Effects of dietary astaxanthin on growth performance, immunity, and tissue composition in largemouth bass, Micropterus salmoides
- 1Department of Aquaculture, Zhejiang Ocean University, Zhoushan, China
- 2Guangdong Yuehai Feed Group Co., Ltd., Zhanjiang, China
- 3College of Life Science, Huzhou University, Huzhou, China
This study examined the effects of feeding largemouth bass (Micropterus salmoides) with diets containing different doses of astaxanthin (0, 50, 100, 150, and 200 mg/kg) for 8 weeks. The results showed that the values of weight gain significantly increased from 620.32 ± 50.38% to 826.14 ± 33.49% as dietary astaxanthin levels increased from 0 mg/kg to 100 mg/kg. When the astaxanthin level exceeded 150mg/kg, the weight gain rate showed a downward trend, but there was no significant difference among of the 100, 150 and 200 mg/kg groups. The feed conversion ratio (FCR) and protein efficiency ratio (PER) were also improved by adding astaxanthin to diets (P < 0.05). Meanwhile, adding astaxanthin to the feed increased the length and thickness of intestinal villus and muscle layer thickness (P < 0.05). The astaxanthin supplementation increased the expression of the NF-E2-related factor (Nrf2) gene and reduced malondialdehyde (MDA) content and the expression of apoptosis genes Caspase-9 and Caspase-3 (P < 0.05), indicating that it has a good antioxidant ability. Furthermore, adding astaxanthin increased the content of non-specific immune markers and decreased the expression levels of the inflammatory factors interleukin-15 (IL-15) and tumor necrosis factor-α (TNF-α). Moreover, fish fed diets with astaxanthin exhibited lower blood cortisol levels (P < 0.05). The proportions of C20:4n6 (ARA) and C20:5n3 (EPA) in the liver decreased with increasing dietary astaxanthin levels. Based on WGR and SGR values, the optimal addition level of astaxanthin in largemouth bass feed is 134.8 mg/kg ~ 135.75 mg/kg. In summary, the appropriate dietary astaxanthin enhanced the antioxidant capacity and immune response of largemouth bass and had a positive effect on its intestinal health.
1 Introduction
Due to changing socio-economic landscapes, intensive aquaculture systems have been developed (Lin et al., 2023). Although intensive aquaculture systems have their advantages, they also bring various stress factors, including temperature fluctuations, water quality deterioration, intra-species competition, and overcrowding etc (Xie et al., 2008). These stressors make aquatic organisms more vulnerable to diseases and stress-related responses, ultimately leading to a significant decrease in yield (Tal et al., 2018). Particularly in the summer, rising temperatures can further exacerbate the situation, increasing the risk of various diseases related to fish stress (Grant et al., 2003). Therefore, researchers have made it a priority to find ways to alleviate the adverse effects of these stressors, such as using fatty acids (Todorčević et al., 2010), herbal compounds (Tan et al., 2017), and vitamins (El-Sayed and Izquierdo, 2021) and so on.
Astaxanthin is an unsaturated compound known as a ketocarotenoid or terpenoid, which is an important feed additive for aquatic animals. It has multiple biological functions, such as antioxidant activity, modulation of immune responses, and enhancing disease resistance (Martin et al., 1999). Earlier research showed that astaxanthin possesses superior antioxidant capability compared to other carotenoids (Naguib and Yousry, 2000). Despite synthetic astaxanthin products are available, Haematococcus pluvialis (algae) is still the most efficient natural source of astaxanthin. Scientific analyses demonstrated that astaxanthin derived from this alga exhibits better stability and antioxidant properties compared to synthetic sources (Ambati et al., 2014). It has been recognized that astaxanthin is an important feed ingredient in aquaculture, which can significantly reduce the hazards associated with environmental stress in fish farming (Jagruthi et al., 2014). Dietary astaxanthin could enhance growth performance, antioxidant defense, and immunity in numerous species, including the large yellow croaker (Larimichthys crocea) (Li et al., 2014) and banded catfish (Tachysurus fulvidraco) (Liu et al., 2016).
The largemouth bass (Micropterus salmoides) is a popular freshwater fish widely cultivated in China due to its rapid growth, easy breeding, few bones, and delicious taste (Kou et al., 2023). In 2022, its production reached 802,486 tons in China, a 14.30 percent increase over the production of 702.093 tons in 2021 (China Fishery Statistical Yearbook, 2023). However, the intensive farming of largemouth bass faces enormous challenges, such as harsh aquatic environment and increased risks of pathogen proliferation (Guo et al., 2020). Previous research showed that astaxanthin can enhance growth performance and tolerance to oxidative stress, and reduce cellular apoptosis in largemouth bass fed high-fat diets (Xie et al., 2020). However, the impact of adding astaxanthin to the normal diet on largemouth bass has not been reported. Therefore, the purpose of this research is to investigate the effects of dietary astaxanthin, derived naturally from Haematococcus pluvialis, on the growth performance, immune ability, and tissue composition of largemouth bass. The research intends to provide useful insights into the potential advantages of astaxanthin as a functional substance for aquatic species.
2 Materials and methods
2.1 Diets
Different doses of astaxanthin were added to five isonitrogenous and isolipidic diets to conduct experiments. The doses were 0 mg/kg (AX0), 50 mg/kg (AX50), 100 mg/kg (AX100), 150 mg/kg (AX150), and 200 mg/kg (AX200), respectively. The formulation and composition of diets are listed in Table 1. The fatty acid profiles of the diets are shown in Table 2. The main protein and lipid sources of experimental diets were fish meal, fish oil and soybean oil, respectively. The dry powdered feed ingredients were meticulously weighed and thoroughly mixed before adding mixed fish and soybean oils. Subsequently, approximately 30% distilled water was added to the mixture. After that, the dough was extruded into 1.5-mm pellets using a twin-screw extruder, and all produced feeds were placed in an air-conditioned chamber at 16°C to be blown dry using cold air, and then stored in a freezer at -20°C for future use.
2.2 Animals
An 8-week feeding study was carried out at Zhejiang Ocean University’s Nutrition Laboratory (Zhoushan, China). The juvenile fish were supplied by a commercial company (Zhengda Aquaculture Co., Huzhou, Zhejiang). They were temporarily raised in 2m3 culture tank and given commercial feed (48% crude protein, 8% crude lipid) for 2 weeks. After the temporary rearing period, 315 juvenile fish (4.21 ± 0.04 g/fish) with uniform size and vigorous viability were randomly selected using lottery method and allocated to 15 tanks (100L). All tanks were divided into five groups, with three replicates in each treatment group (21 fish per tank). The fish were fed the experimental diets at 8:00 and 18:00 every day until they were satisfied. Throughout the experiment period, the water temperature was 25–29°C, the ammonia nitrogen level was < 0.05 mg/L, and the dissolved oxygen content was > 7 mg/L. The photoperiod was 12L:12D. The trial was carried out in static water (aerated tap water), which was changed twice daily at 60% for each time.
