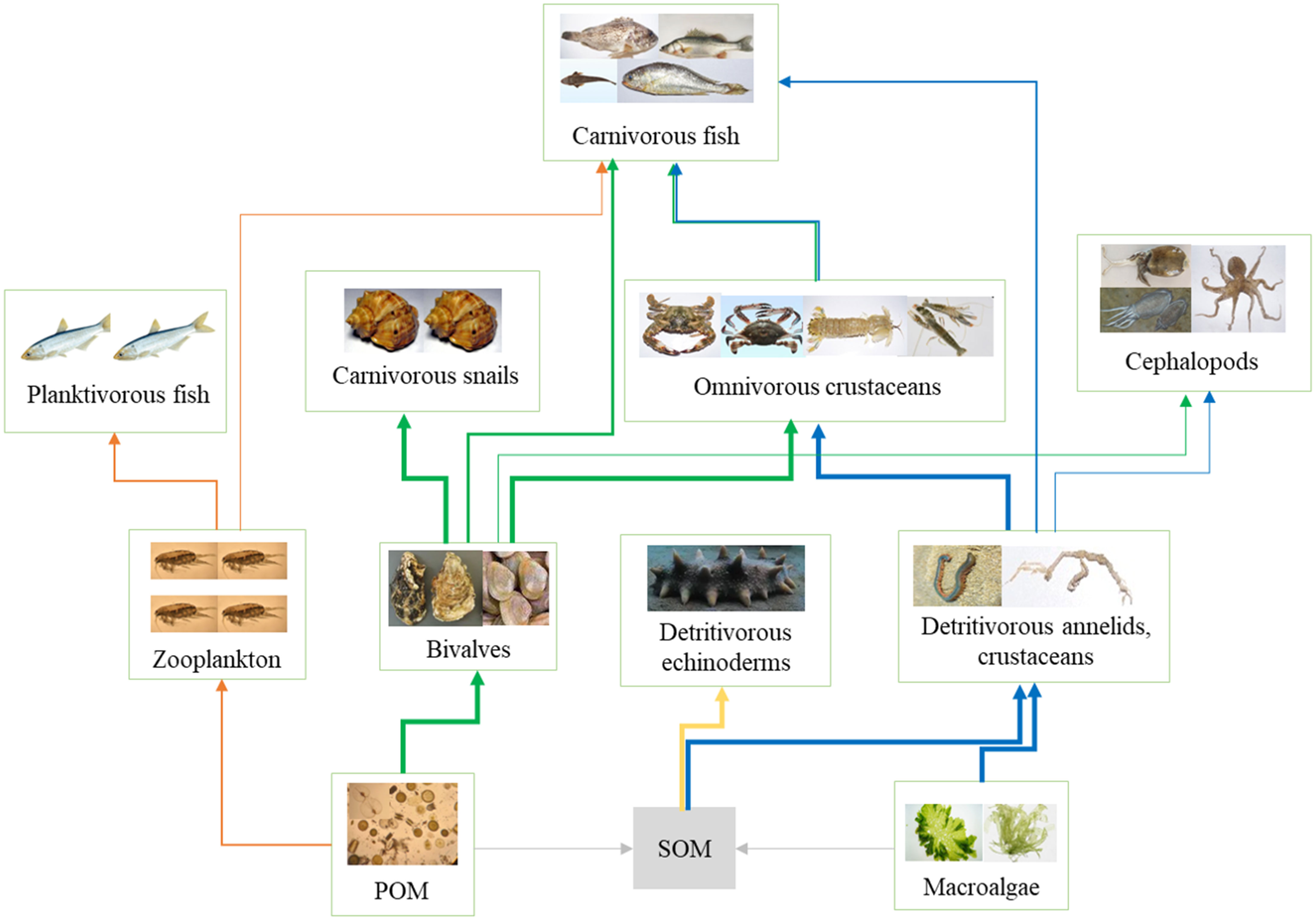
Figure 6 Conceptual model of the trophic structure and energy flow of the artificial reef ecosystems. Thick arrows with orange, green, yellow, and blue colors demonstrate the main energy flow pathways.
4.4 The clues for “producer” or “attractor” of the AR
The AR ecosystem is the “producer” or “attractor” for swimming creatures is a controversial issue ((Bohnsack and Sutherland, 1985; Bortone, 1998; Wilson et al., 2001; Cresson et al., 2014; Brickhill et al., 2015). In this study, most crustaceans showed similar δ13C values, and were close to those of POM and macroalgae. The fish species showed similar δ13C values, and their δ15N values showed good continuity concerning crustaceans, indicating that the AR ecosystem may produce most crustaceans and fish species. However, it is worth noting that these native fish species tend to prey around ARs (Prince and Gotshall, 1976; Hueckel and Buckley, 1987), so the AR and its adjacent waters both play the role of producers. Species of M. planipes, E. crenata, O. vulgaris, S. maindroni, and L. japonicus, which are not typical species of the AR ecosystem, showed lower δ13C values than other typical reef species, may only spend part of their lives in the AR. Another stable isotopic analysis research (unpublished data) revealed that the δ13C of POM values from near shore in Rushankou were higher than those in the AR, and the δ13C of POM values from the outer sea area were lower than those in the AR. It is quite probable that these species immigrated from the near-shore area.
