Nutrient enrichment alters phytoplankton biomass and composition via silicon limitation
- 1Marine Ecology, GEOMAR Helmholtz Centre for Ocean Research Kiel, Kiel, Germany
- 2Department of Biology, Dalhousie University, Halifax, NS, Canada
Despite management efforts, anthropogenic nutrient enrichments continue to enhance phytoplankton blooms worldwide. Release of nitrogen and phosphorus compounds not only provides surplus of nutrients but also disbalances their stoichiometry. Declines in the relative availability of dissolved silicon might induce limitation in diatoms, major primary producers with silicified shells. We studied experimentally how nutrient enrichment and resulting decline in dissolved silicon to nitrogen ratios (Si:N) affect the structure and functioning of natural plankton communities. Nitrate was added to create a range of Si:N ratios and phosphate was supplied in Redfield ratio to nitrogen. We also manipulated copepod abundance to understand the top-down effects on communities experiencing nutrient enrichment. Nitrogen and phosphorus additions resulted in a steep phytoplankton biomass increase, followed by a post-bloom decline. Phytoplankton bloom biomass was higher in high nitrogen treatments but during the post-bloom period this trend switched. Biomass was sustained longer in high Si:N treatments, indicating that silicon limitation terminates the bloom. Many diatom species did not benefit from nitrogen and phosphorus enrichment and diatom dominance ceased below Si:N of 0.4:1. Under high grazing pressure, silicate was taken up faster suggesting that silicification is important in diatom defense. Copepods shaped plankton communities via feeding on dinoflagellates, chlorophytes and the diatom Skeletonema costatum but there was no significant effect of nitrogen and phosphorus enrichment on copepod abundance. Our results, combined with previous studies, show that while nutrient concentrations define the total phytoplankton bloom biomass, resource ratios are important in sustaining biomass and determining community structure and composition.
1 Introduction
Over the past century, anthropogenic nitrogen fixation has doubled the global cycling of nitrogen (Fowler et al., 2013; Stevens, 2019). Similarly, phosphorus influx to the oceans has seen a comparable increase, doubling in comparison to levels before humans (Filippelli, 2008). Major sources of anthropogenic nitrogen and phosphorus pollution include the use of fertilisers and release of human and animal waste. Combustion of fossil fuels is a source of atmospheric nitrogen compounds and increased deforestation and soil loss result in the further release of phosphorus compounds (Filippelli, 2008; Conant et al., 2013; Stevens, 2019). Climate change might further accelerate nitrogen (N) and phosphorous (P) pollution due to predicted increases in precipitation in many regions (Sinha et al., 2017). The supply of dissolved silicon (Si), originates mainly from natural sources, such as rock weathering. Silicon supply has not changed or even decreased over the last decades due to damming-related increases in sedimentation (Treguer and de la Rocha, 2013).With increased N and P concentrations and slower river flow more of the bioavailable Si is taken up by silicifying phytoplankton and exported to sediments before reaching coastal waters (Friedl and Wüest, 2002). Additionally, ocean acidification may reduce silicon availability due to decreasing chemical solubility of silica (Taucher et al., 2022).
Both the reduction in Si availability and the increase in N and P can result in lowered Si:N and Si:P ratios in coastal and marine waters. While phosphorus is often limiting primary production in freshwater systems, in marine systems nitrogen is frequently a more constraining factor (Elser et al., 2007; Capone et al., 2008). For this reason, in this paper, we will concentrate on the effects of changes in Si:N ratios, while it is important to note that Si:P ratios change simultaneously when both N and P availability increases.
Changes in nutrient supply ratios are important as they can affect community composition of primary producers. As mechanistically explained in Tilman’s resource ratio theory, ratios between limiting resources can predict the outcome of species competition (Tilman, 1982). Thus, when Si:N ratios are lowered, non-silicifying phytoplankton have the potential to outcompete silicifyers, such as diatoms. This has been shown in several experiments where silicate concentration was manipulated to achieve reduction in Si:N ratios (Egge and Aksnes, 1992; Sommer, 1998; Sommer et al., 2005; Sommer, 2009; Moriceau et al., 2018; Makareviciute-Fichtner et al., 2020).
Yet in nature, increasing nitrogen concentrations rather than declining silicate may be the more important reason behind lowered Si:N ratios in coastal areas (Downing et al., 2016). Nitrogen, as opposed to silicon, is an essential nutrient for all phytoplankton groups and increasing nitrogen (and phosphorus) concentrations are known to increase total phytoplankton biomass (Smith, 2006). While nitrogen fixing cyanobacteria can use atmospheric N2 as a nitrogen source, all other phytoplankton rely on combined and dissolved nitrogen sources, mainly nitrate (NO3-) and ammonium (NH4+). High phytoplankton biomass can increase shading and as a result light rather than nutrients may become the limiting resource structuring phytoplankton community composition (Brauer et al., 2012). While nitrogen enrichment effects on phytoplankton have been studied extensively, only a few studies have simultaneously addressed the changing relative availability of silicate (Schöllhorn and Granéli, 1996; Kuosa et al., 1997; Granéli et al., 1999; Gilpin et al., 2004). Thus, there is limited knowledge on the mechanistic regulation of the composition and biomass of unicellular plankton over a gradient of Si:N ratios under nitrogen enrichment.
Another overlooked aspect is the influence of differential mesozooplankton grazing pressure on communities experiencing silicon limitation. It is known that thickly silicified cell walls provide effective defence against grazers (Pancic et al., 2019) but it is not understood to what extent silicon limitation could enhance accessibility of diatoms to their predators in natural communities. If diatoms are replaced by non-silicifying unicellular plankton, they can be either beneficial, nuisance or toxic for copepods. Thus, it is important not only to differentiate the influence of nutrient fertilization and silicon limitation on phytoplankton composition but also to investigate selective feeding and the effects of changing diet on copepods.
We set out to experimentally assess how diatoms, non-silicifying phytoplankton, microzooplankton and copepods respond to nitrogen and phosphorus enrichment and the resulting decline in relative silicate availability. We also tested whether this bottom-up driven response can be altered by changing top-down control. We discuss the importance of ratios and concentrations of nutrient components while comparing the results of this study to an analogous experiment (Makareviciute-Fichtner et al., 2020) where the same Si:N ratios were achieved by changing silicate concentrations instead of nitrogen.
2 Methods
2.1 Experimental design
The experiment was conducted in a small mesocosm system in Kiel Fjord Germany (54°19’46.9”N 10°08’59.4”E) between June 19th and July 7th 2017. Natural Baltic Sea water was filtered using 125µm mesh size filters to exclude larger mesozooplanktonic grazers and distributed among twenty 20L transparent plastic water-bags which served as experimental units. In these, we factorially manipulated nutrient concentrations with grazer density. Precisely, five different nutrient Si:N ratios (Table 1) were realized by adding nitrogen as nitrate (NaNO3) while keeping silicate constant. To exclude possible effects of phosphorus limitation, phosphate (KH2PO4) was added to keep N:P ratio according to Redfield proportions (16:1) (Redfield, 1934) in all treatment levels. N, comparing to P, is often the more limiting nutrient in marine systems (Elser et al., 2007; Capone et al., 2008), thus throughout the manuscript we refer to manipulations in Si:N:P as Si:N (with N:P constant). Nutrient conditions at the start of the experiment were close to seasonal high nutrient conditions (the period before the spring bloom or during wind-induced upwelling). The experimental units were randomly placed in four bigger containers equipped with a flow through system with fjord-water to keep the ambient temperatures and a plastic lid to reduce harmful UV light levels at the surface (allowing ~50% of solar radiation to reach experimental bags).
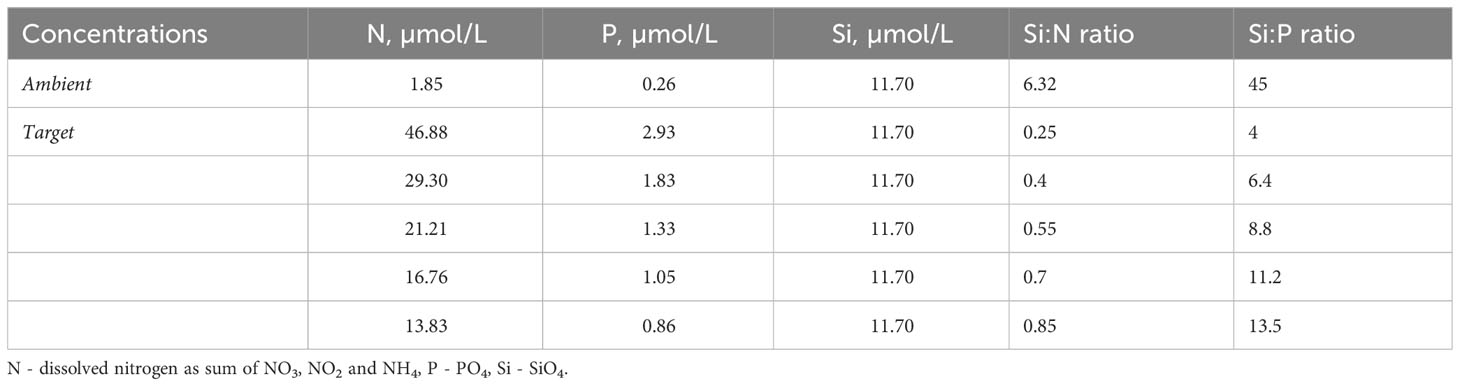
Table 1 Nutrient concentrations before experimental manipulation (ambient) and target concentrations for achieving a range of Si:N ratios.
Once a phytoplankton bloom had established (22nd of June), copepods were added to half of the experimental units at a target concentration of 30 individuals per liter (high copepod treatments). Remaining bags had lowered copepod concentrations due to filtration at the start of the experiment (low copepod treatments). All treatment combinations were realized in duplicates. Copepods were dominated by the species Eurytemora affinis and were collected with a bongo plankton net (Hydro-Bios) pulled behind a research vessel in Kiel Canal. They were kept in culture in 300L aerated buckets with filtered sea water and fed daily with Rhodomonas algae until experimental manipulation.
2.2 Sampling
Fluorescence, temperature, pH and salinity were measured six times per week to follow the development of the experiment. Experimental bags were gently shaken before taking samples to ensure mixing. Relative in vivo chlorophyll a fluorescence was measured using a fluorometer 10-AU (Turner Design) immediately after sampling according to Welschmeyer (1994).
