The outbreak of Drupella snails and its catastrophic effects on coral reefs: a comprehensive review
- 1Hainan Institute of Zhejiang University, Sanya, China
- 2Ocean College, Zhejiang University, Zhoushan, China
- 3College of Marine Life Sciences, Ocean University of China, Qingdao, China
- 4Hainan Yazhou Bay Seed Laboratory, Sanya, China
The recurring outbreaks of Drupella snails are a significant threat to coral reef ecosystems on a global scale. However, research on Drupella snails is currently very fragmentary. This article explores the ecological impacts and mitigation methods of Drupella snails and its outbreaks, and draws the following main conclusions. 1) Drupella snails inhabit the tropical oceans of the Indo-Pacific, utilizing their specialized radula to feed on coral polyps, and they predominantly spawn during summer, attaching egg capsules onto coral skeletons. 2) An outbreak of Drupella snails is defined by a density of > 2 ind/m2, but large aggregations alone do not necessarily indicate an outbreak. 3) The damage of coral reef ecosystem is the primary cause of Drupella snail outbreaks, with the hypotheses of “terrestrial nutrient input” and “overfishing of predator” being widely accepted but not fully explaining all outbreak events. 4) Countering Drupella snail outbreaks involves improving the health of coral reef ecosystems, protected areas, biological control and manual removal, though manual removal is the most direct and effective in short-term. 5) The understanding of the physiological and ecological characteristics of Drupella snails is inadequate at present, with larval development and population ecology being areas of particular under-investigation. 6) Studies of Drupella snail outbreaks have focused mainly on mid-outbreak stages, with little attention to early warnings beforehand or adverse outcomes afterwards. Despite significant challenges, addressing knowledge gaps in the biology of Drupella snails is crucial to determine the true causes of their outbreaks and identify corresponding solutions.
1 Introduction
The coral reef is renowned for being the “tropical rainforest of the ocean” due to its high productivity and immense biodiversity, making it one of the world’s most diverse ecosystems (Apprill et al., 2023; Nama et al., 2023). It is not only an essential habitat for marine organisms, but also a significant source of ecological, economic, and cultural value for human societies (Hein et al., 2015; Macneil et al., 2019; Mellin et al., 2020; Apprill et al., 2023). Coral reefs are one of the most vulnerable ecosystems that have been severely damaged by global climate change and human activities such as overfishing and pollution (Dutra et al., 2021; Hill and Hoogenboom, 2022; Ouedraogo et al., 2023; Rahman et al., 2023). While the effects of environmental stressors like temperature and pH on coral reefs are well-studied (Rice et al., 2019), the detrimental impact of corallivores, whose feeding behaviors can cause minor to severe damage, further exacerbates the decline in coral reef health and significantly alters the coral reef ecosystem (Rogers and Plaganyi, 2022). Corals are subject to predation by various macro-consumers, such as fish (Cole et al., 2008), echinodermata, (Kroon et al., 2021) and gastropod (Dalton and Godwin, 2006). For example, coral mortality and a reduction in coral cover of up to 80% have been caused by outbreaks of the crown-of-thorns starfish (Acanthaster spp., CoTS) since 1980s (Pratchett et al., 2017). The feeding activity of corallivorous gastropods not only has the potential to damage coral and spread coral diseases but can also worsen the spread of existing ciliates on the coral and create additional infections by providing entry points for pathogens through feeding scars (Montano et al., 2022).
The destructive impact of corallivorous Drupella snails has also gradually attracted widespread attention, the high-density presence of these gastropods can decimate vast areas of coral reefs (Boucher, 1986; Cumming, 2009a; Marimuthu and Tripathy, 2018; Thaha and Rathod, 2019). Although climate change threatening coral is widely publicized as a global issue, the havoc wreaked by Drupella snails poses an equal danger to coral reef ecosystem. Massive outbreaks of Drupella snails have resulted in a decline of over 75% in coral reef coverage in the back-reef areas of Ningaloo Reef, Western Australia (Turner, 1994). Currently, there is limited research on Drupella snail outbreaks, leading to an incomplete understanding of the overall situation. This review provides an overview of the existing studies on Drupella snail outbreaks and their impacts on coral reefs. It begins by summarizing the physiology and ecology of Drupella snails. Subsequently, the outbreak events are examined, and the underlying causes are analyzed. Finally, rational strategies for prevention and mitigation are proposed.
2 Physiological and ecological characteristics of Drupella snails
2.1 The taxonomic and morphological characteristics of Drupella snails
Drupella snails belong to the family Muricidae within the phylum Mollusca (Tan, 2000). The main Drupella species include Drupella cornus (Röding, 1798), D. eburnea (Küster, 1862), D. fragum (Blainville, 1832), D. rugosa (Born, 1778) and D. margariticola (Broderip, 1833) (Claremont et al., 2011a) (Figure 1). Studies have documented their average length ranging from 6.4 to 36.3 mm, with average shell and dry body weights of 1335 and 33 mg, respectively (Ismail et al., 2000). The conical shell of Drupella snails typically features small, granular nodules on its surface. The small aperture contains a row of teeth along the inner edge of the outer lip. Additionally, the inner lip axis possesses 3-4 rib-like teeth, while surface nodules are prominent (Johnson and Cumming, 1995; Ruan, 2021). Identifying Drupella snails in the field proved challenging due to heavy encrustation by coralline algae and assorted epifauna (Saponari et al., 2021). Drupella utilizes a distinct radula structure for feeding on coral polyps. Furthermore, some species exhibit sexual dimorphism where males possess more complex radulae and larger rachidian teeth than females (Tan, 2000). D. rugosa in particular has specialized oral structures including an externally keratinized proboscis and lateral teeth, providing morphological adaptations against coral nematocysts and efficient feeding (Turner, 1992; Claremont et al., 2011a).
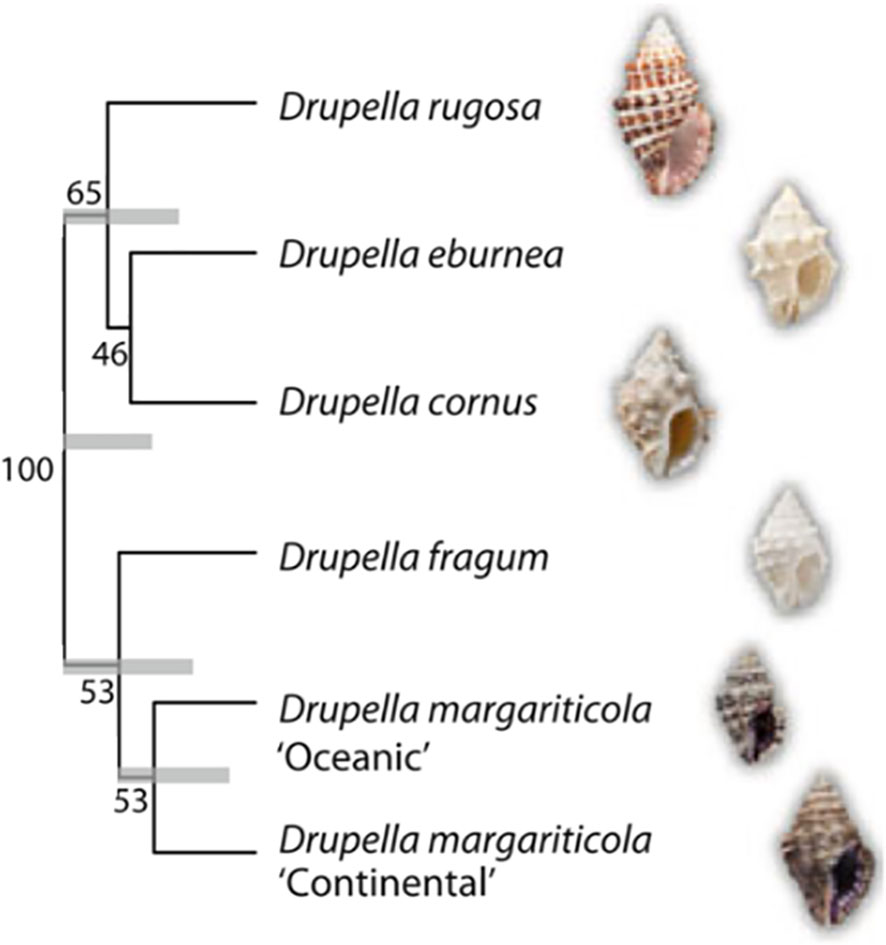
Figure 1 BEAST phylogeny of Drupella snails. Phylogenetic analysis was conducted based on a combined analysis of the 28S rRNA, 12S rRNA, and cytochrome c oxidase subunit I genes. Support values are indicated as BEAST posterior probabilities, while grey bars represent the 95% highest posterior density intervals on nodes with support exceeding 50% (Reproduced from ref. (Claremont et al., 2011a), Copyright © 2011, Springer).
