Sponge abundance and diversity patterns in the shallow and mesophotic reefs of the northern Red Sea
- 1School of Zoology, Faculty of Life Sciences, Tel- Aviv University, Tel-Aviv, Israel
- 2The Steinhardt Museum of Natural History, Tel- Aviv University, Tel-Aviv, Israel
Accumulating data on the increasing degradation of coral reefs worldwide has led to a focus on the unique mesophotic coral ecosystem (MCE) as a potential refuge for threatened shallow-water species (i.e., the deep reef refugia hypothesis: DRRH). Sponges play a crucial role in coral-reef functioning but are often overlooked in benthic surveys. This knowledge gap is especially true for the Red Sea, where data on sponge abundance, coverage and species composition are scarce. Furthermore, to date, no study has examined mesophotic sponges in the Red Sea. Here we compared sponge abundance and diversity metrics between shallow and mesophotic reefs at two sites in the Gulf of Aqaba, northern Red Sea. We also examined the role of biotic and abiotic parameters in determining sponge variability between sites and depths. Sponge diversity metrics significantly varied with increasing depth and between sites. Sponge species composition also differed significantly between depth and sites. However, parameters measured in the water column, that is, Total Organic Carbon (TOC), Particulate Organic Carbon (POC), NOx, etc., did not differ between depths and sites, except for Synechococcus and Prochlorococcus concentration, which did. The findings indicated that site characteristics and biotic interactions may play an essential role in determining sponge diversity and community composition. Between-site differences in sponge fauna revealed in the study highlight the importance of considering the variability in the benthic communities and the factors controlling it when designing management tools for coral reefs, particularly for the northern Red Sea.
1 Introduction
Mesophotic Coral Ecosystems (MCEs) are benthic reefs typically occurring below shallow coral reefs (< 30 m) and down to the bottom of the photic zone (~150 m) (Lesser et al., 2018; Pyle and Copus, 2019). MCEs support diverse benthic communities, composed of scleractinian corals, octocorals, sponges and many other associated organisms that dwell among them (Garcia-Sais, 2010; Lesser and Slattery, 2018; Pyle and Copus, 2019; Goren et al., 2021). Some communities in the MCEs constitute a direct extension of the shallow coral reef (Slattery et al., 2011), while others are both taxonomically and functionally unique to the mesophotic depths (Loya et al., 2016; Kahng et al., 2017). The abiotic conditions undergo changes from shallow to mesophotic depths, as light, temperature and turbulence flow decrease, while nutrient concentrations increase (Lesser et al., 2018; Kahng et al., 2019). Also, due to their (usually) relatively far distance from the shore and sea surface, MCEs have been considered less vulnerable to anthropogenic disturbances, such as ocean warming. This has led to the ‘deep reef refugia hypothesis’ (DRRH), which suggests that MCEs could act as sanctuaries and source of propagules for endangered shallow-water species (Bongaerts and Smith, 2019). Nevertheless, even though the potential promise of this hypothesis for the preservation of endangered shallow-water species, in many reefs around the world, MCEs remain largely unexamined (Smith et al., 2016; Bongaerts et al., 2017; Medeiros et al., 2021) and DRRH role in species conservation is highly controversial. Thus, while some studies support its significance (Kramer et al., 2019; Gasbarro et al., 2022; Sturm et al., 2022), others challenge its validity, mainly at local scales (Slattery et al., 2018; Eyal et al., 2022; Loiseau et al., 2023). Moreover, MCEs have traditionally received less scientific attention compared to their shallow counterparts worldwide (Menza et al., 2008), and particularly so in the Indo-Pacific reefs (Kahng et al., 2010; Dumalagan et al., 2019). This limited exploration of MCEs is due to the logistical and technical constraints involved in research at depths beyond the limits of conventional SCUBA diving (Kahng et al., 2014; Sinniger et al., 2016). This situation emphasizes the important need to reveal the ecological aspects of MCEs, such as biodiversity patterns and the related factors shaping them along the shallow to mesophotic depth. Such studies are particularly urgent in light of the recent evidence of the rapid degradation of shallow coral reefs worldwide (Glynn, 1996; Hughes et al., 2003; Shlesinger and Loya, 2019).
Sponges (Porifera) are a conspicuous component of the benthic fauna on both shallow and mesophotic reefs, exceeding coral biomass and abundance at some locations in the Caribbean (Diaz and Rützler, 2001). Sponges play major structural and functional roles in coral reefs, such as nutrient cycling, as they accumulate dissolved organic matter (DOM) and then release particulate organic matter (POM) back into the surrounding environment. Organisms at other trophic levels further consume the POM, a pathway termed “the sponge-loop” (De Goeij et al., 2013; Rix et al., 2018). Sponges also enhance reef biodiversity by acting as a shelter and providing a microhabitat for many of the reef’s micro and macro fauna (Fiore and Jutte, 2010; Goren et al., 2021). The importance of sponges in coral reefs highlights the significance of characterizing sponge diversity and abundance in these ecosystems. In recent years, sponges’ ecological roles, function, and community structure metrics have been extensively studied in several locations worldwide, including MCEs sites (Lesser, 2006; Pawlik et al., 2016; Lesser and Slattery, 2018; Scott et al., 2019; Macartney et al., 2021). However, in the Indo-Pacific reefs, particularly the Red Sea, data regarding sponges such as, ecology and distribution patterns, remain limited. Several factors contribute to this gap, including a lack of taxonomic knowledge, the frequently cryptic growth form of sponges, and difficulties in field identification, which are just some of the reasons that sponges are often overlooked (Wulff, 2001). This gap of knowledge is especially pronounced in Red Sea MCEs, where data on sponge abundance, community structure, and coverage are notably sparse (Bell et al., 2015; Eyal et al., 2019; Wooster et al., 2019).
The Red Sea, known for its diverse coral reefs, is considered a marine biodiversity hotspot due to the high level of species richness and endemism (Wooster et al., 2019). Nevertheless, most studies conducted on sponges in this region have been related to bioactive compounds (reviewed in Wooster et al., 2019), with only a few publications from recent years engaging with sponge biodiversity (Ilan et al., 2004; Helmy and Van Soest, 2005; Erpenbeck et al., 2016; Voigt et al., 2017; Van Soest and De Voogd, 2018). Additionally, no quantitative data focused on sponge abundance, richness or species composition are available from the region, with the exception of a few studies of individual species (Meroz and Ilan, 1995a; Yahel et al., 1998), or general studies of the benthic fauna with only minor reference to sponges (Benayahu and Loya, 1981; Ellis et al., 2017; Khalil et al., 2017). Additionally, to our knowledge, no study to date has focused on the mesophotic sponge diversity composition in the Red Sea.
In the present study we therefore assessed the abundance and diversity patterns of the sponge fauna along a depth gradient from the shallow to the mesophotic reef at two sites in the Gulf of Aqaba, northern Red Sea. We also addressed the role of abiotic factors (water chemistry parameters) in shaping the variability in sponge diversity and community structure between sites and depths.
2 Materials and methods
2.1 Study sites
The Aqaba (Eilat) coral reef, extending along approximately 10 km from north to south, is characterized by a fringing reef and fragmented structure, exposed to various anthropogenic and natural disturbances (Genin et al., 1995; Loya, 2004; Katz et al., 2015; Tamir et al., 2017). Both shallow and mesophotic habitats are dominated by hermatypic scleractinian corals composed of generalized and specialist fauna. Among them, the coral families Acroporidae, Pocilloporidae, and Merulinidae, as well as Agariciidae, Lobophylliidae, Euphylliidae, and Poritidae, are the most prevalent scleractinian corals in the shallow and mesophotic reef, correspondingly. Crustose coralline algae (CCA) were also reported to flourish in the mesophotic reef in higher abundances compared to the shallow reef (Eyal et al., 2019).
Sponge surveys were carried out in July 2018 at two sites in the Gulf of Aqaba, differing in their geomorphological structure: the Interuniversity Institute for Marine Sciences in Eilat (IUI, 29°30’06.7”N 34°55’02.9”E); and the oil jetty shore (OJ, 29°31’21.7”N 34°55’56.6”E) (Figure 1A). The first site is located within Eilat’s Coral Beach Nature Reserve and is a rich reef, reaching down to 70 m along a steep slope. Unlike its shallow fringing reef, the mesophotic reef at this site is characterized by a gravel-like cover. The second sampling site is located off the OJ site, north of the nature reserve, and is composed of coral knolls distributed among grass/sand patches. It extends down to a continuous boulder-like ancient coral reef at the mesophotic depth.
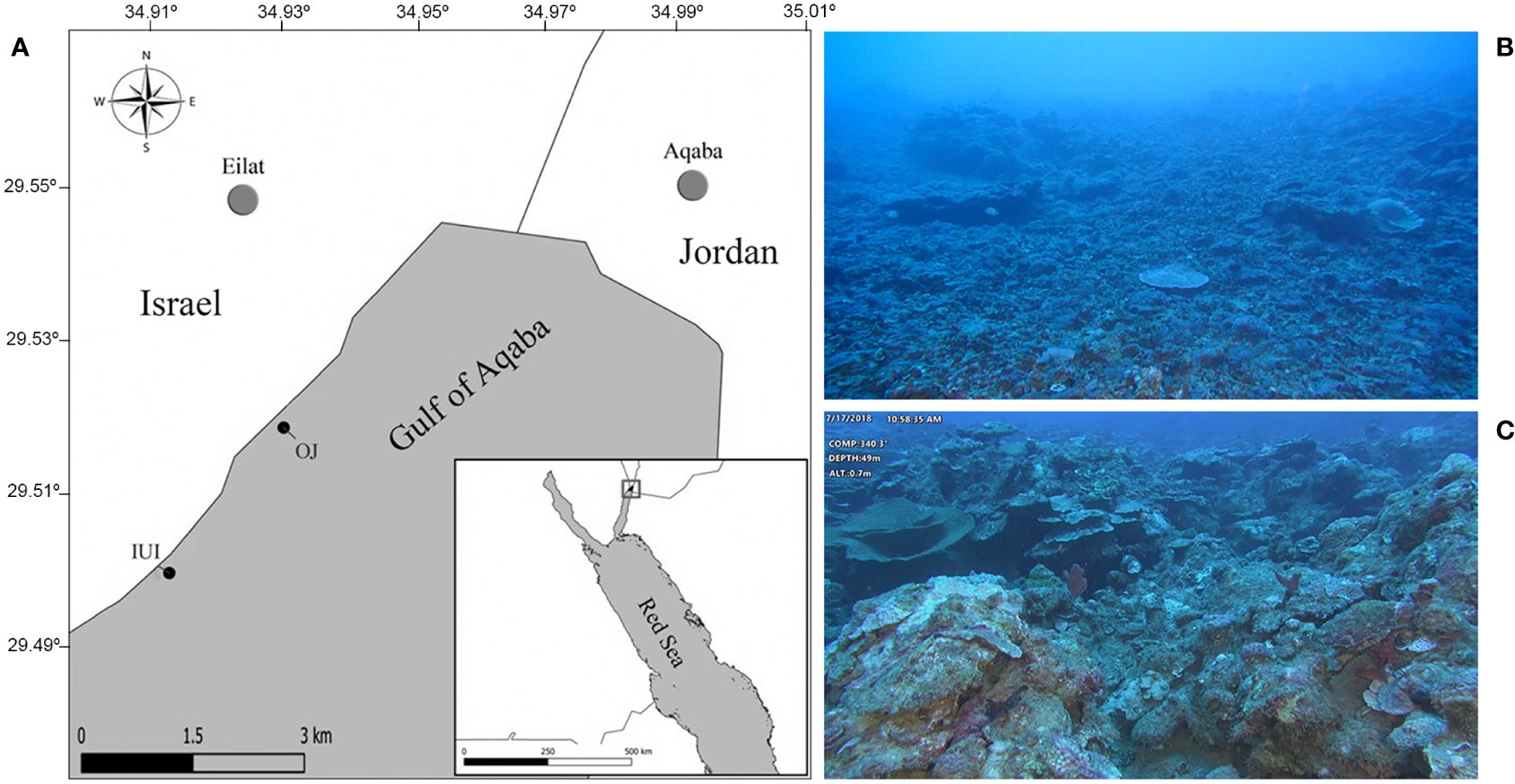
Figure 1 (A) Gulf of Eilat/Aqaba map showing the surveyed sites: IUI – The Interuniversity Institute for Marine Sciences in Eilat. OJ – Oil Jetty. WGS84 projection (B) Typical view of the mesophotic reef (60 m) at the IUI survey site, characterized by a steep slope and gravel-like cover (C) Image of the reef at the OJ survey site (50 m) characterized by large boulders mostly covered with encrusting sponges and algae.