Samples for nutrient, pico-, nano- and microplankton (further referred to as “plankton”) and bacteria analyses were taken three times per week (Monday, Wednesday, and Friday). Water samples for dissolved nutrient analysis (NO3-/NO2-, NH4+, SiO4- and PO43-) were filtered using pre-washed (10% HCl) cellulose acetate filters (pore size 0.45 µm), frozen and kept at -20°C until nutrient measurement according to protocols by Hansen and Koroleff (1999) with an auto-analyser (Skalar, SANPLUS; Breda/Netherlands).
Photosynthetic picoplankton and bacteria were counted using flow-cytometer (FACScalibur, Becton Dickinson). For picoplankton enumeration, 3mL of water were analysed at a flow rate of 35µL min-1 for bacteria counts – 2mL at 8µL min-1. Bivariate plots of forward scattering (FSC) and auto-fluorescence were used to manually gate the different populations. Water samples for nano- and microplankton counts were fixed with acidic Lugol’s iodine solution and later counted according to Utermöhl’s (Utermöhl, 1958) inverted microscope method. For most common species at least 100 individuals were counted giving minimum 20% accuracy (Andersen, 2005). For each species, 20 randomly selected cells were measured and biovolume was calculated according to their closest geometric forms (Hillebrand et al., 1999). Biovolume was converted to carbon according to the allometric conversion suggested by Menden-Deuer and Lessard (2000) for all plankton groups except picoplankton, where conversion with a factor of 0.157 pg C µm3 was used in order to avoid overestimation of small species biomass (Sommer et al., 2012).
For copepod counts, water from experimental bags at the end of the experiment was filtered by 125 µm mesh size filter, fixed using Lugol’s iodine solution and stored in the dark. Copepods were counted using a dissecting microscope and identified to species level (adults) or family level (copepodites and nauplii).
2.3 Statistical analysis
To examine treatment effects on total plankton carbon content and biomass of different plankton groups we performed analysis of covariance (ANCOVA). The effects were assessed during two periods of the experiment: “bloom” – which we defined as the period including and surrounding the peak of plankton biomass (days 5-10) and “post-bloom”, where plankton biomass decline after the peak slowed down in most of the treatments (days 15-19). Due to inhomogeneous variances, bacteria abundances were analysed using generalized linear models (GLM) with Gamma family and log link. Statistical analysis and graphical representations were performed in R version 3.2.4 (R Core Team, 2019) including packages ggplot2 (Wickham, 2016) and reshape2(Wickham, 2017).
3 Results
3.1 Dissolved nutrients
Dissolved nutrients were depleted fast and reached low levels already by day 5 of the experiment (Figure 1). Only in the lowest Si:N treatments dissolved nitrogen (N) and phosphorus (P) remained relatively high until day 8, while in the remaining treatments N and P concentrations were low and decreased further with increasing Si:N ratios on day 5 of the experiment (Figure 2). In general, silicate was taken up a little slower than nitrogen and small amounts were still available on day 5 (Figure 1). This led to Si:N ratios becoming even higher in high Si:N treatments (Figures 1, 2) than initially manipulated. Silicate was taken up faster in the high copepod treatments (Figure 2, df=1, χ2 = 7.191, p=0.001). As a result the ratio between dissolved silicon and dissolved nitrogen was higher under low copepod treatments in high Si:N ratio treatments, while the difference in the lowest Si:N treatments was marginal (Figure 2; Supplementary Table S1). Particulate nutrients (N, P and C) increased following the bloom formation and declined slowly afterwards (Supplementary Figure S1).
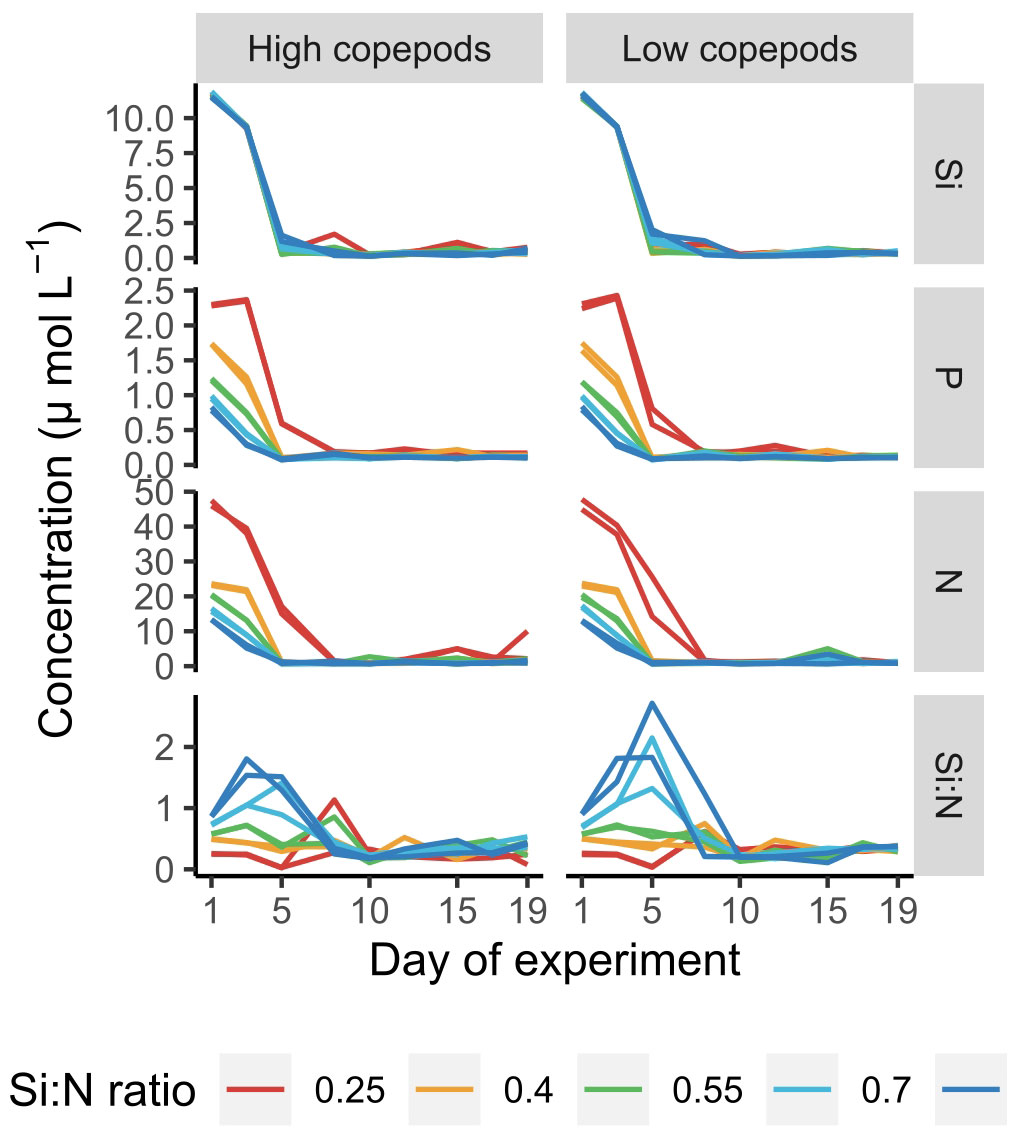
Figure 1 Dissolved nutrient concentrations and Si:N ratio values over the course of experiment. Each line represents an experimental unit. Si - SiO4-, P - PO43-, N - sum of NO3-, NO2- and NH4+, Si:N - molar ratio between Si and N.
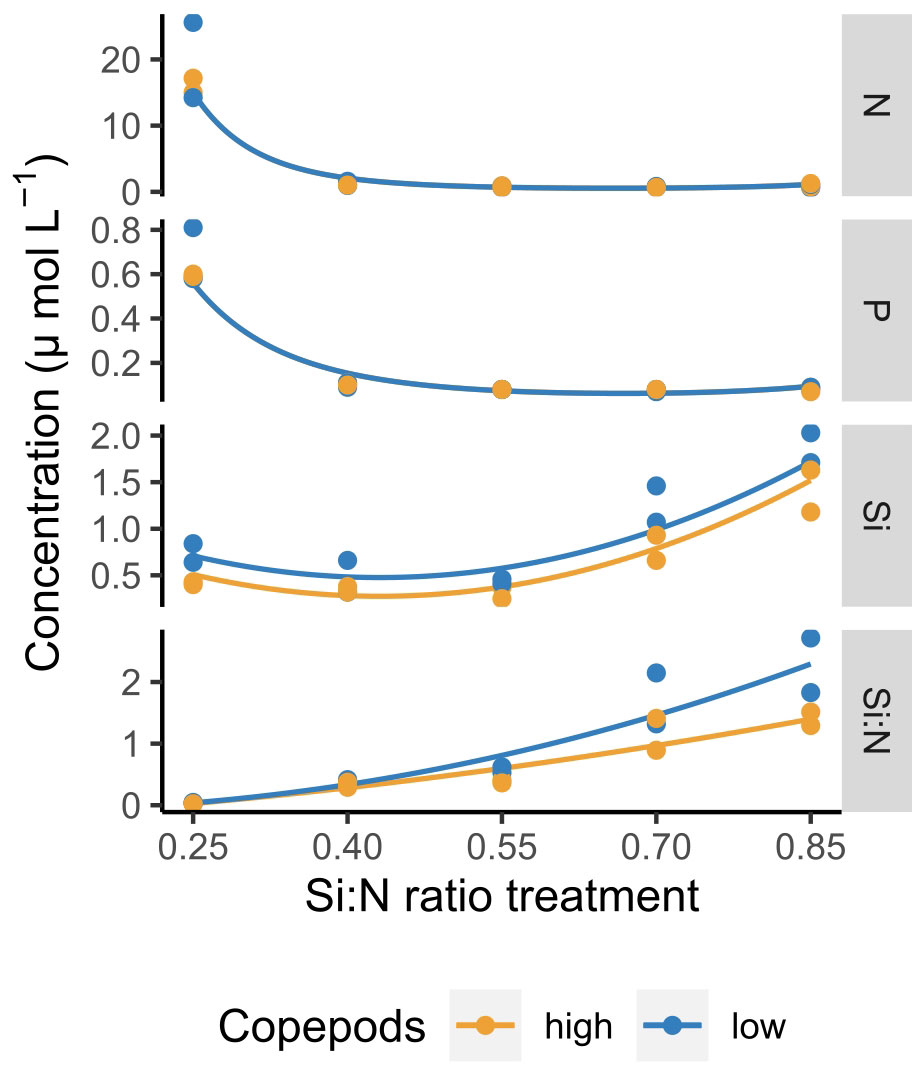
Figure 2 Dissolved nutrient concentrations and Si:N ratios across Si:N ratio treatments on day 5 of the experiment. Lines indicate statistically significant effect of Si:N treatment, colours of the lines are different when the effect of copepod grazing was statistically significant (p<0.05). Statistical details are available in Supplementary Table S1.
