Rare earth elements distribution in the Gulf of Cádiz (SW Spain): geogenic vs. anthropogenic influence
- 1Instituto de Investigacións Mariñas (IIM-CSIC), Vigo, Spain
- 2Centro Oceanográfico de Vigo (IEO-CSIC), Vigo, Spain
- 3Instituto de Ciencias Marinas de Andalucía (ICMAN-CSIC), Puerto Real, Cádiz, Spain
- 4Centro Oceanográfico de Cádiz (IEO-CSIC), Cádiz, Spain
There is a current interest in the study of the geochemical behavior of the rare earth elements (REE) in aquatic systems since their identification as potential microcontaminants in natural waters. In this context, here we report the concentrations and patterns of dissolved REE (dREE) in the waters of the Gulf of Cádiz (GoC) and its major estuaries (Guadiana, Tinto-Odiel and Guadalquivir). Contamination in this area by metals has been extensively reported, linked to acid-mine inputs resulting from the mineralization of the Iberian Pyrite Belt, discharged mainly to the Tinto-Odiel river. However, the impact of these inputs on the concentrations and distribution of the dREE in the coastal waters of the GoC has not been addressed yet. Accordingly, elevated concentrations of dREE were obtained in the Tinto-Odiel estuary compared to the Guadiana and Guadalquivir, reflecting the impact of acid-mine drainage on this system. Only a significant fraction of anthropogenic gadolium (Gd) was observed in the Guadalquivir estuary, as a result of the input from major urban areas, where anthropogenic Gd fractions higher than 70% over the total dissolved pool were obtained for the freshwater end-member. Regarding the surface waters of the GoC, typical NE Atlantic distribution patterns of dREEs were obtained, but modified by two different end-members within this region. Accordingly, and despite the low water discharge of the Tinto-Odiel river, its elevated concentrations lead to an increase in the concentrations of dREEs in the nearshore waters of the GoC and producing a distinctive signature as observed for the MREE anomalies and HREE/LREE ratios. At the easternmost stations, close to the Strait of Gibraltar, these signals are also impacted by the inflow of Mediterranean waters and also by the discharge of the Guadalquivir river.
Introduction
Current interest on the geochemical behavior of the rare earth elements (REE) from an environmental perspective has arisen since their identification as potential microcontaminants in aquatic systems (Kulaksız and Bau, 2007; 2011). The REE are a group of seventeen chemical elements consisting of yttrium and the 15 lanthanide elements (lanthanum, cerium, praseodymium, neodymium, promethium, samarium, europium, gadolinium, terbium, dysprosium, holmium, erbium, thulium, ytterbium, and lutetium); scandium is also generally classified as a rare earth element as it is commonly found in REE deposits (King, 2023). They are a group of chemically similar metallic elements with a gradual decrease in their ionic radii with increasing atomic number. Based on their separability and ionic radii, rare earth elements can be subdivided into light REE (LREE: La, Ce, Pr, Nd, Sm), medium REE (MREE: Eu, Gd, Tb, Dy), and heavy REE (HREE: Ho, Er, Tm, Yb, Lu). All REE occur in the trivalent state in nature, with the exception of Eu and Ce, which can also display +2 and +4 valences, respectively. REE are geochemically fractionated in the environment in a subtle and predictable manner and are thus suitable for their application as tracers of natural processes (Andrade et al., 2020; Neira et al., 2022). The use of REEs in current high-tech applications – e.g. computer memories, rechargeable batteries (including those in electric vehicles), cell phones, catalytic converters, magnets or fluorescent lighting, among others – has led to a marked increase in their production in the last decade. Accordingly, whereas during the period 2005-2017 the production remained relatively constant at around 120,000 metric tons per year (as rare earth oxide equivalent), it has continuously increased in the recent years reaching a production of 300,000 metric tons per year in 2022 (King, 2023). Despite of the anthropogenic uses of the REE, a widespread contamination has only been observed for gadolinium (Gd). The observation of these Gd anthropogenic anomalies has been demonstrated to be linked to the use of this metal in contrasting agents for magnetic resonance imaging (MRI), and are especially relevant in aquatic systems receiving effluents from densely populated areas with developed medical and healthcare systems (Kulaksız and Bau, 2007; 2011). These anthropogenic Gd anomalies can then be used as a pseudo-natural tracer of water discharge from wastewater treatment plants since the Gd organic complex used for medical purposes is not removed by common sewage treatment technologies (Andrade et al., 2020).
Elevated dissolved metal concentrations have been historically reported in estuarine and coastal waters of the Gulf of Cádiz (GoC; South Spain). These elevated concentrations have been claimed to be the result of the mineralization of the Iberian pyrite belt, which is one of the most important sulphide deposits in the World, and whose acidic and metal rich drainage discharge is transported by the Tinto-Odiel river. Accordingly, highest dissolved metal concentrations in the GoC are generally found at the mouth of the Tinto-Odiel river (Elbaz-Poulichet et al., 2001; González-Ortegón et al., 2019). On the other hand, the Guadalquivir river has also been identified as another significant source of metals into GoC; despite having typical lower concentrations than the Tinto-Odiel, its greater discharge (>2000 hm3 year−1 vs. 100–473 hm3 year−1 of the Tinto-Odiel; Elbaz-Poulichet et al., 2001) results in a higher flux of certain metals (e.g., Cu, Ni; González-Ortegón et al., 2019) into the continental shelf than that derived from the Tinto-Odiel. Both main sources result in the presence of a metal-enriched water mass that seasonally impacts the Atlantic inflow through the Strait of Gibraltar (Elbaz-Poulichet et al., 2001).
However, there is still little information to date on the impact of these inputs on the geochemistry of dissolved Rare Earth Elements (dREEs) in the GoC. Available studies are restricted to the Tinto-Odiel river and its estuary, where concentrations exceeding 10 mg L-1 are found in the upper, acidic (pH<2) reaches of the river decreasing downstream as the water pH increases and upon mixing within the estuary (Lecomte et al., 2017; Olías et al., 2018; Cánovas et al., 2020). Concentrations and patterns of dREEs in the other major freshwater inputs to this coastal area, the Guadiana and Guadalquivir estuaries, are unknown at present, as well as for the coastal waters of the GoC. In this study we present the results of the distribution of dREEs in the outer part the major estuarine areas of the GoC (Guadiana, Tinto-Odiel and Guadalquivir) as well as in the surface coastal waters of the GoC, with the aim of (i) provide an assessment of the concentrations and patterns of dREEs in the estuarine areas of the GoC, (ii) evaluate the geogenic and anthropogenic impacts on their distributions, and (iii) determine the major sources controlling the distribution of dREEs in the GoC coastal waters.
Materials and methods
Study area
The Gulf of Cádiz (GoC) in southwest Spain is a partially enclosed area where the movement of water is primarily influenced by interactions between different environmental basins: the Mediterranean and Atlantic basins, as well as the coastal system (Sánchez-Leal et al., 2017). The GoC receives freshwater input from three major rivers: the Guadiana, Tinto-Odiel, and Guadalquivir (Figure 1). In the semiarid environment surrounding these rivers, the flow of freshwater into the Guadiana, Tinto-Odiel, and Guadalquivir estuaries is completely regulated by dams. Both the Guadiana and Guadalquivir rivers have a higher freshwater input (>2000 hm3 year−1) compared to the Tinto-Odiel estuary (100–473 hm3 year−1) (Elbaz-Poulichet et al., 2001).
