Who lives in a pear tree under the sea? A first look at tree reefs as a complex natural biodegradable structure to enhance biodiversity in marine systems
- 1Department of Coastal Systems, Royal Netherlands Institute for Sea Research (NIOZ), Den Hoorn, Netherlands
- 2Conservation Ecology Group, Groningen Institute for Evolutionary Life Sciences (GELIFES), University of Groningen, Groningen, Netherlands
- 3Amsterdam Institute for Life and Environment (A-LIFE), Faculty of Science, Vrije Universiteit Amsterdam, Amsterdam, Netherlands
- 4Department of Aquatic Ecology, Waardenburg Ecology, Culemborg, Netherlands
- 5Department of Estuarine and Delta Systems, Royal Netherlands Institute for Sea Research (NIOZ), Yerseke, Netherlands
- 6Department of Physical Geography, Faculty of Geosciences, Utrecht University, Utrecht, Netherlands
Hard substrates play an important role in global marine systems as settlement surface for sessile reef-forming species such as corals, seaweeds, and shellfish. In soft-sediment systems, natural hard substrates such as stones, bedrock and driftwood are essential as they support diverse assemblages of reef-associated species. However, availability of these hard substrates has been declining in many estuaries and shallow seas worldwide due to human impacts. This is also the case in the Dutch Wadden Sea, where natural hard substrates have gradually disappeared due to burial by sand and/or active removal by humans. In addition, driftwood that was historically imported from rivers has been nullified by upstream logging and coastal damming of estuaries. To investigate the historic ecological role of wood presence in the Wadden Sea as settlement substrate and fish habitat, we constructed three meter high artificial reefs made of felled pear trees. Results demonstrate that these reefs rapidly developed into hotspots of biodiversity. Within six months, the tree-reefs were colonized by sessile hard substrate associated species, with a clear vertical zonation of the settled species. Macroalgae and barnacles were more abundant on the lower parts of the reef, while bryozoans were more dominant on the upper branches. In addition, six fish species were observed on the reefs, while only two species were caught on sandy control sites. Moreover, the abundance of fish on the reefs was five times higher. Individuals of the most commonly caught species, the five-bearded rockling Ciliata mustela, were also larger on the reef. These patterns also hold true for common prawn, Palaemon serratus, which were also larger and ten times more numerous on the reefs. Present findings indicate that the reintroduction of tree-reefs as biodegradable, structurally complex hard substrates can increase local marine biodiversity in soft-sediment systems within relatively short time scales.
Introduction
Reefs greatly enhance biodiversity by attenuating currents and waves and by providing stable, spatially complex substrate which serves as habitat, shelter, foraging and nursing grounds, all of which expand niche availability to marine life (Coolen et al., 2020; Ponti et al., 2021; Nauta et al., 2023). This is particularly important in otherwise structurally homogeneous soft-bottomed marine systems. In these environments, both geogenic and biogenic reefs (i.e., made up of reef-building species like corals, bivalves or tube-worms) act as biodiversity hotspots (Coolen et al., 2020; van der Reijden et al., 2021). Over the last centuries, however, the extent of these reef types has greatly declined worldwide due to human impact. Both have declined because of habitat destruction caused by land reclamation, the removal of hard structures by mining as well as destructive fisheries such as dynamite fishing or bottom-trawling (Lotze, 2007; Sguotti et al., 2016). In addition, pollution and climate change-related extreme events may also cumulatively add to these direct effects by impacting reef-building organisms that make up biogenic reefs (Spalding and Brown, 2015; Stuart-Smith et al., 2015; Ponti et al., 2021).
In line with observed declines worldwide, reefs in the soft-sediment dominated North Sea area have been declining over the last 150 years (Houziaux et al., 2011; Molen et al., 2018). The southern North Sea in particular has seen a well-documented loss of approximately 25,000 km2 of oyster reefs in the late 19th and early 20th centuries related to overfishing (Houziaux et al., 2011; Temming and Hufnagl, 2015; Sguotti et al., 2016). The Wadden Sea, located within the southern expanses of the North Sea region, biogenic reefs have also greatly declined. Disappearance of reefs formed by the tubeworm Sabellaria spinulosa in the 1950s has been linked to shrimp fishing activities (Reise, 1982), oyster reefs disappeared due to disease in the 1960s (Beck et al., 2011), and overfishing reduced the extent of intertidal mussel reefs in the Dutch parts in the late 1980s and early 1990s (Lotze et al., 2005; Eriksson et al., 2010). Moreover, whereas artificial hard substrates have increased along the shorelines due to the construction of dikes and dams, the opposite is the case for natural hard substrates: rocky glacial deposits, dead shells, and woody remains have greatly declined in the North and Wadden Sea systems (Pogoda et al., 2020). Rocky glacial deposits known as erratics were removed by fisheries and dredging whilst in the present day, shells are exploited for mining purposes (Beukema and Cadée, 1999). Hard substrate occurrence and availability has further decreased by ongoing burial due to continued sediment import and altered bathymetry of the Wadden Sea related to the changes in geomorphology and associated hydrodynamic shifts caused by large-scale damming (Giesen et al., 1990; van Katwijk, 2003).
Apart from reducing the occurrence of already present bio- and geogenic reefs in the North and Wadden Sea, human activities can also hamper the formation of new reefs. As biogenic reefs require benthic stability, the establishment of new mussel, oyster, and tubeworm reefs can be inhibited or rendered impossible by seafloor destabilizing activities such as bottom trawling and dredging (Fariñas-Franco et al., 2018; Tiano et al., 2022). Continuous disturbance of the sea floor therefore generally prevents a stable state system where slow-growing shellfish may be able to create new reefs (Fitzsimons et al., 2020). The addition of new natural hard substrates to the Wadden Sea is nowadays limited by extensive landscape domestication and coastal hardening; this restricts riverine inputs of geogenic substrates such as pebbles, gravel, and stones (Veenstra, 1969; Kondolf and Matthews, 1986). In the past, organic substrates such as floating peat rafts and driftwood were also transported to sea by rivers (Evans and Warburton, 2001; Murphy et al., 2021); this is now also nullified by coastal hardening. Recent work demonstrated that prior to human intervention in riverine systems, rivers exported massive quantities of driftwood into estuaries and marine environments worldwide (Wohl and Iskin, 2021). This was also the case in Europe prior to widescale landscape domestication approximately 2500 years ago (Lotze et al., 2005). Some of this wood became waterlogged and sank, providing settlement opportunities and habitat for shellfish (Kano et al., 2013; Schlining et al., 2013) and other sessile organisms. What little wood that currently remains in the Wadden Sea is of historical origin, being either remnants of Holocene bog forests, or more recently, the remains of shipwrecks or wooden dykes (Franken et al., 2023) as natural import has ceased due to the damming of rivers.
Because of the vital ecological functions provided by reefs in coastal systems, both governments and nongovernmental stakeholders worldwide are increasingly investing in halting the ongoing decline of various reef types, seagrass meadows, and mangroves and restoring these vital habitats, further encouraged by the UN Decade on Ecosystem Restoration (Tan et al., 2020; Waltham et al., 2020). This is also the case in the North Sea region. Clear examples are recent attempts to build or restore native oyster reefs in nature reserves (Didderen et al., 2018) and wind farms (Kamermans et al., 2018), and mussel bed restoration experiments in the Wadden Sea (de Paoli et al., 2015), along with the Scheldt estuary (Schotanus et al., 2020a; Schotanus et al., 2020b). Although the importance of reefs of both geogenic and biogenic origin are well-recognized, conservation and restoration action have thus far typically focused on either biogenic reefs (zu Ermgassen et al., 2020) or the introduction of novel hard substrates (Lima et al., 2019; Guo et al., 2021). Typical examples of the latter are concrete-based reef structures (Borsje et al., 2011) for the ecological enhancement of dikes and dams, and the repurposing of abandoned oil and gas platforms (Bull and Love, 2019). Yet, the potential for reintroducing organic hard substrates largely remains to be investigated.
The current lack of research is somewhat surprising as wood has travelled to sea for millions of years; fossil records of biological communities on marine wood falls stretch back to the Jurassic period, approximately 175 million years ago (Kaim, 2011). Prior to large scale landscape domestication, vast amounts of wood entered the sea via rivers (Wohl and Iskin, 2021). As recently as the 1850’s, surveyors reported numerous massive trees of 50 meters long with five meter circumferences on the shores of estuaries in the Pacific Northwest; this was still found to be the case a century later (Gonor et al., 1988). Early survey records from the coast of Oregon show ‘large rafts of drift trees’ off the mouth of the Columbia River (Gonor et al., 1988), which would either eventually wash ashore or sink. Historically, large wood has been removed from river systems globally (Nagayama and Nakamura, 2010) though it is well documented that large woody debris creates excellent habitat for fish in aquatic systems (McMahon and Holtby, 1992) such as rivers, lakes, and estuaries. This is also found to be the case in marine environments (Gonor et al., 1988; Masuda et al., 2010; Alam et al., 2020). However, studies on the effect of large woody debris on fish and sessile life in marine systems are very limited in comparison with studies on the biological effect of wood in riverine and estuarine environments.
In this paper, we explore the potential for marine restoration by creating biodiversity hotspots by introducing biodegradable reefs from organic material to mimic the presence of sunken driftwood in the subtidal benthic environments of the Wadden Sea. As wood was naturally present within the Wadden system in the past, both in the form of in-situ stumps (Franken et al., 2023) as well as riverine and ocean borne sunken driftwood (Wohl and Iskin, 2021), we chose to create biodegradable reefs out of fruit orchard trees to simulate historical natural substrates. Moreover, short-stemmed fruit trees are widely available in large quantities from commercial orchards when the trees reach the end of their economically productive lifespan. Whereas the use of wood to create reef-like structures has been well established in freshwater systems like rivers and lakes (Lehane et al., 2002; Whiteway et al., 2010), to our knowledge only few such studies have been conducted in marine environments (Lima et al., 2019). These studies show that wooden reefs undergo rapid colonization and increased fish use compared to traditional artificial reef material such as concrete (Chapman and Clynick, 2006; Alam et al., 2020). Previous work on wood reintroduction within rivers has demonstrated a positive increase in fish habitat in areas with wood placement, leading to a large increase in fish biomass and density (Lehane et al., 2002; Whiteway et al., 2010). In this study, we test if structures made of trees may be successful in enhancing local biodiversity and biota abundances in a temperate coastal sea. Specifically, we 1) quantified which sessile benthic organisms settle on these tree-reefs, 2) investigate whether these organisms show patterns of vertical zonation depending on distance from the sea floor, and 3) test if the structures have effects on mobile species in the vicinity of the tree-reefs.