3.2 Plankton biomass and composition
Unicellular plankton biomass increased sharply after nutrient manipulation and reached peak values between days 5 and 10 of the experiment (bloom period) (Figure 3A). After the bloom period, plankton biomass declined first sharply and then slowly (post-bloom period) (Figure 3A). During the bloom period plankton biomass was highest in the lowest Si:N treatment (i.e. with highest nitrogen and phosphorus concentration) and decreased with increasing initial Si:N ratios (df=1, F=86.52, p<0.001, Figure 3B). However, during the post-bloom period this trend switched and plankton carbon concentrations increased with increasing Si:N ratios (i.e. with decreasing initial nitrogen and phosphorus concentrations) (df=1, F=7.51, p=0.015, Figure 3C). High copepod abundance had a negative effect on plankton biomass during the bloom period (df=1, F=19.15, p<0.001, Figure 3B) but not during the post-bloom (df=1, F=0.87, p=0.36, Figure 3C).
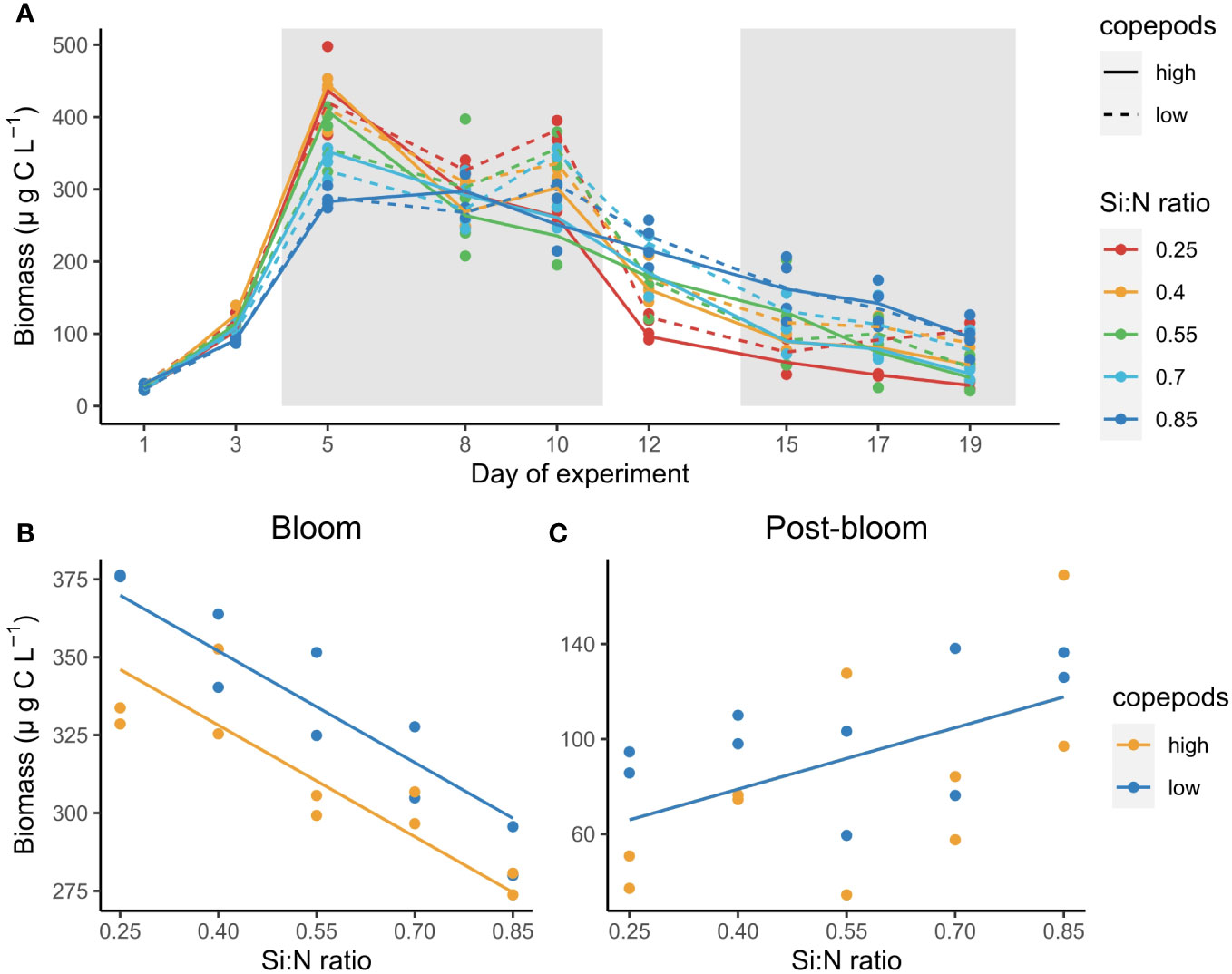
Figure 3 Total plankton biomass change over the course of experiment (A), and average plankton biomass during the bloom (B) and post-bloom (C) periods as a function of Si:N ratio. Grey shaded areas in (A) indicate bloom (days 5-10) and post-bloom (days 15-19) periods. Lines in (B, C) indicate statistically significant effect of Si:N treatment, colours of the lines are different when the effect of copepod grazing was statistically significant (p<0.05).
Plankton composition was relatively diverse at the start of the experiment, but by day 5 diatoms became dominant and formed the major part of the bloom biomass in all treatments (Supplementary Figure S2; Supplementary Table S2). Diatom biomass during the bloom period decreased only slightly along the Si:N gradient while the biomass of picoplankton, dinoflagellates and chlorophytes decreased more strongly (Figure 4). High copepod grazing did not affect diatom and ciliate biomass but resulted in lowered biomass of dinoflagellates, chlorophytes and picoplankton during the bloom period (Figure 4).
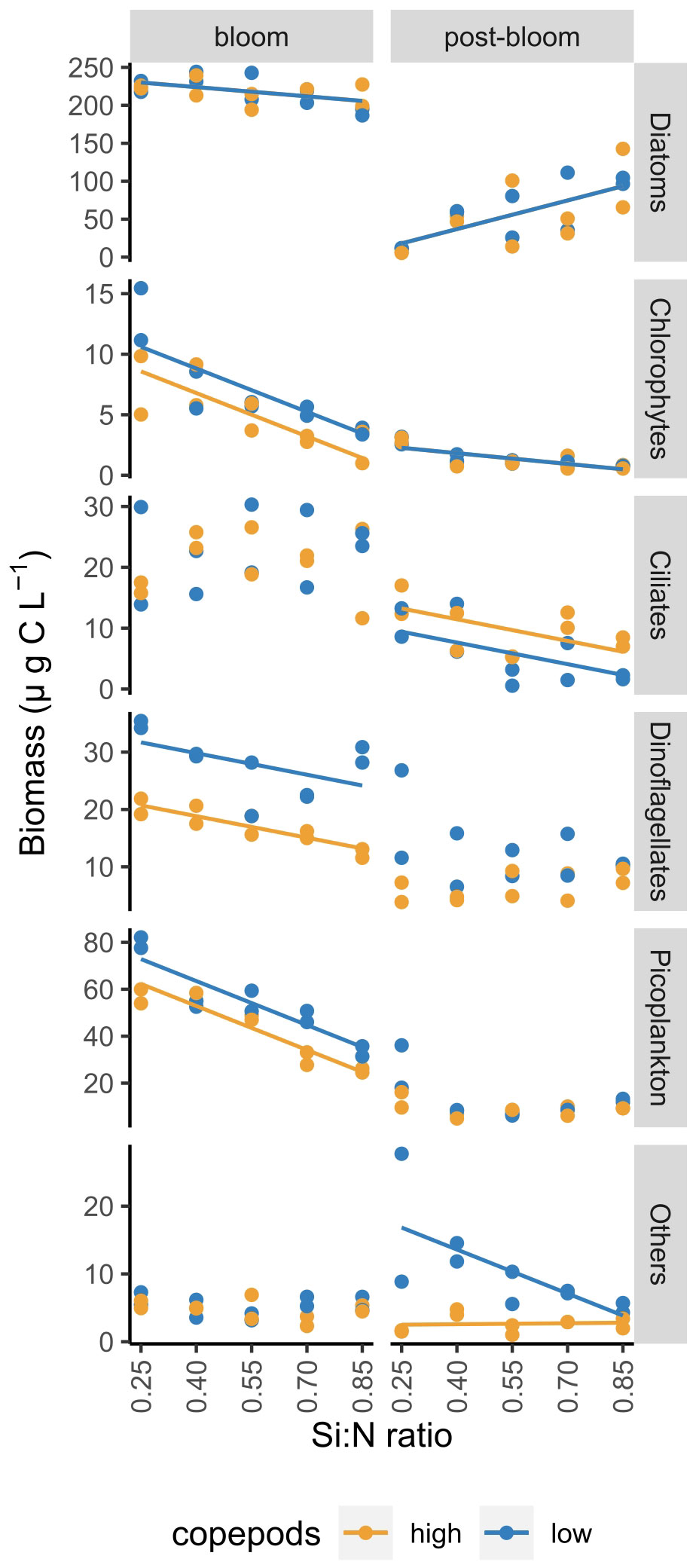
Figure 4 Average biomass of different plankton groups during bloom and post-bloom periods as a function of Si:N ratio. Lines indicate statistically significant effect of Si:N treatment, colours of the lines are different when the effect of copepod grazing was statistically significant (p<0.05). See Supplementary Table S3 for all statistical results.
After the bloom, diatoms remained relatively abundant in all but the lowest Si:N treatment (0.25). Here photosynthetic picoplankton, dinoflagellates and ciliates formed a large part of plankton biomass (Supplementary Figure S2). Total diatom biomass during post-bloom period increased with increasing Si:N ratio, while ciliates, chlorophytes and sum of less common plankton groups (others, under low copepod grazing) decreased with increasing Si:N ratio (Figure 4).
During the bloom period, high copepod grazing significantly affected dinoflagellate, chlorophyte and picoplankton biomass negatively but had no significant effects on diatoms, ciliates and other plankton (Figure 4). During the post-bloom period, ciliate biomass was higher under high copepod grazing, the biomass of other plankton (dominated by unidentified amoeboids) was lower, and diatom, chlorophyte, dinoflagellate and picoplankton biomass were not affected by grazing during this stage of the experiment.