2.3 Sample collection
After the acclimatization period, 10 juveniles were randomly selected and euthanized with overdose MS-222 (250 mg/L in culture water buffered with 200 mg/L of sodium bicarbonate), and then stored in a freezer at -80°C for subsequent analysis of the whole-body composition. At the end of the 8-week feeding trial, all fish in each tank were bulk weighed and counted after a 24-hour fasting. Six fish were then randomly selected from each tank, euthanized with MS-222 (250 mg/L in culture water buffered with 200 mg/L of sodium bicarbonate), and placed in an ultra-low-temperature refrigerator for body composition analysis. After anesthetized with MS-222 (125 mg/L) for another nine fish, blood was obtained from the tail vein and allowed to clot at 4°C for 2 hours. Blood samples were centrifuged at a rate of 4000 g for 10 min at 4°C to obtain serum. Another three fish from each tank were humanely euthanized with overdose MS-222 (250 mg/L in culture water buffered with 200 mg/L of sodium bicarbonate) and then their body length, and weight of viscera and liver were recorded to calculate the condition indices (CF, VSI and HSI). All samples were frozen promptly in liquid nitrogen, then stored at -80°C until analysis. The intestines of three fish from each tank were soaked in 4% paraformaldehyde for histological examination.
2.4 Proximate composition analysis of the sample
The proximate composition of the samples was determined based on the method of the AOAC (1995). The moisture content of the feed was determined by drying it to a constant weight in a 105°C drying oven. The moisture content of the whole fish body was measured using a freeze dryer (LL1500, Thermo Scientific, Waltham, USA) at -110°C. Using a Kjeldahl nitrogen analyzer (K355/K437, Buchi, Flawil, Switzerland) to measure crude protein content (N × 6.25). Crude fat was determined with petroleum ether using a Soxhlet extractor (E816, Buchi, Flawil, Switzerland). The sample was put in a muffle furnace (KSW, Kewei, Beijing, China) at 550°C for around 12 hours to determine the ash content. According to the method of Liu et al. (2021), the fatty acid composition was measured using a gas chromatograph (GC7890B, Agilent Technologies Inc., CA, USA).
2.5 Histological testing
Samples from the intestines were fixed in paraformaldehyde solution for 24 hours, and partial midguts were picked and dehydrated in a graded alcohol series, then sliced into thin sections (5μm) after being embedded in paraffin. Tissue sections were stained with hematoxylin and eosin to analyze gut morphology parameters, and the gut morphology was observed under a microscope (OLYMPUS, U-LS30–3). According to the region-specific measuring technique reported by Zhao et al. (2020), the length and thickness of villi were measured using Image J software.
2.6 Determination of physio-biochemical indicators
The physio-biochemical indicators were analyzed spectrophotometrically using commercial test kits such as total protein (No. A045–4-1), total antioxidant capacity (T-AOC, No. A015–2-1), catalase (CAT, No. A007–1-1), superoxide dismutase (SOD, No. A001–3-2), malondialdehyde (MDA, No. A003–1-2), acid phosphatase (ACP, No. A060–2-1), alkaline phosphatase (AKP, No. A059–2-1), and lysozyme (LZM, No. A050–1-1). Cortisol content was determined employing the competitive method of ELISA with a fish-specific serum reagent kit. All testing processes were carried out in accordance with the manufacturer’s instructions, and were performed by a microplate reader (iMark, Bio-Rad, Hercules, USA). All test kits were purchased from Nanjing Jiancheng Bioengineering Institute (Nanjing, China).
2.7 Gene expression
Following the method of Chomczynski and Sacchi (2006), total RNA from the liver was extracted utilizing TRIzol (Invitrogen, CA, USA) and other reagents. Total RNA concentration and purity were assess using a spectrophotometer (Nanodrop 2000, Thermo Scientific, Waltham, USA) and 1% agarose gel electrophoresis, respectively. According to the manufacturer’s instructions, cDNA was synthesized using 500 ng total RNA by PrimeScriptTM RT Reagent Kit (Takara, Dalian, China). The relative expression of target genes was determined by a Real-Time PCR equipment (Life Technologies, Carlsbad, USA, QuantStudioTM 6 Flex). With β-actin as the reference gene, the expression level of the target gene was calculated by the 2-ΔΔCT technique (Livak and Schmittgen, 2001). The specific primers used in this study are shown in Table 3.
2.8 Data analysis
All data were statistically analyzed using SPSS 26.0 software. Homogeneity was checked by Levene’s test. If the data was homogeneous, one-way ANOVA and Duncan’s multiple range test were used to analyze the difference of means across all treatments. If the variances were found to be non-homogeneous, the Kruskal-Wallis test was used. Data were presented as standard deviation (mean ± S.D.), with a significance level set at P < 0.05. The composition of tissue fatty acids were studied through principal component analysis (PCA). The graphs were produced via R software (version 4.1.2). To determine the optimal dose of astaxanthin supplementation in the diet, a quadratic polynomial regression model was employed.
3 Results
3.1 Growth performance, feed utilization, and morphological indicators
The results of growth and feed utilization are shown in Table 4. After 8 weeks of culture trials, it was found that adding astaxanthin to diets had a considerable favorable influence on the growth performance of largemouth bass. The weight gain rate (WGR) and specific growth rate (SGR) of the group that received AX100 diet were significantly higher than those received the AX0 and AX50 diets. Although there was a downward trend, the WGR and SGR values were not statistically significant difference among the three groups of AX100, AX150 and AX200. As the level of astaxanthin in the diet increases, the feed conversion ratio (FCR) tends to decrease, with the FCR of the AX150 group significantly lower than that of the AX0 group (P < 0.05). The protein efficiency ratio (PER) value of the AX0 group was significantly lower than those of the AX100, AX150, and AX200 groups (P < 0.05). However, no significant changes in PER values were observed among the groups supplemented with astaxanthin (P > 0.05). Additionally, no significant differences (P > 0.05) were observed in the condition factor (CF), hepatosomatic index (HSI), and viscera-somatic index (VSI) among all groups. Through quadratic polynomial regression analysis of WGR (Figure 1) and SGR (Figure 2), it was determined that the optimal astaxanthin supplementation level in the feed for largemouth bass was between 134.8 ~ 135.75 mg kg-1.
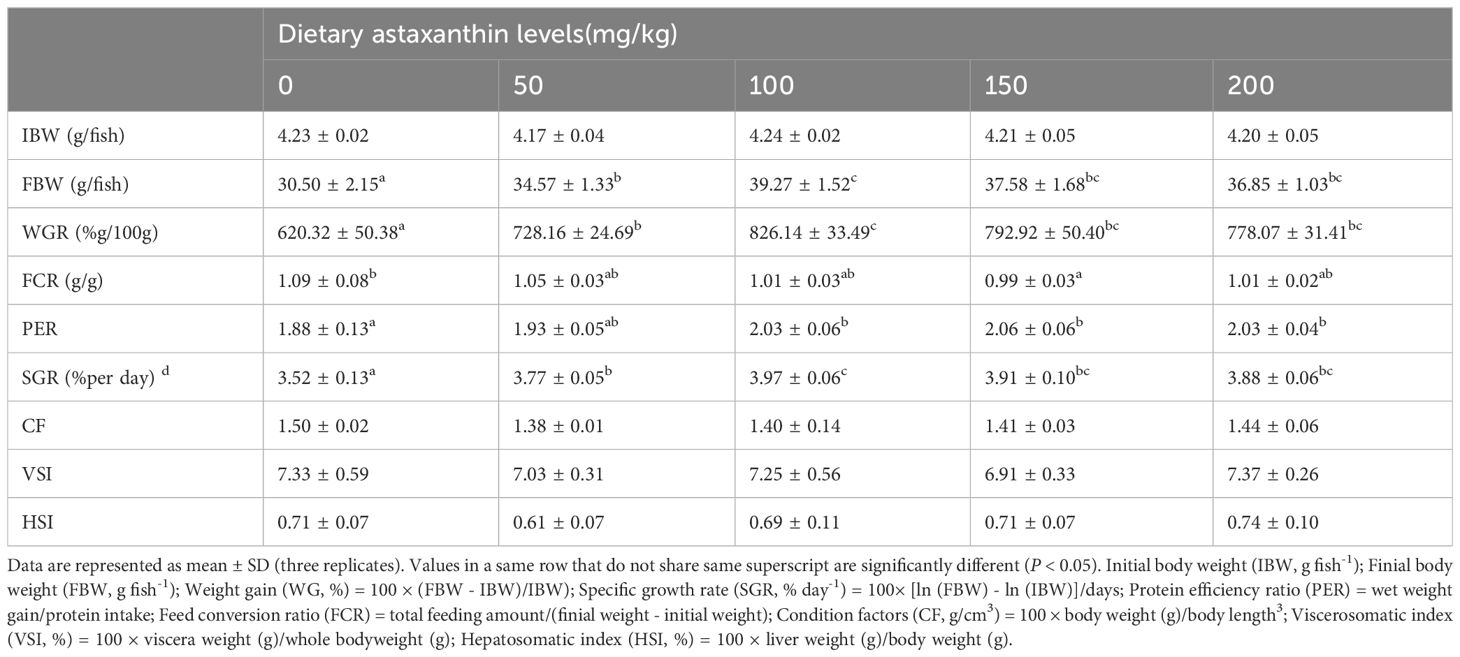
Table 4 Growth performances of largemouth bass fed with diets containing different astaxanthin levels.