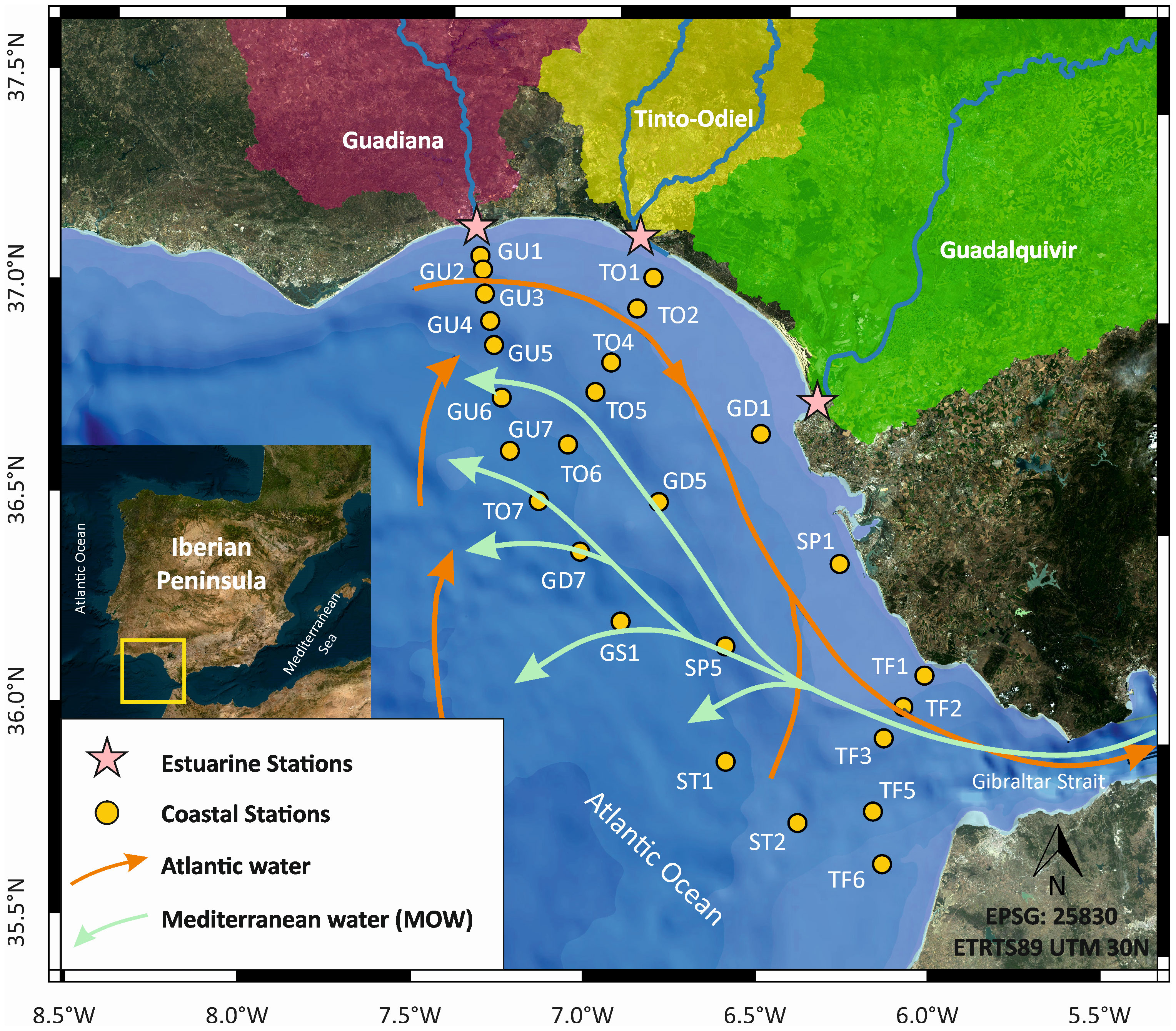
Figure 1 Map showing the stations in the continental shelf of the Gulf of Cádiz and the fixed stations in the estuaries of the Guadiana, Tinto-Odiel and Gudalquivir rivers. Also indicated the dominant surface currents in the area.
The estuary formed by the confluence of the Odiel and Tinto rivers stretches along the southwestern coast of the Guadalquivir sedimentary basin. Historically, the Tinto-Odiel river drained the world’s largest sulphide deposit that have been mined for over 4500 years, making it one of the oldest mining regions globally (Olías and Nieto, 2015). Due to natural changes in these sulphide deposits and mining activities, the Tinto-Odiel river has become severely contaminated, with extremely low pH levels (<2) in their upper reaches and extremely elevated concentrations of metals (Grande et al., 2000). Previous studies conducted in the Tinto-Odiel estuary found that it serves as a source of certain metals (such as Cu, Zn, and Cd) to the GoC (Elbaz-Poulichet et al., 2001; Beckers et al., 2007). The high levels of dissolved metals and metalloids, coupled with low pH values indicates that this river can be a significant source on the influx of metals into the Mediterranean through the Strait of Gibraltar (Elbaz-Poulichet et al., 2001).
The Guadiana estuary is characterized by its rocky shoreline (Garel and Ferreira, 2013), and contamination from acidic mine drainage enters the river system through leachate seepage from a small number of active mines (Delgado et al., 2009) with additional inputs of contaminants in the estuarine area derived from port activities in the estuarine area, tourism developments and the irrigation of intensive crops in the lands adjacent to the estuary introduce significant quantities of contaminants and nutrients (González-Ortegón et al., 2019). The Guadalquivir estuary exhibits a high sediment load, resulting in increased water turbidity (González-Ortegón et al., 2015). The Guadalquivir river basin, with its intensive agriculture in former marshlands and large population centers, experiences a significant influx of nutrients into its waters (González-Ortegón and Drake, 2012). Despite the historic presence of mines in the Guadalquivir basin, metal contamination has not been detected in its estuary, even after the Aznalcóllar toxic spill into two of its lower tributaries in 1998 (Tornero et al., 2014).
Sampling
A detailed description of the sampling protocols can be found in González-Ortegón et al. (2019). Briefly, estuarine samples were collected at a fixed station, following a 24-h sampling cycle in order to evaluate the variation of water composition during a tidal cycle, on 24th–25th February 2016 in the Guadalquivir Estuary (lat. 36.7923 N; long. −6.35623 W) and Tinto-Odiel Estuary (lat. 37.13271 N; long. −6.8426 W) and 17th March 2016 in the Guadiana Estuary (lat. 37.1989 N, long −7.4095 W) for the wet season. For the dry season, samples were collected during 5th-9th July 2016. A peristaltic pump with acid-cleaned Teflon tubing coupled to a C-flex tubing at the pump head, filtered in-line through an acid-cleaned polypropylene cartridge filter (0.22 μm; MSI, Calyx®) was used. Coastal surface water samples in the Gulf of Cádiz (GoC) were collected during 3rd–8th March 2016 using a Teflon towfish system deployed from the research vessel. Seawater was pumped into a class-100 HEPA laminar flow hood through acid-cleaned Teflon tubing coupled to C-flex tubing using a Teflon diaphragm pump, filtered online (0.22 μm). For both estuarine and coastal locations, filtered samples were collected in 0.5 L LDPE bottles and acidified at the onshore lab to pH<2 with ultrapure grade HCl (Merck) in a class-100 HEPA laminar and stored double-bagged (ziplock) pending analysis.