The responses of individual diatom species to nutrient addition and resulting changes in Si:N ratios were not uniform. The biomass of Skeletonema costatum increased sharply after nutrient manipulation and declined afterwards relatively fast in all Si:N treatments (Figure 5). During bloom period, biomass of S.costatum was highest in the lowest Si:N treatments, and decreased with initial Si:N ratio (Figure 6). The biomass of Cerataulina pelagica increased sharply after the start of the experiment and declined fast in low Si:N ratio treatments, yet it sustained moderate biomass until the end of the experiment at high Si:N ratio treatments (Figure 5). Dactyliosolen fragilissimus was the most dominant diatom and its biomass increased slower but remained high also during the post-bloom period in most of the treatments with the exception of the lowest Si:N ratio treatments (Figure 5). The average biomass of D. fragilissimus and C. pelagica increased with initial Si:N ratios already during the bloom period (Figure 6). The bloom of Thalassionema nitzchioides occurred later than of the other species and, while its biomass declined sharply in the lowest Si:N ratio treatments, it sustained its biomass in higher Si:N treatments until the end of the experiment (Figure 5). The average biomass of T. nitzchioides was not affected by Si:N manipulation during the bloom period, but during post-bloom, it’s biomass increased with increasing Si:N ratios, just as the biomass of D. fragilissimus and S. costatum (Figure 6).
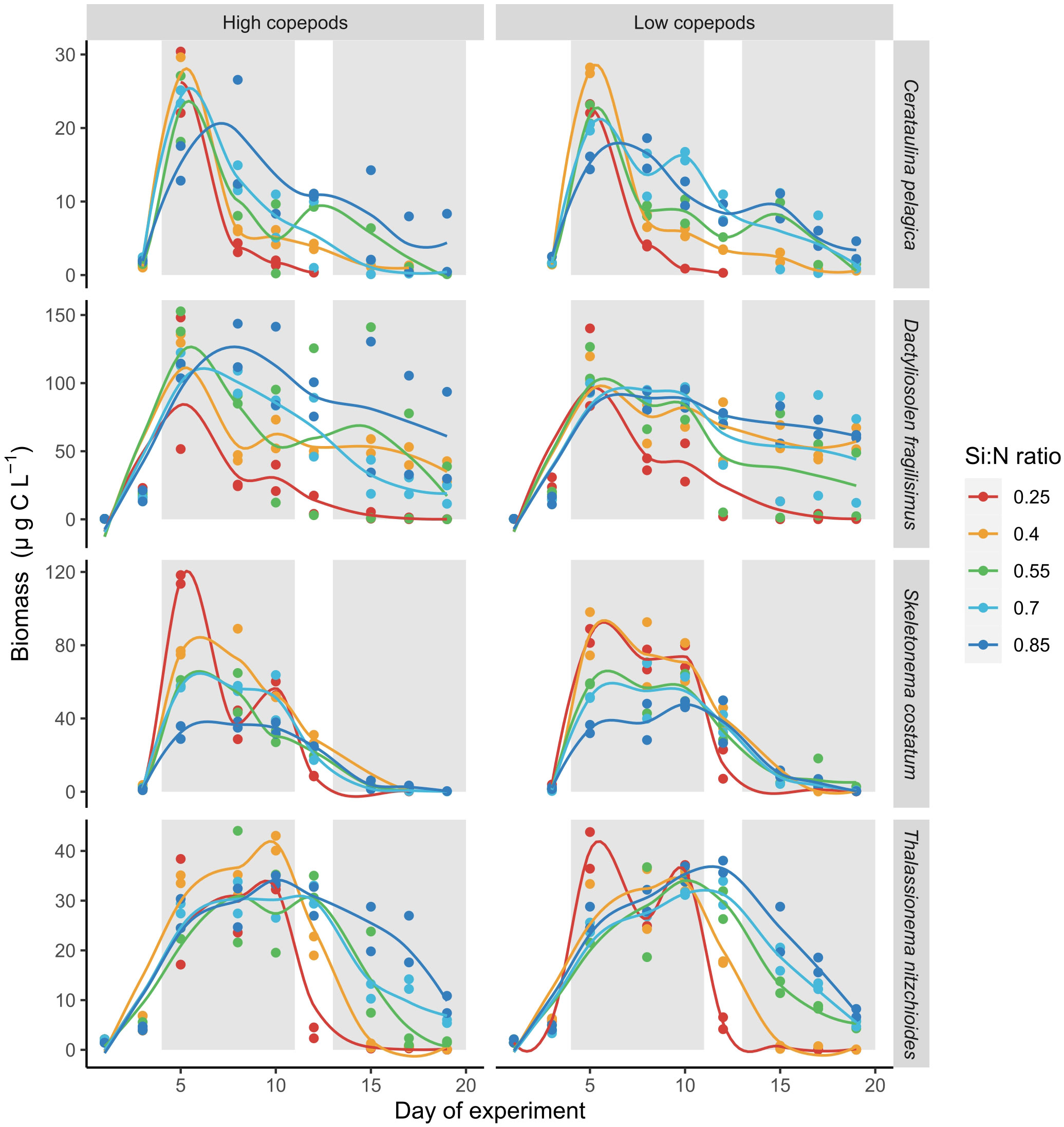
Figure 5 Carbon biomass development of four most common diatom species over the course of experiment. Lines represent smoothed local regression model for each treatment. Gray shaded areas indicate bloom and post-bloom periods.
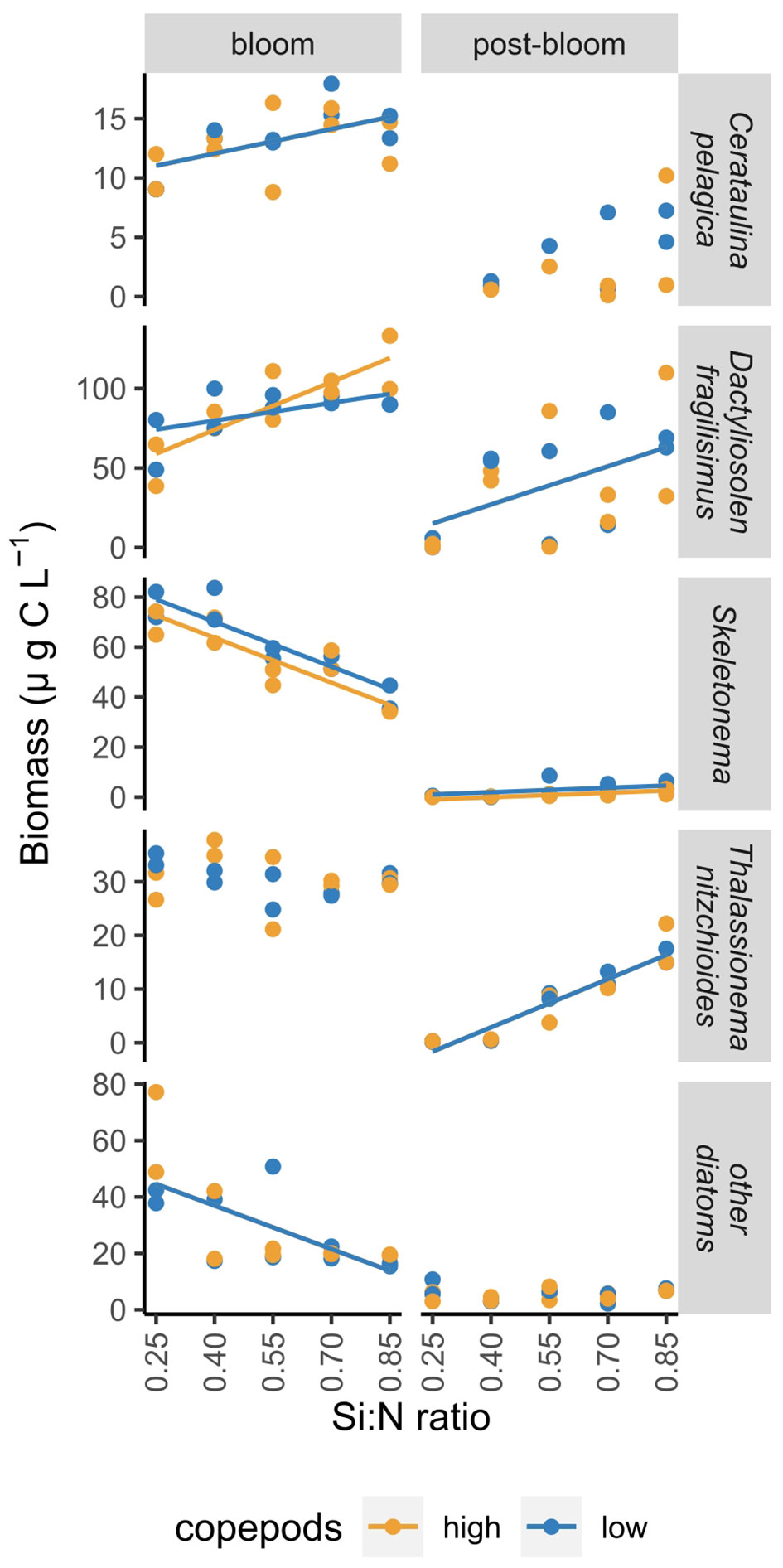
Figure 6 The change in the biomass of most common diatom species over a range of Si:N treatments during bloom and post-bloom periods. Lines indicate where Si:N ratio treatments significantly affected carbon content of different groups, colours of the lines are different when the effect of copepod grazing was statistically significant (p<0.05). See Supplementary Table S4 for all statistical results.
Bacterial abundance increased first during the bloom period and after a slight decline increased even stronger during the post bloom period (Figure 7A). Lowered Si:N ratios affected bacterial abundance positively both during bloom (χ2 = 2.2, df=2, P<0.001, Figure 7B) and post-bloom periods (χ2 = 1.8, df=2, P<0.001, Figure 7C). During the bloom, bacteria abundance was higher in high copepod treatments (χ2 = 0.02, df=1, P<0.001), yet during post-bloom copepods affected bacteria abundance negatively, especially in low Si:N treatments (χ2 = 0.79, df=2, P<0.001, Figure 7C). Neither nauplii, copepodites nor adult copepod abundance responded to Si:N ratio treatments (see Supplementary Figure S3; Supplementary Table S5).