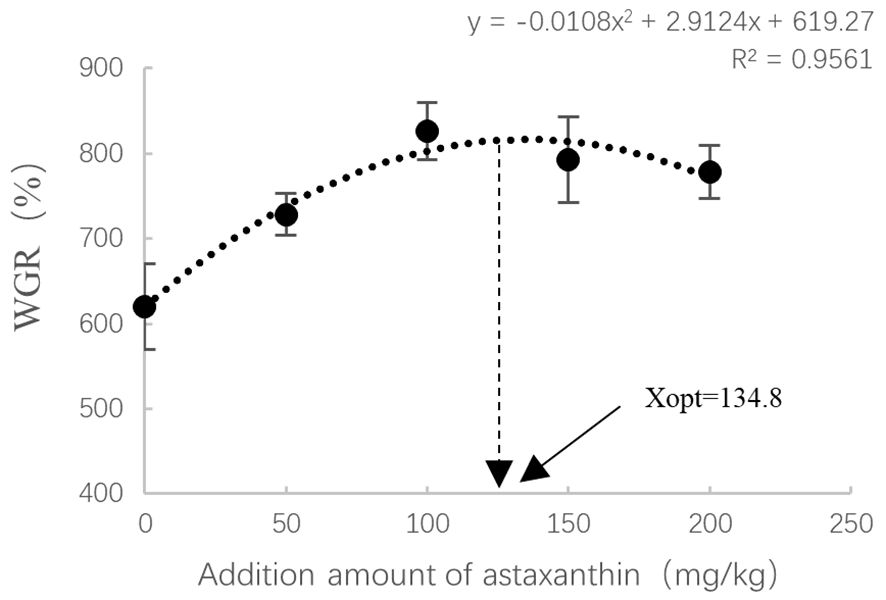
Figure 1 Relationship between astaxanthin supplementation levels and weight gain rate in largemouth bass. Where Xopt represents the optimal dietary astaxanthin level for the maximum WGR.
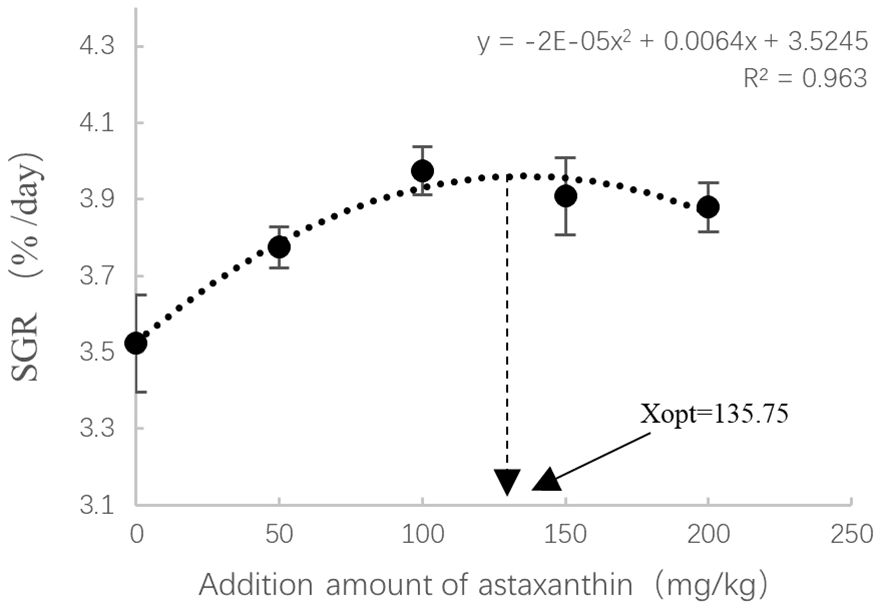
Figure 2 Relationship between astaxanthin supplementation levels and special growth ratio in largemouth bass. Where Xopt represents the optimal dietary astaxanthin level for the maximum SGR.
3.2 Proximate composition of whole body
As shown in Table 5, the whole-body moisture, crude protein, crude fat, or ash contents were not significantly affected by the feed treatments (P > 0.05).
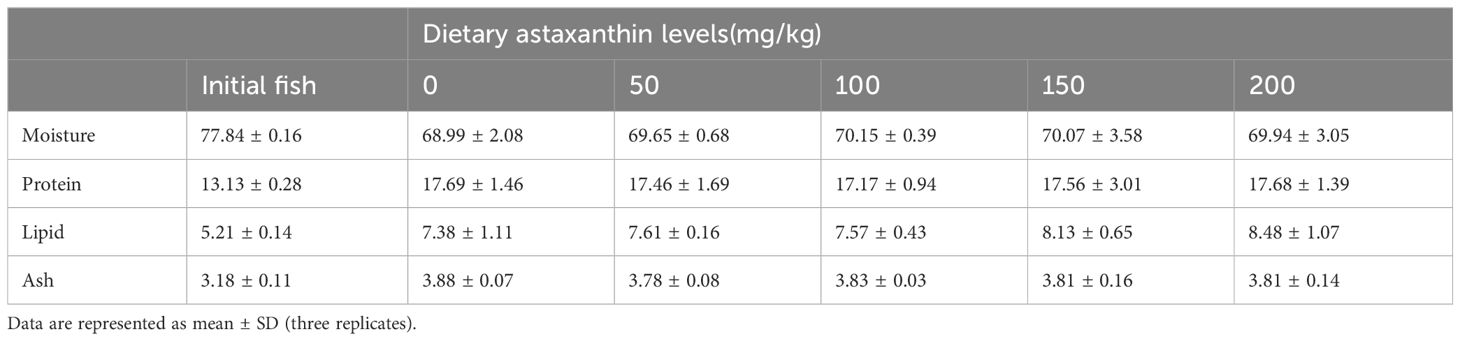
Table 5 Body proximate composition in largemouth bass fed with diets containing different astaxanthin levels.
3.3 Organizational analysis
The inspection results of the intestinal histomorphology are presented in Figure 3. The addition of astaxanthin to feed has a significant impact on the intestinal morphology of largemouth bass. Compared with the AX0 and AX50 groups, the villi length of the AX150 group was significantly increased (P < 0.05). And, the villus thickness of the AX100, AX150, and AX200 groups was significantly higher (P < 0.05) than that of control group (AX0). In addition, the muscular layer thickness was considerably higher in the AX100 and AX150 groups as that of AX0 group (P < 0.05).
