Connectivity between white shark populations off Central California, USA and Guadalupe Island, Mexico
- 1Depatment of Ecology, Montana State University, Bozeman, MT, United States
- 2California White Shark Project, Inverness, CA, United States
- 3Monterey Bay Aquarium, Monterey, CA, United States
- 4Coastal Oregon Marine Experiment Station, Oregon State University, Newport, OR, United States
- 5Pelagios-Kakunjá, La Paz, BCS, Mexico
- 6Fins Attached, Colorado Springs, CO, United States
- 7Department of Wildlife, Fish, and Conservation Biology, University of California, Davis, CA, United States
- 8Instituto Politécnico Nacional, Centro Interdisciplinario de Ciencias Marinas (CICIMAR), La Paz, BCS, Mexico
- 9Department of Biology, Hopkins Marine Station, Stanford University, Pacific Grove, CA, United States
- 10Department of Marine Science, California State University, Monterey Bay, CA, United States
Marine animals often move beyond national borders and exclusive economic zones resulting in a need for trans-boundary management spanning multiple national jurisdictions. Highly migratory fish vulnerable to over-exploitation require protections at international level, as exploitation practices can be disparate between adjacent countries and marine jurisdictions. In this study we collaboratively conducted an analysis of white shark connectivity between two main aggregation regions with independent population assessment and legal protection programs; one off central California, USA and one off Guadalupe Island, Mexico. We acoustically tagged 326 sub-adult and adult white sharks in central California (n=210) and in Guadalupe Island (n=116) with acoustic transmitters between 2008-2019. Of the 326 tagged white sharks, 30 (9.20%) individuals were detected at both regions during the study period. We used a Bayesian implementation of logistic regression with a binomial distribution to estimate the effect of sex, maturity, and tag location to the response variable of probability of moving from one region to the other. While nearly one in ten individuals in our sample were detected in both regions over the study period, the annual rate of trans-regional movement was low (probability of movement = 0.015 yr-1, 95% credible interval = 0.002, 0.061). Sub-adults were more likely than adults to move between regions and sharks were more likely to move from Guadalupe Island to central California, however, sex, and year were not important factors influencing movement. This first estimation of demographic-specific trans-regional movement connecting US and Mexico aggregations with high seasonal site fidelity represents an important step to future international management and assessment of the northeastern Pacific white shark population as a whole.
1 Introduction
Informed wildlife resource use and management decisions are derived from an understanding of population structure and life-history parameters. Demarcating populations of marine animals on ocean-basin scales can be difficult due to large home ranges and inability to observe animals directly in the ocean habitat. However, advances in tagging technologies have increased our capacity to observe movements and ocean-scale migratory paths for multiple species across taxa (Block et al., 1998; Block et al., 2005; Block et al., 2011). These technologies have provided bounds on many highly mobile species, indicating predictable species-specific migratory pathways and high-use areas where focused sampling efforts can provide important population characteristic data.
The white shark (Carcharodon carcharias) is an apex marine predator with a circumglobal distribution. Tagging studies in tandem with genetic techniques provide evidence that natal philopatry is strong (Jorgensen et al., 2010), which results in discrete population structure with clearly defined population segments that have been identified in South Africa (Pardini et al., 2000), Australia-New Zealand (Blower et al., 2012), the northeast (Jorgensen et al., 2010; Bernard et al., 2018) and northwest Pacific Ocean (Tanaka et al., 2011), the Mediterranean Sea (Gubili et al., 2010), and the northwest Atlantic Ocean (O’Leary et al., 2015). Within the northeastern Pacific segment, estimated to be isolated from other Pacific populations for some 200k years (Jorgensen et al., 2010; Bernard et al., 2018), evidence for further genetic structure is mixed. Indications of mitochondrial DNA structure between Central California, USA and Guadalupe Island, Mexico reported in one study (Oñate-González et al., 2015) run contrary to a second study which found no Mitochondrial DNA structure (Santana-Morales et al., 2020). A third transcriptome-derived microsatellite study also reported a lack of support for NEP substructure (Bernard et al., 2018) leaving the question of connectivity across the U.S. – Mexico regions inconclusive.
Off the western U.S. coast, white sharks in the northeastern Pacific are thought to give birth in the Southern California Bight (area between Point Conception and San Diego). This assumption is based on incidental catches of neonate and young-of-the-year white sharks (120 -150 cm) in near-shore waters (<3 nm from shore,<50 m depth) from gill-net fisheries that target other fishes such as California Halibut (Paralichthys californicus), White Seabass (Atractoscion nobilis), and Pacific Angel Shark (Squatina californica) (Klimley, 1985; Lowe et al., 2012; Lyons et al., 2013). Just to the south in Baja, Mexico, a nursery ground has been described in Bahia Sebastian Vizcaino (Oñate-González et al., 2017). Additional nursery areas in the Gulf of California have been proposed based on the movement of large females, although captures of young-of-the-year sharks there are rare (Galván-Magaña et al., 2011; Domeier and Nasby-Lucas, 2013) White sharks are hypothesized to spend ~three years in these nursery grounds feeding upon cephalopods, teleosts and elasmobranchs until they reach lengths of ~ 200-250 cm. As larger juveniles and sub-adults these white sharks recruit primarily to the north of Point Conception, CA and tags along with photo ID indicates they are present in central California or to Guadalupe Island ~250 km offshore of northern Mexico, with large aggregations of pinnipeds residing in both regions (Weng et al., 2007a; Oñate-González et al., 2017).
Multiple tagging studies (Boustany et al., 2002; Weng et al., 2007a; Domeier and Nasby-Lucas, 2008; Jorgensen et al., 2010; Domeier and Nasby-Lucas, 2012) have provided evidence that sub-adult and adult white sharks undertake predictable seasonal migrations in the northeastern Pacific between three main focal areas: (i) North American shelf waters, (ii) the slope and offshore waters of the Hawaiian archipelago, and (iii) the offshore white shark Café, located ~ 1500 km offshore between Baja, Mexico and Hawaii, USA (Weng et al., 2007a; Domeier and Nasby-Lucas, 2008; Jorgensen et al., 2010; Andrzejaczek et al., 2022). While sharks from both coastal regions overlap in the White Shark Café and Hawaii, satellite and acoustic tagged white sharks have been shown to consistently return to their respective tagging region in central California or Guadalupe Island (Domeier and Nasby-Lucas, 2008; Jorgensen et al., 2010; Chapple et al., 2016).