4.5 Implications for AR management
Scientific management plays an important role in the sustainable development of AR ecosystems. Oyster C. gigas transferred a large part of POM to the bottom, which played a key role in coupling the pelagic-benthic environment and sustaining the benthic energy flow pathway. Therefore, it is necessary to protect it from excessive predation by predators, such as R. venosa. The fishing activities targeted for the migratory species (e.g., O. vulgaris and L. japonicus) should be conducted with sufficient caution, because excessive harvesting may make the AR a trap attracting these species, resulting in the decline of these resources in the surrounding waters. It is necessary to evaluate the carrying capacity and maximum sustainable yield of economic species in the AR, and the sustainable yields of the migratory species should be evaluated in an area larger than the AR. S. maindroni was once an important economic species on China’s coast, almost disappearing in China’s northern coast in the past 20 years due to habitat degradation and overfishing (Wu et al., 2010). It was discovered that this AR ecosystem was a spawning ground for this species (Zhang et al., 2020), indicating that the construction of ARs was beneficial for recovering the population of this species on the northern coast of China. Managers can specially design and introduce suitable spawning reefs to improve the resource restoration efficiency of this species.
4.6 Future prospects
This study shows that stable isotope technology is a useful way to investigate the food sources of organisms in AR. The investigated results can provide important clues for exploring the “production” or “attraction” issue of AR, and provide important data for constructing the energy flow model of the AR ecosystem. However, as the sampling area is limited in the marine ranching ecosystem, it is unable to clarify the possible impact of the adjacent sea area on the food web of the AR, which makes the research results have certain limitations. By measuring the distribution of stable isotopes of biotic and abiotic samples in the adjacent area of the marine ranching, we can further clarify the effects of nutrient inputs and biological migration on the food web structure of the AR. In addition, based on SIA, we can only roughly determine the possible food sources of consumers, but cannot determine the specific food composition. Combined with other methods, such as stomach content analysis and fatty acid analysis, we can further determine the food sources and composition of the consumers with higher accuracy to optimize the research results.
Data availability statement
The original contributions presented in the study are included in the article/supplementary material. Further inquiries can be directed to the corresponding authors.
Ethics statement
The animal study was reviewed and approved by the Experimental Animal Ethics Committee of Institute of Oceanology, Chinese Academy of Sciences.
Author contributions
JF, XLZ, FB, WZ, and LZ: investigation, methodology, and writing - original draft; JLX and TZ: supervision, conceptualization, and writing- review and editing; HS, MJY, ZH, CZ, PS, PPH, and PZM: sample analysis; PFS and HJ: writing-reviewing. All authors contributed to manuscript revision, read, and approved the submitted version.
Funding
This research was supported by the China National Key R&D Program of China(2022YFD2401301), National Natural Science Foundation of China (42106102), the Primary Research and Development Plan of Guangxi Province (2021AB34014), the Qingdao Postdoctoral Application Research Project (QDBSH20220201045), the program supported by China National Offshore Oil Corporation Marine Environment and Ecological Protection Public Welfare Foundation (CF-MEEC/TR/2023-7), and the Youth Science and Technology Talent Project supported by Ministry of Natural Resources (recipient: JLX).
Acknowledgments
The authors thank Lu qi, Wencai Xie, and Yaoyang Liu for preparing the samples. The authors also thank Haizhou Li for providing the sampling boats.
Conflict of interest
Author HJ was employed by the company Shandong Fuhan Marine Technology Co., Ltd.
The remaining authors declare that the research was conducted in the absence of any commercial or financial relationships that could be construed as a potential conflict of interest.
Publisher’s note
All claims expressed in this article are solely those of the authors and do not necessarily represent those of their affiliated organizations, or those of the publisher, the editors and the reviewers. Any product that may be evaluated in this article, or claim that may be made by its manufacturer, is not guaranteed or endorsed by the publisher.
References
Boaventura D., Moura A., Leitão F., Carvalho S., Monteiro C. C. (2006). Macrobenthic colonisation of artificial reefs on the southern coast of Portugal (Anco, Algarve) (Netherlands: Springer).
Bohnsack J. A., Sutherland D. L. (1985). Artificial reef research: a review with recommendations for future priorities. Bull. Mar. Science. 37 (1), 11–39. doi: 10.1515/botm.1985.28.11.501
Bohnsack J. A., Johnson D. L., Ambrose R. F. (1991). “Ecology of artificial reef habitats and fishes,” in Artificial habitats for marine and freshwater fisheries. Eds. Seaman W. Jr., Sprague L. M. (San Diego: Academic Press), 61–107. doi: 10.1515/botm.1985.28.11.501
Bohnsack J. A., Harper D. E., McClellan D. B., Hulsbeck M. (1994). Effects of reef size on colonization and assemblage structure of fshes at artifcial reefs off southeast-ern Florida, U.S.A. Bull. Mar. Sci. 55, 796–823.