2.2 Habitat and distribution of Drupella snails
Drupella snails mainly inhabit tropical oceans within the Indo-Pacific, with some species harming coral reefs. Species within this genus commonly occurs on Acropora and Montipora corals or hard substrates in lower intertidal and shallow sublittoral zones (Johnson et al., 1993; Boneka et al., 1999). More sheltered reef slopes favoring branching corals also predominated D. rugosa (Cumming, 2009b). The preference for Acropora spp. by Drupella snails may be linked to their selective feeding on damaged coral tissue (Morton, 2002), fast-growing Acropora spp. are prone to damage and breakage, and increased mucus release from these injured corals may attract predators (Johnson et al., 2008). Meanwhile, massive corals like Platygyra acuta utilize robust defenses against biological fouling and predation under normal conditions (Tsang and Ang, 2019). However, branching Acropora spp. prioritize growth over defenses, resulting in low resistance to predation and disease (Hobbs and Frisch, 2010). Studies found Drupella snails aggregating more on larger corals. Coverage on corals ≥30 cm in diameter nearly doubled that of 5-29 cm corals (Bruckner et al., 2017). Predation behavior of Drupella snails was generally not found on the coral colonies with a diameter smaller than 5 cm (Saponari et al., 2021). Notably, Drupella snails exhibited depth preferences, with most predation recorded at 3 m (Dehnert et al., 2022).
2.3 Reproduction of Drupella spp.
Understanding Drupella snail reproduction, recruitment, and early life history is essential to monitor and manage their outbreaks. Sexual maturity occurs around 2.5 years, with an expected lifespan of 7-8 years (Turner, 1994; Ismail et al., 2000; Claremont et al., 2011b). Drupella snails start as veliger larvae, then metamorphose into crawling juveniles (Holborn et al., 1994). Drupella snails lay kidney-shaped egg capsules directly onto dead coral skeletons (D’Asaro, 1986). The embryo development (Sam et al., 2017) and veliger larvae stages (Sam et al., 2016) are critical periods in the lifecycle of Drupella snails. However, insufficient research on veliger metamorphosis in labs leads to high mortality (Turner, 1994). Another study found all veligers died within 30 days of hatching under lab conditions (Haslam et al., 2023). Positive phototaxis likely contributed to veliger deaths prior to metamorphosis due to high energy costs of swimming in undefined feeding ecologies and limited nutrients in labs (Turner, 1994).
Understanding spawning times of different Drupella snail populations enables targeted larval number control and outbreak management by applying measures during peak periods. Table 1 shows spawning occurs year-round, peaking in summer. In the southern hemisphere, peak spawning is December, in the northern hemisphere it is June. Observations at Rottnest Island, Australia over two consecutive summers (2020-2022) found D. cornus laid egg capsules during local summer (January-March) (Haslam et al., 2023). Furthermore, large juvenile aggregations were discovered at Albion, Mauritius in June 2010, supporting reproduction during southern summer (Pillay et al., 2011). The temperature of the water may be the key factor that limits the development of Drupella snails, given that research has demonstrated that their embryos can develop normally inside egg capsules, even during the winter season when the water temperature drops to 18°C (Koido et al., 2017). A January-April water temperature reduction also inhibited the spawning of D. fragum (Kitamura et al., 2022). Species vary in spawning quantities and embryos per capsule. Specifically, D. cornus, D. fragum, D. margariticola, and D. rugosa produce with 596 (Turner, 1992), 106-336 (Sam et al., 2017), 118 ± 18 (Middelfart, 1996), and 67 ± 8 (Sam et al., 2016) embryos per capsule, respectively. D. rugosa produces comparatively larger embryos and veligers despite the lowest quantity, this trade-off may be a mechanism to increase the survival rate of the juveniles (Turner, 1992; Sam et al., 2016). Hatching times also differ: 15 days for D. rugosa, 22-36 days for D. fragum, and 16-23 days for D. cornus (Sam et al., 2017). Seawater temperature plays a role in the developmental time of juveniles, as higher temperatures can increase the metabolic rate of embryos and thus accelerate their development (Przeslawski, 2004).
2.4 Drupella snails’ population dynamics and structure
According to established method, Drupella snails can be divided into recruits (<1cm), juveniles (1-2 cm), and adults (>2 cm) based on size (Turner, 1994). Studies have shown that the population structure of Drupella snails deviates from the standard unimodal distribution, with over 90% of individuals observed in the survey being adults, which attributed to the cryptic lifestyle of juvenile individuals (Saponari et al., 2021). Small bodies and nocturnal activity further obscure juveniles (Furushima et al., 2012; Tsang and Ang, 2019). Habitat preferences may also differ between juveniles and adult snails. Large gatherings of juvenile Drupella snails have been reported forming clusters among Acropora coral debris on a shallow fringing reef in Puerto Galera, Mindoro Island, Philippines, at a depth of 1-2 meters, while adults inhabit reef flats and slopes (Cumming, 2009b). Dwindling coral and constrained living space may exacerbate intraspecific rivalry and confer larger Drupella snails a competitive benefit across generations (De Roos et al., 2003). Species-specific structures also exist. Few juveniles characterize D. rugosa and D. cornus populations, whereas juveniles comprise half of D. fragum populations (Cumming, 1999). D. rugosa is predominantly found on sheltered reef slopes and D. fragum is mostly found on exposed reef crests, and the unique environmental conditions in these habitats such as eddying currents and restricted water flow across coral branches can influence the larvae’s dispersal and settlement choices, thereby resulting in distinct population structures (Cumming, 1999). The aggregation of Drupella snails is influenced by the season, with notably higher densities in summer potentially relating to breeding behavior (Morton and Blackmore, 2009).
3 The outbreaks of Drupella snails
3.1 The threshold of outbreaks
The outbreak criteria for Drupella snails, although challenging to define solely based on density, play a crucial role in understanding their impact on coral reefs. The threshold for the outbreak of Drupella snails is defined as > 2 ind/m2, based on their feeding rate and the growth rate of coral (Cumming, 2009b). Studies have shown that for Drupella snail species with a strong preference for feeding, such as A. spicifera, an outbreak is considered to have occurred when the density of Drupella snails exceeds 0.62 ind/m2. At this density, their feeding rate on corals surpasses the growth rate of corals, causing a negative growth rate in corals (Bessey et al., 2018). Furthermore, Drupella snails exhibit heterogeneity and high levels of clustering in their distribution, with their density potentially exceeding the outbreak threshold only in certain belt transects, while the average density across the entire reef area is significantly lower than the outbreak threshold (Lei et al., 2022). While the outbreak criteria of Drupella snails are difficult to quantify due to the differences in coral coverage and dominant species across various reef areas, the density of Drupella snails can still serve as a useful indicator to some extent.
3.2 Overview of Drupella snail outbreaks
Drupella snail outbreaks have occurred worldwide, impacting coral reefs in different regions. Massive outbreaks in the South Pacific Ocean and Asia-Pacific have been documented, with population densities reaching millions of individuals (Table 2). Four major outbreaks of Drupella snails have occurred in South Pacific Ocean, in 1983, 1989, 1993, and 2014-2016, respectively. The coral reef of Ningaloo Marine Park, spanning 2,240 km2, once saw a population of up to 500 million D. cornus individuals (Ayling and Ayling, 1987). The multi-year intervals between outbreaks may be attributed to the recovery rate of coral reefs. Furthermore, the outbreak patterns of Drupella snail and CoTS show a degree of coincidence, suggesting different corallivorous species may interact to exacerbate coral degradation (Pratchett, 2010). Drupella snail outbreaks have occurred in several areas within the Asia-Pacific region. In November 2006, a total of 300 colonies of P. acuta and P. carnosus, covering an area of 700 m2, were discovered to have suffered severe bioerosion at their bases by Drupella snails at Coral Beach in Hoi Ha Wan Marine Park, Hong Kong (Lam et al., 2007). After a severe coral bleaching event in Koh Tao Island in the western Gulf of Thailand in 2010, a large-scale outbreak of Drupella snails was detected in the same area in 2011 through local monitoring (Hoeksema et al., 2013). Under certain extreme conditions, the outbreak density of D. cornus can reach as high as 3000 ind/m2 (Moyer et al., 1982). While mass gatherings of Drupella snails have also been documented in Hainan Island, China, with a maximum of 38 individuals found on a single coral species (A. hyacinthus), the density of Drupella snails (0.09 ± 0.03 to 1.78 ± 0.39 ind/m2) did not reach the so-called outbreak threshold (Lei et al., 2022). Outbreaks of Drupella snails have also impacted various regions within the Indian Ocean. The extensive coral bleaching that occurred in South Male’, Maldives in April/May 2016 resulted in the loss of up to 80% of live coral, facilitating a massive subsequent outbreak of Drupella snails with densities of up to 250 individuals recorded per surviving coral colony (Bruckner et al., 2017). A large-scale outbreak of Drupella snails also occurred in the reef areas of Mauritius between 2010 and 2016, with higher average outbreak densities during the summer months compared to winter. In 2016, the outbreak density was as high as 158.14 ± 13.85 ind/m2, which may be related to the continuous decline in local coral reef health and climate change (Kaullysing et al., 2016; Kaullysing et al., 2017). Density disparities of Drupella snails likewise exist between sites within reef systems.