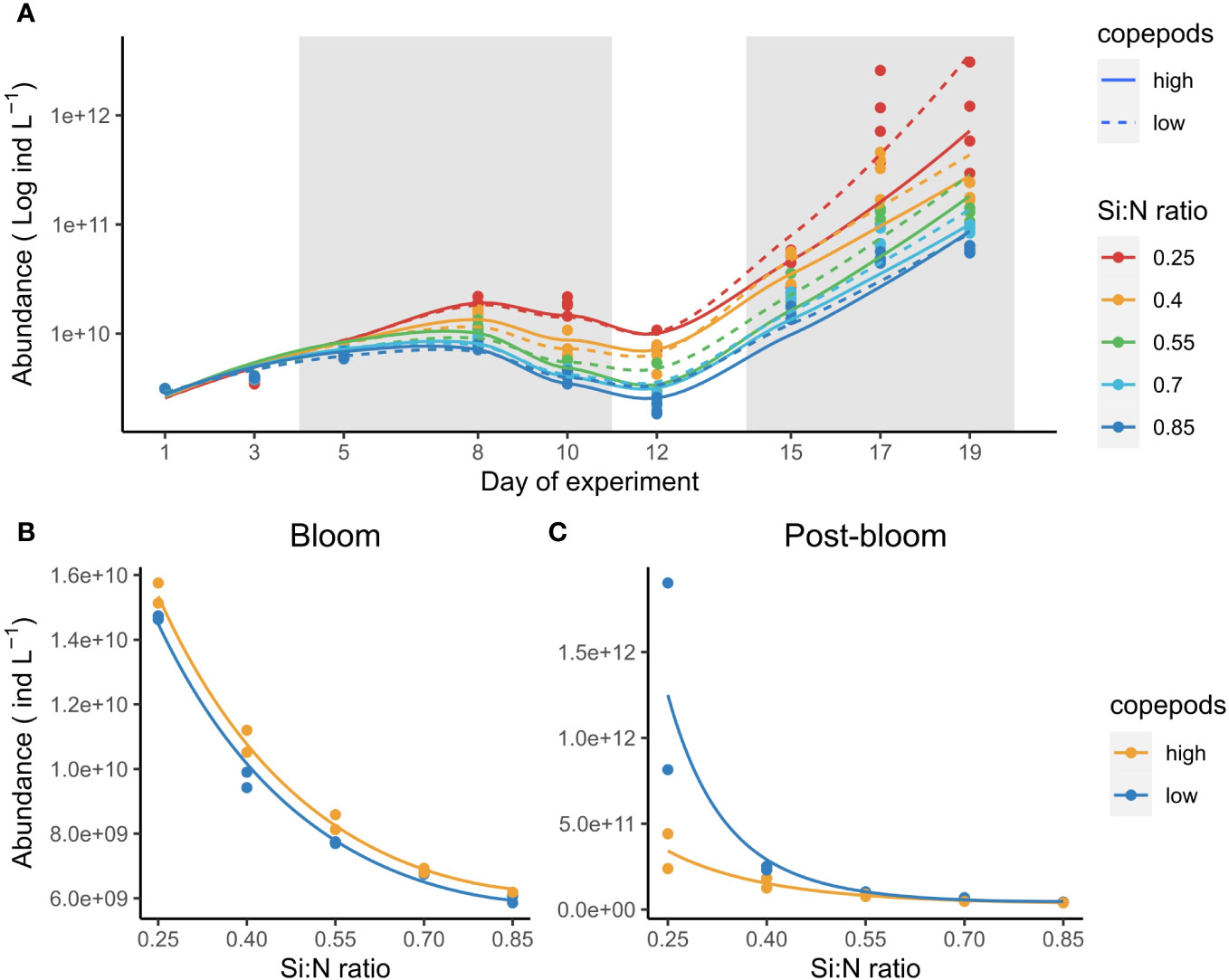
Figure 7 Bacteria abundance over the course of experiment (A) and average abundance during bloom (B) and post-bloom (C) periods. Gray-shaded areas mark plankton bloom and post-bloom periods. Note that y axis in (A) is on log scale to highlight the two bloom stages in bacterial population. Lines in (B, C) indicate statistically significant effect of Si:N treatment, colours of the lines are different when the effect of copepod grazing was statistically significant (p<0.05).
4 Discussion
It is well known that nitrogen and phosphorus enrichment can lead to eutrophication and degradation of aquatic systems (Nixon and Fulweiler, 2009). The role of changing nutrient ratios, however, is debated (Brauer et al., 2012). Our results show that lowered Si:N and Si:P ratios due to nitrogen and phosphorus addition have strong effects on diatoms with consequences for total plankton biomass and likely other groups of plankton, especially during the period after a phytoplankton bloom.
4.1 Plankton response to the increase in nutrient supply and resulting decline in Si:N ratio
Nutrient enrichment, as expected, led to an increase in phytoplankton bloom biomass. While initial phytoplankton community was diverse, diatom proportion increased following experimental manipulation – possibly due to initial silicon availability at the system (Table 1). Coinciding with the build-up of the bloom (day 5) silicate was used up most rapidly in high nutrient (low Si:N) treatments. During the bloom period, total diatom biomass responded to nutrient addition less strongly than other groups (Figure 4). This indicates that already during the bloom period diatoms were affected by silicon limitation in high nutrient treatments (low Si:N).
However, individual diatom species responded differently to nutrient supply. S. costatum did benefit from nitrogen and phosphorus addition: during the bloom period, their biomass was higher in high nitrogen and phosphorus treatments. The growth of S. costatum was characterized by a fast increase followed by a sharp decrease of their biomass once the nutrients were depleted. The biomass of two other common species, D. fragilissimus and C. pelagica, in contrast, did not benefit from nitrogen and phosphorus addition as much - their biomass increased with increasing Si:N ratio treatments already during the bloom. They both grew slower than S. costatum and their biomass declined later. This could possibly be explained by different nutrient uptake and growth rates. S. costatum is smaller than the latter two species and therefore has likely higher growth rates and could take up nutrients more rapidly and grow faster, which coincides with its ability to form blooms under nutrient surplus (Hu et al., 2011). D. fragilissimus and C. pelagica are likely able to store nutrients (Phlips et al., 2010) which would explain their persistent abundance until the end of experiment in high Si:N treatments. In general, biomass of all dominant diatom species persisted longer in higher Si:N treatments. This is in accordance with observations in nature where diatom blooms can be terminated by low silicate concentrations (Krause et al., 2019). Consequently, silicate availability and high Si:N ratios in nutrient supply seem to be essential in sustaining diatoms and in the case of this experiment also total phytoplankton biomass under nutrient limitation.
Silicate is also thought to play a substantial role in diatom defence against copepod grazing as there is experimental evidence from single-species studies that grazing is reduced when cells are heavily silicified (Liu et al., 2016; Pancic et al., 2019). Additionally, Grønning and Kiørboe (2020) reported that diatom cell silicification increases when copepod cues are present and suggested that this could lead to earlier silica limitation of diatom blooms under high copepod grazing pressure. Our study supports this hypothesis with evidence from natural phytoplankton communities – when dissolved nutrients started to be limiting (day 5 of the experiment), silicon was indeed taken up faster under high copepod grazing (Figure 2). This could suggest that diatoms would be more susceptible to grazing under low Si:N ratios. However, grazing did not affect total biomass of diatoms in the current study. Only fast-growing S. costatum was negatively affected by grazing during bloom period (Figure 6). The absence of significant differences between biomass of other diatom species in low and high copepod treatments and higher diatom proportion in plankton under high copepod grazing (Figure 6 indicate that in general diatoms were not preferential food sources for copepods. Diatoms were originally thought to be crucial and nutritious food for mesozooplankton (Mann, 1993), yet in the last two decades evidence has accumulated that diatom diet can cause reduction in copepod egg production, hatching success and post-embryonic development (Carotenuto et al., 2002; Ianora et al., 2003). While copepods do feed on diatoms (Olson et al., 2006), an avoidance of several dominant diatom species has also previously been observed (e.g. Thalassiosira spp. and D. fragilissimus) (Leising et al., 2005; Olson et al., 2006; Makareviciute-Fichtner et al., 2020). This illustrates further need to investigate copepod preferential feeding on a species level, diatom defence mechanisms and their role in copepod nutrition.
Our results show that non-silicifying phytoplankton groups benefited from higher nitrogen and phosphorus availability and possibly reduced competition with diatoms due to lower relative availability of silicon (low Si:N ratio treatments) during the bloom period. However, during post-bloom either the slope of biomass decline with increasing initial Si:N was lower (chlorophytes) or not significant anymore (picoplankton, dinoflagellates). High copepod abundance had a negative effect on dinoflagellate, chlorophyte and picoplankton biomass. Dinoflagellates are a nutritious food source for copepods (Jónasdóttir, 2019), chlorophytes were also a suitable size prey, thus their lower biomass in high copepod treatments could indicate preferential feeding on these groups. Lower picoplankton biomass under high grazing pressure is, however, counter-intuitive. Picoplankton is usually considered poorly accessible food source for copepods due to their small size (Berggreen et al., 1988) and an increase in picoplankton abundance has been observed under high copepod grazing due to trophic cascade effects (copepods feed on major picoplankton grazers – microzooplankton) (Sundt-Hansen et al., 2006; Makareviciute-Fichtner et al., 2020; Zhao et al., 2020). Heterotrophic nanoplankton (HNF) are also important grazers of picoplankton and bacteria (Sherr and Sherr, 2002; Zöllner et al., 2009), however, their abundance was not assessed in our study. HNF can present an additional trophic level between microzooplankton and picoplankton or bacteria (Zöllner et al., 2009) and consequently this could explain the lower picoplankton and bacteria abundance under high copepod grazing pressure during post-bloom. Direct feeding of copepods on picoplankton, however, has also been reported by some studies, presumably via agglomeration or colony formation (Wilson and Steinberg, 2010; Motwani and Gorokhova, 2013). While we are not able to conclude whether higher picoplankton abundance resulted from more complex trophic interactions or due to direct feeding, our results show that increasing Si:N and Si:P ratios might lower the availability of prey in suitable size for copepods.
Bacterial abundance was positively affected by nitrogen and phosphorus enrichment (lower Si:N ratios) possibly due to more decomposition processes occurring following higher biomass production (bloom-period) or higher proportion of detritus (post-bloom period). We hypothesise that the different copepod effects during bloom and post-bloom periods could be either due to trophic or resource control. During bloom, bacterial growth could have been released from grazing pressure by dinoflagellates due to enhanced copepod grazing in high copepod treatments. The increase of bacterial abundance with decreasing initial Si:N ratios during post-bloom is likely related to accumulation of organic matter, of which the amount of detritus was likely highest in low-copepod, low Si:N treatments. A switch in microbial food-web structure could have also played a role: as Zöllner et al. (2009) has shown, bacteria abundance can increase with increasing copepod numbers due to trophic cascade effects via ciliates and HNF.
