Invasive Spartina alterniflora alters sediment organic carbon mineralization dynamics in a coastal wetland of Southeastern China
- 1PowerChina Huadong Engineering Corporation Ltd., Hangzhou, China
- 2Institute of Soil and Water Resources and Environmental Science, College of Environmental and Resource Sciences, Zhejiang University, Hangzhou, China
- 3Key Laboratory for Humid Subtropical Eco-Geographical Processes of the Ministry of Education, School of Geographical Sciences, Fujian Normal University, Fuzhou, China
- 4Frontiers Science Center for Deep Ocean Multispheres and Earth System, and Key Laboratory of Marine Chemistry Theory and Technology, Ministry of Education, Ocean University of China, Qingdao, China
- 5School of Geography Science and Geomatics Engineering, Suzhou University of Science and Technology, Suzhou, China
Invasive Spartina alterniflora has significant impacts on sediment carbon pool and turnover in the tidal wetlands of estuaries and coasts. Yet, how this exotic S. alterniflora affects sediment organic carbon mineralization dynamics remains poorly understood. In this study, sediment geochemical properties, organic carbon fractions, and mineralization dynamics were examined in a native Cyperus malaccensis habitat and three invasive S. alterniflora habitats (6-, 10-, and 14-year-old) in summer and winter. We found that invasive S. alterniflora generally increased sediment total organic carbon and their labile fraction contents. The organic carbon mineralization rates and cumulative carbon mineralization amounts were significantly influenced by invasive S. alterniflora, and their values increased with this exotic plant invasion chronosequences. The mineralization rates and cumulative mineralization amounts were also characterized by higher values in surface sediment (0 – 10 cm depth) compared to subsurface sediment (10 – 20 cm depth) and by seasonal variations with higher values in summer than in winter. The sediment organic carbon labile fractions, rather than total organic carbon, were the most important factor affecting carbon mineralization dynamics. The cumulative carbon mineralization amounts exhibited an excellent fit to the first-order kinetic equation (R2 ≥ 0.93). The changes in modeled kinetic parameters (potential carbon mineralization amounts (C0) and carbon mineralization rate constant (k)) among these four habitats were similar to carbon mineralization rates, implying invasive S. alterniflora promoted the availabilities of organic compounds for microbial respiration metabolism. Overall, our findings highlighted the importance of S. alterniflora invasion in accelerating organic carbon decomposition and carbon dioxide release potential, although it also increases carbon accumulation.
1 Introduction
Tidal wetlands of estuaries and coasts as important carbon pools, playing a crucial role in the global carbon biogeochemical balance and associated climate feedback (Castillo et al., 2017; Xia et al., 2021). Sediment total organic carbon (TOC) dynamics in intertidal wetlands can provide the basis for a deep understanding of the source and sink mechanism of carbon in global ecosystems (Yang et al., 2013; Hinson et al., 2019). Sediment TOC consists of a diverse array of compounds, varying greatly in chemical complexity, and exhibits a mean turnover time that spans from mere days to several decades or even centuries (Williams and Rosenheim, 2015). The TOC pools can be divided into recalcitrant and labile fractions based on their sensitivity to environmental factors and turnover rate (Roldán et al., 2005; Hinson et al., 2019). Generally, recalcitrant carbon fractions account for a substantial proportion of TOC and are important for carbon sequestration and quality in ecosystems because they stay in the sediment for a long time (Lu and Xu, 2014; Villoslada et al., 2022). In contrast, labile carbon fractions constitute a relatively small fraction of TOC, but they play a vital role in driving the early changes in organic pools due to the high biochemical activities (Belay-Tedla et al., 2009). Thus, labile carbon fractions may be more important characterization parameters of the organic carbon budget compared to recalcitrant carbon. Herein, easily oxidizable carbon (EOC), dissolved organic carbon (DOC), and microbial biomass carbon (MBC) are three representative labile carbon fractions and have been commonly applied in the study of carbon dynamics (Lu and Xu, 2014; Grasset et al., 2017; Mou et al., 2018). Additionally, the mineralization of sediment organic carbon is a pivotal process of carbon cycles, which directly affects carbon release and greenhouse gas formation (Chapman et al., 2019; Dong et al., 2022). Therefore, sediment organic carbon fractions and mineralization dynamics as well as their influencing mechanisms in tidal wetlands of estuaries and coasts have received much attention over the last decades.
Exotic plant invasions, one of the important components of global environmental change, have unavoidable effects on native ecosystem functioning (Bernik et al., 2016; Jin et al., 2017). In 1979, Spartina alterniflora, a perennial C4 grass, was deliberately introduced to intertidal wetlands in China to enhance siltation and protect coastlines. Over the past three decades, this invasive plant has spread across an area of about 344.51 km2, emerging as the predominant plant species along the Chinese coastal regions (Yang et al., 2016a; Banerjee et al., 2022). It currently presents a danger to the long-term sustainability of ecosystems in such sensitive wetlands. Previous works have noted that invasive S. alterniflora has obvious effects on sediment geochemical properties, microbial activities, carbon pool, and greenhouse gas emissions (Zhang et al., 2010; Throop et al., 2013; Yang et al., 2016a; Jin et al., 2017; Gao et al., 2019; Chen et al., 2023; He et al., 2023). Their results showed that this exotic S. alterniflora generally promotes sediment TOC accumulation and greenhouse gas emissions. However, the understanding of changes in sediment organic carbon fractions and mineralization characteristics along the chronosequences of S. alterniflora invasion is still very limited. It has been noted that organic carbon fractions in the sediment of estuarine and coastal wetlands are tightly associated with plant net primary productivity, and the changes in their fractions would further affect organic carbon decomposition and mineralization (Mou et al., 2018; Chapman et al., 2019; Steinmuller and Chambers, 2019; Dong et al., 2022). In this case, S. alterniflora invasion can theoretically alter organic carbon fractions and mineralization dynamics due to the fact that it enhances plant biomass, microbial diversities, and carbon and nitrogen substrate availabilities. Hence, gaining a deeper comprehension of how invasive S. alterniflora affects organic carbon fractions and mineralization dynamics in the sediment is crucial for devising effective strategies to manage plant invasions in estuarine and coastal wetlands.
Spartina alterniflora has rapidly expanded and replaced some native plants since it was included in the intertidal wetlands of the Minjiang River estuary (Zhang et al., 2011; Gao et al., 2017). In addition, previous work has confirmed that this region with a large sediment carbon pool (Tong et al., 2011). Here, we conducted a study to explore the impacts of this invasive S. alterniflora on sediment organic carbon fractions and mineralization dynamics in tidal wetlands of the Minjiang River estuary in China. The primary goals of the present study were to (1) investigate the effects of invasive S. alterniflora on sediment geochemical variables and organic carbon fractions (TOC, MBC, DOC, and EOC); (2) elucidate the changes in mineralization characteristics of organic carbon in different chronosequences of S. alterniflora invasion; (3) elucidate the key factors altering organic carbon fractions and mineralization as well as their eco-environmental significance in coastal wetland after S. alterniflora invasion. This work provides valuable insight into the influences of S. alterniflora invasion on carbon dynamics and highlights that the expansion of this exotic plant should be appropriately controlled.
2 Materials and methods
2.1 Site description and sample collection
The present study was carried out in the Nature Reserve of Shanyutan, which is the larger intertidal wetlands in the Minjiang estuary, Fujian Province, Southeastern China (119°34′12″ – 119°41′40″E, 26°00′36″ – 26°03′42″N, Figure 1). The climate here belongs to the typically subtropical monsoon climate, and the annual mean rainfall and temperature are approximately 1300 mm and 19°C, respectively (Mou et al., 2018). The area has enriched vegetation types, and the predominant native species contain Scirpus triqueter, Cyperus malaccensis, and Phragmites australis, which mainly grow in low, middle, and high intertidal zones, respectively (Gao et al., 2017). In 2002, the S. alterniflora was introduced to the study area, expanded rapidly, and occupied native C. malaccensis habitat where in the middle intertidal zone for the next 20 years (Mou et al., 2014).
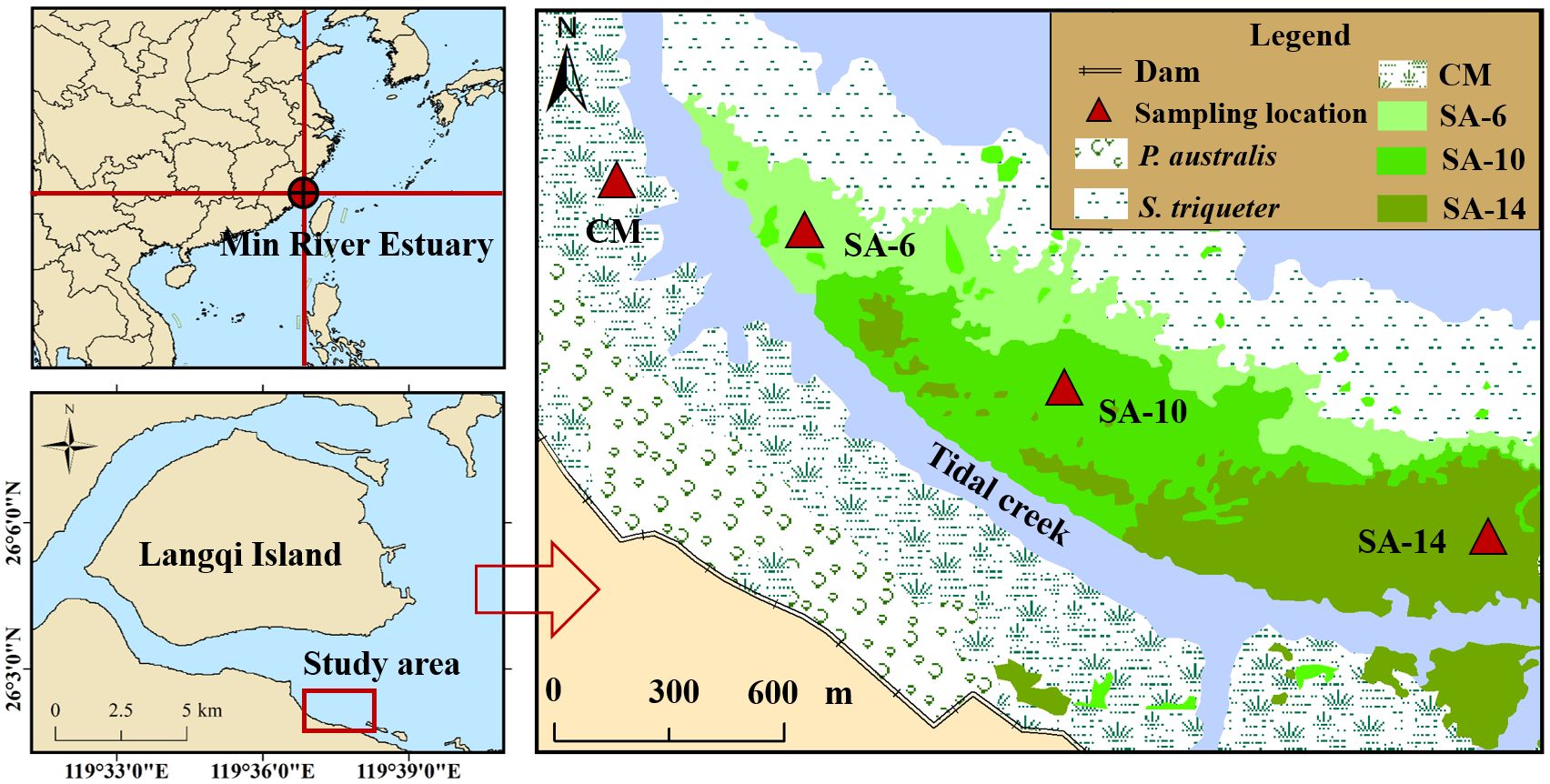
Figure 1 Geographical location of the survey region and sampling points. The CM denotes C. malaccensis habitat, and the SA-6, SA-10, and SA-14 denote S. alterniflora habitats for 2 – 6 years, 6 – 10 years, and 10 – 14 years, respectively.