Analysis of dissolved REEs
The determination of dissolved REEs was attained by the use of a seaFAST (Elemental Scientific) preconcentration module coupled on-line to an ICP-MS (7900, Agilent) (Romero-Freire et al., 2020). In order to produce narrower peaks and lower the analysis time, a 2 cm-long mini-column of 27 μL of volume (Global FIA) was used instead of the original default seaFAST 200 µL column. The mini-column was packed with a chelate resin modified with carboxymethylated polyethylenimine (Fujifilm Wako, Presep® PolyChelate) (Cobelo-García et al., 2021), which was used as the solid phase material for REEs preconcentration in the seaFAST module. The on-line preconcentration method consisted in a 10-mL sample loop; the loaded acidified sample was buffered using 2M ammonium acetate (pH 6.0) and then passed through the column. After preconcentration, the column was rinsed with the buffer solution in order to remove remaining salts and then eluted on-line using 1.5M HNO3, obtaining a chromatographic signal for the target masses. The following isotopes were used for REEs determination: 139La, 140Ce, 141Pr, 146Nd, 147Sm, 153Eu, 157Gd, 159Tb, 163Dy, 165Ho, 166Er, 169Tm, 172Yb, and 175Lu. There was no need to correct for the typical interference of Ba (137Ba16O+, 136Ba16O1H+) on 153Eu, as alkaline earth elements are not retained in the column at the working pH. Potential interferences on certain REEs due to the formation of oxides of lighter REEs during the ICP-MS determination was assessed and accounted for – when necessary – following a similar approach of that given by Babechuk et al. (2020). Accordingly, typical interferences were observed for 157Gd (141Pr16O+, 140Ce16O1H+), 159Tb (143Nd16O+, 142Ce16O1H+), 163Dy (147Sm16O+, 146Nd16O1H+), 165Ho (149Sm16O+, 148Nd16O1H+), 166Er (150Nd16O+, 150Sm16O+), 169Tm (152Sm16O1H+, 153Eu16O+), 172Yb (156Gd16O+), 175Lu (159Tb16O+). In general, interferences accounted from ~0.1% (163Dy, 165Ho) to ~3% (157Gd). All the samples were analyzed in duplicate, with a relative standard deviation for both determinations lower than 4%; average concentrations are presented. Detection limits, expressed as three times the standard deviation of the blanks, ranged from 0.02 to 0.001 ng L-1 depending on the given analyte, making the methodology appropriate for the determination of REEs in natural waters. The reference materials CASS-4 and CASS-6 (Coastal Sea Water, National Research Council, Canada) were analyzed following the same analytical procedures, obtaining values in agreement with previously reported concentrations (Supplementary Tables S1, S2, Supporting Information).
Normalization of REEs concentrations and calculation of Ce, Eu and Gd anomalies
REE concentrations were normalized to European Shale (EUS) as reported by Bau et al. (2018). Calculation of anomalies based on the normalized concentrations, in order to gain insight on fractionation processes (e.g. Ce, Eu) or anthropogenic inputs (e.g. Gd), followed the equations (Lawrence et al., 2006; Rétif et al., 2023):
where La*, Ce*, Eu* and Gd* represent the respective anomalies, and the subscript N the normalized concentrations.
Results and discussion
Concentrations and patterns of dissolved REEs in the Guadiana, Tinto-Odiel and Guadalquivir estuaries
Dissolved REEs concentrations were higher in the sampling campaign conducted in the wet season, especially for the Tinto-Odiel Estuary (Table 1) where a 4-fold enrichment was observed compared with the dry season. This estuary also contains the highest concentrations of REEs compared to the Guadiana and Guadalquivir estuaries, as expected for the results of other dissolved trace metals in these systems (e.g. González-Ortegón et al., 2019). The Tinto-Odiel river flow through different materials of the Iberian Pyrite Belt (IPB) and is greatly impacted by its metal-bearing bedrock and historical mining activities, explaining their elevated dissolved REEs concentrations observed in its estuary; accordingly, concentrations above 106 ng/L (Lecomte et al., 2017; Olías et al., 2018; Ruiz Cánovas et al., 2021) have been reported in the upper reaches of the watercourse where pHs around or below 2 are commonly found. The Guadiana river is subject, although to a lesser extent, to the impact of the IBP, but the industrial, urban and agricultural activities in the watershed appear to have a greater influence on the levels of trace metals within its estuary (González-Ortegón et al., 2019). The 2-fold higher concentrations obtained for dissolved REEs in the Guadiana estuary compared to the Guadalquivir (Table 1) – which is not impacted by the IBP and only subject to urban and anthropogenic activities – suggests that for REEs the IBP may still be responsible for its higher content in the Guadiana.
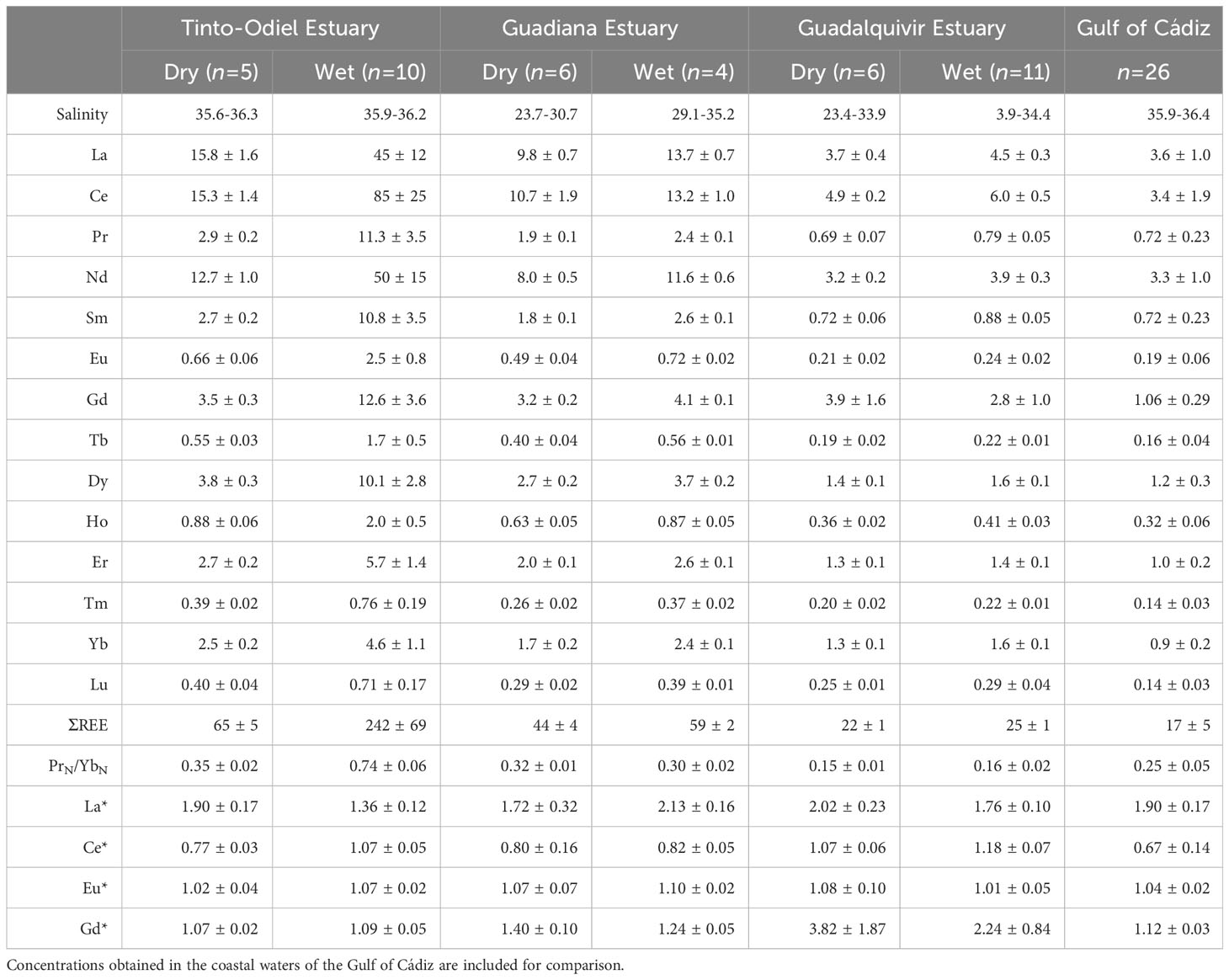
Table 1 Average dissolved REE concentrations (ng L-1) in the Tinto-Odiel, Guadiana and Guadalquivir estuaries for sampling campaigns conducted in the dry and wet season.