4.2 Increasing nitrate or decreasing silicate – does it matter which component of the ratio changes?
Nutrient stoichiometry is defined by ratios among potentially limiting nutrients. While nutrient stoichiometry and resource ratio theory are well established ecological concepts, they do receive criticism, in particular as the importance of the absolute concentrations of nutrients is not accounted for (Brauer et al., 2012). For instance, Flynn (2010) wrote: “Ratios can be dangerous tools; they may be elevated because the denominator is low or the numerator is high”. Here we discuss the evidence from two experiments where we applied the same Si:N ratios while manipulating either silicon (Makareviciute-Fichtner et al., 2020) or nitrogen (this study) concentrations (Figure 8) and argue, that while absolute concentrations do play a role, the general trends of changing Si:N ratio on plankton community structure are similar when either silicon or nitrogen are manipulated.
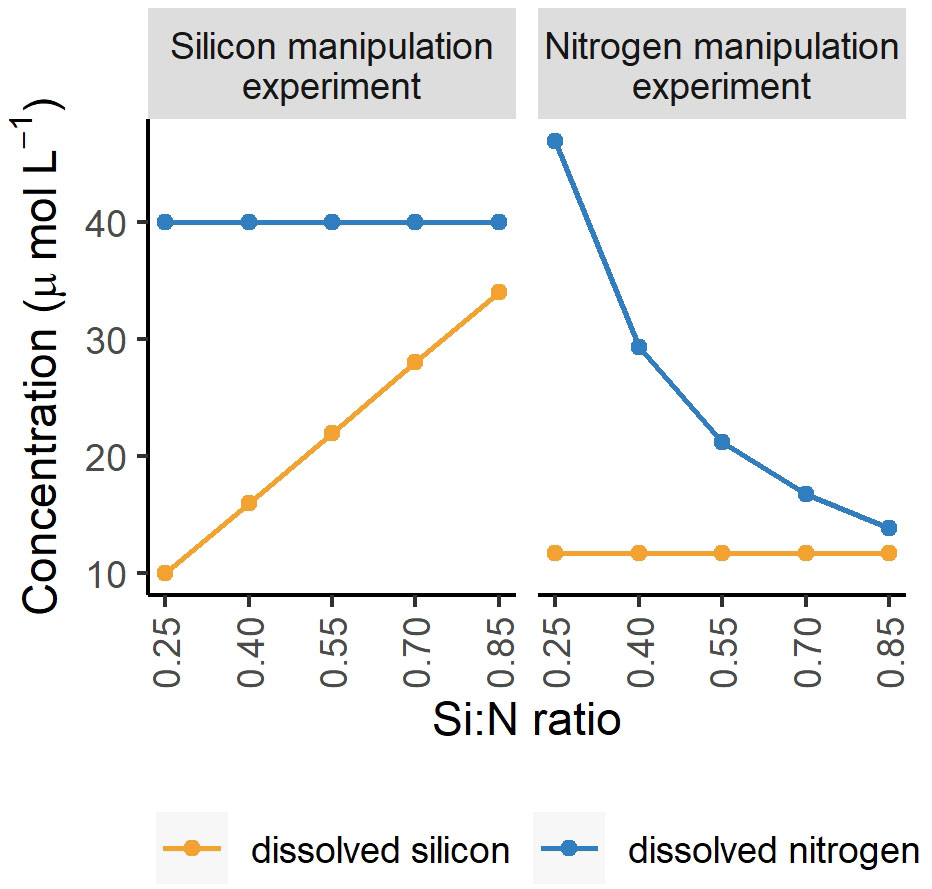
Figure 8 Experimental nutrient manipulations in two experiments to achieve the following Si:N ratios: 0.25, 0.4, 0.55, 0.7, 0.85. The nitrogen experiment refers to this study and the silicon experiment to Makareviciute-Fichtner et al. (2020).
In the current study, we manipulated nitrogen concentrations and showed that with increasing Si:N ratio, diatom proportion increases (Figure 9; Supplementary Figure S2). In an experiment where we manipulated silicate concentration to achieve the same Si:N ratios (Makareviciute-Fichtner et al., 2020), a similar increase of diatom proportion with increasing Si:N ratio could be observed (Figure 9). While experiments were conducted in two different years meaning that starting plankton communities, including the relative contribution of diatoms, were not identical, the slopes of diatom proportion over initial Si:N ratios were similar both during bloom and post-bloom periods (with an exception to the high copepod treatments during bloom period in the nitrogen manipulation experiment) (Figure 9). The increase of diatom proportion with increasing Si:N ratio treatments is, however, not linear: the decrease of diatom proportion with decreasing Si:N ratio is first slow or even non-significant, but when the ratios get lower than 0.4, diatom relative biomass drops strongly. For instance, during the post-bloom period, diatom relative carbon biomass was around 25% lower in the lowest Si:N ratio treatment of 0.25 compared to the remaining Si:N treatments. A similar trend has also been observed by Gilpin et al. (2004) who noted that experimental bags with N:Si of 4 experienced silicon exhaustion before nitrate which resulted in substantial silicon limitation. This suggests that Si:N ratio of 0.25 could be critical for sustaining diatoms within phytoplankton communities.
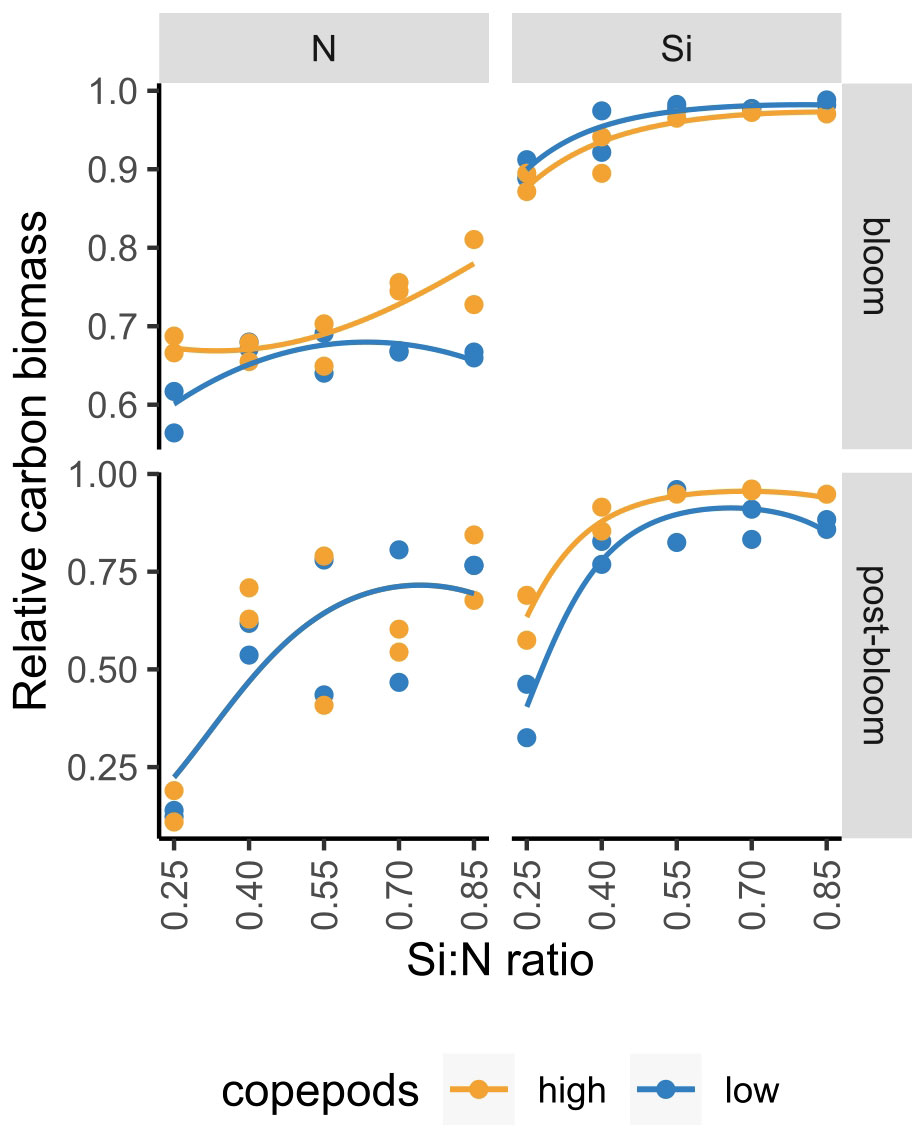
Figure 9 Diatom proportion in nitrogen (N) and silicon (Si) manipulation experiments during bloom and post-bloom periods. Figure is partially adapted from Makareviciute-Fichtner et al. (2020) (for silicon manipulation experiment).
Additionally, changing Si:N ratio affected not only diatoms but also the other major plankton groups (dinoflagellates, picoplankton, ciliates and bacteria) in the same direction both when silicon and nitrogen were manipulated (Table 2). Yet, as anticipated, the manipulation of N versus Si in Si:N ratios can have opposite effects on total phytoplankton biomass during bloom period (Table 2). Nitrogen is an essential component of the biomass of all phytoplankton, while silicate is essential only for diatoms, silicoflagellates and radiolarians. Therefore, nitrogen (and phosphorus) enrichment leads to higher biomass of phytoplankton blooms, which may include diatoms, as long as silicate is not limiting. However, after the phytoplankton bloom, initial nitrogen concentration seems to play a less important role and both diatom and total phytoplankton biomass increased with increasing initial Si:N ratios in both experiments (Table 2; Figure 4).