Methodology
Study site
The Wadden Sea formed 7500 years ago as sea levels rose at the southern expanses of the North Sea region. It is bounded to the south by the European mainland and to the north by a chain of barrier islands (Reise, 2005; Lotze, 2007). Today, it is a shallow and dynamic soft bottom marine system, consisting of approximately 56% intertidal flats, and 44% submerged flats and tidal channels (Ricklefs et al., 2022). Strong tidal currents feature prominently as the tide moves in and out of the Wadden Sea twice a day through the tidal inlets between the barrier islands, carving gullies up to 50m deep between the barrier islands where they enter the system. These gullies then split into smaller channels, ultimately flowing onto the shallow subtidal and intertidal flats. The movement of water in the channels leads to significant sediment entrainment and constant force against any objects, whether they are on the bottom or floating such as navigational buoys. Based on these characteristics of the system, we chose to perform this experiment towards the ends of four subtidal gullies (Figure 1A), where the bathymetric morphology is relatively stable. In addition, we chose the locations in areas closed to trawling and away from main shipping routes to minimize the inherent navigational hazards of submerged structures for ships. The precise locations were chosen to have a minimal water depth of three meters during low tide; the tidal amplitude is approximately 1.5 meters. This research took place in the tidal basin ‘Eijerlandse Gat’, in between the islands of Dutch barrier islands Texel and Vlieland (Figure 1A). At each of the four sites, we selected two plots – one reef plot and a paired control 200 meters away from the reef plot at a similar depth.
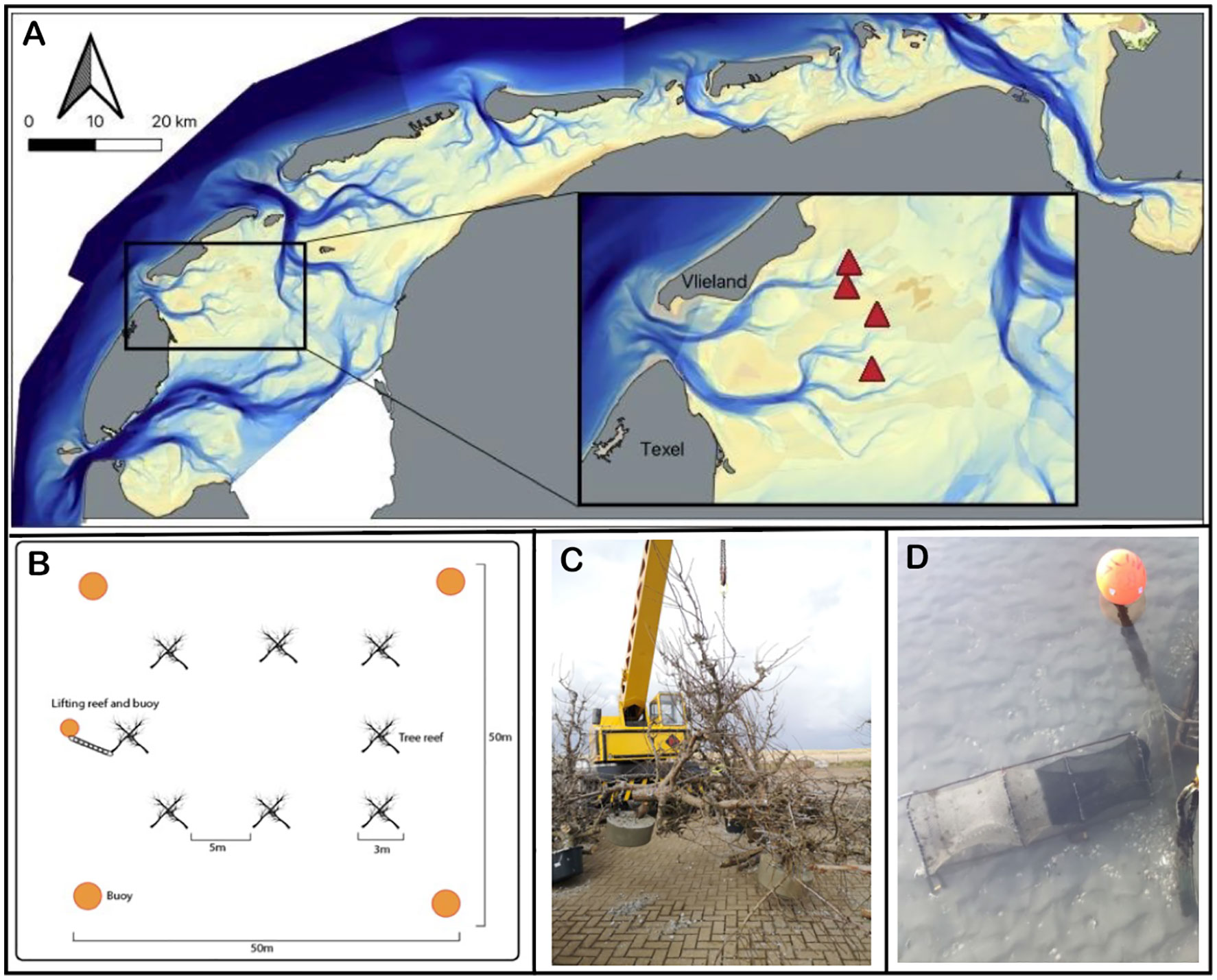
Figure 1 (A) Location of the tree-reefs (marked by triangles) in the Dutch part of the Wadden Sea. The inset provides a zoom in on the study area, showing that the tree-reef blocks (i.e., experimental plots marked by triangles) are located towards the ends of four subtidal gullies, where the morphology is relatively stable. (B) Within each tree-reef block, we placed eight tree-reef units with five meter distance in between. (C) Each tree-reef unit consisted of six short-stemmed fruit trees, bolted together to form a pyramid shape. That is, three trees (upright legs) had a concrete weight at their base, and were bolted to three other trees (crossbeams) to create a self-reinforcing series of triangles that comprise the overall pyramid. (D) Picture of the barrel-fyke style net, referred to as traps, used to catch fish both nearby and away from the tree-reef.
Construction of tree-reef units
To build the reefs, we chose to use short-stemmed pear trees as these have high structural complexity, are sufficiently wide and sturdy enough to withstand constant force in the tidal gullies, and are relatively easy to obtain from orchards that are removing economically non-viable trees at the age of around 25-35 years old. By using short-stemmed trees, we reduce drag encountered in the tidal gullies of the Wadden Sea whilst maintaining strong structural stability of the tree-reefs. The pear trees used were approximately 2.5-3.5m tall, sourced from an orchard in the southwest of the Netherlands. Approximately 200 trees were loaded into four 40m3 shipping containers, taking care to preserve as many branches as possible. These trees were shipped to the Royal Netherlands Institute for Sea Research on the island of Texel (53.006 N, 4.792 E) where they were assembled into 32 individual tree-reef units of six trees, each occupying approximately three cubic meters of total space, though not volume due to the large amount of interstitial spacing between tree trunks and branches. Three trees were secured together in a pyramid shape; these were joined with another three trees as horizontal crossbeams. Once the six trees were attached, concrete feet of approximately 100kg each were poured on each vertical tree leg, and a lifting chain was added for retrieval, observation and re-deployment (Figure 1C). See Supplementary Materials for more details on construction.
Placement of tree-reef blocks
In April 2022, eight tree-reef units per trip were loaded onto the deck of a cockle fishing ship. These were deployed at the study sites in a square pattern of approximately 25 m2 (Figure 1B). For navigational safety, four large yellow buoys of 1.12 m were deployed 25 m out from each corner of the reef square: these were attached to 125 kg concrete weights of approximately 80 cm diameter and 30 cm height, secured by eight meters of 20 mm chain. Control plots were also marked by these buoys, though only two buoys were used since there are no hazards to navigation in control plots.
Tree reef sampling: sessile organisms
Within each of the experimental plots of eight tree-reefs, we created one tree-reef unit that can be repeatedly lifted on deck of a ship and returned to the sea after monitoring. After construction, these units were monitored once during summer, as calm seas are an important safety consideration in the lifting process. In August 2022, four months after placement, we performed our first monitoring round. After lifting, the tree-reef unit was subsequently surveyed for sessile organisms. Transects denoting sessile organisms living on the wood as well as snagged macroalgae were taken from the base of the concrete weights to the top of the branches using 10 cm sections, i.e., 0-10, 11-20, 21-30 cm and so on, until the top of the tree was reached. The height of the upright trees ranged from 260 to 370 cm, depending on the height of the uppermost twigs. Towards the base of the vertical tree-legs, the diameter of the trees widened. We choose to limit the area examined for sessile organisms to five cm on either side of the transect tape, i.e. the maximum surveyed area per segment was 100 cm2.
We carried out this process for each of the three upright tree-reef legs. Where applicable, relevant abiotic factors such as sand, anoxic sand, dead barnacles and peat were also noted. When monitoring was complete, the reef unit was returned to the reef block. Monitoring sessions were carried out in less than an hour in order to minimize sessile organism mortality.
Tree reef sampling: fish populations
Visually monitoring fish populations in the Wadden Sea channels is generally not possible due to extremely limited visibility (~30 cm) and strong currents. For assessing fish populations around tree-reef blocks, we therefore elected to use fish traps; these exert fishing pressure mainly on benthic fish. These are one-meter-long free-floating barrel fyke style nets, see Figure 1D. An individual trap measured 1m x 25 cm x 25 cm and has a 15 cm net opening, with 14mm mesh sizing. It was attached to a 15 kg concrete weight with a rope to allow it to turn and stabilize in the tidal currents. We carried out our first fish monitoring round in October 2022, six months into the experiment. At each location, we deployed three traps at the reef perimeter, while three were placed at the bare sediment control site. We maintained about 20 meter spacing between the individual traps at both reef and control sites. The traps were lifted after 24 hours after which the catch was tallied, measured, and emptied. Each trap was then replaced back at the same location to repeat the same procedure. After a second 24 hour period, the traps were removed from the water and the catch was again assessed. All individuals were identified, counted and their length measured. All fish and other mobile species that were caught were returned to the water after measurement.
Statistical analysis
All statistical analyses were performed in R (version 4.1.2, R Core Team, 2022) using the RStudio (version 2022.12.0) interface. To create an overview of observed sessile organisms, all instances where a species was recorded per 10 cm section of transects along the three upright tree leg transects was scored as ‘present’ and was summed per reef block. We fitted a generalized linear model with a Poisson distribution in lme4 (Bates et al., 2015) to check for differences in observed organisms, followed by a post-hoc Tukey test using the ‘glht’ function from the multcomp package (Hothorn et al., 2008). Subsequently, the vertical distribution of total cover percentage, summed for all observed taxonomic groups along the legs of the tree-reefs was tested. We used a linear mixed effects model, with a quadratic term for heights to allow for an optimum in the distribution. As a random effect the individual tree-legs were nested within tree-reef. For the graphs, the predicted fits are based on the models without nesting factors. For the most dominant taxa, this analysis was repeated in order to obtain estimates for the vertical distributions of individual taxonomic groups.
