Escarpments within Mediterranean seagrass Posidonia oceanica meadows increase habitat heterogeneity and structural complexity enhancing fish diversity and biomass
- 1Centre d’Estudis Avançats de Blanes, Consejo Superior de Investigaciones Científicas, Blanes, Spain
- 2BIOSFERA, Research and Conservation, Girona, Spain
- 3Universitat de Barcelona, Barcelona, Spain
- 4School of Science and Centre for Marine Ecosystems Research, Edith Cowan University, Joondalup, WA, Australia
Seagrass meadows provide important ecosystem services including carbon sequestration, coastal protection from erosion, and sustained biodiversity and fisheries thereby improving the wellbeing and livelihoods of coastal communities. The erosion of millenary deposits of intertwined roots and rhizomes of Posidonia results in the formation of three-dimensional structures named escarpments that constitute a biogenic reef habitat. However, the natural history of seagrass escarpments including their formation processes and their role as habitat for reef fauna and flora remains poorly understood. This research located and characterized Posidonia oceanica escarpments in Menorca (Balearic Islands) and compared structural complexity and fish assemblages among seagrass escarpments, seagrass meadows, rocky substrates and bare sand with emphasis on its role as habitat and shelter for typical rocky fish. Fish abundance and biomass were similar between seagrass escarpments and rocky substrates (P > 0.05), but significantly lower in seagrass meadows (P < 0.001). The large number of caves found along seagrass escarpments provide shelter to fish, including species only associated to rocky substrates. Seagrass meadows form a rather homogenous habitat within their canopy, but the presence of seagrass escarpments enhances habitat heterogeneity and structural complexity along with fish abundance and biomass at the seascape level. This study enhances understanding on the ecological importance of seagrass escarpments.
1 Introduction
Seagrass meadows are considered one of the most productive ecosystems on the planet and providers of important benefits for human well-being, including carbon sequestration, protection from coastal erosion and reservoir of biodiversity (Boudouresque and Meinesz, 1983; Rappe, 2010; Cullen-Unsworth and Unsworth, 2013; Nordlund et al., 2018). Seagrasses form dense meadows providing habitat for a variety of organisms, sustaining complex food webs that maintain high levels of marine biodiversity through nutritional and structural functions (Duffy, 2006; Morrison et al., 2014; Hyman et al., 2019). Hundreds of species live as epiphytes on seagrass leaves and rhizomes, whereas others find shelter within their canopy (Boudouresque, 1974; Ballesteros, 1987, 1992; Templado et al., 2004; Piazzi et al., 2016). In particular, large numbers of juvenile fish find shelter within the meadows compared with nearby bare substrates (Heck and Orth, 1980; Bell and Harmelin-Vivien, 1982; Pollard, 1984; Rozas and Odum, 1988; Francour, 1997; Guidetti, 2000). Previous studies revealed more than 600 different species in one-hectare of seagrass meadow including gastropods, bivalves, cephalopods, tunicates, echinoderms, fish and algae (Boudouresque et al., 2012; Piazzi et al., 2016). Meiofauna is also an important, yet often overlooked, component of biodiversity in seagrass meadows (Bell et al., 1984), with a total of 672 species found in Posidonia oceanica meadows (García-Gómez et al., 2022). Scientific evidence suggests that 20% of commercial fish species rely on seagrass as resident or occasional inhabitants throughout their life cycles (Ambo-Rappe et al., 2013; Jänes et al., 2020). The high biodiversity supported by the meadows includes a large number of protected, endangered, charismatic and commercially important species (Larkum et al., 2006; Santos de Moura and Vianna, 2020), thereby seagrass conservation contributes to protect marine biodiversity and support food provision to humans (Morrison et al., 2014).
Posidonia oceanica is an endemic species in the Mediterranean Sea that ranks amongst the most productive seagrass species, and forms highly complex habitats that contain micro-niches for multitude of taxonomic groups (Boudouresque, 2004; Piazzi et al., 2016; Bellisario et al., 2019; Dressel, 1899; Boudouresque, 1974; Boudouresque and Meinesz, 1983; Blas Aritio, 1990; Corbera et al., 1996; Francour, 1997; Ferreira et al., 2001; Charbonnel et al., 2002; Bodilis et al., 2003; Boudouresque, 2004; Comas, 2004; Contreras et al., 2006; Duffy, 2006; Boström et al., 2011; Boudouresque et al., 2012; Fourqurean et al., 2012; Friedman et al., 2012; Cullen-Unsworth and Unsworth, 2013; Bonhomme et al., 2015; Bussotti et al., 2018; García-Gómez et al., 2022). Seagrasses grow vertically to avoid burial, which can result in soil elevation rates ranging from 1 to 10 cm yr-1 that result in the formation of peat-like sedimentary deposits that can reach several meters in thickness (Mateo et al., 1997). This structure consisting of live and dead root and rhizome debris embedded within a mineral matrix that fills the interstices, is called matte (Pérès and Picard, 1975). Due to the persistent bottom currents, heavy storms, and human activity within coastal areas, portions of the meadows can be eroded revealing the organic-rich matte in the form of seagrass escarpments (i.e., blowouts and reef-like formations; Patriquin, 1975; Bonhomme et al., 2015). It seems likely that the increased frequency and magnitude of storm surges predicted with climate change could enhance the formation of seagrass escarpments in the near future. Seagrass escarpments are structurally complex formations with cavities, ledges and crevices that constitute a distinct habitat from the typically monotonous seagrass canopy. Dead rhizomes exposed along the escarpments are colonized by algae, other seagrass species including Cymodocea nodosa, bryozoans and ascidians, among others (Tomasello et al., 2009; Piazzi et al., 2016).
The structural complexity and habitat heterogeneity of marine seascapes is a key factor affecting abundance and richness of taxa, including fish assemblages (Reñones et al., 1997; Kaiser et al., 1998; Ferreira et al., 2001). The habitat structural complexity is the result of the surface topography of the substrata and the organisms that grow on it (e.g., coral, kelp or seagrass). Many studies on coral reefs revealed relationships between habitat structural complexity and abundance and richness of fish assemblages (Ferreira et al., 2001; Charbonnel et al., 2002; Gratwicke and Speight, 2005). Similarly, the fish abundance and diversity is driven by the three-dimensional topography of seagrass habitats (Thiriet et al., 2014; Máñez-Crespo et al., 2022), together with habitat heterogeneity (Murphey and Fonseca, 1995; Hyndes et al., 2003; Boström et al., 2011). Previous studies showed that the presence of escarpments within P. oceanica, P. australis, and Thalassia testudinum increase the heterogeneity of the meadows and likely enhances fish biomass and diversity (Macia and Robinson, 2005; Serrano et al., 2016, 2020). However, the influence of escarpments within seagrass meadows on the abundance and biodiversity of fish assemblages remains unexplored.
In this study, we characterize the location, dimensions, and morphology of P. oceanica escarpments along the north and south regions of Menorca Island (Spain), together with the number and volume of cavities within seagrass escarpments. In addition, with the study of fish assemblages in P. oceanica escarpments, seagrass meadows, sandy and rocky substrates we decipher the role of escarpments as habitat for fish. It is hypothesized that fish abundance, biomass, and diversity in seagrass escarpments will be similar to that of rocky substrates, and higher than in seagrass meadows and sandy habitats due to the lower structural complexity in the latter. This study aims at enhancing current knowledge on marine phanerogams and thereby, to promote ocean and seagrass conservation.
2 Materials and methods
2.1 Study area
This study was conducted in Menorca (Balearic Islands, North-western Mediterranean). Menorca became a Biosphere Reserve by UNESCO in 1993 for its natural landscape and great biodiversity (Menorca Biosphere Reserve, 2020). The coastline of Menorca is relatively well preserved with large terrestrial and marine protected areas (Comas, 2004). Most of the perimeter of the island is fringed by seagrass meadows where P. oceanica and C. nodosa species coexist in very shallow waters, and P. oceanica thrive in deeper waters down to 35 m. The northern coastline is very irregular with steep cliffs and large sheltered bays, whereas the southern coastline is straight with rocky cliffs and narrow coves (Llompart and Rosell, 2002). Sampling on the northern side of the island took place in shallow waters (between 1 to 7 m) within small, sheltered coves, whereas on the southern side sampling was performed at deeper depths (between 15 to 18 m).
A total of five study sites were sampled, with three sites (Sanitja, S’Estany and Sa Torreta) located on the northern side of the island, and two sites (Cala Galdana and Son Bou) located on the southern side of the island (Figure 1). Within each study site, fish surveys and structural complexity were assessed in each habitat type: seagrass meadow, seagrass escarpment, sand, and rocky substrates, except for Sanitja site where suitable rocky substrates and bare sand habitat were not found. Escarpments sitting in front or on top of visible rock were not sampled.