Bortone S. A. (1998). Resolving the attraction-production dilemma in artificial reef research: some yeas and nays. Fisheries. 23 (3), 6–10. doi: 10.1577/1548-8446(1998)023<0006:RTADIA>2.0.CO;2
Bortone S. A. (2008). "Coupling fisheries with ecology through marine artificial reef deployments". in. Reconciling Fisheries Conservation: Proc. Fourth World Fisheries Congress (Bethesda, Maryland), 917–924.
Bortone S. A., Tassell J. V., Brito A., Falcon J. M., Mena J., Bundrick C. M. (1994). Enhancement of the nearshore fsh assemblage in the canary islands with artifcial habitats. Bull. Mar. Sci. 55, 602–608.
Briand M. J., Bonnet X., Goiran C., Guillou G., Letourneur Y. (2015). Major sources of organic matter in a complex coral reef lagoon: identification from isotopic signatures (delta13C and delta15N). PloS One 10 (7), e0131555. doi: 10.1371/journal.pone.0131555
Brickhill M. J., Lee S. Y., Connolly R. M. (2015). Fishes associated with artificial reefs: attributing changes to attraction or production using novel approaches. J. Fish Biol. 67 (sB), 53–71. doi: 10.1111/j.0022-1112.2005.00915.x
Byon J. Y., Moon H. T., Son M. H., Hwang C. H., Kim D. I. (2015). Predation of neptunea cumingii crosse on the young disk abalone, haliotis discus hannai (ino) in the eastern coast of Korea. Korean J. Malacol. 31 (3), 165–170. doi: 10.9710/kjm.2015.31.3.165
Cahoon L. B. (1999). The role of benthic microalgae in neritic ecosystems. Oceanogr. Mar. Biol. 37, 47–86.
Charbonnel E., Serre E., Ruitton S., Harmelin J. G., Jensen A. C. (2002). Effects of increased habitat complexity on fish assemblages associated with large artificial reef units (French Mediterranean coast). ICES J. Mar. Science. 59, S208–S213. doi: 10.1006/jmsc.2002.1263
Chen Y., Qing Y. C., Zhang G. S., Zhang S. (2002). The environmental function and fish gather effect of atificial reefs. J. Dalian Fish. University. 11 (1), 64–69. doi: 10.3969/j.issn.1000-9957.2002.01.012
Chen L., Wang X. M., Zhang X. L. (2018). Research on the formation of green tide of Enteromorpha prolifera in qingdao offshore. J. Sci. Teachers′College University. 038 (009), 37–42. doi: CNKI:SUN:GLKX.0.2018-09-009
Cheung S. G., Wai H. Y., Shin P. K. S. (2010). Fatty acid profile of benthic environment associated with artificial reef in subtropical Hong Kong. Mar. Pollut. Bull. 60, 303–308. doi: 10.1016/j.marpolbul.2009.12.001
Claisse J. T., Pondella D. J., Love M., Zahn L., Williams C., Williams J., et al. (2014). Oil platforms off California are among the most productive marine fish habitats globally. Proc. Natl. Acad. Sci. United States America 111 (43), 15462–15467. doi: 10.1073/pnas.1411477111
Cresson P., Direach L. E., Rouanet E., Goberville E., Astruch P., Ourgaudd M., et al. (2019). Functional traits unravel temporal changes in fish biomass production on artificial reefs. Mar. Environ. Res. 145, 137–146. doi: 10.1016/j.marenvres.2019.02.018
Cresson P., Ruitton S., Fontaine M. F., Harmelin-Vivien M. (2012). Spatio-temporal variation of suspended and sedimentary organic matter quality in the bay of marseilles (nw mediterranean) assessed by biochemical and isotopic analyses. Mar. pollut. Bulletin. 64 (6), 1112–1121. doi: 10.1016/j.marpolbul.2012.04.003
Cresson P., Ruitton S., Harmelin-Vivien M. (2014). Artificial reefs do increase secondary biomass production: mechanisms evidenced by stable isotopes. Mar. Ecol. Progress. 509 (5), 15–26. doi: 10.3354/meps10866
Cui Y., Wu Y., Jing Z., Wang N. (2012). Potential dietary influence on the stable isotopes and fatty acid compositions of jellyfishes in the yellow Sea. J. Mar. Biol. Assoc. UK. 92, 1325–1333. doi: 10.1017/S0025315412000082
Decottignies P., Beninger P. G., Rincé Y., Riera P. (2007). Trophic intractions between two introduced suspension-feeders, Crepidula fornicata and Crassostrea gigas, are influenced by seasonal effects and qualitative selection capacity. J. Exp. Mar. Biol. Ecol. 342, 231–241. doi: 10.1016/j.jembe.2006.10.005
Deng J. Y., Jiang W. M., Yang J. M., Li J. (1997). Species interaction and food web structure of major predator species in the bohai Sea. J. Fishery Sci. China 4 (4), 1–7. doi: CNKI: SUN: ZSCK.0.1997-04-000