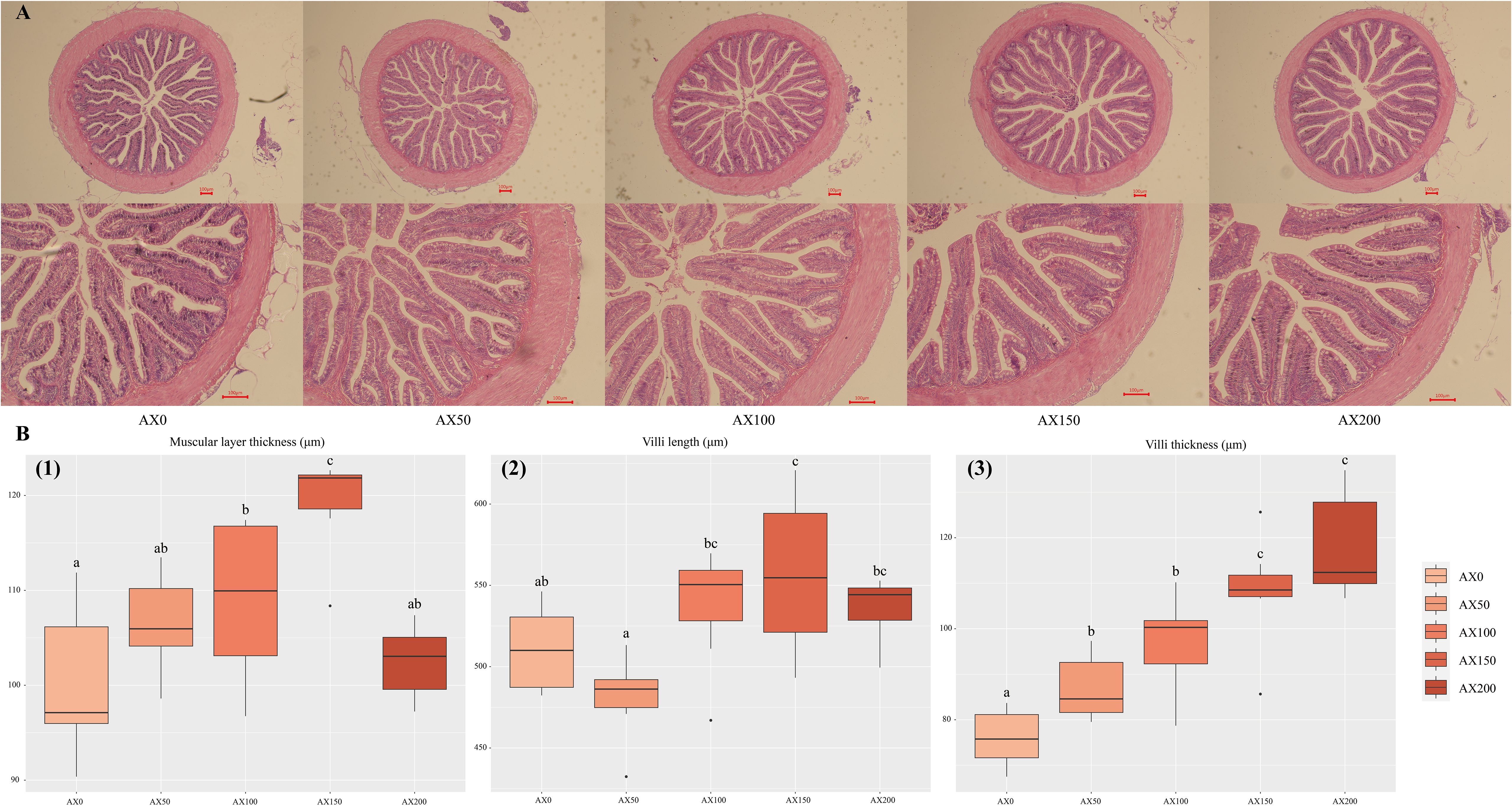
Figure 3 Intestinal histology (A) and morphometry (B) of largemouth bass intestine. (A) First row magnification 40x, second row magnification 100x; Scale bar: 100 μm. (B) (1) muscular layer thickness (μm); (2) villus length (μm); (3) villus thickness (μm). Values were presented as mean ± SD (n = 9), different letters indicate significant differences (P < 0.05).
3.4 Physio-biochemical parameters
The effects of dietary astaxanthin levels on the antioxidant and immune performance of largemouth bass are showed in Figure 4 and Supplementary Table 1. As the dose of astaxanthin increased, so did the levels of T-AOC in the serum and liver. T-AOC levels of both tissues in the AX150 group were significantly higher than those in the control group (P < 0.05). The activities of SOD and CAT in the liver and serum exhibited an increasing trend with the rising levels of dietary astaxanthin, with CAT activity in the liver of the AX200 group significantly higher than that of the control group (P < 0.05). As the astaxanthin level increased from 0 to 150 mg/kg, the MDA content in serum and liver significantly decreased, with the AX150 group having significantly lower values than the control group (P < 0.05). Astaxanthin supplementation can significantly enhance the immunity of largemouth bass. Specifically, serum LZM activity was highest in the AX100 and AX150 groups (P < 0.05). ACP activity also increased significantly with increasing dietary astaxanthin levels (P < 0.05). Compared with the control group, the AKP levels in the AX100, AX150, and AX200 groups were significantly higher (P < 0.05). In addition, the cortisol contents in the serum in the astaxanthin-added groups were significantly lower than that in the AX0 group (P < 0.05).
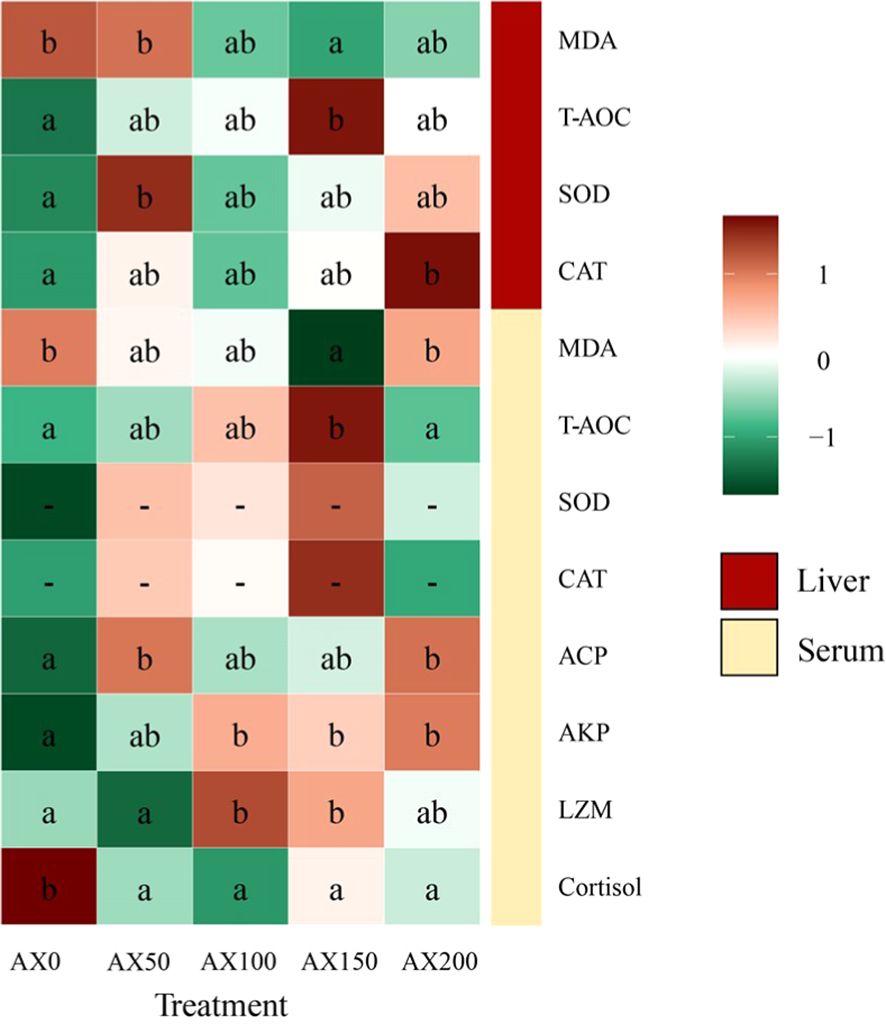
Figure 4 Physio-biochemical parameters in serum and liver of largemouth bass fed experimental diets for 8 weeks. Different letters indicate significant differences in the same row (P < 0.05).