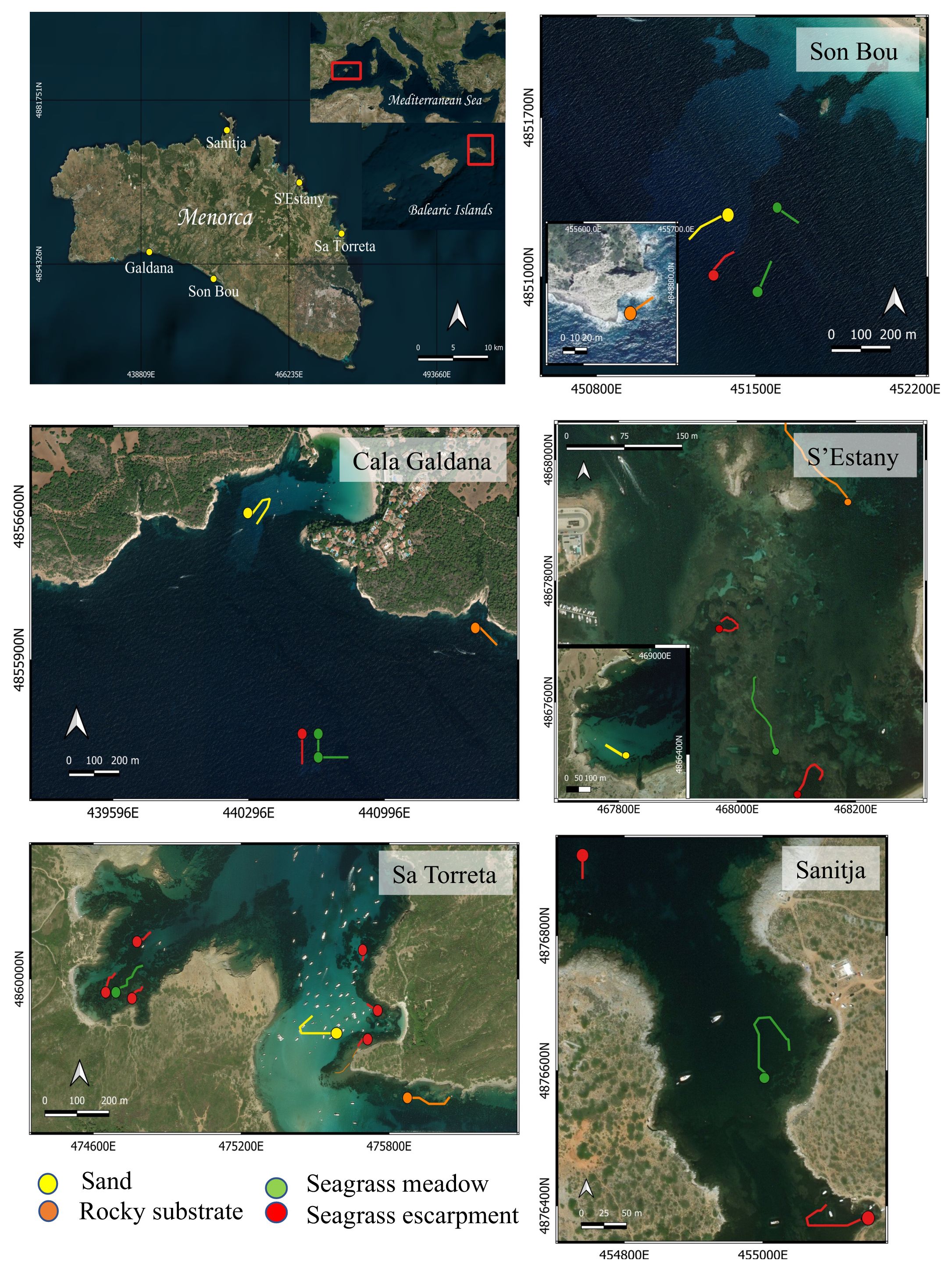
Figure 1 Map of sampling sites in the southern (Cala Galdana and Son Bou) and northern coastline (Sanitja, S’Estany and Sa Torreta) of Menorca. The transects within each habitat type (seagrass meadow, seagrass escarpment, sand, and rocky substrates) are indicated in colors.
2.2 Visual fish census
We performed visual fish surveys using SCUBA in May 2022 following standard procedures (Harmelin-Vivien et al., 1985; Giakoumi et al., 2012). All fish data were collected by E.B. and E.G. For seagrass meadows, rocky substrates and bare sand within each site, at least three transects of 50 m were conducted, with a total 23 transects in P. oceanica seagrass meadows, 12 transects in bare sand, and 12 transects in rocky substrates. The transect length in seagrass escarpments varied from 10 to 50 m owing to the scarcity of long escarpments in some study sites, with a total of 18 transects in P. oceanica seagrass escarpments (Supplementary Table 1). We excluded bare sand habitat from further assessments because fish counts equaled zero in all 12 transects, which in part could be linked to the lack shelter in bare sand that results in the fishes remaining beyond the observer sight.
At each transect, the diver laid out a 50 m line-transect and simultaneously identified, counted, and sized all fish (at 5 cm intervals) (La Mesa and Vacchi, 1999). The observer swam at a constant speed (~10 min/50 m transect) and all fish passing within 2 m from each side of the transect in seagrass meadows and rocky substrates were recorded, whereas at seagrass escarpments fish were recorded within 1.5 m from the escarpment wall. Transects were randomly positioned in seagrass meadows and rocky bottoms but followed the escarpment contour in the escarpments. All censuses were conducted between 10 am to 4 pm to minimize time effects on fish behavior and fish counts.
We assigned fish species to a trophic category based on diet (Moranta et al., 2006) and trophic level (Pauly and Froese, 2006): herbivores, macrophagous, mesophagous, microphagous, and omnivores (Supplementary Table 2). The biomass of each fish species was estimated using allometric length–weight relationship based on FishBase (Pauly and Froese, 2006) and Morey et al. (2003): W = a * (TL^b), where parameters a and b are species-specific constants, TL is total length in cm, and W is weight in grams. Parameters a and b were selected from the study conducted in the nearest site to our study area (Morey et al., 2003). Fish abundance and total fish biomass were calculated for each transect and trophic category.
2.3 Habitat structural complexity
For each transect within each habitat where fish surveys were conducted, the seafloor structural complexity was estimated. The chain rugosity method (Hill and Wilkinson, 2004; Guidetti et al., 2016); was used for seagrass meadows, sand and rocky habitats, whereas the height and cavity volume were measured along the escarpments to estimate the habitat complexity. A 30 m chain was deployed three times along each transect to measure structural complexity, by deploying the chain carefully over the undulating substrate in a straight line and registering the real lineal length used. Chain surface rugosity index was calculated by dividing the 30 m real chain length by the linear distance covered (Friedman et al., 2012). In seagrass escarpment habitats, the escarpment height was measured every 5 m, together with the width, height and depth of all cavities and ledges with an opening greater than 20 cm in diameter. The volume of cavities was estimated using the formula of a pyramid (V = (1/3) * w * d * h; where w is width, d is depth and h is height), whereas the volume of ledges was estimated using the formula of a rectangular prism (V = w * h * l; where w is width, h is height and l is length). The structural complexity in seagrass escarpments was estimated using an area-based surface rugosity index, by dividing the three-dimensional topography by the projected planar area (Friedman et al., 2012). The three-dimensional topography is the result of the sum of the area of the escarpment (i.e., transect length multiplied by the average escarpment height), plus the area of cavities and ledges. The projected planar area was estimated as the area of the escarpment (i.e., transect length multiplied by the average escarpment height).
2.4 Statistical analyses
Differences in fish assemblage assessments between the two observers were discarded based on the lack of significant differences in nine duplicate fish surveys (t-test; P = 0.064). Data on fish abundance, total number of species, fish biomass and Shannon´s diversity Index (H’) were standardized per unit area (m2). Differences on fish abundance (number of fish m-2), total number of species (number of species m-2), total biomass (g m-2), Shannon´s diversity Index (H’), biomass of fish size categories (at 5 cm intervals), trophic categories (macrophagous, mesophagous, microphagous, omnivorous and herbivorous), and structural complexity among habitats were assessed using univariate non-parametric analysis of variance (PERMANOVA), which allows heteroscedasticity of samples and non-normality of the residuals. Data from each trophic category and fish size were square root transformed, and the resemblance matrix for each variable was computed using Euclidean distance resemblance matrix. Sum of squares type III (partial) was applied after 9999 permutations of residuals under a reduced model. Any significant factors were analyzed using post-hoc pair-wise tests. In the experimental design, study site was considered as a random factor and habitat type (n = 3; seagrass meadow, seagrass escarpment, rocky substrate) was considered a fixed factor.
Differences in fish species, trophic categories, and fish size categories among habitats were also assessed using multivariate PERMANOVA. Data were square root transformed to down-weight the influence of highly abundant species, and the Bray-Curtis similarity matrix was used. PERMANOVA post-hoc pair-wise tests were conducted on significant factors. To visualize the role of fish species and trophic category in driving differences in fish biomass among habitats, Principal Coordinate Analyses (PCO) were computed (Anderson et al., 2009). Pearson correlation was used to assess potential relationships between habitat structural complexity, fish abundance, fish biomass and Shannon´s diversity Index among habitats. All statistical analyses were performed using PRIMER v6 statistical software with add-in PERMANOVA (Anderson et al., 2009).
3 Results
Fish assemblages and structural complexity were surveyed along 2,911 lineal meters across 63 transects at the five study sites in the coast of Menorca (Supplementary Table 1). A total of 2,727 fish individuals belonging to 33 fish species from 10 families were recorded, with 1,568 individual fish reported in rocky substrates, 683 in seagrass escarpments and 476 in seagrass meadows. Of the trophic categories identified, 102 were classified as macrophagous 1,295 as mesophagous, 288 as omnivorous, 120 as herbivorous, and 922 as microphagous (Supplementary Table 2).
