Genetic types and provenance indication of clastic amphibole in the South Yellow Sea
- 1Qingdao Institute of Marine Geology, China Geological Survey, Qingdao, China
- 2School of Resources and Environmental Engineering, Ludong University, Yantai, China
- 3Ministry of Education Key Laboratory for Coast and Island Development, School of Geography and Ocean Science, Nanjing University, Nanjing, China
- 4State Key Laboratory of Estuarine and Coastal Research, East China Normal University, Shanghai, China
The main focus of studying sediment sources in marine areas is to determine the properties of clastic minerals originating from different rivers. Variations in the characteristics of clastic minerals occur due to differences in the types of source rocks within the watershed. Determining the source rock of clastic minerals in the marine area can improve the accuracy of provenance analysis. Electron probe microanalysis of 258 amphibole grains from six surface stations in the South Yellow Sea (SYS) was conducted to calculate the numerical and characteristic values of cations in the crystal structure. The results showed that amphibole in the SYS is mainly magnesiohornblende (52.2% ~ 81.4%) in the calcic amphibole subgroup, followed by tschermakite. The source rock types of amphibole are mainly different types of magmatic (ultrabasic, basic, and intermediate acidic) and metamorphic rocks. Amphibole derived from intermediate-acid intrusive rocks accounts for a large proportion (>40%). The genetic analysis of clastic amphibole in the SYS showed that most of the medium-acid invading amphibole belong to crust-mantle type, followed by mantle type. Most of the amphibole from metamorphic origins are of medium-low pressure type (± 80%). Combined with electron probe analysis of amphibole from the Huanghe River (the Yellow River, HH) and Changjiang River (the Yangtze River, CJ), the amphibole in the SYS exhibits characteristics inherited from these two rivers. The northern sea area has a strong resemblance to the sediments from the HH, while the southern area is more influenced by sediments supply from the CJ. The central area represents a mixed zone with a higher sediment supply from the HH. The clastic amphibole deduces the type of source rock in different watersheds, serving as a crucial link between the source rock, watershed, and marine area, providing a basis for provenance analysis.
1 Introduction
Sediments transported by rivers rapidly accumulate in estuaries, then are resuspended into the sea by the action of waves and tides, and transported over long distances by water mass and circulation, with most of them settling in the shelf areas of marginal seas (Milliman and Meade, 1983; McKee et al., 2004). The western shelf area of the South Yellow Sea (SYS) is a convergence zone with a wide shelf and multiple terrestrial sediments. The sediments from the Huanghe River (HH), Changjiang River (CJ), and several other small and medium-sized rivers converge, resuspend, transport, and deposit in this area, making it a natural laboratory for studying marine sedimentary environments and terrestrial sediment characteristics.
Since the 1980s, significant progress has been made in the study of the differentiation and distribution of terrestrial sediments in the SYS shelf area (Qin, 1989; He, 2006). This includes analyzing the differences in sediment composition between the CJ and the HH based on heavy mineral assemblages and the proportion of clay minerals, as well as determining the distribution area of river-derived clay minerals in the sea (Soo-Chul et al., 2000; Han et al., 2022). By analyzing whole samples or fine-grained geochemical elements, various indices such as trace elements, rare earth elements, and their ratios have been identified to discriminate river-derived sediments in the SYS (Yang and Li, 2000; Yang and Youn, 2007; Zhou et al., 2015). Additionally, studies have found that parameters such as Ti, Cr/Th, Ti/Nb, and Zr/Nb in sediments can reliably identify the contributions of the CJ, the HH, and Korean rivers to the sediment composition in the Yellow Sea (Yang et al., 2003a, b). However, the high degree of mixing of different materials in whole samples or the influence of different mineral types at limited grain sizes may introduce uncertainties in source analysis.
With the advancement of provenance analysis, some heavy minerals have been found to reflect differences in source rock types and origins, providing a basis for determining specific material sources (Morton et al., 2005; Nie et al., 2010; Safonova et al., 2010; Krippner et al., 2016; Wang et al., 2018; Shang et al., 2021; Jin et al., 2022). Among them, the combination of geochronology and mineral geochemical elements of garnet can identify the sources of magmatic origin components, while monazite can be used to analyze the sources of metamorphic origin components (Guo et al., 2020). Zircon U-Pb ages can quantitatively analyze the contribution of the Huanghe River to the Changjiang River subaqueous delta (Shang et al., 2021). Yang et al. (2004) conducted a study on the chemical composition of magnetite in surface sediments in the eastern South China Sea, TiO2-Al2O3-MgO genetic classification diagram method indicates that the magnetite in the sediments is mainly derived from intermediate-acidic and basic magmatic rocks. However, most of these individual minerals are accessory minerals and only exist in certain types of rocks, which may result in the loss of some source signals.
Amphibole, as one of the major rock-forming minerals, is widely distributed in sediments from rivers and marine areas worldwide (Carver et al., 1972; Yue et al., 2018; Fan et al., 2021). In addition, amphiboles in rivers at mid to high latitudes have low weathering degrees and show little variation in chemical elements. Amphiboles derived from different rocks under different diagenetic and mineralization conditions have specific compositions and structural characteristics, which can clearly reflect the differences in source rock types and sedimentary environments, providing a basis for provenance analysis (Liu, 1986; Zhao et al., 1993; Hawthorne et al., 2012; Jin et al., 2013; Fan et al., 2021). The Mg-(Na+K+Ca)-(Fe2++Fe3+) ternary diagram can be used to differentiate the genetic types of amphiboles (Chen et al., 1988). The analysis of different rivers such as the Liaohe River, Yalu River, Huanghe River, Changjiang River, and Huaihe River, have provided a systematic study of the origin characteristics, crystallization temperature and pressure conditions, and geochemical features of amphiboles in mid-high latitude rivers in China (Jin et al., 2014; Wang, 2019; Fan et al., 2021). With the deepening of research on the source-to-sink system, further studies on the sedimentary clastic amphiboles in marginal seas and continental shelves are needed.
In this study, we analyze the source rock types and depositional environments of clastic minerals in the continental shelf area, tracing them back to the rock types in the watershed region for provenance identification. Using this approach, we conduct electron probe element analysis on clastic amphiboles in the SYS, classify the amphibole species, and match them with the characteristics of riverine amphiboles. This allows us to verify the backward tracing of riverine sediments from sink to source, connecting the watershed-continental shelf sediment transport system. This research has significant implications for understanding the evolution of this important source-sink system from rivers to the ocean.
2 Samples and methods
2.1 Samples
This study utilized surface sediment samples from the western South Yellow Sea. Six groups of sediment samples were collected from the SYS during the summer comprehensive cruise in 2014, as part of the Major Scientific Research Program of the Ministry of Science and Technology. DGPS positioning was used, the accuracy was about 1 m. The sediment samples were collected using a box corer with a sampling volume of about 2 kg for each sample, which was stored in self-sealing bags. The study area and sampling points are shown in Figure 1.