3.5 Gene expressions
As shown in Figure 5, the expression levels of TNF-α decreased with increasing astaxanthin levels in diets. Compared to the control group, the values of the AX150 and AX200 groups were significantly lower (P < 0.05). The expression level of IL-15 gene showed a decreasing trend with increasing astaxanthin supplementation (P > 0.05), but there was no significant difference among the groups. The levels of Caspase-3 and Caspase-9 in the groups added astaxanthin were significantly lower than those in the group non-added astaxanthin (P < 0.05). The expression level of Nrf2 gene in the AX150 group was the highest among all groups and significantly higher than the value in the AX0 group (P < 0.05).
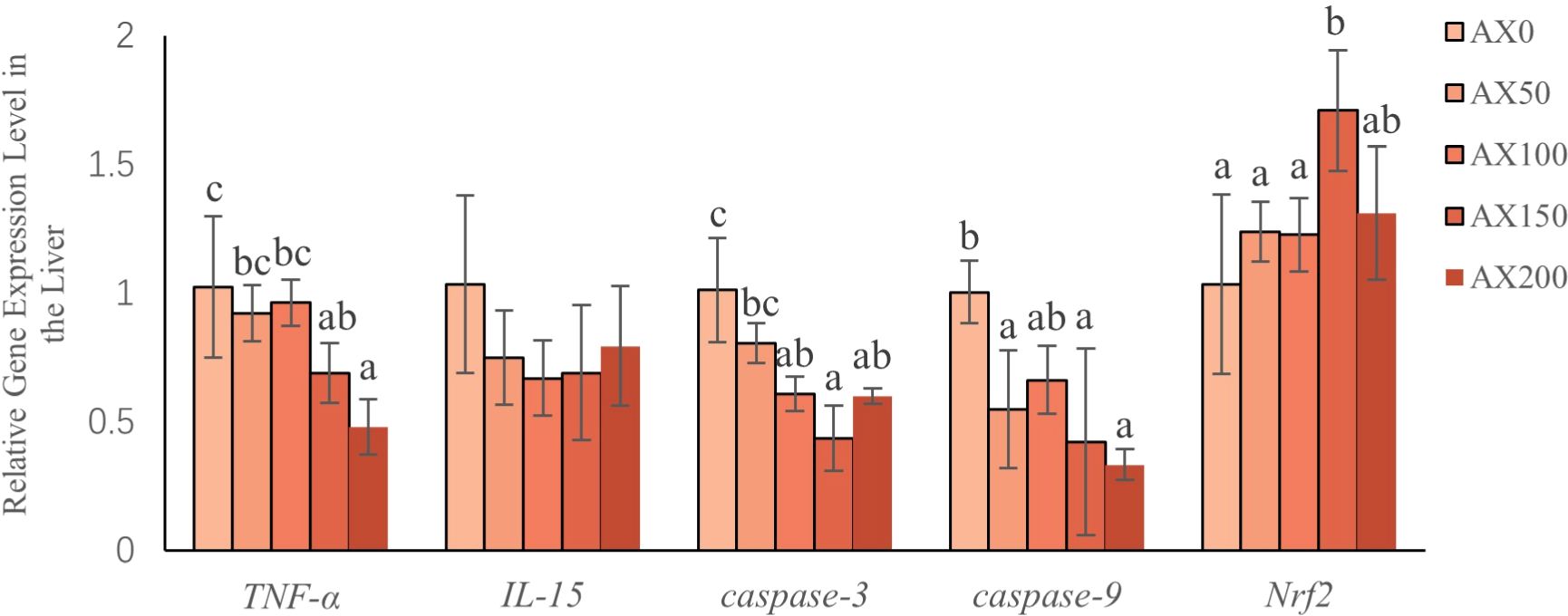
Figure 5 Gene expression (Nrf2, Caspase-3, Caspase-9, TNF-α, IL-15) in the liver after feeding different levels of astaxanthin. Different letters indicate significant differences between dietary groups within each gene (P < 0.05).
3.6 Fatty acid profile
The effects of feeding different levels of astaxanthin on tissue fatty acid composition of largemouth bass are showed in Figure 6 and Supplementary Tables 2, 3. The results showed that dietary astaxanthin levels had a significant effect on the contents of C16:0 and C20:5n3 (EPA) in muscle and most fatty acid contents in the liver, including SFA, MUFA, n-6PUFA, and n-3PUFA. However, the C22:6n3 (DHA) content in muscle and liver did not change significantly among treatments. With the increase of astaxanthin levels in the diet, the EPA content in muscle and liver decreased significantly (P < 0.05). Moreover, the content of C20:4n6 (ARA) in the liver significantly decreased with the increase of astaxanthin levels in the diets (P < 0.05). The liver content of 18:2n-6 and 18:3n-3 increased with increasing dietary astaxanthin levels (P < 0.05). The results of the principal component analysis (PCA) showed a significant separation of the liver fatty acid compositions between the control group and the groups with supplementation levels above 50 mg kg-1 (Figure 6).