DeNiro M. J., Epstein S. (1981). Influence of diet on the distribution of nitrogen isotopes in animals. Geochimica Cosmochimica Acta 45, 341–351. doi: 10.1016/0016-7037(81)90244-1
Feng J., Tian X. L., Dong S. L., Li D. S., He R. P., Zhang K., et al. (2018). Trophic interaction in a Portunus rituberculatus polyculture ecosystem based on carbon and nitrogen stable isotope analysis. J. Ocean Univ. China. 017 (6), 1432–1440. doi: 10.1007/s11802-018-3655-y
Feng J. X., Gao Q. F., Dong S. L., Sun Z. L., Zhang K. (2014). Trophic relationships in a polyculture pond based on carbon and nitrogen stable isotope analyses: A case study in jinghai bay, China. Aquaculture s428–429 (11), 258–264. doi: 10.1016/j.aquaculture.2014.03.008
France R. L. (1995). Carbon-13 enrichment in benthic compared to planktonic algae: food web implications. Mar. Ecol. Prog. Series. 124, 307–312. doi: 10.3354/meps124307
Fry B., Arnold C. (1982). Rapid 13C/12C turnover during growth of brown shrimp (Penaeus aztecus). Oecologia (Berl). 54, 200–204. doi: 10.1007/BF00378393
Fry B., Wainright S. C. (1991). Diatom sources of 13c-rich carbon in marine food webs. Mar. Ecol. Prog. Series. 76 (2), 149–157. doi: 10.3354/meps076149
Gearing J. N., Gearing P. J., Rundnick D. T., Requejo A. G., Hutchins M. J. (1984). Isotopic variability of organic-carbon in a phytoplankton-based, temperate estuary. Geochimica Et Cosmochimica Acta 48 (5), 1089–1098. doi: 10.1016/0016-7037(84)90199-6
Grégori G., Colosimo A., Denis M. (2001). Phytoplankton group dynamics in the bay of Marseilles during a 2-year survey based on analytical flow cytometry. Cytometry 44, 247–256. doi: 10.1002/1097-0320(20010701)44:3<247::AID-CYTO1117>3.0.CO;2-Z
Grey J. (2016). The incredible lightness of being methane-fueled: stable isotopes reveal alternative energy pathways in aquatic ecosystems and beyond. Front. Ecol. Evolution. 4. doi: 10.3389/fevo.2016.00008
Grossman G. D., Jones G. P., Seaman W. J. (1997). Do artificial reefs increase regional fish production? a review of existing data. Fisheries 22, 17–23. doi: 10.1577/1548-8446(1997)0222.0.CO;2
Guo X. W., Tang Q. (2000). Consumption and ecological conversion efficiency of. S. Thrissa kammalensis. J. Fisheries China. 24 (5), 422–427. doi: 10.3321/j.issn:1000-0615.2000.05.007
Gusha M., Dalu T., Mcquaid C. D. (2021). Interaction between small-scale habitat properties and short-term temporal conditions on food web dynamics of a warm temperate intertidal rock pool ecosystem. Hydrobiologia 848, 1517–1533. doi: 10.1007/s10750-021-04535-8
Harmelin J. G., Bellan-Santini D. (1997). “Assessment of biomass and production of artificial reef communities,” in European Artificial reef research, proceedings of the 1st EARRN conference. Ed. Jensen A. C. (Southampton Oceanography Centre, Ancona: Chapman and Hall), 305–322.
Harmelin-Vivien M., Loizeau V., Mellon C., Beker B., Arlhac D., Bodiguel X., et al. (2008). Comparison of c and n stable isotope ratios between surface particulate organic matter and microphytoplankton in the gulf of lions (NW Mediterranean). Cont. Shelf Res. 28 (15), 1911–1919. doi: 10.1016/j.csr.2008.03.002
Hixon M. A., Beets J. P. (1989). Shelter characteristics and caribbean fish assemblages: experiments with artificial reefs. Bull. Mar. Science. 44 (2), 666–680. doi: 10.1515/botm.1989.32.2.181
Hobson K., Welch H. (1992). Determination of trophic relationships within a high Arctic marine food web using δ13C and δ15N analysis. Mar. Ecol. Prog. Series. 84, 9–18. doi: 10.3354/meps084009
Hobson K. A., Welch H. E. (1992). Determination of trophic relationships within a high artic marine food web using δ13C and δ15N analysis. Mar. Ecol. Prog. Series. 84, 9–18. doi: 10.3354/meps084009
Hueckel G. J., Buckley R. M. (1987). The influence of prey communities on fish species assemblages on artificial reefs in puget sound, Washington. Environ. Biol. Fish. 19 (3), 195–214. doi: 10.1007/BF00005349
Ji D. P. (2014). Study on the fishery biology of hexagrammos agrammus and hexagrammos otakii in lidao rongcheng, doctoral dissertation (Qingdao: Ocean University of China).