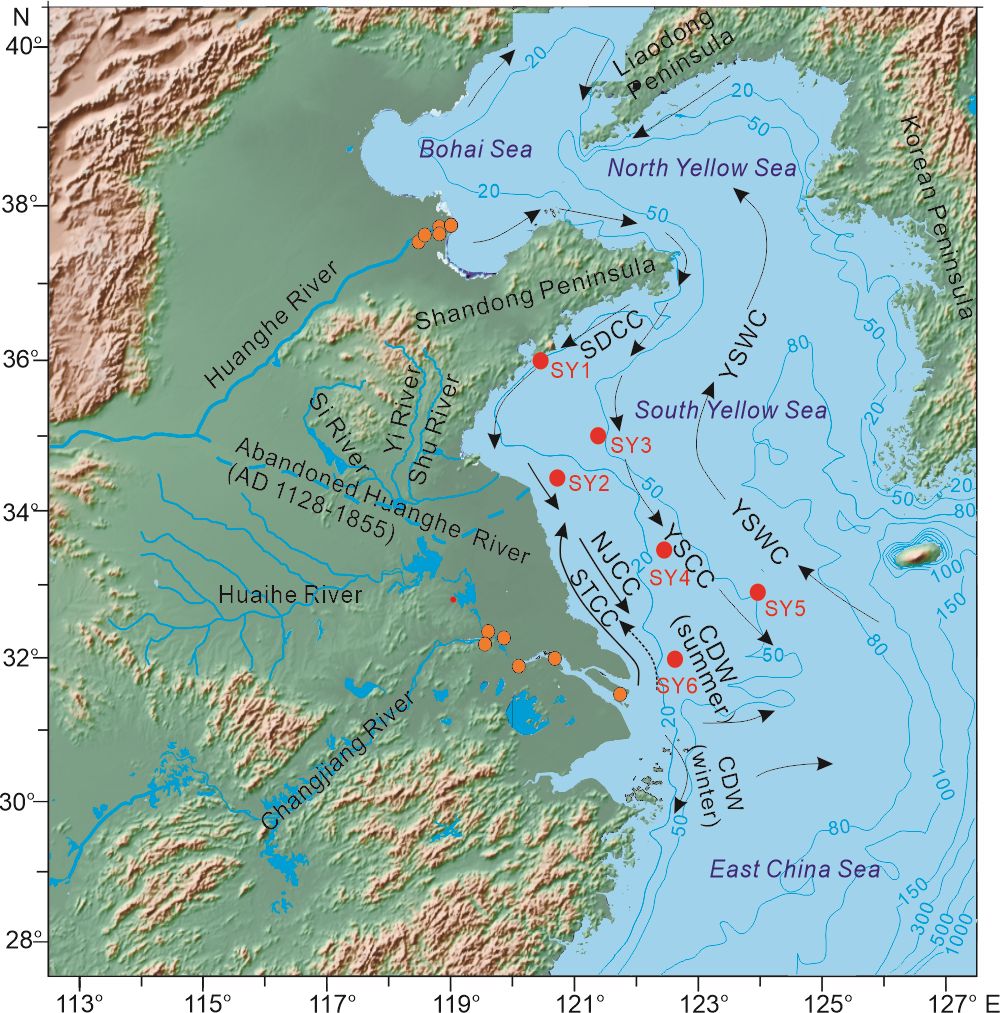
Figure 1 Location map showing the study area and sampling sites of sediments. The red dots in the figure were the sampling points of the surface samples of the South Yellow Sea in this paper. Yellow dots were watershed sampling points, and the data is from Wang, 2019; Fan et al., 2021. Circulation information cited in Qiao, 2011. SDCC, Shandong Coastal Current; YSWC, Yellow Sea Warm Current; YSCC, Yellow Sea Coastal Current; NJCC, North Jiangsu Coastal Current; STCC, Subei tide-induced coastal current; CDW, Changjiang Diluted Water.
2.2 Methods
2.2.1 Clastic amphibole selection
Approximately 500 g of each surface sediment samples from the SYS were collected. Wet sieving was performed using sieves with apertures of 3Φ (0.125 mm) and 4Φ (0.063 mm). The sediment, which had been sieved to a grain size of 3Φ - 4Φ, was subsequently dried and its mass was measured. Following this, the resulting fine-grained clastic sand was subjected to a light-heavy mineral separation process using bromoform (CHBr3), which has a density of 2.89 g/cm3. The mineral separation lab was maintained at a constant temperature of 20°C, and the sediment was stirred three times with a glass rod at 15-minute intervals, combined with centrifugal force to allow heavy minerals to settle. After 8 hours of settling, the floating and settled minerals were separated, washed with anhydrous ethanol, dried, and weighed to obtain the light and heavy mineral samples.
2 g of mineral samples were placed in diiodomethane (CH2I2, density of 3.32 g/cm3) for amphibole flotation. The floated samples were rinsed by dimethyl sulfoxide, dried, and then examined under a stereomicroscope for the selection of amphibole mineral particles. In each sample group, 200 randomly selected amphibole particles were chosen, excluding those with severe alteration, weathering, or inclusions.
2.2.2 Amphibole electron microprobe elemental analysis
As a means of in-situ micro analysis, electron probe analysis can rapidly determine the elemental composition of solid sample surfaces (Reed, 1996). We conducted in-situ micro analysis on each amphibole grain to test for the content of main elements such as SiO2, FeO, MgO, CaO, Al2O3, Na2O, TiO2, K2O, MnO. Using 23 oxygen atoms as a reference, the cationic numerical values and characteristic values in the amphibole crystal structure formula were calculated to classify the amphibole, assign names, and determine the source rock type.
Each sample was randomly selected to obtain approximately 60 grains of amphibole for thin section preparation. The mineral grains were first mounted on double-sided adhesive tape and covered with a target mold coated with petroleum jelly. Epoxy resin and curing agent were poured into the mold and cured in a constant temperature oven (60°C) for 24 hours. The amphibole grains were then polished to expose a flat surface, approximately 1/3 or 1/2 of the grain, and coated with a carbon film about 20 nm thick. Subsequently, in situ geochemical analysis of the amphibole was conducted using the electron probe microanalyzer (JEOL JXA-8100) equipped with four spectrometers. The quantitative analysis of amphibole geochemical elements was performed under the following conditions: acceleration voltage of 15 kV, beam current of 10 nA, and beam spot diameter of 3 μm. The standard samples used were as follows: jadeite (Na), olivine (Mg), albite (Al, Si), orthoclase (K), diopside (Ca), rutile (Ti), rhodonite (Mn), and hematite (Fe). The aforementioned experiments were carried out at the Laboratory of Marine and Coastal Geology, Third Institute of Oceanography, Ministry of Natural Resources.
3 Results
3.1 Geochemical elements characteristics of amphiboles in the SYS
A total of 258 very fine sand-sized (3Φ - 4Φ) clastic amphibole grains from six stations in the surface sediments of the SYS were analyzed using an electron probe microanalyzer to determine the constant elements in each amphibole grain (Table 1). The clastic amphiboles in the South Yellow Sea have the highest SiO2 content, with an average content exceeding 45% and a maximum value of 46.9%. The next highest element is FeO, with a content ranging from 14.6% to 16.3%, showing a decreasing trend from north to south. The average content of MgO and CaO in the SYS amphiboles is above 11%, with little difference between the two. The content of MgO increases from north to south, opposite to the trend of FeO. The average content of Al2O3 is around 9.7%, varying between 9.1% and 10.3%. The content of Na2O shows little variation and is below 1.3%. The average content of TiO2 and K2O is relatively low, at 0.8% and 0.7% respectively, both less than 1%. The lowest content is MnO, ranging from 0.30% to 0.35%, with an average content of 0.33%. Overall, clastic amphiboles in the surface sediments of the SYS exhibit high silicon, high calcium, high iron and magnesium, and low sodium and potassium content in different locations.
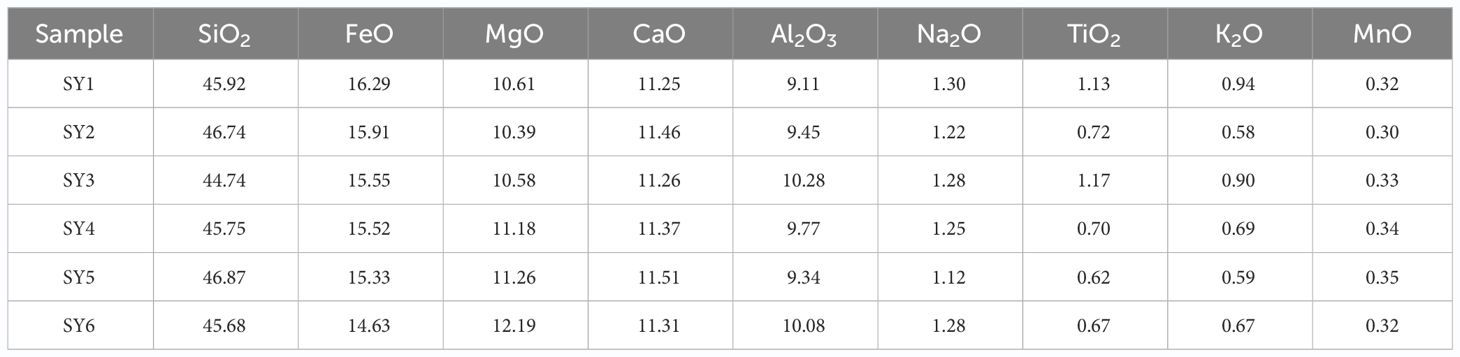
Table 1 Average content of major elements in clastic amphibole in the surface of the South Yellow Sea (%).