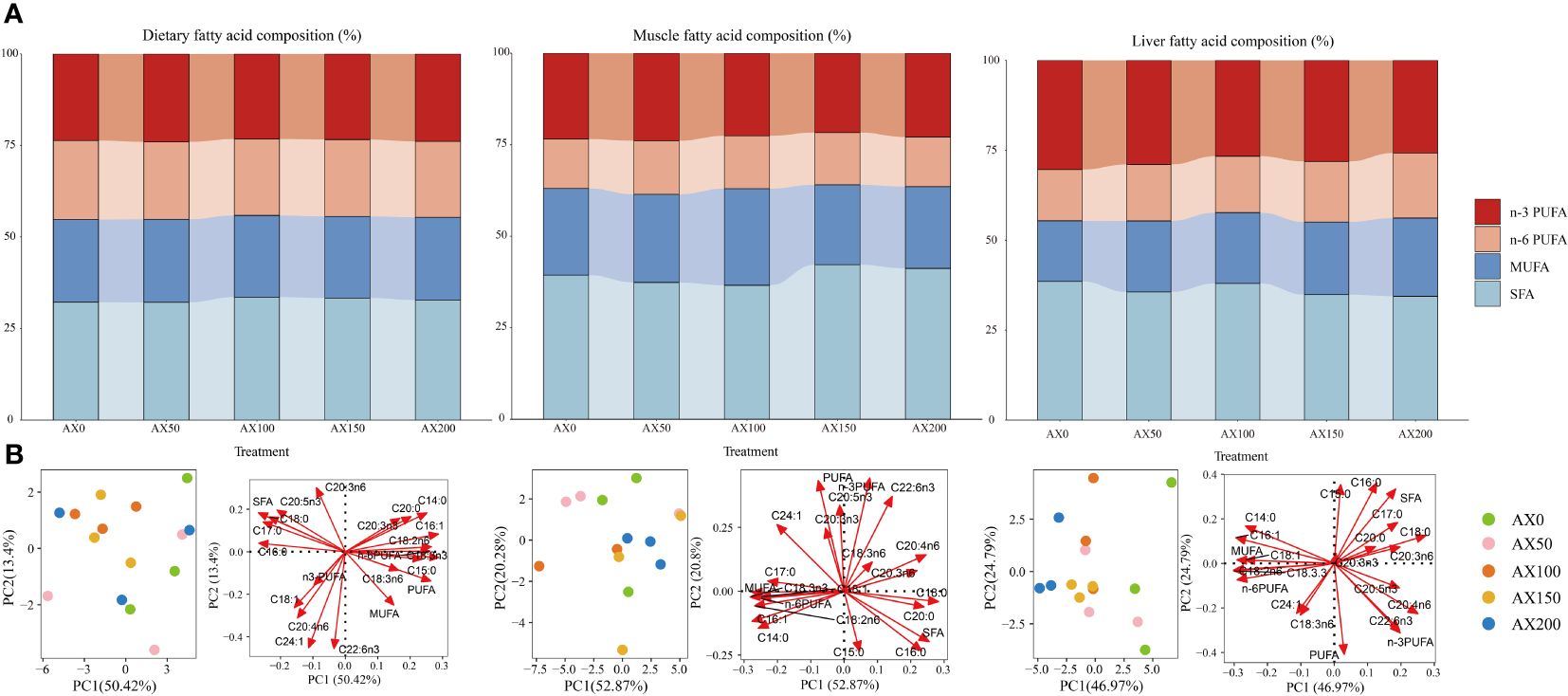
Figure 6 The fatty acid profile in the muscle, liver of largemouth bass and the experimental feeds. (A) Fatty acid composition (% of total fatty acids); (B) principal component analysis (PCA) score plot (left) and loading plot (right). SFA: saturated fatty acids. MUFA: monounsaturated fatty acids. PUFA: polyunsaturated fatty acids.
4 Discussion
The biological effects of astaxanthin on aquatic species, such as promoting growth, reproduction, pigmentation, and immune activation, have received increasing attention in recent years (Elbahnaswy and Elshopakey, 2024). In this study, it was found that the addition of astaxanthin can improve the growth performance of largemouth bass. Fish fed with diets containing 100 and 150 mg kg-1 astaxanthin exhibited significantly higher growth performances than those in the control group without astaxanthin supplementation. Chen et al. (2020) also confirmed that the diet supplemented with 100 mg/kg astaxanthin significantly improved the WGR and SGR values of loach (Paramisgurnus dabryanus), but the growth showed a downward trend when the addition level was higher. In addition, adding astaxanthin also improved the FCR and PER values of largemouth bass; these values in the AX150 group were significantly higher than those in the AX0 group. Similar results can be found in the report on rainbow trout (Oncorhynchus mykiss) (Zhao et al., 2022b). By analyzing the data on WGR and SGR using quadratic polynomial regression, the optimal supplementation level of astaxanthin in the diet of largemouth bass is between 134.8 and 135.75 mg/kg. This is consistent with studies on some species, such as 150 mg/kg for Asian seabass (Lates calcarifer) (Lim et al., 2019), and 100 mg/kg for swordtail fish (Xiphophorus helleri) (Rashidian et al., 2020). However, several studies also showed that the addition of astaxanthin has no effect on the growth performance of certain species, such as catfish (Lophiosilurus alexandri) (Costa et al., 2022) and coral trout (Plectropomus leopardus) (Zhu et al., 2022). According to Cheng et al. (2018), the variation may be caused by some factors such as the source of astaxanthin, species, fish size, and dietary palatability.
The examination of intestinal morphology is an effective method to evaluate the health status and digestive system function of fish (Han et al., 2015). Morphological changes, such as increased dimensions of villi, could expand the surface area, allowing for better nutrient absorption in the intestine (Lin et al., 2020). The thickness of the muscular layer is closely related to the peristalsis ability of the intestine, which plays a crucial role in promoting the passage of food through the intestine and completing digestion (Yu et al., 2020). The results indicated that astaxanthin can improve the intestinal development and enhance the digestion and absorption capacity of largemouth bass. Compared to other treatment groups, the length and thickness of villis in the AX100 and AX150 groups were significantly increased, and the thickness of muscle layer in the AX150 group was the highest among all groups. This may be one of the reasons why adding 100–150mg/kg astaxanthin resulted in the optimal FCR and PER values. It was reported that astaxanthin is beneficial for improving the intestinal morphology of rainbow trout, particularly by increasing villi length and thickness and muscular layer thickness (Zhao et al., 2022b). Similarly, adding astaxanthin led to an increase in the length of midgut villi in the pompano (Trachinotus ovatus) (Zhao et al., 2022a).
The antioxidant defense system is the primary defense mechanism of cells against oxidative stress. Some indicators, such as T-AOC, SOD, CAT, GSH-PX, and MDA, are important biomarkers for evaluating antioxidant activity in vivo. SOD and CAT directly participate in the elimination of reactive oxygen species, protecting the organism from oxidative damage (Zhang et al., 2013). MDA is an indirect sign of cellular damage caused by free radicals. T-AOC represents the total antioxidant capacity in the body (Ghosh et al., 2016). In this study, with the increase of astaxanthin levels in diets, the SOD, CAT, and T-AOC values in serum and liver all increased. And, the highest T-AOC value appeared in the AX150 group. Meanwhile, the MDA levels in both tissues showed a decreased trend with increasing dietary astaxanthin levels. Similar results can also be seen in blood parrot (Cichlasoma citrinellum × Cichlasoma synspilum) (Li et al., 2018). These results indicated that astaxanthin can reduce MDA content and enhance antioxidant capacity of largemouth bass.
The expression of antioxidant enzymes is predominantly regulated by Nrf2 gene (Kobayashi et al., 2006; Keum, 2012). Previous research on rainbow trout (Zhao et al., 2023) demonstrated that Nrf2 controls the transcriptional activity of antioxidant enzymes by binding to antioxidant response elements. In this study, the addition of astaxanthin to the diet resulted in a significant increase in Nrf2 gene expression. Combined with the results of antioxidant enzymes, it is suggested that astaxanthin may enhance cellular defense mechanisms by regulating the Nrf2 signaling pathway. Xie et al. (2019) also pointed out that astaxanthin can upregulate Nrf2 gene expression, improving the antioxidant capacity of the body and reducing the potential for apoptosis. In response to apoptotic signals, activation of endogenous apoptotic pathways can influence gene expression pertinent to anti-apoptotic and pro-apoptotic processes. Relevant studies showed that astaxanthin can activate the Nrf2/HO-1 (Heme Oxygenase-1) pathway, significantly inhibit cell apoptosis, and reduce the gene expression of Caspase-9 and Caspase-3 (Azita et al., 2021). The current research also indicated that dietary astaxanthin significantly reduced the expression of Caspase-9 and Caspase-3 gene in largemouth bass.