Adults from both locations predictably travel offshore and have similar timing for departure from and return to coastal areas (Domeier and Nasby-Lucas, 2008; Jorgensen et al., 2010). Sub-adults in Guadalupe Island, however, mostly remain coastal (Hoyos-Padilla et al., 2016), and sub-adults that do travel offshore do not appear to have offshore movements that are synchronized with seasonal departures by adults to offshore areas (Domeier, 2012). The onset of migratory behavior remains largely unknown for the sub-adult phase of life for white sharks in central California due to a scarcity of research on this specific transition phase. In both regions, transition of sub-adults making temporally predictable and direct migrations remains unclear but could be a cumulative experience of annual attempts to hone in on an efficient migratory pattern.
Prior to recruitment at adult aggregating sites, tagging indicates that some juvenile white sharks seasonally migrate back and forth between Vizcaino Bay, Mexico and Southern to central California (Weng et al., 2007a; Benson et al., 2018). Additionally, five acoustically tagged sub-adult sharks were detected in both central California and Guadalupe Island (Jorgensen et al., 2012a; Hoyos-Padilla et al., 2016). To date, a comprehensive analysis of tagging data has been lacking across both regions to quantify the extent of the connectivity, its frequency, and the potential demographic factors (e.g. sex, year, size).
To date, assessments of the NEP white shark population have been conducted separately for central California (Chapple et al., 2011; Kanive et al., 2021) and Guadalupe Island (Sosa-Nishizaki et al., 2012). To date, assessments of the entire NEP segment, along with the potential for source/sink or rescue effects between these two regions remains an important conservation goal. Estimating abundance for the combined northeastern Pacific region could be artificially inflated if individuals use both regions and were counted twice. Therefore, it is important to accurately define annual rates of overlap between both regions to accurately quantify multi-regional population parameters.
Quantifying the connectivity between these two transboundary regions needs to be considered when making informed management decisions about the overall regional population size. Additionally, jurisdictional differences between Mexico and the US are important to consider in monitoring the population structure and trajectory of this protected species. In this study, we conducted a comprehensive analysis of acoustic tagging data collected over 12 years of two populations that bound international waters. Through collaboration between several research groups using parallel tagging methods and technology, we quantify movement between these two important aggregations in USA and Mexico and explore demographic-specific migration rates and drivers of movement for sub-adult and adult white sharks.
2 Materials and methods
2.1 Study area and tagging methods
From 2008 to 2018, we acoustically tagged sharks at known sub-adult and adult aggregation regions in California, USA (Southeast Farallon Island, Año Nuevo Island, and Tomales Point) and Guadalupe Island, Mexico (Figure 1) during periods (September – February) of peak coastal residency (Klimley, 1985; Jorgensen et al., 2010). White sharks were attracted to a research boat using a seal decoy, and motivated to circle the boat with a small (<2kg) piece of salvaged marine mammal blubber tethered against the boat at the water line (Kanive et al., 2015) in central California. In Guadalupe Island similar techniques are used but the attraction is a whole yellowfin tuna or portions of the fish. Individual sharks were identified from photo and video images of the natural and unique patterns on the trailing edge of their dorsal fin as fin morphological patterns have been validated for stability over periods exceeding 25 years (Anderson et al., 2011). Total lengths of the sharks were estimated from one to three experienced researchers using the known length of the research vessel as a reference measurement as the shark swam close to the vessel. If there was a disparity in an estimate of the total length among the researchers, the mean was used as the estimate. Maturity was assigned based on published values where adult males ≥ 380cm total length (TL), sub-adult males < 380cm TL, adult females ≥ 440cm TL, and sub-adult females < 440cm TL (Francis, 1996; Pratt, 1996). Sex was determined by the presence (male) or absence (female) of claspers.
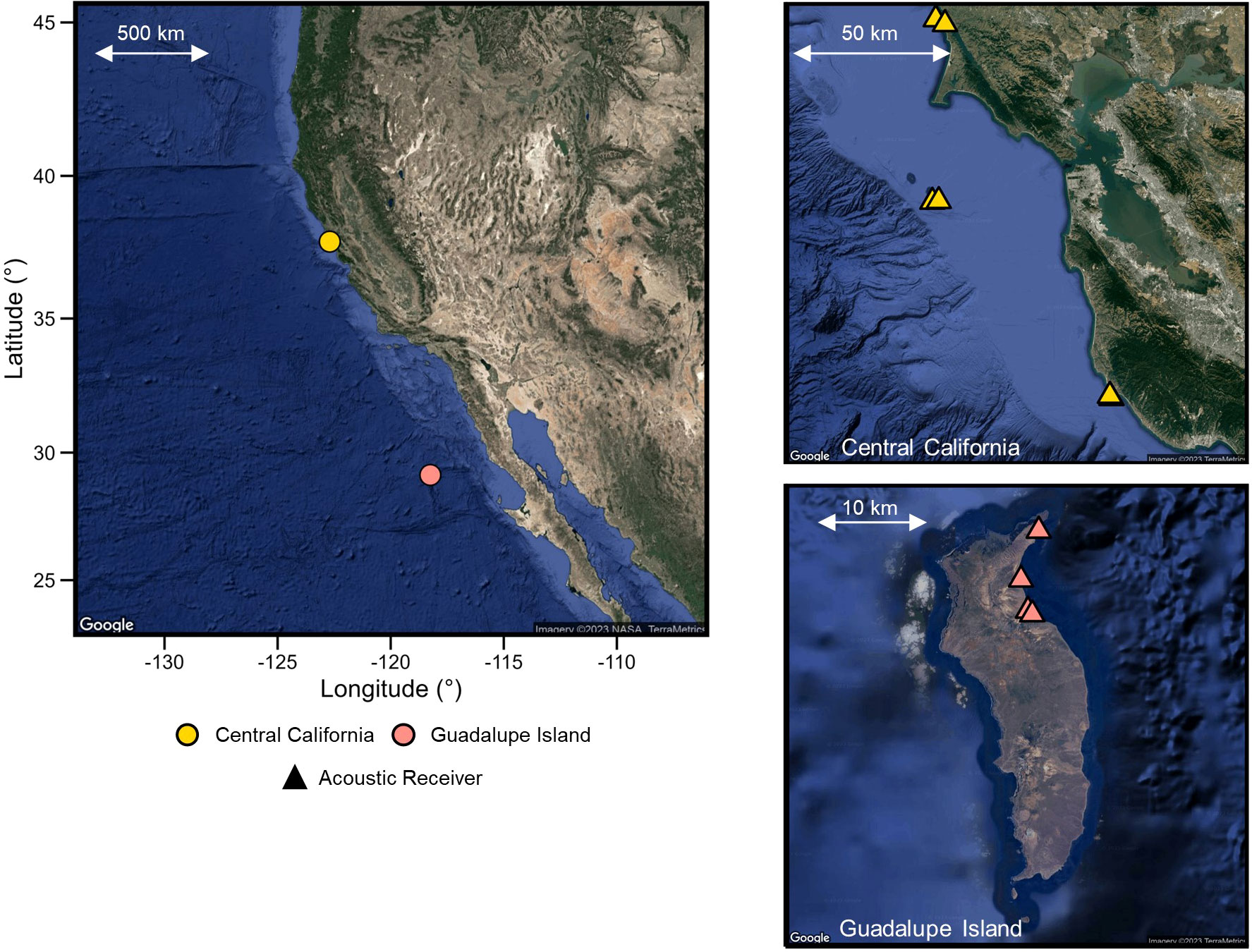
Figure 1 Map of the Northeast Pacific that includes the seasonal coastal aggregation sites of white sharks off central California, USA (yellow) and Guadalupe Island, Mexico (red). Circles represent coastal/island aggregation sites. Triangles indicate receiver locations at each study site.