For statistics on mobile species richness, the data from both 24 hour sets of three traps per reef block - treatment combination were aggregated and this value was used in a paired t-test. For the numbers of caught individuals of both fish and crustaceans, as well as for the differences in length of the most commonly caught fish species, five-bearded rockling (Ciliata mustela), the six traps per reef block - treatment combination were considered as pseudoreplicates. These were tested with a linear mixed effects model, with treatment (reef versus control) as fixed effect, and reef block as random effect using package lmerTest (Kuznetsova et al., 2017). All assumptions for the used statistical tests were visually checked using the ‘check_model’ function from the performance package (Lüdecke et al., 2021), and all plots were created using the ggplot2 package (version 3.3.6, Wickham, 2016).
Results
Vertical zonation of sessile organisms
In total, 15 taxa of sessile organisms were observed on the diagonal legs of the tree-reefs. There were large differences in the number of occurrences of species on the tree-reefs (2(14) = 701, p < 0.001; Figure 2). Barnacles and hydroid polyps were clearly the most frequently observed taxa (z-value 8.2 and 7.9, respectively; p < 0.001 for both; Figure 2). The next most dominant groups (Molgula, Bryozoa, Ulva sp. and Asterias rubens) all had an average of > 10 occurrences on the tree-reefs. Additionally, the total percentage cover along the diagonal legs of the tree-reefs showed increased percentage cover with elevations higher above the sediment, which leveled off towards the highest sections of the tree-reefs (Figure 3). The full model was able to explain 27% of the observed variation (conditional R2 = 0.27), while both height and the quadratic term of height were highly significant in explaining the fit of the model (t(414) = 5.94, p < 0.001 and t(414) = -6.45, p < 0.001, respectively). The highest total cover percentage of sessile organisms was found slightly below 200 cm along the diagonal (upright) tree legs, which, at an angle of ~50, corresponds to approximately 1.5 meters above the sediment.
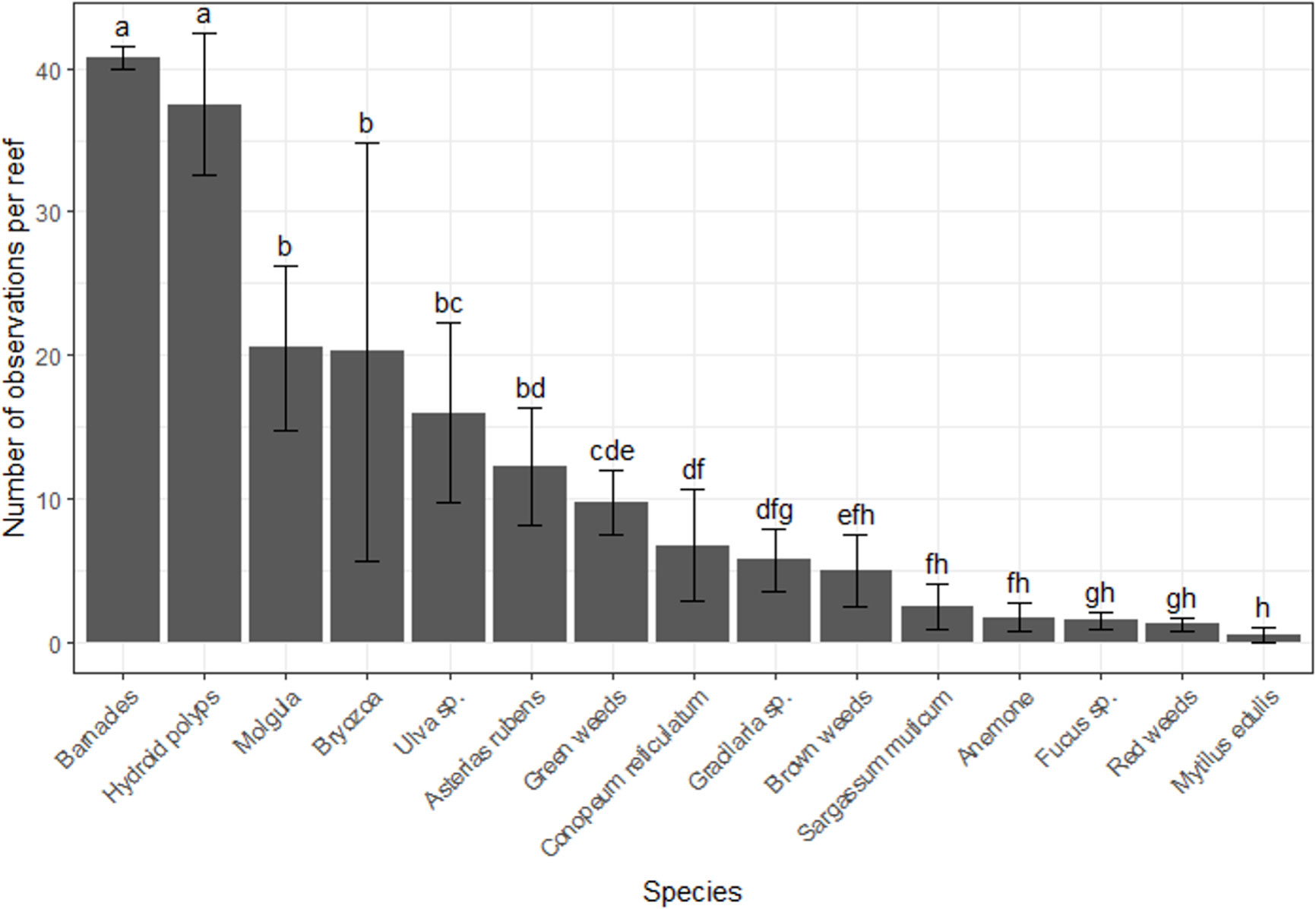
Figure 2 Number of 10 cm sections on the vertical tree legs on which species were observed. A species was recorded as present in a tree-leg segment if the cover exceeded 1%, the minimal value that was recorded in the field. Error bars indicate the standard error, and differences in letters indicate significant differences between the taxa at the p < 0.05 level.
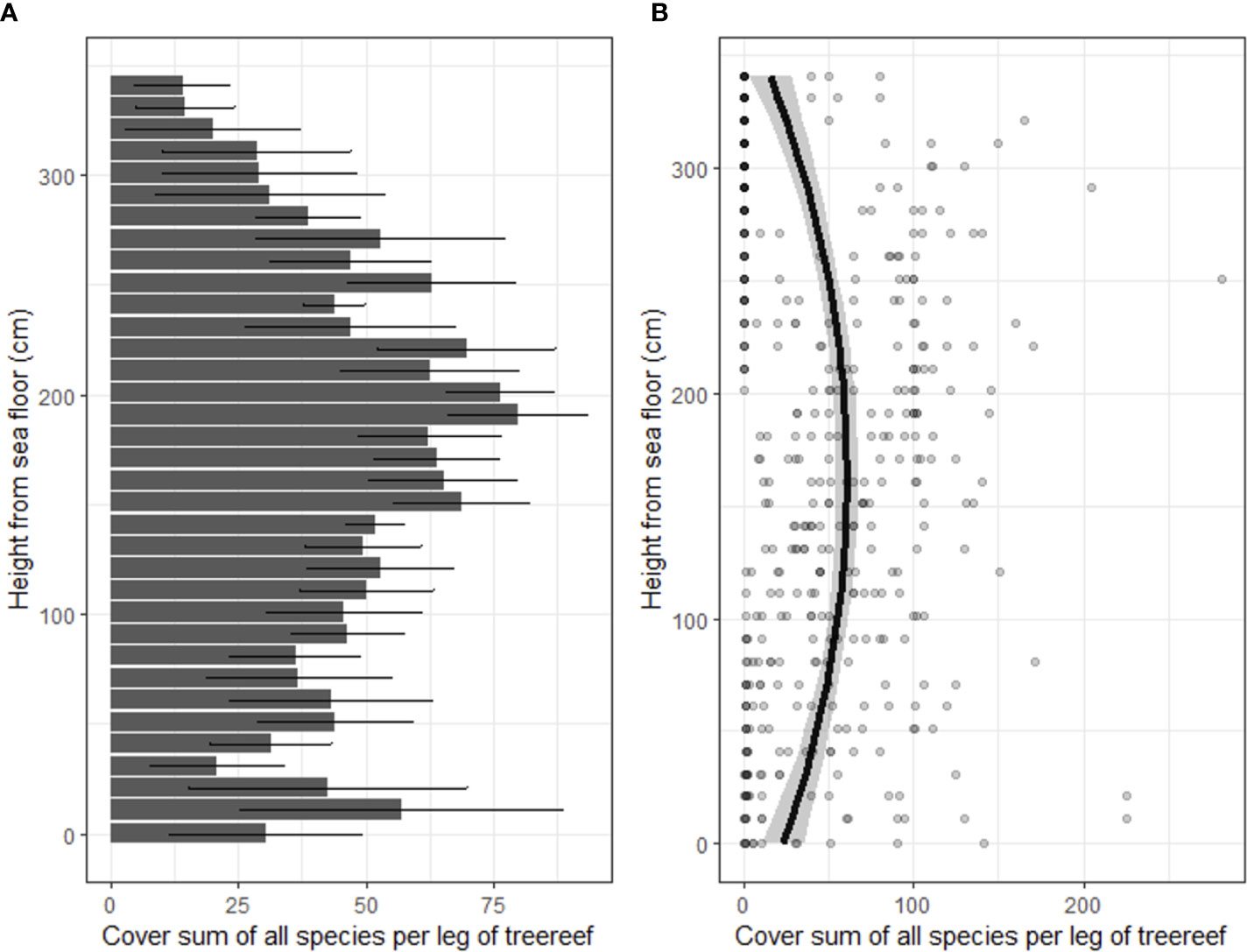
Figure 3 Total cover of all observed species combined and expressed as average of the tree legs and the reef blocks. (A) Mean cover with error bars representing the standard error. (B) Cover per height as individual points, including model fit with confidence intervals.
We found a biota settlement gradient in optimal elevation from the seafloor along the upright legs of the tree reefs, and that this optimal vertical distribution is species specific. The optima of occurrence of the different taxa along the tree-legs ranged from 221 cm for Bryozoans to 101 cm for Ulva sp. (Table 1). The six most abundant taxa (Figure 4) showed a highly significant association with the quadratic term for height in the logistic regression, indicating that there is an optimum of occurrence (Figure 2; Table 1).