The superimposition analysis of the Landsat 8 image (2014), SPOT5 image (2010), and aerial image (2006) were used to identify the sampling location with different chronosequences of invasive S. alterniflora (Jin et al., 2017). The locations of sample collection included a native habitat (CM, C. malaccensis) and three invasive S. alterniflora habitats that replaced the former in 2010 – 2014 (SA-6), 2006 – 2010 (SA-10), and 2002 – 2006 (SA-14), respectively (Figure 1). The above-mentioned four habitats were chosen as sample points, and these represent the different invasion degrees of S. alterniflora. Over the past several years, the study area was noticeably divided by a tidal creek, and the CM habitats on the east/northeast/north side of the creek have been occupied by this invasive plant (Figure 1). Thus, the sampling location of CM habitats in our study was situated in another side of the creek. These sampling locations (CM, SA-6, SA-10, and SA-14) were situated in the middle intertidal zone, which had similar sediment and hydrodynamic characteristics before S. alternifolia invasion. Since a large portion of the root biomass of S. alterniflora is distributed within a depth of 0 – 20 cm, where the sediment has higher TOC contents and is more susceptible to environmental changes (Tong et al., 2011; Jin et al., 2017), this depth was chosen to explore the response of organic carbon mineralization dynamics to S. alterniflora invasion. In each sampling habitat, three sediment samples at two depths (0 – 10 cm and 10 –20 cm) were collected randomly based on a stainless steel sediment cylinder (10 cm diameter) on July 23, 2016, and December 22, 2016 from approximately 7:00 to 9:00, during the diurnal ebb-tide. Sediment temperatures were recorded immediately based on a portable sediment thermometer. After removing plant roots, residues, and recognizable stones, the samples were put into sterile ziplock bags. Then, sediment samples were stored in a thermostat at approximately 4 °C and returned to the analyzer room within 4 h. After that, sediment samples were homogenized and separated into two fractions: one fraction was stored at 4 °C to measure sediment MBC and DOC contents, and the second fraction was freeze-dried for determination of other carbon fractions (TOC and EOC) and geochemical variables as well as organic carbon mineralization dynamics.
2.2 Sediment geochemical properties analysis
The portable thermometer was used to measure sediment temperatures. The pH and electrical conductivity (EC) of sediment were determined by the handheld pH (IQ Scientific Instruments, USA) and EC tester (Spectrum Technologies Inc., USA), respectively (Gao et al., 2017). The oven-drying and cutting-ring methods were used to analyze sediment moisture and density, respectively. Total nitrogen (TN) contents were measured based on a CHNS analyzer (Vario EL, Elementar, Germany) (Jin et al., 2017). The potassium chloride solution (KCl) of 2 M was applied to extract sediment ammonium (NH4+), nitrate (NO3–) and nitrite (NO2–), and their contents were measured by an automatic injection analyzer (Skalar SAN++, the Netherlands) (Mou et al., 2018).
2.3 Measurements of sediment organic carbon fractions
Sediment TOC contents were analyzed based on a CHNS analyzer (Vario EL, Elementar, Germany) after 1 M hydrochloric acid (HCl) acidification (Jin et al., 2017). The methods of chloroform fumigation extraction were used to determine MBC content in sediment (Song et al., 2012). In brief, two fresh sediment samples (10 g) were placed into 100 mL beakers, and these samples were incubated for 24 h in a dark fumigation dish, with one of them being fumigated with chloroform. The sediments with and without fumigation were extracted with 40 mL potassium sulfate (K2SO4) (0.5 M), and the mixed solutions were filtered through a 0.45-µm filter membrane. Subsequently, TOC concentration in the filtrate was measured by a TOC analyzer (TOC-VCPH, Shimadzu). Sediment MBC contents were calculated based on the TOC concentration in the filtrate with and without fumigation (Song et al., 2012). Sediment DOC contents were measured by a TOC analyzer (TOC-VCPH, Shimadzu) after extraction with deionized water (Liu et al., 2022). The EOC contents in sediment were quantified based on the potassium permanganate (KMnO4) oxidation method (Song et al., 2012). Freeze-dried sediment samples (1 g) were extracted with 333 M KMnO4 solution (25 mL), and the supernatant was collected after 3 h standing. Then, the absorbance in the supernatant at 565 nm was determined based on the UV-Vis spectrophotometers (UV-2450, Shimadzu). Meanwhile, the blank sample without sediment was also included in the analysis. Sediment EOC contents were estimated according to the KMnO4 concentrations in sediment and blank samples.
2.4 Sediment organic carbon mineralization determination
A series of incubation experiments were designed to study the impacts of invasive S. alterniflora on organic carbon mineralization dynamics. Freeze-dried sediment samples of 20 g were put into 120 mL glass vials equipped with two triple valves, and they were pre-wetted to approximately 60 ± 3% water-holding capacity. All glass vials were preincubated in a thermostatic incubator for 7 days to restore microbial activities before closed incubation. Then, these glass vials were incubated at a constant temperature (summer: 32°C, winter: 17°C) in the dark incubator, and the organic carbon mineralization rates on days 1, 3, 5, 7, 10, 14, 21, 28, 38 and 56 were determined. On each sampling date, the incubation glass vials were flushed with high-purity nitrogen gas through three-way stopcocks for 5 min, and the 3 mL gas samples (initial values) were collected immediately after closed. After 24 h incubation, gas samples (3 mL) were obtained again via a sterile injector, and these were marked as final values. The gas chromatograph (GC-2014, Shimadzu) was used to measure the carbon dioxide (CO2) concentrations in collected gas, and the organic carbon mineralization rates at each incubation day were calculated based on initial and final CO2 concentrations in the headspace of glass vilas (Equation (1)) (Mou et al., 2018):
where F is sediment organic carbon mineralization rates (μg g-1 d-1); represents the changes in CO2 concentrations (μL L-1 h-1). The headspace volume of the incubation glass vila (L) and the sediment weight (g) were expressed as VH and MS, respectively. The MV and MW refer to the molecular volume(L mol-1) and weight (g mol-1) in an ideal gas, respectively; T is the temperature across the incubation period (°C). In addition, a first-order kinetic model was applied to determine the mineralization kinetics and characteristics of organic carbon, which was calculated by Equation (2) (Cooper et al., 2011):
where Ct is the cumulative carbon mineralization amounts at time t (mg g-1). The C0 denotes potential carbon mineralization amounts (mg g-1). The t represents incubation days (d), and k is the carbon mineralization rate constant.
2.5 Statistical analysis
All analysis was conducted based on the SPSS 19.0 and Origin 2021 software package. These data were examined for normal distribution and the need for any necessary transformations before statistical analysis. The one-way analysis of variance followed by the LSD was conducted to detect the statistical differences in sediment organic carbon fractions across four habitats in the same season. A first-order kinetic model in Origin 2021 was used to estimate the potential carbon mineralization amounts and the mineralization rate constant. Pearson’s related analysis was performed to reveal the associations between sediment geochemical properties, organic carbon fractions, and their mineralization dynamics.
3 Results
3.1 Sediment geochemical variables
Sediment temperature varied from 16.80 to 33.83°C, and summer temperature (32.95 ± 1.09°C) was significantly higher than that in winter (17.28 ± 0.47°C) (p< 0.05, Table 1). In summer, the highest and lowest temperatures of sediment occurred in SA-10 and SA-6 habitats, respectively, but there were no statistical differences across different habitats in winter (p > 0.05, Table 1). Sediment pH in summer ranged from 5.18 − 5.91, which was highest in SA-6 habitats, followed by the CM habitats, and lowest in the SA-14 and SA-10 habitats. While in winter, sediment pH was significantly higher in S. alterniflora habitats (SA-14, SA-6, and SA-10) than in C. malaccensis habitat (CM) (p< 0.05). No obvious differences in pH were observed between 0 − 10 cm and 10 − 20 cm depth (Table 1). Sediment EC in summer and winter ranged from 0.70 to 1.73 and 2.17 to 2.91, respectively, and the highest values were observed in SA-14 habitats (Table 1). In summer, sediment water content (37.09 − 50.68%) and bulk density (1.31 − 1.67 g cm-3) were generally lower and higher respectively, in C. malaccensis habitat (CM) as compared to S. alterniflora habitats (SA-14, SA-10 and SA-6), but the values did not present similar change patterns in winter (Table 1). Sediment TN, NH4+, NO3− and NO2− contents were in the range of 1.60 − 2.30 g kg-1, 21.61 − 48.89 mg kg-1, 0.78 − 1.72 mg kg-1 and 12.06 − 34.27 μg kg-1, and their values generally increased with the invasion degree of S. alterniflora (Table 1). Additionally, sediment TN, NH4+, NO3− and NO2− contents at the surface (0 − 10 cm) were higher than those at the subsurface (10 − 20 cm), except for winter NH4+ in SA-10 and SA-14 habitats (Table 1).