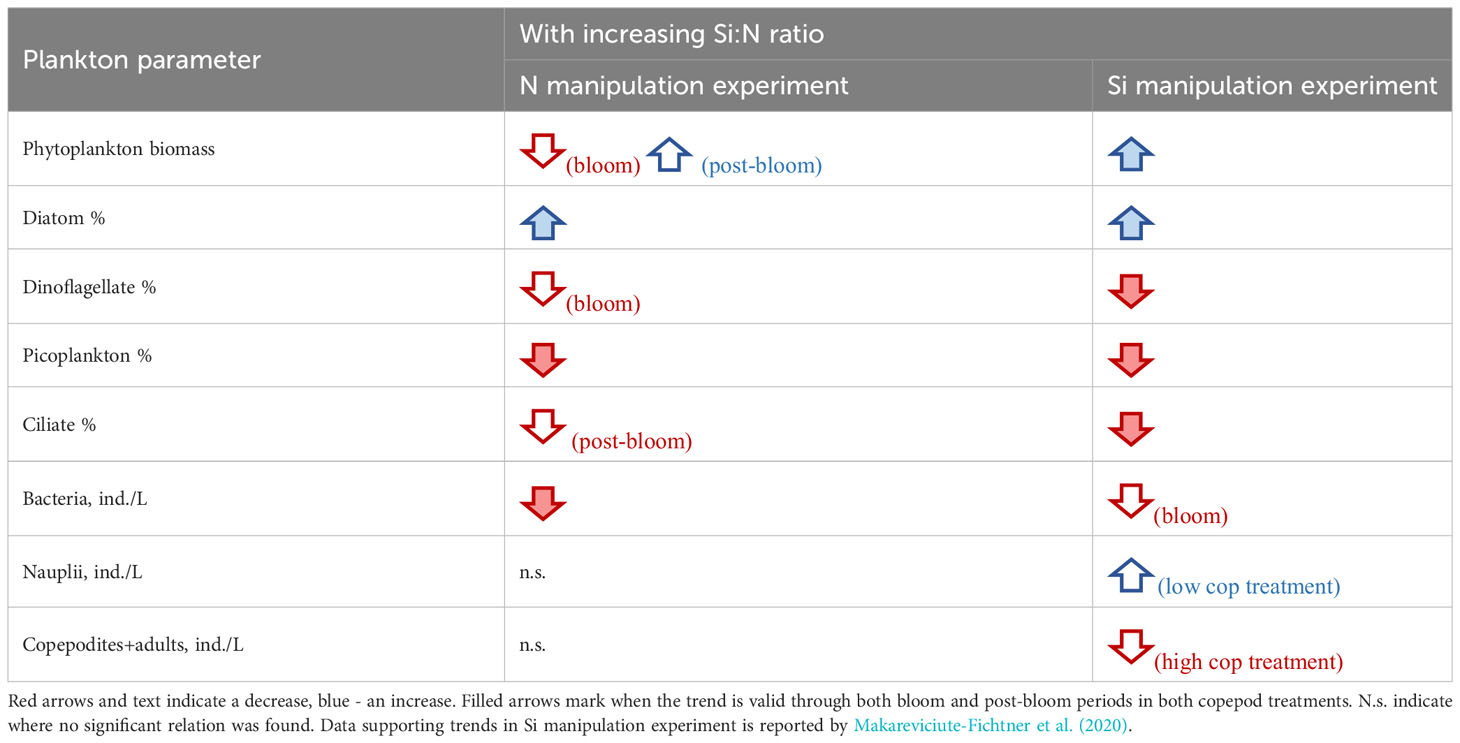
Table 2 Response of different plankton parameters to increasing Si:N ratio in an experiment where nitrogen or silicon concentrations were manipulated.
In this study, we used initial Si:N ratios to indicate the treatments and to discuss their effects on phytoplankton both during bloom and post-boom conditions. It is important to acknowledge that actual nutrient concentrations and ratios diverged from these initial conditions as the experiment progressed, as depicted in Figure 1. It is known that there is a time lag between stimuli and subsequent algal growth and changes in their composition (Duarte, 1990; Olden, 2000). Initial conditions had pronounced effects on phytoplankton biomass and composition throughout the experiment duration – for over 20 days. These likely include both direct and indirect effects of nutrient manipulation (i.e. nutrient storage and recycling) and highlights the importance to consider not only the immediate nutrient conditions but also possible previous changes, in particular in the context of phytoplankton blooms.
4.3 The role of phosphorus
Historically it was considered that phosphorus is the limiting nutrient in freshwater systems while nitrogen is more important in marine waters (Smith, 1984). Currently, we know that N and P can often co-limit phytoplankton growth in the ocean and in some marine areas phosphorus may be more limiting that nitrogen (Elser et al., 2007; Howarth et al., 2021). The effects of changes in Si:N ratio in our study are simultaneously the effects of changes in Si:P ratio as N:P were supplied in 16:1 ratio in all treatments. Further research is necessary to unravel the impacts of changes in Si:P ratios compared to changes in Si:N ratios and their impact in aquatic systems.
5 Conclusions
A decline in relative silicate availability due to nitrogen and phosphorus enrichment resulted in an increase of phytoplankton bloom biomass, but a decrease of the proportion of diatoms. High copepod abundance caused an earlier silicon limitation, likely due to enhanced diatom silicification under higher grazing pressure. Non-silicifying plankton groups, such as dinoflagellates, picoplankton, ciliates and bacteria benefited from nitrogen and phosphorus enrichment and lowered Si:N and Si:P ratios.
Si:N ratios can decline either due to an increase in nitrogen availability or a decline in silicon concentrations (or both). Here, we show that while nitrogen and silicon manipulation can have opposite effects on phytoplankton bloom biomass, plankton community composition responds to the decline in Si:N ratios in a comparable way no matter which of the nutrients is changing.
Data availability statement
The datasets presented in this study can be found in online repositories. The names of the repository/repositories and accession number(s) can be found below: https://doi.pangaea.de/10.1594/PANGAEA.961150, https://doi.pangaea.de/10.1594/PANGAEA.961149.
Author contributions
KM: Conceptualization, Funding acquisition, Visualization, Writing – original draft, Writing – review & editing. BM: Conceptualization, Funding acquisition, Resources, Supervision, Writing – review & editing. HL: Conceptualization, Methodology, Supervision, Writing – review & editing. US: Conceptualization, Funding acquisition, Methodology, Resources, Supervision, Writing – review & editing.
Funding
The author(s) declare financial support was received for the research, authorship, and/or publication of this article. This work was supported by a PhD fellowship to K.M.F. through the Helmholtz Research School on Ocean System Science and Technology (www.hosst.org) and Inge-Lehmann Fond at GEOMAR Helmholtz Centre for Ocean Research Kiel (VH-KO-601) as well as Gordon and Betty Moore Foundation (GBMF3788 to A.Worden).
Acknowledgments
We would like to thank Thomas Hansen, Cordula Meyer and Bente Gardeler for their technical assistance and nutrient analysis. Special thanks to Barbara Sa, Emily Chen, Stephanie Ismar and the students in the 2017 summer practical course for their help in setting up and carrying out the experiment.
Conflict of interest
The authors declare that the research was conducted in the absence of any commercial or financial relationships that could be construed as a potential conflict of interest.
Publisher’s note
All claims expressed in this article are solely those of the authors and do not necessarily represent those of their affiliated organizations, or those of the publisher, the editors and the reviewers. Any product that may be evaluated in this article, or claim that may be made by its manufacturer, is not guaranteed or endorsed by the publisher.
Supplementary material
The Supplementary Material for this article can be found online at: https://www.frontiersin.org/articles/10.3389/fmars.2024.1289768/full#supplementary-material
References
Berggreen U., Hansen B., Kiørboe T. (1988). Food size spectra, ingestion and growth of the copepodAcartia tonsa during development: Implications for determination of copepod production. Mar. Biol. 99, 341–352. doi: 10.1007/BF02112126
Brauer V. S., Stomp M., Huisman J. (2012). The nutrient-load hypothesis: patterns of resource limitation and community structure driven by competition for nutrients and light. Am. Nat. 179, 721–740. doi: 10.1086/665650
Capone D. G., Bronk D. A., Mulholland M. R., Carpenter E. J. (2008). Nitrogen in the marine environment (Amsterdam: Elsevier).
Carotenuto Y., Ianora A., Buttino I., Romano G., Miralto A. (2002). Is postembryonic development in the copepod Temora stylifera negatively affected by diatom diets? J. Exp. Mar. Biol. Ecol. 276, 49–66. doi: 10.1016/S0022-0981(02)00237-X
Conant R. T., Berdanier A. B., Grace P. R. (2013). Patterns and trends in nitrogen use and nitrogen recovery efficiency in world agriculture. Global Biogeochem. Cycles 27, 558–566. doi: 10.1002/gbc.20053
Downing J. A., Cherrier C. T., Fulweiler R. W. (2016). Low ratios of silica to dissolved nitrogen supplied to rivers arise from agriculture not reservoirs. Ecol. Lett. 19, 1414–1418. doi: 10.1111/ele.12689
Duarte C. M. (1990). Time lags in algal growth: generality, causes and consequences. J. Plankton Res. 12, 873–883. doi: 10.1093/plankt/12.4.873
Egge J. K., Aksnes D. L. (1992). Silicate as regulating nutrient in phytoplankton competition. Mar. Ecol. Prog. Ser. 83.2, 281–289. doi: 10.3354/meps083281
Elser J. J., Bracken M. E., Cleland E. E., Gruner D. S., Harpole W. S., Hillebrand H., et al. (2007). Global analysis of nitrogen and phosphorus limitation of primary producers in freshwater, marine and terrestrial ecosystems. Ecol. Lett. 10, 1135–1142. doi: 10.1111/j.1461-0248.2007.01113.x
Filippelli G. M. (2008). The global phosphorus cycle: past, present, and future. Elements 4, 89–95. doi: 10.2113/GSELEMENTS.4.2.89
Flynn K. J. (2010). Do external resource ratios matter?: Implications for modelling eutrophication events and controlling harmful algal blooms. J. Mar. Syst. 83, 170–180. doi: 10.1016/j.jmarsys.2010.04.007
Fowler D., Coyle M., Skiba U., Sutton M. A., Cape J. N., Reis S., et al. (2013). The global nitrogen cycle in the twenty-first century. Philos. Trans. R. Soc. B: Biol. Sci. 368, 20130164. doi: 10.1098/rstb.2013.0164
Friedl G., Wüest A. (2002). Disrupting biogeochemical cycles-Consequences of damming. Aquat. Sci. 64, 55–65. doi: 10.1007/s00027-002-8054-0
Gilpin L. C., Davidson K., Roberts E. (2004). The influence of changes in nitrogen: silicon ratios on diatom growth dynamics. J. Sea Res. 51, 21–35. doi: 10.1016/j.seares.2003.05.005
Granéli E., Carlsson P., Turner J. T., Tester P. A., Béchemin C., Dawson R., et al. (1999). Effects of N:P:Si ratios and zooplankton grazing on phytoplankton communities in the northern Adriatic Sea. I. Nutrients, phytoplankton biomass, and polysaccharide production. Aquat. Microb. Ecol. 18, 37–54. doi: 10.3354/ame018037
Grønning J., Kiørboe T. (2020). Diatom defence: Grazer induction and cost of shell-thickening. Funct. Ecol. 34, 1790–1801. doi: 10.1111/1365-2435.13635
Hansen H. P., Koroleff F. (1999). “Determination of nutrients,” in Methods of seawater analysis. Eds. Grasshoff K., Kremling K., Ehrhardt M. (Weinheim: Wiley), 159–228.