2.2 Quantitative survey of shallow and mesophotic sponge communities
Sponge abundance, diversity and coverage were assessed in the shallow coral reefs of IUI and OJ at depths of 5, 10 and 20 m, and in the mesophotic reef at 50, 60 and 70 m and 50 and 60 m at OJ and IUI (correspondingly). For each combination of site and depth, a quantitative survey was conducted using approximately 30-35 photo-quadrats (each of 70x50 cm), covering a total area of ~10.5 m² per site and depth and a total of ~115.5 m² across both sites. The MCE survey was conducted from the R/V Sam Rothberg using a Remotely Operated Vehicle (ROV ECA-Robotics H800) equipped with FHD camera, four video lights (4000 lumens) and two parallel laser beams for scale (7.5 cm apart). The ROV’s path was continuously tracked using an acoustic underwater positioning system (USBL) and the HYPACK® navigation system. Photo-quadrats were captured at 10 m intervals to ensure there was no overlap in the surveyed area. In addition, to maintain the camera’s perpendicular orientation to the substrate, the ROV’s position was adjusted to the slope angle and fixed along the surveyed depth. This ensured that the ROV’s HD camera was aimed directly downward at a 90° angle, approximately 1 meter above the seabed. In instances where it was feasible, the ROV was positioned directly on the seafloor to capture images in front of vertical substrates (such as walls or sheer drops). In these cases, the ROV’s camera was kept horizontal (90° angle) to the photographed surface, maintaining about 1 m distance.
A similar methodology was performed at the shallow reefs, using SCUBA diving, with photo-quadrats taken ~1 m above the reef using a CANON G12/16 camera mounted on a tray with two video lights (3500 lumens) and two parallel laser beams for scale (7.5 cm apart). To ensure a uniform survey of the area and to minimize diver’s bias, photo-quadrats were taken at a consistent depth perpendicular to the target surface (seabed or wall). These were captured at intervals corresponding to every five ‘fine strikes’ aligned parallel to the shoreline. At both habitats, additional close-up pictures of the surveyed area (in each photo-quadrat) were taken in order to facilitate identification of the living organisms on the substrate. Photo-quadrats size was adjusted using ImageJ software (Abràmoff et al., 2004) to create image collections of equal size (70*50 cm). Only clear and sharp images were used for the analyses of the photo-quadrats. Furthermore, images were cropped to remove any blurry edges and lens distortion, concentrating on the central area of the image where the camera angle’s effect is minimized.
For sponge coverage calculation, 100 sampling points were overlaid on each image (50x70 cm) in a stratified random manner (Shihavuddin et al., 2013) using CoralNet online software (Lozada-Misa et al., 2017). Benthos components beneath each point were classified into one of five categories: sponge, coral, algae, other biotic components (“Other”) and substrate which included dead corals, soft and hard substrate. Each component’s coverage was calculated in relation to the others (relative abundance). To assess sponge diversity at each site/depth (e.g., richness, abundance, Shannon, evenness), the sponge species were counted and identified in each image to the lowest possible taxonomic level. To facilitate accurate identification of sponges in the photo-quadrats, where required and feasible, specimens were collected during the survey. Subsequent sponge taxonomic classification was performed through preparation and inspection of spicules and tissue sections (Rützler and Macintyre, 1978) supported by the relevant sponge taxonomic literature (Row, 1911; Hooper and Van Soest, 2002; Ilan et al., 2004; World Porifera Database, 2024). However, when taxonomic identification and/or sampling were not possible, sponges were defined according to their morphology and referred to as “morphospecies”.
2.3 Environmental conditions at the study sites
Because seawater composition may affect sponge community structure, we sampled seawater adjacent to the reef (<50 cm from the substrate) at 50 and 5 m depth at the two survey sites, using horizontal 12 L Niskin bottle (Supplementary Figure 1). Samples were collected during winter (Nov 2018-Mar 2019) and summer (Jun-Sep of 2019) and processed for: Total Organic Carbon (TOC), Particulate Organic Carbon (POC) and inorganic nutrients (NOx-, SiO2, PO43-), and fluorescence-activated cell-sorting (FACS) was used to sort the cells (following the protocols in Morganti et al., 2016 and Baetge et al., 2020). Briefly:
2.3.1 TOC analysis
Pre-combusted EPA vials (40 mL) with PTFE-lined septa were pre-washed twice with the sampled water. Twenty mL of sampled water were then added to the bottle and preserved together with 80 µL of HCL 32%. Samples were immediately stored at -80°C until processing and analysis at the Carlson Microbial Oceanography Lab at the Marine Science Institute, UC, Santa Barbara.
2.3.2 POC analysis
For this analysis duplicates of 4 L seawater from two Niskin bottle were collected and filtered through 100 µm plankton mesh to remove large particles, followed by filtration through pre-combusted (400°C for 4 hours) GF/F filters (nominal pore size 0.7 µm, 25mm). Immediately thereafter the filters were stored at -80°C and dried at 60°C for 24 h prior to analysis using a Total Organic Carbon analyzer (Shimadzu Instruments).
2.3.3 Inorganic nutrients (NOtotal, SiO2, PO4-3)
For each nutrient, duplicates of 15 mL water samples were collected from two Niskin bottles. The collection tubes and filters were twice pre-washed with the water sample before use. First, the samples were filtered through a 100 µm plankton mesh to remove large particles. They were then filtered through 0.22 membrane filters and frozen at -20°C until analysis. Nutrient concentrations were measured using a QuikChem 8000 flow injection analyzer (Lachat Instruments, Milwaukee, USA).
2.3.4 Ultra-plankton concentration
FACS analysis was used to assess the concentration of non-photosynthetic bacteria (Bac), Prochlorococcus (Pro), Synechococcus (Syn) and eukaryotic algae (Euk) cells. For this purpose, 1.8 µL of sampled water were preserved in cryovials with 0.2% EM grade glutaraldehyde, to a final concentration of 0.1%. The cryovials were then flash-frozen in liquid nitrogen and stored at -80°C until analysis using an Attune NXT® acoustic focusing flow cytometer (Applied Biosystems, Waltham, USA).
2.4 Statistical analysis
All statistical analyses and data visualizations were performed with Rstudio version 2021.09.0 + 351 (RStudio, T, 2020) in R version 4.1.0 (Team, R. C, 2020). Sponge diversity, species composition, and coverage were analyzed and compared between shallow and mesophotic reefs, as well as between sites (OJ and IUI). Coverage data were additionally compared across depths within each site. The decision to pool the diversity data into shallow (5,10,20 m) and mesophotic (50, 60, 70 m) categories, rather than ‘shallow’, ‘upper’ and ‘lower’ mesophotic, is based on previous studies from the Gulf of Eilat. These studies identified light zonation of ‘upper’ mesophotic at 30-80 m and ‘lower’ at 80-160 m, corresponding with changes in benthic community composition (Tamir, 2015; Eyal et al., 2019; Tamir et al., 2019). As our current study surveyed only depths within the ‘upper’ mesophotic, we referred to it collectively as ‘mesophotic’. In addition, to mitigate the potential bias in our results for sponge diversity indices and community composition calculations, we addressed the issue of zero-inflated data in the count data as following: data were pooled from every ten photo-quadrats per site and depth, creating one larger photo-quadrat of 3.5m², which we refer to as a ‘transect’. This approach was not necessary for the coverage analysis, which did not exhibit the same issue. Consequently, the coverage analysis was conducted using individual photo-transect units, where the average sponge diversity per site or depth was calculated based on the averaged values per transect. Sponge community structure was inferred from Bray–Curtis dissimilarity distances species-by-site matrix, calculated using the ‘metaMDS’ function. Diversity indices (e.g., richness, abundance, Shannon, evenness) were estimated using the ‘diversity’ function, all available on the vegan package (Oksanen et al., 2013). Differences in the sponge coverage and diversity indices between sites and depths were examined using permuted nested two-way ANOVA (‘aovp’ function,Wheeler et al., 2016), followed by Benjamini-Hochberg post-hoc pair-wise permutation tests, where depths were nested within sites (‘dunn_test’ function, Mangiafico and Mangiafico, 2017). A pairwise correlation matrix, based on Spearman’s correlation rank coefficient, was built to examine the relations between sponge diversity (coverage and diversity indices) and depth, as well as biotic parameters, including algae and coral coverage (‘cor’ function, Team, R. C, 2018). Rarefaction curves were built and statistically compared using the functions ‘specaccum’ and ‘EcoTest.sample’ available in the packages vegan and rareNMtests, respectively (Oksanen et al., 2013; Cayuela et al., 2015).
Sponge community composition was visualized using non-metric multidimensional scaling (nMDS), and possible differences between sites and depths were examined using ‘adonis’ function for permutation multivariate analysis of variance (PERMANOA) (Anderson, 2001; Anderson and Walsh, 2013), followed by SIMPER analysis, to identify the contribution of each sponge species to the overall dissimilarity between depths or/and sites. P. value <0.05 was defined as the level of significance (Oksanen et al., 2013).
Seawater content/composition differences in relation to season, sites and depths were assessed using permuted repeated measures ANOVA (‘aovperm’ function, nperm=5000, method=“Rd_kheradPajouh_renaud”), where site was defined as a nested factor within season (Frossard and Renaud, 2021). Nevertheless, unfortunately, only inorganic nutrients (NOx-, SiO2, PO43-) were analyzed in relation to season since the summer samples of TOC and FACS were corrupted. FACS parameters were analyzed using two-way ANOVA, after log transformation of Prochlorococcus (Pro) and Synechococcus (Syn) data to meet ANOVA’s test assumptions for normal distribution and homoscedasticity. Data visualization was performed with the following packages: ggplot2 (Wickham, 2016), ggrepel (Slowikowski et al., 2018), gridExtra (Auguie et al., 2017) and ggpubr (Kassambara and Kassambara, 2020).
3 Results
3.1 Sponge quantitative surveys
Forty-one morphospecies and twenty-three species were recorded during the quantitative surveys across depths and sites (a sum of sixty-four overall). Of these, 40 were unique to the mesophotic reef, nine unique to the shallow and 15 species/morphospecies were shared by both (Supplementary Figure 2). Among these shared species, seven are common across both shallow waters and the depths of 50, 60, and 70 m. Two species are found in both the shallow reef and at 60 m, while three are unique to both shallow and 70 m depths. The remaining species are shared between shallow waters and depths of 50 and 60 m. In addition, most sponges at the mesophotic sites were encrusting (70%), compared to only 37% at the shallow ones.
3.1.1 Sponge abundance, diversity and coverage
Sponge richness was found to be depth-dependent at both surveyed sites (Figures 2A, E) and was significantly higher in the mesophotic reef compared to the shallow reef (permuted nested two-way ANOVA, p<0.05, Figure 2A, Table 1). Thus, the highest richness was found at 60 m depth (n=30 species/morphospecies), followed by 70 m (n=29 species/morphospecies) and 50 m (n=25 species/morphospecies). At the shallow depths, 20 and 10 m displayed a similar number of sponge species (n=15 species/morphospecies), while the 5 m depth had the lowest richness (n=6 morphospecies/species). In addition, while at the shallow depths (20 and 5 m) the rarefaction curves reached a plateau, indicating sufficient sampling effort, at the mesophotic depths the curves were not asymptotic and thus far from saturation, indicating insufficient sampling effort for these depths (Figure 2E). Both sponge abundance and Shannon diversity indices were found to be significantly higher in the mesophotic compared to the shallow reef (mesophotic: 61.7 ± 15.4 SE and 4.9 ± 0.3 SE individuals/transect, respectively, shallow: 5.9 ± 0.6 SE and 1.8 ± 0.2 SE, respectively. Figures 2B, C, Table 1). The sponge community in the mesophotic reef was less even than that at the shallow depth (0.36 ± 0.0 SE and 0.68 ± 0.0 SE, respectively. Figure 2D, Table 1). In addition, while sponge abundance, Shannon and evenness were similar between sites (OJ and IUI), the mean richness was significantly higher at the OJ site compared to the IUI site (6.5 ± 1 SE vs. 3 ± 0.5 SE morphospecies/transect, respectively, Table 1).