Jin X. S., Dou S. Z., Shan X. J., Wang Z. Y., Wang R. J., Bian X. D. (2015). Hot spots of frontiers in the research of sustainable yield of Chinese inshore fishery. Prog. Fish. Sci. 36 (1), 124–131. doi: 10.11758/yykxjz.20150119
Kang C. K., Choy E. J., Hur Y. B., Myeong J. (2009). Isotopic evidence of particle size-dependent food partitioning in cocultured sea squirt Halocynthia roretzi and pacific oyster Crassostrea gigas. Aquat. Biol. 6 (1), 289–302. doi: 10.3354/ab00126
Kurata K., Minami H., Kikuchi E. (2001). Stable isotope analysis of food sources for salt marsh snails. Mar. Ecol. Prog. Ser. 223 (1), 167–177. doi: 10.3354/meps223167
Lei Y. Z. (2006). Aquaculture water environmental chemistry experiment (Beijing: China Agriculture Press), 49–56.
Letourneur Y., Lison de Loma T., Richard P., Harmelin-Vivien M. L., Cresson P., Banaru D., et al. (2013). Identifying carbon sources and trophic position of coral reef fishes using diet and stable isotope (δ15N and δ13C) analyses in two contrasted bays in moorea, French Polynesia. Coral Reefs. 32 (4), 1091–1102. doi: 10.1007/s00338-013-1073-6
Lin H. J., Qin C. X., Li X. G., Chen P. M., Mo B. L., Ma H., et al. (2018). Food web analysis in zhelin bay marine ranching. J. Fish. China. 1026–1039 42 (7), 1026–2039. doi: 10.11964/jfc.20170410814
Lin C. G., Yang H. S., Chen Y., Jin X. S., Chen B., Li W., et al. (2020). Construction and development of modern marine ranching–academic review of the 230th shuangqing forum [J/OL]. Bull. Natl. Natural Sci. Foundation China 35 (1), 143–152. doi: 10.16262/j.cnki.1000-8217.20200825.005
Liu J. Y. (2013). Status of marine biodiversity of the China seas. PloS One 8 (1), e50719–e50719. doi: 10.1371/journal.pone.0050719
Lorenzen C., Jeffrey S. (1980). Determination of chlorophyll in seawater. UNESCO Tech. Pap. Mar. Science. 35, 1–20. doi: 10.1556/Nano.2008.00001
Mao Z. G., Gu X. H., Zeng Q. F., Pan G. (2014). Seasonal and spatial variations of the food web structure in a shallow eutrophic lake assessed by stable isotope analysis. Fish. Science. 80 (5), 1045–1054. doi: 10.1007/s12562-014-0771-5
Marín Leal J. C., Dubois S., Orvain F., Galois R., Blin J. L., Ropert M., et al. (2008). Stable isotopes (δ13C, δ15N) and modeling as tools to estimate the trophic ecology of cultivated oysters in two contrasting environments. Mar. Biol. 153, 673–688. doi: 10.1007/s00227-007-0841-7
Michener R. H., Schell D. M. (1994). "Stable isotope ratios as tracers in marine aquatic food webs". Stable Isotopes Ecol. Environ. Sci. (Oxford, UK), 138–158.
Minagawa M., Wada E. (1984). Stepwise enrichment of δ15N along food chains: further evidence and the relation between δ15N and animal age. Geochimica Et Cosmochimica Acta 48 (5), 1135–1140. doi: 10.1016/0016-7037(84)90204-7
Okano T., Takeda M., Nakagawa Y., Hirata K., Mitsuhashi K., Kawaguchi S., et al. (2011). “Artificial reefs to induce upwelling to increase fishery resources”. in Artificial Reefs in Fisheries Management Bortone S.A., Brandini F.P., Fabi G., Otake S. (Boca Raton, FL: CRC Press LLC), 265–278.
Okumura S., Kayano Y., Kusaka K., Tsumura S., Maruyama K. (2003). Experimental release of juvenile red spotted grouper Epinephelus akaara into the artificial reefs made of scallop Patinopecten yessoensis shells. Nsugaf 69 (6), 917–925. doi: 10.2331/suisan.69.917
Padilla D. K. (2010). Context-dependent impacts of a non-native ecosystem engineer, the pacific oyster Crassostrea gigas. Integr. Comp. Biol. 50 (2), 213–225. doi: 10.1093/icb/icq080
Park K. D., Kang Y. J., Huh S. H., Kwak S. N., Lee H. W. (2007). Feeding ecology of sebastes schlegeli in the tongyeong marine ranching area. Korean J. Fish. Aquat. Sci. 40 (5), 1–7. doi: 10.5657/kfas.2007.40.5.308
Parnell A. C., Inger R., Bearhop S., Jackson A. L. (2010). Source partitioning using stable isotopes: coping with too much variation. PloS One 5 (3), e9672. doi: 10.1371/JOURNAL.PONE.0009672
Peterson B. J., Fry B. (1987). Stable isotopes in ecosystem studies. Annu. Rev. Ecol. System. 18, 293–320. doi: 10.1146/annurev.es.18.110187.001453
Phillips D. L., Inger R., Bearhop S., Jackson A. L., Moore J. W., Parnell A. C., et al. (2014). Best practices for use of stable isotope mixing models in food web studies. Can. J. Fish. Aquat. Sci. 835, 823–835. doi: 10.1139/cjz-2014-0127
Pitcher T. J., Seaman W. J. (2000). Petrarch’s principle: how protected human-made reefs can help the reconstruction of fisheries and marine ecosystems. Fish Fish. 1, 73–81. doi: 10.1046/j.1467-2979.2000.00010.x
Polovina J. J., Sakai I. (1989). Impacts of artificial reefs on fishery production in shimarmaki, Japanese. Bull. Mar. Sci. 44, 997–1003.