3.2 Sediment organic carbon fractions
Sediment TOC contents varied from 11.33 to 30.51 g kg-1, and S. alterniflora invasion generally increased the values, especially in SA-10 and SA-14 habitats (Figure 2). In CM habitats, TOC contents at 0 − 10 cm sediment layer were larger than those at 10 − 20 cm sediment layer, but no obvious changes were observed between the two sediment layers in S. alterniflora habitats (SA-14, SA-10, and SA-6) (p > 0.05). Seasonally, sediment TOC contents were higher in summer than in winter in these four habitats (Figure 2). The changes in sediment MBC contents varied among four habitats at different depths. Wherein, sediment MBC contents at the surface (0 − 10 cm) were significantly higher in SA-14 and SA-10 habitats than in CM habitats both in summer and winter (Figure 2) (p< 0.05). While at 10 − 20 cm depth, summer sediment MBC did not differ among four habitats (p > 0.05), but highest values were observed in SA-14 habitats in winter. Sediment DOC contents ranged from 74.89 to 113.47 mg kg-1, and summer sediment DOC contents in S. alterniflora habitats (SA-14, SA-10, and SA-6) were generally higher than in C. malaccensis habitat (CM), but no statistical differences across different habitats in winter (Figure 2) (p > 0.05). The DOC contents at 0 − 10 cm sediment layer were higher than those at 10 − 20 cm sediment layer both in summer and winter. In addition, no obvious seasonal differences in sediment DOC contents were found among four different habitats (p > 0.05). The EOC contents varied from 3.87 to 8.53 g kg-1, and the values in SA-10 and SA-14 habitats were higher than those in SA-6 and CM habitats, although some differences did not reach a level of statistical significance (Figure 2). Sediment EOC contents at the surface (0 − 10 cm) were also higher than those at the subsurface (10 − 20 cm). The EOC contents with little variation between summer and winter in CM and SA-6 habitats, while the values in summer were larger than those in winter in SA-14 and SA-10 habitats (Figure 2).
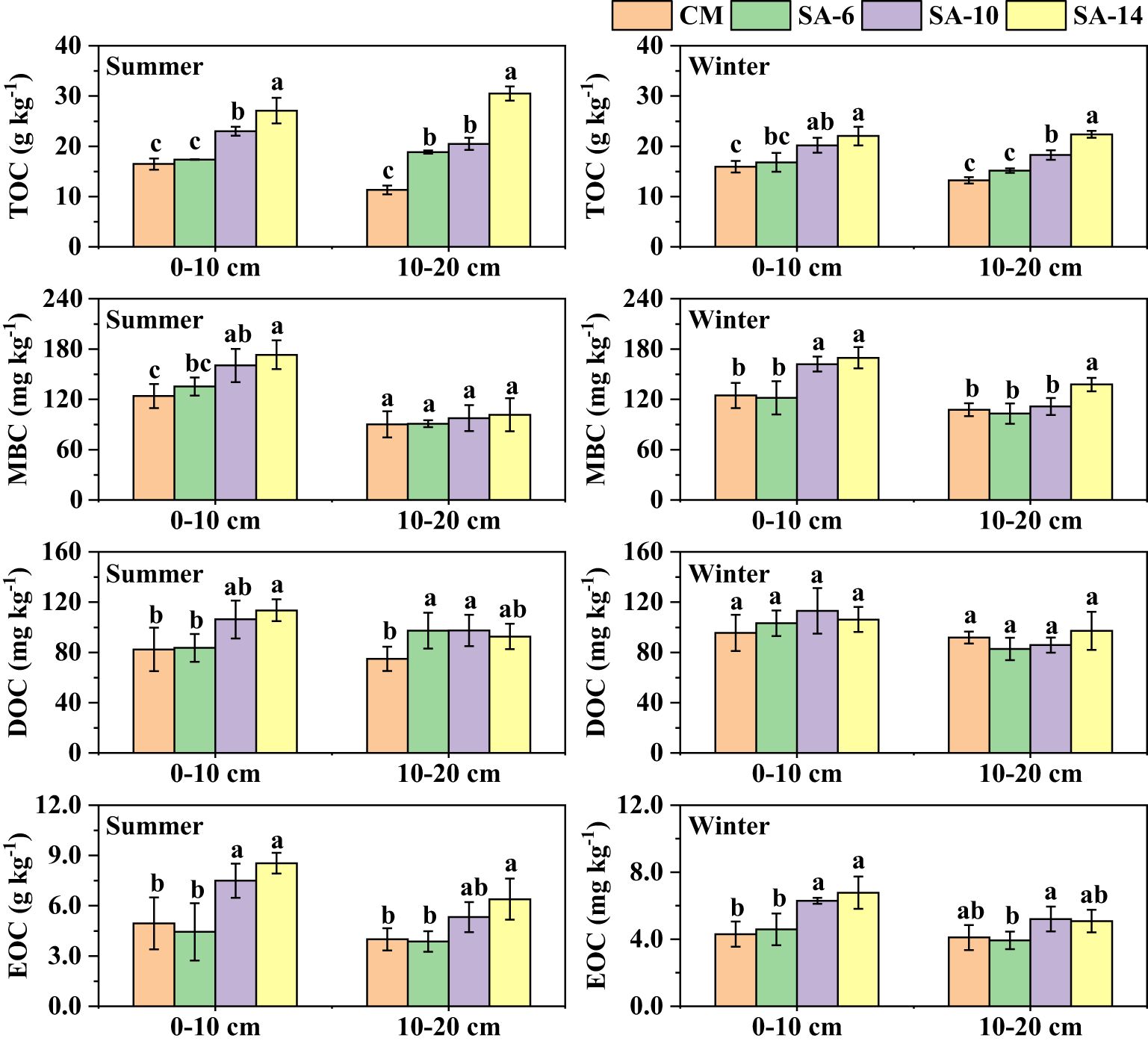
Figure 2 The effects of invasive S. alternifolia on sediment organic carbon fractions. The CM denotes C. malaccensis habitat, and the SA-6, SA-10, and SA-14 denote S. alterniflora habitats for 2 – 6 years, 6 – 10 years, and 10 – 14 years, respectively. The presence of different lowercase letters indicates statistical differences (p< 0.05) across four habitats in the same depth.
3.3 Sediment organic carbon mineralization characteristics
The mineralization rates of sediment organic carbon in SA-14, SA-10, SA-6, and were in the range of 64.22 − 770.99 μg g-1 d-1, 65.21 − 624.82 μg g-1 d-1, 64.73 − 420.16 μg g-1 d-1, and 60.97 − 362.45 μg g-1 d-1, respectively, with large variations across different habitats and incubation time (Figure 3). During the 56-day incubation period, organic carbon mineralization rates in early stage (days 1 − 7) were relatively higher, and the largest values occurred at day 3. In the middle and late stages (days 7 − 56), the organic carbon mineralization rates decreased with incubation time and tended to be stable (Figure 3). Sediment organic carbon mineralization rates in S. alterniflora habitats (SA-14, SA-10, and SA-6) were larger than those in C. malaccensis habitat (CM), and exhibited an increasing tendency with the invasion degree of S. alterniflora (Figure 3). In addition, organic carbon mineralization rates at the surface (0 − 10 cm) were generally higher than those at subsurface (10 − 20 cm), especially in the early stage of incubation. Seasonally, organic carbon mineralization rates were larger in summer compared to winter in both two sediment layers (Figure 3). During the 56-day incubation, the mean rates of organic carbon mineralization among four habitats under different seasons and depths generally displayed the above-mentioned change characteristics, although some differences did not reach a level of statistical significance (Figure 4).
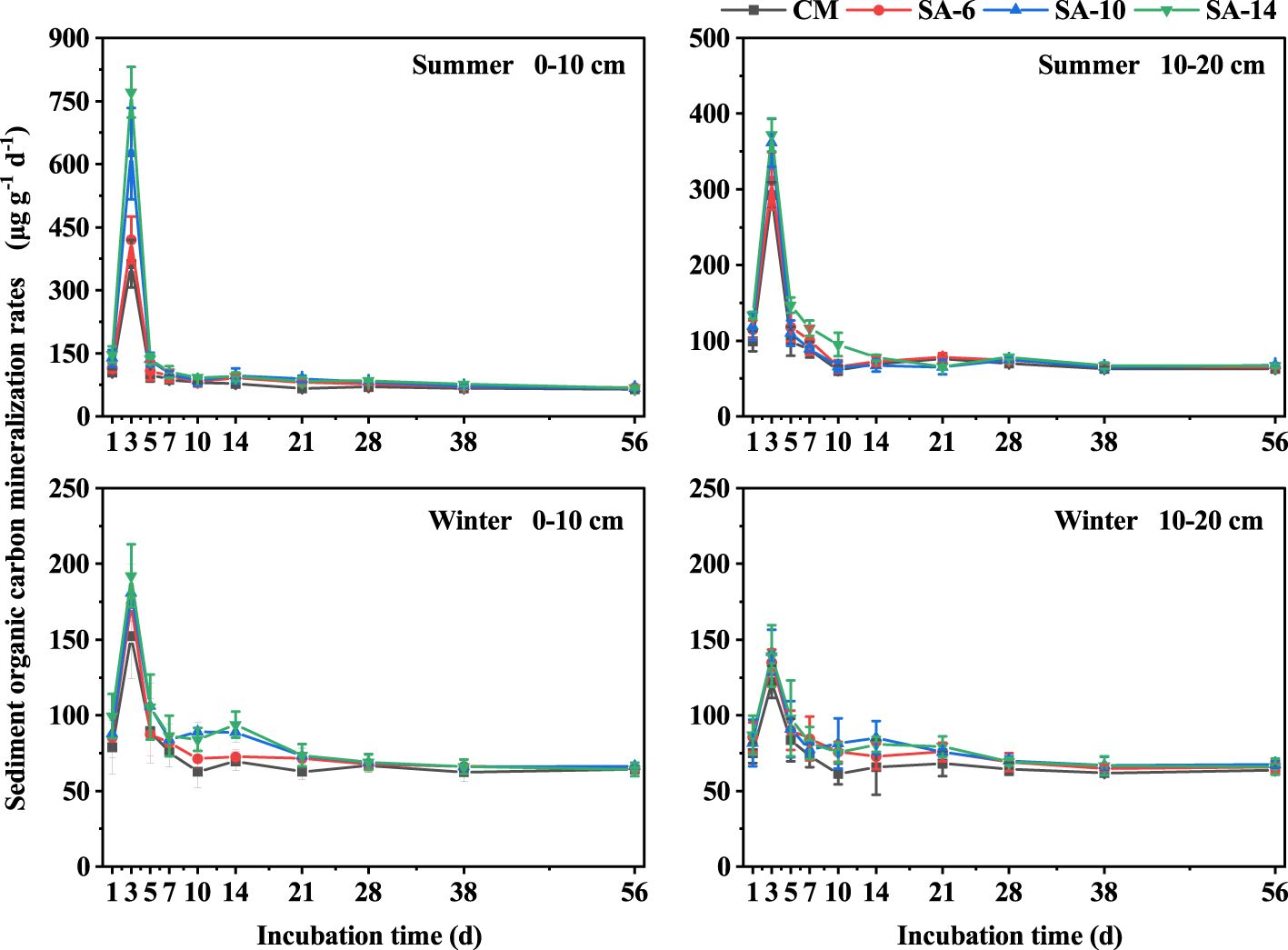
Figure 3 The effects of invasive S. alternifolia on sediment organic carbon mineralization rates. The CM denotes C. malaccensis habitat, and the SA-6, SA-10, and SA-14 denote S. alterniflora habitats for 2 – 6 years, 6 – 10 years, and 10 – 14 years, respectively.