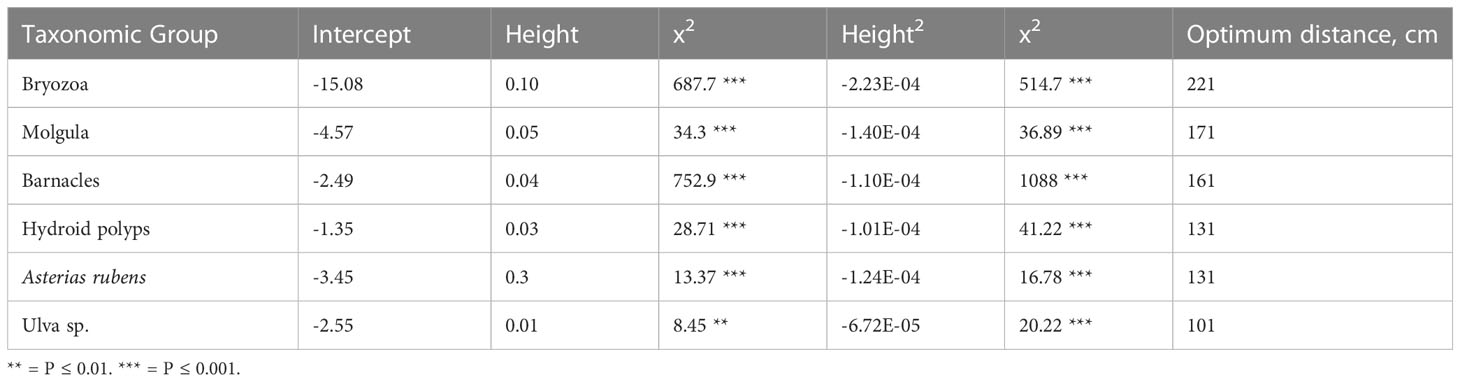
Table 1 Fit of logistic regressions of presence and absence of the six most dominant taxonomic groups over the diagonal (upright) legs of the tree reefs, ordered from the highest to the lowest optimum distance along the tree legs.
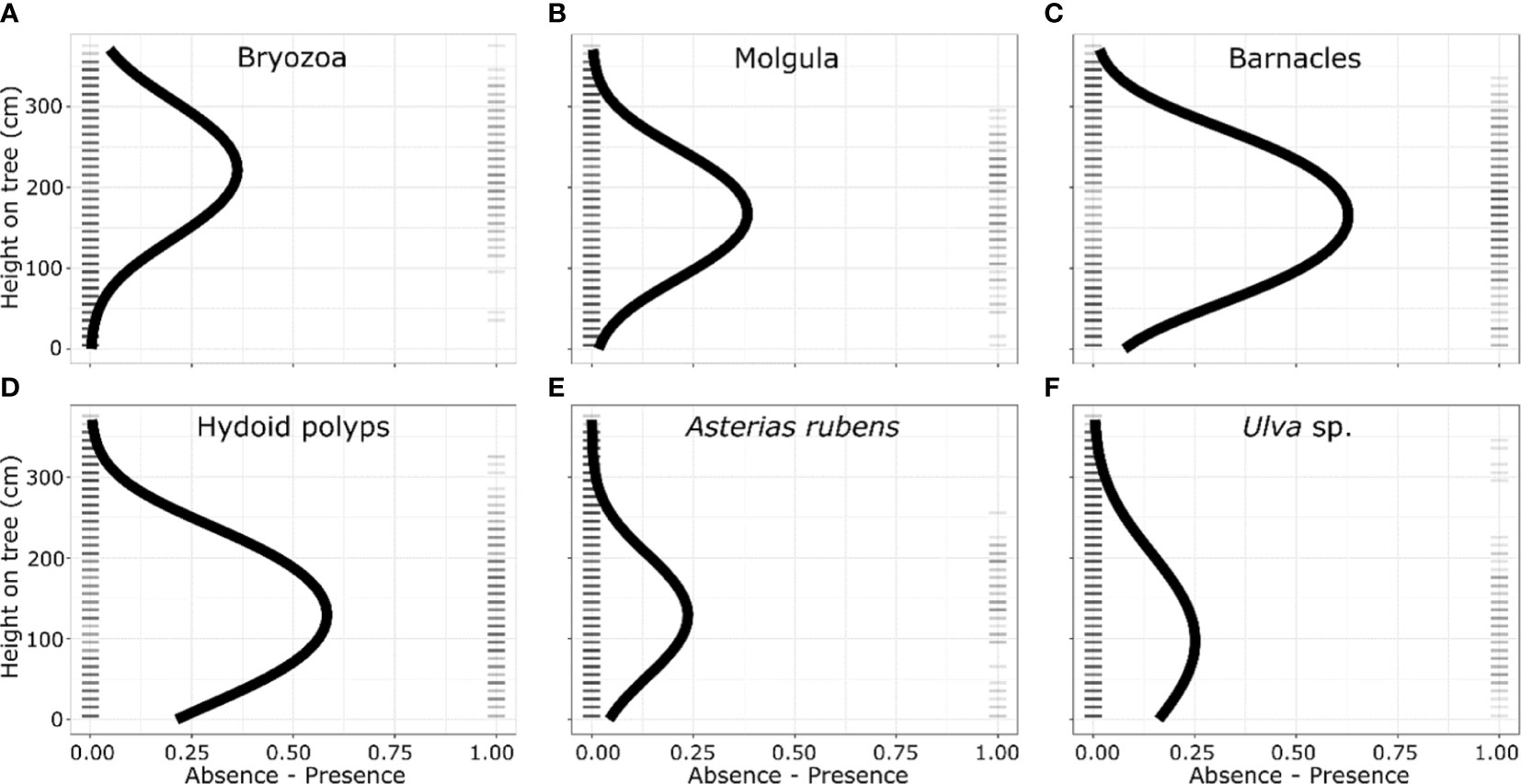
Figure 4 Vertical distribution of the presence and absence of the six most dominant taxa/species observed on the diagonal legs of the tree reefs, ordered from the highest to the lowest optimum distance along the tree legs: (A) Barnacles, (B) Hydroid polyps, (C) Molgula, (D) Bryozoa, (E) Ulva sp. and (F) Asterias rubens. Ticks indicate the presence and absence of the taxonomic group in a 10 cm section; while the curve is the predicted distribution based on logistic regression.
Tree reefs increase the number of mobile species
There were major differences in the catch of mobile species, such as fish, crabs, shrimp and prawns between the locations with tree reefs versus their control sites. These differences existed both in terms of the number of total caught individuals as well as the number of species that were caught (Figures 5, 6A). While the number of observed unique species on all reefs combined (10 mobile species, six of which were fish) was higher than the control sites (seven mobile species, two of which were fish), this difference was not significant when richness was paired per reef block (paired t-test: t(3) = 1.57, p = 0.22; t(3) = 1.99, p = 0.14; and t(3) = 0.52, p = 0.64 for all mobiles species combined, fish only and crustaceans only, respectively).
The total number of individual fish caught was about five times greater across all reef blocks (230 fish) compared to control sites (44 fish). On average, 10 fish were caught per trap on the reef sites, versus 2.9 in the control sites (t(34.2) = 4.728, p < 0.001; Figure 6B). Interestingly, this was not the case when looking at the catch of crabs, with the total number of individuals caught on (116) and off (109) reef being fairly equal. The average number of crabs caught per trap was 5.3 on reef sites, versus 5.0 on control sites; this was not found to be significant (t(39.0) = 0.663, p < 0.51; Figure 6C).
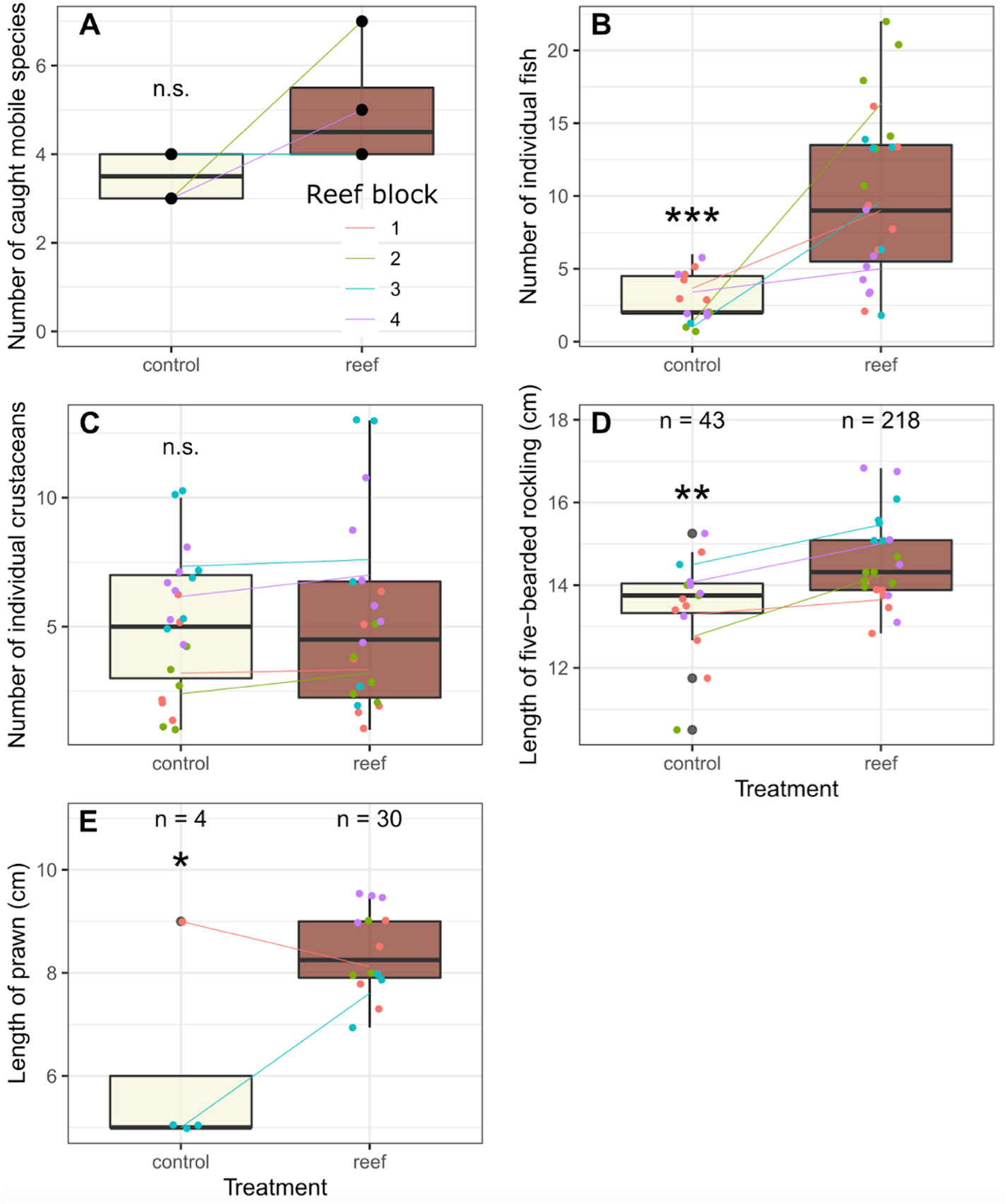
Figure 6 Results from fishing on Reef versus Control locations. (A) Total species richness observed at each location, where the data from the individual traps were combined in the analysis. (B) Total catch of individual fish. (C) The total catch of individual crabs. (D) Length of the most abundant fish species, the five bearded rockling (Ciliata mustela). (E) Length of prawn (Palaemon serratus) In all panels the colored lines indicate the differences between the control and reef sites within a reef block. For panel (B–D), the dots represent individual traps which are considered pseudoreplicates in the statistical analysis. * = P ≤ 0.05. ** = P ≤ 0.01. *** = P ≤ 0.001. ns = P > 0.05.