Hillebrand H., Dürselen C. D., Kirschtel D., Pollingher U., Zohary T. (1999). Biovolume calculation for pelagic and benthic microalgae. J. Phycol. 35, 403–424. doi: 10.1046/j.1529-8817.1999.3520403.x
Howarth R. W., Chan F., Swaney D. P., Marino R. M., Hayn M. (2021). Role of external inputs of nutrients to aquatic ecosystems in determining prevalence of nitrogen vs. phosphorus limitation of net primary productivity. Biogeochemistry 154, 293–306. doi: 10.1007/s10533-021-00765-z
Hu H., Zhang J., Chen W. (2011). Competition of bloom-forming marine phytoplankton at low nutrient concentrations. J. Environ. Sci. 23, 656–663. doi: 10.1016/S1001-0742(10)60459-7
Ianora A., Poulet S. A., Miralto A. (2003). The effects of diatoms on copepod reproduction: a review. Phycologia 42, 351–363. doi: 10.2216/i0031-8884-42-4-351.1
Jónasdóttir S. H. (2019). Fatty acid profiles and production in marine phytoplankton. Mar. Drugs 17, 151. doi: 10.3390/md17030151
Krause J. W., Schulz I. K., Rowe K. A., Dobbins W., Winding M. H., Sejr M. K., et al. (2019). Silicic acid limitation drives bloom termination and potential carbon sequestration in an Arctic bloom. Sci. Rep. 9 (1), 8149. doi: 10.1038/s41598-019-44587-4
Kuosa H., Autio R., Kuuppo P., Setälä O., Tanskanen S. (1997). Nitrogen, silicon and zooplankton controlling the Baltic spring bloom: an experimental study. Estuar. Coast. Shelf Sci. 45, 813–821. doi: 10.1006/ecss.1997.0241
Leising A. W., Pierson J. J., Halsband-Lenk C., Horner R., Postel J. (2005). Copepod grazing during spring blooms: does Calanus pacificus avoid harmful diatoms? Prog. Oceanogr. 67, 384–405. doi: 10.1016/j.pocean.2005.09.008
Liu H., Chen M., Zhu F., Harrison P. J. (2016). Effect of diatom silica content on copepod grazing, growth and reproduction. Front. Mar. Sci. 3, 89. doi: 10.3389/fmars.2016.00089
Makareviciute-Fichtner K., Matthiessen B., Lotze H. K., Sommer U. (2020). Decrease in diatom dominance at lower Si:N ratios alters plankton food webs. J. Plankton Res. 42, 411–424. doi: 10.1093/plankt/fbaa032
Mann K. (1993). Physical oceanography, food chains, and fish stocks: a review. ICES J. Mar. Sci. 50, 105–119. doi: 10.1006/jmsc.1993.1013
Menden-Deuer S., Lessard E. J. (2000). Carbon to volume relationships for dinoflagellates, diatoms, and other protist plankton. Limnol. Oceanogr. 45, 569–579. doi: 10.4319/lo.2000.45.3.0569
Moriceau B., Iversen M. H., Gallinari M., Evertsen A. J. O., Le Goff M., Beker B., et al. (2018). Copepods boost the production but reduce the carbon export efficiency by diatoms. Front. Mar. Sci. 5, 82. doi: 10.3389/fmars.2018.00082
Motwani N. H., Gorokhova E. (2013). Mesozooplankton grazing on picocyanobacteria in the Baltic Sea as inferred from molecular diet analysis. PloS One 8, e79230. doi: 10.1371/journal.pone.0079230
Nixon S., Fulweiler R. (2009). Nutrient pollution, eutrophication, and the degradation of coastal marine ecosystems. Global loss Coast. habit.: rates causes consequences, 23–58.
Olden J. D. (2000). An artificial neural network approach for studying phytoplankton succession. Hydrobiologia 436 (1–3), 131–143.
Olson M. B., Lessard E. J., Wong C. H. J., Bernhardt M. J. (2006). Copepod feeding selectivity on microplankton, including the toxigenic diatoms Pseudo-nitzschia spp., in the coastal Pacific Northwest. Mar. Ecol. Prog. Ser. 326, 207–220. doi: 10.3354/meps326207
Pancic M., Torres R. R., Almeda R., Kiorboe T. (2019). Silicified cell walls as a defensive trait in diatoms. Proc. R. Soc. B 286, 20190184. doi: 10.1098/rspb.2019.0184
Phlips E. J., Badylak S., Christman M. C., Lasi M. A. (2010). Climatic trends and temporal patterns of phytoplankton composition, abundance, and succession in the Indian River Lagoon, Florida, USA. Estuaries Coasts 33, 498–512. doi: 10.1007/s12237-009-9166-8
R Core Team (2019). R: A language and environment for statistical computing (Vienna, Austria: R Foundation for Statistical Computing). https://www.R-project.org/.
Redfield A. C. (1934). On the proportions of organic derivatives in sea water and their relation to the composition of plankton (Liverpool: University press of Liverpool).
Schöllhorn E., Granéli E. (1996). Influence of different nitrogen to silica ratios and artificial mixing on the structure of a summer phytoplankton community from the Swedish west coast (gullmar fjord). J. Sea Res. 35, 159–167. doi: 10.1016/S1385-1101(96)90743-1
Sherr E. B., Sherr B. F. (2002). Significance of predation by protists in aquatic microbial food webs. Antonie Van Leeuwenhoek 81, 293–308. doi: 10.1023/A:1020591307260
Sinha E., Michalak A. M., Balaji V. (2017). Eutrophication will increase during the 21st century as a result of precipitation changes. Science 357, 405–408. doi: 10.1126/science.aan2409
Smith S. V. (1984). Phosphorus versus nitrogen limitation in the marine environment, Limnol. Oceanogr 29 (6), 1149–1160. doi: 10.4319/lo.1984.29.6.1149
Smith V. H. (2006). Responses of estuarine and coastal marine phytoplankton to nitrogen and phosphorus enrichment. Limnol. Oceanogr. 51, 377–384. doi: 10.4319/lo.2006.51.1_part_2.0377
Sommer U. (1998). From algal competition to animal production: Enhanced ecological efficiency of Brachionus plicatilis with a mixed diet. Limnol. Oceanogr. 43, 1393–1396. doi: 10.4319/lo.1998.43.6.1393
Sommer U. (2009). Copepod growth and diatoms: insensitivity of Acartia tonsa to the composition of semi-natural plankton mixtures manipulated by silicon:nitrogen ratios in mesocosms. Oecologia 159, 207–215. doi: 10.1007/s00442-008-1193-9
Sommer U., Hansen T., Blum O., Holzner N., Vadstein O., Stibor H. (2005). Copepod and microzooplankton grazing in mesocosms fertilised with different Si:N ratios: no overlap between food spectra and Si:N influence on zooplankton trophic level. Oecologia 142, 274–283. doi: 10.1007/s00442-004-1708-y
Sommer U., Lengfellner K., Lewandowska A. (2012). Experimental induction of a coastal spring bloom early in the year by intermittent high-light episodes. Mar. Ecol. Prog. Ser. 446, 61–71. doi: 10.3354/meps09486
Stevens C. J. (2019). Nitrogen in the environment. Science 363, 578–580. doi: 10.1126/science.aav8215
Sundt-Hansen L. E., Olsen Y., Stibor H., Heldal M., Vadstein O. (2006). Trophic cascades mediated by copepods, not nutrient supply rate, determine the development of picocyanobacteria. Aquat. Microb. Ecol. 45, 207–218. doi: 10.3354/ame045207
Taucher J., Bach L. T., Prowe A. E. F., Boxhammer T., Kvale K., Riebesell U. (2022). Enhanced silica export in a future ocean triggers global diatom decline. Nature 605, 696–700. doi: 10.1038/s41586-022-04687-0
Tilman D. (1982). Resource competition and community structure (Princeton, N.J.: Princeton University Press). doi: 10.1515/9780691209654
Treguer P. J., de la Rocha C. L. (2013). The world ocean silica cycle. Ann. Rev. Mar. Sci. 5, 477–501. doi: 10.1146/annurev-marine-121211-172346
Utermöhl H. (1958). Zur Vervollkommnung der quantitativen Phytoplankton-Methodik: Mit 1 Tabelle und 15 abbildungen im Text und auf 1 Tafel. Internationale Vereinigung für theoretische und angewandte Limnol.: Mitt. 9, 1–38.
Welschmeyer N. A. (1994). Fluorometric analysis of chlorophyll a in the presence of chlorophyll b and pheopigments. Limnol. Oceanogr. 39, 1985–1992. doi: 10.4319/lo.1994.39.8.1985
Wickham H. (2016). ggplot2: Elegant Graphics for Data Analysis (New York: Springer-Verlag). doi: 10.1007/978-3-319-24277-4
Wickham H. (2017). reshape2: flexibly reshape data: a reboot of the reshape package (R package version 1).
Wilson S., Steinberg D. (2010). Autotrophic picoplankton in mesozooplankton guts: evidence of aggregate feeding in the mesopelagic zone and export of small phytoplankton. Mar. Ecol. Prog. Ser. 412, 11–27. doi: 10.3354/meps08648
Zhao Y., Dong Y., Li H., Lin S., Huang L., Xiao T., et al. (2020). Grazing by microzooplankton and copepods on the microbial food web in spring in the southern Yellow Sea, China. Mar. Life Sci. Technol. 2, 442–455. doi: 10.1007/s42995-020-00047-x
Keywords: plankton, Si:N, nutrient ratios, diatoms, copepods, stoichiometry
Citation: Makareviciute-Fichtner K, Matthiessen B, Lotze HK and Sommer U (2024) Nutrient enrichment alters phytoplankton biomass and composition via silicon limitation. Front. Mar. Sci. 11:1289768. doi: 10.3389/fmars.2024.1289768
Received: 06 September 2023; Accepted: 27 February 2024;
Published: 12 March 2024.
Edited by:
Cosimo Solidoro, National Institute of Oceanography and Applied Geophysics, ItalyReviewed by:
Lasse Mork Olsen, University of Bergen, NorwayDedmer B. Van de Waal, Netherlands Institute of Ecology (NIOO-KNAW), Netherlands
Copyright © 2024 Makareviciute-Fichtner, Matthiessen, Lotze and Sommer. This is an open-access article distributed under the terms of the Creative Commons Attribution License (CC BY). The use, distribution or reproduction in other forums is permitted, provided the original author(s) and the copyright owner(s) are credited and that the original publication in this journal is cited, in accordance with accepted academic practice. No use, distribution or reproduction is permitted which does not comply with these terms.
*Correspondence: Kriste Makareviciute-Fichtner, kmakareviciute@geomar.de