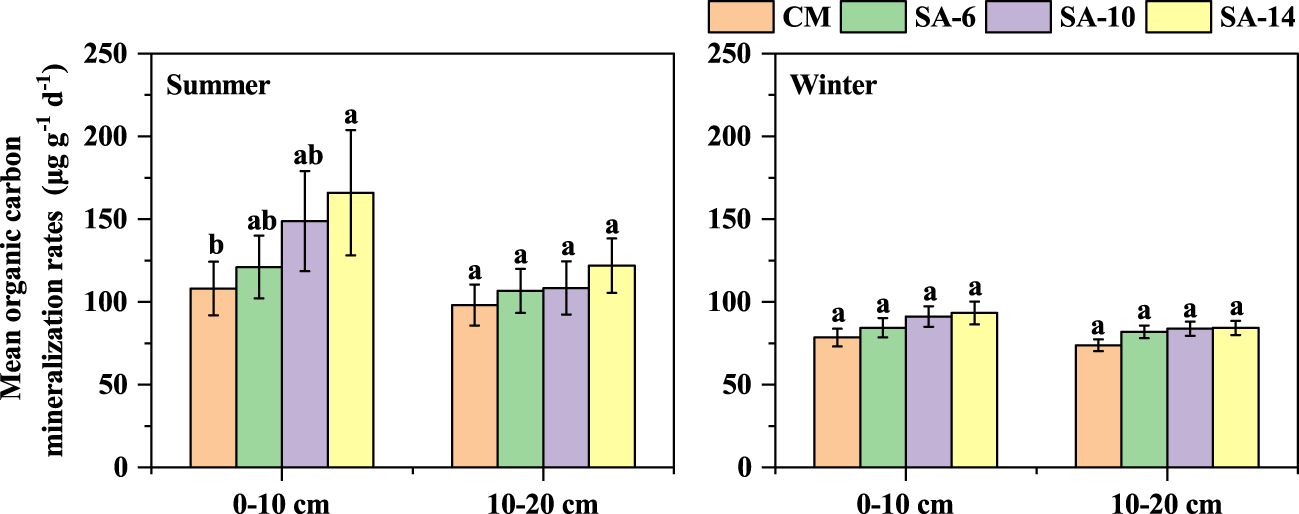
Figure 4 The effects of invasive S. alternifolia on sediment mean organic carbon mineralization rates. The CM denotes C. malaccensis habitat, and the SA-6, SA-10, and SA-14 denote S. alterniflora habitats for 2 – 6 years, 6 – 10 years, and 10 – 14 years, respectively. The presence of different lowercase letters indicates statistical differences (p< 0.05) across four habitats in the same depth.
The cumulative carbon mineralization amounts (Ct) increased rapidly in the early stage of incubation and then gradually tended to be stable (Figure 5). After 56-day incubation, the Ct ranged from 738.47 to 1658.41 μg g-1, which was highest in SA-14 habitats, followed by SA-10 and SA-6 habitats, and lowest in the CM habitats. Also, the Ct was larger in summer compared to winter, and higher at the surface (0 − 10 cm) compared to the subsurface (10 − 20 cm) (Figure 5). In addition, organic carbon mineralization kinetics were described well by the first-order equation, and the correlation coefficients varied from 0.93 to 0.98 (Table 2). Potential carbon mineralization amounts (C0) varied from 695.62 to 1523.28 μg g-1, which was in the order of SA-10 > SA-10 >SA-6 > CM. The variations in estimated C0 among four habitats under different seasons and depths generally were similar to measured cumulative organic carbon mineralization (Table 2). The carbon mineralization rate constant (k) ranged from 0.09 to 0.22. In summer, the k values in S. alterniflora habitats (SA-14, SA-10, and SA-6) were higher than those in C. malaccensis habitat (CM), but with little variation in winter (Table 2).
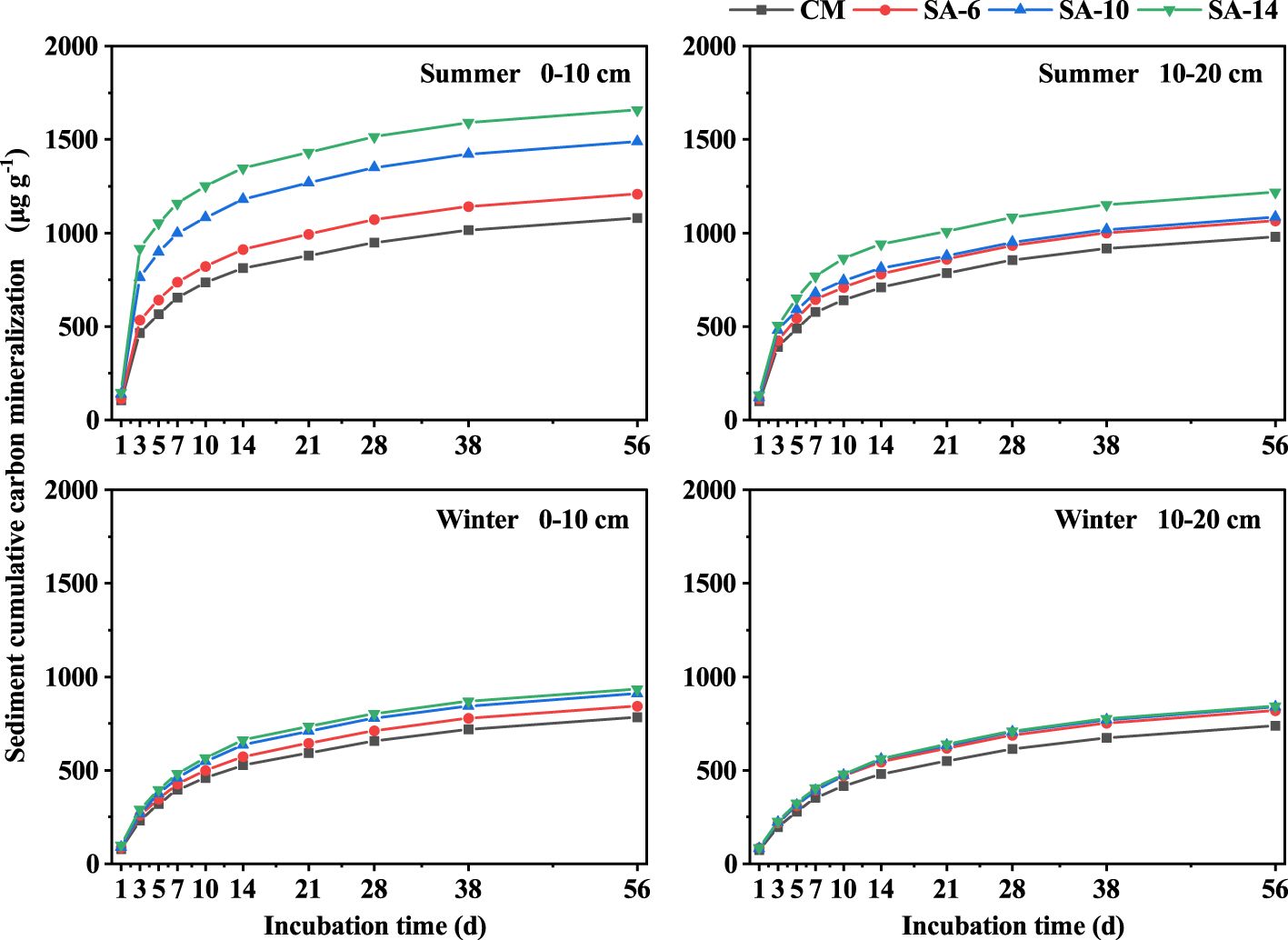
Figure 5 The effects of invasive S. alternifolia on sediment cumulative carbon mineralization. The CM denotes C. malaccensis habitat, and the SA-6, SA-10, and SA-14 denote S. alterniflora habitats for 2 – 6 years, 6 – 10 years, and 10 – 14 years, respectively.
3.4 Sediment properties affecting organic carbon fractions and mineralization
Sediment TOC contents were significantly related to DOC, EOC, bulk density, TN, NH4+, NO3−, and NO2− (p< 0.05) (Table 3). The MBC contents in sediment were dramatically associated with DOC, EOC, TN, NH4+, and NO3− (p< 0.05). Sediment DOC contents were positively correlated with MBC, EOC, TN, and NO3−, but negatively related to bulk density (p< 0.05) (Table 3). Sediment EOC contents were significantly related to TN and inorganic nitrogen (NH4+, NO3−, and NO2−) (p< 0.05), but not associated with other measured geochemical properties (p >0.05). The Ct and C0 were significantly correlated with TOC, EOC, temperature, EC, NO3−, and NO2− (p< 0.05) (Table 3). Also, the k values were positively related to TOC, EOC, temperature, NO3−, and NO2−, but negatively associated with pH and EC (p< 0.05). In addition, there are pairwise correlations among the Ct, C0, and k values (Table 3).