3.2 Classification of amphibole species in the SYS
The charge arrangement and B-site cation types, recognized by the International Mineralogical Association (IMA), are used for the classification and naming of amphiboles (Hawthorne et al., 2012). The sum of B-site cations is ΣB = BLi + BNa + BΣM2+ + BCa, where BΣM2+ = BMg + BFe2+ + BMn2+. It was found that among the 258 very fine sand-sized amphibole grains in the surface sediments of six stations in the South Yellow Sea, B(Ca + ΣM2+)/ΣB ≥ 0.75 and BCa/ΣB ≥ BΣM2+/ΣB, indicating that they belong to the calcium amphibole subfamily.
To further classify the amphibole species, the cation values in the A-site and C-site of the amphibole crystal structure was used as a criterion, with the total charge written as: A+ = A (Na + K + 2Ca), C+ = C (Al + Fe3+ + 2Ti4+), where cations are expressed in apfu. The diagram uses C (Al + Fe3+ + 2Ti4+) apfu as the x-axis and A (Na + K + 2Ca) apfu as the y-axis, completing the nomenclature of amphibole species in the South Yellow Sea (Figure 2). Finally, the number of each amphibole species was counted to calculate their respective proportions.
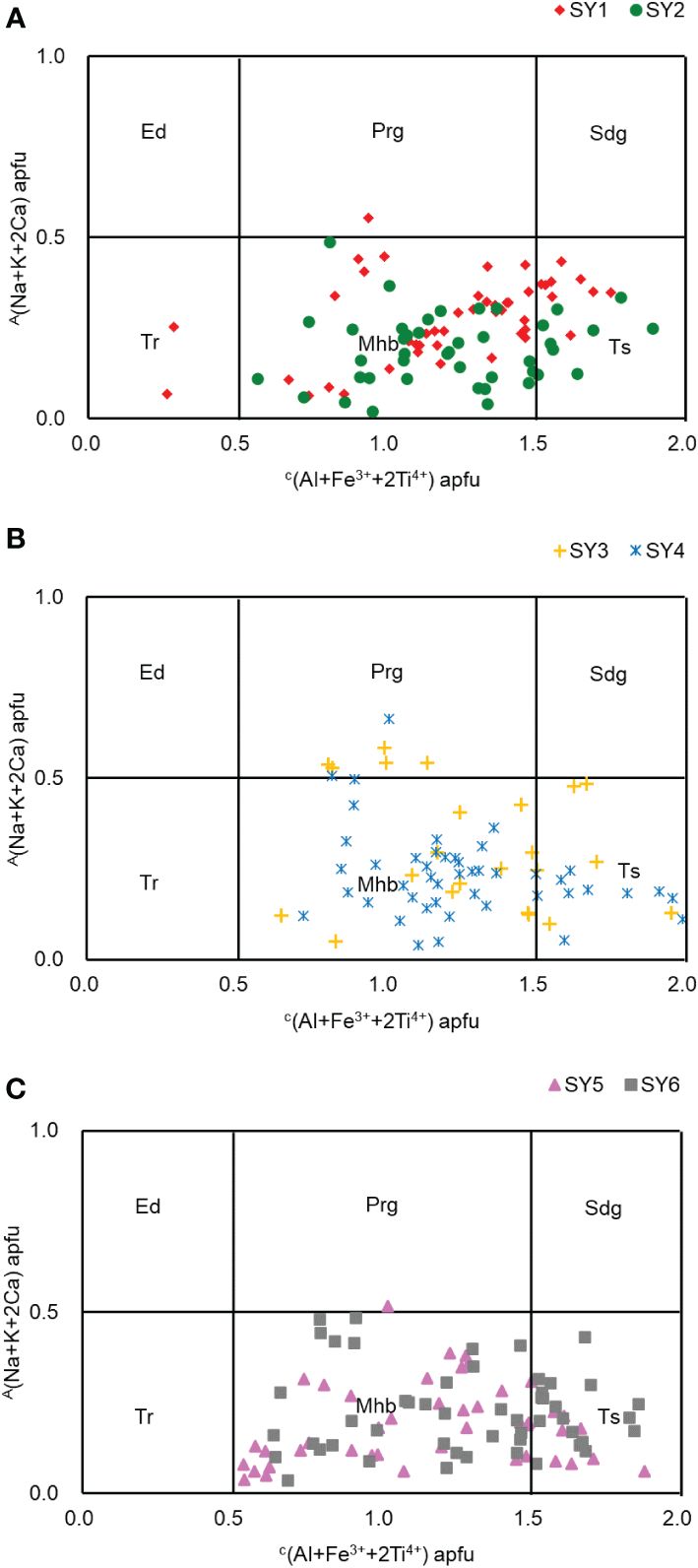
Figure 2 Classification of clastic amphibole in the surface sediments of the South Yellow Sea (referenced from Hawthorne et al., 2012). Ed, Edenite; Prg, Pargasite; Sdg, Sadanagaite; Tr, Tremolite; Mhb, Magnesiohornblende; Ts, Tschermakite. (A–C) represent the different classification of amphibole from different sites in the South Yellow Sea.
The quantity and content of various amphibole in surface samples of the SYS were classified and analyzed. Among the samples, the content of magnesiohornblende was the highest, ranging from 52.2% to 81.4%, with the majority being above 70%. The average content of magnesiohornblende in the northern part of the SYS (SY1, SY2, SY3) was 70%, while in the southern part (SY4, SY5, SY6) it was 72%. The second most abundant amphibole was tschermakite with the highest content found in sample SY6 at 35.3%, and the lowest in sample SY1 at 18.4%. The average percentage of tschermakite in surface sediments of the South Yellow Sea was 23.4%, showing an increasing trend from north to south. Additionally, a certain amount of pargasite was found in the samples, with the highest content of 21.7% in SY3, and an average of around 3% in SY1, SY4, and SY5. Furthermore, SY1 also contains 4.1% of tremolite.
3.3 Source rock types indicated by amphibole in the SYS
In amphibole, Ti and Si are generally present in the form of tetravalent cations in the T and C sites, which are constant elements in amphibolite. Ti is greatly influenced by changes in crystallization temperature, with higher temperatures in crystal formation and geological processes resulting in higher Ti content. The Si content in amphibolite varies in different magma types, including ultrabasic, basic, intermediate, acidic or alkaline igneous rocks, as well as different metamorphic rocks (Chen et al., 1988). Therefore, the Si-Ti variation diagram can clearly reflect the source rock genesis type of amphibolite.
Projecting the Si and Ti cation counts of amphibole in the surface samples of the SYS onto a scatter plot of amphibole genesis (Figure 3). The majority of amphiboles in surface samples from the South Yellow Sea are derived from medium-acidic intrusive rocks and metamorphic rocks, with a small portion originating from ultrabasic-basic magmatite and retrogressive metamorphic or metasomatic rocks, and a few amphiboles derived from volcanic rocks. In terms of details, the proportions of different rock types indicated by amphiboles vary in different regions of the SYS. For example, SY1 from the northern part of the SYS, the content of amphiboles in medium-acidic intrusive rocks is 28.3%, and the proportion of metamorphic clastic amphiboles is around 24%. SY3 from the northern part of the estuary of the Huanghe River, which has similar characteristics to SY1, has a content of metamorphic and medium-acidic intrusive amphiboles of 32%; both samples have a few amphiboles falling into Class I, which belong to volcanic amphiboles in volcanic rocks. The content of amphiboles of different origins in samples SY2 and SY4 is similar, with amphiboles in medium-acidic intrusive rocks exceeding 50%. In comparison, SY2 has more retrogressive metamorphic or metasomatic genesis amphiboles than SY4. SY5 and SY6 are located in the southeastern and southern parts of the SYS, respectively. The content of amphiboles in medium-acidic intrusive rocks is around 43%, with the rest being metamorphic amphiboles; both samples have very little content of ultrabasic-basic volcanic rock amphiboles.