Astaxanthin not only enhances antioxidant capacity, but also has a substantial impact on fish immune function. Lysozyme, an alkaline enzyme, plays a crucial role in defense mechanisms by degrading and removing foreign substances (Liu et al., 2010). Some studies showed that astaxanthin can increase lysozyme activity in a variety of species, including large yellow croaker (Li et al., 2014) and snakehead (Channa argus) (Zhu et al., 2020). Similarly, serum lysozyme activity increased significantly as dietary astaxanthin levels increased in this study. AKP and ACP are essential for immune defense mechanisms as they can remove invasive pathogens and promote phagocytosis and degradation of foreign bodies (Zhao et al., 2018). AKP also plays a significant role in the growth of aquatic organisms by contributing to the assimilation, utilization of nutrients, and protein synthesis (Wu et al., 2021). In this research, the ACP activity was positively correlated with increasing levels of dietary astaxanthin. Furthermore, astaxanthin can modulate the immune responses of aquatic species by affecting the expression of key inflammatory cytokine (Xie et al., 2020). It was demonstrated that astaxanthin can reduce the production of apoptosis markers and inflammatory cytokines in fish, including Caspase-3, Caspase-9, IL-15, and TNF-α (Song et al., 2017). Unsurprisingly, dietary astaxanthin reduced the gene expression of TNF-α in largemouth bass.
Previous research showed that adding astaxanthin can affect the metabolism of fatty acids in fish (Kalinowski et al., 2019; Trine et al., 2021). It is worth noting that the contents of EPA in the muscle and liver of the largemouth bass are significantly lower than that of DHA. And, the percentage contents of EPA in muscle and liver are only half of that in feed. As the dose of astaxanthin in diets increased, the concentration of EPA in both tissues decreased significantly, while DHA levels remained relatively stable. Sargent et al. (2002) suggested that DHA was more important than EPA for fish growth and development. In addition, the unique properties of DHA structure can help it resist environmental stressors and reduce the risk of oxidative degradation. In this Study, increasing the dietary astaxanthin content led to a decrease in the levels of ARA and n-3PUFA in the liver. Similarly, Zhao et al. (2022b) reported that adding astaxanthin to the diet can inhibit the biosynthesis of n-3 unsaturated fatty acids in rainbow trout. Bell et al. (2000) suggested that the absence of fat-soluble antioxidants might trigger increased activity of fatty acid elongases and desaturases due to stimulation by peroxides, thereby enhancing PUFA synthesis. Long-chain polyunsaturated fatty acids (Lc-PUFA) are an important component of cell membranes and are highly susceptible to attacks from reactive oxygen species. The accumulation of Lc-PUFA oxidation products poses potential risks to cellular and organ function (Sies, 1997). Therefore, when dietary antioxidant levels are low, the increase in PUFA synthesis leads to an excessive accumulation of oxidation products, which is harmful to the organism itself (Nematipour and Gatlin, 1993). Astaxanthin supplementation may help prevent excessive oxidation and decomposition of Lc-PUFA, as high levels of MDA content in the liver and serum and high expression of Caspase-9 and Caspase-3 genes were found in the control group. These results indicate that astaxanthin supplementation can reduce excessive oxidation and decomposition of Lc-PUFA.
As is well known, Lc-PUFA plays a key role in regulating immune function by affecting cell membrane composition, signaling pathways, and related enzyme activities (Qi et al., 2022). There is ample evidence that certain polyunsaturated fatty acids, particularly ARA and EPA, are precursors to eicosanoid metabolites, including prostaglandins (PG), thromboxanes (TX), leukotrienes (LT), and lipoxins (LX). These metabolites play pivotal roles in modulating inflammation and immune responses in fish (Tocher, 2010; Pedro et al., 2021). Interestingly, the proportions of ARA and EPA in liver fatty acids in the groups with astaxanthin addition were lower compared with the control group without astaxanthin supplementation. Combined with the evaluation of non-specific immune parameters, it can be concluded that dietary astaxanthin can enhance the immune function of largemouth bass by regulating fatty acid metabolism. However, further research on this topic is warranted to clarify the underlying mechanisms controlling these interactions.
Cortisol is a hormone that helps organisms resist environmental stress (Donaldson, 1981). For instance, under stress conditions, fish will increase cortisol secretion through the hypothalamic-pituitary-adrenal (HPA) axis to maintain body homeostasis (Hsieh et al., 2003). However, prolonged elevated levels of cortisol can have adverse effects on immune function, leading to a decrease in lymphocyte and white blood cell count, and damage to immune organs such as the spleen and thymus (Valle et al., 1995; Demers and Bayne, 1997). This will make organisms susceptible to pathogen infections. In this study, fish fed diets containing astaxanthin had significantly lower blood cortisol levels than those in the control group. The results indicate that dietary astaxanthin can enhance stress resilience and mitigate stress-induced immunosuppression in largemouth bass. This is consistent with the study conducted on banded catfish (Liu et al., 2016).
5 Conclusion
Adding astaxanthin to the diet can not only improve growth performance, but also have a positive impact on intestinal structure of largemouth bass. Astaxanthin can enhance the activity of antioxidant enzymes in the liver and serum by regulating the Nrf2 signaling pathway, resulting in decreased cortisol and MDA levels in these tissues. Moreover, dietary astaxanthin can alter tissues fatty acid content and significantly boosts the immunity of largemouth bass. According to the results of a quadratic regression analysis of WGR and SGR, the optimal level of astaxanthin supplementation for largemouth bass feed is 134.8 ~ 135.75 mg/kg.
Data availability statement
The raw data supporting the conclusions of this article will be made available by the authors, without undue reservation.
Ethics statement
The animal study was approved by Institutional Animal Care and Use Committee of Zhejiang Ocean University. The study was conducted in accordance with the local legislation and institutional requirements.
Author contributions
JZ: Data curation, Formal analysis, Investigation, Methodology, Writing – original draft, Writing – review & editing. YY: Conceptualization, Investigation, Methodology, Writing – original draft. HX: Conceptualization, Data curation, Investigation, Methodology, Writing – review & editing. XL: Conceptualization, Data curation, Investigation, Methodology, Writing – review & editing. FD: Conceptualization, Data curation, Investigation, Methodology, Writing – review & editing. QC: Conceptualization, Investigation, Methodology, Writing – review & editing. TH: Conceptualization, Funding acquisition, Methodology, Project administration, Supervision, Writing – review & editing. JW: Conceptualization, Funding acquisition, Methodology, Project administration, Supervision, Writing – original draft, Writing – review & editing. CW: Conceptualization, Investigation, Methodology, Writing – review & editing.
Funding
The author(s) declare financial support was received for the research, authorship, and/or publication of this article. This work was supported by Major scientific and technological research projects of Zhoushan (2023C13017), Zhejiang Province “three agricultural six-party” science and technology collaboration program (2024SNJF059), The Key Research and Development Program of Zhejiang Province (2021C04016), National Natural Science Foundation of China (32273151, 32303023).
Conflict of interest
Author XL was employed by the company Guangdong Yuehai Feed Group Co., Ltd.
The remaining authors declare that the research was conducted in the absence of any commercial or financial relationships that could be construed as a potential conflict of interest.
Publisher’s note
All claims expressed in this article are solely those of the authors and do not necessarily represent those of their affiliated organizations, or those of the publisher, the editors and the reviewers. Any product that may be evaluated in this article, or claim that may be made by its manufacturer, is not guaranteed or endorsed by the publisher.