3.1 Differences in fish abundance, biomass and diversity among habitats
Significant effects of the factor habitat type (seagrass meadow, seagrass escarpment, rocky substrate) on fish abundance, total number of species, total fish biomass, and Shannon´s diversity Index were detected (P < 0.001; Supplementary Table 3). Total fish abundance was similar between rocky substrates (mean ± SE; 0.65 ± 0.07 fish m-2) and escarpments (0.78 ± 0.19 fish m-2; P = 0.739), but up to 5-fold higher than in seagrass meadows (0.10 ± 0.02 fish m-2; P < 0.001; Figure 2A). Similarly, total fish biomass was comparable between rocky substrates (11.66 ± 3.03 g m-2) and seagrass escarpments (5.17 ± 1.28 g m-2; P = 0.059), but 18-fold higher than in seagrass meadows (0.64 ± 0.15 g m-2; P < 0.001; Figure 2B). On the other hand, total number of fish species and Shannon´s diversity Index were significantly higher in rocky substrates (0.053 ± 0.003 species m-2; H’ = 1.73 ± 0.06, respectively) than in seagrass escarpments (0.024 ± 0.002 species m-2; H’ = 1.08 ± 0.07, respectively; Figure 2C). In addition, total number of species and Shannon´s diversity Index in seagrass escarpments were significantly higher than in seagrass meadows (0.016 ± 0.001 species m-2; H’ = 0.82 ± 0.06, respectively; Figure 2D).
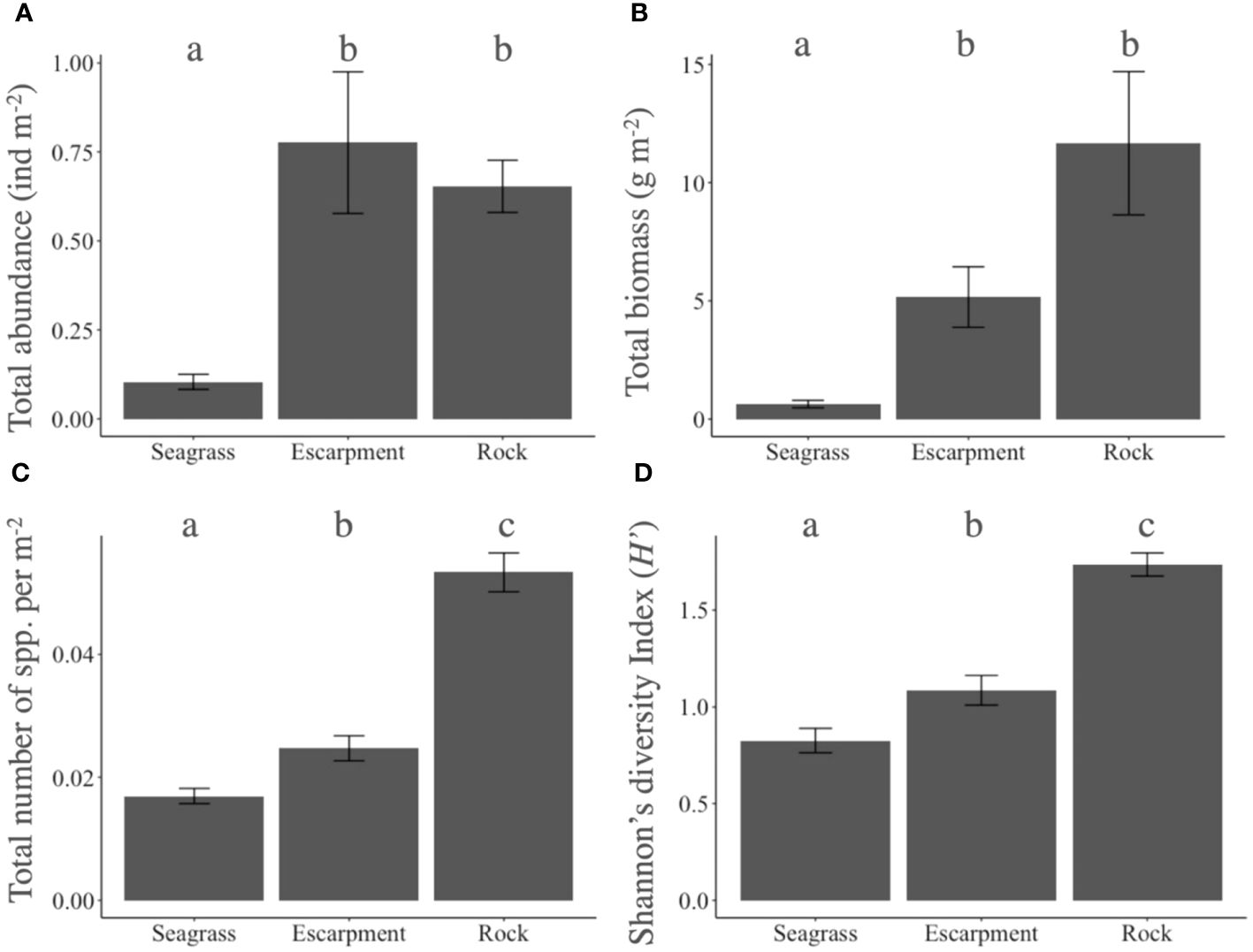
Figure 2 Mean ± standard error of (A) total fish abundance (number of fish m-2), (B) total fish biomass (g m-2), (C) total number of species (number of species m-2) and (D) Shannon diversity Index (H’) in seagrass meadows, seagrass escarpments and rocky substrates. Significant differences among habitat types are denoted with letters a–c.
3.2 Differences in fish biomass among trophic and size categories, fish species among habitats
Significant differences in fish biomass among habitats were observed for all size categories (P < 0.001; Supplementary Table 3). Fish biomasses of small size categories 0–5, 5–10 and 10–15 cm were significantly higher in seagrass escarpments (0.05 ± 0.02, 2.54 ± 0.72 and 1.41 ± 0.37 g m-2, respectively) and rocky substrates (0.05 ± 0.02, 1.39 ± 0.13 and 2.32 ± 0.36 g m-2, respectively) compared to seagrass meadows (0.006 ± 0.002, 0.23 ± 0.07 and 0.33 ± 0.13 g m-2, respectively). On the other hand, fish biomass of size category 15–20 cm was significantly higher in rocky substrates (2.17 ± 0.71 g m-2) compared to seagrass meadows and escarpments (0.07 ± 0.03 and 0.59 ± 0.33 g m-2, respectively). Fish biomass of size category 20–25 cm was significantly higher in rocky substrates (2.81 ± 1.43 g m-2) than in seagrass escarpments (0.13 ± 0.10 g m-2), whereas biomass of fish size 25–30 cm was similar between rocky substrates and seagrass escarpments (1.74 ± 1.32 and 0.46 ± 0.35 g m-2; respectively; P = 0.238). Fish larger than 20 cm in size were absent in seagrass meadows (Supplementary Table 4; Figure 3A).
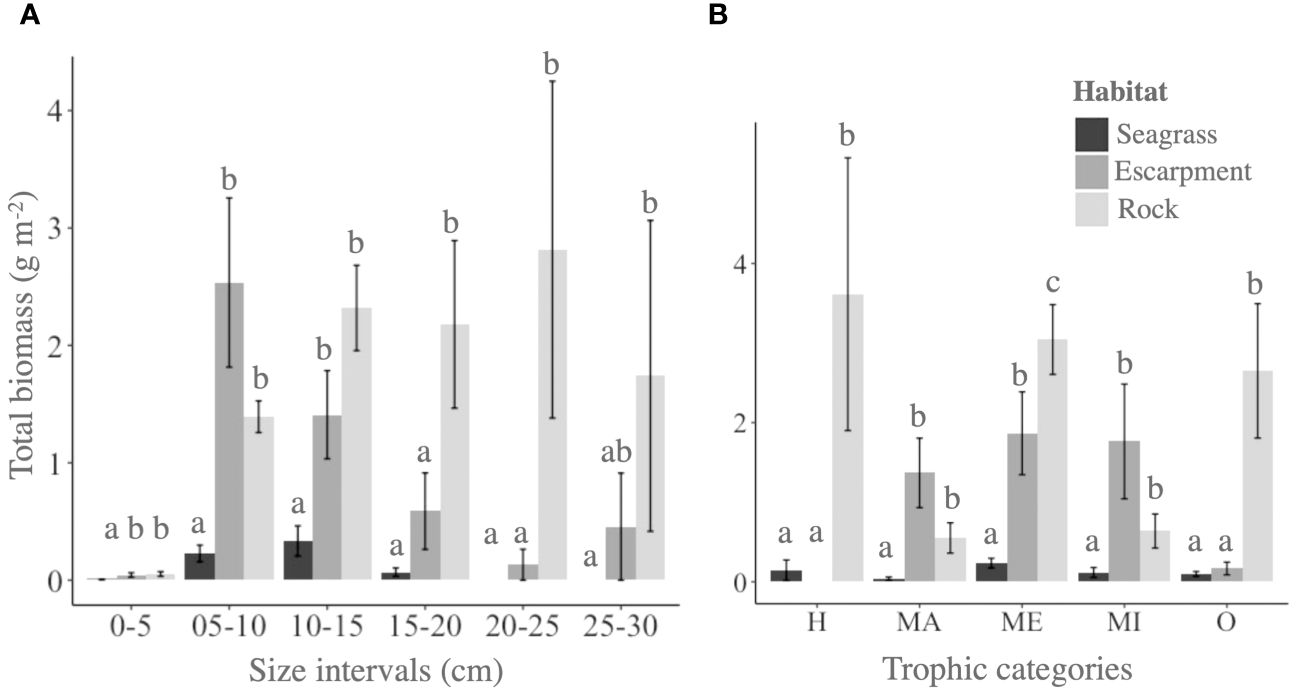
Figure 3 Total biomass (g m-2) of (A) fish length size categories (in 5 cm intervals from 0 to 30 cm) and (B) trophic categories (herbivores, H; macrophagous, MA; mesophagous, ME; microphagous, MI; and omnivores) across all fish species surveyed across habitat types: seagrass meadows, seagrass escarpments and rocky substrates. Significant differences among habitat types are denoted with letters a–c.