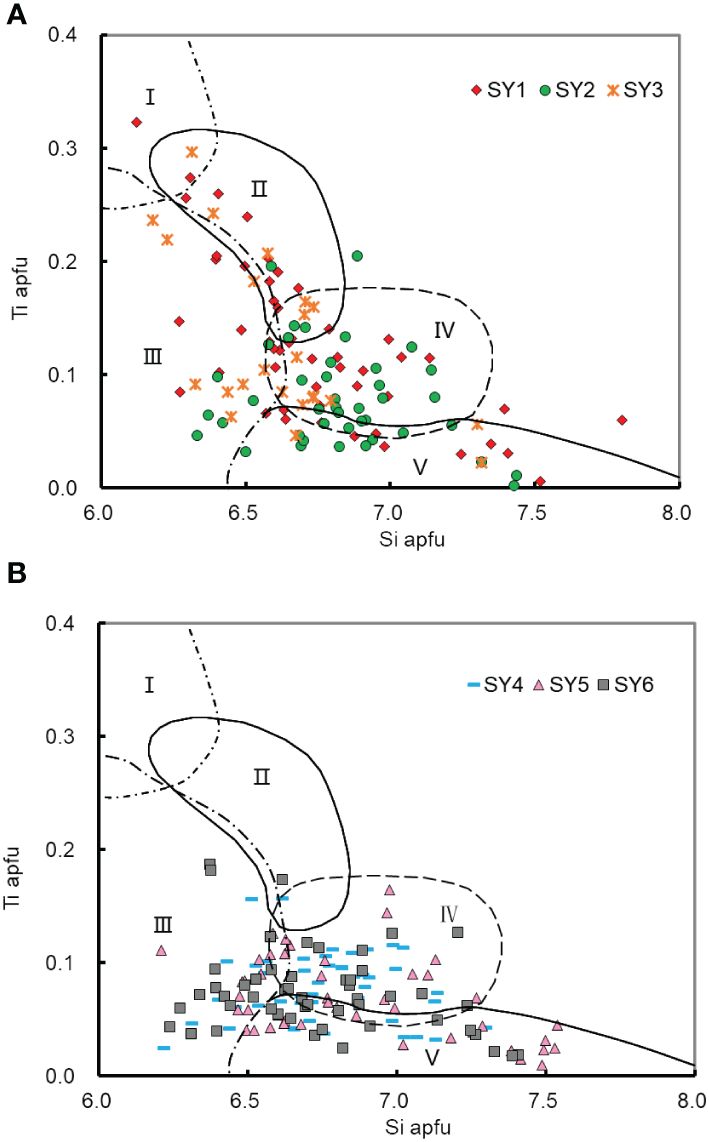
Figure 3 Si-Ti variation diagram and genetic classification of amphiboles in surface sediments of the South Yellow Sea (referenced from Chen et al., 1988). I = Magmatic amphiboles in volcanic rocks; II = Magmatic amphiboles in ultrabasic-basic rocks; III = Amphiboles in metamorphic rocks; IV = Amphiboles of magmatic genesis in mid-acid intrusive rocks; V = Amphiboles of retrogressive metamorphic or metasomatic genesis, (A, B) represent the different genetic types of surface samples from different sites in the South Yellow Sea.
4 Discussion
4.1 Genetic analysis of clastic amphibole in SYS
4.1.1 Genesis of magmatic amphibole
In the surface sediments of different stations in the South Yellow Sea, 120 medium-acid intrusive amphiboles were projected onto a Ca-Fe2++Fe3+-Mg ternary diagram, and divided into different magma source types (Figure 4). Among them, the crust-mantle hybrid amphiboles accounted for 80% in samples SY1 and SY2, with amphibole species being magnesiohornblende and edenite; the mantle-derived amphibole accounted for 20%, all of which were magnesiohornblende species in the calcium amphibole subfamily. Similarly, in SY3 located in the northern sea area of the study area, the proportion of crust-mantle hybrid amphibole was 77.8%, mostly edenite and magnesiohornblende; the mantle-derived amphibole accounted for 22.2%, and the amphibole species belonged to magnesiohornblende. SY4 and SY5 located in the southern part of the western shelf area of the SYS, the magma source types of medium-acid intrusive amphiboles were similar, with the proportions of crust-mantle hybrid amphiboles being 88.5% and 88.2% respectively, and the proportions of mantle-derived amphiboles being less than 12% in both samples. SY6 was located in the offshore area near the estuary of the CJ with the proportion of crust-mantle hybrid amphiboles from medium-acid intrusive magma sources being 87%, and the proportion of mantle-derived amphiboles being 13%. In summary, the medium-acid intrusive clastic amphiboles in different stations in the SYS are mostly crust-mantle hybrid amphiboles, followed by mantle-derived amphiboles, and no crust -derived amphiboles were found. The proportion of mantle-derived amphiboles in the northern part of the western shelf area of the SYS is higher than that in the southern part, with an average difference of about 10%.
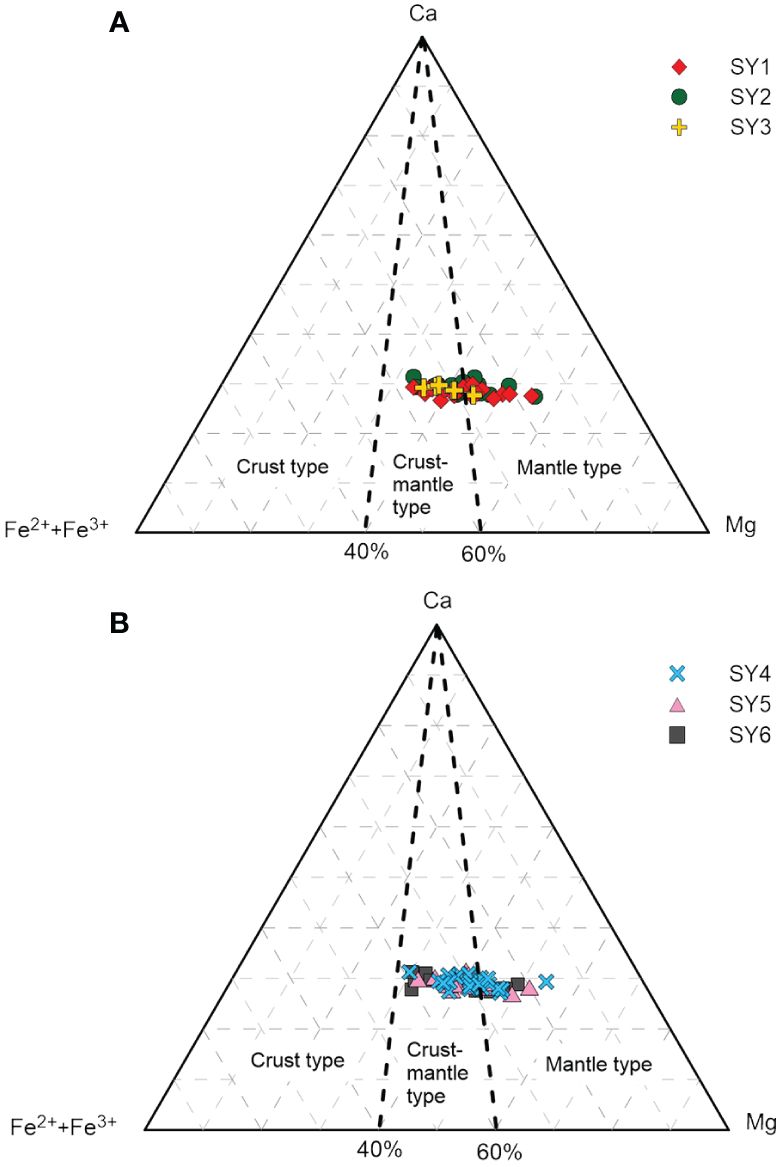
Figure 4 Magmatic source species of acidic intrusion type igneous amphibole in the surface sediments of the South Yellow Sea (referenced from Xue, 1991). (A, B) represent the magma source types of surface samples from different sites in the South Yellow Sea.