Of the fish species, the five bearded rockling (Ciliata mustela) was found to be the most dominant species by far, with 218 individuals caught on the reefs, versus 43 individuals at control sites, thereby constituting 95% of the total fish catch. Only one individual of another species (Myoxocephalus scorpius) was caught at the control sites. Other fish species that were found in low numbers on the reef locations were Trisopterus luscus (whiting-pout, n = 8), M. scorpius (shorthorn sculpin or bullrout, n = 1), Pholis gunnellus (rock gunnel, n = 1), Pomatoschistus microps (common goby, n = 1) and Anguilla anguilla (European eel, n = 1).
As C. mustela was abundant in both treatments, we included a comparison of body size between the individuals between the reef and control location. Individuals caught on reef sites were nearly one cm larger on average (14.4 vs 13.6 cm; t(33.7) = 2.76, p < 0.01; Figure 6D). The same pattern was observed for the prawn P. serratus, with 40 individuals observed on the reefs and only 4 in control sites. In addition, the length of the caught prawns differed between the sites, with larger individuals on the reefs compared to the control sites (8.0 vs 6.0 cm; t(14.4) = 2.88, p < 0.05; Figure 6E).
Discussion
The damming of rivers and the logging of forests along their banks has terminated the outflux of wood into estuaries of the last centuries, thereby severely limiting the availability of this natural hard substrate for marine reef communities. In line with wood reintroduction in rivers, we show that using short-stemmed fruit trees to build reefs that mimic the presence of historical sunken driftwood strongly increases the biodiversity and abundance of both sessile and mobile species on and around these tree reefs within six months after placement. Current findings highlight that initial colonization of natural tree-reefs is rapid and suggest that recovery of communities associated with woody substrates may be possible by active restoration. However, these results reflect developments over the first six months following reef introduction; further monitoring is required to gauge the longer-term performance of natural tree-reefs as a coastal restoration management tool.
The advantageous effect of tree-reef height on sessile organisms
Similar to other experimental studies introducing geogenic or biogenic hard substrates into soft-sediment coastal ecosystems (Alam et al., 2020) we found that the tree-reefs quickly developed into hotspots of sessile epibenthos biodiversity. Notably, we found a clear vertical zonation of species over the reefs’ vertical gradient. Bryozoans, molgula and barnacles grow well on the tree reefs, with a seeming preference for habitat approximately 100 to 250 cm above the sea floor. Hydroid polyps and algae, on the other hand, were found closer to the base of the reefs. This zonation may have several causes. The vertical height of the tree-reefs may facilitate recruitment as their profile increases the vertical substrate surface throughout the water column, which increases the probability of broadcast spawned larvae to encounter the structures compared to a low-profile reef (Wilhelmsson et al., 2006). The relative distancing from the seafloor as offered by tree-reefs likely also improved the survivability of sessile organisms, particularly for vulnerable bryozoans and molgula, as sand scour and burial probability decreases with reef height (Fodrie et al., 2014; Pondella et al., 2018). Moreover, apart from abiotic stress, predation pressure from benthic predators such as crabs, benthic fish, shrimp and sea stars likely also decreases with height (Motro et al., 2005). Overall, the addition of height by using trees, in this case pear trees, to build reefs offers advantages versus reef-building structures that lack this addition of height.
Sessile species occurrence and first signs of the start of succession
Bryozoans dominated the higher portions of the tree-reefs within four months of placement; these fast growing filter feeders thrive in strong currents (Hermansen et al., 2001) and provide nursery grounds and habitat for other species such as amphipods (Wood et al., 2012). They also provide additional surface area for some sessile organisms to attach to and hide within. Indeed, anecdotal observations on our tree-reefs revealed juvenile mussels residing within clumps of bryozoans. The solitary tunicate Molgula sp. was also frequently found, but was not yet observed in similarly high densities as the bryozoans. We expect tunicates and shellfish to increase in abundance as the tree-reefs develop, analogous to observations in studies describing ecological succession on other substrate types (Greene and Grizzle, 2007). We also expect Mytilus edulis to become dominant on the mid to upper reaches of the tree-reefs in the coming years, outcompeting bryozoans and tunicates for space in those areas where both physical stress and predation pressure are relatively low. Whether it is bryozoans, tunicates, or mussels, we expect these organisms to continue to enhance complexity, shelter, nursery habitat (Wood et al., 2012) and foraging opportunities for fish, copepods and other nekton (Rowden et al., 2007). We argue that the vertical structure promotes habitat in locations with reduced stress within the water column and that the 3D-complexity of the tree-reefs coupled with the internal interstitial spacing are key aspects in stimulating these ecological functions.
Tree-reef effects on fish and nekton
The Wadden Sea has seen fish population trends generally decline since the start of accurate record keeping in the 1960’s, with the most marked decline from the 1980’s onwards (van Walraven et al., 2017). In the last decade, estuarine fish and marine juvenile populations have generally stabilized at significantly lower than historic levels, while diadromous and endangered fish species trends are mainly in decline, stable, or uncertain (Ricklefs et al., 2022). It is important to note this is a generalization as specifics cannot be discussed in this paper; the takeaway message here is that fish populations are significantly less than what they once were throughout the Wadden Sea.
The abundance and species richness of the fish community and the abundance of prawn was greatly elevated around the reef sites. We found fish populations within the reef blocks to be at least fivefold more numerous than control sites when averaged across all locations, while the number of species was also three times higher. It is clear that the fish we caught at reef sites not only have a strong preference for reefs versus sandy bottoms, but are also bigger and comprised of more species, although for most species their count was too low to draw significant conclusions for the individual species. However, the most numerous caught fish was Ciliata mustela (five-bearded rockling), an important food source for species such as cod (Dando, 1975), herring (Hinrichs, 1985) and seals (Kavanagh et al., 2010; Aarts et al., 2019); showing the presence of C. mustela may be an important underlying link within the local food web. Overall, the witnessed increase in fish abundance around our tree-reefs is in line with findings from other hard substrates within predominantly soft bottom systems as well. This is borne out by studies in the North Sea (Reubens et al., 2013), Portugal (Coelho et al., 2012), Florida (Bohnsack et al., 1994) and others.
C. mustela and T. luscus were the only two fish species that were caught more than once per treatment in our fishing surveys, with 84% of C. mustela and 100% of T. luscus associated with the reef treatments. Both species cover a broad geographical range that includes the Wadden Sea areas - C. mustela is found from Portugal to northern Norway (Froese and Pauly, 2023) and T. luscus is found from Morocco to southern Norway (Froese and Pauly, 2023). Indeed, both species are known to be associated with structure (Dando, 1975) and hard substrate (Reubens et al., 2011; Reubens et al., 2013), explaining why these species were retrieved mostly on the tree reefs rather than at control sites. However, based on our data, we cannot say for certain as to whether the tree-reefs are simply aggregating them or if they also increasing the overall abundance of these fish in the area. As many of the fish caught were adults older than the reefs themselves, these early results suggests that aggregation is certainly one of the underlying mechanisms. Yet, it is possible that over time, the reefs also increase the overall abundance of these species by facilitating survival and/or growth by increasing foraging and hunting opportunities, as well as serving shelter from predation and hydrodynamics, or a combination of these factors. Determining which specific factor that influences fish density around these reefs is too early to say and may not be possible in the current experimental set up.
Alongside the increased number of fish found at tree reef sites, the patterns of prawn (Palaemon serratus) distribution show that there are approximately tenfold as many prawns at reef versus control sites; furthermore, the prawns at reef sites are larger. This increase may have important implications for their predators such as cod. Both C. mustela and P. serratus, themselves primary and secondary consumers, play an important role linking trophic levels. By consuming primary and secondary producers, they facilitate energy transfer through the food web to higher trophic levels, such as the threatened and commercially important cod Gadus morhua (Dando, 1975; Haig et al., 2014).
Although our monitoring revealed clear differences in fish communities between reefs and bare areas, our catch method may have underestimated the differences for cryptic fish species. When we lifted the reefs on deck to monitor sessile organisms, gobies (P. microps) and gunnels (P. gunnelus) fell out of the trees onto the deck of the ship – approximately tenfold more than what appeared in our catches. Although we cannot surmise the actual populations of these fish living around the reefs due to probable selectivity of the traps, we expect that the true populations of these fish around the tree-reefs are higher than quantified in this paper.
Further inferences from the fish catch data indicate that it is not solely juvenile fish that have been using these reefs within the first six months. The mean size of rockling caught on reef (14.4 cm), with some rockling reaching 19 cm shows that many of these fish are older than one year; the average one-year size of a rockling varies from 11-14 cm, depending on sex (Munk and Nielsen, 2007). The measured Trisopterus luscus (whiting-pout) caught on reef strengthens this inference; the average size of T. luscus was 17.2 cm. This fish reaches about 15 cm after one year (Dipper, 2001). These findings reinforce the idea that both juveniles and fish that are >1 year old are utilizing these structures, showing that fish do not necessarily have to spawn near the reefs to find and use them.
It is important to note that our data encompasses only a single sampling point in time (October 2022 for fish populations and August 2022 for sessile organisms). Therefore, seasonal migration and growth patterns of organisms are not included in this study, while they are likely to play a significant role. We expect that the fish populations and communities of sessile organisms will change through the seasons, as changes in water temperature will affect biological growth, fish migration patterns and larval availability and settlement (Wilhelmsson et al., 2006; van Walraven et al., 2017). Also, successional changes over time may take place. For example, it was found that while whiting-pout colonized wind turbine scour protection rock armour in the North Sea almost immediately, it took approximately a year for cod to follow suit (Reubens et al., 2013). We expect that as the tree-reef communities mature over the coming years, fish and sessile community composition will also change over time.