Existing statistical results indicate that the major species involved in outbreaks are D. cornus and D. rugosa. The consumption rates of corals by different types of Drupella snails vary. Reports indicated that each individual of D. cornus can consume 2.6 cm2 of live branching coral per day, with the consumption rate increasing with temperature, although body size associated feeding rates did not exhibit a significant difference (Turner, 1992; Al-Horani et al., 2011). Consumption rates of coral tissue also differed between D. rugosa (1.81 cm2 per day) and D. margariticola (0.51 cm2 per day) (Samsuri et al., 2018). Similar results have also been detected in other studies on coral-feeding snails. Marine gastropod consumption of Acropora tissue averages 1.9 cm2 per individual per day, but peaks at 6.5 cm2 for some Caribbean species (Bruckner et al., 1997). In Florida, the estimated long-term feeding rate for marine snails (average length 29 mm) was 1.07 cm2 of tissue per individual per day (Baums et al., 2003). In addition, it is worth noting that coral communities contain various coral morphologies and species, and the consumption rates of Drupella snails may vary with the preferred prey species selection.
3.3 Outbreak mode of Drupella snails
The limited research on the outbreak mode of Drupella snails underscores the importance of understanding their outbreak patterns for effective management, as the factors triggering the initial onset of outbreaks can be subtle and challenging to detect. Given their comparable feeding habits, living environments, planktonic larval stage and metamorphosis, insights from the better-studied outbreak mechanism of CoTS can provide valuable information on potential Drupella snail outbreaks. The CoTS outbreak is categorized into primary and secondary outbreaks. Primary outbreaks are characterized by a multimodal distribution of individual sizes and a large variation in size. On the other hand, secondary outbreaks exhibit a unimodal distribution of population size, with individuals of similar size comprising the outbreak population (Birkeland et al., 1990; Pratchett, 2005; Pratchett et al., 2014). It is likely that the outbreak of Drupella snails falls under the secondary outbreak category. Studies indicate that Drupella snail populations primarily consist of mature individuals of comparable sizes, possibly originating from either a single large-scale recruitment event or the gradual accumulation of snails from multiple cohorts (Saponari et al., 2021). Another plausible explanation was that smaller individuals grow rapidly in the early stages of the outbreak, and most current research focused on the middle stages of the outbreak when juvenile individuals were already similar in size to adults. For example, Drupella snails that were 15 mm long grew by 5.2 mm over six months at a site in the early stages of an outbreak, whereas at a site with an established outbreak, they only grew by 3.8 mm (Black and Johnson, 1994). Snails that were larger than 35 mm showed very little growth.
3.4 Drivers of Drupella snail outbreaks
Marine ecosystems are complex and dynamic, and the outbreak of Drupella snails is a multifaceted issue that has been studied extensively. The causes of Drupella snail outbreaks are not yet fully understood, but various factors have been suggested as possible contributors, including terrestrial runoff, overfishing of Drupella predators, and increased reef damages (Turner, 1994). The following hypotheses have gained the most widespread acceptance.
3.4.1 Terrestrial nutrient input hypothesis
Coastal runoff containing nutrients can drive Drupella snail outbreaks by stressing corals through lowering salinity and increasing nutrients, thus developing conditions favorable for snail proliferation. Drupella snails were notably more drawn to corals that were experiencing stress and exposed to low salinity water, the formation of feeding aggregations can explain the outbreaks of Drupella snails previously reported in Hong Kong (Morton, 2002). The phenomenon of increased phytoplankton levels due to warmer temperatures and nutrient pollution potentially enhancing the survival and growth of Drupella larvae and juveniles, and contributing to outbreaks, has also been proposed as a contributing factor in outbreaks of CoTS (Kamya et al., 2018). An ample supply of phytoplankton ensured that veligers of Drupella snails had enough energy to complete metamorphosis and transform into crawling juveniles (Turner, 1994). Furthermore, high nutrient input exacerbates the overgrowth of macroalgae (Leckraz, 2015). The macroalgae’s (Padina boryana) attachment to and overgrowth of A. muricata releases organic carbon that promotes microbe proliferation, weakening the coral’s resistance and reducing the photosynthesis efficiency of its zooxanthellae by 56% (Kaullysing et al., 2016). Other researcher also identified the synergistic impact of corallivorous Hermodice carunculata and macroalgae Halimeda opuntia on reef-building coral Orbicella faveolata in the Caribbean, potentially inducing coral predation and tissue decay (Wolf and Nugues, 2013). As the health condition of corals deteriorates, their resistance and immunity decrease, making Drupella snails more easily capture and prey on coral tissues and leading to their rapid reproduction and outbreak on a large scale (Morton, 2002).
3.4.2 Overfishing of predator hypothesis
Overfishing that reduced balistids, labrids and invertebrate predators may have contributed to higher Drupella snail populations regionally by lifting controls on their numbers. Predators of Drupella snails, such as the fish Coris aygula, were seldom observed in investigated region, which may account for the behavior of Drupella snails foraging on the upper surfaces of branching corals and exposed massive or encrusting corals during the daytime, without being constrained by “top-down” control (Shafir et al., 2008). Napoleon wrasses (Cheilinus undulatus), considered one of the natural predators of Drupella snails, can suck the snails out of coral and crush their hard shells using pharyngeal bones (Ratianingsih et al., 2017). However, another study has shown a significant decline in Napoleon wrasses populations in coral reefs (Sadovy et al., 2003). The decline of herbivorous fishes further exacerbates the overgrowth of macroalgae, resulting in the deterioration of coral reef ecosystems (Leckraz, 2015). Corals can also be protected from attack to some extent by other small predators, such as Alpheus lottini and Cymo andreossyi, as their larger claws can crush the shells of Drupella snails (Williams et al., 2001; Gokul and Venkataraman, 2010).
3.4.3 Climate change hypothesis
Climate change impacts like thermal-stressed bleaching and extreme weather events may contribute to Drupella snail outbreaks through weakened coral resilience. Reports of high densities of Drupella snails have risen in recent years, notably after thermal stress triggered mass bleaching events (Bruckner et al., 2017). The cyclical occurrence of Drupella outbreaks indicates a possible correlation with oceanographic oscillations (e.g., El Niño) (Turner, 1994). In a similar vein, Drupella snails had a more significant impact on corals that were damaged by cyclone Ivor in 1990 on the Great Barrier Reef (Ayling and Ayling, 1992). Periodic climate change may also be responsible for the cyclical occurrences of Drupella outbreaks. Other studies have suggested that as early as the 1940s, there were large populations of other corallivorous animals present, which implies that the cyclical outbreaks may have existed even in the absence of significant human impacts on the marine environment (Pratchett et al., 2014).
In addition, other factors have also exacerbated the outbreak of Drupella snails. The high amount of visitation to dive sites has exacerbated the damage to corals, and the mucus secreted by broken coral fragments has attracted Drupella snails to feed, leading to significant aggregations of Drupella snails and contributing to outbreaks to some extent (Armstrong, 2009). Studies have shown that areas with higher levels of human disturbance tend to have greater densities of Drupella snails, while less impacted areas generally show lower abundances (Boneka and Mamangkey, 2013). While the outbreak of Drupella snails can be attributed to both human-induced environmental degradation and cyclical climate change, the precise mechanisms underlying these outbreaks remain unclear. Therefore, further research is necessary to obtain a better understanding of this phenomenon and its impact on marine ecosystems.
4 Coral destruction induced by Drupella snail outbreaks
4.1 Direct destruction for corals
Widespread coral predation by Drupella snails can severely degrade reefs through slowing coral growth and directly reduce coral coverage (Figure 2). The outbreak of Drupella snails has brought devastation to coral reefs, destroying 35 m2 of coral reef on Great Barrier Reef in just two months, resulting in extensive coral rubble areas and a shift from coral-dominated to macroalgae-dominated distribution (Cumming, 2009b; Kayal et al., 2012). Moreover, Drupella snails will move on to nearby coral reefs to cause destruction after completely devastating a coral reef (Shafir et al., 2008). CoTS (A. planci) is widely acknowledged as a coral predator. The outbreak of CoTS in Moorea Island from 2003 to 2010 led to a drastic decline in live coral cover in an area of 132 km2, from over 40% to less than 1%, causing approximately 96% of coral mortality in the region (Kayal et al., 2012). The destructive capacity of Drupella snails on coral reefs is no less than that of CoTS, 0.9 Drupella snails per m2 was equivalent to 10 CoTS per hectare, calculated using regression relationships between coral-grazing surface area of reef and surface area of coral branches (Cumming, 2009b). Between 1987 and 1989, the live coral cover along a 280 km stretch of Ningaloo Reef in Western Australia decreased by up to 75% due to predation by Drupella snails (Baird, 1999). Following the Drupella snails attack, the survival rate of A. formosa decreased to 66%, while that of P. damicornis dropped to 79% (Pillay et al., 2011). Different species of Drupella snails show varying feeding rates on corals, with D. rugosa consuming coral tissue at a significantly higher rate (1.81 ± 0.95 cm2/day) than D. margariticola (0.51 ± 0.75 cm2/day) (Samsuri et al., 2018).