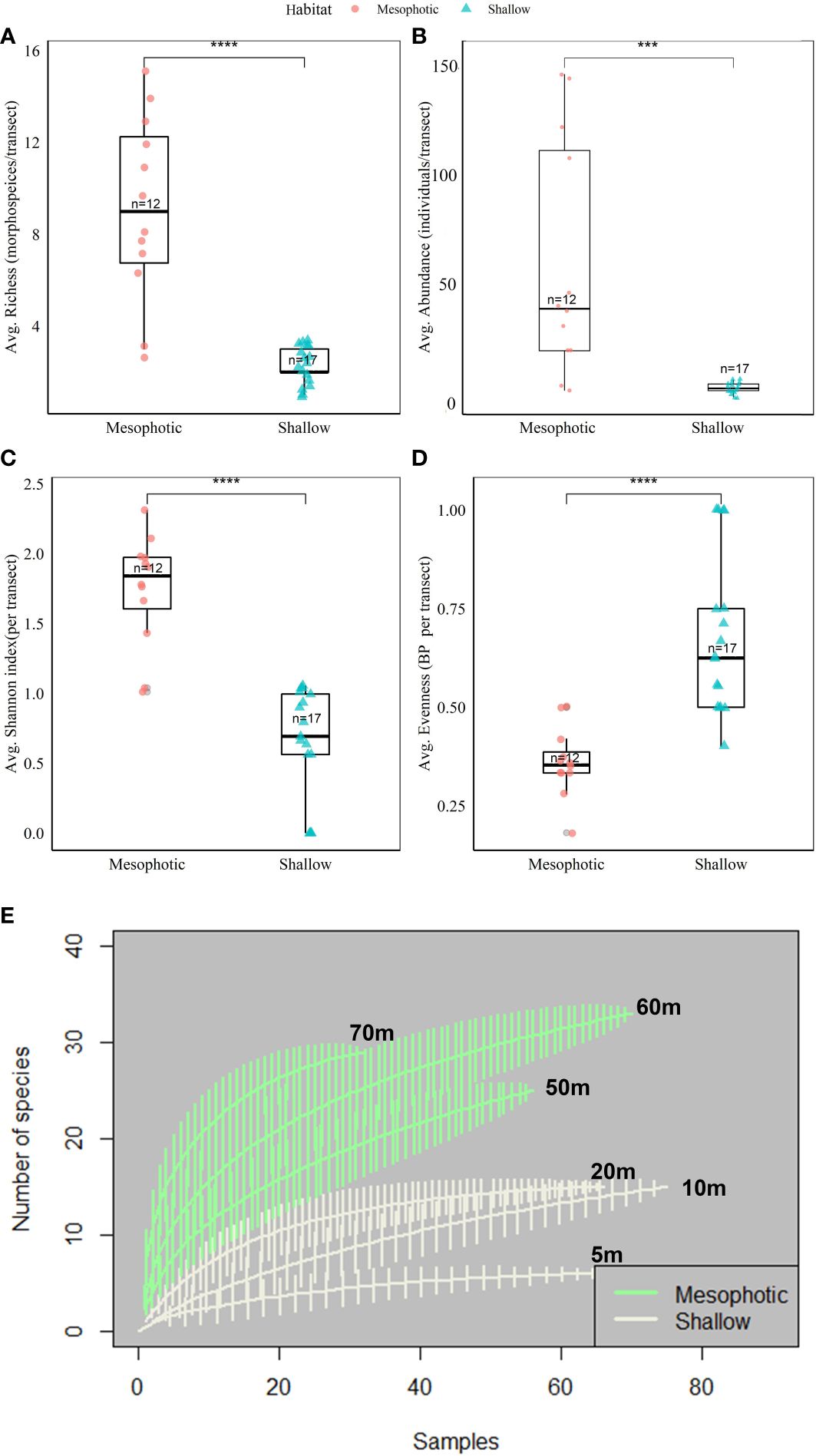
Figure 2 Mean values of sponge diversity indices – A comparison between shallow (n=17 transects) and mesophotic (n=12 transects) reefs at both study sites (IUI & OJ) pooled together. (A) Sponge richness (morphospecies/transect) (B) Sponge abundance (individuals/transect) (C) Shannon diversity index (D) Berger-Parker evenness index. Asterisks represent significant difference (p<0.05, permuted two-way ANOVA, depths nested within sites, Table 1). Interquartile range of 25th and 75th percentile of the data is represented by the box. The median value is indicated by the line inside the box. (E) Sample-based Rarefaction curves of sponge species and morphospecies richness in the mesophotic (green curves) and shallow (grey curves), sites pooled. Permutations no. = 1000 per curve. The vertical lines indicate confidence interval of 95%. *** p.value <0.001, **** p.value <0.0001.
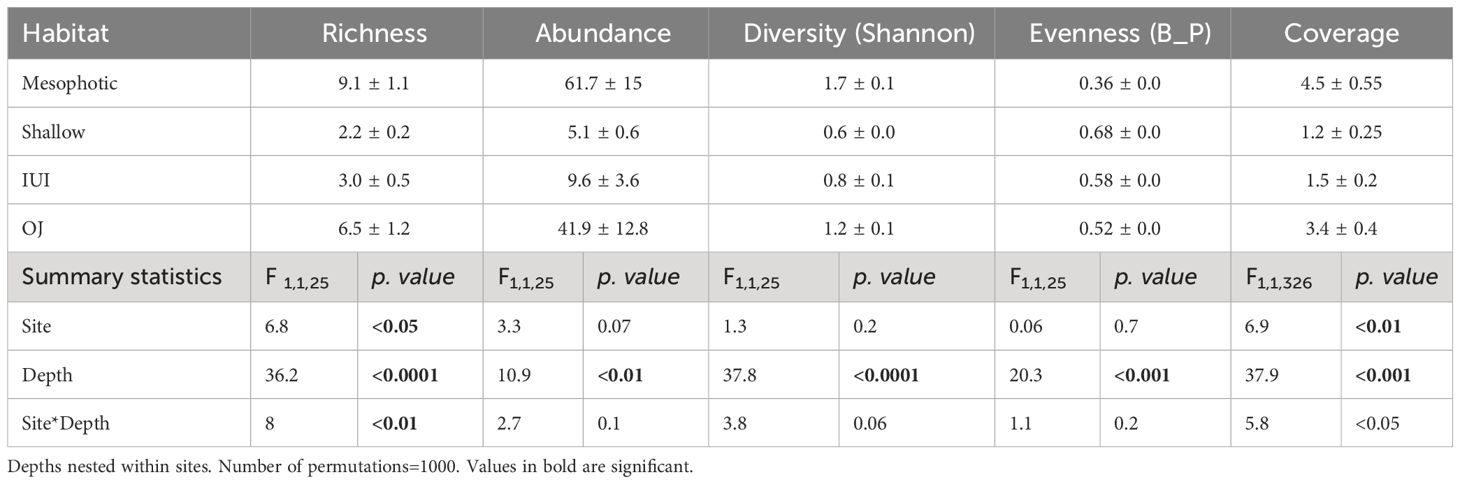
Table 1 Mean values and summary statistics of permuted nested two-way ANOVA results for depth and site effect on sponge richness, abundance, Shannon diversity index, evenness index and sponge coverage.
Sponge coverage significantly increased with depth and varied between sites. Also, a significant interaction was found between depth x site effect for sponge coverage (Table 1, Figure 3A). The highest sponge coverage was found at 70 m (mean: 8.4% ± 1.5 SE) and the lowest at 5 m (<1%). Moreover, sponge coverage was higher at the OJ site (mean:3.4% ± 0.4 SE) compared to the IUI (mean: 1.5% ± 0.2 SE) and varied between sites at the same depths: for example, sponge coverage was two-fold higher at the OJ mesophotic reef compared to the IUI mesophotic (mean: 5.5% ± 0.7 SE vs. 2.6% ± 0.6 SE, Figures 3A, B; Supplementary Table 1). In addition, at both sites sponge coverage significantly increased between depths (IUI: F1,144 = 10.4, p<0.05, OJ: F1,182 = 36.8, p<0.001, Figures 3A, B). At the OJ site sponge coverage significantly changed along depths, peaking at 20 m, followed by a decrease and then peaking again at 70 m, whereas at IUI, sponge coverage peaked at 50 m and decreased at 60 m (Figures 3A, B; Supplementary Table 1). Algal and coral coverage also differed between depths but with an opposite trend. While algal coverage increased with depth (F1,326 = 645, p<0.001) that of coral decreased (F1,326 = 45, p<0.001). Thus, out of the total coverage, algae dominated the mesophotic depths at both sites (~50%) whereas corals were a prominent biotic component on the shallow reefs (~25%). In addition, differences in coral and algal coverage were also observed between sites. For example, coral coverage at the IUI mesophotic depth was 19% ± 1.8 SE, compared to 9.2% ± 1.1 SE at the OJ site (Supplementary Table 1). In addition, significant positive correlations were found between depth and sponge Shannon index (Spearman’s rank, R2 = 0.83, p<0.05), sponge abundance (Spearman’s rank R2 = 0.94, p<0.05) and algal cover (Spearman’s rank R2 = 0.83, p<0.05, Table 2). Conversely, depth had a significant negative correlation with coral cover (Spearman’s rank R2=-0.83 p<0.05) and sponge community evenness (Spearman’s rank R2=-0.83, p<0.05, Table 2). Significant negative correlations were also found between sponge abundance and coral cover (Spearman’s rank R2=-0.77, p<0.05), and sponge community evenness (Spearman’s rank R2=-0.94, p<0.05, Table 2).
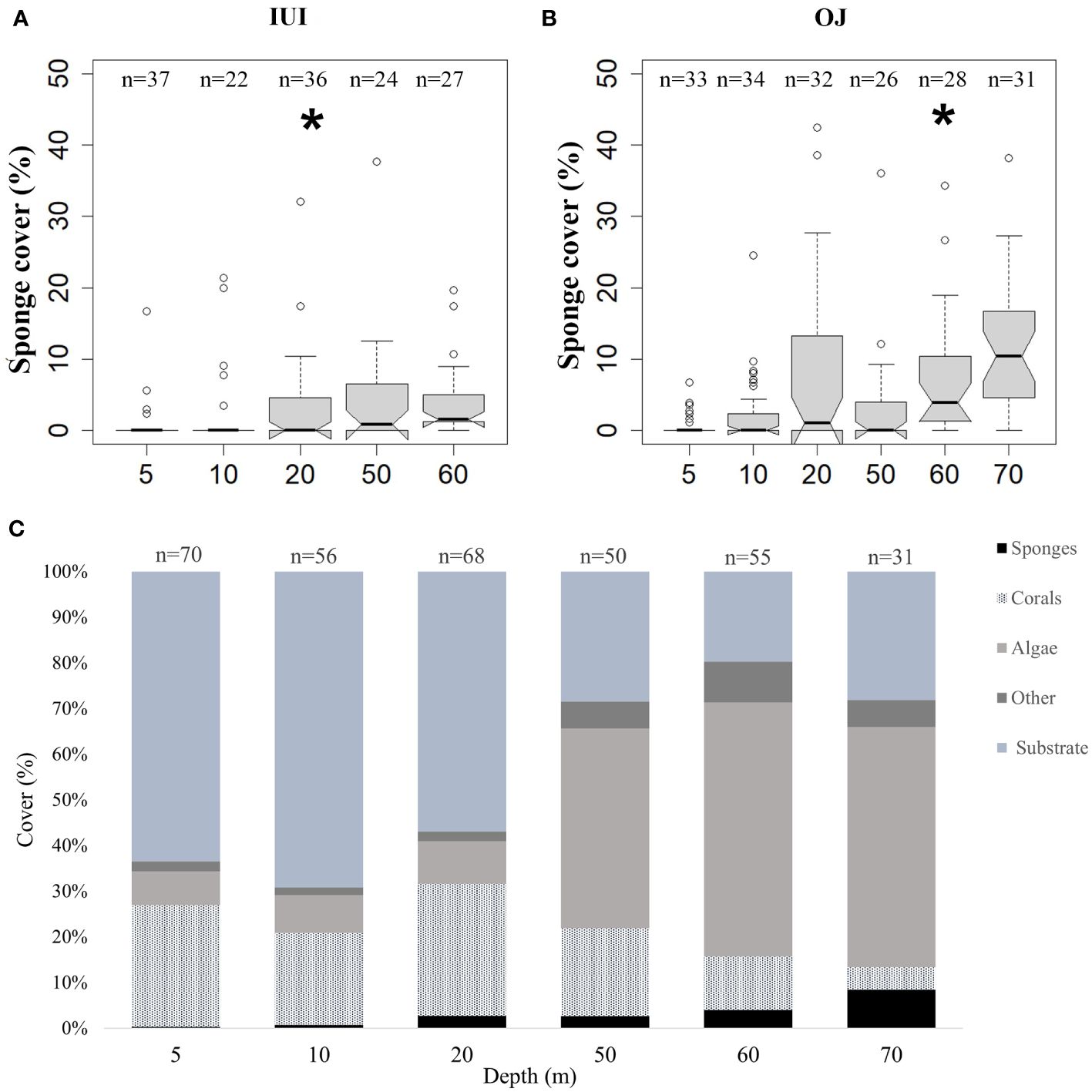
Figure 3 Sponge, corals, algae, other biotic components (“Other”), and substrate coverage (%), across the shallow (5-30 m) and mesophotic (50-70 m) depths at the survey sites (IUI & OJ). (A) Sponge coverage at IUI (permuted ANOVA, F1,144 = 10.44, p<0.05) (B) Sponge coverage at OJ (permuted ANOVA, F1,182 = 36.8, p<0.0001). (C) Mean coverage of sponges, corals, algae, other biotic components (“Other”) and substrate at the shallow and mesophotic depths. Asterisks indicate significant difference. Interquartile range of 25th and 75th percentile of the data is represented by the box. The median value is indicated by the line within the box.