With the exception of the Tinto-Odiel estuary in the sampling carried out in the dry season, the normalized patterns (Figure 2) show a typical increase from LREE to HREE as typical for estuarine and coastal waters (e.g. Andrade et al., 2020). Highest LREE/HREE fractionation, as indicated by the PrN/YbN ratios (Table 1), was found in the Guadalquivir estuary with values even lower than those observed in the GoC coastal waters. The dREE pattern in the Tinto-Odiel estuary in the wet season (Figure 2) was unexpected for a water mass with salinities typical for coastal seawater, and was similar to those reported for upstream waters more influenced by the inputs of the acid mine drainage (AMD), where a bell-shaped distribution with and enrichment in the MREE compared to the LREE and HREE are observed (Olías et al., 2018). This suggests a pulse of AMD in the watercourse of the river that reached the estuary with no time to equilibrate (e.g. estuarine fractionation of the dREEs) to ambient conditions.
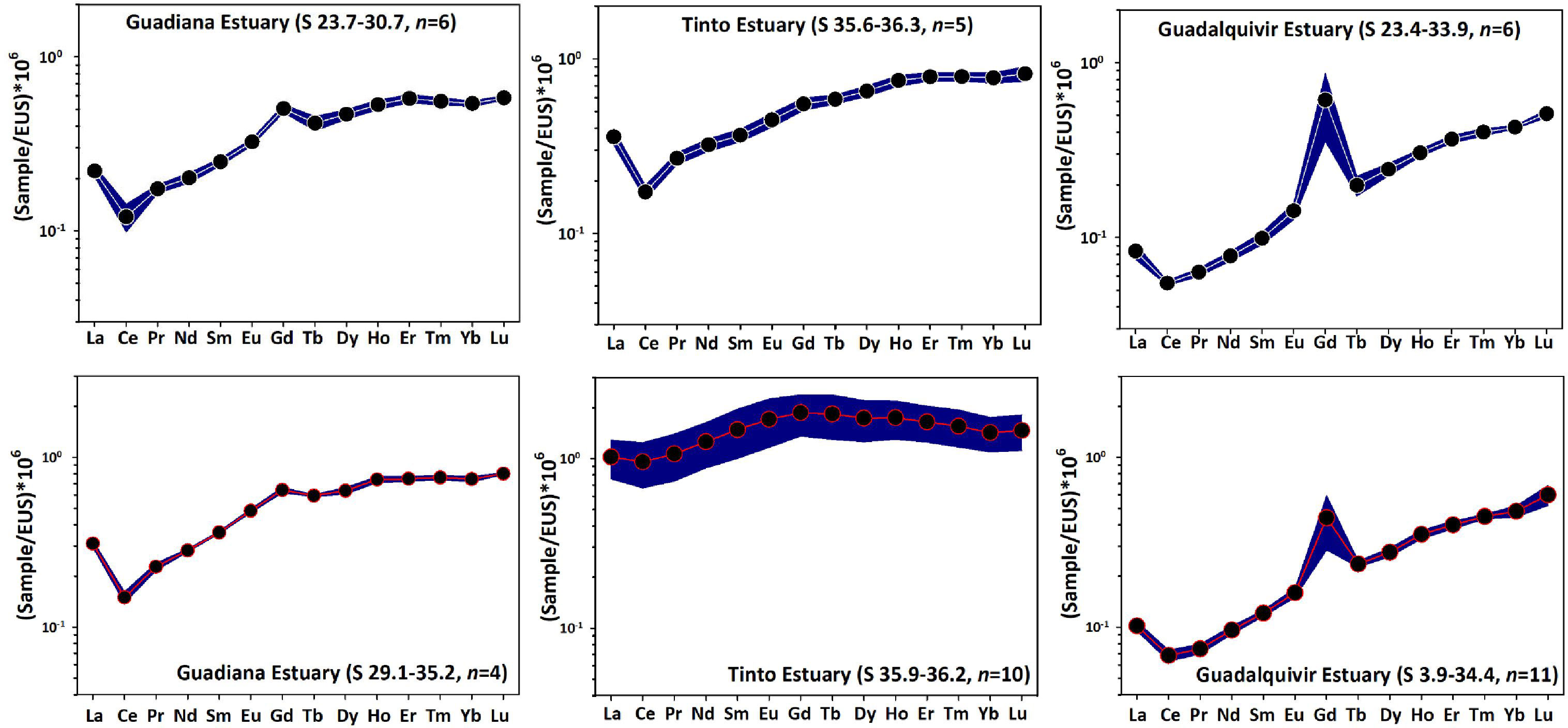
Figure 2 EUS-normalized pattern of dissolved REE in the Guadiana, Tinto-Odiel and Guadalquivir estuaries for the sampling conducted during the dry (upper panel) and wet (lower panel) season. Dots represent the average concentrations obtained, whereas the blue shaded area represents the maximum and minimum concentrations for each element.
Anthropogenic Gd in the estuaries of the GoC
No anthropogenic Gd signal was observed within the Tinto-Odiel estuary (Table 1, Figure 2), which reflects the absence of a significant urban pressure within this system and the ‘natural’ elevated concentrations that may also lead to an effective dilution effect of any anthropogenic contribution. In the case of the Guadiana estuary, the presence of a minor signal of anthropogenic Gd was observed (Figure 2); accordingly, the values calculated were Gd* = 1.40 ± 0.10 and 1.24 ± 0.05 for the dry and wet season respectively (note that anomalies up to 1.5 are considered within the range of the natural variability; Brünjes and Hofmann, 2020).
A significant input of anthropogenic Gd was evident in the case of the Guadalquivir estuary (Figure 2). This river flows through major cities (e.g. Córdoba and Seville, with a population of 325,000 and 692,000) and thus is subject to wastewater inputs that are behind this anthropogenic source. The calculated concentration of anthropogenic (Gdant), expressed as Gdant = (GdN – GdN/Gd*)·GdEUS where GdEUS is the Gd concentrations in the European Shale (Bau et al., 2018), follows a conservative mixing within the estuary (Figure 3), as expected by the unreactive Gd-complexes used as a contrast agent for magnetic resonance imaging (MRI), which is the major contamination source of this element (Neira et al., 2022). The fraction of Gdant over total dissolved Gd was higher during the dry season, accounting for a greater dilution during the periods of elevated water discharge in the wet season. Nevertheless, Gdant represented a significant fraction in both sampling campaigns (Figure 3), increasing above 70% as salinity decreases, which indicates that, at the freshwater end-member, the anthropogenic inputs dominate the total dissolved concentrations of Gd in the Guadalquivir river.