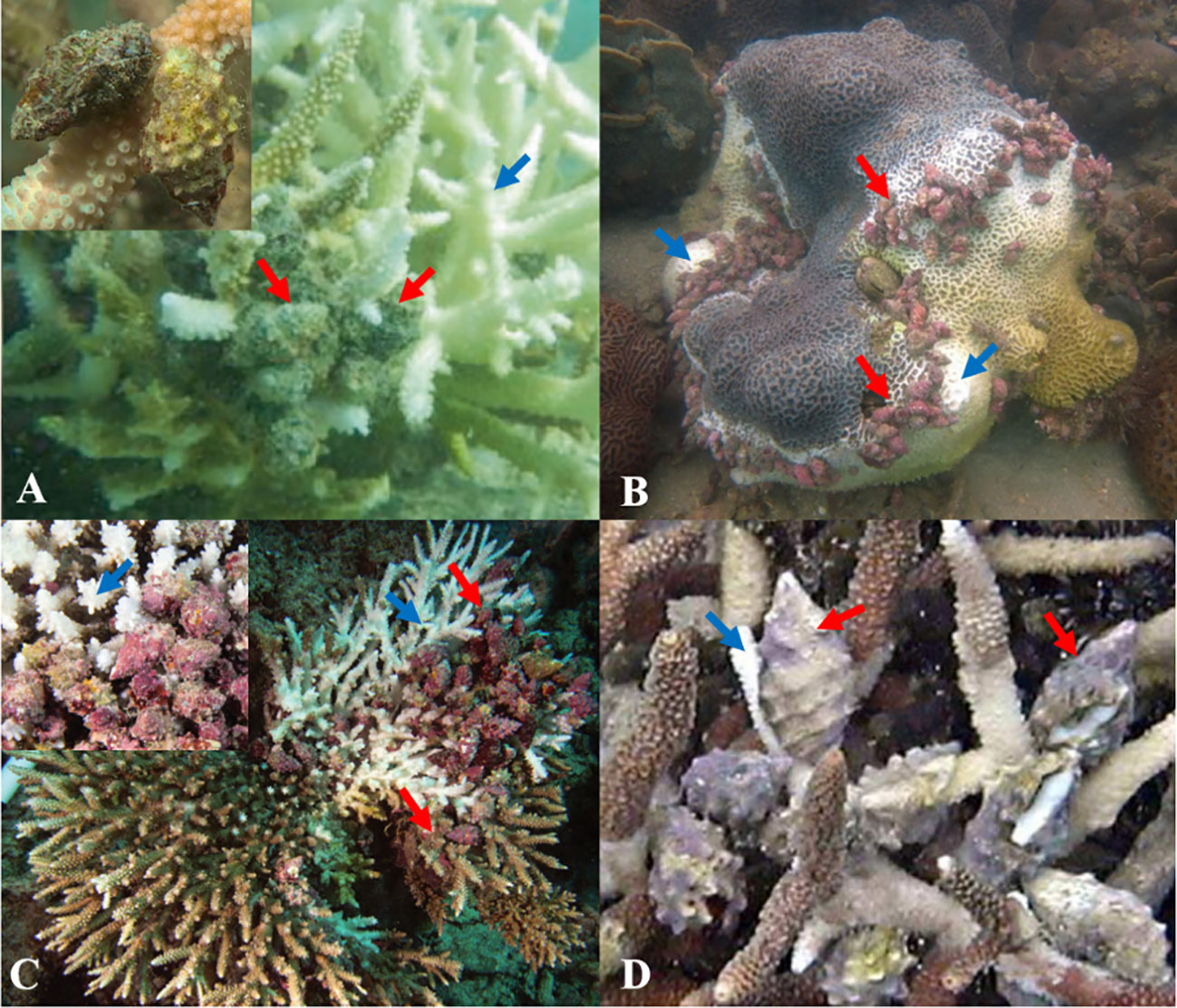
Figure 2 The devastation of corals amid Drupella outbreaks. (A) indicate D. rugosa predation on A. formosa in the Van Phong Bay, Vietnam (Reproduced from ref. (Bettarel et al., 2018), Copyright © 2018, Springer). (B) indicate aggregation of D. rugosa on an P. acuta colony in Hong Kong (Reproduced from ref. (Tsang and Ang, 2019), Copyright © 2019, Elsevier). (C) indicate Drupella spp. consuming half colony of Acropora in Maldives (Reproduced from ref. (Bruckner et al., 2017), Copyright © 2017, Springer). (D) indicate the aggregation of D. cornus (Reproduced from ref. (Kita et al., 2005), Copyright © 2005, Elsevier). The red arrows point to the snails, and the blue arrows point to the coral ailment.
By using their specialized radula to rasp live tissue from the coral skeletons, these snails create grazing scars that have the potential to significantly impact the growth rate and overall health of the corals. On the one hand, Drupella snails inflicted injuries cause coral colonies to prioritize tissue regeneration over colony growth, and prolonged or recurrent injuries may ultimately result in colony mortality (Pillay et al., 2011). On the other hand, the disruption of the bacteriome and virome by Drupella snails ultimately leads to a reduction in the coral’s overall fitness (Bettarel et al., 2018; Nguyen et al., 2023). Drupella snails can create feeding scars and disrupt the coral’s superficial mucus layer, which can provide an environment conducive to the growth and spread of pathogens that cause diseases (skeletal eroding, band disease) (Lamb et al., 2014). Therefore, the occurrence of Drupella snails has been linked to a number of coral diseases (Widiastuti, 2019), such as white syndrome (Antonius and Riegl, 1998), skeletal eroding band disease and black band disease (Onton et al., 2011), and brown band disease (Nicolet et al., 2013; Nicolet et al., 2018). The gastropod Drupella snails, which feeds on coral, proved to be a highly efficient carrier of brown band disease, as it infected over 40% of the experimental colonies (Nicolet et al., 2013). Studies have also demonstrated that Drupella snail predation can alter the composition of the coral’s symbiotic microbial community, leading to an increase in the abundance of potentially harmful bacteria (Bettarel et al., 2018). Specifically, the community composition of active epibiotic bacteria has shifted towards more pathogenic taxa, including those belonging to the Vibrionales, Clostridiales, Campylobacterales and Alteromonadales orders (Bettarel et al., 2018). Likewise, the feeding behavior of Drupella snails also has an impact on the reproduction of corals. The removal of coral recruits by Drupella snails (10%) underscored the importance of coral recruitment in maintaining the population structure of adult corals and promoting the recovery of coral reefs following disturbances (Tsang et al., 2018).
Coral reefs affected by coral diseases or large-scale bleaching are particularly vulnerable to further degradation, and the feeding of corallivorous animals hinders the recovery of coral reefs (Bruckner et al., 2017). D. cornus exhibit a greater attraction to conspecifics than to undamaged coral, and even show a preference for corals damaged by conspecifics over those damaged artificially (Hamman, 2018). Under the threat of climate change, outbreaks of Drupella snails may devastate the resilience of coral reefs. The ongoing predation by Drupella snails may lead to the extinction of once-dominant coral species, including genets that have tolerance to higher sea water temperatures (Bruckner et al., 2017). The presence of Drupella snails is likely to diminish the resilience of these reefs, as many of the corals that are targeted by Drupella snails were previously able to withstand bleaching during periods of elevated water temperatures.
4.2 Indirect destruction
Drupella snails can strongly impact reefs through selective yet adaptive feeding that facilitates coral composition shifting. Drupella snails’ feeding behavior is selective, with a preference for branching corals over massive corals during the initial outbreak (Saponari et al., 2021). Their selective feeding can cause changes in the composition and diversity of coral communities (Lachs et al., 2019). Drupella snails exhibit strong adaptability and modify their feeding preferences based on the availability of food (Hoeksema et al., 2013; Moerland et al., 2016). After their preferred species are depleted, they continue to feed on other species, ultimately leading to the death of most corals. However, the decrease in coral coverage has minimal impact on the distribution of Drupella snails (Saponari et al., 2021). After a major coral bleaching event in Thailand, there have been reports of Drupella snail shifting their dietary preferences from their favored genera Acropora spp. and Pocillopora spp. to less palatable fungiid corals (Hoeksema et al., 2013; Moerland et al., 2016). After the massive death of corals, the coverage of macroalgae rapidly increases due to the enlarged living space, and occupies the ecological niche of corals, hindering coral recovery (Lesser, 2021). Therefore, the outbreak of Drupella snails indirectly alters the community structure of coral reef ecosystems, reduces their recovery capacity, and indirectly leads to the reduction or even disappearance of certain coral reef fish species.
5 Strategies to address Drupella snail outbreaks
Recurrent outbreaks of Drupella snails significantly contribute to the degradation of coral reef ecosystems. However, limited documentation exists regarding methods to control Drupella snails. By implementing effective management strategies, it is possible to mitigate the risk of Drupella outbreaks and protect coral reef ecosystems. Here are strategies that can be employed.