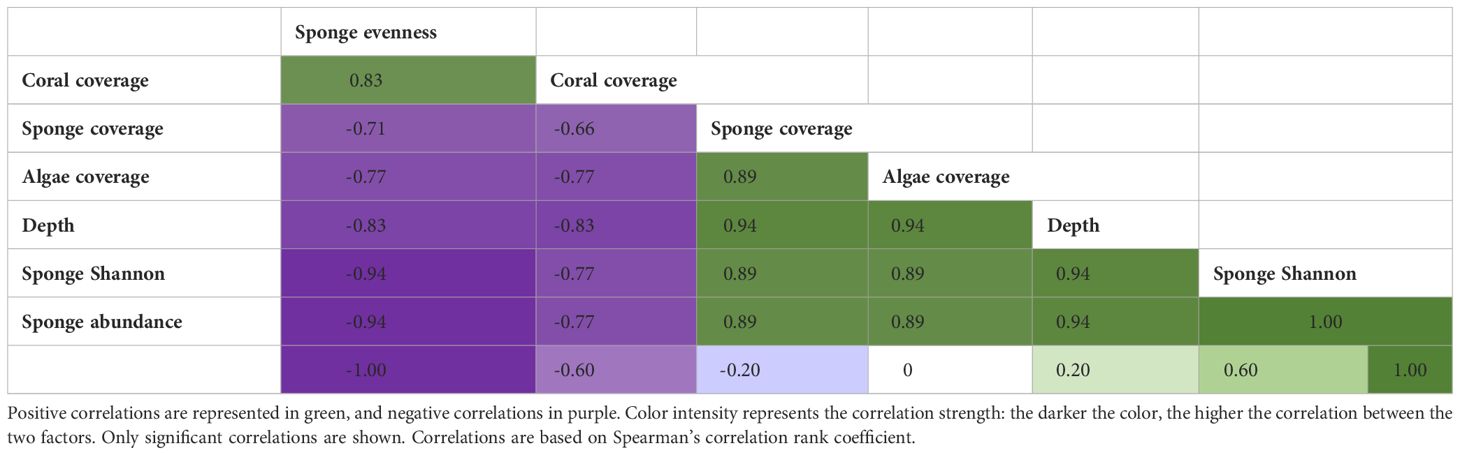
Table 2 Correlation matrix for comparisons between Depth (m), Sponge evenness (Berger-parker index), Sponge coverage, Sponge abundance, Sponge, Shannon index, Algae and Coral coverage.
3.1.2 Sponge community composition
NMDS ordination revealed a clear separation between sites and between mesophotic and shallow communities within each site (PERMANOVA, R2Sites=0.19, R2Sites/Depths=0.39, p<0.01). Site and depth had a strong interaction on the community composition, meaning that at each depth (shallow vs mesophotic), sponge communities clustered according to the site origin rather than to a specific depth (Figure 4). Furthermore, SIMPER analysis revealed that Mycale (Carmia) fistulifera (Row, 1911) and Crella (Grayella) cyathophora (Carter, 1869) explained 24% of the difference between the IUI and OJ sites (Figure 5; Supplementary Table 2), while the morphospecies ‘yellow.gre.enc.thin’ and ‘beige.enc.flower’, which occur only at the mesophotic depths, explained 23% of the difference between the shallow and mesophotic habitats (Figure 5; Supplementary Table 3).
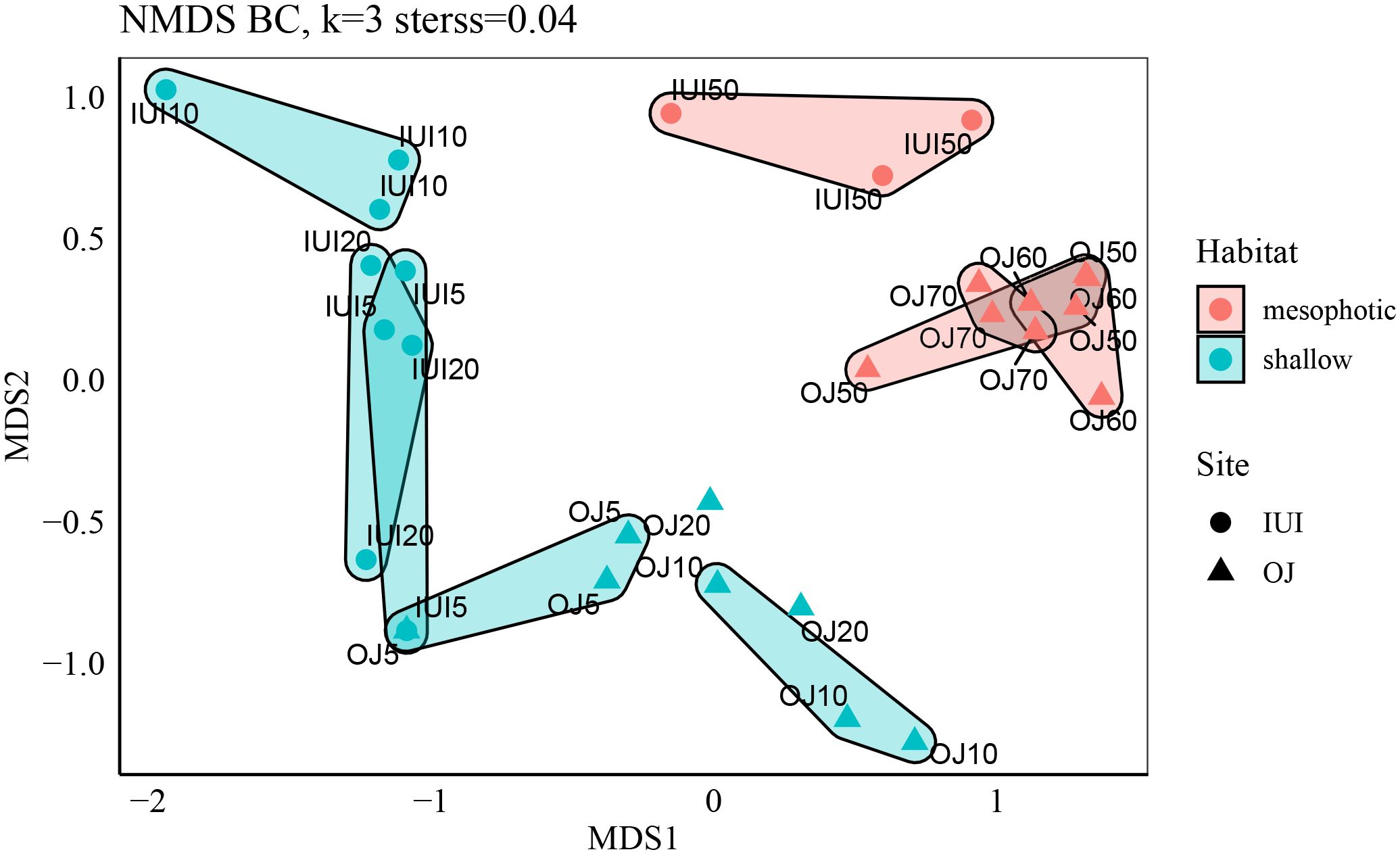
Figure 4 NMDS ordination of mesophotic and shallow sites by species, calculated using Bray Curtis dissimilarity matrix and Hellinger transformation. Sponge species composition significantly differs between depths and sites (IUI vs. OJ, Permutational Multivariate Analysis of Variance (Adonis): Depths R2 = 0.39, p<0.01 sites/depths: R2 = 0.19, p<0.01).
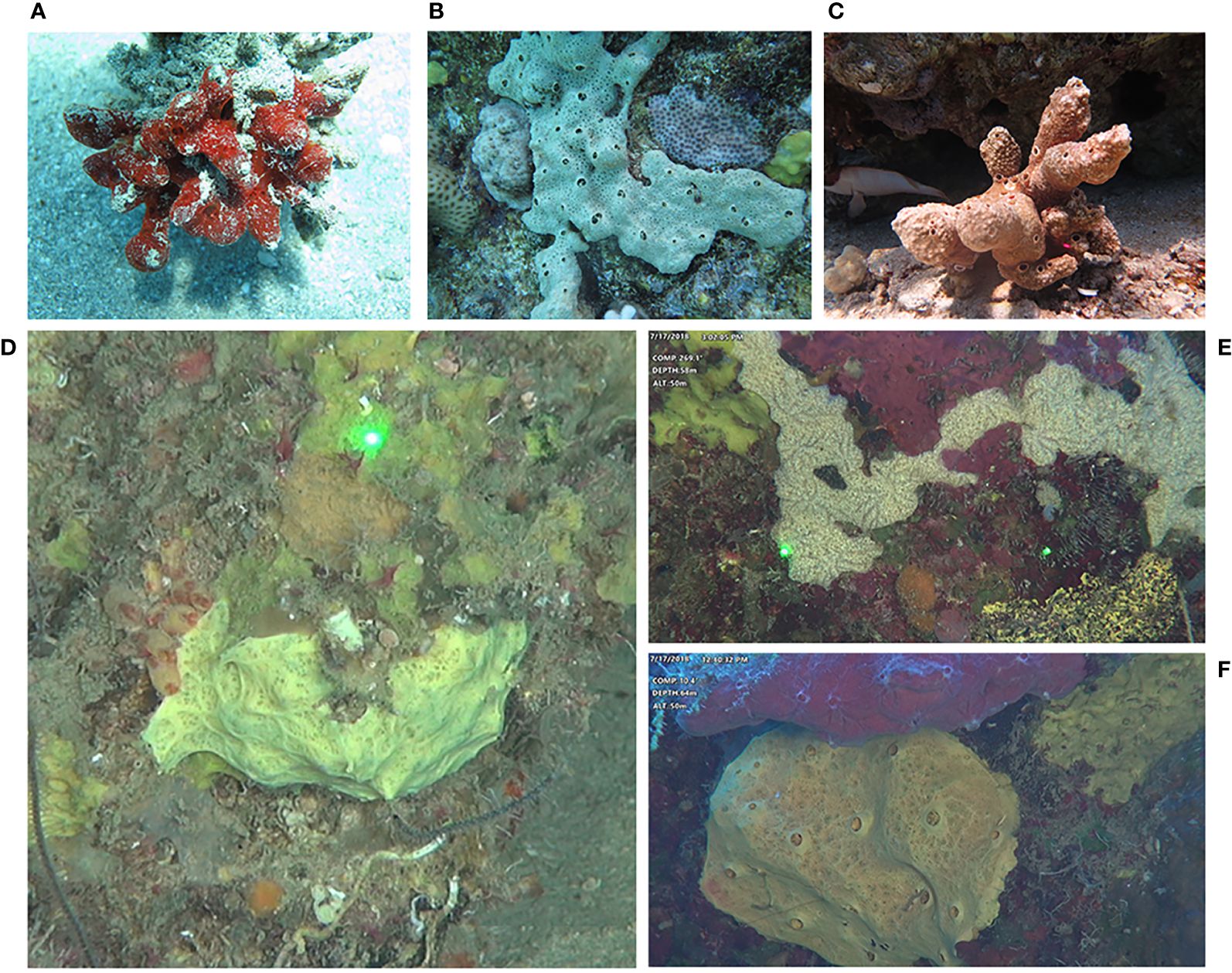
Figure 5 In-situ photographs of the sponge species/morphospecies most contributing to the explained variance in sponge community composition according to SIMPER analysis between sites and depths (shallow vs mesophotic, see also Supplementary Tables 2 and 3). (A) Mycale fistulifera (B) Crella cyathophora (C) Diacarnus erythraeanus (D) ‘Yellow.gre.enc.thin’ morphospecies (E) ‘Beige.enc.flower’ morphospecies (F) ‘Yellow.enc.oscula’ morphospecies.
3.2 Water sampling results
Generally, water parameters were found to be homogenous between sites, depths, and seasons (for inorganic nutrients), except for Prochlorococcus and Synechococcus concentrations (Supplementary Table 4). Prochlorococcus concentration was significantly higher at the mesophotic depths (3.38 ± 0.0 SE µM/L vs. 3.0 ± 0.0 SE µM/L in the shallow, log-transformed data), but was similar between sites. In contrast, Synechococcus concentration was significantly higher at the OJ site compared to the IUI (depths pooled, 4.0 ± 0.0 SE µM/L and 3.8 ± 0.0 SE µM/L, log-transformed respectively) and within each site it was significantly higher at the shallow reef (Supplementary Table 4).