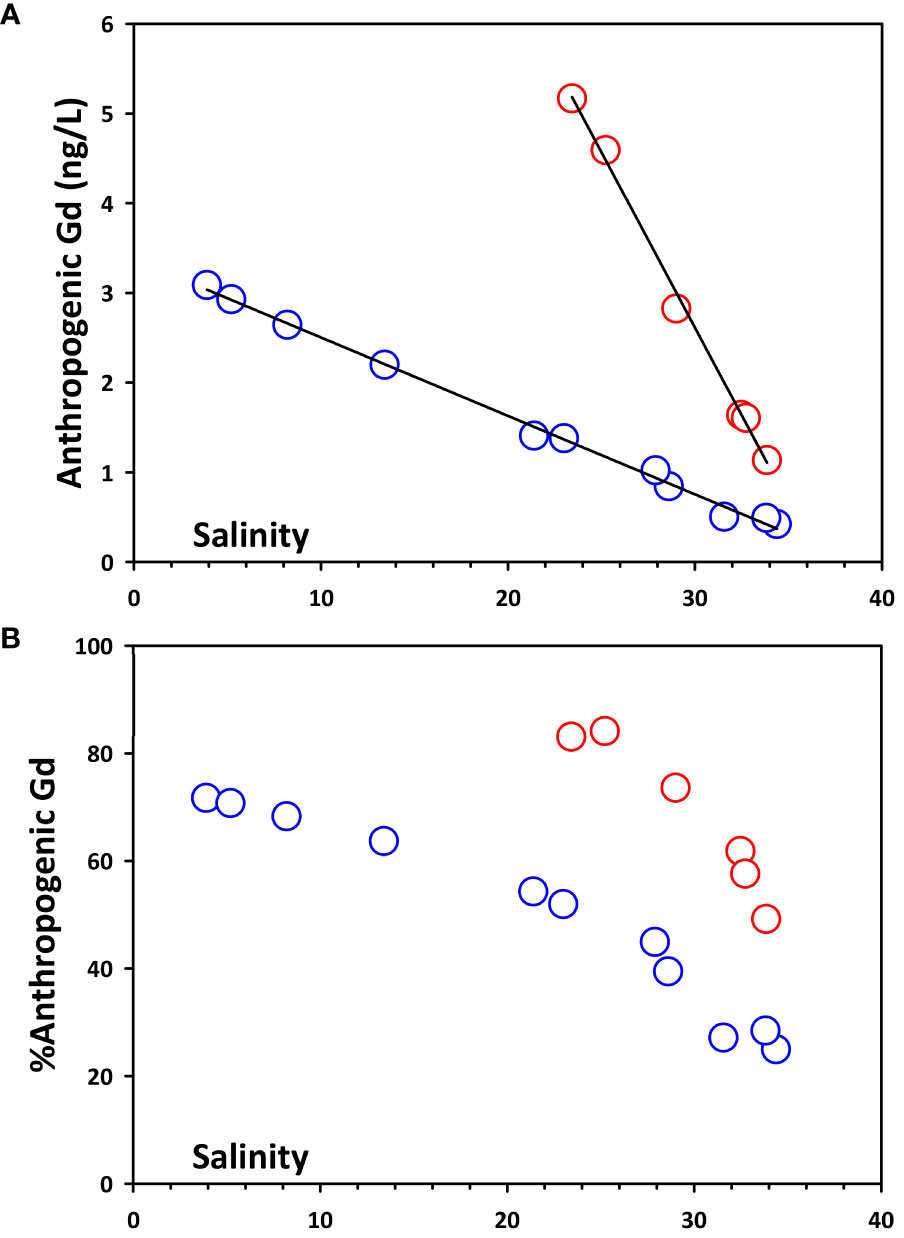
Figure 3 (A) Conservative mixing of dissolved anthropogenic Gd in the Guadalquivir estuary obtained in the sampling campaigns during the wet (blue dots) and dry (red dots) seasons. (B) Anthropogenic fraction over total dissolved Gd.
Dissolved REE distribution in the GoC
The highest surface total dREEs in the GoC were obtained in the near-shore area between the outflow of the Tinto-Odiel and Guadalquivir rivers (Figure 4), responding to the inputs of elevated concentrations of dREEs by the Tinto-Odiel which are then transported eastwards by the surface coastal currents (Figure 1). The fractionation of dREEs, regarding the LREE/HREE and MREE/HREE ratios (expressed as PrN/YbN and GdN/YbN, respectively; Figure 4), display the highest values in the area of the influence of the Tinto-Odiel discharge, with values decreasing southeastwards to the Strait of Gibraltar. A similar distribution pattern showing a distinctive signal due to this freshwater source is also observed for the La, Ce and Eu anomalies (La*, Ce*, Eu*; Figure 4).
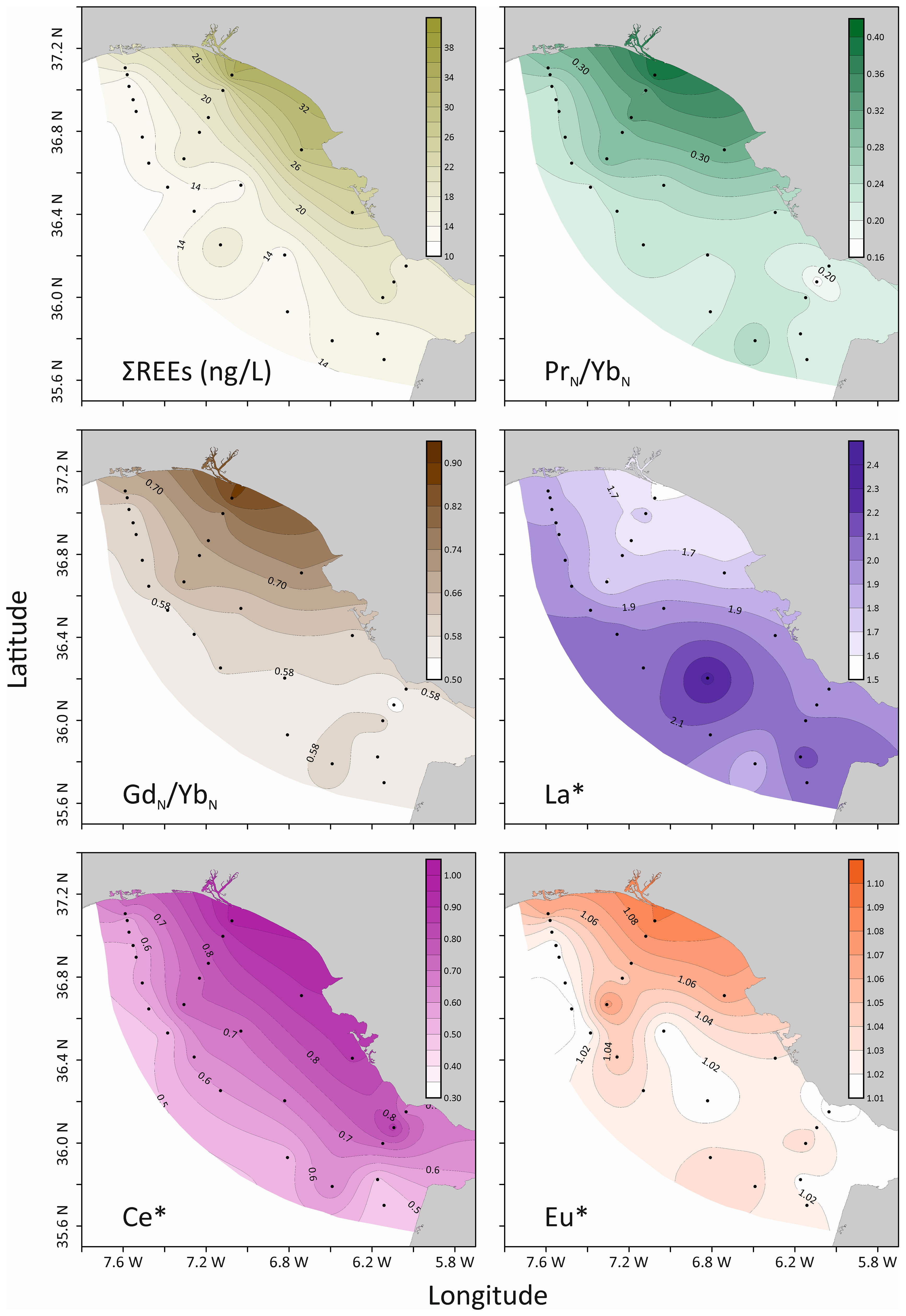
Figure 4 Contour plots of the surface spatial distribution of total dREEs, the LREE/HREE (as expressed as PrN/YbN) and MREE/HREE (expressed as GdN/YbN) ratios, and the anomalies of La (La*), Ce (Ce*) and Eu (Eu*) in the continental shelf of the Gulf of Cádiz. Dots indicated the location of the sampling stations.