5.1 Protection of coral reef ecosystems
Reducing anthropogenic impacts and promoting the health of coral reef ecosystems are key to mitigating future Drupella snail outbreaks and maintaining their overall well-being (Armstrong, 2009; Boneka and Mamangkey, 2013). By improving water quality in the Great Barrier Reef, such as through the reduction of agricultural runoff, it is possible to reduce macroalgal cover by an average of 39% and increase the richness of hard corals and phototrophic octocorals (De’ath and Fabricius, 2010). Increasing the richness of coral can enhance the resilience and stress resistance of coral reef ecosystems. For example, the presence of P. cylindrica has reduced the predation of Drupella snails on P. frondifera to some extent (Lachs et al., 2019). Enhancing the protection of coral reef fish and increasing their abundance and diversity, especially herbivorous fish, can control the growth of macroalgae, improve the health and resilience of coral reef ecosystems, and promote their resistance to Drupella snail outbreaks (Balasubramanian et al., 2014; Sheppard et al., 2023). Additionally, no-take marine protected areas can contribute to the well-being of coastal societies and the sustainability of coral reef ecosystems by increasing the abundance of reef organisms and protecting key ecosystem functions (Graham et al., 2011). The development of habitat connectivity restoration technology has the potential to enhance the connectivity of indigenous marine biological communities, promote nutrient cycling and material flow across multiple ecosystems, and improve the health and organization of coral reef ecosystems while also boosting biological abundance and diversity (Wang et al., 2022).
5.2 Biological control
Biological control methods can be utilized to manage Drupella snail outbreaks. Certain organisms can act as predators or competitors of Drupella snails, helping to control their population. The giant triton snail (Charonia tritonis) is considered a predator of various corallivorous harmful organisms (Bose et al., 2017; Ratianingsih et al., 2017). The Australian government has invested $568,000 in studying the reproductive biology of giant triton snails and successfully bred 100,000 juveniles, which will be used for the biological control of corallivorous harmful organisms (Chadwick, 2017). The coral guard crabs (Trapezia spp.) are commonly found among the branches of live Acropora spp. and Pocillopora spp. colonies, and they exhibit high levels of territorialism and aggression towards corallivores. They effectively discourage the invasion of A. planci by clipping and pinching the soft tissues of intruders (Glynn, 2013). The feeding rate of D. rugosa was reduced by 22.9% in the presence of the coral guard crab (Trapezia cymodoce) (Samsuri et al., 2018). It is important to exercise caution during biological control and conduct a comprehensive assessment of the selected species to prevent ecological disasters in coral reef ecosystems.
5.3 Manual removal
Manual removal is a recommended method for controlling Drupella snail outbreaks under current conditions. Manual removal, which involves clearing both juveniles and adults, the former involves inspecting exposed coral skeletons on the reef for early detection of Drupella snails’ egg capsules and removing them to decrease the likelihood of outbreaks. Moreover, manual removal has been implemented successfully in various regions. According to other researchers, it is advisable to carry out extermination activities in southwestern Shikoku (Japan) between February and March, prior to the spawning season (Kitamura et al., 2022). By implementing the extermination program of Drupella snails, Japan effectively controlled the number of Drupella snails, causing the density of Acropora spp. to remain high even in the cold winter, despite Acropora spp. being more sensitive to low temperatures and more prone to predation under cold stress conditions. This precisely illustrated the importance of manually removing Drupella snails (Kimura et al., 2005). The local government is employing methods like manual removal of Drupella snails and implementing restrictions on human activities to assist in the restoration of coral reefs (Lam et al., 2007). The manual removal of 14,000 individuals by volunteer divers effectively mitigated the outbreak of Drupella snails in Thailand, resulting in a significant decrease in Drupella snails density from 7.9 ind/m2 in October 2010 to 5.6 ind/m2 in April 2021 (Scott et al., 2017a). Removing a distinct species of corallivorous snail, C. abbreviata, from coral reefs resulted in a significant increase in the area of live coral tissue in comparison to areas where the snail clusters were left untouched (Miller, 2001). Manual removal is the most immediately effective method in the short term. However, it also has certain restrictions. For example, it can only temporarily alleviate the damage caused by outbreaks and cannot solve the issue fundamentally. Manual removal is expensive, and its operational depth is limited, making it impossible to remove snails in deeper waters. Additionally, its effectiveness in controlling widespread outbreaks of Drupella snails is not significant.
5.4 Alternative methods and perspectives
In addition to the aforementioned strategies, alternative methods can be utilized for the control of Drupella snails. For example, a Japanese research team has developed specific antibodies for the planktonic larvae of Drupella snails, which can be used for low-cost and rapid on-site detection. This can help better monitor the population dynamics of Drupella snails and predict their outbreaks (Kitamura et al., 2021).
To address the outbreak of Drupella snails in the long term, further research and comprehensive studies are necessary. Exploring the physiological and reproductive characteristics of different species of Drupella snails can provide a basis for early outbreak management. Initially, researchers believed that only a single species was responsible for the outbreaks. However, as knowledge advanced, it was discovered that sometimes multiple species of Drupella snails were involved in the outbreak, and there were significant physiological differences among different species of Drupella snails. For example, D. margariticola does not have specialized mouth structures and can only feed on coral that has already been attacked by other Drupella species (Claremont et al., 2011a). Therefore, understanding the physiological and reproductive characteristics of different species of Drupella snails, especially the duration of their planktonic larvae, metamorphosis, and feeding habits, can provide a basis for early outbreak management. Additionally, establishing population dynamic models, identifying environmental triggers, and studying the interactions between Drupella snails and their predators will contribute to developing more effective control strategies.
Furthermore, based on the aggregation characteristics of Drupella snails, it is possible to identify the main components of specific pheromones (such as mucus released during conspecific feeding, coral fragment secretions, etc.) associated with Drupella snails’ aggregation. By analyzing the main components, targeted trapping devices can be designed (Kita et al., 2005). Additionally, Drupella snails possess the characteristic of evading areas with natural predators. By analyzing the main components of pheromones released by their natural predators, it is feasible to create dispersal devices that may serve as potential control strategies for outbreaks.
Notably, it is crucial to raise awareness among local communities, policymakers, and stakeholders about the ecological importance of coral reef ecosystems and the impacts of Drupella snail outbreaks. Education and outreach programs can promote sustainable practices and encourage community involvement in the conservation and restoration of coral reefs.
6 Conclusion
Addressing the recurrent outbreaks of Drupella snails requires a multifaceted approach (Figure 3). Protecting coral reef ecosystems, implementing biological control methods, utilizing manual removal, and exploring alternative techniques are all important strategies. Additionally, conducting further research, raising awareness, and promoting community involvement will contribute to the long-term management and conservation of coral reef ecosystems affected by Drupella snail outbreaks.
Author contributions
FZ: Conceptualization, Data curation, Validation, Writing – original draft. XJ: Validation, Writing – review & editing. ZL: Validation, Writing – review & editing. YJ: Validation, Writing – review & editing. MQ: Conceptualization, Funding acquisition, Supervision, Validation, Visualization, Writing – review & editing.
Funding
The author(s) declare financial support was received for the research, authorship, and/or publication of this article. This work was supported by the research funding from Sanya Yazhou Bay Science and Technology City (SCKJ-JYRC-2022-50) and Hainan Yazhou Bay Seed Laboratory (B21Y10404, B22C10401) and Guangzhou Basic and Applied Basic Research Foundation (202201010207).
Conflict of interest
The authors declare that the research was conducted in the absence of any commercial or financial relationships that could be construed as a potential conflict of interest.
Publisher’s note
All claims expressed in this article are solely those of the authors and do not necessarily represent those of their affiliated organizations, or those of the publisher, the editors and the reviewers. Any product that may be evaluated in this article, or claim that may be made by its manufacturer, is not guaranteed or endorsed by the publisher.
References
Al-Horani F. A., Hamdi M., Al-Rousan S. A. (2011). Prey selection and feeding rates of Drupella cornus (Gastropoda: Muricidae) on corals from the Jordanian Coast of the Gulf of Aqaba, Red Sea. Jordan J. Biol. Sci. 4 (4), 191–198.
Al-Moghrabi S. M. (1997). Bathymetric distribution of Drupella cornus and Coralliophila neritoidea in the Gulf of Aqaba (Jordan). Coral Reefs, 1345–1350.
Antonius A., Riegl B. (1998). Coral diseases and Drupella cornus invasion in the Red Sea. Coral Reefs 17, 48–48. doi: 10.1007/s003380050093
Apprill A., Girdhar Y., Mooney T. A., Hansel C. M., Long M. H., Liu Y. Q., et al. (2023). Toward a new era of coral reef monitoring. Environ. Sci. Technol. 57 (13), 5117–5124. doi: 10.1021/acs.est.2c053695117
Armstrong S. (2009). The status of the coral predator Drupella cornus at Ningaloo Marine Park. Ed. Conservation D. (Western Australia: Science Division).
Ayling A. M., Ayling A. L. (1987). Ningaloo Marine Park: preliminary fish density assessment and habitat survey, with information on coral damage due to Drupella cornus grazing (Western Australia: Department of Conservation and Land Management).
Ayling A. M., Ayling A. L. (1992). Preliminary information on the effects of Drupella spp. grazing on the Great Barrier Reef. Ed. Management C. (Como, Western Australia: Proc Workshop Department of Conservation and Land Management), 37–42.
Baird A. (1999). A large aggregation of Drupella rugosa following the mass bleaching of corals on the Great Barrier Reef. Reef Res. 9 (2), 6–7.
Balasubramanian K., Jeevamani J., Nagendran A., Pandiaraja D., Chandrasekaran S. (2014). Impact of removal of invasive species Kappaphycus alvarezii from coral reef ecosystem in Gulf of Mannar, India. Curr. Sci. 106, 1401–1408.