Tree reefs as compared to existing traditional artificial reefs
Artificial reefs are used near-globally for habitat enhancement and to recover biodiversity (Scarcella et al., 2015; Paxton et al., 2020); these reefs are composed of many different materials and designs. Along with intentional shipwrecks and oil platforms, artificial reefs are also built of concrete, metal, rock, plastic, tires, industrial material, clay, fibreglass, and most pertinently, wood (Lima et al., 2019; Guo et al., 2021). This latter material has been used in artificial reefs for its low cost, lack of toxicity, low-to-no carbon emissions required for construction, and general compatibility with marine environments. In other words, wood has generally been used as a material of convenience rather than a mimic of historical natural substrates such as trees (Wohl and Iskin, 2021) that entered rivers and floated to sea, some of which became waterlogged, colonized, and eventually non-buoyant, sinking to the sea floor. As a material of convenience, most of this wood used for artificial reefs is processed timber. As a result of this, however, it loses much of the natural complexity created by branches and roots. Moreover, wood used in marine environments is often chemically treated to increase its longevity. Although this has not been found to be problematic for epifaunal settlement (Brown, 2005) it is noted that exposure of epifaunal life to toxins leaching from artificial substrates can affect community composition (Wilding, 2002). In the case of tires and some metallic structures, toxic substances leaching from these reefs has proven to negatively affect the biota (Aleksandrov et al., 2002; Lima et al., 2019). Wood, even if treated, is a highly suitable substrate for epifaunal assemblages (Brown, 2005), and wooden artificial reefs are colonized significantly more quickly by more species and larger fish than alternatives such as PVC or steel (Masuda et al., 2010; Alam et al., 2020).
Currently, our tree-reefs are still young in terms of reef succession and physical degradation. Existing reefs made using nearly-whole trees are not widespread if present at all; the nearest comparison is the relatively common practice of using old Christmas trees as fish attraction devices and fish habitat (Tugend et al., 2002). However, compared to hardwood fruit trees such as cherry, pear, and apple, these softwood trees such as pine, spruce, and fir degrade more quickly. Though the density of each tree species varies, in general hardwoods are much denser and are therefore more durable and less buoyant. In freshwater, twigs and branches of Christmas trees, generally a spruce or fir, can decompose within eighteen months and the majority of the tree itself within five years (D. Nygren, personal communication, 2022). Similar degradation of finer branches and leaves was noted in marine environments, with the branches of Japanese cypress trees, a softwood, decomposing within the space of 18 months (Alam et al., 2020). Consequently, complex softwood types prove only a short-term solution as an artificial reef. Conversely, larger and denser wood, such as many hardwoods, can last decades or centuries in suitable marine environments (Jurgens and Blanchette, 2005).
Consequences of tree-reef biodegradability for long-term development and OSPAR
Wood is a natural material that has been present in marine systems for millions of years; communities have evolved to live on and around sunken wood-falls (Kano et al., 2013). Along with providing habitat, however, it is also a food source for marine boring worms (Terinidae sp.) which actively consume wood. The rate of consumption depends on the density and size of wood, proximity to existing populations of Terinidae, water temperature, oxygen levels and existing immediate presence of wood worms (Quayle, 1992). The rate of consumption by Terinidae also varies whether it is heartwood or sapwood being consumed; heartwood is noticeably more durable in most species (Ajuong et al., 2014). In other words, the internal structure of the tree is generally longer lasting than the perimeter sapwood. Though wood will eventually be consumed and/or decompose within most marine environments, it will take years to decades of time; long enough for biogenic shellfish reefs to establish and mature on the wooden reef ‘skeleton’. Along with a variety of hard substrates, mussels have also been found to settle on tubeworm beds, individual cockle shells, and even bare sand and silt. They accumulate mud which may be up to 0.5m thick, thus completely outgrowing their initial substrate (Brinkman et al., 2002; de Paoli et al., 2015) while maintaining a shellfish reef. After this settlement and eventual collapse of tree-reefs, it is likely that the established reef community will by continue to function, based on the structures generated by the reef communities themselves, especially when habitat-forming species such as mussels are part of the community.
In fact, the temporary nature of these biodegradable reefs may be beneficial. Indeed, OSPAR guidelines (OSPAR Commission, 2013) indicate that all artificial reefs must be removable. Although this legislation is regarded by some people to be outdated, it is a factor to consider. In the case of tree-reefs, ‘removable’ can be synonymous with ‘degradable’ as the reef community itself will conduct the ‘removal work’ at no cost or effort to humans while facilitating nutrient cycling. As such, introduction of woody substrates via tree-reefs can allow humans to build with nature to restore benthic hard substrate-associated communities in soft-sediment ecosystems with little risk and at relatively low costs.
The benefit of using short-stemmed fruit trees for tree-reef construction
Along with the fact that tree-reefs act as a mimic of historical wood within marine systems (Kaim, 2011; Kano et al., 2013; Voight, 2015; Wohl and Iskin, 2021), our tree-reefs are made out of waste product: economically nonviable orchard trees. Short-stemmed trees cultivated for fruit production within orchards have a limited economically productive lifespan: depending on conditions and type of trees, this can be anywhere from 10-35 years. Once these hardwood trees such as pear, cherry or apple are no longer economically viable, they are removed from orchards. Generally, these trees are then turned into firewood, wood-pellets for fuel, composted or simply sent to landfill. As such, orchard trees past their economic ‘best-by’ date are a low value product and can therefore be acquired relatively cheaply and in large numbers. This experiment comprised a total of 192 pear trees harvested from approximately 0.25 hectares. In the Netherlands alone, approximately 400 hectares of fruit trees are cleared annually (T. Bouma, personal communication, 2021). Therefore there is a large and renewable supply of these trees nearly anywhere fruit orchards are cultivated, making them a relatively local, natural, biodegradable low-carbon-emission and highly productive material to build artificial reefs with. From an engineering perspective, it is an advantage that fruit trees are nowadays all short-stemmed. It allows for easier transport and limits the drag forces encountered in the sea while improving the structural integrity of the structures; the use of taller trees, while possible, would require more effort to construct tree-reefs.
Management implications and perspectives for tree reefs
The relative profusion of life on and around the tree-reefs in the first six months of deployment has shown that despite the fact that driftwood is no longer present in the Wadden Sea in historical abundance, sunken wooden structures can fulfil an important role in hosting and maintaining biodiverse ecosystems in what is now a predominantly sandy-bottomed system. Tree-reefs may have important ecological ramifications for nature managers in the Wadden Sea specifically, and in shallow temperate coastal seas in general. It is clear that even this relatively small-scale introduction of tree-reefs has had a positive impact on the diversity and abundance of local biological communities - from bryozoans to prawns to predatory fish. Further monitoring is ongoing, and community shifts are anticipated as the reefs mature. Though not all benthic systems will be suitable for tree-reefs, our first results suggest that they offer a new and exciting tool for nature managers and restoration practitioners to enhance biodiversity and restore local food webs.
Data availability statement
The raw data supporting the conclusions of this article will be made available by the authors, without undue reservation.
Author contributions
JD wrote the majority of the paper and invented the tree-reefs along with experiment design, conducted fieldwork, data entry, sourced materials, etc. OF conducted the majority of the statistical analysis and helped deploy the tree-reefs. MW conducted some fieldwork and assisted with construction and deployment. BM assisted with construction, deployment and monitoring of tree-reefs. SH helped with permit applications to government authorities, along with material sourcing. All of OF, MW, LG, TH, and TB contributed to editing and suggestions within the paper. TB had the inspiration to stick pear trees in the water as reefs. All authors contributed to the article and approved the submitted version.
Funding
This study was funded by Waddenfonds, Rijkswaterstaat, and the Dutch provinces of North Holland, Friesland and Groningen and is registered as ‘Waddentools: habitatheterogeniteit’ (also known as Waddenmozaïek) with reference number WF2018-187059.
Acknowledgments
The authors wish to thank Captains Jouke Visser of the HA-2, Willem-Anton Schagen of the TX-96, as well as Jan Hottentot and Pieter Slik of the TX-56 for their invaluable assistance in deploying and monitoring the tree-reefs. Furthermore, our thanks are extended to the numerous volunteers who helped with the loading and unloading of trees at the onset of this experiment. We thank the editor and reviewers for their helpful comments on an earlier version of this manuscript.
Conflict of interest
The authors declare that the research was conducted in the absence of any commercial or financial relationships that could be construed as a potential conflict of interest.
Publisher’s note
All claims expressed in this article are solely those of the authors and do not necessarily represent those of their affiliated organizations, or those of the publisher, the editors and the reviewers. Any product that may be evaluated in this article, or claim that may be made by its manufacturer, is not guaranteed or endorsed by the publisher.
Supplementary material
The Supplementary Material for this article can be found online at: https://www.frontiersin.org/articles/10.3389/fmars.2023.1213790/full#supplementary-material
References
Aarts G., Brasseur S., Poos J. J., Schop J., Kirkwood R., Kooten T., et al. (2019). Top-down pressure on a coastal ecosystem by harbor seals. Ecosphere 10. doi: 10.https://doi.org/10.1002/ecs2.2538
Ajuong E., Freitag C., Morrell J. J. (2014). Decay resistance and extractive content of second-growth port orford cedar (Chamaecyparis lawsoniana) wood. Wood AND FIBER Sci. 46, 502–509.
Alam J. F., Yamamoto T., Umino T., Nakahara S., Hiraoka K. (2020). Modeling the efficacy of three types of artificial timber reefs in mitsu bay, Japan. Water 12, 2013. doi: 10.3390/w12072013
Aleksandrov B. G., Minicheva G. G., Strikalenko T. V. (2002). Ecological aspects of artificial reef construction using scrap tires. Russian J. Mar. Biol. 28.
Bates D., Maechler M., Bolker B., Walker S. (2014). Fitting linear mixed-effects models using lme4. J. Stat. Software 67 (1), 1–48. doi: 10.18637/jss.v067.i01
Beck M. W., Brumbaugh R. D., Airoldi L., Carranza A., Coen L. D., Crawford C., et al. (2011). Oyster reefs at risk and recommendations for conservation, restoration, and management. BioScience 61, 107–116. doi: 10.1525/bio.2011.61.2.5
Beukema J. J., Cadée G. C. (1999). An estimate of the sustainable rate of shell extraction from the Dutch Wadden Sea. J. Appl. Ecol. 36, 49–58. doi: 10.1046/j.1365-2664.1999.00380.x
Bohnsack J. A., Harper D. E., McClellan D. B., Hulsbeck M. (1994). Effects of reef size on colonization and assemblage structure of fishes at artificial reefs off southeastern florida, U.S.A. University of Miami - Rosenstiel School of Marine, Atmospheric & Earth Science 55, 796–823.