Significant differences in fish biomass among habitats were observed for each trophic category (P < 0.003; Supplementary Table 3). Macrophagous and microphagous fish biomasses were significantly higher in seagrass escarpments (1.37 ± 0.44 and 1.76 ± 0.72 g m-2, respectively) and rocky substrates (0.55 ± 0.19 and 0.64 ± 0.21 g m-2, respectively) compared to seagrass meadows (0.04 ± 0.02 and 0.12 ± 0.06 g m-2, respectively). On the other hand, omnivorous and herbivorous biomasses were significantly higher in rocky substrates (2.65 ± 0.84 and 3.61 ± 1.71 g m-2, respectively) compared to seagrass escarpments (0.17 ± 0.08 g m-2 omnivorous biomass whereas herbivorous fish were absent) and seagrass meadows (0.10 ± 0.08 and 0.14 ± 0.13 g m-2, respectively). Mesophagous fish biomass was significantly lower in seagrass meadows (0.23 ± 0.06 g m-2) than in seagrass escarpments (1.87 ± 0.52 g m-2), and significantly higher in rocky substrates (3.04 ± 0.44 g m-2) than in seagrass meadows and escarpments (Supplementary Table 4; Figure 3B). Two principal components of the PCO showing differences in fish biomass across habitat types according to trophic categories explained 59% of the total variance (Figure 4A). The first component (PCO1; 39% of the variance) differentiated seagrass escarpments and rocky substrates from seagrass meadows based on the lower fish biomasses across all trophic categories in the latter. PCO2 (20% of the variance) mostly differentiated seagrass escarpments from rocky substrates based on the larger abundance of herbivorous and omnivorous fish in the latter.
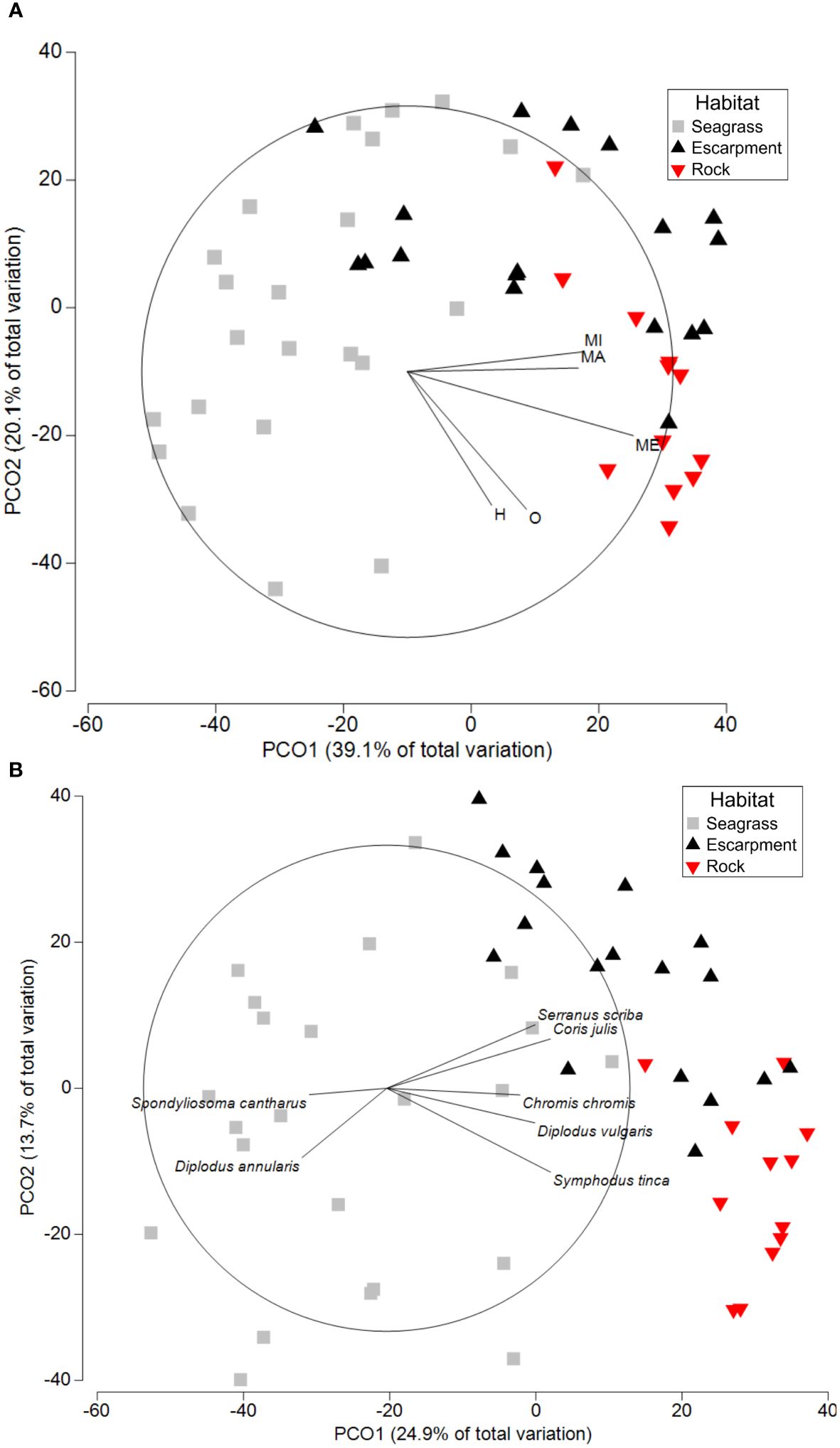
Figure 4 Principal Coordinate Analysis (PCO) showing differences in fish biomass among habitat types (seagrass meadows, seagrass escarpments and rocky substrates) based on (A) trophic category (herbivores, H; macrophagous, MA; mesophagous, ME; microphagous, MI; and omnivores, O); and (B) fish species. Relevant fish species driving PCO1 and PCO2 scores are overlayed.
The PCO based on the biomass of fish species explained 39% of the total variance (Figure 4B). The first component (PCO1; 25% of the variance) differentiated seagrass meadows from both seagrass escarpments and rocky substrates based on the higher abundance of Serranus scriba, Coris julis, Symphodus tinca and Chromis chromis in rocky substrates, and the higher abundance of Spondyliosoma cantharus and Diplodus annularis in seagrass meadows. PCO2 (14% of the variance) differentiated seagrass escarpments from rocky substrates based on the greater abundance of S. scriba and C. julis in seagrass escarpments, and the higher abundance of Diplodus vulgaris and S. tinca in rocky substrates.
3.3 Correlations between structural complexity and fish assemblages
A total of 169 cavities were measured across all seagrass escarpments in order assess habitat structural complexity. Structural complexity was different among habitats (P (perm) < 0.001, Supplementary Table 3), with significantly higher values in rocky substrates than in seagrass meadows and escarpments (8.3 ± 1.7, 13.1 ± 3.3, 19.7 ± 1.7, respectively). Weak positive relationships between habitat structural complexity and Shannon´s diversity Index, total number of fish species and total fish biomass across all habitats were observed (r = 0.46, 0.44 and 0.38, respectively; Figure 5). The abundance of fish was not correlated with habitat structural complexity (r = 0.20).
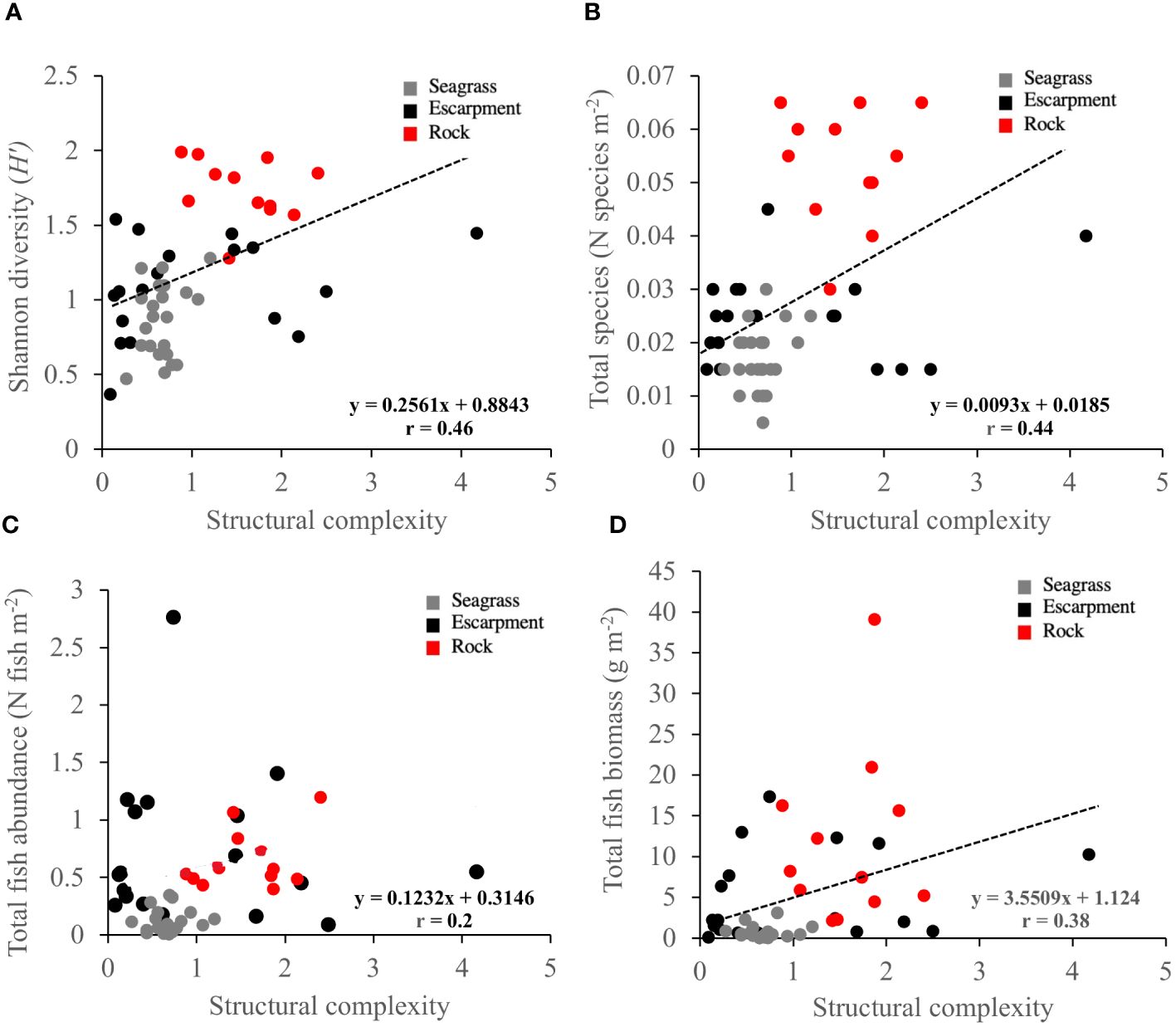
Figure 5 Pearson correlation relationships between habitat structural complexity, and (A) Shannon´s diversity Index (H’), (B) total number of species (number of species m-2), (C) fish abundance (number of fish m-2), and (D) fish biomass (g m-2) among habitats. The regression equation and r values are indicated. Habitat types are denoted in colors: seagrass meadows (grey), seagrass escarpments (black) and rocky substrates (red).