Sources of REEs to the GoC
Estuarine fluxes of dREEs to the GoC, estimated from the average concentrations obtained for each estuary and the freshwater discharge at the sampling date, are given in Table 2. Highest fluxes of LREE were derived from the Tinto-Odiel estuary during the wet season; during the dry season, the Guadiana and Guadalquivir were a higher source of LREE to the GoC. A higher flux for the heavier REE were obtained for the Guadiana and/or the Guadalquivir estuaries compared to the Tinto-Odiel.
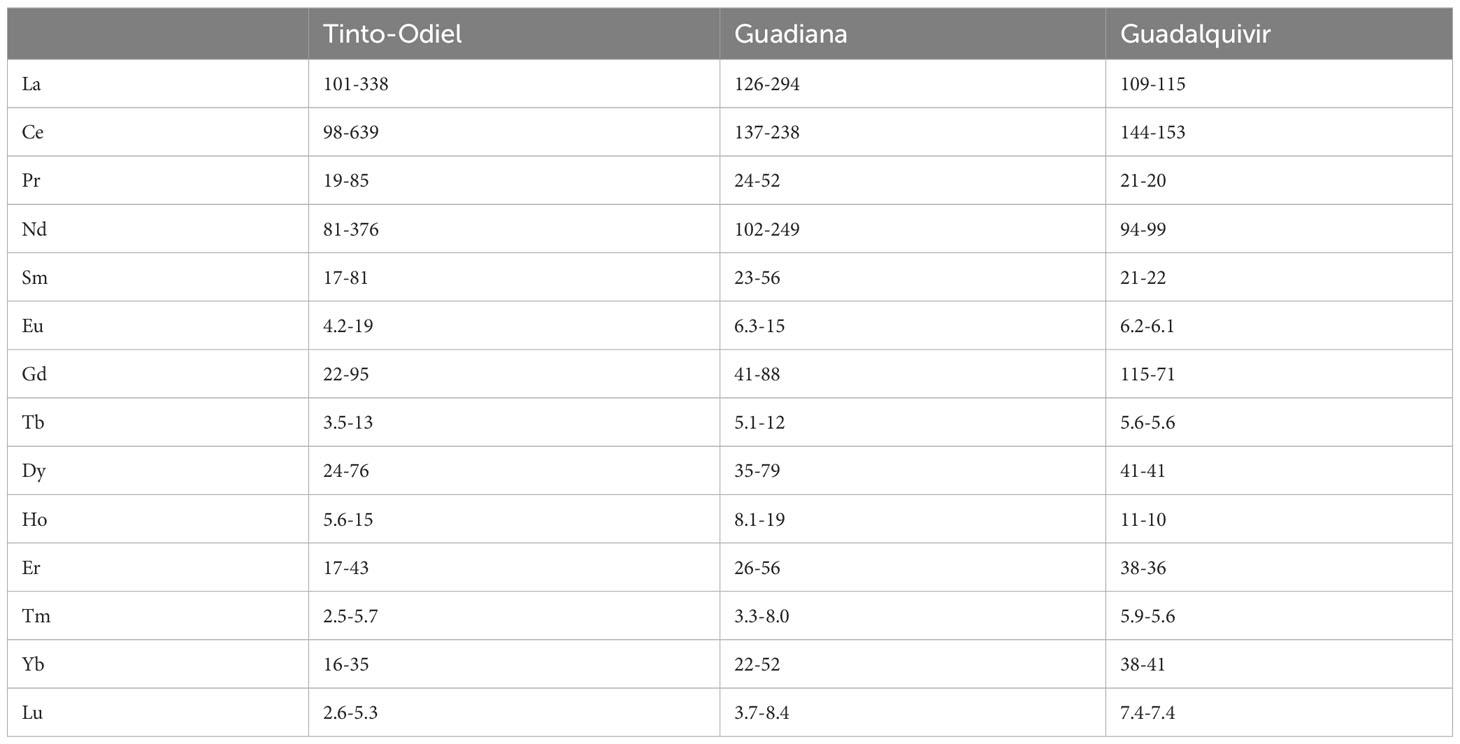
Table 2 Fluxes of dREEs to the Gulf of Cádiz (in µg/s) from the Tinto-Odiel, Guadiana and Guadalquivir estuaries for the sampling campaigns conducted in the dry and wet seasons (d-w).
In order to constrain the impact of these continental sources of dREEs on their distribution in the GoC, the relative differences in EUS-normalized concentrations presented as the MREE anomaly vs. the HREE/LREE ratio (Figure 5) were used (Crocket et al., 2018). Note that, in order to avoid the inference of Gdant in the calculation of the MREE anomaly, the Gd concentrations were not used in the calculations (see caption of Figure 5). In the water samples of the GoC (Figure 5, left), results show a trend of increasing MREE/MREE* values as the HREE/LREE decreases. The two samples with highest MREE/MREE* (>0.75) correspond to TO1 and GD1 (Figure 1), that is, the two most influenced by the Tinto-Odiel discharge (note that GD1 is affected due to the coastal eastward surface currents in this area; Figure 1), whereas lowest MREE/MREE* ratios (and highest HREE/LREE) occurred at the easternmost stations close to the Strait of Gibraltar. In order to assess the control of the potential sources on the distribution of dREEs in the GoC, the values obtained in the estuaries were also included (Figure 5, right). It can be observed that both the Guadiana and Tinto-Odiel (in the dry season) display a similar REE signature, overlapping with several stations in the GoC. The data from the Tinto-Odiel in the wet season, due to the likely presence of a pulse of non-fractionated AMD within the estuary, results in significantly higher values for MREE/MREE* and lower for HREE/LREE, but maintaining the continuum in the trend; this increase in MREE/MREE* for the AMD-impacted estuarine waters in the Tinto-Odiel estuary are in agreement with the elevated values reported for the AMD end-member (Olías et al., 2018; Figure 5). Data of the Guadalquivir estuary provides a distinctive end-member, with the lowest MREE/MREE* ratios (and highest HREE/LREE), and are within the typical ratios reported for the NW Mediterranean (García-Solsona and Jeandel, 2020; Figure 5). The majority of ratios in the open waters of the GoC are, however, typical of those for surface NE Atlantic waters (shown in Figure 5 the data of the easternmost stations of the Extended Ellett Line in Crocket et al., 2018), indicating that the typical signature of NE atlantic surface waters in the GoC is altered by two different end-members, i.e., the Tinto-Odiel signal leading to high MREE/MREE* and low HREE/LREE ratios, and the Mediterranean and Guadalquivir inputs resulting in lower MREE anomalies and higher HREE/LREE ratios.