4 Discussion
The current study provides valuable insights into the sponge community structure at mesophotic depths in the Gulf of Aqaba. It demonstrates that sponge coverage and diversity significantly increase with depth and vary between adjacent sites (Figures 2, 3A, B, Table 1; Supplementary Table 1). We also found that the overall sponge community underwent change between sites and within sites at different depths (Figure 4), with the mesophotic reef presenting a flourishing sponge community composed of 40 unique morphospecies. A total of 64 morphospecies/species were found for both shallow and mesophotic reefs together at the two surveyed sites (Supplementary Figure 2). This number, however, is probably an underestimation since many sponge species are cryptic or endolithic and, therefore, invisible to standard visual benthic (especially ROV-dependent) surveys and thus frequently overlooked (Pearman et al., 2018; Timmers et al., 2022). The rarefaction curves in our results also indicate an underestimation of sponge community richness, particularly for the mesophotic depths, where the rarefaction curves were far from saturation (Figure 2E). This suggests that additional sampling would result in higher richness. Another limitation that should be noticed when assessing benthic diversity using orthorectified imagery (such as ROV) is the potential errors due to the variability in the captured images derived from ROV- camera to substate angles (Lesser and Slattery, 2021; Lesser et al., 2023). To address these, our study employed two specific strategies to reduce distortion in ROV imagery. Unlike typical ROV surveys that rely on video-derived orthorectified images, we used photo-quadrats for the mesophotic survey (see methods section). We ensured a consistent perpendicular angle between the camera and the substrate by deploying the ROV on the seafloor, facing vertical substrates, to align the camera horizontally with the target. In steep slope areas like IUI, or under challenging conditions preventing vehicle settlement, we adjusted the ROV and camera angles constantly downwards across the surveyed depth to reduce angular discrepancies. In addition, during image post-processing, we concentrated on the central part of the photo-quadrats where angle distortion is the least. Our results also indicate minimal image bias, as evidenced by the lack of significant differences in most sponge diversity indices and coverage between sites despite their bathymetric variation (Table 1) (Bell et al., 2023).
Finally, our results highlight that site-specific features, such as geomorphology, type of substrate, human influences, and biotic interactions, might be crucial in determining the variability in sponges’ diversity and composition at different depths and sites.
4.1 Sponge abundance and diversity patterns along the shallow to mesophotic depths
Sponge abundance, diversity indices, as well as coverage, were generally significantly higher at the mesophotic depths (Figures 2, 3A, B, Table 1) and were similar at the same depths at the different sites.
In addition, as expected, we found that sponges comprised only a small part (1-4%) of the shallow reef cover, where coral coverage is the main component of the benthic live assemblage (Figure 3C; Supplementary Table 1). Although only two sites were surveyed in this study, we found it to agree with findings of previous studies from the Red Sea (<5 m, Wagler and Brümmer, 2009; Khalil et al., 2017; Eyal et al., 2019), as well as from other Indo-Pacific areas such as Wakatobi Marine National Park, Indonesia (Powell et al., 2010), Moorea, French Polynesia (Freeman and Easson, 2016) and Eastern India (McClanahan et al., 2009). In the above-mentioned studies, sponge coverage was 1-11% while coral coverage was 3%-51%. In contrast, in some reefs off Indonesia, exceptional higher sponge coverage was documented, reaching 29% of the benthic live coverage (Bell and Smith, 2004). Sponge coverage in the Caribbean is also high, with a mean of ~ 16% and a large range of 2.2-74.4% (Loh and Pawlik, 2014). While historical isolation events might have contributed to shaping the difference in the overall biodiversity between these biogeographic regions, local-scale factors, such as geomorphology and differences in biotic interactions, have also been suggested to contribute to the observed differences in sponge distribution patterns (reviewed in Slattery and Lesser, 2012).
Although at the studied sites sponge coverage was generally low, on the mesophotic reef sponges were more dominant and prevalent members of the benthic community, with a mean coverage of 5.5% and 2.6% at the OJ site and IUI site, respectively (Figures 3A, B, Tables 1; Supplementary Table 1). Moreover, in numerous photo-quadrats of the mesophotic reef, sponges exceeded 40% of the live benthic coverage (OJ, 70 m, Figure 3B). Nevertheless, our observations of the relatively low sponge coverage in the mesophotic reef are congruent with the general pattern of sponge coverage in the Indo-Pacific. In the Philippines, for example, sponge coverage (assessed together with other biotic components) was lower than 10% for all the mesophotic sites (Dumalagan et al., 2019). At our study sites, we also found that the encrusting shape to be the dominant morphology of sponges at the mesophotic depths (~70%), which suggests that although sponge coverage is higher at these depths, the total sponge biomass is not necessarily higher in comparison to that in the shallower habitats (Slattery and Lesser, 2012; Scott and Pawlik, 2019).
Previous studies have shown that light conditions, rather than depth solely explain more adequately benthic community changes along shallow to mesophotic gradient. Hence, many benthic communities “break” around 60 m depth, marked by a transition of the typical shallow-reef community to the mesophotic one. The change in benthic composition appears to be primarily driven by diminishing light levels, closely associated with the range of optical depths where surface irradiance reduces from 10% to 1% (Lesser et al., 2019; Laverick et al., 2020). In the Gulf of Aqaba, two distinct coral assemblages were characterized: shallow (<40 m) and mesophotic (40-150 m). Where branching corals predominantly inhabited shallower regions (< 36 m), other coral species (such as Leptoseris glabra and Euphyllia paradivisa) thrived in the upper mesophotic zone (40-80 m), and one coral species, Leptoseris fragilis was typically found at the lower mesophotic zone, exceeding 80 m (Tamir et al., 2019). In the current study, such a pattern is problematic to detect since only the upper-mesophotic depths were surveyed, and most of the shared species between the shallow and the mesophotic were observed down to the upper mesophotic depths limit (that is, 70 m, see results section). Nevertheless, our data show that several photoautotrophic sponges can be found in the lower mesophotic depths (for example Theonella swinhoei (Gray, 1868, 75 m, 87 m) and Crella cyathophora (Carter, 1869, 76 m, 78 m). Hence, it is plausible that these species switch their trophic ecology to rely more on heterotrophic consumption (Morrow et al., 2016; Macartney et al., 2021), enabling these species to extend their distribution into the lower mesophotic depths, beyond the typical patterns observed in coral communities. This assumption is also supported by the increase of sponge richness, abundance, and diversity with depth (Figures 2, 3A, B, Table 1). The last corresponds to the well-studied pattern documented from the Caribbean reefs that indicated an increase in sponge abundance and coverage with depth (Liddell and Ohlhorst, 1988; Reed and Pomponi, 1997; Kahng et al., 2010; Lesser and Slattery, 2011; Slattery and Lesser, 2012; Lesser and Slattery, 2018; Scott et al., 2019). In the Indo-Pacific region, however, the biodiversity of sponges in the MCEs has barely been studied (Loya et al., 2019), other than some data collected from the mesophotic depths in the Gulf of Aqaba (Eyal et al., 2019). A study conducted in Kenting (Taiwan), reported sponges as a common component in the mesophotic habitats, but with no additional quantitative information (Dai et al., 1992). Other studies from the Philippines, Japan and Fiji only reported on the presence of sponges on the mesophotic reef, while occasionally noting a specific species. However, no additional data regarding their abundance, richness or any quantitative data have been reported from these geographic locations (Cabaitan et al., 2019; Pyle, 2019; Sinniger et al., 2019).
Several factors have been attributed to controlling sponge community patterns along the shallow to mesophotic depths. In the Caribbean, for example, an increase in the availability of trophic resources (such as carbon and nitrogen) with depth was suggested to act as a main driver influencing the distribution and abundance of sponges from shallow to mesophotic depths (bottom-up control) (Lesser, 2006; Lesser et al., 2019, 2020). Sponges are remarkable filter-feeders that consume particles as well as dissolved organic matter (DOM) (Ribes et al., 2003; Yahel et al., 2003). Consequently, some studies have speculated that since sponges have greater access to food on the mesophotic reef, this might support a higher sponge growth rate and, therefore, the higher biomass documented at these depths (Lesser and Slattery, 2018). Others, however, argued that there is insufficient evidence to support the theory that sponge biomass, as well as diversity, increase with depth in the Caribbean (e.g., Scott and Pawlik (2019)). It was also suggested that sponge diversity varies at different depths in various locations throughout the Caribbean, and that it is a function of additional factors such as substratum type, slope and orientation, rather than depth per se. In continuation, Pawlik et al. (2018) argued that predation pressure (top-down control) structured by fish and hawksbill turtles is likely to have a significant effect on the relative abundance of sponges in the Caribbean reefs (Pawlik et al., 2013). The findings of the current study indicate a general increase in sponge diversity and coverage (Figures 2, 3A, B). Nevertheless, no difference in the examined water components (e.g. POC, TOC, NOx) was detected between the shallow and mesophotic reefs, with the exception of Prochlorococcus concentration, which was higher at the mesophotic depths (Supplementary Table 4). Our finding of elevated levels of Prochlorococcus in mesophotic depths corroborates similar observations made in Caribbean studies (for example, Lesser et al., 2019, 2020). In contrast, we observed a homogeneous distribution of other water components, such as Particulate Organic Carbon (POC), non-photosynthetic bacteria (BAC), and Nitrogen components (NOx), across both shallow and mesophotic depths. The different water component patterns between our study and the ones reported from the Caribbean may be a result of the distinct water column dynamics at the Gulf of Aqaba, characterized by vertical mixing and restratification. Seasonal water displacement (following cooling of surface waters) along with the impact of semi-diurnal internal tides that cause significant water displacements (up to around 50 m), likely facilitate this mixing process (Carlson et al., 2014). Such dynamics could be instrumental in maintaining the uniformity of water components across various depths, particularly in the 5-50 m range that was the focus of our study.
In addition, given the restricted sampling size and depth range (5 vs. 50 m), solely at two sites, the comparability of these findings with other studies addressing this topic (see Pawlik et al., 2013, 2018) is limited. Consequently, it is challenging to establish concrete conclusions about the effect of bottom-up factors on the distribution patterns of sponges from shallow to mesophotic depths in this case.
Another plausible explanation for the observed increase in sponge diversity metrics from the shallow to mesophotic depths could be driven by biotic interactions between sponges and other benthic components. Such interactions could include competition for space or food, or a positive synergism (Scott et al., 2019; Slattery and Lesser, 2021), or preditation (Pawlik et al., 2018). As for the last, sponge-eating fishes are not prevalent in the Gulf of Aqaba, and signs of sponge-tissue consumption are rare (M. Ilan personal obs). Hence, there is no strong evidence for top-down control in this area. However, other biotic interactions might explain sponge diversity patterns; for example, in the current study, we found that algal coverage also significantly increased from the shallow to the surveyed upper mesophotic depths, reaching ~50% of the total coverage (Figure 3C, Supplementary Table 1). The ability of algae to efficiently adapt to a low light regime (Kirk, 1994; Vroom and Smith, 2001), as found at the mesophotic depth, might have contributed to their dominant occurrence at this depth (Spalding, 2012; Pyle et al., 2016). In addition, algal coverage was positively correlated with sponge abundance and diversity (indicated by the Shannon diversity index; Table 2), further reinforcing the idea that a positive feedback loop might exist between sponges and algae, as previously suggested Pawlik et al. (2016). According to those authors there is a reciprocal interaction between sponges, algae, and microbe, in which the sponges consume dissolved organic carbon (DOC) released by the algae, releasing onto the reef carbon and nutrients that enhance algae growth. These interactions have been suggested to alter microbial activity, which in turn negatively affects the coral microbiomes. This theory was originally posited to explain the low coral resilience and coral decline in the Caribbean coral reefs. In environments with reduced light, such as MCEs, where autotrophic corals face the decreased ability to compete for space, this idea of positive interaction between sponges and algae might also explain the increase in coverage with depth of both sponges and algae and the decrease of corals coverage in the Gulf of Eilat/Aqaba, as also observed recently off the Caribbean reefs in regard to macroalgae and sponge cover (Scott et al., 2019). Our findings also indicate that corals dominate the benthic live cover at shallow depths, where sponge coverage was relatively low, while the opposite pattern emerged with increasing depth, where coral coverage decreased and sponge coverage increased (Figure 3C; Supplementary Table 1). A similar phenomenon was described for Indonesia (Wakatobi Marine National Park), where coral coverage decreased from the shallow to mesophotic depth, while sponge coverage markedly increased (Bell et al., 2019). However, contrary to our observations, crustose coralline algae (CCA) coverage at Wakatobi decreased with depth. The reciprocal coverage trends of corals and algae along the depth gradient reported in our study also agree with those of previous surveys from the Gulf of Eilat/Aqaba. For example, Eyal et al., 2019, reported a general increase in macroalgae coverage from shallow (10 m) to mesophotic (60 m) depths and a decrease in scleractinian coral coverage for the same depths. Similarly, Abesamis et al. (2018) reported that both stony and soft corals were characteristic of shallow sites in the Philippines, while sponges and macroalgae (among other benthic components) were more abundant at the mesophotic sites. Other factors that undergo change along a depth gradient are light intensity and temperature, both of which are also important factors in determining sponge distribution and diversity patterns (Lesser et al., 2018; Kahng et al., 2019; Pyle and Copus, 2019), and were previously shown to have a significant effect on sponge communities worldwide (Beepat et al., 2020; Idan et al., 2020; Vargas et al., 2021). Regarding the coral reefs in the Gulf of Aqaba, only ~1% of the surface light penetrates to the mesophotic depths (30-76 m). Autotrophic sponges, which rely on photosynthetic-carbon products generated by Cyanobacteria as part of their energetic budget, are likely to be significantly affected by this diminished light availability. The reduced light also impacts photosynthetic organisms such as corals and algae, which compete with sponges for the available substrate. Consequently, the lower light levels might favour sponge that can shift to heterotrophic feeding, since the latter do not require light and can thus thrive in these darker environments (Morrow et al., 2016; Lesser and Slattery, 2018; Lesser et al., 2018; Macartney et al., 2021).