4 Discussion
4.1 Natural history of P. oceanica escarpments
The high productivity of P. oceanica meadows together with their capacity to trap particles contributes to the accretion of carbon-rich soils over centuries to millennia at an average rate of 2.1 mm yr-1 (Fourqurean et al., 2012; Serrano et al., 2016), resulting in the formation of the matte (Bonhomme et al., 2015). The presence of vertical cuts within P. oceanica meadows can originate from either natural or anthropogenic factors, but it is overall rare. The dimensions of the escarpments surveyed had an average length of 37 ± 1.4 m, 1.4 ± 0.2 m in height, and 0.6 ± 0.1 cavities per m2 of escarpment. Overall, Posidonia escarpments are barrier reefs that resemble a rocky habitat in aspect and constitute unique formations, some of which have been recognized as a protected National Monument under the IUCN (Blas Aritio, 1990).
The cliffs along a coastline are structurally very similar to seagrass escarpments and thereby, it is intuited that the formation of escarpments is analogous to that of vertical cliffs. The erosion of the escarpment, owing to wave and current action, likely erodes the base of the matte creating a ledge where the upper portion overhangs from the wall. Then after, the overhang collapses resulting on the retreat of the escarpment over variable time scales. Field observations of ledges towards the base of the escarpment and collapsed fragments of matte (up to 1 m3 in size) in front of the escarpments support this hypothesis (Figures 6A, B).
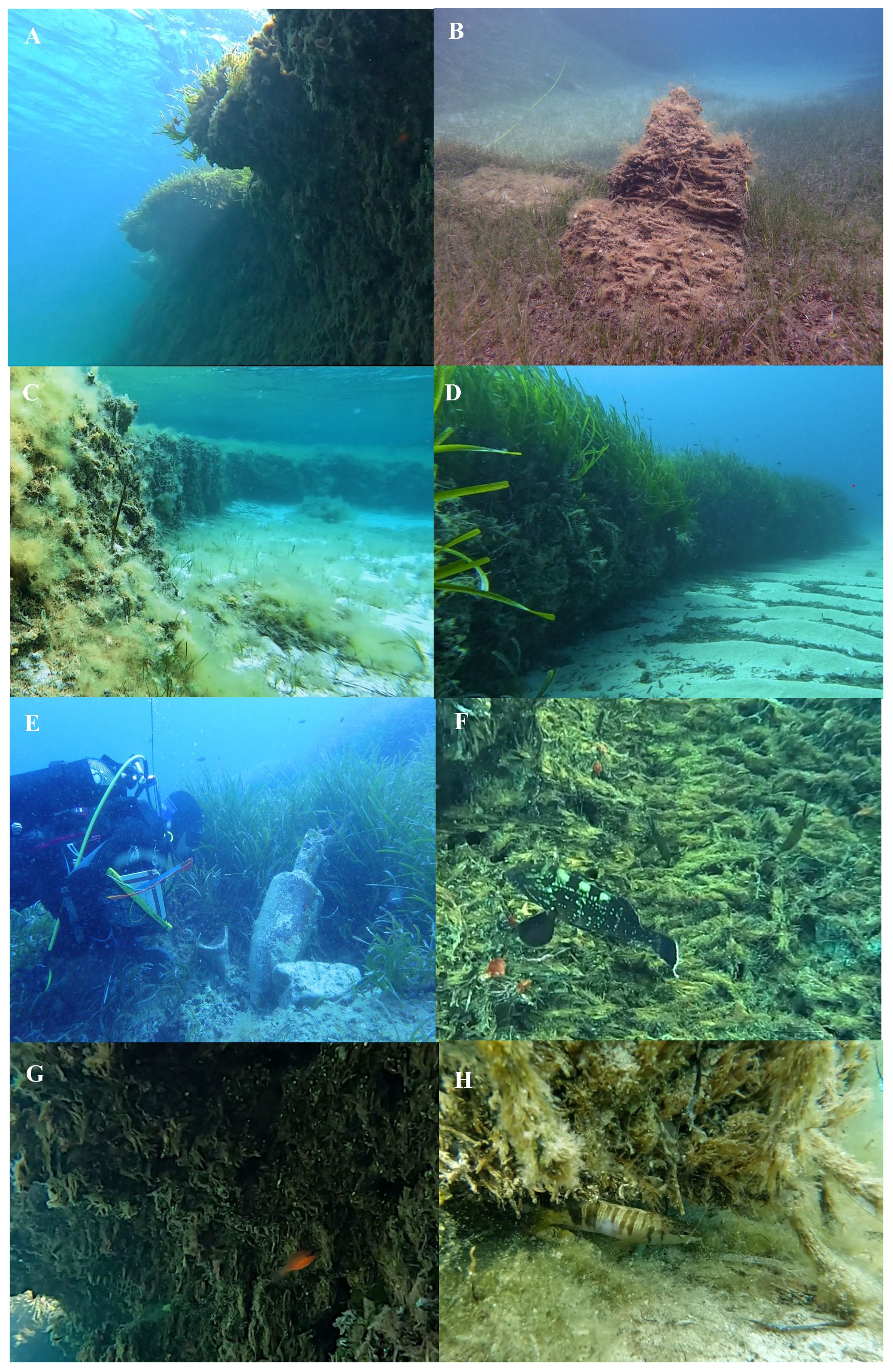
Figure 6 Seagrass escarpments and archaeological heritage. (A) Shallow escarpment in Sa Torreta with an overhang; (B) portion of matte detached from the escarpment wall in Sa Torreta); (C) shallow escarpment colonized by seaweed in S’Estany; (D) deep seagrass escarpment in Son Bou; (E) ancient ceramic amphora embedded in an escarpment; (F) rocky fish Epinephelus marginatus in front of a cavity within an escarpment; (G) rocky fish Apogon imberbis under an escarpment overhang; and (H) rocky fish Serranus scriba hidden under a ledge at the base of an escarpment.
The geomorphology of seagrass escarpments differed among sites, likely according to the interaction of physical variables (e.g., water depth, exposure to hydrodynamic forces and dominant winds, water flow, and terrestrial inputs), biological variables (e.g., seagrass primary production and meadow structure) and anthropogenic disturbances. Typically, the deep escarpments surveyed were more scarce, shorter, and narrower than shallower escarpments (Figures 6C, D). It seems plausible that the relatively higher seagrass productivity, biomass, and soil accumulation rates in shallow meadows results in higher soil accretion and matte build up (Serrano et al., 2016), thereby likely allowing the formation of higher escarpments than in deeper areas. All escarpments observed, except those located in waters deeper than 12 m, were oriented in parallel to the coast, which could indicate a natural mechanism of formation linked to hydrodynamic forces. Shallow seagrass escarpments likely form by either i) exposure to continuous hydrodynamic forces that results in the opening of channels across the meadows to facilitate water outflow (Bonhomme et al., 2015); ii) extreme storm events that rework sediment and dislodge portions of the matte (Gera et al., 2014; Oprandi et al., 2020); iii) anchor damage followed by erosion of the edges of the meadow; or iv) dredging to open a channel into shallower areas. Indeed, hydrodynamic energy and anchoring are typically more intense in shallow environments making shallow meadows more susceptible to erosion. This hypothesis is supported by multiple observations of large portions of matte detached from the escarpment wall in shallow settings compared to the scarcity of detached matte in deeper settings, which could also indicate faster rates of formation and evolution of escarpments in the former. At the deeper escarpments surveyed, in addition to occasional extreme hydrodynamic events (e.g., storm surges), it seems plausible that anchors deployed by big boats opened a scar within the continuous meadow (typically found at depths >10 m), favoring the expansion of the scar, resulting in the large and linear escarpments observed. It may also seem plausible that fish could actively erode the escarpment when digging to create shelters, as previously observed following deep sea oil and gas piping deployment (Twichell et al., 1985). This hypothesis is supported by observations during field surveys of species from genus Gobius and Symphodus eroding the escarpment wall to maintain or create shelter.