Borsje B. W., van Wesenbeeck B. K., Dekker F., Paalvast P., Bouma T. J., van Katwijk M. M., et al. (2011). How ecological engineering can serve in coastal protection. Ecol. Eng. 37, 113–122. doi: 10.1016/j.ecoleng.2010.11.027
Brinkman A., Dankers N., Van Stralen M. (2002). An analysis of mussel bed habitats in the Dutch Wadden Sea. Helgol Mar. Res. 56, 59–75. doi: 10.1007/s10152-001-0093-8
Brown C. J. (2005). Epifaunal colonization of the Loch Linnhe artificial reef: Influence of substratum on epifaunal assemblage structure. Biofouling 21, 73–85. doi: 10.1080/08927010512331344197
Bull A. S., Love M. S. (2019). Worldwide oil and gas platform decommissioning: A review of practices and reefing options. Ocean Coast. Manage. 168, 274–306. doi: 10.1016/j.ocecoaman.2018.10.024
Chapman M. G., Clynick B. G. (2006). Experiments testing the use of waste material in estuaries as habitat for subtidal organisms. J. Exp. Mar. Biol. Ecol. 338, 164–178. doi: 10.1016/j.jembe.2006.06.018
Coelho R., Monteiro P., Abecasis D., Blot J. Y., Gonçalves J. M. S. (2012). Macrofauna assemblages in a XVIIth century shipwreck: comparison with those on natural reefs and sandy bottoms. Braz. J. oceanogr. 60, 447–462. doi: 10.1590/S1679-87592012000400004
Coolen J. W. P., van der Weide B., CuPerus J., Blomberg M., Van Moorsel G. W. N. M., Faasse M. A., et al. (2020). Benthic biodiversity on old platforms, young wind farms, and rocky reefs. ICES J. Mar. Sci. 77, 1250–1265. doi: 10.1093/icesjms/fsy092
Dando P. R. (1975). On the northern rockling Ciliata septentrionalis (Collett) in the Plymouth area. J. Mar. Biol. Ass. 55, 925–931. doi: 10.1017/S002531540001780X
de Paoli H., van de Koppel J., van der Zee E., Kangeri A., van Belzen J., Holthuijsen S., et al. (2015). Processes limiting mussel bed restoration in the Wadden-Sea. J. Sea Res. 103, 42–49. doi: 10.1016/j.seares.2015.05.008
Didderen K., Lengkeek W., Kamermans P., Deden B., Reuchlin-Hugenholtz E., Bergsma J. H., et al (2018). Pilot to actively restore native oyster reefs in the North Sea: Comprehensive report to share lessons learned in 2018 Bureau Waardenburg / WWF, Culemburg / Zeist, Netherlands.
Eriksson B. K., van der Heide T., Van De Koppel J., Piersma T., van der Veer H. W., Olff H. (2010). Major changes in the ecology of the wadden sea: human impacts, ecosystem engineering and sediment dynamics. Ecosystems 13, 752–764. doi: 10.1007/s10021-010-9352-3
Evans M., Warburton J. (2001). Transport and dispersal of organic debris (peat blocks) in upland fluvial systems. Earth Surf. Process. Landforms 26, 1087–1102. doi: 10.1002/esp.256
Fariñas-Franco J. M., Pearce B., Mair J. M., Harries D. B., MacPherson R. C., Porter J. S., et al. (2018). Missing native oyster (Ostrea edulis L.) beds in a European Marine Protected Area: Should there be widespread restorative management? Biol. Conserv. 221, 293–311. doi: 10.1016/j.biocon.2018.03.010
Fitzsimons J. A., Branigan S., Gillies C. L., Brumbaugh R. D., Cheng J., DeAngelis B. M., et al. (2020). Restoring shellfish reefs: Global guidelines for practitioners and scientists. Conservat Sci. Prac 2. doi: 10.1111/csp2.198
Fodrie F. J., Rodriguez A. B., Baillie C. J., Brodeur M. C., Coleman S. E., Gittman R. K., et al. (2014). Classic paradigms in a novel environment: inserting food web and productivity lessons from rocky shores and saltmarshes into biogenic reef restoration. J. Appl. Ecol. 51, 1314–1325. doi: 10.1111/1365-2664.12276
Franken O., Dickson J., Holthuijsen S., Witte S., Smeele Q., Olff H., et al. (2023). Natuurlijk hard substraat belangrijk voor biodiversiteit Waddenzee (De Levende Natuur).
Giesen W. B. J. T., van Katwijk M. M., den Hartog C. (1990). Eelgrass condition and turbidity in the Dutch Wadden Sea. Aquat. Bot. 37, 71–85. doi: 10.1016/0304-3770(90)90065-S
Gonor J. J., Sedell J. R., Benner P. A. (1988). Chapter 4. What we know about large trees in estuaries, in the sea, and on coastal beaches. USDA For. Serv. Gen. Tech. Rep. GTR-PNW-229, Pacific Northwest Res. Sta. 83–112.
Greene J. K., Grizzle R. E. (2007). Successional development of fouling communities on open ocean aquaculture fish cages in the western Gulf of Maine, USA. Aquaculture 262, 289–301. doi: 10.1016/j.aquaculture.2006.11.003
Guo Z., Wang L., Cong W., Jiang Z., Liang Z. (2021). Comparative analysis of the ecological succession of microbial communities on two artificial reef materials. Microorganisms 9, 120. doi: 10.3390/microorganisms9010120
Haig J., Ryan N. M., Williams K. F. (2014). A review of the Palaemon serratus fishery: biology, ecology & management. Fisheries and Conservation Report. (Bangor: Bangor University).
Hermansen P., Larsen P. S., Riisg H. U. (2001). Colony growth rate of encrusting marine bryozoans žElectra pilosa and Celleporella hyalina/. J. Experimental Mar. Biol. Ecol. 263, 1–23.
Hinrichs R. (1985). Quantity and composition of food of herring and sprat in the Baltic Sea from 1980-1981. Fisch. Forsch 23, 70–81.
Hothorn T., Bretz F., Westfall P. (2008). Simultaneous inference in general parametric models. Biometrical J. 50, 346–363.
Houziaux J.-S., Fettweis M., Francken F., Van Lancker V. (2011). Historic, (1900) seafloor composition in the Belgian–Dutch part of the North Sea: A reconstruction based on calibrated visual sediment descriptions. Continental Shelf Res. 31, 1043–1056. doi: 10.1016/j.csr.2011.03.010
Jurgens J. A., Blanchette R. A. (2005). Characterization of wood destroying microorganisms in archaeological woods from marine environments. (Chile: Presented at the International Academy of Wood Science Annual Meeting).
Kaim A. (2011). Non-actualistic wood-fall associations from Middle Jurassic of Poland: Non-actualistic wood-fall associations. Lethaia 44, 109–124. doi: 10.1111/j.1502-3931.2010.00236.x
Kamermans P., Walles B., Kraan M., van Duren L., Kleissen F., van der Have T., et al. (2018). Offshore wind farms as potential locations for flat oyster (Ostrea edulis) restoration in the dutch north sea. Sustainability 10, 3942. doi: 10.3390/su10113942
Kano Y., Fukumori H., Brenzinger B., Waren A. (2013). Driftwood as a vector for the oceanic dispersal of estuarine gastropods (Neritidae) and an evolutionary pathway to the sunken-wood community. J. Molluscan Stud. 79, 378–382. doi: 10.1093/mollus/eyt032
Kavanagh A. S., Cronin M. A., Walton M., Rogan E. (2010). Diet of the harbour seal ( Phoca vitulina vitulina ) in the west and south-west of Ireland. J. Mar. Biol. Ass. 90, 1517–1527. doi: 10.1017/S0025315410000974
Kondolf G. M., Matthews W. V. G. (1986). Transport of tracer gravels on a coastal California river. J. Hydrology 85, 265–280. doi: 10.1016/0022-1694(86)90060-0
Kuznetsova A., Brockhoff P., Christensen R. (2017). lmerTest package: tests in linear mixed effects models. J. Stat. Software 82, 1–26. doi: 10.18637/jss.v082.i13
Lehane B. M., Giller P. S., O’Halloran J., Smith C., Murphy J. (2002). Experimental provision of large woody debris in streams as a trout management technique. Aquat. Conserv: Mar. Freshw. Ecosyst. 12, 289–311. doi: 10.1002/aqc.516
Lima J. S., Zalmon I. R., Love M. (2019). Overview and trends of ecological and socioeconomic research on artificial reefs. Mar. Environ. Res. 145, 81–96. doi: 10.1016/j.marenvres.2019.01.010
Lotze H. K. (2007). Rise and fall of fishing and marine resource use in the Wadden Sea, southern North Sea. Fisheries Res. 87, 208–218. doi: 10.1016/j.fishres.2006.12.009
Lotze H. K., Reise K., Worm B., van Beusekom J., Busch M., Ehlers A., et al. (2005). Human transformations of the Wadden Sea ecosystem through time: a synthesis. Helgol Mar. Res. 59, 84–95. doi: 10.1007/s10152-004-0209-z
Lüdecke D., Ben-Shachar M. S., Patil I., Waggoner P., Makowski D. (2021). An R package for assessment, comparison and testing of statistical models. J. Open Source Software 6, 31–39. doi: 10.21105/joss.03139
Masuda R., Shiba M., Yamashita Y., Ueno M., Kai Y., Nakanishi A., et al. (2010). Fish assemblages associated with three types of artificial reefs: density of aseemblages and possible impacts on adjacent fish abundance.
McMahon T. E., Holtby L. B. (1992). Behaviour, Habitat Use, and Movements of Coho Salmon ( Oncorhynchus kisutch ) Smolte during Seaward Migration. Can. J. Fish. Aquat. Sci. 49, 1478–1485. doi: 10.1139/f92-163
Molen J., García-García L. M., Whomersley P., Callaway A., Posen P. E., Hyder K. (2018). Connectivity of larval stages of sedentary marine communities between hard substrates and offshore structures in the North Sea. Sci. Rep. 8, 14772. doi: 10.1038/s41598-018-32912-2
Motro R., Ayalon I., Genin A. (2005). Near-bottom depletion of zooplankton over coral reefs: III: vertical gradient of predation pressure. Coral Reefs 24, 95–98. doi: 10.1007/s00338-004-0451-5
Murphy E., Nistor I., Cornett A., Wilson J., Pilechi A. (2021). Fate and transport of coastal driftwood: A critical review. Mar. pollut. Bull. 170, 112649. doi: 10.1016/j.marpolbul.2021.112649
Nagayama S., Nakamura F. (2010). Fish habitat rehabilitation using wood in the world. Landscape Ecol. Eng. 6, 289–305. doi: 10.1007/s11355-009-0092-5
Nauta J., Christianen M. J. A., Temmink R. J. M., Fivash G. S., Marin-Diaz B., Reijers V. C., et al. (2023). Biodegradable artificial reefs enhance food web complexity and biodiversity in an intertidal soft-sediment ecosystem. J. Appl. Ecol. 14348, 1365–2664. doi: 10.1111/1365-2664.14348
OSPAR Commission (2013). OSPAR_Guidelines on Artificial Reefs in relation to Living Marine Resources.