Free-swimming sharks were tagged with individually coded acoustic transmitter tags (V16-4H; and V16-6H; Innova Sea, Inc formerly Vemco, Halifax, Nova Scotia) in central California using a 59 mm titanium dart with an 18-20 cm 136 kg test monofilament leader protected by hollow braided Dacron and shrink-wrap (Jorgensen et al., 2010; Wilson et al., 2015). The darts were inserted into the dorsal musculature using a tagging pole (Boustany et al., 2002; Weng et al., 2007a) as the shark swam by the research vessel. In Guadalupe Island, sharks were tagged with individually coded acoustic transmitter tags (V16-4H; Vemco, Halifax, Nova Scotia) using a stainless steel dart and a steel leader with shrink-wrap.
Acoustic receivers (Vemco; VR-2, VR-3UM, VR-4UM) were placed at known white shark aggregation sites in central California and Guadalupe Island (Figure 1). These receivers archived the acoustic detections of each shark with an individual acoustic number as they swam within ~500 m of the receiver. The data from the receivers could then be downloaded in situ (VR-3), physically retrieved (VR-2) or remotely transmitted (VR-4UM).
2.2 GLMM analysis
We modeled the probability of transitioning from one region (Guadalupe Island or central California) site to the other using logistic regression with a binomial distribution for the response variable (moved from one aggregation site [central California or Guadalupe Island] to the other) and a logit-link between the response variable and covariates of interest. We used a Bayesian approach to implement one general model that included five features that we predicted might be associated with variation in an individual’s probability of movement. These included sex, maturity class, and the aggregation site at which it was observed at the start of the binomial trial. Because some individuals provided data for multiple binomial trials and because multiple individuals were studied within a year, we also included random effect of individual and year in our models. As the annual migratory pattern consists of an offshore phase followed by a coastal phase, our model defines a ‘movement’ as either (i) a shark migrates offshore from one region and returns to the coast at the other region or (ii) a shark returned to where it departed at previous time step, then moved to the other region within the season. Individual sharks were coded as ‘1’ if the shark moved or ‘0’ if the shark did not move between sites in a given season. Therefore, the probability of ‘moving’ to the other site at least one time in a season can be defined as:
We implemented the model in the R software (R Core Team, 2019) environment using the ‘rstanarm’ (Goodrich et al., 2020) and ‘shinystan’ (Gabry, 2018) packages (Muth et al., 2018). We ran four chains using diffuse priors and ran each chain for 1,000 iterations after a burn-in of 1,000 iterations was completed and discarded (Gelman et al., 1996). We used the standard priors set by ‘rstanarm’. We used normal distributions for the priors for random effects of individual and year (random effects were ~N[0, ] and ~N[0, ]]). After 1,000 burn-in iterations, we ran an additional 1,000 iterations per chain, resulting in 4,000 total samples from the posterior distribution. We assessed model convergence by inspecting the trace plots and Geweke diagnostics (Geweke, 1991) and evaluating whether the Gelman-Rubin statistic, , was<1.1 for each monitored parameter (Gelman and Rubin, 1992). We evaluated how well predicted values from the fitted model corresponded with actual observations using posterior predictive checks (Gelman et al., 2000). Specifically, we evaluated how well distributions of predicted values corresponded to the mean, standard deviation, density, and 2.5% and 97.5% quantiles of the observed data. We also examined the distribution of discrepancies between predicted and observed values to evaluate whether there was evidence that some observations were not predicted well by the model.
3 Results
In California, from 2008 to 2018, a total of 249 tags were deployed onto 210 individual white sharks (some sharks were tagged multiple times) that ranged in estimated total length from 240 cm to 550 cm in total length (mean = 401.75 cm; SD = 75.14). Of the sharks tagged, 81 were adult males, 46 were sub-adult males, 43 were adult females, and 40 were sub-adult females.
In Guadalupe Island, from 2008 to 2018, 122 acoustic tags were deployed onto 116 sharks that ranged in estimated total length from 200 cm to 570 cm in total length (mean = 374.27 cm; SD = 80.44). Of those sharks tagged, there were 26 adult males, 33 sub-adult males, 18 adult females, and 39 sub-adult males.
The mean length of time that acoustic tags remained attached to a shark and had a functional battery that transmitted a signal was 572 days (SD = 508; range = 6 to 2983 days). Over the course of the study, we recorded a total of 6,190,866 detections from receivers placed at both locations in central California and Guadalupe Island. In central California, there were a total of 666,560 detections from receivers (VR-2, VR-3, and VR-4) and 5,524,306 detections from receivers (VR-2) in Guadalupe Island (Figure 1). A summary of the number of sharks with functioning acoustic tags for each year of the study can be found in Table 1.
Of the 326 total sharks tagged in the study, 210 (64.42%) were tagged in central California while the other 116 (35.58%) were tagged in Guadalupe Island. Of the 326, 30 (9.20%) were detected in both locations, and 296 (90.80%) were detected only at the aggregation sites (central California or Guadalupe Island) where the tag was originally applied. Of the 30 sharks detected at both locations, 20 (66.67%) were tagged in central California and included four adult males, seven sub-adult males, two adult females, and seven sub-adult females (1.22 males for every female). The 10 (33.33%) sharks tagged in Guadalupe Island that were detected at both locations included one adult male, five sub-adult males, one adult female, and three sub-adult females (1.5 males for every female). The proportion of sharks tagged at each region was similar to the proportion of sharks that moved from each region.