4.2 Sponge abundance and diversity patterns between sites
In contrast to the significant increase in sponge diversity metrics from the shallow to mesophotic depths, between the OJ and IUI sites, sponge abundance and diversity indices were similar (Table 1). The sponge community composition however significantly differed between sites (Figure 4, PERMANOVA, p<0.01), as did sponge richness and coverage, which were significantly higher at the OJ site (Table 1). Such differences might be related to a local-scale variance between sites, such as substrate type, slope angle, orientation or rugosity (Barnes and Bell, 2002). The mesophotic reef at the OJ site is composed of large boulders, while that of the IUI is mainly covered with coral rubble (Figures 1B, C). Hence, the OJ site is characterized by a high abundance of vertical walls or inclined substrate, experiencing a relatively low rate of sedimentation, and is therefore favoured by sponges (Maldonado and Young, 1997; Santín et al., 2018). Solar irradiance is also lower on vertical surfaces, inhibiting the growth of macroalgae and other phototrophic organisms and promoting the recruitment of sponges (Lesser et al., 2018). In addition, the rocky boulders at the OJ site offer a more stable substrate than the coral rubble substrate at the IUI, consequently potentially supporting higher sponge coverage and richness compared to the IUI (Duckworth, 2015). Nevertheless, unlike the IUI, which is part of the protected marine nature reserve, the OJ site has been subjected to heavy anthropogenic pressures such as oil spills (Loya and Rinkevich, 1980), intense marine traffic, and metal waste accumulation from nearby port activities. Such anthropogenic interferences typically negatively impact sponge diversity, which flourishes in well-preserved ecosystems (Easson et al., 2015; Turon et al., 2019). Contrary to this, our findings revealed higher sponge richness and coverage at OJ compared to IUI, suggesting that other dominant factors, such as substrate type or flow regime, might play a more crucial role in determining sponge diversity between these sites. Sponge species composition also significantly varied between the OJ and IUI sites (Figures 4, 5). SIMPER analysis indicated that Mycale fistulifera was the sponge species that contributed most to the dissimilarity between these sites (Supplementary Table 2, mean abundance 34% vs. 18%, IUI and OJ, respectively). Interestingly, a study examining the life-history characteristics of M. fistulifera in the 1990s at the same sites found a similar pattern, in which this species was more abundant at the IUI than at the OJ (then referred to as the Japanese Gardens – JG). This difference was attributed to: 1) higher availability of the sponge’s preferred substrate – the coral Stylophora pistillata (Esper, 1792) at the IUI; and 2) higher coral density at the OJ site that led to higher competition, which might have reduced the abundance of M. fistulifera (Meroz and Ilan, 1995b). In contrast, Crella cyathophora was more abundant at the OJ site (31% vs. 0.01% at IUI). C. cyathophora is an opportunistic sponge, thriving on artificial substrates (Perkol-Finkel and Benayahu, 2005) and common at disturbed sites. It is possible that the high level of artificial substrate at the OJ site further facilitated the establishment and expansion of an opportunistic species such as C. cyathophora at this site.
5 Conclusion
The findings from this study add valuable knowledge to the scarce data regarding the distribution and diversity of sponges in the Red Sea in general and in the Gulf of Aqaba in particular. In the current study, only two sites were surveyed, yet they reflect the findings from other benthic studies from the Indo-Pacific region, indicating that visible sponge coverage and abundance are much lower in this region than in the Caribbean reefs. However, as recently shown, the contribution of the sponge community in the reef cryptic habitats in the northern Red Sea greatly expands the overall benthic community diversity (Van Hoytema et al., 2023). This study, which is the first to assess the diversity, abundance and species composition of mesophotic sponges in the Red Sea, has revealed that the mesophotic reef at the surveyed sites features a unique and distinct sponge community. Our findings indicate that both local factors, such as site characteristics (geomorphology, substrate type, anthropogenic influences, etc.), and biotic interactions, may play an essential role in shaping the differences in sponge diversity and community composition between depths and sites.
Finally, the findings from this study emphasize the importance of investigating previously overlooked habitats, such as those at the mesophotic depths, which hold promise for encountering novel species as well as ecological or pharmacological functions, and may also act as biodiversity reservoirs in an increasingly dynamic world. This latter possibility is even more crucial considering the global threats to coral-reef biodiversity and functioning, in the face of future anthropogenic and climate-driven changes.
Data availability statement
The raw data supporting the conclusions of this article will be made available by the authors, without undue reservation.
Ethics statement
The manuscript presents research on animals that do not require ethical approval for their study.
Author contributions
LR-N: Conceptualization, Data curation, Formal Analysis, Investigation, Methodology, Project administration, Visualization, Writing – original draft, Writing – review & editing. LG: Formal Analysis, Methodology, Project administration, Writing – review & editing. SS: Conceptualization, Investigation, Methodology, Project administration, Writing – review & editing. MI: Conceptualization, Funding acquisition, Investigation, Project administration, Supervision, Writing – review & editing.
Funding
The author(s) declare that financial support was received for the research, authorship, and/or publication of this article. This research was supported by the ISF-NSFC joint research program (Grant No. 2577/18 to MI). LR-N was supported by a Lev Tzion scholarship from the Israeli Council for Higher Education. LR-N received the Inter University Institute for Marine Science in Eilat fellowship for doctoral students.
Acknowledgments
The authors wish to express their profound gratitude to the members of Ilan’s lab for their invaluable support in conducting the fieldwork, in both dives and cruises, aboard the research vessel. We thank N. Paz for scientific editorial assistance and Y. Shmuel for the assistance with collecting the water samples. We acknowledge T. Rivlin and I. Kolesnikov for their support in the water chemical analyses. We appreciate G. Yahel’s lab members for their advice regarding water collection and analyses. Our special thanks to the staff of the R/V Sam Rothberg, the IUI diving center staff, and the Interuniversity Institute for Marine Sciences in Eilat (IUI) for their continuous assistance and provision of access to facilities. The authors also acknowledge the environmental NGO EcoOcean and EDT personnel for providing and operating the Remotely Operated Vehicle that enabled the mesophotic sponge surveys. Finally, we extend our sincere gratitude to the reviewers for their valuable contributions which have significantly enhanced the quality of this manuscript.
Conflict of interest
The authors declare that the research was conducted in the absence of any commercial or financial relationships that could be construed as a potential conflict of interest.
Publisher’s note
All claims expressed in this article are solely those of the authors and do not necessarily represent those of their affiliated organizations, or those of the publisher, the editors and the reviewers. Any product that may be evaluated in this article, or claim that may be made by its manufacturer, is not guaranteed or endorsed by the publisher.
Supplementary material
The Supplementary Material for this article can be found online at: https://www.frontiersin.org/articles/10.3389/fmars.2024.1370089/full#supplementary-material
References
Abesamis R. A., Langlois T., Birt M., Thillainath E., Bucol A. A., Arceo H. O., et al. (2018). Benthic habitat and fish assemblage structure from shallow to mesophotic depths in a storm-impacted marine protected area. Coral Reefs 37, 81–97. doi: 10.1007/s00338-017-1635-0
Abràmoff M. D., Magalhães P. J., Ram S. J. (2004). Image processing with imageJ. Biophotonics Int. 11, 36–42.
Anderson M. J. (2001). A new method for non-parametric multivariate analysis of variance. Austral Ecol. 26 (1), 32–46. doi: 10.1111/j.1442-9993.2001.01070.pp.x
Anderson M. J., Walsh D. C. (2013). PERMANOVA, ANOSIM, and the Mantel test in the face of heterogeneous dispersions: what null hypothesis are you testing? Ecol. Monogr. 83, 557–574. doi: 10.1890/12-2010.1
Auguie B., Antonov A., Auguie M. B. (2017) Package ‘gridExtra’. Miscellaneous functions for “Grid” Graphics. Available online at: https://cran.r-.org/web/packages/gridExtra/index.html.
Baetge N., Graff J. R., Behrenfeld M. J., Carlson C. A. (2020). Net community production, dissolved organic carbon accumulation, and vertical export in the Western North Atlantic. Front. Mar. Sci. 7. doi: 10.3389/fmars.2020.00227
Barnes D. K., Bell J. J. (2002). Coastal sponge communities of the West Indian Ocean: morphological richness and diversity. Afr. J. Ecol. 40, 350–359. doi: 10.1046/j.1365-2028.2002.00388.x
Beepat S. S., Davy S. K., Woods L., Bell J. J. (2020). Short-term responses of tropical lagoon sponges to elevated temperature and nitrate. Mar. Environ. Res. 157, 104922. doi: 10.1016/j.marenvres.2020.104922
Bell J., Jompa J., Haris A., Werorilangi S., Shaffer M., Mortimer C. (2019). Domination of mesophotic ecosystems in the Wakatobi Marine National Park (Indonesia) by sponges, soft corals and other non-hard coral species. J. Mar. Biol. Assoc. U. K. 99, 771–775. doi: 10.1017/S0025315418000917
Bell J. J., McGrath E., Biggerstaff A., Bates T., Cárdenas C. A., Bennett H. (2015). Global conservation status of sponges. Biol. Conserv. 29, 42–53. doi: 10.1111/cobi.12447
Bell J. J., Micaroni V., Strano F., Broadribb M., Wech A., Harris B., et al. (2023). Testing the impact of Remotely Operated Vehicle (ROVs) camera angle on community metrics of temperate mesophotic organisms: A 3D model-based approach. Ecol. Inform. 76, 102041. doi: 10.1016/j.ecoinf.2023.102041
Bell J. J., Smith D. (2004). Ecology of sponge assemblages (Porifera) in the Wakatobi region, south-east Sulawesi, Indonesia: richness and abundance. J. Mar. Biol. Assoc. U. K. 84, 581–591. doi: 10.1017/S0025315404009580h
Benayahu Y., Loya Y. (1981). Competition for space among coral-reef sessile organisms at Eilat, Red Sea. Bull. Mar. Sci. 31, 514–522.
Bongaerts P., Riginos C., Brunner R., Englebert N., Smith S. R., Hoegh-Guldberg O. (2017). Deep reefs are not universal refuges: reseeding potential varies among coral species. Sci. Adv. 3, e1602373. doi: 10.1126/sciadv.1602373
Bongaerts P., Smith T. B. (2019). “Beyond the “Deep reef refuge” Hypothesis: A conceptual framework to characterize persistence at depth",” in Mesophotic coral ecosystems. Eds. Loya Y., Puglise K. A., Bridge T. C. L. (Springer International Publishing, Cham), 881–895.
Cabaitan P. C., Quimpo T. J. R., Dumalagan E. E., Munar J., Calleja M. A. C., Olavides R. D. D., et al. (2019). “The Philippines,” in Mesophotic coral ecosystems. Eds. Loya Y., Puglise K. A., Bridge T. C. L. (Springer International Publishing, Cham), 265–284.
Carlson D. F., Fredj E., Gildor H. (2014). The annual cycle of vertical mixing and restratification in the Northern Gulf of Eilat/Aqaba (Red Sea) based on high temporal and vertical resolution observations. Deep Sea Res. Part I: Oceanographic Res. Papers 84, 1–17. doi: 10.1016/j.dsr.2013.10.004
Carter H. J. (1869). On grayella cyathophora, a new genus and species of sponges. Ann. Mag. Nat. Hist. 4 (21), 189–197.
Cayuela L., Gotelli N., Colwell R. K. (2015). Ecological and biogeographic null hypotheses for comparing rarefaction curves. Ecol. Monogr. 85.3, 437–455. doi: 10.1890/14-1261.1
Dai C.-F., Stewart L., Cooper R., Sprunk H. (1992). Distribution of substrates and macrobenthos at depths between 35 and 120 m in southern Taiwan. Acta Oceanographica Taiwanica. 28), 1–18.
De Goeij J. M., Van Oevelen D., Vermeij M. J., Osinga R., Middelburg J. J., de Goeij A. F., et al. (2013). Surviving in a marine desert: the sponge loop retains resources within coral reefs. Science 342, 108–110. doi: 10.1126/science.1241981
Diaz M. C., Rützler K. (2001). Sponges: an essential component of Caribbean coral reefs. Bull. Mar. Sci. 69, 535–546.
Duckworth A. R. (2015). Substrate type affects the abundance and size of a coral-reef sponge between depths. Mar. Freshw. Res. 67, 246–255. doi: 10.1071/MF14308
Dumalagan J. E. E., Cabaitan P. C., Bridge T. C., Go K. T., Quimpo T. J. R., Olavides R. D. D., et al. (2019). Spatial variability in benthic assemblage composition in shallow and upper mesophotic coral ecosystems in the Philippines. Mar. Environ. Res. 150, 104772. doi: 10.1016/j.marenvres.2019.104772
Easson C. G., Matterson K. O., Freeman C. J., Archer S. K., Thacker R. W. (2015). Variation in species diversity and functional traits of sponge communities near human populations in Bocas del Toro, Panama. PeerJ. 3, e1385. doi: 10.7717/peerj.1385
Ellis J., Anlauf H., Kürten S., Lozano-Cortés D., Alsaffar Z., Cúrdia J., et al. (2017). Cross shelf benthic biodiversity patterns in the Southern Red Sea. Sci. Rep. 7, 1–14. doi: 10.1038/s41598-017-00507-y
Erpenbeck D., Voigt O., Al-Aidaroos A. M., Berumen M. L., Büttner G., Catania D., et al. (2016). Molecular biodiversity of Red Sea demosponges. Mar. pollut. Bull. 105, 507–514. doi: 10.1016/j.marpolbul.2015.12.004
Esper E. J.C. (1788-1830). “Die Pflanzenthiere in Abbildungen nach der Natur mit Farben erleuchtet nebst Beschreibungen,” in Raspischen Buchhandlung, Nuremberg. 3, 2.