4.1.2 Genesis of metamorphic amphibole
During metamorphism, high content of IVAl in amphibole indicates a strong isomorphism with Si, representing a higher temperature during mineral formation; high content of VIAl indicates greater pressure during mineral formation (Xue, 1991). By projecting the metamorphic amphiboles (70 particles) into a diagram showing the relationship between VIAl-Si regional metamorphic pressure (Figure 5), it can be observed that most of the surface metamorphic amphiboles in the SYS have pressures below 500 mPa, indicating a medium to low-pressure metamorphic origin. A small amount of amphiboles belong to a high-pressure metamorphic origin. Specifically, all the metamorphic amphiboles in sample SY1 are of low-pressure origin, while sample SY3 has the highest proportion of high-pressure metamorphic amphiboles, accounting for 33%. Amphiboles of high-pressure metamorphic origin in samples SY4 and SY6 rank second, accounting for 25% and 23.5% respectively. The content of high-pressure metamorphic amphiboles in SY2 and SY5 is 16.7% and 15.4% respectively, showing a trend of lower content of nearshore high-pressure metamorphic amphiboles overall.
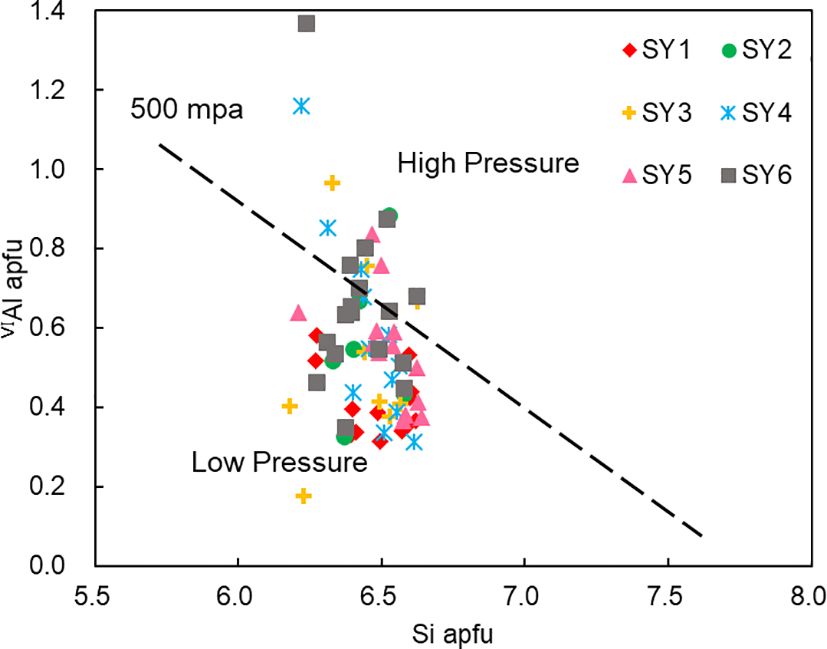
Figure 5 Illustration of the metamorphic genesis of amphibole VIAl-Si in the surface of the South Yellow Sea (referenced from Johnson and Rutherford, 1989).
4.2 Significance of the provenance of amphibole in the SYS
4.2.1 Identification of seaward stream sediments
The electron probe element analysis of clastic amphibole from the Huanghe River and Changjiang River has been systematically studied. There are differences in the FeO and MgO contents of amphibole from the Changjiang and Huanghe rivers. The FeO and MgO contents in amphibole from the CJ are 14.4% and 12%, respectively, while those from the HH are 16.1% and 10.6%, respectively (Jin et al., 2013; Wang, 2019; Fan et al., 2021). When projecting the amphibole from different stations in the South Yellow Sea onto a FeO-MgO scatter plot (Figure 6A), distinct zones are observed for amphibole from the CJ and HH. The samples from stations (SY1, SY2, and SY3) in the northwestern part of the South Yellow Sea are closer to the HH, while the SY6 sample in the southern research area of the Yellow Sea is located near the CJ, indicating a greater influence from the CJ in this region. The SY4 and SY5 samples are located between the CJ and HH, indicating a combined influence from both rivers. Among them, the SY4 sample tends to be more influenced by the Huanghe River, while the SY5 sample is more influenced by the CJ.
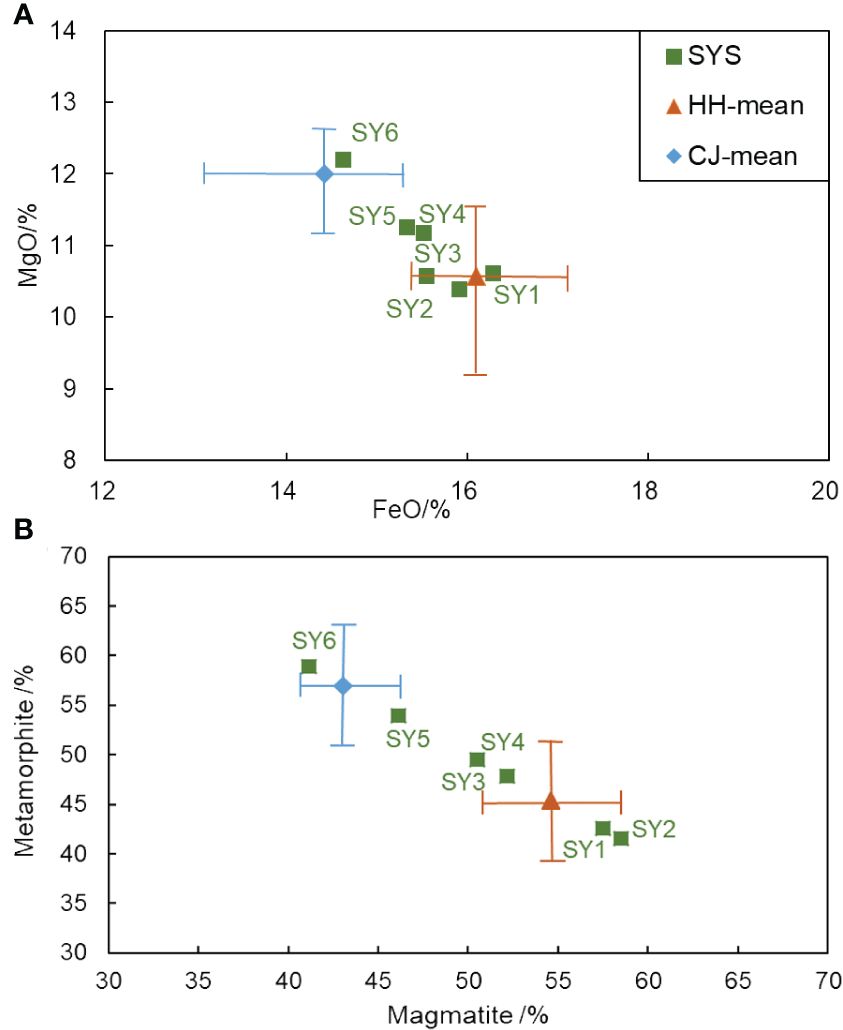
Figure 6 Scatterplot of provenance identification of amphibole in the South Yellow Sea (SYS). (A), FeO-MgO content; (B), proportion of source rock types. Average value of Huanghe River (HH-mean) and Changjiang River (CJ-mean) referenced from Jin et al., 2013; Wang, 2019; Fan et al., 2021.
The clastic amphibole in the HH and CJ originates from different types of magmatic and metamorphic rocks. By analyzing the proportions of source rock types indicated by clastic amphibole at the estuaries of the CJ and HH, it is found that the content of clastic amphibole of metamorphic origin in the CJ is slightly higher than that in the HH, accounting for 56.9% and 45.4% respectively. The Huanghe River, on the other hand, is characterized by a higher content of clastic amphibole of magmatic origin, accounting for over 54% (Figure 6B). Regarding the content of source rock types indicated by clastic amphibole in different stations in the SYS, samples from SY1, SY2, and SY3 located in the northern part of the western shelf area of the SYS are closer to the characteristic composition of the HH source rock types. The amphibole in these samples are mostly columnar and short columnar, appearing in dark green, grass green, and occasionally brown colors, with subangular shapes and some subrounded shapes, exhibiting similarities to the characteristics of amphibole s in Huanghe River sediments, indicating a significant influence from the HH.
The sediment of the HH is influenced by the Shandong Coastal Current (SDCC), flowing eastward from the northern part of the Shandong Peninsula along the Bohai Bay and turning south at Chengshan Head, entering the SYS; then flowing southward along the Subei tide-induced coastal current (STCC), with the front reaching as far as near 30°N (Qin et al., 2018). The central region of the SYS is influenced by both the HH and the CJ. In sample SY4, the average proportions of clastic amphibole of magmatic and metamorphic genesis are 50.5% and 49.4% respectively, with a larger contribution from the HH. In sample SY5, the average proportions of clastic amphibole of magmatic and metamorphic genesis are 46.1% and 53.9% respectively, indicating a larger contribution from the CJ.