Baomar J., Al-Sofyani A., Wilson J. (2016). “Spawning behavior of corallivorous gastropod Drupella cornus in Obhur Creek, Jeddah,” in International Conference on the Marine Environment of the Red Sea (Jeddah, Saudi Arabia: King Abdullah University of Science and Technology).
Baums I., Miller M., Szmant A. (2003). Ecology of a corallivorous gastropod, Coralliophila abbreviata, on two scleractinian hosts. II. Feeding, respiration and growth. Mar. Biol. 142, 1093–1101. doi: 10.1007/s00227-003-1053-4
Bessey C., Babcock R. C., Thomson D. P., Haywood M. D. E. (2018). Outbreak densities of the coral predator Drupella in relation to in situ Acropora growth rates on Ningaloo Reef, Western Australia. Coral Reefs 37 (4), 985–993. doi: 10.1007/s00338-018-01748-7
Bettarel Y., Halary S., Auguet J., Mai C. T., Bui V. N., Bouvier T., et al. (2018). Corallivory and the microbial debacle in two branching scleractinians. ISME J. 12, 1109–1126. doi: 10.1038/s41396-017-0033-5
Birkeland C., Lucas J. S., Birkeland C., Lucas J. S. (1990). Acanthaster planci: major management problems of coral reefs. Q. Rev. Biol. 67 (3).
Black R., Johnson M. S. (1994). Growth rates in outbreak populations of the corallivorous gastropod Drupella cornus (Röding 1798) at Ningaloo Reef, Western Australia. Coral Reefs 13 (3), 145–150. doi: 10.1007/bf00301190
Boneka F., Mamangkey N. (2013). Abundance of coral-polyp-eating gastropods Drupella cornus in Bunaken National Park, Indonesia: indicating anthropogenic impact? Aquat. Sci. Manage. 1, 17–20. doi: 10.35800/jasm.1.1.2013.1961
Boneka F. B., Lalamentik L. T. X., Cussoy D. (1999). Feeding niche of Drupella (Muricidae: Prosobranchia) in the coral reefs of Bunaken Island, Indonesia. Phuket Mar. Biol. Center Special Publ. 19 (1), 55–59.
Bose U., Wang T., Zhao M., Motti C., Hall M., Cummins S. (2017). Multiomics analysis of the giant triton snail salivary gland, a crown-of-thorns starfish predator. Sci. Rep. 7, 1–14. doi: 10.1038/s41598-017-05974-x
Boucher L. (1986). Coral predation by muricid gastropods of the genus Drupella at Enewetak, Marshall Islands. Bull. Mar. Sci. 38 (1), 9–11.
Bruckner R. J., Bruckner A. W., Williams E. (1997). Life history strategies of Coralliophila abbreviata Lamarck (Gastropoda: Coralliophilidae) on the southwest coast of Puerto Rico. Proc. 8th Int. Coral Reef Sym. 1, 627–632.
Bruckner A., Coward G., Bimson K., Rattanawongwan T. (2017). Predation by feeding aggregations of Drupella spp. inhibits the recovery of reefs damaged by a mass bleaching event. Coral Reefs 36 (4), 1181–1187. doi: 10.1007/s00338-017-1609-2
Chadwick E. (2017). Giant sea snails join the fight to save the reef. Ed. Science A.. Available at: https://www.aims.gov.au/information-centre/news-and-stories/giant-sea-snails-join-fight-save-reef.
Claremont M., Reid D. G., Williams S. T. (2011a). Evolution of corallivory in the gastropod genus Drupella. Coral Reefs 30 (4), 977–990. doi: 10.1007/s00338-011-0788-5
Claremont M., Williams S., Barraclough T., Reid D. (2011b). The geographic scale of speciation in a marine snail with high dispersal potential. J. Biogeogr. 38, 1016–1032. doi: 10.1111/j.1365-2699.2011.02482.x
Cole A., Pratchett M., Jones G. (2008). Diversity and functional importance of coral-feeding fishes on tropical coral reefs. Fish Fisheries 9, 286–307. doi: 10.1111/j.1467-2979.2008.00290.x
Cumming R. (1999). Predation on reef-building corals: Multiscale variation in the density of three corallivorous gastropods, Drupella spp. Coral Reefs 18, 147–157. doi: 10.1007/s003380050170
Cumming R. L. (2009a). Case study: Impact of Drupella spp. on reef-building corals of the Great Barrier Reef (Townsville, Qld: Great Barrier Reef Marine Park Authority).
Cumming R. L. (2009b). Population outbreaks and large aggregations of Drupella on the Great Barrier Reef (Townsville, Qld; Great Barrier Reef Marine Park Authority, Australian Government).
Dalton S., Godwin S. (2006). Progressive coral tissue mortality following predation by a corallivorous nudibranch (Phestilla sp.). Coral Reefs 25, 529–529. doi: 10.1007/s00338-006-0139-0
D’Asaro C. (1986). Egg capsules of eleven marine prosobranchs from northwest Florida. Bull. Mar. Sci. 39, 76–91.
De’ath G., Fabricius K. (2010). Water quality as a regional driver of coral biodiversity and macroalgae on the Great Barrier Reef. Ecol. Appl. 20 (3), 840–850. doi: 10.1890/08-2023.1
Dehnert I., Galli P., Montano S. (2022). Ecological impacts of coral gardening outplanting in the Maldives. Restor. Ecol. 31, e13783. doi: 10.1111/rec.13783
De Roos A. M., Persson L., McCauley E. (2003). The influence of size-dependent life-history traits on the structure and dynamics of populations and communities. Ecol. Lett. 6 (5), 473–487. doi: 10.1046/j.1461-0248.2003.00458.x
Dutra L. X. C., Haywood M. D. E., Singh S., Ferreira M., Johnson J. E., Veitayaki J., et al. (2021). Synergies between local and climate-driven impacts on coral reefs in the Tropical Pacific: A review of issues and adaptation opportunities. Mar. pollut. Bull. 164, 111922. doi: 10.1016/j.marpolbul.2020.111922
Forde M. J. (1992). Populations, behaviour and effects of Drupella cornus on the Ningaloo Reef, Western Australia (Western Australia: Conservation and Land Management), 92, 45–50.
Furushima Y., Maruyama T., Suzuki S. (2012). Coral fluorescence monitoring system camera photographed feeding damage by a snail (Drupella spp.). Midoriishi 23, 25–30.
Glynn P. (2013). Fine-scale interspecific interactions of coral reef: Functional roles of small and cryptic metazoans. Smithsonian Contributions to Mar. Sci. 39, 229–248.
Gokul A., Venkataraman K. (2010). Taxonomy and systematics of coral associated Brachyuran crabs in Gulf of Mannar Marine Biosphere Reserve. Records Zool. Survey India 110 (3), 61–76. doi: 10.26515/rzsi/v110/i3/2010/158934
Graham N., Ainsworth T., Baird A., Ban N., Bay L., Cinner J., et al. (2011). From microbes to people: Tractable benefits of no-take areas for coral reefs. Oceanogr. Mar. Biol.: Annu. Rev. 49, 105–136. doi: 10.1201/b11009-4
Hamman E. (2018). Aggregation patterns of two corallivorous snails and consequences for coral dynamics. Coral Reefs 37, 851–860. doi: 10.1007/s00338-018-1712-z
Haslam V., Bessey C., Chaplin J., van Keulen M. (2023). Evidence of corallivorous Drupella cornus breeding on the higher latitude reefs of Rottnest Island (32° S), Western Australia. Mar. Biol. 171 (1), 1–28. doi: 10.21203/rs.3.rs-2905663/v1
Hein M. Y., Lamb J. B., Scott C., Willis B. L. (2015). Assessing baseline levels of coral health in a newly established marine protected area in a global scuba diving hotspot. Mar. Environ. Res. 103, 56–65. doi: 10.1016/j.marenvres.2014.11.008
Hill T. S., Hoogenboom M. O. (2022). The indirect effects of ocean acidification on corals and coral communities. Coral Reefs 41 (6), 1557–1583. doi: 10.1007/s00338-022-02286-z
Hobbs J. P., Frisch A. (2010). Coral disease in the Indian Ocean: taxonomic susceptibility, spatial distribution and the role of host density on the prevalence of white syndrome. Dis. Aquat. Organisms 89, 1–8. doi: 10.3354/dao02181
Hoeksema B., Scott C., True J. (2013). Dietary shift in corallivorous Drupella snails following a major bleaching event at Koh Tao, Gulf of Thailand. Coral Reefs 32, 423–428. doi: 10.1007/s00338-012-1005-x
Holborn K., Johnson M., Black R. (1994). Population genetics of the corallivorous gastropod Drupella cornus at Ningaloo Reef, Western Australia. Coral Reefs 13, 33–39. doi: 10.1007/BF00426432
Hsieh H., Chen K., Lin Y., Huang Y. C., Baird A., Tsai W., et al. (2011). Establishment of a no-take area (NTA) could not guarantee the preservation of coral communities in Chinwan Inner Bay, Penghu, Taiwan. Zool. Stud. 50, 443–453.