Eyal G., Laverick J. H., Ben-Zvi O., Brown K. T., Kramer N., Tamir R., et al. (2022). Selective deep water coral bleaching occurs through depth isolation. Sci. Total Environ. 844, 157180. doi: 10.1016/j.scitotenv.2022.157180
Eyal G., Tamir R., Kramer N., Eyal-Shaham L., Loya Y. (2019). “"The red sea: Israel”,” in Mesophotic coral ecosystems. Eds. Loya Y., Puglise K. A., Bridge T. C. L. (Springer International Publishing, Cham), 199–214.
Fiore C. L., Jutte P. C. (2010). Characterization of macrofaunal assemblages associated with sponges and tunicates collected off the southeastern United States. Invertebr. Biol. 129, 105–120. doi: 10.1111/j.1744-7410.2010.00184.x
Freeman C. J., Easson C. G. (2016). Sponge distribution and the presence of photosymbionts in Moorea, French Polynesia. PeerJ. 4, e1816. doi: 10.7717/peerj.1816
Frossard J., Renaud O. (2021). Permutation tests for regression, ANOVA, and comparison of signals: the permuco package. J. Stat. Software 99, 1–32. doi: 10.18637/jss.v099.i15
Garcia-Sais J. R. (2010). Reef habitats and associated sessile-benthic and fish assemblages across a euphotic–mesophotic depth gradient in Isla Desecheo, Puerto Rico. Coral Reefs. 29, 277–288. doi: 10.1007/s00338-009-0582-9
Gasbarro R., Sowers D., Margolin A., Cordes E. E. (2022). Distribution and predicted climatic refugia for a reef-building cold-water coral on the southeast US margin. Glob. Change Biol. 28, 7108–7125. doi: 10.1111/gcb.16415
Genin A., Lazar B., Brenner S. (1995). Vertical mixing and coral death in the Red Sea following the eruption of Mount Pinatubo. Nature. 377, 507–510. doi: 10.1038/377507a0
Glynn P. W. (1996). Coral reef bleaching: facts, hypotheses and implications. Glob. Change Biol. 2, 495–509. doi: 10.1111/j.1365-2486.1996.tb00063.x
Goren L., Idan T., Shefer S., Ilan M. (2021). Macrofauna inhabiting massive demosponges from shallow and mesophotic habitats along the Israeli Mediterranean Coast. Front. Mar. Sci. 7. doi: 10.3389/fmars.2020.612779
Gray J. E. (1868). Note on Theonella, a new genus of coralloid sponges from Formosa. Proc. Zool. Soc. Lond. 3, 565–566. doi: 10.3389/fmars.2020.612779
Helmy T., Van Soest R. (2005). Amphimedon species (Porifera: Niphatidae) from the Gulf of Aqaba, Northern Red Sea: Filling the gaps in the distribution of a common pantropical genus. Zootaxa 859, 18. doi: 10.11646/zootaxa.859.1.1
Hooper J. N., Van Soest R. W. (2002). “Systema Porifera. A guide to the classification of sponges,” in Systema porifera (Springer, Boston, MA), 1–7. doi: 10.1007/978-1-4615-0747-5
Hughes T. P., Baird A. H., Bellwood D. R., Card M., Connolly S. R., Folke C., et al. (2003). Climate change, human impacts, and the resilience of coral reefs. Science. 301, 929–933. doi: 10.1126/science.1085046
Idan T., Goren L., Shefer S., Ilan M. (2020). Sponges in a changing climate: Survival of Agelas oroides in a warming Mediterranean Sea. Front. Mar. Sci. 7. doi: 10.3389/fmars.2020.603593
Ilan M., Gugel J., Van Soest R. (2004). Taxonomy, reproduction and ecology of new and known Red Sea sponges. Sarsia: North Atlantic Mar. Science. 89, 388–410. doi: 10.1080/00364820410002659
Kahng S. E., Akkaynak D., Shlesinger T., Hochberg E. J., Wiedenmann J., Tamir R., et al. (2019). “Light, temperature, photosynthesis, heterotrophy, and the lower depth limits of mesophotic coral ecosystems” in Mesophotic coral ecosystems. Eds. Loya Y., Puglise K. A., Bridge T. C. L. (Springer International Publishing, Cham), 801–828.
Kahng S., Copus J., Wagner D. (2014). Recent advances in the ecology of mesophotic coral ecosystems (MCEs). COSUST 7, 72–81. doi: 10.1016/j.cosust.2013.11.019
Kahng S., Copus J. M., Wagner D. (2017). Mesophotic coral ecosystems. Mar. Anim. Forests. 10, 978–973. doi: 10.1007/978-3-319-21012-4_4
Kahng S., Garcia-Sais J., Spalding H., Brokovich E., Wagner D., Weil E., et al. (2010). Community ecology of mesophotic coral reef ecosystems. Coral Reefs. 29, 255–275. doi: 10.1007/s00338-010-0593-6
Kassambara A., Kassambara M. A. (2020) Package ‘ggpubr’. R package version 0.1 6. Available online at: https://CRAN.R-project.org/package=ggpubr.
Katz T., Ginat H., Eyal G., Steiner Z., Braun Y., Shalev S., et al. (2015). Desert flash floods form hyperpycnal flows in the coral-rich Gulf of Aqaba, Red Sea. EPSL 417, 87–98. doi: 10.1016/j.epsl.2015.02.025
Khalil M. T., Bouwmeester J., Berumen M. L. (2017). Spatial variation in coral reef fish and benthic communities in the central Saudi Arabian Red Sea. PeerJ. 5, e3410. doi: 10.7717/peerj.3410
Kirk J. T. (1994). Light and photosynthesis in aquatic ecosystems (Cambridge university press). doi: 10.1017/CBO9780511623370
Kramer N., Eyal G., Tamir R., Loya Y. (2019). Upper mesophotic depths in the coral reefs of Eilat, Red Sea, offer suitable refuge grounds for coral settlement. Sci. Rep. 9, 1–12. doi: 10.1038/s41598-019-38795-1
Laverick J. H., Tamir R., Eyal G., Loya Y. (2020). A generalized light-driven model of community transitions along coral reef depth gradients. Glob. Ecol. Biogeogr 29, 1554–1564. doi: 10.1111/geb.13140
Lesser M. P. (2006). Benthic–pelagic coupling on coral reefs: feeding and growth of Caribbean sponges. J. Exp. Mar. Bio. Ecol. 328, 277–288. doi: 10.1016/j.jembe.2005.07.010
Lesser M. P., Mueller B., Pankey M. S., Macartney K. J., Slattery M., De Goeij J. M. (2020). Depth-dependent detritus production in the sponge, Halisarca caerulea. Limnol. Oceanogr. 65, 1200–1216. doi: 10.1002/lno.11384
Lesser M. P., Slattery M. (2011). Phase shift to algal dominated communities at mesophotic depths associated with lionfish (Pterois volitans) invasion on a Bahamian coral reef. Biol. Invasions. 13, 1855–1868. doi: 10.1007/s10530-011-0005-z
Lesser M. P., Slattery M. (2018). Sponge density increases with depth throughout the Caribbean. Ecosphere. 9, e02525. doi: 10.1002/ecs2.2525
Lesser M., Slattery M. (2021). Mesophotic coral reef community structure: the constraints of imagery collected by unmanned vehicles. Mar. Ecol. Prog. Ser. 663, 229–236. doi: 10.3354/meps13650
Lesser M. P., Slattery M., Laverick J. H., Macartney K. J., Bridge T. C. (2019). Global community breaks at 60 m on mesophotic coral reefs. Glob. Ecol. Biogeogr. 28, 1403–1416. doi: 10.1111/geb.12940
Lesser M. P., Slattery M., Macartney K. J. (2023). Quantifying sponge communities from shallow to mesophotic depths using orthorectified imagery. Mar. Biol. 170, 111. doi: 10.1007/s00227-023-04258-5
Lesser M. P., Slattery M., Mobley C. D. (2018). Biodiversity and functional ecology of mesophotic coral reefs. Annu. Rev. Ecol. Evol. 49, 49–71. doi: 10.1146/annurev-ecolsys-110617-062423
Liddell W. D., Ohlhorst S. L. (1988). Hard substrata community patterns, 1-120 M, North Jamaica. Palaios 3 (4), 413–423. doi: 10.2307/3514787
Loh T. -L., Pawlik J. (2014). Chemical defenses and resource trade-offs structure sponge communities on Caribbean coral reefs. Proc. Natl. Acad. Sci. U. S. A., 111. doi: 10.1073/pnas.1321626111
Loiseau N., Villéger S., Le Bozec C., Gimenez M., Kawahara S. L., Claverie T. (2023). Mesophotic reefs are not refugia for neither taxonomic nor functional diversity of reef fishes. Coral Reefs. 42, 63–75. doi: 10.1007/s00338-022-02311-1
Loya Y. (2004). “The coral reefs of Eilat—past, present and future: three decades of coral community structure studies,” in Coral health and disease (Springer, Berlin, Heidelberg) 3(2), 1–34. doi: 10.3354/meps003167
Loya Y., Eyal G., Treibitz T., Lesser M. P., Appeldoorn R. (2016). Theme section on mesophotic coral ecosystems: advances in knowledge and future perspectives. Coral Reefs 35, 1–9. doi: 10.1007/s00338-016-1410-7
Loya Y., Puglise K. A., Bridge T. C. (2019). Mesophotic coral ecosystems (New York: Springer). doi: 10.1007/978-3-319-92735-0
Loya Y., Rinkevich B. (1980). Effects of oil pollution on coral reef communities. Mar. Ecol. Prog. Se. 3 (2), 167–180. doi: 10.3354/meps003167
Lozada-Misa P., Schumacher B., Vargas-Ángel B. (2017). “Analysis of benthic survey images via CoralNet: a summary of standard operating procedures and guidelines,” in U.S. Department of Commerce, National Oceanic and Atmospheric Administration, National Marine Fisheries Service, Pacific Islands Fisheries Science Center. doi: 10.7289/V5/AR-PIFSC-H-17-02
Macartney K. J., Slattery M., Lesser M. P. (2021). Trophic ecology of Caribbean sponges in the mesophotic zone. AIOL. 66, 1113–1124. doi: 10.1002/lno.11668
Maldonado M., Young C. (1997). Bathymetric patterns of sponge distribution on the Bahamian slope. Oceanogr. Lit. Rev. 4, 368. doi: 10.1016/0967-0637(96)00042-8
Mangiafico S., Mangiafico M. S. (2017) Package ‘rcompanion’. Available online at: https://CRAN.R-project.org/package=rcompanion.
McClanahan T. R., Muthiga N. A., Maina J., Kamukuru A. T., Yahya S. A. (2009). Changes in northern Tanzania coral reefs during a period of increased fisheries management and climatic disturbance. Aquat. Conserv.: Mar. Freshw. 19, 758–771. doi: 10.1002/aqc.1020
Medeiros A. P., Ferreira B. P., Alvarado F., Betancur-R R., Soares M. O., Santos B. A. (2021). Deep reefs are not refugium for shallow-water fish communities in the southwestern Atlantic. Ecol. Evol. 11, 4413–4427. doi: 10.1002/ece3.7336
Menza C., Kendall M., Hile S. (2008). The deeper we go the less we know. Rev. Biol. Trop. 56, 11–24. doi: 10.15517/rbt.v56i0.5575
Meroz E., Ilan M. (1995a). Cohabitation of a coral reef sponge and a colonial scyphozoan. Mar. Biol. 124, 453–459. doi: 10.1007/BF00363919
Meroz E., Ilan M. (1995b). Life history characteristics of a coral reef sponge. Mar. Biol. 124, 443–451. doi: 10.1007/BF00363918
Morganti T., Yahel G., Ribes M., Coma R. (2016). VacuSIP, an improved InEx method for in situ measurement of particulate and dissolved compounds processed by active suspension feeders. JoVE. 114), e54221. doi: 10.3791/54221-v
Morrow K. M., Fiore C. L., Lesser M. P. (2016). Environmental drivers of microbial community shifts in the giant barrel sponge, Xestospongia muta, over a shallow to mesophotic depth gradient. Environ. Microbiol. 18, 2025–2038. doi: 10.1111/1462-2920.13226
Oksanen J., Blanchet F. G., Kindt R., Legendre P., Minchin P. R., O’hara R., et al. (2013) Package ‘vegan’. Community ecology package, version. Available online at: https://CRAN.R-project.org/package=vegan.