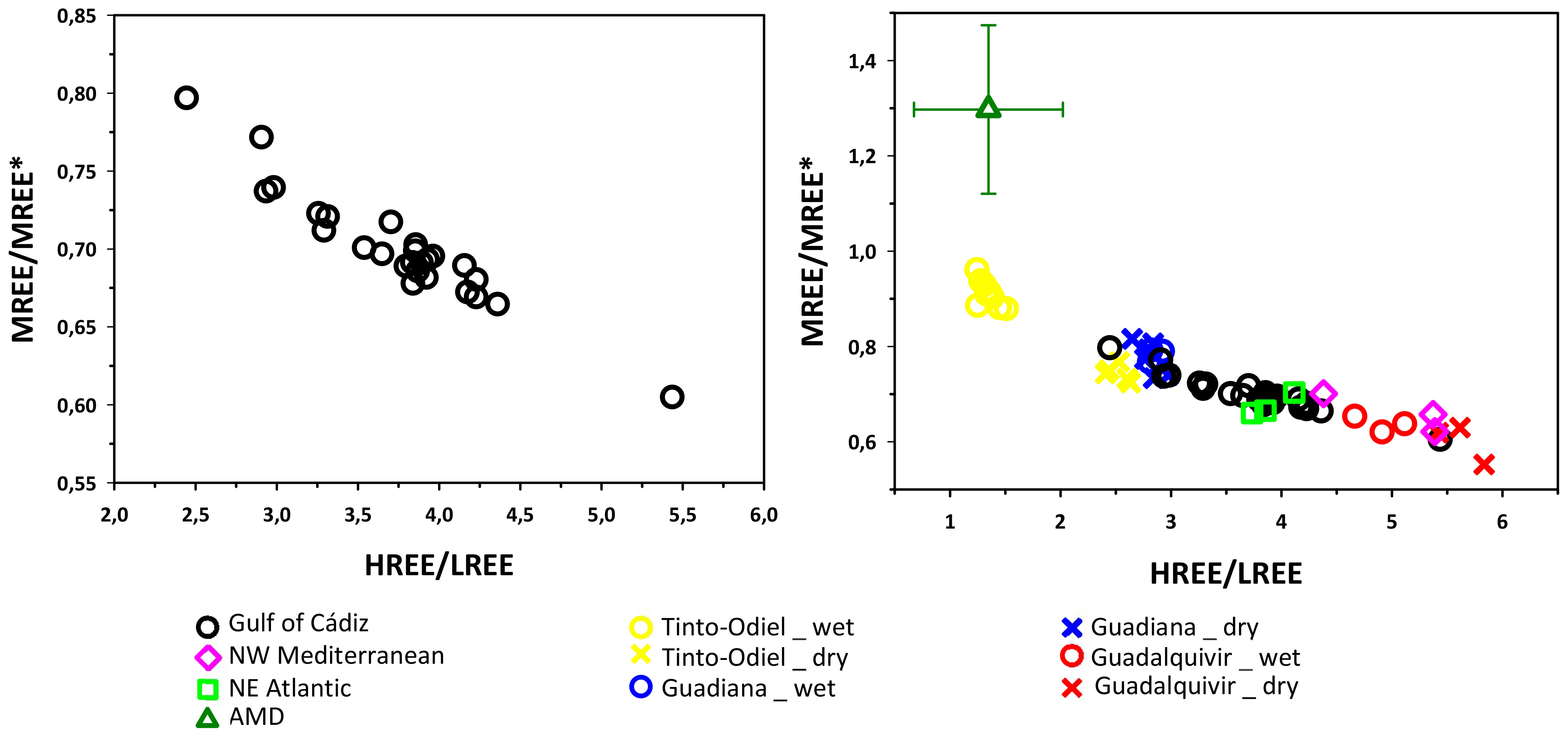
Figure 5 EUS-normalized dREE concentrations expressed as the MREE anomaly (MREE/MREE* = (TbN+DyN)/[(LaN+PrN+NdN+TmN+YbN+LuN)/2]) vs. the HREE/LREE ratio (HREE/LREE= (TbN+YbN+LuN)/(LaN+PrN+NdN)) in waters of the Gulf of Cádiz (left) and (right) including the different sources to the basin. For the estuarine data, only samples with salinity>30 were plotted. Note that for the calculation of the MREE, GdN was not considered due to the presence of an anthropogenic anomaly affecting the Guadalquivir estuary. Values for the sub-surface NW Mediterranean (100m depth) were taken from García-Solsona and Jeandel (2020), for the surface NE Atlantic from Crocket et al., 2018 (<10m depth) and for the AMD inputs in the Tinto-Odiel from Olías et al. (2018).
Conclusions
Elevated dissolved metal concentrations have been previously reported in estuarine and coastal waters of the GoC, as a result of the mineralization of the Iberian pyrite belt, and whose acidic and metal rich drainage discharge is transported by the Tinto-Odiel river. However, this impact on the concentrations and distribution of dREEs in this coastal area has not been addressed yet. Here, we have observed that surface waters of the GoC display typical NE Atlantic distribution patterns of dREEs, which are modified by two different end-members within this region. Accordingly, and despite the low water discharge of the Tinto-Odiel river, its elevated concentrations lead to an increase in the concentrations of dREEs in the nearshore waters of the GoC and producing a distinctive signature as observed for the MREE anomalies and HREE/LREE ratios. At the easternmost stations, close to the Strait of Gibraltar, these signals are also impacted by the inflow of Mediterranean waters and also by the discharge of the Guadalquivir river.
The presence of anthropogenic Gd in the estuaries was significant in the Guadalquivir estuary, likely due to the wastewater discharge from large cities (e.g. Córdoba, Seville) to this river, representing a major fraction of total dissolved Gd (>70%) in the freshwater end-member. In the case of the Guadiana estuary, a slight Gd anomaly was detected but was within the typical values of natural variability, whereas in the Tinto-Odiel no anomaly was observed.
Data availability statement
The raw data supporting the conclusions of this article will be made available by the authors, without undue reservation.
Author contributions
AC: Conceptualization, Data curation, Formal analysis, Methodology, Writing – original draft, Writing – review & editing. PB: Data curation, Formal analysis, Writing – review & editing. CM: Formal analysis, Writing – review & editing. EG: Writing – original draft. DS: Writing – review & editing. RS: Writing – review & editing. AT: Funding acquisition, Supervision, Writing – review & editing.
Funding
The author(s) declare that financial support was received for the research, authorship, and/or publication of this article. This study was funded by the Spanish Ministry of Science and Innovation under the project CTM2014-59244-C3-3-R.
Acknowledgments
CM acknowledges the FPU scholarship granted by the Spanish Ministry of Science, Innovation and Universities.
Conflict of interest
The authors declare that the research was conducted in the absence of any commercial or financial relationships that could be construed as a potential conflict of interest.
The author(s) declared that they were an editorial board member of Frontiers, at the time of submission. This had no impact on the peer review process and the final decision.
Publisher’s note
All claims expressed in this article are solely those of the authors and do not necessarily represent those of their affiliated organizations, or those of the publisher, the editors and the reviewers. Any product that may be evaluated in this article, or claim that may be made by its manufacturer, is not guaranteed or endorsed by the publisher.