Post D. M. (2002). Using stable isotopes to estimate trophic position: models, methods, and assumptions. Ecology 83 (3), 703–718. doi: 10.1890/0012-9658(2002)083[0703:USITET]2.0.CO;2
Powers S. P., Grabowski J. H., Peterson C. H., Lindberg W. J. (2003). Estimating enhancement of fish production by offshore artificial reefs: uncertainty exhibited by divergent scenarios. Mar. Ecol. Prog. Ser. 264, 265–277. doi: 10.3354/meps264265
Prince E. D., Gotshall D. W. (1976). Food of the copper rockfish, Sebastes caurinus Richardson, associated with an artificial reef in south Humboldt bay, California. California Fish Game. 62, 274–285.
Qi L. (2017). Study on characterization of three digestive enzymes and preliminary purification of salivary gland protease from Rapana venosa (Qingdao: Master dissertation, ShanDong University).
Qu P., Wang Q., Pang M., Zhang Z., Liu C., Tang X. X. (2016). Trophic structure of common marine species in the bohai strait, north china sea, based on carbon and nitrogen stable isotope ratios. Ecol. Indicators. 66, 405–415. doi: 10.1016/j.ecolind.2016.01.036
Rau G. H., Teyssie J. L., Rassoulzadegan F., Fowler S. W. (1990). 13C/12C and 15N/14N variations among size-fractionated marine particles: implications for their origin and trophic relationships. Mar. Ecol. Prog. Series. 59, 33–38. doi: 10.3354/meps059033
Riisgard H. U., Larsen P. S. (2000). Comparative ecophysiology of active zoobenthic filter feeding, essence of current knowledge. J. Sea Res. 44, 169–193. doi: 10.1016/S1385-1101(00)00054-X
Sahm R., Sünger E., Burmann L., Zubrod J. P., Schulz R., Fink P. (2021). Compound-specific δ15N analyses of amino acids for trophic level estimation from indigenous and invasive freshwater amphipods. Int. Rev. Hydrobiol. 106, 41–47. doi: 10.1002/iroh.202002058
Sato T., Miyajima T., Ogawa H., Yu U., Koike. I. (2006). Temporal variability of stable carbon and nitrogen isotopic composition of size-fractionated particulate organic matter in the hypertrophic sumida river estuary of Tokyo bay, Japan. Estuar. Coast. Shelf Sci. 68 (1/2), 245–258. doi: 10.1016/j.ecss.2006.02.007
Slater M. J., Jeffs A. G., Carton A. G. (2009). The use of the waste from green-lipped mussels as a food source for juvenile sea cucumber, Australostichopus mollis. Aquaculture 292, 219–224. doi: 10.1016/j.aquaculture.2009.04.027
Smith J. A., Lowry M. B., Champion C., Suthers I. M. (2016). A designed artificial reef is among the most productive marine fish habitats: new metrics to address “production versus attraction. Mar. Biol. 163, 188. doi: 10.1007/s00227-016-2967-y
Song J. P., Fang J. B., Song H., Zhang T., Ban S. J., Pan Y., et al. (2016). Effect of feeding preferences, temperature, and size on food intake of Rapana venosa. Mar. Sci. 1, 48–53. doi: CNKI:SUN:HYKX.0.2016-01-007
Sriwoon R., Pholpunthin P., Lirdwitayaprasit T., Kishino M., Furuya K. (2010). Population dynamics of green Noctiluca scintillans (Dinophyceae) associated with the monsoon cycle in the upper gulf of Thailand. J. Phycol. 44 (3), 605–615. doi: 10.1111/j.1529-8817.2008.00516.x
Stewart S. D., Kelly D., Biessy L., Laroche O., Wood S. A. (2021). Individual diet specialization drives population trophic niche responses to environmental change in a predator fish population. Food Webs. 27 (6), e00193. doi: 10.1016/j.fooweb.2021.e00193
Sugie K., Suzuki K. (2015). Size of dominant diatom species can alter their evenness. PloS One 10 (6), e0131454. doi: 10.1371/journal.pone.0131454
Sun Z. L., Gao Q. F., Dong S. L., Shin P. K. S., Wang F. (2012). Estimates of carbon turnover rates in the sea cucumber Apostichopus japonicus (Selenka) using stable isotope analysis: the role of metabolism and growth. Mar. Ecol. Progress. 457, 101–112. doi: 10.3354/meps09760
Uhlig G., Sahling G. (1990). Long-term studies on N. scintillans in the German bight population dynamics and red tide phenomena. Netherlands J. Sea Res. 25, 101–112. doi: 10.1016/0077-7579(90)90012-6