Of the 30 sharks that moved, 20 made one move to the other region and apparently remained there for the remainder of tag function. There were eight sharks that made two movements, meaning they moved to the other region and then returned to the original location. In addition, one shark made three movements and one shark made four movements between the regions (Figure 2).
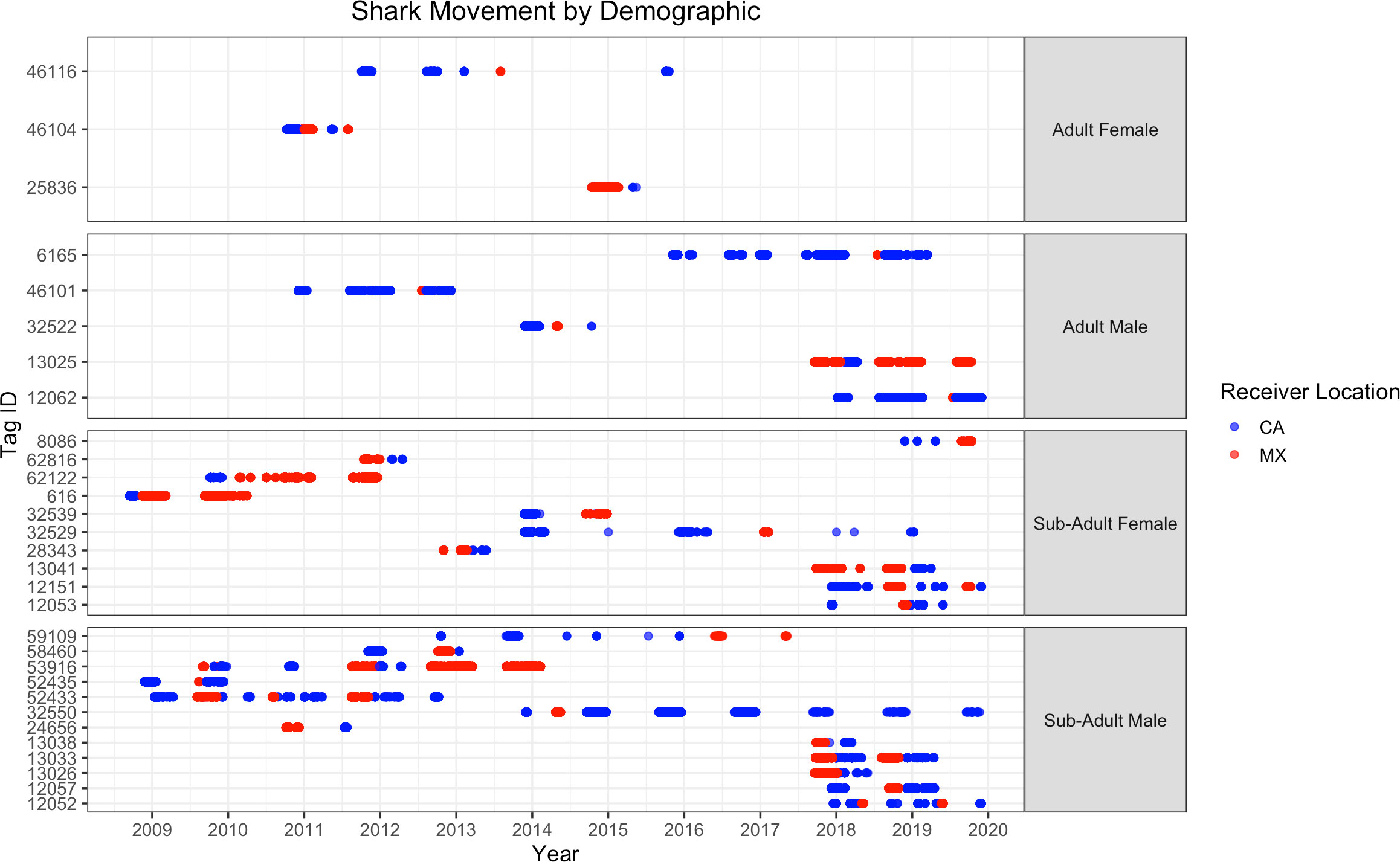
Figure 2 Detections of white sharks by class at receivers in central California, USA and Guadalupe Island, Mexico over the course of the study period. The Tag ID is the acoustic code of the tag applied to the individual shark.
There were three sharks that made more than one transition within a season (shark 53916, 350-cm male; shark 46104, 450-cm female; shark 32539, 300-cm female). The straight-line distance from the southernmost location in central California to Guadalupe Island is ~ 950 km. The mean length of time of within season movement between central California and Guadalupe Island ranged from 8 to 91 days (mean = 32.56 days; SD = 24.81). Therefore, the fastest minimum rate of travel was estimated to be 133 km/day at 1.31 m/s.
3.1 Model results
The model converged successfully, and the posterior distribution for model coefficients generated predicted values that corresponded well with features of the observed data. The model results indicated that the predicted probability of moving in between central California and Guadalupe Island (regardless of direction) was rare (α = -4.16; SE = 0.86; 90% credible intervals [CI] = -6.11 to -2.72). The model indicated that males and females were quite similar with respect to movement probability (βs = 0.15; SE = 0.63; 90% CI = -1.15 to 1.37). Adults were less likely to move than sub-adults (βm = -2.10; SE = 0.71; 90% CI = -3.63 to -0.79), and sharks were similar in movement probability given their starting location (βl = 0.55; SE = 0.51; CI = -1.54 to 0.47). The estimated variance term for the random effect for individual sharks was larger ( = 7.65; 90% credible intervals = 2.68 to 16.67) than the random effect for years ( = 0.12; CI = 0.00 to 0.64). As only 30 of the 326 sharks in this 12-year study moved to the other region, accordingly, the individual random effect-outcomes predicted for all but those 30 sharks were small and below 0, whereas the remaining 30 tended to be small and positive with only a very few sharks predicted to have positive random effects that notably increased their probability of movement. Predicted probabilities of moving between central California and Guadalupe Island for each of the 30 individuals that moved averaged 0.39 (SE = 0.22), whereas movement probabilities for the other individuals averaged<0.02 (SE = 0.05).