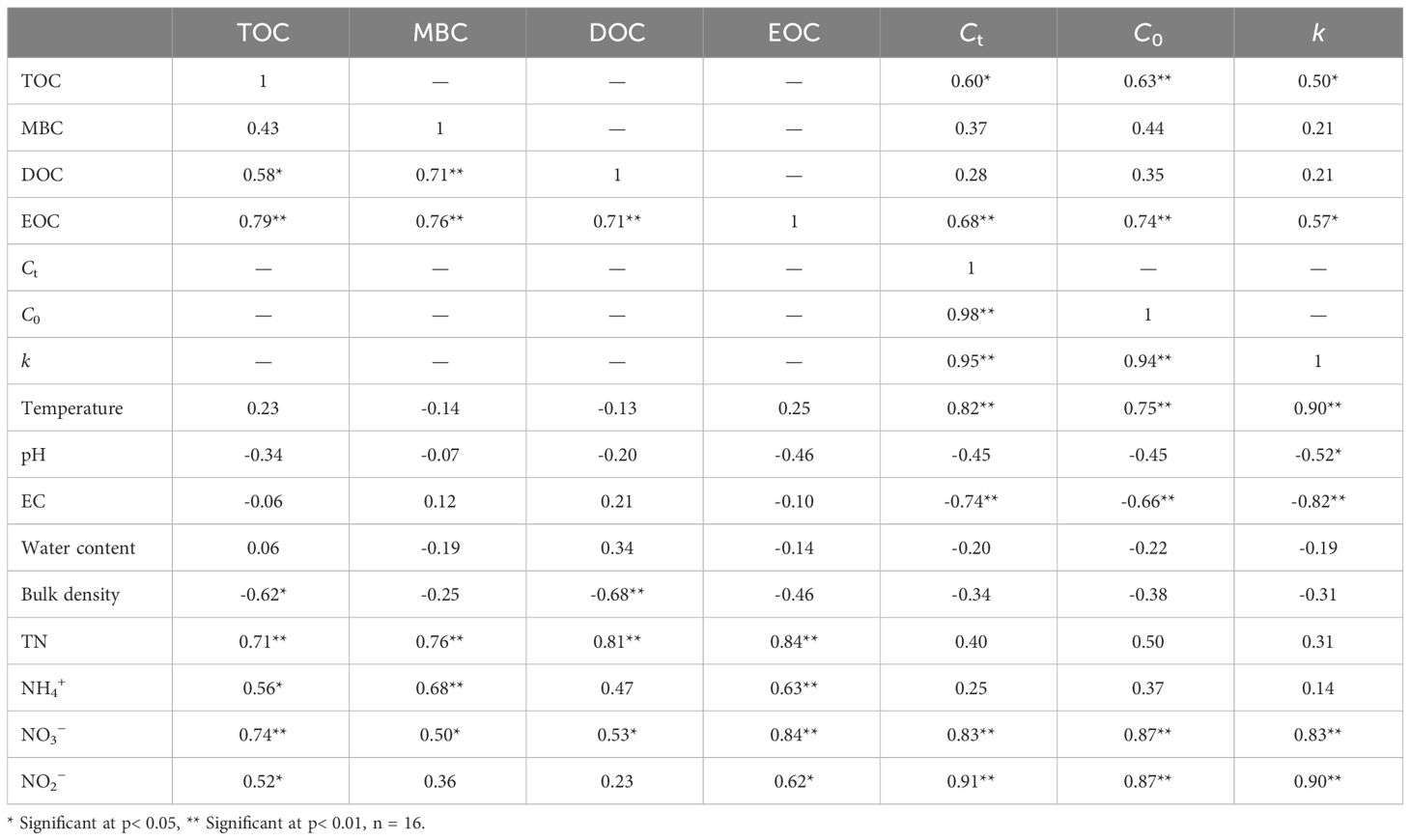
Table 3 The relationship between sediment geochemical variables, organic carbon fractions, and mineralization kinetic parameters.
4 Discussion
4.1 Effects of invasive S. alterniflora on organic carbon fractions
Exotic plant invasion has obvious effects on sediment geochemical properties and biogenic element contents (Gao et al., 2019; Xia et al., 2021). Our newly revealed results indicate that invasive S. alterniflora changes sediment organic carbon fractions, which improves the understanding of carbon dynamics in intertidal wetlands of estuaries and coasts. In this study, sediment TOC contents were larger in S. alterniflora habitats than in C. malaccensis habitat, and the values increased with the invasion degree of S. alterniflora (Figure 2). Generally, sediment TOC contents in intertidal wetlands of estuaries and coasts are tightly related to vegetation growth, and higher net primary production can increase their contents via roots and litter inputs (Tong et al., 2011; Li et al., 2019; Liu et al., 2021; Zheng et al., 2023). Indeed, invasive S. alterniflora had greater aboveground and root biomass compared to C. malaccensis (Gao et al., 2017), which explained well the aforementioned changes in TOC. Previous studies also found that sediment TOC contents in other intertidal wetlands in China (e.g., Jiaozhou Bay wetlands, Yellow River Estuary wetlands, Yancheng wetlands of Jiangsu, Yangtze Estuary wetlands, and Hangzhou Bay wetlands) were higher in S. alterniflora habitat than in native salt marsh habitats (Zhang et al., 2010; Yang et al., 2013; Zhang et al., 2018; Gao et al., 2019; Liu et al., 2021), demonstrating that S. alterniflora invasion increased organic carbon accumulation in such ecosystems. However, sediment TOC contents in the S. alterniflora habitat in Jiulong River Estuary wetlands and/or Pearl River Estuary wetlands) were lower than those in the mangrove habitat (Yu et al., 2015; Xia et al., 2021), which indicated that S. alterniflora invasion into the mangrove caused organic carbon loss. This phenomenon was mainly attributed to the changes in biomass, and mangroves have larger biomass, leading to a greater accumulation of organic carbon (Yu et al., 2015). In the vertical profile, sediment TOC contents were larger at the surface (0 − 10 cm) than at subsurface (10 − 20 cm) in C. malaccensis habitat, but there were comparable between the two depths in S. alterniflora habitats (Figure 2). Theoretically, sediment TOC contents in the surface layer would be higher compared to the subsurface layer due to the input of aboveground plant litter (Xia et al., 2021). This discrepant result might be attributed to the differences in root systems among these habitats (Emery and Fulweiler, 2014; Jin et al., 2017). Field survey showed that S. alternifolia has more developed root systems compared to C malaccensis, resulting in no significant decrease in TOC contents at the subsurface sediment layer (10 − 20 cm depth) in the former. In general, the root length of S. alternifolia can be more than 1 m, while C malaccensis is about 0.5 m (Liao et al., 2007; Gao et al., 2017). Hence, S. alternifolia invasion altered the vertical distribution characteristics of sediment TOC. Seasonally, sediment TOC contents in these four habitats were larger in summer compared to winter (Figure 2), which might be due to the fact that summer serves as the plant growing season, possessing higher primary productivity (Tong et al., 2011; Gao et al., 2019). Sediment TOC contents continued to increase with invasion chronosequences of S. alterniflora (Figure 2), indicating that sediment carbon accumulation had not yet reached its maximum value at SA-14 habitats, and this finding aligned with the results observed in the Yancheng wetlands of Jiangsu Province and Yellow River Estuary wetlands (Yang et al., 2016b; Zhang et al., 2018). Hence, the changes in sediment TOC contents after S. alterniflora invasion should be further followed up to recognize its carbon storage potential (He et al., 2024).
Sediment labile carbon fractions (e.g., MBC, DOC, and EOC) are sensitive to changes in environmental conditions and have commonly been used as indicators for assessing sediment carbon turnover (Jin et al., 2017; Vidya et al., 2023). In our study, we also discovered that invasive S. alterniflora generally increased sediment MBC, DOC, and EOC contents, although some differences were statistically insignificant (Figure 2). It has been noted that sediment TOC consists of labile and recalcitrant carbon fractions, and the labile carbon fractions are more reflective of the variations in TOC (Song et al., 2012; Fu et al., 2023), resulting in similar change patterns for TOC and their labile fractions. This result was also supported by the positive relationship between measured labile carbon fractions with TOC (p< 0.05, Table 3). However, sediment MBC, DOC, and EOC contents across four habitats (SA-14, SA-10, SA-6, and CM) were higher at the surface (0 − 10 cm) compared to the subsurface (10 − 20 cm), which was different from the vertical distribution of TOC in S. alterniflora habitats (Figure 2). This is because the labile fractions are more associated with fresh organic matter, and the availability of plant litter on the surface is generally larger compared to the subsurface (Jin et al., 2017; Xia et al., 2021). Previous works mainly focused on the impact of S. alterniflora invasion on sediment organic carbon contents and stocks in intertidal wetlands, with less research conducted on the changes in its active fractions, and the present study can provide important insight into carbon dynamics in such ecosystems. Compared to carbon contents, the proportions of labile carbon fractions in TOC can better reflect sediment carbon pool turnover (Zhang et al., 2010; Kuzmina et al., 2022). Here, we further calculated the ratios of sediment MBC, DOC, and EOC to TOC. The results showed that MBC/TOC, DOC/TOC, and EOC/TOC values did not differ among four habitats at 0 − 10 cm depth, but these values were lower in S. alterniflora habitats than in C malaccensis habitats at 10 − 20 cm depth, especially in SA-14 (Figure 6). This implied that the changes were less pronounced for carbon turnover rates than carbon contents after S. alterniflora invasion (Jin et al., 2017). Herein, the MBC/TOC ratios are regarded as a microbial quotient (MQ), which characterizes the microbial carbon use efficiency (Cotrufo et al., 2013; Fang et al., 2022). The different change trends in sediment MQ and TOC along invasion chronosequences of S. alterniflora were observed in our study (Figure 2, Figure 6), which was consistent with previous results that MQ was negatively related to TOC (Yang et al., 2013; Jin et al., 2017).