Pawlik J. R., Burkepile D. E., Thurber R. V. (2016). A vicious circle? Altered carbon and nutrient cycling may explain the low resilience of Caribbean coral reefs. Bioscience. 66, 470–476. doi: 10.1093/biosci/biw047
Pawlik J. R., Loh T.-L., McMurray S. E. (2018). A review of bottom-up vs. top-down control of sponges on Caribbean fore-reefs: what’s old, what’s new, and future directions. PeerJ. 6, e4343. doi: 10.7717/peerj.4343
Pawlik J. R., Loh T.-L., McMurray S. E., Finelli C. M. (2013). Sponge communities on Caribbean coral reefs are structured by factors that are top-down, not bottom-up. PloS One 8, e62573. doi: 10.1371/journal.pone.0062573
Pearman J. K., Leray M., Villalobos R., Machida R., Berumen M. L., Knowlton N., et al. (2018). Cross-shelf investigation of coral reef cryptic benthic organisms reveals diversity patterns of the hidden majority. Sci. Rep. 8, 1–17. doi: 10.1038/s41598-018-26332-5
Perkol-Finkel S., Benayahu Y. (2005). Recruitment of benthic organisms onto a planned artificial reef: shifts in community structure one decade post-deployment. Mar. Environ. Res. 59, 79–99. doi: 10.1016/j.marenvres.2004.03.122
Powell A. L., Hepburn L. J., Smith D. J., Bell J. J. (2010). Patterns of sponge abundance across a gradient of habitat quality in the Wakatobi Marine National Park, Indonesia. Open J. Mar. Sci. 4, 31–38.
Pyle R. L. (2019). “Fiji,” in Mesophotic coral ecosystems. Eds. Loya Y., Puglise K. A., Bridge T. C. L. (Springer International Publishing, Cham), 369–385.
Pyle R. L., Boland R., Bolick H., Bowen B. W., Bradley C. J., Kane C., et al. (2016). A comprehensive investigation of mesophotic coral ecosystems in the Hawaiian Archipelago. PeerJ. 4, e2475. doi: 10.7717/peerj.2475
Pyle R. L., Copus J. M. (2019). “Mesophotic coral ecosystems: introduction and overview,” in Mesophotic coral ecosystems. Eds. Loya Y., Puglise K. A., Bridge T. C. L. (Springer International Publishing, Cham), 3–27.
Reed J., Pomponi S. (1997). “Biodiversity and distribution of deep and shallow water sponges in the Bahamas,” in Proc 8th Int Coral Reef Symp, 1387-1392.
Ribes M., Coma R., Atkinson M., Kinzie III, R.A. (2003). Particle removal by coral reef communities: picoplankton is a major source of nitrogen. Mar. Ecol. Prog. Ser. 257, 13–23. doi: 10.3354/meps257013
Rix L., de Goeij J. M., Van Oevelen D., Struck U., Al-Horani F. A., Wild C., et al. (2018). Reef sponges facilitate the transfer of coral-derived organic matter to their associated fauna via the sponge loop. Mar. Ecol. Prog. Ser. 589, 85–96. doi: 10.3354/meps12443
Row R. H. (1911). Reports on the marine biology of the Sudanese Red Sea.—XIX. Report on the sponges collected by Mr. Cyril Crossland in 1904-5. Part II. Non-Calcarea. Zool. J. Linn. Soc 31, 287–400. doi: 10.1111/zoj.1911.31.issue-208
RStudio, T (2020). RStudio: integrated development for R (Boston, MA: Rstudio Team, PBC). Available at: http://www.rstudio.com.
Rützler K., Macintyre I. G. (1978). Siliceous sponge spicules in coral reef sediments. Mar. Biol. 49, 147–159. doi: 10.1007/BF00387114
Santín A., Grinyó J., Ambroso S., Uriz M. J., Gori A., Dominguez-Carrió C., et al. (2018). Sponge assemblages on the deep Mediterranean continental shelf and slope (Menorca Channel, Western Mediterranean Sea). Deep Sea Res. Part I: Deep-Sea Res. I: Oceanogr. Res. 131, 75–86. doi: 10.1016/j.dsr.2017.11.003
Scott A. R., Battista T. A., Blum J. E., Noren L. N., Pawlik J. R. (2019). Patterns of benthic cover with depth on Caribbean mesophotic reefs. Coral Reefs. 38, 961–972. doi: 10.1007/s00338-019-01824-6
Scott A. R., Pawlik J. R. (2019). A review of the sponge increase hypothesis for Caribbean mesophotic reefs. Mar. Biodivers. 49, 1073–1083. doi: 10.1007/s12526-018-0904-7
Shihavuddin A. S. M.,, Gracias N., Garcia R., Gleason A. C., Gintert B. (2013). Image-based coral reef classification and thematic mapping. Remote Sens 5 (4), 1809–1841. doi: 10.3390/rs5041809
Shlesinger T., Loya Y. (2019). Breakdown in spawning synchrony: A silent threat to coral persistence. Science 365, 1002–1007. doi: 10.1126/science.aax0110
Sinniger F., Ballantine D., Bejarano I., Colin P., Pochon X., Pomponi S., et al. (2016). “Biodiversity of mesophotic coral ecosystems,” in Mesophotic coral ecosystems—a lifeboat for coral reefs? (The United Nations Environment Programme and GRID-Arendal, Nairobi and Arendal), ISBN: 978-82-7701-150-9. 98 p.
Sinniger F., Harii S., Humblet M., Nakamura Y., Ohba H., Prasetia R. (2019). “Ryukyu islands, Japan,” in Mesophotic coral ecosystems. Eds. Loya Y., Puglise K. A., Bridge T. C. L. (Springer International Publishing, Cham), 369–385.
Slattery M., Lesser M. (2012). “Mesophotic coral reefs: a global model of community structure and function,” in Proceedings of the 12th International Coral Reef Symposium. 9–13, (ICRS Cairns).
Slattery M., Lesser M. P. (2021). Gorgonians are foundation species on sponge-dominated mesophotic coral reefs in the caribbean. Front. Mar. Sci. 8. doi: 10.3389/fmars.2021.654268
Slattery M., Lesser M., Brazeau D., Stokes M., Leichter J. (2011). Connectivity and stability of mesophotic coral reefs. J. Exp. Mar. Bio. Ecol. 408, 32–41. doi: 10.1016/j.jembe.2011.07.024
Slattery M., Moore S., Boye L., Whitney S., Woolsey A., Woolsey M. (2018). The Pulley Ridge deep reef is not a stable refugia through time. Coral Reefs. 37, 391–396. doi: 10.1007/s00338-018-1664-3
Slowikowski K., Schep A., Hughes S., Lukauskas S., Irisson J.-O., Kamvar Z. N., et al. (2018) Package ggrepel. Automatically position non-overlapping text labels with ‘ggplot2. Available online at: https://github.com/slowkow/ggrepel.
Smith T. B., Gyory J., Brandt M. E., Miller W. J., Jossart J., Nemeth R. S. (2016). Caribbean mesophotic coral ecosystems are unlikely climate change refugia. Glob. Change Biol. 22, 2756–2765. doi: 10.1111/gcb.13175
Spalding H. L. (2012). Ecology of mesophotic macroalgae and Halimeda kanaloana meadows in the main Hawaiian Islands (Honolulu: University of Hawaii at Manoa).
Sturm A. B., Eckert R. J., Carreiro A. M., Voss J. D. (2022). Population genetic structure of the broadcast spawning coral, Montastraea cavernosa, demonstrates refugia potential of upper mesophotic populations in the Florida Keys. Coral Reefs 41, 587–598. doi: 10.1007/s00338-021-02112-y
Tamir R. (2015). Spatial and seasonal dynamics of the light field in the Gulf of Aqaba (Eilat). Bar-Ilan University, Israel.
Tamir R., Eyal G., Kramer N., Laverick J. H., Loya Y. (2019). Light environment drives the shallow-to-mesophotic coral community transition. Ecosphere 10, e02839. doi: 10.1002/ecs2.2839
Tamir R., Lerner A., Haspel C., Dubinsky Z., Iluz D. (2017). The spectral and spatial distribution of light pollution in the waters of the northern Gulf of Aqaba (Eilat). Sci. Rep. 7, 1–10. doi: 10.1038/srep42329
Team, R. C (2018) Package stats. The R Stats Package. Available online at: https://CRAN.R-project.org/package=STAT.
Team, R. C (2020) A language and environment for statistical computing (Vienna, Austria: R Foundation for Statistical Computing). Available online at: www.r-project.org (Accessed Febuary 14, 2019).
Timmers M. A., Vicente J., Webb M., Jury C. P., Toonen R. J. (2022). Sponging up diversity: Evaluating metabarcoding performance for a taxonomically challenging phylum within a complex cryptobenthic community. Environ. DNA 4, 239–253. doi: 10.1002/edn3.163
Turon M., Cáliz J., Triadó-Margarit X., Casamayor E. O., Uriz M. J. (2019). Sponges and their microbiomes show similar community metrics across impacted and well-preserved reefs. Front. Microbiol. 10. doi: 10.3389/fmicb.2019.01961
Van Hoytema N., de Goeij J. M., Kornder N. A., El-Khaled Y., Van Oevelen D., Rix L., et al. (2023). A carbon cycling model shows strong control of seasonality and importance of sponges on the functioning of a northern Red Sea coral reef. Coral Reefs, 1–15. doi: 10.1007/s00338-022-02339-3
Van Soest R. W., De Voogd N. J. (2018). Calcareous sponges of the western Indian Ocean and Red Sea. Zootaxa. 4426, 1–160-161–160. doi: 10.11646/zootaxa.4426.1.1
Vargas S., Leiva L., Wörheide G. (2021). Short-term exposure to high-temperature water causes a shift in the microbiome of the common aquarium sponge Lendenfeldia chondrodes. Microb. Ecol. 81, 213–222. doi: 10.1007/s00248-020-01556-z
Voigt O., Erpenbeck D., González-Pech R. A., Al-Aidaroos A. M., Berumen M. L., Wörheide G. (2017). Calcinea of the Red Sea: providing a DNA barcode inventory with description of four new species. Mar. Biodivers. 47, 1009–1034. doi: 10.1007/s12526-017-0671-x
Vroom P. S., Smith C. M. (2001). The challenge of siphonous green algae. Am. Sci. 89, 525–531. doi: 10.1511/2001.6.524
Wagler M., Brümmer F. (2009). “Competitive interactions of sponges and corals in the Gulf of Aqaba” in 1st international workshop research in shallow marine and fresh water systems, vol. 21 (FOG - Freiberg Online Geoscience), 26–31.
Wheeler B., Torchiano M., Torchiano M. M. (2016) Package ‘lmPerm’. R package version. Available online at: https://CRAN.R-project.org/package=lmPerm.
Wickham H. (2016). ggplot2: elegant graphics for data analysis (New York: Springer-Verlag). doi: 10.1007/978-3-319-24277-4
Wooster M. K., Voigt O., Erpenbeck D., Wörheide G., Berumen M. L. (2019). “Sponges of the red sea,” in Coral reefs of the red sea, vol. 11 . Eds. Voolstra C., Berumen M. (Springer, Cham).
World Porifera Database (2024). Available online at: https://www.marinespecies.org/porifera/ (Accessed March 04 2024).
Wulff J. (2001). Assessing and monitoring coral reef sponges: why and how? Bull. Mar. Sci. 69, 831–846.
Yahel G., Post A. F., Fabricius K., Marie D., Vaulot D., Genin A. (1998). Phytoplankton distribution and grazing near coral reefs. Limnol. Oceanogr. 43, 551–563. doi: 10.4319/lo.1998.43.4.0551
Keywords: sponges, mesophotic, sponge community structure, sponge coverage, diversity, Red Sea, coral reefs, MCEs
Citation: Raijman-Nagar L, Goren L, Shefer S and Ilan M (2024) Sponge abundance and diversity patterns in the shallow and mesophotic reefs of the northern Red Sea. Front. Mar. Sci. 11:1370089. doi: 10.3389/fmars.2024.1370089
Received: 13 January 2024; Accepted: 08 April 2024;
Published: 30 April 2024.
Edited by:
Manuel Maldonado, Spanish National Research Council (CSIC), SpainReviewed by:
Andreu Santin Muriel, Spanish National Research Council (CSIC), SpainMarc Slattery, University of Mississippi, United States
Joseph R. Pawlik, University of North Carolina Wilmington, United States
Copyright © 2024 Raijman-Nagar, Goren, Shefer and Ilan. This is an open-access article distributed under the terms of the Creative Commons Attribution License (CC BY). The use, distribution or reproduction in other forums is permitted, provided the original author(s) and the copyright owner(s) are credited and that the original publication in this journal is cited, in accordance with accepted academic practice. No use, distribution or reproduction is permitted which does not comply with these terms.
*Correspondence: Lilach Raijman-Nagar, ralilah2@gmail.com