While all demographics from both locations moved to the other location at some point during the study period, the sub-adult demographic from both central California and Guadalupe Island had the higher probabilities of movement to the other location (Table 2). For sub-adults, these probabilities ranged from 0.073 (90% CI = 0.000 to 0.25) to 0.155 (90% CI = 0.000 to 0.405) compared to the lower probabilities of movement for the adult demographic that ranged from 0.017 (90% CI = 0.000 to 0.088) to 0.031 (90% CI = 0.000 to 0.141). Interestingly, sub-adult males tagged in Guadalupe Island had the highest probability of moving 0.155 (90% CI = 0.000 to 0.779) and the adult males tagged in Guadalupe Island had the lowest probability of moving 0.006 (90% CI = 0.000 to 0.080).
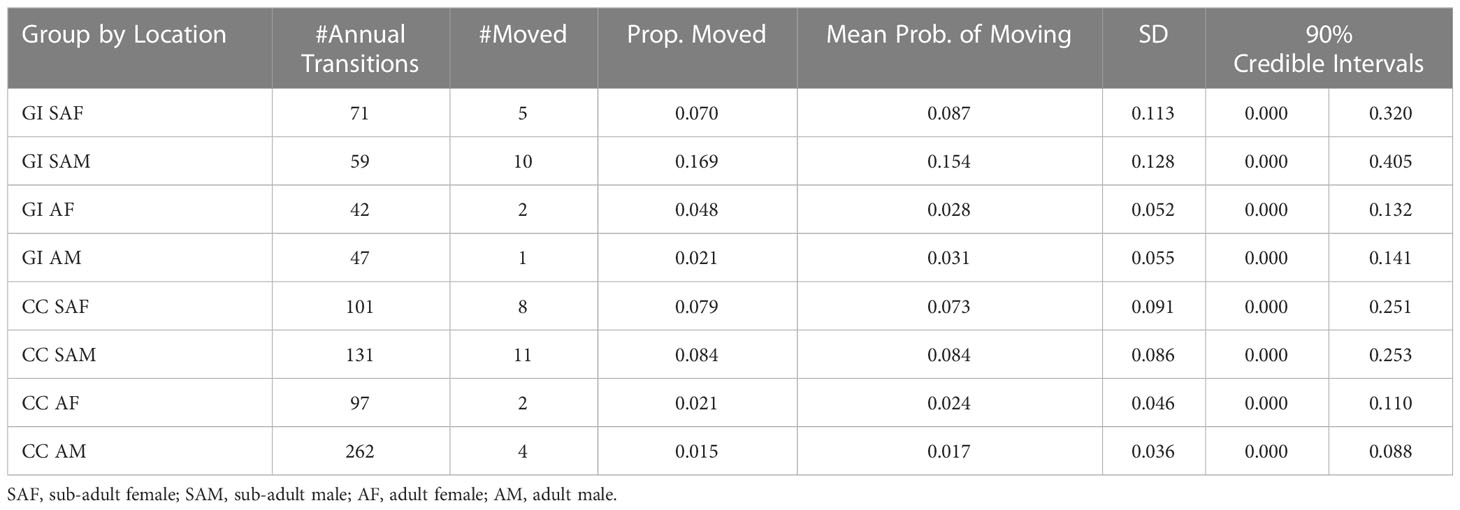
Table 2 Summary of results from the GLMM analysis of movement probabilities for each demographic at central California, USA and Guadalupe Island, Mexico.
4 Discussion
The purpose of this study was to better understand the connectivity and movement of sub-adult and adult white sharks between the two main aggregations that comprise the northeastern Pacific population. Our results indicate that the majority of sharks have high annual fidelity to their respective foraging aggregation site where they were first tagged. Over the 12-year study, ~10% of sharks utilized both aggregation sites, yet the overall probability of movement was relatively low. Understanding the rate of exchange will be important to inform how to best integrate mark-recapture data from each site and an help understand ambiguity and genetic structure.
Individuals from all classes (male and female sub-adult and adults) were shown to have moved to the other location from where they were tagged. Sub-adult sharks were more likely than adults to be using or exploiting both aggregation sites. These results are consistent with previous studies that have found sub-adult sharks visiting both major aggregations (Jorgensen et al., 2012a; Hoyos-Padilla et al., 2016). Sub-adult connectivity has also been hypothesized to be a source of genetic mixing between the two aggregations (Domeier, 2012). In this study, however, we also detected eight adult white sharks (five male, three female) making transregional movements, a first for this population and a finding that puts aside an early hypothesis that only sub-adults move between the regions (Domeier, 2012). The mechanisms driving a small number of individual sharks to exploit both areas remain unknown, but could be associated with low prey availability and/or high density of white sharks at a respective aggregation area leading to increased intraspecific competition.
Mating locations and dynamics in the NEP have not been confirmed and thus the potential population-level implications for these movements detected by tagging remain unknown. Mating likely occurs at either coastal locations (Domeier, 2012; Domeier and Nasby-Lucas, 2012; Domeier and Nasby-Lucas, 2013) or in the White Shark Café (Jorgensen et al., 2012b), ~1500 km between Baja, Mexico and Hawaii, USA. However, a single migrant per generation over time can be sufficient to homogenize genetic structure between two otherwise isolated populations (Morjan and Rieseberg, 2004; Hartl and Clark, 2006). During the 12-year period of this study we documented 30 individuals (of 329 tagged) moving between Guadalupe Island and central California. Therefore, this study supports the findings of Bernard et al. (2018) and Santana-Morales et al. (2020), which detected no genetic structure (nuclear and mitochondrial DNA respectively) between these regions, rather than with those of Oñate-González et al. (2015), which indicated significant mitochondrial DNA structure. Until future studies resolve the genetics of the population conclusively as well as the mating dynamics, the relationships between the individual trans-regional movement and overall population structure in this case remains uncertain.
Sub-adult white sharks are a demographic of which relatively little is known. During this life stage, white sharks undergo an ontogenetic shift in diet and habitat (Tricas and Mccosker, 1984) and transition to aggregations where adult white sharks are well established and have been successfully exploiting these areas for food resources (Weng et al., 2007b). There is likely intense intraspecific competition for recruiting sub-adult sharks prospecting for new resources around pinniped prey concentrations to fulfill their increased physiological demand in the colder nearshore waters. It has been shown that the smaller size classes in central California have substantially lower apparent survival rates than larger conspecifics (Kanive et al., 2019). However, since mortality is confounded with permanent emigration in apparent survival, this could mean that smaller sub-adults may fail to recruit at adult aggregating sites resulting in either mortality or emigration to secondary locations. Grievous wounds have been observed on multiple sub-adult sharks that appear to have been inflicted by a larger white shark (Kanive et al., 2019). Sub-adults could be motivated to explore other habitats if they experience such aggression while attempting to recruit at an established, predominately adult hunting ground. The results of this study where the sub-adults have the highest degree of movement support these possibilities.