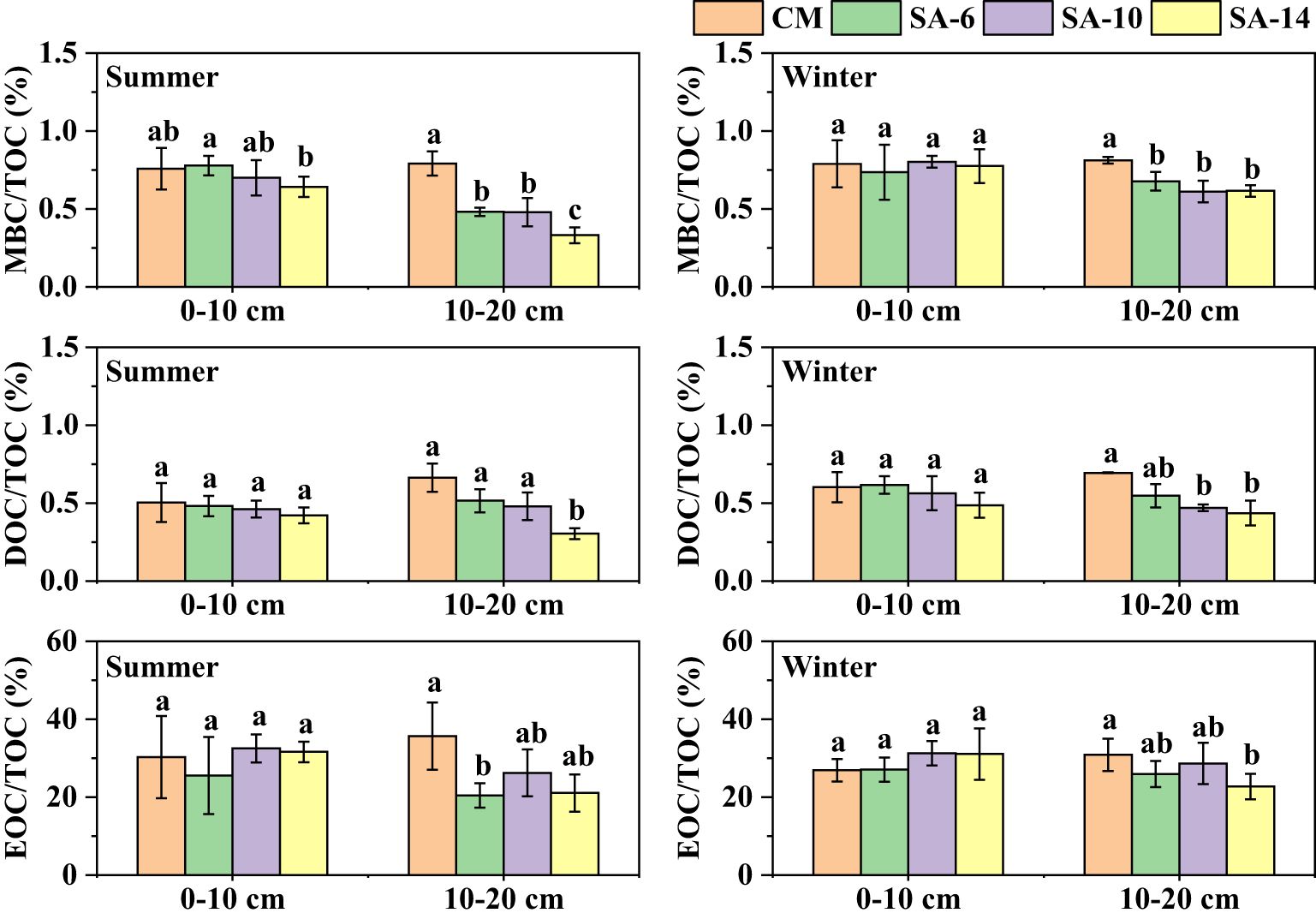
Figure 6 The ratios of sediment MBC, DOC, and EOC to TOC. The CM denotes C. malaccensis habitat, and the SA-6, SA-10, and SA-14 denote S. alterniflora habitats for 2 – 6 years, 6 – 10 years, and 10 – 14 years, respectively. The presence of different lowercase letters indicates statistical differences (p< 0.05) across four habitats in the same depth.
4.2 Effects of invasive Spartina alterniflora on organic carbon mineralization dynamics
Sediment organic carbon mineralization means that microorganisms directly use biogenic elements to complete their metabolism, decomposing organic matter and releasing carbon dioxide (CO2) (Mou et al., 2018; Gaudel et al., 2024). Thus, changes in mineralization characteristics of sediment organic carbon affected by S. alterniflora invasion have the potential to alter organic carbon pools and greenhouse gas emissions in intertidal wetlands of estuaries and coasts (Xia et al., 2021). In this study, sediment organic carbon mineralization rates and cumulative carbon mineralization amounts in S. alterniflora habitats were larger compared to C. malaccensis habitat, and their values increased with S. alterniflora invasion chronosequences, especially at the surface layer in summer (Figure 3, 4, 5). Previous studies noted that sediment substrate availabilities were the main factor influencing organic carbon mineralization, and higher substrate contents can stimulate microbial activities and carbon mineralization (Chapman et al., 2019; Tito et al., 2021). Invasive S. alterniflora increased sediment TOC and labile fraction contents (Figure 2), which might be an important reason for the increase in carbon mineralization rates and their cumulative mineralization amounts. This result was also supported by the positive relationship between cumulative carbon mineralization amounts (Ct) with TOC and EOC (Table 3). In addition, our study showed that cumulative carbon mineralization amounts (Ct) were significantly associated with EC, which was similar to the findings of Weston et al. (2011) who noted that salt-water intrusion altered organic carbon dynamics via microbial decomposition. In addition, Yang et al. (2016b) found that invasive S. alterniflora stimulated various microbial groups and microbial respiration, and this is also an important reason for the increase in organic carbon mineralization. Sediment organic carbon mineralization rates and cumulative carbon mineralization amounts across four habitats were significantly higher at the surface layer compared to the subsurface layer (Figures 3, 5). Sediment TOC contents in C. malaccensis habitat were also larger at the surface than at the subsurface layer, but this was not the case in S. alterniflora habitats (Figure 2). Hence, the vertical distributions of organic carbon mineralization cannot be explained based on changes in TOC contents. Notably, sediment carbon labile fractions (MBC, DOC, and EOC) were larger at the surface than at the subsurface layer (Figure 2). This result implied that sediment organic carbon labile fractions might be the more dominant factor in affecting carbon mineralization dynamics (Mou et al., 2018).
During the 56-day incubation, sediment organic carbon mineralization dynamics were similar across four habitats, and the largest values occurred on day 3 (Figure 3). These changes were tightly associated with the availability of sediment organic carbon. In the early stage (days 1 − 7), sediment carbon labile fractions were rapidly decomposed to provide substrate for microorganisms, which in turn accelerated carbon decomposition and CO2 release (Mou et al., 2018; Ni et al., 2024). While in the middle and late stages (days 7 − 56), sediment carbon labile fractions decreased, and recalcitrant carbon was not easily utilized by microorganisms, resulting in a decrease in organic carbon mineralization and a tendency toward stabilization (Chapman et al., 2019).
Generally, sediment organic carbon mineralization has obvious seasonal differences. In the present study, the organic carbon mineralization rates and cumulative carbon mineralization amounts were larger in summer compared to winter (Figures 3, 5), and this was primarily attributed to the temperature changes. Previous studies noted that microbial activities increase with increasing temperature within a certain range (Gao et al., 2018; Kim et al., 2020). In our study area, mean sediment temperatures in winter and summer were 17.28 ± 0.47°C and 32.95 ± 1.09°C, respectively (Table 1), which explained well the aforementioned results.
The mathematical characterization of organic carbon mineralization kinetics in incubation experiments helps to understand the supply and balance ability of organic carbon (Cooper et al., 2011; Luo et al., 2019). The first-order equation in the present study reflected well the organic carbon mineralization kinetics in four different habitats (Table 2). The potential carbon mineralization amounts (C0) varied from 695.62 to 1523.28 μg g-1, which was generally higher than the previous findings (Llorente and Turrión, 2010; Mou et al., 2018). The changes in estimated C0 in our study were consistent with those of Ct (Table 2), and there was a positive correlation between the two parameters (Table 3), which indicated that sediment carbon decomposition ability was significantly enhanced after S. alterniflora invasion (Lu and Xu, 2014; Rodríguez-Salgado et al., 2017). The carbon mineralization rate constant (k) in S. alterniflora habitats was higher compared to C. malaccensis habitat in summer, further confirming that invasive S. alterniflora raised the effectiveness of organic compounds decomposed by microbial respiration (Mou et al., 2018). Significant relationships were observed between C0 and k (Table 3), implying that the changes in k values among four habitats might be caused by the carbon pool size (Goberna et al., 2006). Overall, the results indicated that invasive S. alterniflora increased sediment organic carbon contents in estuarine and coastal wetlands, which may be conducive to carbon accumulation and storage (Wang et al., 2023). On the other, organic carbon decomposition and mineralization potential also increased with the invasion degrees of S. alterniflora, and this will accelerate CO2 emission and subsequently affect climate change (Bu et al., 2015; Gaudel et al., 2024). From this perspective, invasive S. alterniflora does not promote carbon accumulation by reducing carbon decomposition. Hence, the increased release of carbon-containing greenhouse gases caused by S. alterniflora invasion should attract our attention. In recent years, various measures (biological substitution, physical control, or chemical herbicides) were adopted across China to control S. alterniflora expansion (Zheng et al., 2023), which may have substantial importance in mitigating carbon emissions from estuarine and coastal wetlands. However, there are some disputes regarding the eco-environmental significance after the S. alterniflora invasion (Zheng et al., 2023), which certainly looks worthy of further work. In addition, to better understand the responses of carbon dynamics to this invasive S. alterniflora, the ongoing study should also focus on sediment microbial communities related to carbon turnover. Nevertheless, this study provided valuable insight into sediment carbon dynamics and the management of invasive plants in intertidal wetlands of estuaries and coasts.