Ishida S. (2004). Life history of the Muricid Gastropod, Cronia margariticola (Broderip 1833): Growth mode transition with season and sexual maturity. Benthos Res. 59, 35–44. doi: 10.5179/benthos1996.59.1_35
Ismail N. S., Elkarmi A., Al-Moghrabi S. M. (2000). Population structure and shell morphometrics of the corallivorous gastropod Drupella cornus (Gastropoda: Prosobranchia) in the Gulf of Aqaba, Red Sea. Indian J. Mar. Sci. 29, 165–170.
Johnson M. S., Cumming R. L. (1995). Genetic distinctness of three widespread and morphologically variable species of Drupella (Gastropoda, Muricidae). Coral Reefs 14 (2), 71–78. doi: 10.1007/BF00303426
Johnson M. S., Holborn K., Black R. (1993). Fine-scale patchiness and genetic heterogeneity of recruits of the corallivorous gastropod Drupella cornus. Mar. Biol. 117 (1), 91–96. doi: 10.1007/BF00346429
Johnson K., Jackson J., Budd A. (2008). Caribbean reef development was independent of coral diversity over 28 million years. Science 319, 1521–1523. doi: 10.1126/science.1152197
Kamya P., Byrne M., Mos B., Dworjanyn S. (2018). Enhanced performance of juvenile crown of thorns starfish in a warm-high CO2 ocean exacerbates poor growth and survival of their coral prey. Coral Reefs 37, 751–762. doi: 10.1007/s00338-018-1699-5
Kaullysing D., Gopeechund A., Mattan-Moorgawa S., Taleb-Hossenkhan N., Kulkarni B., Bhagooli R. (2016). “Increased density of the corallivore Drupella cornus on Acropora muricata colonies overgrown by Padina boryana,” in Proceedings of the 13th International Coral Reef Symposium (Honolulu), 288–304.
Kaullysing D., Mehrotra R., Arnold S., Ramah S., Allchurch A., Haskin E., et al. (2020). Multiple substrates chosen in mass in situ egg deposition by Drupella in Mauritius, a first record for the western Indian Ocean. J. Molluscan Stud. 86, 1–4. doi: 10.1093/mollus/eyaa023
Kaullysing D., Taleb-Hossenkhan N., Kulkarni B., Bhagooli R. (2017). A first field report of various coral-eating gastropods and associated infestations around Mauritius Island, Western Indian Ocean. Western Indian Ocean J. Mar. Sci. 1, 73–75.
Kayal M., Vercelloni J., Lison de Loma T., Bosserelle P., Chancerelle Y., Geoffroy S., et al. (2012). Predator crown-of-thorns starfish (Acanthaster planci) outbreak, mass mortality of corals, and cascading effects on reef fish and benthic communities. PloS One 7, e47363. doi: 10.1371/journal.pone.0047363
Kimura Y., Furuse H., Okazaki S., Suzuki Y. (2005). Endoscope-assisted tonsillectomy. J. Japan Soc. Head Neck Surg. 15 (2), 165–168. doi: 10.5106/jjshns.15.165
Kita M., Kitamura M., Koyama T., Teruya T., Matsumoto H., Nakano Y., et al. (2005). Feeding attractants for the muricid gastropod Drupella cornus, a coral predator. Tetrahedron Lett. 46 (49), 8583–8585. doi: 10.1016/j.tetlet.2005.09.182
Kitamura T., Iwai T., Shigematsu Y., Miura C., Miura T. (2021). Production of specific polyclonal antibody against the larvae of corallivorous gastropod Drupella fragum for prediction of outbreaks. Sustainability 13, 11713. doi: 10.3390/su132111713
Kitamura T., Shigematsu Y., Iwai T., Miura C., Miura T. (2022). The spawning season of Drupella fragum in southwestern Shikoku. Biogeography 24, 32–38.
Koido T., Oku Y., Fukuda M., Nakano A., Fukami H. (2017). Drupella outbreak in a large coral community off the coast of Cape Toi, Miyazaki, Japan. Galaxea J. Coral Reef Stud. 19, 31–32. doi: 10.3755/galaxea.19.1_31
Kroon F., Barneche D., Emslie M. (2021). Fish predators control outbreaks of crown-of-thorns starfish. Nat. Commun. 12, 6986. doi: 10.1038/s41467-021-26786-8
Lachs L., Johari N., Le D. Q., Safuan C., Duprey N., Tanaka K., et al. (2019). Effects of tourism-derived sewage on coral reefs: Isotopic assessments identify effective bioindicators. Mar. pollut. Bull. 148, 85–96. doi: 10.1016/j.marpolbul.2019.07.059
Lam K., Shin P. K. S., Hodgson P. (2007). Severe bioerosion caused by an outbreak of corallivorous Drupella and Diadema at Hoi Ha Wan Marine Park, Hong Kong. Coral Reefs 26 (4), 893–894. doi: 10.1007/s00338-007-0288-9
Lamb J., True J., Piromvaragorn S., Willis B. (2014). Scuba diving damage and intensity of tourist activities increases coral disease prevalence. Biol. Conserv. 178, 88–96. doi: 10.1016/j.biocon.2014.06.027
Leckraz S. K. (2015). Spatio-temporal variations in the community structure at two coral reefs sites around Mauritius Island (Scotland, UK: M.Sc., University of Aberdeen), p. 34.
Lei X., Liu C., Zhang Y., Yu X., Yang J., Luo Y., et al. (2022). Spatial variability in the abundance and prey selection of the corallivorous snail Drupella spp. in the southeastern Hainan Island, China. Front. Mar. Sci. 9. doi: 10.3389/fmars.2022.990113
Lesser M. (2021). Eutrophication on coral reefs: What is the evidence for phase shifts, nutrient limitation and coral bleaching. BioScience 71, 1216–1233. doi: 10.1093/biosci/biab101
Macneil A., Mellin C., Matthews S., Wolff N., McClanahan T., Devlin M., et al. (2019). Water quality mediates resilience on the Great Barrier Reef. Nat. Ecol. Evol. 3, 620–627. doi: 10.1038/s41559-019-0832-3
Marimuthu N., Tripathy B. (2018). First report of Drupella cornus Röding 1798 (Gastropoda: Muricidae), a biological indicator of coral reef habitat of Lakshadweep Archipelago, India. Records Zool. Survey India 118 (1), 97–99. doi: 10.26515/rzsi/v118/i1/2018/122305
Mellin C., Peterson E. E., Puotinen M., Schaffelke B. (2020). Representation and complementarity of the long-term coral monitoring on the Great Barrier Reef. Ecol. Appl. 30 (6), e02122. doi: 10.1002/eap.2122
Middelfart P. (1996). Egg capsules and early development of ten muricid gastropods from Thai waters. Phuket Mar. Biol. Center Special Publ. 16, 103–130.
Miller M. (2001). Corallivorous snail removal: Evaluation of impact on Acropora palmata. Coral Reefs 19, 293–295. doi: 10.1007/PL00006963
Moerland M., Scott C., Hoeksema B. (2016). Prey selection of corallivorous muricids at Koh Tao (Gulf of Thailand) four years after a major coral bleaching event. Contributions to Zool. Bijdragen tot dierkunde 85, 291–309. doi: 10.1163/18759866-08503003
Montano S., Aeby G., Galli P., Hoeksema B. (2022). Feeding behavior of Coralliophila sp. on corals affected by Caribbean ciliate infection (CCI): A new possible vector? Diversity 14, 363. doi: 10.3390/d14050363
Morton B. (2002). Corallivory and prey choice by Drupella rugosa (Gastropoda: Muricidae) in Hong Kong. J. Molluscan Stud. 68, 217–223. doi: 10.1093/mollus/68.3.217
Morton B., Blackmore G. (2009). Seasonal variations in the density of and corallivory by Drupella rugosa and Cronia margariticola (Caenogastropoda: Muricidae) from the coastal waters of Hong Kong: ‘plagues’ or A’ggregations’? J. Mar. Biol. Assoc. United Kingdom 89, 147–159. doi: 10.1017/S002531540800218X
Moyer J. T., Emerson W. K., Ross M. (1982). Massive destruction of scleractinian corals by the muricid gastropod, Drupella, in Japan and the Philippines. Nautilus 96, 69–82.
Nama S., Shanmughan A., Nayak B. B., Bhushan S., Ramteke K. (2023). Impacts of marine debris on coral reef ecosystem: A review for conservation and ecological monitoring of the coral reef ecosystem. Mar. pollut. Bull. 189, 114755. doi: 10.1016/j.marpolbul.2023.114755
Nguyen D. H., Chu N. H., Bettarel Y., Auguet J. C., Bouvier T., Chu H. H., et al. (2023). Metagenomic data of bacterial communities associated with Acropora species from Phu Quoc Islands, Vietnam. Data Brief 47, 108977. doi: 10.1016/j.dib.2023.108977
Nicolet K., Chong-Seng K., Pratchett M., Willis B., Hoogenboom M. (2018). Predation scars may influence host susceptibility to pathogens: Evaluating the role of corallivores as vectors of coral disease. Sci. Rep. 8 (1), 5258. doi: 10.1038/s41598-018-23361-y
Nicolet K. J., Hoogenboom M. O., Gardiner N. M., Pratchett M. S., Willis B. L. (2013). The corallivorous invertebrate Drupella aids in transmission of brown band disease on the Great Barrier Reef. Coral Reefs 32 (2), 585–595. doi: 10.1007/s00338-013-1010-8
Onton K., Page C., Wilson S., Neale S., Armstrong S. (2011). Distribution and drivers of coral disease at Ningaloo reef, Indian Ocean. Mar. Ecol. Prog. Ser. 433, 75–84. doi: 10.3354/meps09156
Ouedraogo D. Y., Mell H., Perceval O., Burga K., Domart-Coulon I., Hedouin L., et al. (2023). What are the toxicity thresholds of chemical pollutants for tropical reef-building corals? A systematic review. Environ. Evid. 12 (1), 1–38. doi: 10.1186/s13750-023-00298-y
Pillay R., Suraj B. G., Vishwakalyan B., Curpen S. (2011). Adapting coral culture to climate change: the Mauritian experience. Western Indian Ocean J. Mar. Sci. 10, 155–167.