SY6 shows similar characteristics to the CJ in terms of the proportions of source rock types of clastic amphibole, with a higher proportion of clastic amphibole of metamorphic origin than magmatic origin, indicating that the southern sea area of the SYS is mainly supplied by the CJ. Furthermore, in SY6 sample, the amphiboles are predominantly columnar, green in color with some light green, and a relatively higher proportion of brown amphiboles. They exhibit poor roundness, subangular shapes, low degree of weathering, and are more similar to the characteristics of amphibole particles in the CJ sediments. The sediment entering the SYS from the CJ mainly undergoes long-distance transportation under the influence of the Changjiang Diluted Water (CDW), Subei tide-induced coastal current (STCC), and the Yellow Sea Warm Current (YSWC) (Qiao, 2011). The northward expansion of these water masses is significantly influenced by the wind field, with the East Asian monsoon playing an important role in the material entering the SYS from the CJ (Hori, 2001).
In summary, the northwestern part of the South Yellow Sea shelf area (SY1, SY2, and SY3) is influenced by the sediments from the HH, while the southern sea area (SY6) is mainly supplied by the CJ. The central part of the study area (SY4) is a mixed zone of sediments from the HH and CJ, with a slightly higher supply of sediments from the HH. In the southeastern part (SY5), the supply of sediments from the CJ is slightly higher than that from the HH. Furthermore, both the HH and the CJ contain calcic amphiboles, mainly consisting of magnesiohornblende. These amphiboles are predominantly derived from medium-acid intrusive rocks, followed by metamorphic rocks and retrograde or metasomatic rocks. The amphiboles derived from medium-acid plutonic rocks are mostly of crust-mantle origin, followed by mantle origin. Most of the metamorphic-derived amphiboles belong to the medium-low pressure metamorphic type (Fan et al., 2021). The above characteristics of amphiboles in rivers are reflected in the SYS. Therefore, the types and genetic characteristics of amphiboles are well inherited from rivers to the sea, making them a “powerful tool” for provenance analysis.
4.2.2 Tracing of source rock types
Tracing the bedrock and sediment types in the areas where the HH and CJ (Figure 7). The main source of sediment in the HH comes from the Loess Plateau in the middle reaches of the river, which covers about 2/3 of the total area of the Huanghe River basin (Wu et al., 2020). The sediment consists mainly of thick loess deposits, ranging from 100 to 200 meters in thickness, with some Triassic and Cretaceous sandstone debris. The grain size and mineral composition of the HH sediment largely inherit the characteristics of the loess. The majority of the amphiboles in the river also originate from the loess, which in turn originated from the Gobi Desert and surrounding orogenic belts in western China, where Paleozoic granitic rocks and pre-Cambrian metamorphic rocks (mainly gneiss) are widely developed (Liu, 1985). In addition, the magmatic rocks in the HH basin are fully developed, and granite is widely exposed, while there are also some metamorphic rocks, limestone and clastic rocks (Zhai, 2012). This results in a diverse range of amphibole types and complex origins in the HH, making it a representative of the mineral composition of the crust in the mid-latitude Asian continent.
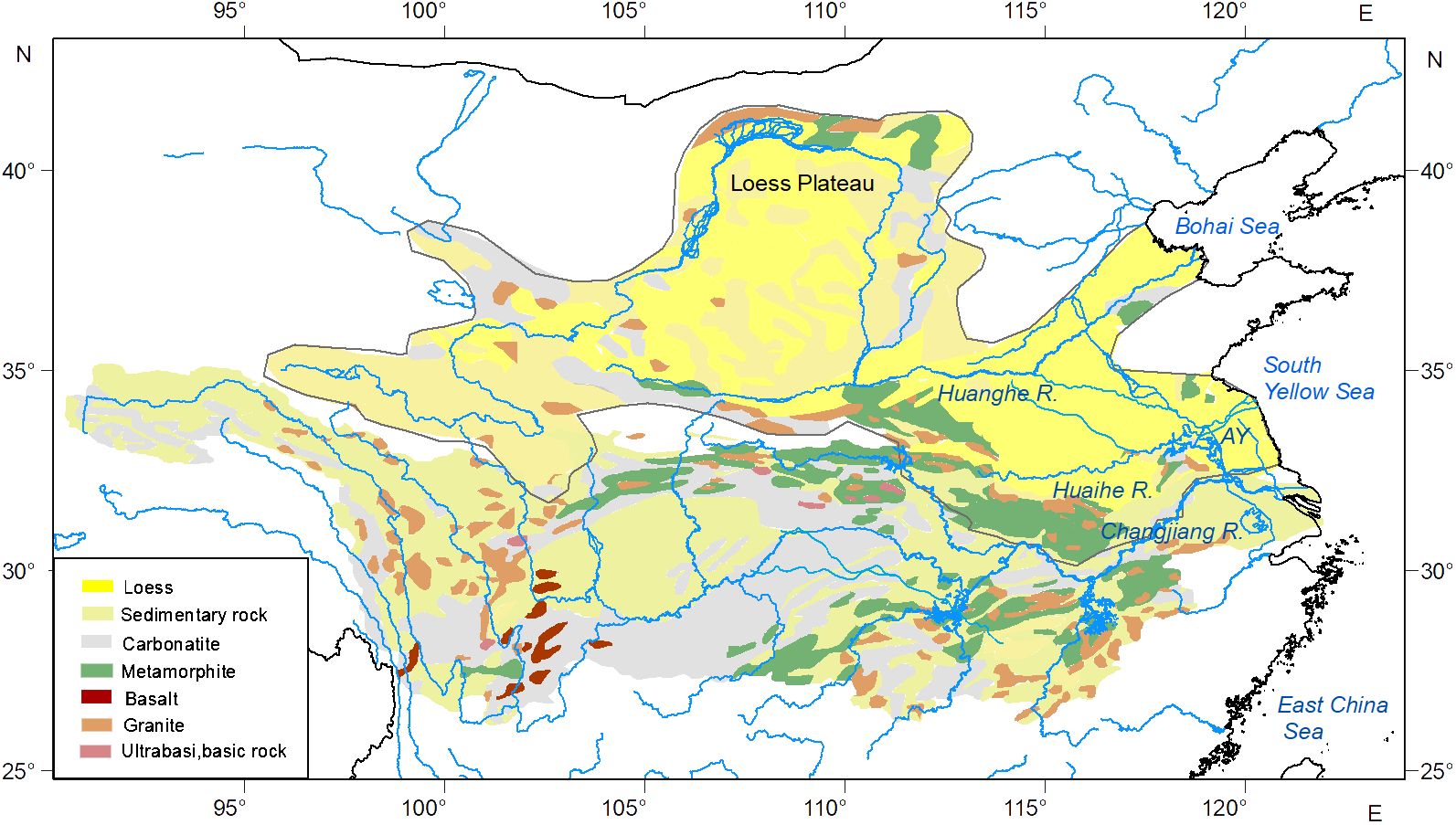
Figure 7 Geological map of the Changjiang, Huanghe - Huaihe rivers basin. Modified from Liu and Wang, 2002.
In the Changjiang River basin sedimentary rocks, metamorphic rocks and magmatic rocks are exposed, and the tributaries flow through various geological structural zones, and the different layers are well-developed in different areas, resulting in a complex and diverse range of rock types (Ministry of Water Resources, 1999). In the upper reaches of the CJ, the prominent rock formations are the Emei Mountain basalt and the Neogene granite. Other exposed rock formations include carbonate rocks, metamorphic sandstone, red clastic rocks, and potassium-rich granite (Hou et al., 1999). In the middle reaches of river, the main rock types are metamorphic rocks and Early Paleozoic medium-acid intrusive rocks. Quaternary loose sediments and lacustrine sedimentary rocks are widely distributed, with fewer occurrences of carbonate rocks and sporadic occurrences of ultrabasic-basic rocks (Yang and Li, 1999). In the lower reaches of the CJ, the dominant rock type is Quaternary loose sediments, with a small amount of Cretaceous medium-acid intrusive rocks and acidic magmatic rocks (Chen et al., 2001). The sediment in the CJ mainly originates from the middle and upper reaches, where basic volcanic rocks and calcium-magnesium carbonate are widely distributed in the upper reaches, and the middle reaches are characterized by abundant medium-acid intrusive rocks and metamorphic rocks (Vezzoli et al., 2016). These areas are also associated with extensive magmatic activity, hydrothermal activity, and metamorphism, which are the main causes of the development of amphiboles in the CJ. The clastic amphiboles in the HH and CJ estuaries inherit the characteristics of their source rocks, and can be used as geochemical trace element indicators to further determine the contribution and diffusion range of riverine sediments in the marine area.