5 Conclusions
The present study reported the variations in sediment organic carbon fractions and mineralization dynamics along invasion degrees of S. alterniflora in a subtropical estuarine wetland of China. Invasive S. alterniflora generally raised sediment TOC and labile fraction contents. Sediment organic carbon mineralization rates and Ct were also obviously influenced by S. alterniflora invasion, and their values increased with the degree of this exotic plant invasion. The carbon mineralization rates and Ct were characterized by higher values at the surface compared to the subsurface layer and by seasonal changes with higher values in summer than in winter. Compared to TOC, organic carbon labile fractions were found to be the more key factors influencing carbon mineralization dynamics. The first-order kinetic model provided an excellent description of organic carbon mineralization dynamics. The changes in kinetic parameters (C0 and k) in these four habitats were similar to carbon mineralization rates, indicating an increased ability for organic carbon decomposition following S. alterniflora invasion. Overall, our findings showed the importance of S. alterniflora invasion in facilitating organic carbon decomposition and carbon dioxide release potential, which provided the scientific basis for the management of intertidal wetlands of estuaries and coasts.
Data availability statement
The original contributions presented in the study are included in the article/supplementary material. Further inquiries can be directed to the corresponding authors.
Author contributions
YJ: Formal Analysis, Funding acquisition, Investigation, Writing – original draft, Writing – review & editing. DY: Writing – review & editing. JH: Writing – review & editing, Formal Analysis, Funding acquisition, Methodology, Supervision. YW: Writing – review & editing. HT: Writing – review & editing. JX: Writing – review & editing. XL: Writing – review & editing, Investigation. DS: Investigation, Writing – review & editing, Validation. DG: Investigation, Writing – review & editing, Conceptualization, Funding acquisition, Project administration, Supervision, Writing – original draft.
Funding
The author(s) declare that financial support was received for the research, authorship, and/or publication of this article. This work was supported by the Natural Science Foundation of China (grant number: 42206237) and Science and Technology Project of Huadong Engineering (Fujian) Corporation (ZKY2022-FJ-02-02). It was also funded by the Public Welfare Project of Fujian Science and Technology Department (2022R1002007).
Acknowledgments
We are grateful to the editor and reviewers for constructive comments on this manuscript.
Conflict of interest
Authors YJ, DY, YW, HT, were employed by Huadong Engineering (Fujian) Corporation.
The remaining authors declare that the research was conducted in the absence of any commercial or financial relationships that could be construed as a potential conflict of interest.
The author(s) declared that they were an editorial board member of Frontiers, at the time of submission. This had no impact on the peer review process and the final decision.
The authors declare that this study received funding from Huadong Engineering (Fujian) Corporation. The funder had the following involvement in the study: the study design, collection, analysis, interpretation of data, the writing of the article, and the decision to submit the article for publication.
Publisher’s note
All claims expressed in this article are solely those of the authors and do not necessarily represent those of their affiliated organizations, or those of the publisher, the editors and the reviewers. Any product that may be evaluated in this article, or claim that may be made by its manufacturer, is not guaranteed or endorsed by the publisher.
References
Banerjee A. K., Liang X. R., Harms N. E., Tan F. X., Lin Y. T., Feng H., et al. (2022). Spatio-temporal pattern of cross-continental invasion: Evidence of climatic niche shift and predicted range expansion provide management insights for smooth cordgrass. Ecol. Indic. 140, 109052. doi: 10.1016/j.ecolind.2022.109052
Belay-Tedla A., Zhou X. H., Su B., Wan S. Q., Luo Y. Q. (2009). Labile, recalcitrant, and microbial carbon and nitrogen pools of a tallgrass prairie soil in the US Great Plains subjected to experimental warming and clipping. Soil Biol. Biochem. 41, 110–116. doi: 10.1016/j.soilbio.2008.10.003
Bernik B. M., Li H., Blum M. J. (2016). Genetic variation of Spartina alterniflora intentionally introduced to China. Biol. Invasions 18, 1485–1498. doi: 10.1007/s10530-016-1096-3
Bu N. S., Qu J. F., Li G., Zhao B., Zhang R. J., Fang C. M. (2015). Reclamation of coastal salt marshes promoted carbon loss from previously-sequestered soil carbon pool. Ecol. Eng. 81, 335–339. doi: 10.1016/j.ecoleng.2015.04.051
Castillo J. A. A., Apan A. A., Maraseni T. N., Salmo S. G. (2017). Soil C quantities of mangrove forests, their competing land uses, and their spatial distribution in the coast of Honda Bay, Philippines. Geoderma 293, 82–90. doi: 10.1016/j.geoderma.2017.01.025
Chapman S. K., Hayes M. A., Kelly B., Langley J. A. (2019). Exploring the oxygen sensitivity of wetland soil carbon mineralization. Biol. Lett. 15, 20180407. doi: 10.1098/rsbl.2018.0407
Chen S. T., Gao D. Z., Li X. F., Niu Y. H., Liu C., Sun D. Y., et al. (2023). Invasive Spartina alterniflora accelerates the increase in microbial nitrogen fixation over nitrogen removal in coastal wetlands of China. Eco-Environ. Health 2, 184–192. doi: 10.1016/j.eehl.2023.07.007
Cooper J. M., Burton D., Daniell T. J., Griffiths B. S., Zebarth B. J. (2011). Carbon mineralization kinetics and soil biological characteristics as influenced by manure addition in soil incubated at a range of temperatures. Eur. J. Soil Biol. 47, 392–399. doi: 10.1016/j.ejsobi.2011.07.010
Cotrufo M. F., Wallenstein M. D., Boot C. M., Denef K., Paul E. (2013). The microbial efficiency-matrix stabilization (MEMS) framework integrates plant litter decomposition with soil organic matter stabilization: do labile plant inputs form stable soil organic matter? Glob Change Biol. 19, 988–995. doi: 10.1111/gcb.12113
Dong X. F., Liu C., Wu X. D., Man H. R., Wu X. W., Ma D. L., et al. (2022). Linking soil organic carbon mineralization with soil variables and bacterial communities in a permafrost-affected tussock wetland during laboratory incubation. Catena 221, 106783. doi: 10.1016/j.catena.2022.106783
Emery H. E., Fulweiler R. W. (2014). Spartina alterniflora and invasive Phragmites australis stands have similar greenhouse gas emissions in a New England marsh. Aquat. Bot. 116, 83–92. doi: 10.1016/j.aquabot.2014.01.010
Fang Z. W., Zhou S., Zhang S. H., Xing W. C., Feng X. L., Yang Q. L., et al. (2022). Spatial distribution and influencing factors of urban soil organic carbon stocks in Xi’an City, China. Urban Ecosyst. 26, 677–688. doi: 10.1007/s11252-022-01316-6
Fu Y. H., Hu Z. Q., Zhu Q., Rong Y. (2023). Characteristics of labile organic carbon fractions under different types of subsidence waterlogging areas in a coal mining area: A case study in Xinglongzhuang Coal Mine, China. Catena 232, 107398. doi: 10.1016/j.catena.2023.107398
Gao D. Z., Hou L. J., Li X. F., Liu M., Zheng Y. L., Yin G. Y., et al. (2019). Exotic Spartina alterniflora invasion alters soil nitrous oxide emission dynamics in a coastal wetland of China. Plant Soil 442, 233–246. doi: 10.1007/s11104-019-04179-7
Gao D. Z., Li X. F., Lin X. B., Wu D. M., Jin B. S., Huang Y. P., et al. (2017). Soil dissimilatory nitrate reduction processes in the Spartina alterniflora invasion chronosequences of a coastal wetland of southeastern China: Dynamics and environmental implications. Plant Soil 421, 383–399. doi: 10.1007/s11104-017-3464-x
Gao J., Hou L. J., Zheng Y. L., Liu M., Yin G. Y., Yu C. D., et al. (2018). Shifts in the community dynamics and activity of ammonia-oxidizing prokaryotes along the Yangtze estuarine salinity gradient. J. Geophys. Res-Biogeo. 123, 3458–3469. doi: 10.1029/2017JG004182
Gaudel G., Xing L., Shrestha S., Poudel M., Sherpa P., Raseduzzaman M., et al. (2024). Microbial mechanisms regulate soil organic carbon mineralization under carbon with varying levels of nitrogen addition in the above-treeline ecosystem. Sci. Total Environ. 917, 170497. doi: 10.1016/j.scitotenv.2024.170497
Goberna M., Sánchez J., Pascual J. A., García C. (2006). Surface and subsurface organic carbon, microbial biomass and activity in a forest soil sequence. Soil Biol. Biochem. 38, 2233–2243. doi: 10.1016/j.soilbio.2006.02.003
Grasset C., Rodriguez C., Delolme C., Marmonier P., Bornette G. (2017). Can soil organic carbon fractions be used as functional indicators of wetlands? Wetlands 37, 1195–1205. doi: 10.1007/s13157-017-0951-z
He S. X., Lin J. L., Liu X. M., Jia S. W., Chen S. Q. (2023). Cordgrass Spartina alterniflora acts as a key carbon source to support macrozoobenthos in the salt marsh and nearby mudflat communities. Ecol. Indic. 148, 110052. doi: 10.1016/j.ecolind.2023.110052
He J. Y., Yin J. J., Wu J. P., Christakos G. (2024). Accurate carbon storage estimation for the salt marsh ecosystem based on Bayesian maximum entropy approach-A case study for the Spartina alterniflora ecosystem. J. Environ. Manage. 354, 120278. doi: 10.1016/j.jenvman.2024.120278
Hinson A. L., Feagin R. A., Eriksson M. (2019). Environmental controls on the distribution of tidal wetland soil organic carbon in the continental United States. Global Biogeochem. Cy. 33, 1408–1422. doi: 10.1029/2019GB006179