This study provides critical information for a future estimation and assessment of the overall abundance of the northeastern Pacific that includes both groups, central California and Guadalupe Island, that to date have largely been considered separately. This study confirms connectivity between the two main aggregation of both sub-adults and adults.Consequently, simply summing independent estimates from both locations to estimate overall population characteristics would include a percentage of ‘double-counted’ individuals. Furthermore, a joint method of ‘marking’ or identifying individuals, such as using the long-lasting (>26 years) natural and unique patterns on dorsal fins (Anderson et al., 2011) is needed to incorporate data into a mark-recapture framework, an established method to identify population vital rates (Kanive et al., 2015; Chapple et al., 2016; Kanive et al., 2019) and abundance estimates (Chapple et al., 2011; Towner et al., 2013; Kanive et al., 2021). Lastly, continued and expanded mutually beneficial collaborative efforts and shared information are needed to enable researchers to estimate robust population parameters that can be used for international management.
Data availability statement
The raw data supporting the conclusions of this article will be made available by the authors, without undue reservation.
Ethics statement
The animal study was reviewed and approved by Montana State University Institutional Animal Care and Use Committee.
Author contributions
PK, JR, SJ, MH, and TC contributed to conception and design of the study. PK, SJ, TC, SA, MH-P, FG-M, and BB tagged white sharks in the field and downloaded receivers. PK organized the database. PK and JR performed the statistical analysis. PK wrote the first draft of the manuscript. SA and AK helped with figures. All authors contributed to the article and approved the submitted version.
Funding
Funding was provided by the Monterey Bay Aquarium and Stanford University.
Acknowledgments
In the US, this work was conducted under permission from the California Department of Fish and Wildlife (SCP-2014001349), National Oceanic and Atmospheric Administration (MULTI-2014-013-A1), National Park Service (NPS-PORE-00031), Montana State University (IACUC 2015- 21), and Stanford APLAC and IACUC procedures. PK received fellowship support from the Monterey Bay Aquarium. Support for the tagging was provided by the Tagging of Pacific Pelagics program, the Moore, Packard and Monterey Bay Aquarium. We would like to thank Ron Elliott, Pat Conroy, Tom O’leary, Tom Baty, and Shawn Rhodes for vessel support. We thank Karin Neff for her suppoort and input on visual presentation of data. We thank Mike Castleton for data management of the acoustic data and the technical assistance of the Tuna Research and Conservation Center technician team for leadering and preparing acoustic tags. Vessel operations in support of the white shark program were from Ron Elliott, Pat Conroy, Tom O’leary, Tom Baty, Shawn Rhodes, Monterey Bay Aquarium, Friends of Hopkins Marine Station, Stanford University, Discovery Corporation, and Grady White. Ethics and tagging procedures followed an animal care protocol (Protocol number 16022, UC Davis Institutional Animal Care and Use Committee). In Mexico the authors thank Club Cantamar, Solmar V, Horizon Charters, Islander Charters and Storm for their logistical support. The research was funded by Alianza WWF-Fundacion Telmex-Telcel, Alianza WWF-Fundacion Carlos Slim, Fins Attached, International Community Foundation, Charles Annenberg Foundation and Ocean Blue Tree. FGM thanks to Instituto Politecnico Nacional for fellowships granted (COFAA, EDI). This study was conducted under permits from Secretaría del Medio Ambiente y Recursos Naturales (SEMARNAT): OFICIO 662 NUM.SGPA/DGVS/07052/16 and OFICIO NUM.SG- 663PA/DGVS/06673/17, Comision Nacional de Areas Naturales Protegidas (OFICIO NUM.F00.DRPBCNP.-001002/2017) and Secretaria de Gobernacion (OFICIO NUM.UG/211/0491/2017).
Conflict of interest
The authors declare that the research was conducted in the absence of any commercial or financial relationships that could be construed as a potential conflict of interest.
Publisher’s note
All claims expressed in this article are solely those of the authors and do not necessarily represent those of their affiliated organizations, or those of the publisher, the editors and the reviewers. Any product that may be evaluated in this article, or claim that may be made by its manufacturer, is not guaranteed or endorsed by the publisher.
References
Anderson S. D., Chapple T. K., Jorgensen S. J., Klimley A. P., Block B. A. (2011). Long-term individual identification and site fidelity of white sharks, Carcharodon carcharias, off California using dorsal fins. Mar. Biol. 158 (6), 1233–1237. doi: 10.1007/s00227-011-1643-5
Andrzejaczek S., Chapple T. K., Jorgensen S. J., Anderson S. D., Castleton M., Kanive P. E., et al. (2022). Multi-decadal high-resolution data reveal the cryptic vertical movement patterns of a Large marine predator along the Californian coast. Front. Mar. Sci. 9. doi: 10.3389/fmars.2022.835576
Benson J. F., Jorgensen S. J., O'Sullivan J. B., Winkler C., White C. F., Garcia-Rodriguez E., et al. (2018). Juvenile survival, competing risks, and spatial variation in mortality risk of a marine apex predator. J. Appl. Ecol. 55, 2888–2897. doi: 10.1111/1365-2664.13158
Bernard A. M., Richards V. P., Stanhope M. J., Shivji M. S. (2018). Transcriptome-derived microsatellites demonstrate strong genetic differentiation in pacific white sharks. J. Heredity 109 (7), 771–779. doi: 10.1093/jhered/esy045
Block B. A., Dewar H., Farwell C., Prince E. D. (1998). A new satellite technology for tracking the movements of Atlantic bluefin tuna. Proc. Natl. Acad. Sci. 95 (16), 9384–9389. doi: 10.1073/pnas.95.16.9384
Block B. A., Teo S. L. H., Walli A., Boustany A., Stokesbury M. J. W., Farwell C. J., et al. (2005). Electronic tagging and population structure of Atlantic bluefin tuna. Nature 434 (7037), 1121–1127. doi: 10.1038/nature03463
Block B. A., Jonsen I. D., Jorgensen S. J., Winship A. J., Shaffer S. A., Bograd S. J., et al. (2011). Tracking apex marine predator movements in a dynamic ocean. Nature 475 (7354), 86–90. doi: 10.1038/nature10082
Blower D. C., Pandolfi J. M., Bruce B. D., Gomez-Cabrera del M. C., Ovenden J. R. (2012). Population genetics of Australian white sharks reveals fine-scale spatial structure, transoceanic dispersal events and low effective population sizes. Mar. Ecol. Prog. Ser. 455, 229–244. doi: 10.3354/meps09659
Boustany A. M., Davis S. F., Pyle P., Anderson S. D., Boeuf B. J. L., Block B. A. (2002). Satellite tagging: expanded niche for white sharks. Nature 415 (6867), 35–36. doi: 10.1038/415035b
Chapple T. K., Jorgensen S. J., Anderson S. D., Kanive P. E., Klimley A. P., Botsford L. W., et al. (2011). A first estimate of white shark, Carcharodon carcharias, abundance off central California. Biol. Lett. 7 (4), 581–583. doi: 10.1098/rsbl.2011.0124
Chapple T. K., Chambert T., Kanive P. E., Jorgensen S. J., Rotella J. J., Anderson S. D., et al. (2016). A novel application of multi-event modeling to estimate class segregation in a highly migratory oceanic vertebrate. Ecology 97 (12), 3494–3502. doi: 10.1002/ecy.1589
Domeier M. (2012). A new life-history hypothesis for white sharks, carcharodon carcharias, in the northeastern pacific. In: Global perspectives on the biology and life history of the white shark (CRC Press), 199–224. Available at: http://www.crcnetbase.com/doi/abs/10.1201/b11532-19. (Accessed 4 March 2013).