4.3 Follow-up research
The South Yellow Sea is a region where multiple sources of sediments converge. It is influenced not only by the Huanghe and Changjiang rivers, but also by medium and small - sized rivers, residual sediments, offshore or coastal erosion materials, atmospheric transport, and even cosmic dust. Among these, cosmic dust can be ignored due to its negligible proportion. Meanwhile, there is a lack of suitable end-member samples for atmospheric transport, submarine residual sediments, and coastal erosion materials, making it difficult to identify end-member sources. In addition, the sediments from the open ocean in the SYS are mostly biogenic siliceous and calcareous clay.
The South Yellow Sea is influenced by multiple small and medium-sized rivers such as the abandoned Huanghe River, Huaihe River, and peninsula rivers (Liaodong Peninsula, Shandong Peninsula, Korean Peninsula). The abandoned Huanghe River, formed by historical channel changes of the Huanghe River, has sediment characteristics similar to the Huanghe River. The sediment transport of the Huaihe River is only 7.2 Mt yr-1, with 70% of the sediment entering the Changjiang River and flowing into the sea (Liu et al., 2008). The peninsula rivers have short flow paths and steep gradients, and their sediment supply to the sea is mainly composed of fine sand, making them one of the main sources of coarse-grained sediment in the marginal sea area. Especially in the Shandong Peninsula, which extends deep into the Yellow Sea, the small and medium-sized rivers have long-term stability in delivering land-derived clastic sediments to the sea. Compared to the vast river basins composed of numerous tributaries of major rivers, the Shandong Peninsula has a smaller drainage area, lower complexity of lithology, and relatively uniform natural conditions such as climate and hydrodynamics, making it more suitable for the analysis of end-member characteristics under limited boundary conditions.
Future research can focus on analyzing the content of multiple grain-size heavy minerals and extracting the characteristic identification of amphibole elements that are unique to the small and medium-sized rivers in the Shandong Peninsula. This will help establish a heavy mineral-amphibole provenance tracer system and a mathematical model for quantitative estimation, deepening our understanding of the impact and contribution of the Shandong Peninsula as a source of sediment on the Yellow Sea shelf.
5 Conclusions
(1) Through electron probe testing of the major elements of amphiboles in the SYS, it was found that clastic amphiboles have a general characteristic of high Si and Ca content, high Fe and Mg content, and low Na and K content. The clastic amphiboles belong to the calcic amphibole subfamily, predominantly consisting of magnesiohornblende with a content exceeding 70%. Tschermakite exhibits the second highest abundance, while tremolite and pargasite are present in varying quantities.
(2) Most of the amphibole in the SYS originates from medium-acidic intrusive rocks and metamorphic rocks. Among them, the amphibole in medium-acidic intrusive rocks is mostly mantle-crust type, followed by mantle type, and a few are derived from crustal sources. The amphibole of metamorphic origin is mostly formed under pressures below 500 mPa, belonging to medium-low pressure metamorphic processes.
(3) The genesis characteristics of amphibole in the SYS were compared with those of the HH and CJ, the source rock types in these two river-basins were traced, which confirmed that the main source of the study area sediments are from these two rivers. The western shelf area of the SYS is influenced by different river, forming distinct zones. The northern sea area is influenced by the HH, while the southern sea area is mainly supplied by the CJ. The central area is a mixed zone of HH and CJ, with slightly higher supply from the HH. In the southeastern area, the supply of CJ is slightly higher than that of the HH.
(4) The amphibole inherits different source rock types from various river basins, establishing a common link between the source rocks, watersheds, and marine sediments, making it a powerful tool for terrestrial-marine provenance analysis. Future research should also consider other sediment sources, such as the supply from medium and small-sized rivers in the Shandong Peninsula, in order to deepen and expand the study on the influence and contribution of multiple sources of material in the marine area.
Data availability statement
The raw data supporting the conclusions of this article will be made available by the authors, without undue reservation.
Author contributions
MW: Data curation, Writing – original draft. BJ: Conceptualization, Methodology, Writing – review & editing. JL: Validation, Writing – review & editing. MG: Funding acquisition, Supervision, Writing – review & editing. JG: Resources, Supervision, Writing – review & editing. JJ: Funding acquisition, Writing – review & editing.
Funding
The author(s) declare financial support was received for the research, authorship, and/or publication of this article. This work was financially supported by the National Natural Science Foundation of China (Grant No. U2106203; No. 41977173; No. 41330964; No. 41876092), the National Geological Survey Project of China Geology Survey (No. DD20221775), Qingdao Postdoctoral Applied Research Project (QDBSH20240102122) and the China-ASEAN maritime cooperation fund (Cooperative researches on the marine geo-environments and geo-hazards in the Yangtze River and the Red River deltas).
Conflict of interest
The authors declare that the research was conducted in the absence of any commercial or financial relationships that could be construed as a potential conflict of interest.
Publisher’s note
All claims expressed in this article are solely those of the authors and do not necessarily represent those of their affiliated organizations, or those of the publisher, the editors and the reviewers. Any product that may be evaluated in this article, or claim that may be made by its manufacturer, is not guaranteed or endorsed by the publisher.
References
Carver R., Douglas L., Yoshiki S. (1972). Procedures in sedimentary petrology. Soil Sci. 114, 500. doi: 10.1097/00010694-197212000-00027
Chen G. Y., Sun D. S., Yin H. A. (1988). Genetic mineralogy and prospecting mineralogy (Chongqing: Chongqing Press).
Chen Z. Y., Li J. F., Shen H. T., Wang Z. H. (2001). Yangtze River of China: historical analysis of discharge variability and sediment flux. Geomorphology 41, 77–91. doi: 10.1016/S0169-555X(01)00106-4
Fan S., Jin B. F., Yue W., Dang L. L., Wang M. Y., Kong Q. X. (2021). Type and genesis of amphibole in the Huanghe River and Changjiang River estuaries and significance of its provenance. Geosci J. 25, 575–587. doi: 10.1007/s12303-020-0061-4
Guo R. H., Hu X. M., Garzanti E., Lai W., Mark C. (2020). How faithfully do the geochronological and geochemical signatures of clastic zircon, titanite, rutile and monazite record magmatic and metamorphic events? A case study from the Himalaya and Tibet. Earth-Sci. Rev. 201, 103082. doi: 10.1016/j.earscirev.2020.103082
Han Z. Z., Wang Y. B., Sun Y. G., Ai L. N., Wu X., Yang Y. P. (2022). Composition of minerals in surface sediments of the Yellow Sea and their provenance. Mar. Geol. Frontiers 38, 10–19.
Hawthorne F. C., Oberti R., Harlow G. E., Maresch W. V., Martin R. F., Schumacher J. C., et al. (2012). IMA report: Nomenclature of the amphibole supergroup. Am. Mineralogist 97, 2031–2048. doi: 10.2138/am.2012.4276
Hori K. (2001). Sedimentary facies and Holocene progradation rates of the Changjiang (Yangtze) delta, China. Geomorphology 41, 233–248. doi: 10.1016/S0169-555X(01)00119-2
Hou Z. Q., Wang Y. L., Xia L. Q., Li H. Y., Guo L. J. (1999). Emei large igneous province: Characteristics and origin. Geological review 45, 885–891.
Jin B. F., Dang L. L., Kong Q. X., Wang M. Y., Yue W., Fan S. M. (2022). Comparison of geochemical characteristics of lithophile elements of amphibole: identification of estuarine sediment provenance, Huanghe and Changjiang rivers. Acta Sedimentologica Sinica 40, 149–165.