Jin B. S., Lai D. Y. F., Gao D. Z., Tong C., Zeng C. S. (2017). Changes in soil organic carbon dynamics in a native C4 plant-dominated tidal marsh following spartina alterniflora invasion. Pedosphere 27, 856–867. doi: 10.1016/S1002-0160(17)60396-5
Kim J., Chaudhary D. R., Kang H. (2020). Nitrogen addition differently alters GHGs production and soil microbial community of tidal salt marsh soil depending on the types of halophyte. Appl. Soil Ecol. 150, 103440. doi: 10.1016/j.apsoil.2019.103440
Kuzmina D. M., Lim A. G., Loiko S. V., Shefer N., Shirokova L. S., Julien F., et al. (2022). Dispersed ice of permafrost peatlands represents an important source of labile carboxylic acids, nutrients and metals. Geoderma 429, 116256. doi: 10.1016/j.geoderma.2022.116256
Li N., Li B., Nie M., Wu J. H. (2019). Effects of exotic Spartina alterniflora on saltmarsh nitrogen removal in the Yangtze River Estuary, China. J. Clean. Prod. 271, 122557. doi: 10.1016/j.jclepro.2020.122557
Liao C. Z., Luo Y. Q., Jiang L. F., Zhou X. H., Wu X. W., Fang C. M., et al. (2007). Invasion of spartina alterniflora enhanced ecosystem carbon and nitrogen stocks in the Yangtze estuary, China. Ecosystems 10, 1351–1361. doi: 10.1007/s10021-007-9103-2
Liu C. J., Li P., Xie W. X., Sha M. Q., Ding W. C. (2021). Changes of sulfur fractions in sediment following Spartina alterniflora invasion in a seaward direction in a temperate salt marsh, China. Ecol. Indic. 131, 108217. doi: 10.1016/j.ecolind.2021.108217
Liu C., Xia J. B., Cui Q., Zhang D. J., Liu M., Hou L. J., et al. (2022). Crab bioturbation affects competition between microbial nitrogen removal and retention in estuarine and coastal wetlands. Environ. Res. 215, 114280. doi: 10.1016/j.envres.2022.114280
Llorente M., Turrión M. B. (2010). Microbiological parameters as indicators of soil organic carbon dynamics in relation to different land use management. Eur. J. For. Res. 129, 73–81. doi: 10.1007/s10342-008-0249-z
Lu Y., Xu H. W. (2014). Distribution characteristic of soil organic carbon fraction in different types of wetland in Hongze lake of China. Sci. World J. 2014, 487961. doi: 10.1155/2014/487961
Luo M., Huang J. F., Zhu W. F., Tong C. (2019). Impacts of increasing salinity and inundation on rates and pathways of organic carbon mineralization in tidal wetlands: a review. Hydrobiologia 827, 31–49. doi: 10.1007/s10750-017-3416-8
Mou X. J., Liu X. T., Sun Z. G., Tong C., Huang J. F., Wan S., et al. (2018). Effects of anthropogenic disturbance on sediment organic carbon mineralization under different water conditions in coastal wetland of a subtropical estuary. Chin. Geogr. Sci. 28, 400–410. doi: 10.1007/s11769-018-0956-4
Mou X., Liu X. T., Tong C., Sun Z. G. (2014). Responses of CH4 emissions to nitrogen addition and Spartina Alterniflora invasion in Minjiang River estuary, southeast of China. Chin. Geogr. Sci. 24, 562–574. doi: 10.1007/s11769-014-0692-3
Ni B. B., Yu X. F., Duan X., Zou Y. C. (2024). Wetland soil organic carbon balance is reversed by old carbon and iron oxide additions. Front. Microbiol. 14, 1327265. doi: 10.3389/fmicb.2023.1327265
Rodríguez-Salgado I., Pérez-Rodríguez P., Santás V., Nóvoa-Muñoz J. C., Arias-Estévez M., Díaz-Raviña M., et al. (2017). Carbon mineralization in acidic soils amended with an organo-mineral bentonite waste. J. Soil Sci. Plant Nutt. 17, 624–634. doi: 10.4067/S0718-95162017000300006
Roldán A., Salinas-García J. R., Alguacil M. M., Caravaca F. (2005). Changes in soil enzyme activity, fertility, aggregation and C sequestration mediated by conservation tillage practices and water regime in a maize field. Appl. Soil Ecol. 30, 11–20. doi: 10.1016/j.apsoil.2005.01.004
Song Y. Y., Song C. C., Yang G. S., Miao Y. Q., Wang J. Y., Guo Y. D. (2012). Changes in labile organic carbon fractions and soil enzyme activities after marshland reclamation and restoration in the Sanjiang Plain in Northeast China. Environ. Manage. 50, 418–426. doi: 10.1007/s00267-012-9890-x
Steinmuller H. E., Chambers L. G. (2019). Characterization of coastal wetland soil organic matter: Implications for wetland submergence. Sci. Total Environ. 677, 648–659. doi: 10.1016/j.scitotenv.2019.04.405
Throop H. L., Lajtha K., Kramer M. (2013). Density fractionation and 13C reveal changes in soil carbon following woody encroachment in a desert ecosystem. Biogeochemistry 112, 409–422. doi: 10.1007/s10533-012-9735-y
Tito G. A., Fernandes J. D., Chaves L. H. G., Guerra H. O. C., Dantas E. R. B. (2021). Organic carbon mineralization of the biochar and organic compost of poultry litter in an Argisol. Semin-Cienc. Agrar. 42, 3167–3184. doi: 10.5433/1679-0359
Tong C., Zhang L. H., Wang W. Q., Gauci V., Marrs R., Liu B. G., et al. (2011). Contrasting nutrient stocks and litter decomposition in stands of native and invasive species in a sub-tropical tidal estuarine marsh. Environ. Res. 111, 909–916. doi: 10.1016/j.envres.2011.05.023
Vidya P. V., Rajathy S., Kumar C. S. R. (2023). Composition of sedimentary organic matter in Thrissur Kole wetland, southwest India. Environ. Earth Sci. 82, 174. doi: 10.1007/s12665-023-10854-x
Villoslada M., Sipelgas L., Ward R. D., Reintam E., Astover A., Kumpula T., et al. (2022). Multi-source remote sensing data reveals complex topsoil organic carbon dynamics in coastal wetlands. Ecol. Indic. 143, 109329. doi: 10.1016/j.ecolind.2022.109329
Wang K., Wang S., Zhang X., Wang W. Y., Wang X. Y., Kong F. L., et al. (2023). The amelioration and improvement effects of modified biochar derived from Spartina alterniflora on coastal wetland soil and Suaeda salsa growth. Environ. Res. 240, 17426. doi: 10.2139/ssrn.4572161
Weston N. B., Vile M. A., Neubauer S. C., Velinsky D. J. (2011). Accelerated microbial organic matter mineralization following salt-water intrusion into tidal freshwater marsh soils. Biogeochemistry 102, 135–151. doi: 10.1007/s10533-010-9427-4
Williams E. K., Rosenheim B. E. (2015). What happens to soil organic carbon as coastal marsh ecosystems change in response to increasing salinity? An exploration using ramped pyrolysis. Geochem. Geophy. Geosy. 16, 2322–2335. doi: 10.1002/2015GC005839
Xia S. P., Wang W. Q., Song Z. L., Kuzyakov Y., Guo L. D., Van Zwieten L., et al. (2021). Spartina alterniflora invasion controls organic carbon stocks in coastal marsh and mangrove soils across tropics and subtropics. Global Change Biol. 27, 1627–1644. doi: 10.1111/gcb.15516
Yang W., Jeelani N., Leng X., Cheng X., An S. (2016b). Spartina alterniflora invasion alters soil microbial community composition and microbial respiration following invasion chronosequence in a coastal wetland of China. Sci. Rep. 6, 26880. doi: 10.1038/srep26880
Yang W., Yan Y. E., Jiang F., Leng X., Chen X. L., An S. Q. (2016a). Response of the soil microbial community composition and biomass to a short-term Spartina alterniflora invasion in a coastal wetland of eastern China. Plant Soil 408, 443–456. doi: 10.1007/s11104-016-2941-y
Yang W., Zhao H., Chen X. L., Yin S. L., Cheng X. L., An S. Q. (2013). Consequences of short-term C4 plant Spartina alterniflora invasions for soil organic carbon dynamics in a coastal wetland of Eastern China. Ecol. Eng. 61, 50–57. doi: 10.1016/j.ecoleng.2013.09.056
Yu X. Q., Yang J., Liu L. M., Tian Y., Yu Z. (2015). Effects of Spartina alterniflora invasion on biogenic elements in a subtropical coastal mangrove wetland. Environ. Sci. pollut. Res. 22, 3107–3115. doi: 10.1007/s11356-014-3568-2
Zhang G. L., Bai J. H., Jia J., Wang X., Wang W., Zhao Q. Q., et al. (2018). Soil organic carbon contents and stocks in coastal salt marshes with spartina alterniflora following an invasion chronosequence in the yellow river delta, China. Chin. Geogra. Sci. 28, 374–385. doi: 10.1007/s11769-018-0955-5
Zhang Y. H., Ding W. S., Luo J. F., Donnison A. (2010). Changes in soil organic carbon dynamics in an Eastern Chinese coastal wetland following invasion by a C4 plant Spartina alterniflora. Soil Biol. Biochem. 42, 1712–1720. doi: 10.1016/j.soilbio.2010.06.006
Zhang W. L., Zeng C., Tong C., Zhang Z. C., Huang J. F. (2011). Analysis of the expanding process of the Spartina alterniflora salt marsh in Shanyutan Wetland, Minjiang River estuary by remote sensing. Proc. Environ. Sci. 10, 2472–2477. doi: 10.1016/j.proenv.2011.09.385
Keywords: organic carbon fractions, mineralization, dynamics, Spartina alterniflora, estuarine and coastal wetland
Citation: Jiang Y, Yang D, Huang J, Wen Y, Tang H, Xu J, Lin X, Sun D and Gao D (2024) Invasive Spartina alterniflora alters sediment organic carbon mineralization dynamics in a coastal wetland of Southeastern China. Front. Mar. Sci. 11:1400381. doi: 10.3389/fmars.2024.1400381
Received: 13 March 2024; Accepted: 10 May 2024;
Published: 31 May 2024.
Edited by:
Licheng Peng, Hainan University, ChinaCopyright © 2024 Jiang, Yang, Huang, Wen, Tang, Xu, Lin, Sun and Gao. This is an open-access article distributed under the terms of the Creative Commons Attribution License (CC BY). The use, distribution or reproduction in other forums is permitted, provided the original author(s) and the copyright owner(s) are credited and that the original publication in this journal is cited, in accordance with accepted academic practice. No use, distribution or reproduction is permitted which does not comply with these terms.
*Correspondence: Dengzhou Gao, dzgao@fjnu.edu.cn; Jiafang Huang, wahugeo@fjnu.edu.cn