Domeier M., Nasby-Lucas N. (2008). Migration patterns of white sharks Carcharodon carcharias tagged at Guadalupe island, Mexico, and identification of an eastern pacific shared offshore foraging area. Mar. Ecol. Prog. Ser. 370, 221–237. doi: 10.3354/meps07628
Domeier M., Nasby-Lucas N. (2012). Sex-specific migration patterns and sexual segregation of adult white sharks, carcharodon carcharias, in the northeastern pacific. In: Global perspectives on the biology and life history of the white shark (CRC Press) (Accessed 4 March 2013).
Domeier M. L., Nasby-Lucas N. (2013). Two-year migration of adult female white sharks (Carcharodon carcharias) reveals widely separated nursery areas and conservation concerns. Anim. Biotelemetry 1 (1), 1–10. doi: 10.1186/2050-3385-1-2
Francis M. P. (1996). “Observations on a pregnant white shark with a review of reproductive biology,” in Great white sharks: the biology of carcharodon carcharias (San Diego: Academic Press), 157–172.
Gabry J. (2018). Shinystan: interactive visual and numerical diagnostics and posterior analysis for Bayesian models. Available at: https://CRAN.R-project.org/package=shinystan.
Galván-Magaña F., Hoyos-Padilla E. M., Navarro-Serment C. J., Márquez-Farías F. (2011). Records of white shark, Carcharodon carcharias, in the gulf of California, Mexico. Mar. Biodiversity Records 3, 258–265. doi: 10.1017/S1755267210000977
Gelman A., Goegebeur Y., Tuerlinckx F., Mechelen I. V. (2000). Diagnostic checks for discrete data regression models using posterior predictive simulations. J. R. Stat. Society: Ser. C (Applied Statistics) 49 (2), 247–268. doi: 10.1111/1467-9876.00190
Gelman A., Meng X.-L., Stern H. (1996). Posterior predictive assessment of model fitness via realized discrepancies. Stat. Sin. 6 (4), 733–760.
Gelman A., Rubin D. B. (1992). Inference from iterative simulation using multiple sequences. Stat. Sci. 7 (4), 457–472. doi: 10.1214/ss/1177011136
Geweke J. (1991). Evaluating the accuracy of sampling-based approaches to the calculation of posterior moments (Minneapolis; Federal Reserve Bank of Minneapolis, University of Minnesota Research Department).
Goodrich B., Gabry J., Ali I., Brilleman S. (2020) Rstanarm: Bayesian applied regression modeling via Stan. Available at: https://mc-stan.org/rstanarm.
Gubili C., Bilgin R., Kalkan E., Karhan S. Ü., Jones C. S., Sims D. W., et al. (2010). Antipodean white sharks on a Mediterranean walkabout? historical dispersal leads to genetic discontinuity and an endangered anomalous population. Proc. R. Soc. London B: Biol. Sci. 278, rspb20101856. doi: 10.1098/rspb.2010.1856
Hoyos-Padilla E. M., Klimley A. P., Galván-Magaña F., Antoniou A. (2016). Contrasts in the movements and habitat use of juvenile and adult white sharks (Carcharodon carcharias) at Guadalupe island, Mexico. Anim. Biotelemetry 4, 14. doi: 10.1186/s40317-016-0106-7
Jorgensen S. J., Reeb C. A., Chapple T. K., Anderson S., Perle C., Sommeran V., et al. (2010). Philopatry and migration of pacific white sharks. Proc. R. Soc. B: Biol. Sci. 277 (1682), 679–688. doi: 10.1098/rspb.2009.1155
Jorgensen S., Chapple T., Hoyos M., Reeb C., Block B. (2012a). “Connectivity among white shark coastal aggregation areas in the northeastern pacific,” in Global perspectives on the biology and life history of the white shark. Ed. Domeier M. (CRC Press), 159–168. doi: 10.1201/b11532-16 (Accessed: 4 March 2013).
Jorgensen S. J., Arnoldi N. S., Estess E. E., Chapple T. K., Rückert M., Anderson S. D., et al. (2012b). Eating or meeting? cluster analysis reveals intricacies of white shark (Carcharodon carcharias) migration and offshore behavior. PloS One 7 (10), e47819. doi: 10.1371/journal.pone.0047819
Kanive P. E., Rotella J. J., Jorgensen S. J., Chapple T. K., Anderson S. D., Klimley A. P., et al. (2015). Estimating apparent survival of sub-adult and adult white sharks (Carcharodon carcharias) in central California using mark-recapture methods. Mar. Megafauna 2. doi: 10.3389/fmars.2015.00019
Kanive P. E., Rotella J. J., Jorgensen S. J., Chapple T. K., Hines J. E., Anderson S. D., et al. (2019). Size-specific apparent survival rate estimates of white sharks using mark-recapture models. Can. J. Fish. Aquat. Sci. doi: 10.1139/cjfas-2018-0142carlisle
Kanive P. E., Rotella J. J., Chapple T. K., Anderson S. D., White T. D., Block B. A., et al. (2021). Estimates of regional annual abundance and population growth rates of white sharks off central California. Biol. Conserv. 257, 109104. doi: 10.1016/j.biocon.2021.109104
Klimley A. P. (1985). The areal distribution and autoecology of the white shark, Carcharodon carcharias, of the west coast of north America. Memoirs South. California Acad. Sci. 9, 15–40.