Ushiama S., Smith J. A., Suthers I. M., Lowry M., Johnston E. L. (2016). The effects of substratum material and surface orientation on the developing epibenthic community on a designed artificial reef. Biofouling 32 (9-10), 1049–1060. doi: 10.1080/08927014.2016.1224860
Vanderklift M. A., Ponsard S. (2003). Sources of variation in consumer-diet ?15N enrichment: a meta-analysis. Oecologia 136, 169–182. doi: 10.1007/s00442-003-1270-z
Vander Zanden M. J., Rasmussen J. B. (2001). Variation in δ15N and δ13C trophic fractionation: implications for aquatic food web studies. Limnol. Oceanogr. 46 (8), 2061–2066. doi: 10.4319/lo.2001.46.8.2061
Walker S. J., Schlacher T. A., Schlacher-Hoenlinger M. A. (2010). Spatial heterogeneity of epibenthos on artificial reefs: fouling communities in the early stages of colonization on an East Australian shipwreck. Mar. Ecol. 28 (4), 435–445. doi: 10.1111/j.1439-0485.2007.00193.x
Wei S., Jiang W. M. (1992). Study on food web of fishes in the yellow sea. Oceanol. Limnol. Sinica. 23 (2), 182–192.
Wei H. J., Zhu X. M., Ji Y. N., Jiang Y. Z., Lin N., Wang Y. L. (2013). Study on the food web structure and their trophic levels of marine ranching area in xiangshan harbor. J. Appl. Oceanogr. 32 (2), 250–257. doi: 10.3969/J.ISSN.2095-4972.2013.02.015
Wiencke C., Fischer G. (1990). Growth and stable carbon isotope composition of cold-water macroalgae in relation to light and temperature. Mar. Ecol. Prog. Ser. 65 (3), 283–292. doi: 10.3354/meps065283
Wilson J., Osenberg C. W., Mary C. M. S., Watson C. A., Lindberg W. J. (2001). Artifcial reefs, the attraction–production issue, and density dependence in marine ornamental fshes. Aquac. Aquar. Conserv. 3, 95–105. doi: 10.1023/A:1011343312031
Wu C. W., Dong Z. Y., Chi C. F., Ding F. (2010). Reproductive and spawning habits of Sepiella maindroni off zhejiang,china. Oceanol. Et Limnol. Sinica. 41 (1), 39–46. doi: CNKI:SUN:HYFZ.0.2010-01-006
Xie B., Li Y. K., Zhang H., Zhang S. (2017). Food web foundation and seasonal variation of trophic structure based on the stable isotopic technique in the marine ranching of haizhou bay, China. Chin. J. Appl. Ecol. 28 (7), 2292–2298. doi: 10.13287/j.1001-9332.201707.019
Xu F. X. (2018). The UAV and multi-resource data-based research on green tide monitoring in the yellow Sea, doctoral dissertation (Beijing: University of Chinese Academy of Sciences).
Xu Q. Z., Zhang L. B., Zhang T., Zhang X. L., Yang H. S. (2017). Functional groupings and food web of an artificial reef used for sea cucumber aquaculture in northern China. J. Sea Res. 119, 1–7. doi: 10.1016/j.seares.2016.10.005
Yang J. M. (2001). A study on food and trophic levels of bohai sea fish. Mod. Fish. Inf. 16 (9), 10–19. doi: CNKI: SUN: XYYZ.0.2001-10-001
Yang M. J., Feng J., Yu Z. L., Song H., Hu Z., Zhou C., et al. (2020). Comparative analysis of the feeding habits of Rapana venosa and Neptunea arthritica cumingii near zhangzi island, China, based on stable isotope ratios and fatty acid profiles. Aquacult. Res. 00, 1–9. doi: 10.1111/are.15033
Yang H. S., Huo D., Xu Q. (2016). Views on modern marine ranching. Oceanol. Limnol. Sinica. 47 (6), 1069–1074. doi: 10.11693/hyhz20160900203
Yang H. S., Yang X. Y., Lin C. G., Zhang L. B., Xu Q. (2018). Strive to realize modernization of concept, equipment, technology, and management of modern marince ranching development. Bull. Chin. Acad. Sci. 33 (7), 732–738. doi: 10.16418/j.issn.1000-3045.2018.07
Yokohama H. (2013). Suspended culture of the sea cucumber Apostichopus japonicus below a Pacific oyster raft – potential for integrated multi-trophic aquaculture. Aquaculture Research 46 (4), 1–8. doi: 10.1111/are.12234
Yuan X. T., Yang H. S., Zhou Y., Mao Y. Z., Wang L. L. (2008). Bioremediation potential of apostichopus japonicas (selenka) in coastal bivalve suspension aquaculture system. J. Appl. ecol. 19 (4), 866–872. doi: CNKI: SUN: YYSB.0.2008-04-028