Erosion likely exposed the ancient matte along the edges of the meadows at S’Estany and Sa Torreta, whereas the escarpments at Sanitja likely originated from channels carved in the meadow for boats to enter the coastal settlement during Roman times (Contreras et al., 2006). However, establishing a cause-effect relationship around the origin or evolution of escarpments is difficult owing to the multiple climatic and anthropogenic processes that occurred since millennia, which further accelerated and intensified during the last decades in coastal areas. Physical and geomorphological variables are site-specific and multiple biotic and abiotic factors may have interacted over millennia to shape the currently observed geomorphology of escarpments (Bonhomme et al., 2015).
The walls of the escarpments were colonized by organisms that typically inhabit rocky substrates, and the taxa and their abundance seemed to vary according to differences in irradiance, escarpment slope, rhizome density and orientation. Escarpments located in shaded areas (e.g., north-oriented) with vertical profiles or overhangs were colonized by sciaphilic algae, including Flabellia petiolata and Peyssonnelia squamaria. On the other hand, those exposed to higher irradiance were colonized by photophilic algae including Halopteris scoparia and Dictyota spp., and also by invasive species such as Caulerpa cylindracea and Lophocladia trichoclados. Escarpments were also colonized by other organisms typically found in rocky substrates including ascidians (e.g., Halocynthia papillosa) and bryozoans (e.g., Schizoporella errata). The colonization of living and dead P. oceanica rhizomes by fauna and flora typically inhabiting rocky substrates has been previously reported (Boudouresque, 1974; Ballesteros, 1992; Mabrouk et al., 2014). However, the intrinsic characteristics of escarpments, which differ from seagrass canopies, requires further studies to understand the distribution of fauna and flora assemblages according to differences in escarpment geomorphology.
Posidonia oceanica seagrass meadows are a sink for undiscovered ancient shipwrecks and other archaeological items (Krause-Jensen et al., 2019). The vertical accretion of the meadow can cover entire wrecks after sinking thousands of years ago, and the visual assessment of seagrass escarpments could be used as a preliminary step towards identifying archaeological remains and reveal the presence of cultural heritage. During field work we identified portions of ceramic amphoras embedded in the escarpment wall and other ceramic remains at the blowout near the escarpment, which seem to belong to Roman times (~100 BC; amphora Dressel 1 type, based on Dressel (1899) classification) and showcases the extraordinary capacity of seagrass meadows to colonize new substrates and to conceal ancient history (Figure 6E).
4.2 Enhanced fish diversity and biomass in seagrass escarpments
The choice of habitat by fish species typically responds to specific shelter and food requirements, and seagrasses are well known to fulfil both needs for a number of species (Rozas and Odum, 1988). The results obtained suggest that the higher structural complexity provided by P. oceanica escarpments enhances fish abundance, number of species diversity and biomass compared to homogeneous seagrass meadows. The presence of cavities within the vertical walls provides additional shelter to fish assemblages compared to seagrass canopies and resembling rocky substrates. However, the relatively higher number of species, diversity and size of fish found in rocky substrates compared to seagrass escarpments could be explained by the overall higher structural complexity of rocky habitats, with larger number and size of cavities that can provide shelter to relatively bigger fish (Ferreira et al., 2001). However, the extremely high variability in the structural complexity across the escarpments studied (ranging from 2 to 35%) points to the need to conduct further studies aiming at assessing how different escarpment types (e.g., composition, orientation, depth, fetch, and geomorphology) could affect fish assemblages. Fish diversity and abundance in escarpments seemed to be enhanced by the presence of big cavities and ledges, including overhanging and detached portions of matte, and by reduced incidence of irradiance (i.e., orientated to the north, in our case). However, it was not possible to test these hypotheses due to the heterogeneity of escarpment features along each transect and the unbalanced sampling design.
The presence of fish species linked to cavities from rocky substrates within seagrass escarpments, such as Apogon imberbis (Apogonidae) and Epinephelus marginatus (Serranidae) (Figures 6F, G), supports the hypothesis that escarpments provide suitable habitat for fish similarly to the one supplied by rocky substrates. For example, it seems plausible that A. imberbis, which typically congregates along reef caves and overhangs and has a nocturnal feeding habit (Corbera et al., 1996; Bussotti et al., 2018), uses the escarpments as habitat for residence during daytime and feed in the nearby P. oceanica meadows at night. Owing to the night feeding behavior of A. imberbis, it seems possible that some individuals were not counted because they were hiding inside the cavities of escarpments and rocky substrates. On the other hand, E. marginatus use caves as permanent places of residence but adult individuals are solitary and prefer deep rocky substrates compared to juveniles that are mainly found in shallow waters close to shore (Heemstra and Randall, 1993). E. marginatus is a targeted species for fishers and catalogued as vulnerable by the International Union for Conservation of Nature (IUCN) (Pollard et al., 2018); the location of escarpments within shallow and sheltered bays can constitute suitable nursery grounds for juvenile groupers (Harmelin and Harmelin-Vivien, 1999; Bodilis et al., 2003). These facts are also supported by the observation of multiple juvenile and adult E. coioides individuals hiding along P. australis escarpments in Australia (Serrano et al., 2017), a region that is less overfished than the Mediterranean Sea.
The large number of macrophagous fish found in seagrass escarpments (e.g., S. scriba; Figure 6H) compared to the other habitats surveyed, suggests that escarpments constitute a key habitat for the shelter and likely reproduction and hunting ground for this species. The absence of herbivore fish within escarpments could be explained by limited food availability (i.e., seaweed) and by the risk of exposure to predators when feeding along the escarpments. Seagrass escarpments can be surrounded by either seagrass, sand, or rocky habitats, and the results obtained showed that fish species inhabiting fringing habitats could use the escarpment as a corridor among the heterogenous coastal seascape or even adjust their life cycle and use it as permanent residence. This is a novel hypothesis that brings seagrass meadows into scope for a new-fangled extension of the enhanced biodiversity ecosystem service.
5 Conclusions
This study provides new insights into the natural history of seagrass escarpments, including their plausible origin linked to natural and anthropogenic processes, and the fauna and flora inhabiting these unique habitats. It also showcases that seagrass escarpments are overlooked habitats that sustain fish abundance and biomass comparable to rocky substrates. The presence of strictly rocky fish species within the escarpments together with the similar fish abundance and biomass between rocky substrates and escarpments supports the hypothesis of escarpments acting as three-dimensional complex habitats. In addition, escarpments could play a key connectivity role among habitats in embayment’s with scarcity of rocky substrates (Serrano et al., 2016). Further studies are required to better understand the natural history of escarpments, including differences in fish assemblages according to the nature of escarpments (e.g., geomorphology, orientation, fetch and depth), together with deciphering fish assemblages across multiple seasons and regions with different conservation status. This study provides new evidence supporting the need for the protection and conservation of seagrass meadows.
Data availability statement
The raw data supporting the conclusions of this article will be made available by the authors, without undue reservation.
Ethics statement
Ethical approval was not required for the study involving animals in accordance with the local legislation and institutional requirements because only observational study; no harvesting or interacting with animals.
Author contributions
EG: Conceptualization, Data curation, Formal analysis, Investigation, Methodology, Software, Visualization, Writing – original draft, Writing – review & editing. EB: Conceptualization, Formal analysis, Investigation, Methodology, Writing – original draft, Writing – review & editing. IB: Investigation, Writing – original draft, Writing – review & editing. KI: Data curation, Formal analysis, Visualization, Writing – original draft, Writing – review & editing. MM: Conceptualization, Investigation, Writing – original draft, Writing – review & editing. OS: Conceptualization, Data curation, Formal analysis, Funding acquisition, Investigation, Methodology, Project administration, Resources, Supervision, Validation, Visualization, Writing – original draft, Writing – review & editing.
Funding
The author(s) declare financial support was received for the research, authorship, and/or publication of this article. This project was funded by the Spanish RYC2019-027073-I project. IB was funded by the JAE-Intro ICU fellowship. EG was supported by “Pla de Doctorats Industrials del Departament de Recerca i Universitats de la Generalitat de Catalunya”. OS was supported by I+D+© project PIE HOLOCENO 20213AT014 funded by MCIN/ AEI/10.13039/501100011033 and FEDER.
Acknowledgments
We thank Juancho Movilla, Sebastián Molina and Maria Elena Cefalì (Estació d’Investigació Jaume Ferrer, COB-IEO) for their support in the field. This is a publication of the Benthic Ecology Group (2021 SGR 00405).
Conflict of interest
The authors declare that the research was conducted in the absence of any commercial or financial relationships that could be construed as a potential conflict of interest.
The author(s) declared that they were an editorial board member of Frontiers, at the time of submission. This had no impact on the peer review process and the final decision.
Publisher’s note
All claims expressed in this article are solely those of the authors and do not necessarily represent those of their affiliated organizations, or those of the publisher, the editors and the reviewers. Any product that may be evaluated in this article, or claim that may be made by its manufacturer, is not guaranteed or endorsed by the publisher.
Supplementary material
The Supplementary Material for this article can be found online at: https://www.frontiersin.org/articles/10.3389/fmars.2024.1373074/full#supplementary-material
References
Ambo-Rappe R., Nessa M. N., Latuconsina H., Lajus D. L. (2013). Relationship between the tropical seagrass bed characteristics and the structure of the associated fish community. Open J. Ecol. 3, 331–342. doi: 10.4236/oje.2013.35038
Anderson M. J., Gorley R. N., Clarke K. R. (2009). PRIMER for Windows Version 6.1. 12 & PERMANOVA Version 1.0. 2. Plymouth: PRIMER-E-Ltd.