Supplementary material
The Supplementary Material for this article can be found online at: https://www.frontiersin.org/articles/10.3389/fmars.2024.1304362/full#supplementary-material
References
Andrade R. L. B., Hatje V., Pedreira R. M. A., Böning P. H., Pahnke K. (2020). REE fractionation and human Gd footprint along the continuum between Paraguacu river to coastal South Atlantic waters. Chem. Geology 532, 119303. doi: 10.1016/j.chemgeo.2019.119303
Babechuk M. G., O’Sullivan E. M., McKenna C. A., Rosca C., Nägler T. F., Schoenberg R., et al. (2020). Ultra-trace element characterization of the Central Ottawa River Basin using a rapid, flexible, and low-volume ICP-MS method. Aquat. Geochemistry 26, 327–374. doi: 10.1007/s10498-020-09376-w
Bau M., Schmidt K., Pack A., Bendel V., Kraemer D. (2018). The European Shale: An improved data set for normalization of rare earth element and yttrium concentrations in environmental and biological samples from Europe. Appl. Geochemistry 90, 142–149. doi: 10.1016/j.apgeochem.2018.01.008
Beckers J. M., Achterberg E. P., Braungardt C. (2007). Comparison of high spatial resolution trace metal distributions with model simulations for surface waters of the Gulf of Cadiz. Estuar. Coast. Shelf Sci. 74, 599–609. doi: 10.1016/j.ecss.2006.12.020
Brünjes R., Hofmann T. (2020). Anthropogenic gadolinium in freshwater and drinking water systems. Water Res. 182, 115966. doi: 10.1016/j.watres.2020.115966
Cánovas C. R., Basallote M. D., Macías F. (2020). Distribution and availability of rare earth elements and trace elements in the estuarine waters of the Ría of Huelva (SW Spain). Environ. Pollut. 267, 115506. doi: 10.1016/j.envpol.2020.115506
Cobelo-García A., Mulyani M. E., Schäfer J. (2021). Ultra-trace interference-free analysis of palladium in natural waters by ICP-MS after on-line matrix separation and pre-concentration. Talanta 232, 122289. doi: 10.1016/j.talanta.2021.122289
Crocket K. C., Hill E., Abell R. E., Johnson C., Gary S. F., Brand T., et al. (2018). Rare earth element distribution in the NE Atlantic: evidence for benthic source, longevity of the seawater signal, and biogeochemical cycling. Front. Mar. Sci. 5. doi: 10.3389/fmars.2018.00147
Delgado J., Sarmiento A. M., Condesso de Melo M. T., Nieto J. M. (2009). Environmental impact of mining activities in the southern sector of the Guadiana basin (SW of the Iberian Peninsula). Water Air Soil Pollut. 199, 323–341. doi: 10.1007/s11270-008-9882-x
Elbaz-Poulichet F., Morley N. H., Beckers J.-M., Nomerange P.H. (2001). Metal fluxes through the Strait of Gibraltar: the influence of the Tinto and Odiel rivers (SW Spain). Mar. Chem. 73, 193–213. doi: 10.1016/S0304-4203(00)00106-7
García-Solsona E., Jeandel C. (2020). Balancing rare earth element distributions in the Northwestern Mediterranean Sea. Chem. Geology 532, 119372. doi: 10.1016/j.chemgeo.2019.119372
Garel E., Ferreira O. (2013). Fortnightly changes in water transport direction across the mouth of a narrow estuary. Estuaries Coasts 36, 286–299. doi: 10.1007/s12237-012-9566-z
González-Ortegón E., Baldó F., Arias A., Cuesta J. A., Fernández-Delgado C., Vilas C., et al. (2015). Freshwater scarcity effects on the aquatic macrofauna of a European Mediterranean-climate estuary. Sci. Total Environ. 503-504, 213–221. doi: 10.1016/j.scitotenv.2014.06.020
González-Ortegón E., Drake P. (2012). Effects of freshwater inputs on the lower trophic levels of a temperate estuary: physical, physiological or trophic forcing? Aquat. Sci. 74, 455–469. doi: 10.1007/s00027-011-0240-5
González-Ortegón E., Laiz I., Sánchez-Quiles D., Cobelo-García A., Tovar-Sánchez A. (2019). Trace metal characterization and fluxes from the Guadiana, Tinto-Odiel and Guadalquivir estuaries to the Gulf of Cadiz. Sci. Total Environ. 650, 2454–2466. doi: 10.1016/j.scitotenv.2018.09.290
Grande J. A., Borrego J., Morales J. A. (2000). A study of heavy metal pollution in the Tinto-Odiel estuary in southwestern Spain using factor analysis. Environ. Geology 39, 1095–1101. doi: 10.1007/s002549900080
King H. M. (2023) REE – Rare Earth Elements and their Uses. Available online at: https://geology.com/articles/rare-earth-elements/#uses (Accessed 09/19/2023).
Kulaksız S., Bau M. (2007). Contrasting behavior of anthropogenic gadolinium and natural rare earth elements in estuaries and the gadolinium input into the North Sea. Earth Planetary Sci. Lett. 260, 361–371. doi: 10.1016/j.epsl.2007.06.016
Kulaksız S., Bau M. (2011). Anthropogenic gadolinium as a microcontaminant in tap water used as drinking water in urban areas and megacities. Appl. Geochemistry 26, 1877–1885. doi: 10.1016/j.apgeochem.2011.06.011
Lawrence M. G., Greig A., Collerson K. D., Kamber B. S. (2006). Rare earth element and yttrium variability in south east Queensland waterways. Aquat. Geochemistry 12, 39–49. doi: 10.1007/s10498-005-4471-8
Lecomte K. L., Sarmiento A. M., Borrego J., Nieto J. M. (2017). Rare earth elements mobility processes in an AMD-affected estuary: Huelva estuary (SW Spain). Mar. Pollut. Bull. 121, 282–291. doi: 10.1016/j.marpolbul.2017.06.030
Neira P., Romero-Freire A., Basallote M. D., Qiu H., Cobelo-García A., Cánovas C. R. (2022). Review of the concentration, bioaccumulation, and effects of lanthanides in marine systems. Front. Mar. Sci. 9. doi: 10.3389/fmars.2022.920405
Olías M., Cánovas C. R., Basallote M. D., Lozano A. (2018). Geochemical behavior of rare earth elements (REE) along a river reach receiving inputs of acid mine drainage. Chem. Geology 493, 468–477. doi: 10.1016/j.chemgeo.2018.06.029
Olías M., Nieto J. (2015). Background conditions and mining pollution throughout history in the Río Tinto (SW Spain). Environments 2, 295–316. doi: 10.3390/environments2030295
Rétif J., Zalouk-Vergnoux A., Briant N., Poirier L. (2023). From geochemistry to ecotoxicology of rare earth elements in aquatic environments: diversity and uses of normalization reference materials and anomaly calculation methods. Sci. Total Environ. 856, 158890. doi: 10.1016/j.scitotenv.2022.158890
Romero-Freire A., Lassoued J., Silva E., Calvo S., Pérez F. F., Bejaouib N., et al. (2020). Trace metal accumulation in the commercial mussel M. galloprovincialis under future climate change scenarios. Mar. Chem. 224, 103840. doi: 10.1016/j.marchem.2020.103840
Ruiz Cánovas C., Basallote M. D., Macías F., Olías M., Pérez-López R., Ayora C., et al. (2021). Geochemical behavior and transport of technology critical metals (TCMs) by the Tinto River (SW Spain) to the Atlantic Ocean. Sci. Total Environ. 764, 143796. doi: 10.1016/j.scitotenv.2020.143796
Sánchez-Leal R. F., Bellanco M. J., Fernández-Salas L. M., García-Lafuente J., Gasser-Rubinat M., González-Pola C., et al. (2017). The Mediterranean Overflow in the Gulf of Cadiz: a rugged journey. Sci. Adv. 3, eaao060. doi: 10.1126/sciadv.aao0609
Keywords: rare earth elements (REE), Gulf of Cadiz, Guadalquivir estuary, Guadiana estuary, Tinto-Odiel estuary, anthropogenic gadolinium
Citation: Cobelo-García A, Bernárdez P, Mendoza-Segura C, González-Ortegón E, Sánchez-Quiles D, Sánchez-Leal R and Tovar-Sánchez A (2024) Rare earth elements distribution in the Gulf of Cádiz (SW Spain): geogenic vs. anthropogenic influence. Front. Mar. Sci. 11:1304362. doi: 10.3389/fmars.2024.1304362
Received: 29 September 2023; Accepted: 15 February 2024;
Published: 05 March 2024.
Edited by:
Teba Gil-Díaz, Friedrich Schiller University Jena, GermanyReviewed by:
Pedro Brito, Portuguese Institute for Sea and Atmosphere (IPMA), PortugalMarie-Noëlle Pons, Université de Lorraine, France
Copyright © 2024 Cobelo-García, Bernárdez, Mendoza-Segura, González-Ortegón, Sánchez-Quiles, Sánchez-Leal and Tovar-Sánchez. This is an open-access article distributed under the terms of the Creative Commons Attribution License (CC BY). The use, distribution or reproduction in other forums is permitted, provided the original author(s) and the copyright owner(s) are credited and that the original publication in this journal is cited, in accordance with accepted academic practice. No use, distribution or reproduction is permitted which does not comply with these terms.
*Correspondence: Antonio Cobelo-García, acobelo@iim.csic.es