Zalmon I. R., Sa F. S. D., Neto E. J. D., Rezende C. E. D., Mota P. M., Almeida T. C. M. D. (2014). Impacts of artificial reef spatial configuration on infaunal community structure — southeastern Brazil. J. Exp. Mar. Biol. Ecol. 454, 9–17. doi: 10.1016/j.jembe.2014.01.015
Zamora L., Dollimore J., Jeffs A. (2014). Feasibility of co-culture of the australasian sea cucumber (Australostichopus mollis) with the pacific oyster (Crassostrea gigas) in northern new zealand. New Z. J. Mar. Freshw. Res. 48 (3), 394–404. doi: 10.1080/00288330.2014.901232
Zhang T., Feng J., Song H. (2020). Principles and technology of biological resources maintenance in marine ranching. Sci. Technol. Dev. 16 (2), 206–212. doi: 10.11842/chips.20200316003
Zhang B. L., Guo B., Yu Y., Xu X. F., Wang S. (2019). Study of the food-web structure in the dashentang artificial reef area in tianjin using a stable isotope technique. Prog. Fish. Sci. 40 (6), 25–35. 10.19663/j.issn2095-9869.20180912001
Zhang B., Li Z. Y., Jin X. S. (2014). Food composition and prey selectivity of Sebastes schlegeli. J. Fish. Sci. China 21 (1), 134–141. doi: 10.3724/SP.J.1118.2014.00134
Zhang B. L., Sun D. Y., Bi H. S., Wu Y. Q., Huang B. (1997). Growth and seasonal distribution of sepiella maindroni in the jiaozhou bay and adjacent waters. Mar. Sci 5, 61–64. doi: CNKI:SUN:HYKX.0.1997-05-020
Zhang Y. Q., Xu Q., Alós J., Liu H., Xu Q. Z., Yang H. S. (2015). Short-term fidelity, habitat use and vertical movement behavior of the black rockfish Sebastes schlegelii as determined by acoustic telemetry. PloS One 10 (8), e0134381. doi: 10.1371/journal.pone.0134381
Zhang Z. P., Wang M. Q., Song J. J., Zhao W. X., Yu D. D., Tang X. X., et al. (2020a). Food web structure prior to and following construction of artificial reefs, based on stable isotope analysis. Regional Stud. Mar. Science. 37, 1–11. doi: 10.1016/j.rsma.2020.101354
Zhang Y. Q., Xu Q., Xu Q. Z., Alós J., Zhang H. Y., Yong H. L. (2018). Dietary composition and trophic niche partitioning of spotty-bellied greenlings Hexagrammos agrammus, fat greenlings H. otakii, Korean rockfish Sebastes schlegelii, and Japanese seaperch Lateolabrax japonicus in the yellow Sea revealed by stomach content analysis and stable isotope analysis. Mar. Coast. Fish. 10 (2), 255–268. doi: 10.1002/mcf2.10019
Zhang R. L., Zhang H., Liu H., Zhan J. M. (2021). Differences in trophic structure and trophic pathways between artificial reef and natural reef ecosystems along the coast of the north yellow sea, China, based on stable isotope analyses. Ecol. Indicators. 125, 1–13. doi: 10.1016/j.ecolind.2021.107476
Keywords: artificial reef, food web, stable isotope, marine ranching, system function
Citation: Feng J, Zhao X, Bi F, Zhao W, Zhao L, Song H, Yang M, Hu Z, Zhou C, Shi P, Hu P, Ma P, Sun P, Jiang H, Xu J and Zhang T (2023) Investigating food web structure and system function of an artificial reef ecosystem based on carbon and nitrogen stable isotope analysis: implications for reef management. Front. Mar. Sci. 10:1192173. doi: 10.3389/fmars.2023.1192173
Received: 23 March 2023; Accepted: 12 April 2023;
Published: 10 May 2023.
Edited by:
Kai Zhang, Chinese Academy of Fishery Sciences, ChinaReviewed by:
Bin Xia, Qingdao Agricultural University, ChinaBo Zhou, The Fishery Institute of the Sichuan Academy of Agricultural Sciences, China
Copyright © 2023 Feng, Zhao, Bi, Zhao, Zhao, Song, Yang, Hu, Zhou, Shi, Hu, Ma, Sun, Jiang, Xu and Zhang. This is an open-access article distributed under the terms of the Creative Commons Attribution License (CC BY). The use, distribution or reproduction in other forums is permitted, provided the original author(s) and the copyright owner(s) are credited and that the original publication in this journal is cited, in accordance with accepted academic practice. No use, distribution or reproduction is permitted which does not comply with these terms.
*Correspondence: Jianglin Xu, xu_jiangling@163.com; Tao Zhang, zhangtaocas@126.com