Ballesteros E. (1987). Estructura i dinàmica del poblament algal de les fulles de Posidonia oceanica (L.) Delile als herbeis de Tossa de Mar (Girona). Butlletí la Institució Catalana d’Història Natural 54, 13–30.
Ballesteros E. (1992). Els vegetals i la zonació litoral: espècies, comunitats i factors que influeixen en la seva distribució.Arxius Secció Ciències 101 (Barcelona: Institut d’Estudis Catalans), 616.
Bell J. D., Harmelin-Vivien M. (1982). Fish fauna of French Mediterranean Posidonia oceanica seagrass meadows. I. Community structure. Tethys 10 (4), 337–347.
Bell S. S., Walters K., Kern J. C. (1984). Meiofauna from seagrass habitats: A review and prospectus for future research. Estuaries 7, 331–338. doi: 10.2307/1351617
Bellisario B., Camisa F., Abbattista C., Cimmaruta R. (2019). A network approach to identify bioregions in the distribution of Mediterranean amphipods associated with Posidonia oceanica meadows. Peer J. 7, e7686. doi: 10.7717/peerj.6786
Blas Aritio L. (1990). Guía de los Espacios Naturales de Andalucía (Spain, Junta de Andalucía: Agencia de Medio Ambiente).
Bodilis P., Ganteaume A., Francour P. (2003). Recruitment of the dusky grouper (Epinephelus marginatus) in the North-western Mediterranean Sea. Cybium 27, 123–129.
Bonhomme D., Boudouresque C. F., Astruch P., Bonhomme J., Bonhomme P., Goujard A., et al. (2015). Typology of the reef formations of the Mediterranean seagrass Posidonia oceanica, and the discovery of extensive reefs in the Gulf of Hyères (Provence, Mediterranean). Travaux Scientifiques du Parc Natl. Port-Cros 29, 41–73.
Boström C., Pittman S. J., Simenstad C., Kneib R. T. (2011). Seascape ecology of coastal biogenic habitats: Advances, gaps, and challenges. Mar. Ecol. Prog. Ser. 427, 191–217. doi: 10.3354/meps09051
Boudouresque C. F. (1974). Recherches de bionomie analytique, structurale et expérimentale sur les peuplements benthiques sciaphiles de Méditerranée Occidentale (fraction algale): le peuplement épiphyte des rhizomes des Posidonies (Posidonia oceanica Delile). Bull. du Muséum d’Histoire Naturelle Marseille 54, 269–283.
Boudouresque C. F. (2004). Marine biodiversity in the Mediterranean; status of species, populations and communities. Travaux Scientifiques du Parc Natl. Port-Cros 20, 97–146.
Boudouresque C. F., Bernard G., Bonhomme P., Charbonnel E., Diviacco G., Meinesz A., et al. (2012). Protection and conservation of Posidonia oceanica meadows (Monaco: RAMOGE and RAC/SPA), 202.
Boudouresque C. F., Meinesz A. (1983). Découverte de l’herbier de Posidonies (Cahier du Parc National de Port Cros). 4, 1–79.
Bussotti S., di Franco A., Bianchi C. N., Chevaldonné P., Egea L., Fanelli E., et al. (2018). Fish mitigate trophic depletion in marine cave ecosystems. Sci. Rep. 8, 9183. doi: 10.1038/s41598-018-27491-1
Charbonnel E., Serre C., Ruitton S., Harmelin J. G., Jensen A. (2002). Effects of increased habitat complexity on fish assemblages associated with large artificial reef units (French Mediterranean coast). ICES J. Mar. Sci. 59, 208–213. doi: 10.1006/jmsc.2002.1263
Comas E. (2004). Menorca, reserva de la biosfera, el repte de la sostenibilitat. Atzavara. Oficina de la Reserva de la Biosfera, Consell Insular de Menorca. 2, 69–78. doi: 10.1006/jmsc.2002.1263
Contreras F., Müller R., Joseí F. J. (2006). El asentamiento militar romano de Sanitja (123-45 a.C.): una aproximacioín a su contexto histoírico. Mayurqa 31, 231–249.
Corbera J., Sabatés A., Garcia-Rubies A. (1996). Peces de mar de la Península Ibérica (Planeta), 312.
Cullen-Unsworth L., Unsworth R. (2013). Seagrass meadows, ecosystem services, and sustainability. Environment 55, 14–28. doi: 10.1080/00139157.2013.785864
Dressel H. (1899). Corpus Inscriptionum Latinarum, Vol. XV: Inscriptiones Urbis Romae Latinae (Berlin: Instrumentum domesticum). Available at: https://abc.se/~pa/mar/dressel.htm.
Duffy J. E. (2006). Biodiversity and the functioning of seagrass ecosystems. Mar. Ecol. Prog. Ser. 311, 233–250. doi: 10.3354/meps311233
Ferreira C. E. L., Gonçalves J. E. A., Coutinho R. (2001). Community structure of fishes and habitat complexity on a tropical rocky shore. Environ. Biol. Fishes 61, 353–369. doi: 10.1023/A:1011609617330
Fourqurean J. W., Duarte C. M., Kennedy H., Marbà N., Holmer M., Mateo M. A., et al. (2012). Seagrass ecosystems as a globally significant carbon stock. Nat. Geosci. 5, 505–509. doi: 10.1038/ngeo1477
Francour P. (1997). Fish assemblages of Posidonia oceanica beds at Port-Cros (France, NW Mediterranean). Assessment of composition and long-term fluctuations by visual census. Mar. Ecol. 18, 157–173. doi: 10.1111/j.1439-0485.1997.tb00434.x
Friedman A., Pizarro O., Williams S. B., Johnson-Roberson M. (2012). Multi-scale measures of rugosity, slope and aspect from benthic stereo image reconstructions. PloS One 7, e50440. doi: 10.1371/journal.pone.0050440
García-Gómez G., García-Herrero A., Sánchez N., Pardos F., Izquierdo-Muñoz A., Fontaneto D., et al. (2022). Meiofauna is an important, yet often overlooked, component of biodiversity in the ecosystem formed by Posidonia oceanica. Invertebrate Biol. 141, e12377. doi: 10.1111/ivb.12377
Gera A., Pagès J. F., Arthur R., Farina S., Roca G., Romero J., et al. (2014). The effect of a centenary storm on the long-lived seagrass Posidonia oceanica. Limnology Oceanography 59, 1910–1918. doi: 10.4319/lo.2014.59.6.1910
Giakoumi S., Cebrian E., Kokkoris G. D., Ballesteros E., Sala E. (2012). Relationships between fish, sea urchins and macroalgae: The structure of shallow rocky sublittoral communities in the Cyclades, Eastern Mediterranean. Estuarine Coast. Shelf Sci. 109, 1–10. doi: 10.1016/j.ecss.2011.06.004
Gratwicke B., Speight M. R. (2005). The relationship between fish species richness, abundance and habitat complexity in a range of shallow tropical marine habitats. J. Fish Biol. 66, 650–667. doi: 10.1111/j.0022-1112.2005.00629.x
Guidetti P. (2000). Differences among fish assemblages associated with nearshore Posidonia oceanica seagrass beds, rocky-algal reefs and unvegetated sand habitats in the Adriatic Sea. Estuarine Coast. Shelf Sci. 50, 515–529. doi: 10.1006/ecss.1999.0584
Guidetti P., Baiata P., Ballesteros E., Di Franco A., Hereu B., Macpherson E., et al. (2016). Large-scale assessment of Mediterranean marine protected areas effects on fish assemblages. PloS One 9, e91841. doi: 10.1371/journal.pone.0091841
Harmelin J. G., Harmelin-Vivien M. (1999). A review on habitat, diet and growth of the dusky grouper Epinephelus marginatus (Lowe 1934). Mar. Life 9, 11–20.
Harmelin-Vivien M., Harmelin J. G., Chauvet C., Duval C., Galzin R., Lejeune P., et al. (1985). Evaluation visuelle des peuplements et populations de poissons. Méthodes et problémes. Rev. d’Écologie Terre Vie 40, 467–539. doi: 10.3406/revec.1985.5297
Heck K. L., Orth R. J. (1980). Seagrass habitats: the roles of habitat complexity, competition and predation in structuring associated fish and motile macroinvertebrate assemblages. Estuarine Perspectives, 449–464. doi: 10.1016/B978-0-12-404060-1.50043-5
Heemstra P. C., Randall J. E. (1993). FAO species catalogue, Vol. 16. Groupers of the world (Family Serranidae, Subfamily Epinephelinae). An annotated and illustrated catalogue of the grouper, rockcod, hind, coral grouper and lyretail species known to date. Fisheries synopsis, 125. Rome: FAO.
Hill J., Wilkinson C. (2004). Methods for ecological monitoring of coral reefs (Townsville: Australian Institute of Marine Science), 117.
Hyman A., Frazer T. K., Jacoby C. A., Frost J. R., Kowalewski M. (2019). Long-term persistence of structured habitats: Seagrass meadows as enduring hotspots of biodiversity and faunal stability. Proc. R. Soc. B: Biol. Sci. 286, 1912. doi: 10.1098/rspb.2019.1861
Hyndes G. A., Kendrick A. J., MacArthur L. D., Stewart E. (2003). Differences in the species- and size-composition of fish assemblages in three distinct seagrass habitats with differing plant and meadow structure. Mar. Biol. 142, 1195–1206. doi: 10.1007/s00227-003-1010-2
Jänes H., Macreadie P. I., Zu Ermgassen P. S. E., Gair J. R., Treby S., Reeves S., et al. (2020). Quantifying fisheries enhancement from coastal vegetated ecosystems. Ecosystem Serv. 43, 101105. doi: 10.1016/j.ecoser.2020.101105
Kaiser M. J., Rogers S. I., Ellis J. R. (1998). Importance of benthic habitat complexity for demersal fish assemblages. Am. Fisheries Soc. Symposium Vol 22, 212–223.