Lowe C., Blasius M., Jarvis E., Mason T., Goodmanlowe G., O’Sullivan J. (2012). “Historic fishery interactions with white sharks in the southern California bight,” in Global perspectives on the biology and life history of the white shark. Ed. Domeier M. (CRC Press), 169–186. doi: 10.1201/b11532-17 (Accessed: 4 March 2013).
Lyons K., Jarvis E. T., Jorgensen S. J., Weng K., O’Sullivan J., Winkler C., et al. (2013). The degree and result of gillnet fishery interactions with juvenile white sharks in southern California assessed by fishery-independent and -dependent methods. Fish. Res. 147, 370–380. doi: 10.1016/j.fishres.2013.07.009
Morjan C. L., Rieseberg L. H. (2004). How species evolve collectively: implications of gene flow and selection for the spread of advantageous alleles. Mol. Ecol. 13 (6), 1341–1356. doi: 10.1111/j.1365-294X.2004.02164.x
Muth C., Oravecz Z., Gabry J. (2018). User-friendly Bayesian regression modeling: a tutorial with rstanarm and shinystan. ResearchGate. doi: 10.20982/tqmp.14.2.p099
O’Leary S. J., Feldheim K. A., Fields A. T., Natanson L. J., Wintner S., Hussey N., et al. (2015). Genetic diversity of white sharks, Carcharodon carcharias, in the Northwest Atlantic and southern Africa. J. Heredity 106 (3), 258–265. doi: 10.1093/jhered/esv001
Oñate-González E. C., Rocha-Olivares A., Saavedra-Sotelo N. C., Sosa-Nishizaki O. (2015). Mitochondrial genetic structure and matrilineal origin of white sharks, Carcharodon carcharias, in the northeastern pacific: implications for their conservation. J. Heredity 106 (4), 347–354. doi: 10.1093/jhered/esv034
Oñate-González E. C., Sosa-Nishizaki O., Herzka S. Z., Lowe C. G., Lyons K., Santana-Morales O., et al. (2017). Importance of bahia Sebastian vizcaino as a nursery area for white sharks (Carcharodon carcharias) in the northeastern pacific: a fishery dependent analysis. Fish. Res. 188, 125–137. doi: 10.1016/j.fishres.2016.12.014
Pardini A. T., Jones C. S., Scholl M. C., Noble L. R. (2000). Isolation and characterization of dinucleotide microsatellite loci in the great white shark, Carcharodon carcharias. Mol. Ecol. 9 (8), 1176–1178. doi: 10.1046/j.1365-294x.2000.00954-4.x
Pratt H. L. (1996). “Reproduction in the male white shark,” in Great white sharks: the biology of carcharodon carcharias (San Diego: Academic Press), 131–138.
R Core Team (2019). R: A language and environment for statistical computing. R Foundation for Statistical Computing, Vienna, Austria. Available at: https://www.R-project.org/.
Santana-Morales O., Abadía-Cardoso A., Hoyos-Padilla M., Naylor G. J. P., Corrigan S., Malpica-Cruz L., et al. (2020). The smallest known free-living white shark Carcharodon carcharias (Lamniformes: lamnidae): ecological and management implications. Copeia 108 (1), 39–46. doi: 10.1643/OT-19-233
Sosa-Nishizaki O., Morales-Bojórquez E., Nasby-Lucas N., Oñate-González E., Domeier M. (2012). “Problems with photo identification as a method of estimating abundance of white sharks, carcharodon carcharias”. In: Global perspectives on the biology and life history of the white shark (CRC Press) (Accessed 4 March 2013).
Tanaka S., Kitamura T., Mochizuki T., Kofuji K. (2011). Age, growth and genetic status of the white shark (Carcharodon carcharias) from kashima-nada, Japan. Mar. Freshw. Res. 62 (6), 548–556. doi: 10.1071/MF10130
Towner A. V., Wcisel M. A., Reisinger R. R., Edwards D., Jewell O. J. D. (2013). Gauging the threat: the first population estimate for white sharks in south Africa using photo identification and automated software. PloS One 8 (6), e66035. doi: 10.1371/journal.pone.0066035
Tricas T. C., Mccosker J. E. (1984). Predatory behavior of the white shark (Carcharodon carcharias), with notes on its biology. Proc. California Acad. Sci. 43, 221–238.
Weng K., Boustany A., Pyle P., Anderson S., Brown A., aBlock B. (2007a). Migration and habitat of white sharks (Carcharodon carcharias) in the eastern pacific ocean. Mar. Biol. 152 (4), 877–894. doi: 10.1007/s00227-007-0739-4
Weng K. C., O’Sullivan J. B., Lowe C. G., Winkler C. E., Dewar H., Block B. A. (2007b). Movements, behavior and habitat preferences of juvenile white sharks Carcharodon carcharias in the eastern pacific. Mar. Ecol. Prog. Ser. 338, 211–224. doi: 10.3354/meps338211
Keywords: white shark, California, Guadalupe Island, acoustic telemetry, movement rates, connectivity
Citation: Kanive PE, Rotella JJ, Chapple TK, Anderson SD, Hoyos-Padilla M, Klimley AP, Galván-Magaña F, Andrzejaczek S, Block BA and Jorgensen SJ (2023) Connectivity between white shark populations off Central California, USA and Guadalupe Island, Mexico. Front. Mar. Sci. 10:1210969. doi: 10.3389/fmars.2023.1210969
Received: 23 April 2023; Accepted: 19 June 2023;
Published: 14 July 2023.
Edited by:
Elizabeth Grace Tunka Bengil, University of Kyrenia, CyprusReviewed by:
Luis Cardona, University of Barcelona, SpainMichael Domeier, Marine Conservation Science Institute (MCSI), United States
Copyright © 2023 Kanive, Rotella, Chapple, Anderson, Hoyos-Padilla, Klimley, Galván-Magaña, Andrzejaczek, Block and Jorgensen. This is an open-access article distributed under the terms of the Creative Commons Attribution License (CC BY). The use, distribution or reproduction in other forums is permitted, provided the original author(s) and the copyright owner(s) are credited and that the original publication in this journal is cited, in accordance with accepted academic practice. No use, distribution or reproduction is permitted which does not comply with these terms.
*Correspondence: Paul E. Kanive, paul.kanive@montana.edu