Pratchett M. S. (2005). Dynamics of an outbreak population of Acanthaster planci at Lizard Island, northern Great Barrier Reef, (1995–1999). Coral Reefs 24 (3), 453–462. doi: 10.1007/s00338-005-0006-4
Pratchett M. (2010). Changes in coral assemblages during an outbreak of Acanthaster planci at Lizard Island, northern Great Barrier Reef, (1995–1999). Coral Reefs 29, 717–725. doi: 10.1007/s00338-010-0602-9
Pratchett M., Caballes C., Rivera-Posada J., Sweatman H. (2014). Limits to understanding and managing outbreaks of crown-of-thorns starfish (Acanthaster spp.). Oceanogr. Mar. Biol. 52, 133–200. doi: 10.1201/b17143-4
Pratchett M., Caballes C., Wilmes J., Matthews S., Mellin C., Sweatman H., et al. (2017). Thirty years of research on crown-of-thorns starfish, (1986–2016): Scientific advances and emerging opportunities. Diversity 9, 41. doi: 10.3390/d9040041
Przeslawski R. (2004). A review of the effects of environmental stress on embryonic development within intertidal gastropod egg masses. Molluscan Res. 24, 43–63. doi: 10.1071/MR04001
Rahman M. N., Shozib S. H., Akter M. Y., Islam A. R. M. T., Islam M. S., Sohel M. S., et al. (2023). Microplastic as an invisible threat to the coral reefs: Sources, toxicity mechanisms, policy intervention, and the way forward. J. Hazard. Mater. 454, 131522. doi: 10.1016/j.jhazmat.2023.131522
Ratianingsih R., Ismawati N., Puspita J., Jaya A. (2017). The role of top-predator in the preservation of coral reefs ecosystem. Commun. Biomathematical Sci. 1, 54–61. doi: 10.5614/cbms.2017.1.1.5
Rice M., Ezzat L., Burkepile D. (2019). Corallivory in the anthropocene: Interactive effects of anthropogenic stressors and corallivory on coral reefs. Front. Mar. Sci. 5. doi: 10.3389/fmars.2018.00525
Rogers J., Plaganyi E. (2022). Culling corallivores improves short-term coral recovery under bleaching scenarios. Nat. Commun. 13, 2520. doi: 10.1038/s41467-022-30213-x
Ruan Y. (2021). “Outbreak and prevention of Drupa and Drupella,” in IOP Conference Series: Earth and Environmental Science, Vol. 781. 032066. doi: 10.1088/1755-1315/781/3/032066
Sadovy Y., Kulbicki M., Labrosse P., Lokani P., Donaldson T. (2003). The humphead wrasse, Cheilinus undulatus: Synopsis of a threatened and poorly known giant coral reef fish. Rev. Fish Biol. Fisheries 13, 327–364. doi: 10.1023/B:RFBF.0000033122.90679.97
Sam S. Q., Taira D., Toh T. C., Chou L., Ng C. S. L., Kikuzawa Y. (2016). First observation of Drupella rugosa egg capsules on scleractinian coral Pocillopora damicornis. Bull. Mar. Sci. 92, 353–354. doi: 10.5343/bms.2016.1062
Sam S. Q., Toh T. C., Kikuzawa Y. P., Ng C. S. L., Taira D., Afiq-Rosli L., et al. (2017). Egg capsules and veligers of the corallivorous muricid gastropod Drupella rugosa (Born 1778). Invertebrate Reprod. Dev. 61 (3), 164–171. doi: 10.1080/07924259.2017.1315343
Samsuri A., Kikuzawa Y., Taira D., Sam S. Q., Sim W. T., Ng C. S. L., et al. (2018). The effectiveness of Trapezia cymodoce in defending its host coral Pocillopora acuta against corallivorous Drupella. Mar. Biol. 165, 72–78. doi: 10.1007/s00227-018-3330-2
Saponari L., Dehnert I., Galli P., Montano S. (2021). Assessing population collapse of Drupella spp. (Mollusca: Gastropoda) 2 years after a coral bleaching event in the Republic of Maldives. Hydrobiologia 848 (11), 2653–2666. doi: 10.1007/s10750-021-04546-5
Scott C., Mehrotra R., Hein M., Moerland M., Hoeksema B. (2017a). Population dynamics of corallivores (Drupella and Acanthaster) on coral reefs of Koh Tao, a diving destination in the Gulf of Thailand. Raffles Bull. Zool. 65, 68–79.
Scott C., Mehrotra R., Hoeksema B. (2017b). In-situ egg deposition by corallivorous snails on mushroom corals at Koh Tao (Gulf of Thailand). J. Molluscan Stud. 83, 360–362. doi: 10.1093/mollus/eyx020
Shafir S., Gur O., Rinkevich B. (2008). A Drupella cornus outbreak in the northern Gulf of Eilat and changes in coral prey. Coral Reefs 27 (2), 379–379. doi: 10.1007/s00338-008-0353-z
Sheppard C., Williams G., Exton D., Keith S. (2023). Co-occurrence of herbivorous fish functional groups correlates with enhanced coral reef benthic state. Global Ecol. Biogeogr. 32, 435–449. doi: 10.1111/geb.13638
Tan K. S. (2000). Species checklist of muricidae (Mollusca: Gastropoda) in the South China Sea. Raffles Bull. Zool. 48 (8), 495–512.
Thaha P. P., Rathod J. L. (2019). Predation of corallivorous snail (Drupella sp.) on the hard corals of Chetlat Atoll, Lakshadweep, India. J. Mar. Biol. Assoc. India 61 (2), 79–83. doi: 10.6024/jmbai.2019.61.2.2126-12
Tsang R. H. L., Ang P. O. (2019). Resistance to temperature stress and Drupella corallivory may promote the dominance of Platygyra acuta in the marginal coral communities in Hong Kong. Mar. Environ. Res. 144, 20–27. doi: 10.1016/j.marenvres.2018.11.009
Tsang R. H. L., Chui A. P. Y., Wong K. T., Ang P. (2018). Corallivory plays a limited role in the mortality of new coral recruits in Hong Kong marginal coral communities. J. Exp. Mar. Biol. Ecol. 503, 100–108. doi: 10.1016/j.jembe.2018.03.003
Turner S. J. (1992). The egg capsules and early life history of the corallivorous gastropod Drupella cornus (Röding 1798). Veliger 35, 16–25.
Turner S. J. (1994). The biology and population outbreaks of the corallivorous gastropod Drupella on Indo-Pacific reefs. Oceanogr. Mar. Biol. 32, 461–530.
Wang X., Lin H., Zhang M., Sun T. (2022). Research status and development trend of coral reef ecological restoration technology based on bibliometric analysis. Acta Scientiae Circumstantiae 42 (1), 321–328.
Widiastuti W. (2019). “Status of coral diseases and compromised health syndromes on Pemuteran shallow reefs, North Bali island,” in IOP Conference Series: Earth and Environmental Science, Vol. 236. 012048. doi: 10.1088/1755-1315/236/1/012048
Williams S. T., Knowlton N., Weigt L. A., Jara J. A. (2001). Evidence for three major clades within the snapping shrimp genus Alpheus inferred from nuclear and mitochondrial gene sequence data. Mol. Phylogenet. Evol. 20 (3), 375–389. doi: 10.1006/mpev.2001.0976
Keywords: coral reef deterioration, coral predator, corallivorous gastropod, outbreak hallmark, mitigation strategies
Citation: Zhang F, Jia X, Lin Z, Jiang Y and Qu M (2024) The outbreak of Drupella snails and its catastrophic effects on coral reefs: a comprehensive review. Front. Mar. Sci. 10:1290001. doi: 10.3389/fmars.2023.1290001
Received: 15 September 2023; Accepted: 12 December 2023;
Published: 04 January 2024.
Edited by:
Aldo Cróquer, The Nature Conservancy, Dominican RepublicReviewed by:
Hui Huang, Chinese Academy of Sciences (CAS), ChinaZhongli Chen, Chongqing University, China
Copyright © 2024 Zhang, Jia, Lin, Jiang and Qu. This is an open-access article distributed under the terms of the Creative Commons Attribution License (CC BY). The use, distribution or reproduction in other forums is permitted, provided the original author(s) and the copyright owner(s) are credited and that the original publication in this journal is cited, in accordance with accepted academic practice. No use, distribution or reproduction is permitted which does not comply with these terms.
*Correspondence: Mengjie Qu, mengjiequ@zju.edu.cn