Krause-Jensen D., Serrano O., Apostolaki E. T., Gregory D. J., Duarte C. M. (2019). Seagrass sedimentary deposits as security vaults and time capsules of the human past. Ambio 48, 325–335. doi: 10.1007/s13280-018-1083-2
La Mesa G., Vacchi M. (1999). An analysis of the coastal fish assemblage of the Ustica Island marine reserve (Mediterranean Sea). Mar. Ecol. 20, 147–165. doi: 10.1046/j.1439-0485.1999.00067.x
Larkum A. W. D., Orth R. J., Duarte C. M. (2006). Seagrasses: Biology, ecology and conservation (Netherlands: Springer), 691. doi: 10.1111/j.1439-0485.2006.00138.x
Llompart J., Rosell C. (2002). El naixement d’una illa, Menorca. Guia de geologia pràctica (Montcada i Reixac: Dacs, Indústria Gràfica).
Mabrouk L., Ben Brahim M., Hamza A., Bradai M. N. (2014). Diversity and temporal fluctuations of epiphytes and sessile invertebrates on the rhizomes of Posidonia oceanica in a seagrass meadow off Tunisia. Mar. Ecol. 35, 212–220. doi: 10.1111/maec.12072
Macia S., Robinson M. (2005). Effects of habitat heterogeneity in seagrass beds on grazing patterns of parrotfishes. Mar. Ecol. Prog. Ser. 303, 113–121. doi: 10.3354/meps303113
Máñez-Crespo J., Tomas F., Fernández-Torquemada Y., Royo L., Espino F., Antich L., et al. (2022). Variation in fish abundance, diversity and assemblage structure in seagrass meadows across the Atlanto-Mediterranean province. Diversity 14, 808. doi: 10.3390/d14100808
Mateo M. A., Romero J., Pérez M., Littler M. M., Littler D. S. (1997). Dynamics of millenary organic deposits resulting from the growth of the Mediterranean seagrass Posidonia oceanica. Estuarine Coast. Shelf Sci. 44, 103–110. doi: 10.1006/ecss.1996.0116
Menorca Biosphere Reserve. (2020). Menorca Biosphere Reserve (Spain: UNESCO). Available at: https://en.unesco.org/biosphere/eu-na/menorca.
Moranta J., Palmer M., Morey G., Ruiz A., Morales-Nin B. (2006). Multi-scale spatial variability in fish assemblages associated with Posidonia oceanica meadows in the Western Mediterranean Sea. Estuarine Coast. Shelf Sci. 68, 579–592. doi: 10.1016/j.ecss.2006.03.008
Morey G., Moranta J., Massutı E., Grau A., Linde M., Riera F., et al. (2003). Weight–length relationships of littoral to lower slope fishes from the western Mediterranean. Fisheries Res. 62, 89–96. doi: 10.1016/S0165-7836(02)00250-3
Morrison M. A., Lowe M. L., Grant C. M., Smith P. J., Carbines G., Reed J., et al. (2014). “Seagrass meadows as biodiversity and productivity hotspots,” in New Zealand Aquatic Environment and Biodiversity Report No. 137. New Zealand: Ministry for Primary Industries.
Murphey P. L., Fonseca M. S. (1995). Role of high and low energy seagrass beds as nursery areas for Penaeus duorarum in North Carolina. Mar. Ecol. Prog. Ser. 121, 91–98. doi: 10.3354/meps121091
Nordlund L. M., Jackson E. L., Nakaoka M., Samper-Villarreal J., Beca-Carretero P., Creed J. C. (2018). Seagrass ecosystem services – What’s next? Mar. pollut. Bull. 134, 145–151. doi: 10.1016/j.marpolbul.2017.09.014
Oprandi A., Mucerino L., De Leo F., Bianchi C. N., Morri C., Azzola A., et al. (2020). Effects of a severe storm on seagrass meadows. Sci. Total Environ. 748, 141373. doi: 10.1016/j.scitotenv.2020.141373
Patriquin D. G. (1975). “Migration” of blowouts in seagrass beds at Barbados and Carriacou, West Indies, and its ecological and geological implications. Aquat. Bot. 1, 163–189. doi: 10.1016/0304-3770(75)90021-2
Pauly D., Froese R. (2006). The length-weight relationship of fishes: a review. J. Appl. Ichthyology 22, 241–253. doi: 10.3750/AIP2011.41.4.01
Pérès J. M., Picard J. (1975). Causes de la raréfaction et de la disparition des herbiers de Posidonia oceanica sur les côtes françaises de la Méditerranée. Aquat. Bot. 1, 133–139. doi: 10.1016/0304-3770(75)90018-2
Piazzi L., Balata D., Ceccherelli G. (2016). Epiphyte assemblages of the Mediterranean seagrass Posidonia oceanica: an overview. Mar. Ecol. 37, 3–41. doi: 10.1111/maec.12331
Pollard D. A. (1984). A review of ecological studies on seagrass-fish communities, with particular reference to recent studies in Australia. Aquat. Bot. 18, 3–42. doi: 10.1016/0304-3770(84)90079-2
Pollard D. A., Afonso P., Bertoncini A. A., Fennessy S., Francour P., Barreiros J. (2018). Epinephelus marginatus. The IUCN Red List of Threatened Species 2018, e.T7859A100467602. doi: 10.2305/IUCN.UK.2018-2.RLTS.T7859A100467602.en
Rappe R. (2010). Population and community level indicator in assessment of heavy metal contamination in seagrass ecosystem. Coast. Mar. Sci. 34, 198–204.
Reñones O., Moranta J., Coll J., Morales-Nin B. (1997). Rocky bottom fish communities of Cabrera Archipelago National Park (Mallorca, Western Mediterranean). Scientia Marina 61, 495–506.
Rozas L. P., Odum W. E. (1988). Occupation of submerged aquatic vegetation by fishes: testing the roles of food and refuge. Oecologia 77, 101–106. doi: 10.1007/BF00380932
Santos de Moura M., Vianna M. (2020). A new threat: assessing the main interactions between marine fish and plastic debris from a scientometric perspective. Rev. Fish Biol. Fisheries 30, 623–636. doi: 10.1007/s11160-020-09621-z
Serrano O., Lavery P. S., Bongiovanni J., Duarte C. M. (2020). Impact of seagrass establishment, industrialization and coastal infrastructure on seagrass biogeochemical sinks. Mar. Environ. Res. 160, 104990. doi: 10.1016/j.marenvres.2020.104990
Serrano O., Lavery P. S., Lopez-Merino L., Ballesteros E., Mateo M. A. (2016). Location and associated carbon storage of erosional escarpments of seagrass Posidonia mats. Front. Mar. Sci. 3. doi: 10.3389/fmars.2016.00042
Serrano O., Serrano E., Inostroza K., Lavery P. S., Mateo M. A., Ballesteros E. (2017). Seagrass meadows provide 3D habitat for reef fish. Front. Mar. Sci. 4. doi: 10.3389/fmars.2017.00054
Templado J., Calvo Revuelta M., Luque Á. A., Garvía A., Maldonado M., Moro L. (2004). Guía de los invertebrados peces marinos españoles protegidos por la legislación nacional internacional. MMACSIC, Madrid.
Thiriet P., Cheminée A., Mangialajo L., Francour P. (2014). “How 3D complexity of macrophyte-formed habitats affect the processes structuring fish assemblages within coastal temperate seascapes?,” in Underwater Seascapes (Springer, Cham), 185–199. doi: 10.1007/978-3-319-03440-9_12
Tomasello A., Di Maida G., Calvo S., Pirrotta M., Borra M., Procaccini G. (2009). Seagrass meadows at the extreme of environmental tolerance: the case of Posidonia oceanica in a semi-enclosed coastal lagoon. Mar. Ecol. 30, 288–300. doi: 10.1111/j.1439-0485.2009.00285.x
Keywords: natural history, aquatic plant ecology, fish ecology, biodiversity, ecosystem services, erosion, Balearic Islands
Citation: Gomis E, Ballesteros E, Bernabeu I, Inostroza K, Mateo MA and Serrano O (2024) Escarpments within Mediterranean seagrass Posidonia oceanica meadows increase habitat heterogeneity and structural complexity enhancing fish diversity and biomass. Front. Mar. Sci. 11:1373074. doi: 10.3389/fmars.2024.1373074
Received: 19 January 2024; Accepted: 08 April 2024;
Published: 03 May 2024.
Edited by:
Ibon Galparsoro, Technological Center Expert in Marine and Food Innovation (AZTI), SpainReviewed by:
Tomas Vega Fernandez, Anton Dohrn Zoological Station Naples, ItalyMarco C. Brustolin, Norwegian Institute of Marine Research (IMR), Norway
Copyright © 2024 Gomis, Ballesteros, Bernabeu, Inostroza, Mateo and Serrano. This is an open-access article distributed under the terms of the Creative Commons Attribution License (CC BY). The use, distribution or reproduction in other forums is permitted, provided the original author(s) and the copyright owner(s) are credited and that the original publication in this journal is cited, in accordance with accepted academic practice. No use, distribution or reproduction is permitted which does not comply with these terms.
*Correspondence: Oscar Serrano, oserrano@ceab.csic.es