Paxton A. B., Shertzer K. W., Bacheler N. M., Kellison G. T., Riley K. L., Taylor J. C. (2020). Meta-analysis reveals artificial reefs can be effective tools for fish community enhancement but are not one-size-fits-all. Front. Mar. Sci. 7. doi: 10.3389/fmars.2020.00282
Pogoda B., Merk V., Colsoul B., Hausen T., Peter C., Pesch R., et al. (2020). Site selection for biogenic reef restoration in offshore environments: The Natura 2000 area Borkum Reef Ground as a case study for native oyster restoration. Aquat. Conserv: Mar. Freshw. Ecosyst. 30, 2163–2179. doi: 10.1002/aqc.3405
Pondella D., Williams J., Williams C., Claisse J., Witting D. (2018). Restoring a nearshore rocky reef ecosystem in the challenge of an urban setting. (Symposium, Bethesda, Maryland, USA: American Fisheries Society).
Ponti M., Linares C., Cerrano C., Rodolfo-Metalpa R., Hoeksema B. W. (2021). Editorial: biogenic reefs at risk: facing globally widespread local threats and their interaction with climate change. Front. Mar. Sci. 8. doi: 10.3389/fmars.2021.793038
Quayle D. B. (1992). Marine wood borers in British Columbia, Canadian special publication of fisheries and aquatic sciences. (Ottawa: Dept. of Fisheries and Oceans).
R Core Team (2022). “R: A language and environment for statistical computing,” in R foundation for statistical computing, vol. 2022. (Vienna, Austria). Available at: https://www.R-project.org/.
Reise K. (1982). Long-term changes in the macrobenthic invertebrate fauna of the Wadden Sea: Are polychaetes about to take over? Netherlands J. Sea Res. 16, 29–36. doi: 10.1016/0077-7579(82)90014-X
Reise K. (2005). Coast of change: habitat loss and transformations in the Wadden Sea. Helgol Mar. Res. 59, 9–21. doi: 10.1007/s10152-004-0202-6
Reubens J. T., Braeckman U., Vanaverbeke J., Van Colen C., Degraer S., Vincx M. (2013). Aggregation at windmill artificial reefs: CPUE of Atlantic cod (Gadus morhua) and pouting (Trisopterus luscus) at different habitats in the Belgian part of the North Sea. Fisheries Res. 139, 28–34. doi: 10.1016/j.fishres.2012.10.011
Reubens J. T., Degraer S., Vincx M. (2011). Aggregation and feeding behaviour of pouting (Trisopterus luscus) at wind turbines in the Belgian part of the North Sea. Fisheries Res. 108, 223–227. doi: 10.1016/j.fishres.2010.11.025
Ricklefs K., Franken O., Glorius S., Mascioli F., Nielsen P. (2022). Wadden sea quality status report [WWW Document].
Rowden A. A., Cranfield H. J., Mann P. J., Wood A. C. L. (2007). Benthic macrofauna bycatch of oyster dredging in Foveaux Strait (No. 10). New Zealand Fisheries Assessment Report.
Scarcella G., Grati F., Bolognini L., Domenichetti F., Malaspina S., Manoukian S., et al. (2015). Time-series analyses of fish abundance from an artificial reef and a reference area in the central-Adriatic Sea. J. Appl. Ichthyol. 31, 74–85. doi: 10.1111/jai.12952
Schlining K., von Thun S., Kuhnz L., Schlining B., Lundsten L., Jacobsen Stout N., et al. (2013). Debris in the deep: Using a 22-year video annotation database to survey marine litter in Monterey Canyon, central California, USA. Deep Sea Res. Part I: Oceanographic Res. Papers 79, 96–105. doi: 10.1016/j.dsr.2013.05.006
Schotanus J., Capelle J. J., Paree E., Fivash G. S., van de Koppel J., Bouma T. J. (2020a). Restoring mussel beds in highly dynamic environments by lowering environmental stressors. Restor. Ecol. 28, 1124–1134. doi: 10.1111/rec.13168
Schotanus J., Walles B., Capelle J. J., van Belzen J., van de Koppel J., Bouma T. J. (2020b). Promoting self-facilitating feedback processes in coastal ecosystem engineers to increase restoration success: Testing engineering measures. J. Appl. Ecol. 57, 1958–1968. doi: 10.1111/1365-2664.13709
Sguotti C., Lynam C. P., García-Carreras B., Ellis J. R., Engelhard G. H. (2016). Distribution of skates and sharks in the North Sea: 112 years of change. Glob Change Biol. 22, 2729–2743. doi: 10.1111/gcb.13316
Spalding M. D., Brown B. E. (2015). Warm-water coral reefs and climate change. Science 350, 769–771. doi: 10.1126/science.aad0349
Stuart-Smith R. D., Edgar G. J., Stuart-Smith J. F., Barrett N. S., Fowles A. E., Hill N. A., et al. (2015). Loss of native rocky reef biodiversity in Australian metropolitan embayments. Mar. pollut. Bull. 95, 324–332. doi: 10.1016/j.marpolbul.2015.03.023
Tan Y. M., Dalby O., Kendrick G. A., Statton J., Sinclair E. A., Fraser M. W., et al. (2020). Seagrass restoration is possible: insights and lessons from Australia and New Zealand. Front. Mar. Sci. 7. doi: 10.3389/fmars.2020.00617
Temming A., Hufnagl M. (2015). Decreasing predation levels and increasing landings challenge the paradigm of non-management of North Sea brown shrimp (Crangon crangon). ICES J. Mar. Sci. 72, 804–823. doi: 10.1093/icesjms/fsu194
Tiano J., Depestele J., Van Hoey G., Fernandes J., van Rijswijk P., Soetaert K. (2022). Trawling effects on biogeochemical processes are mediated by fauna in high energy biogenic reef–inhabited coastal sediments (preprint). Biogeochemistry: Coast. Ocean. doi: 10.5194/bg-2022-23
Tugend K., Allen S. M., Webb M. (2002). Use of artificial habitat structures in U.S. Lakes and Reservoirs: A Survey from the Southern Division AFS Reservoir Committee. Fisheries 27, 22–26.
van der Reijden K. J., Koop L., Mestdagh S., Snellen M., Herman P. M. J., Olff H., et al. (2021). Conservation implications of sabellaria spinulosa reef patches in a dynamic sandy-bottom environment. Front. Mar. Sci. 8. doi: 10.3389/fmars.2021.642659
van Katwijk M. M. (2003). Reintroduction of eelgrass (Zostera marina L.) in the Dutch Wadden Sea: a research overview and management vision in: Challenges to the Wadden Sea. Presented at the 10th International Scientific Wadden Sea Symposium. (Gronigen: University of Groningen).
van Walraven L., Dapper R., Nauw J. J., Tulp I., Witte J. I. J., van der Veer H. W. (2017). Long-term patterns in fish phenology in the western Dutch Wadden Sea in relation to climate change. J. Sea Res. 127, 173–181. doi: 10.1016/j.seares.2017.04.001
Veenstra H. J. (1969). Gravels of the southern north sea. Mar. Geology 7, 449–464. doi: 10.1016/0025-3227(69)90017-6
Voight J. R. (2015). Xylotrophic bivalves: aspects of their biology and the impacts of humans. J. Molluscan Stud. 81, 175–186. doi: 10.1093/mollus/eyv008
Waltham N. J., Elliott M., Lee S. Y., Lovelock C., Duarte C. M., Buelow C., et al. (2020). UN decade on ecosystem restoration 2021–2030—What chance for success in restoring coastal ecosystems? Front. Mar. Sci. 7. doi: 10.3389/fmars.2020.00071
Whiteway S. L., Biron P. M., Zimmermann A., Venter O., Grant J. W. A. (2010). Do in-stream restoration structures enhance salmonid abundance? A meta-analysis. Can. J. Fish. Aquat. Sci. 67, 831–841. doi: 10.1139/F10-021
Wilding T. (2002). The physical and chemical performance of artificial reef blocks made using quarry by-products. ICES J. Mar. Sci. 59, S250–S257. doi: 10.1006/jmsc.2002.1267
Wilhelmsson D., Yahya S. A. S., Öhman M. C. (2006). Effects of high-relief structures on cold temperate fish assemblages: A field experiment. Mar. Biol. Res. 2, 136–147. doi: 10.1080/17451000600684359
Wohl E., Iskin E. P. (2021). Damming the wood falls. Sci. Adv. 7, eabj0988. doi: 10.1126/sciadv.abj0988
Wood A. C. L., Probert P. K., Rowden A. A., Smith A. M. (2012). Complex habitat generated by marine bryozoans: a review of its distribution, structure, diversity, threats and conservation: Complex habitat generated by marine bryozoans Aquatic Conserv: Mar. Freshw. Ecosyst. 22, 547–563. doi: 10.1002/aqc.2236
Keywords: artificial reef, biodegradable structures, hard substrate, sessile organisms, tree, wood, marine biodiversity
Citation: Dickson J, Franken O, Watson MS, Monnich B, Holthuijsen S, Eriksson BK, Govers LL, van der Heide T and Bouma TJ (2023) Who lives in a pear tree under the sea? A first look at tree reefs as a complex natural biodegradable structure to enhance biodiversity in marine systems. Front. Mar. Sci. 10:1213790. doi: 10.3389/fmars.2023.1213790
Received: 28 April 2023; Accepted: 18 July 2023;
Published: 25 August 2023.
Edited by:
Margot Hessing-Lewis, Hakai Institute, CanadaReviewed by:
Peter J. Auster, Mystic Aquarium, United StatesAndrew Martin Lohrer, National Institute of Water and Atmospheric Research (NIWA), New Zealand
Copyright © 2023 Dickson, Franken, Watson, Monnich, Holthuijsen, Eriksson, Govers, van der Heide and Bouma. This is an open-access article distributed under the terms of the Creative Commons Attribution License (CC BY). The use, distribution or reproduction in other forums is permitted, provided the original author(s) and the copyright owner(s) are credited and that the original publication in this journal is cited, in accordance with accepted academic practice. No use, distribution or reproduction is permitted which does not comply with these terms.
*Correspondence: Jon Dickson, Jon.Dickson@nioz.nl