Jin B. F., Yue W., Wang K. S. (2013). The Crystallochemistry characteristics and genetic analysis of amphibole in the sediments of the Huanghe River. Acta Oceanologica Sinica 35, 131–143.
Jin B. F., Yue W., Wang K. S. (2014). Chemical composition of clastic amphibole in the sedimental of the Huanghe River, Liao River and Yalu River, and its implication for sediment provenance. Acta Oceanologica Sinica 36, 11–21.
Johnson M. C., Rutherford M. (1989). Experimental calibration of the aluminum-in-hornblende geobarometer with applicable to Long Valley Caldera (California) volcanic rocks. Geol 17, 837–841. doi: 10.1130/0091-7613(1989)017<0837:ECOTAI>2.3.CO;2
Krippner A., Meinhold G., Morton A. C., Schönig J., von Eynatten H. (2016). Heavy minerals and garnet geochemistry of stream sediments and bedrocks from the Almklovdalen area, Western Gneiss Region, SW Norway: Implications for provenance analysis. Sediment Geol. 336, 96–105. doi: 10.1016/j.sedgeo.2015.09.009
Liu J. H. (1986). Genetic classification of hornblendes and its application. (Changchun: Journal of Changchun Institute of Geology). 1, 41–48.
Liu C., He Y., Zhang Y. (2008). Trends analysis of the water and sediment loads of the main rivers in China using water-sediment diagram. Adv. Water Sci. 19, 317–324.
McKee B. A., Aller R., Allison M. A., Bianchi T., Kineke G. (2004). Transport and transformation of dissolved and particulate materials on continental margins influenced by major rivers: benthic boundary layer and seabed processes. Cont. Shelf Res. 24, 899–926. doi: 10.1016/j.csr.2004.02.009
Milliman J. D., Meade R. H. (1983). World-wide delivery of river sediment to the oceans. J. Geol. 91, 1–21. doi: 10.1086/628741
Ministry of Water Resources, Changjiang River Water Resources Commission (1999). Atlas of the Changjiang River Basin (Beijing: China Cartographic Publishing House).
Morton A. C., Whitham A. G., Fanning C. M. (2005). Provenance of Late Cretaceous to Paleocene submarine fan sandstones in the Norwegian Sea: Integration of heavy mineral, mineral chemical and zircon age data. Sediment Geol. 182, 3–28. doi: 10.1016/j.sedgeo.2005.08.007
Nie J. S., Song Y. G., King J. W., Egli R. (2010). Consistent grain size distribution of pedogenic maghemite of surface soils and Miocene loessic soils on the Chinese Loess Plateau. J. Quaternary Sci.: Published Quaternary Res. Assoc. 25, 261–266. doi: 10.1002/jqs.1304
Qiao F. L. (2011). Chinese regional oceanography - physical oceanography (Beijing: China Ocean Press).
Qin Y. C., Xue C., Jiang X. (2018). Tidal current-dominated depositional environments in the central-northern Yellow Sea as revealed by heavy-mineral and grain-size dispersals. Mar. Geol. 398, 59–72. doi: 10.1016/j.margeo.2018.01.004
Reed S. J. (1996). Electron Microprobe analysis and scanning microscopy in geology (Cambridge: Cambridge University Press), 165–197.
Safonova I., Maruyama S., Hirata T., Kon Y., Rino S. (2010). LA-ICP-MS U-Pb ages of clastic zircons from Russia largest rivers: Implications for major granitoid events in Eurasia and global episodes of supercontinent formation. J. Geodynamics 50, 134–153. doi: 10.1016/j.jog.2010.02.008
Shang Y., Nian X. M., Zhang W. G., Wang F. (2021). Yellow River’s contribution to the building of Yangtze delta during the last 500 years - evidence from clastic zircon U-Pb geochronology. Geophys. Res. Lett. 48, 1–10. doi: 10.1029/2020GL091896
Soo-Chul P., Hyun-Hee L., Hyuk-Soo H., Gwang-Hoon L. (2000). Evolution of late Quaternary mud deposits and recent sediment budget in the southeastern Yellow Sea. Mar. Geol. 170, 271–288. doi: 10.1016/S0025-3227(00)00099-2
Vezzoli G., Garzanti E., Limonta M., Ando S., Yang S. Y. (2016). Erosion patterns in the Changjiang (Yangtze River) catchment revealed by bulk-sample versus single-mineral provenance budgets. Geomorphology 261, 177–192. doi: 10.1016/j.geomorph.2016.02.031
Wang M. Y. (2019). Study on characteristics of main clastic minerals in modern sediments of the Hongze Lake reach in the Huaihe River (Yantai: Ludong University).
Wang Z. B., Yang S. Y., Mei X., Lu K. (2018). clastic garnet chemistry of the Changjiang (Yangtze River) sediments and their provenance implication. J. Tongji Univ. (Natural Science) 46, 1455–1472.
Wu X., Wang H. J., Bi N. S., Saito Y., Xu J. P., Zhang Y., et al. (2020). Climate and human battle for dominance over the Yellow River’s sediment discharge: From the Mid-Holocene to the Anthropocene. Mar. Geol. 425, 106188. doi: 10.1016/j.margeo.2020.106188
Yang S. Y., Jung H. S., Lim D. I., Li C. X. (2003a). A review on the provenance discrimination of sediments in the Yellow Sea. Earth-Sci. Rev. 63, 93–120. doi: 10.1016/S0012-8252(03)00033-3
Yang S. Y., Li C. (1999). Element composition and tracer function of modern surface sediments from the Yangtze and Yellow rivers. Prog. Natural Sci. 9, 930–937.
Yang S. Y., Li C. Y. (2000). Elemental composition in the sediments of the Yangtze and the Yellow Rivers and their tracing implication. Prog. Natural Sci.: Materials Int. 10, 616–618.
Yang S. Y., Li C. X., Hoi-Soo J., Dong-Il L. M., Man-Sik C. (2003b). Geochemistry of trace elements in Chinese and Korean river sediments. Mar. geol. Quaternary geol. 23, 6.
Yang Q. H., Lin Z. H., Zhang F. Y., Zhou H. Y. (2004). Mineral characteristics of hornblende and magnetite in surface sediments in the east of the South China Sea and their genesis. Mar. geol. Quaternary geol. 24, 29–35.
Yang S. Y., Youn J. S. (2007). Geochemical compositions and provenance discrimination of the central south Yellow Sea sediments. Mar. Geol. 243, 229–241. doi: 10.1016/j.margeo.2007.05.001
Yue W., Jin B. F., Zhao B. C. (2018). Transparent heavy minerals and magnetite geochemical composition of the Yangtze River sediments: Implication for provenance evolution of the Yangtze Delta. Sediment Geol 364, 42–52. doi: 10.1016/j.sedgeo.2017.12.006
Zhai M. G. (2012). Evolution of the north China craton and early plate tectonics. Acta Geographica Sinica 86, 1335–1349.
Zhao S. Y., Bian Q. J., Yan W. Q. (1993). Crystallography and mineralogy (Beijing: Higher Education Press).
Keywords: sedimentary provenance, clastic amphibole, source rock, genesis of crystallization, South Yellow Sea
Citation: Wang M, Jin B, Liu J, Gao M, Gao J and Jia J (2024) Genetic types and provenance indication of clastic amphibole in the South Yellow Sea. Front. Mar. Sci. 11:1382352. doi: 10.3389/fmars.2024.1382352
Received: 05 February 2024; Accepted: 06 May 2024;
Published: 21 May 2024.
Edited by:
Tianyuan Zheng, Ocean University of China, ChinaReviewed by:
Zhang Bo, Shandong University of Science and Technology, ChinaYunhai Fang, Hefei University of Technology, China
Zhongbo Wang, Shantou University, China
Copyright © 2024 Wang, Jin, Liu, Gao, Gao and Jia. This is an open-access article distributed under the terms of the Creative Commons Attribution License (CC BY). The use, distribution or reproduction in other forums is permitted, provided the original author(s) and the copyright owner(s) are credited and that the original publication in this journal is cited, in accordance with accepted academic practice. No use, distribution or reproduction is permitted which does not comply with these terms.
*Correspondence: Maosheng Gao, gaomsh66@sohu.com; Jianjun Jia, jjjia@sklec.ecnu.edu.cn