Supplementary material
The Supplementary Material for this article can be found online at: https://www.frontiersin.org/articles/10.3389/fmars.2024.1404661/full#supplementary-material
References
Ambati R., Moi P., Ravi S., Aswathanarayana R. (2014). Astaxanthin: sources, extraction, stability, biological activities and its commercial applications-A review. Mar. Drugs 12, 128–152. doi: 10.3390/md12010128
AOAC (1995). “Official methods of analysis of AOAC international,” in Official Analytical Chemists, 16th ed. Ed. Cunniff P. (AOAC International, Arlington, Virginia), pp.1141–1154.
Azita A., Fardin A., Morteza K., Hassan N., Mohammad A. G., Aligholi S. S. (2021). Astaxanthin relieves busulfan-induced oxidative apoptosis in cultured human spermatogonial stem cells by activating the Nrf-2/HO-1 pathway. Reprod. Sci. 29, 374–394. doi: 10.1007/s43032–021-00651-x
Bell J. G., McEvoy J., Tocher D. R., Sargent J. R. (2000). Depletion of α-tocopherol and astaxanthin in Atlantic salmon (Salmo salar) affects autoxidative defense and fatty acid metabolism. J. Nutr. 130, 1800–1808. doi: 10.1093/jn/130.7.1800
Chen X. M., Gao C. S., Du X. Y., Yao J. M., He F. F., Niu X. T., et al. (2020). Effects of dietary astaxanthin on the growth, innate immunity and antioxidant defence system of Paramisgurnus dabryanus. Aquac. Nutr. 26, 1453–1462. doi: 10.1111/anu.13093
Cheng C. H., Guo Z. X., Ye C. X., Wang A. L. (2018). Effect of dietary astaxanthin on the growth performance, non-specific immunity, and antioxidant capacity of pufferfish (Takifugu obscurus) under high temperature stress. Fish Physiol. Biochem. 44, 209–218. doi: 10.1007/s10695–017-0425–5
China Fishery Statistical Yearbook (2023). Compiled by Fishery Bureau of China Agriculture Department. (China Agriculture Press, Beijing) 25.
Chomczynski P., Sacchi N. (2006). The single-step method of RNA isolation by acid guanidinium thiocyanate-phenol-chloroform extraction: twenty-something years on. Nat. Protoc. 1, 581–585. doi: 10.1038/nprot.2006.83
Costa D. P. D., Silva M. J. S., Geraldi R. M., Lorenzini J. P., Mattioli C. C., Oliveira A. D. L., et al. (2022). Effects of the use of synthetic astaxanthin in the feeding of Lophiosilurus alexandri, a neotropical siluriform fish. Anais da Academia Bras. Cienc. 94, e20210434. doi: 10.1590/0001–3765202220210434
Demers N. E., Bayne C. J. (1997). The immediate effects of stress on hormones and plasma lysozyme in rainbow trout. Dev. Comp. Immunol. 21, 0–373. doi: 10.1016/S0145–305X(97)00009–8
Donaldson E. M. (1981). The pituitary-interrenal axis as an indicator of stress in fish. Stress Fish 93, 11–47.
Elbahnaswy S., Elshopakey G. E. (2024). Recent progress in practical applications of a potential carotenoid astaxanthin in aquaculture industry: a review. Fish Physiol. Biochem. 50, 97–126. doi: 10.1007/s10695–022-01167–0
El-Sayed A. F. M., Izquierdo M. (2021). The importance of vitamin E for farmed fish—A review. Rev. Aquac. 14, 688–703. doi: 10.1111/raq.12619
Ghosh K., De S., Das S., Mukherjee S., Sengupta Bandyopadhyay S. (2016). Withaferin A induces ROS-mediated paraptosis in human breast cancer cell-lines MCF-7 and MDA-MB-231. PloS One 11, e0168488. doi: 10.1371/journal.pone.0168488
Grant E. C., Philipp D. P., Inendino K. R., Goldberg T. L. (2003). Effects of temperature on the susceptibility of largemouth bass to largemouth bass virus. J. Aquat. Anim. Health 15, 215–220. doi: 10.1577/h03–009
Guo Z. R., Zhao Z., Zhang C., Jia Y. J., Qiu D. K., Zhu B., et al. (2020). Carbon nanotubes-loaded subunit vaccine can increase protective immunity against rhabdovirus infections of largemouth bass (Micropterus Salmoides). Fish Shellfish Immunol. 99, 548–554. doi: 10.1016/j.fsi.2020.02.055
Han B., Long W. Q., He J. Y., Liu Y. J., Si Y. Q., Tian L. X. (2015). Effects of dietary Bacillus licheniformis on growth performance, immunological parameters, intestinal morphology and resistance of juvenile Nile tilapia (Oreochromis niloticus) to challenge infections. Fish Shellfish Immunol. 46, 225–231. doi: 10.1016/j.fsi.2015.06.018
Hsieh S. L., Chen Y. N., Kuo C. M. (2003). Physiological responses, desaturase activity, and fatty acid composition in milkfish (Chanos chanos) under cold acclimation. Aquaculture 220, 903–918. doi: 10.1016/S0044–8486(02)00579–3
Jagruthi C., Yogeshwari G., Anbazahan S. M., Shanthi Mari L. S., Arockiaraj J., Mariappan P., et al. (2014). Effect of dietary astaxanthin against Aeromonas hydrophila infection in common carp, Cyprinus carpio. Fish Shellfish Immunol. 41, 674–680. doi: 10.1016/j.fsi.2014.10.010
Kalinowski C. T., Larroquet L., Véron V., Robaina L., Izquierdo M. S., Panserat S., et al. (2019). Influence of dietary astaxanthin on the hepatic oxidative stress response caused by episodic hyperoxia in rainbow trout. Antioxidants 8, 626. doi: 10.3390/antiox8120626
Keum Y. S. (2012). Regulation of Nrf2-mediated phase II detoxification and anti-oxidant genes. Biomol. Ther. 20, 144–151. doi: 10.4062/biomolther.2012.20.2.144
Kobayashi A., Kang M. I., Watai Y., Tong K. I., Shibata T., Uchida K., et al. (2006). Oxidative and electrophilic stresses activate Nrf2 through inhibition of ubiquitination activity of Keap1. Mol. Cell. Biol. 26, 221–229. doi: 10.1128/MCB.26.1.221–229.2006
Kou H. Y., Liu X. T., Hu J. R., Lin G., Zhang Y. F., Lin L. (2023). Impact of dietary zinc on the growth performance, histopathological analysis, antioxidant capability, and inflammatory response of largemouth bass Micropterus salmoides. Fish Shellfish Immunol. 141, 109025. doi: 10.1016/j.fsi.2023.109025
Li F., Huang S. Y., Lu X. X., Wang J., Cai M. G. (2018). Effects of dietary supplementation with algal astaxanthin on growth, pigmentation, and antioxidant capacity of the blood parrot (Cichlasoma citrinellum × Cichlasoma synspilum). J. Oceanol. Limnol. 36, 1851–1859. doi: 10.1007/s00343–019-7172–7
Li M., Wu W. J., Zhou P. ,. P., Xie F. J., Zhou Q. C., Mai K. S. (2014). Comparison effect of dietary astaxanthin and Haematococcus pluvialis on growth performance, antioxidant status and immune response of large yellow croaker Pseudosciaena crocea. Aquaculture 434, 227–232. doi: 10.1016/j.aquaculture.2014.08.022
Lim K. C., Yusoff F. M., Shariff M., Kamarudin M. S. (2019). Dietary administration of astaxanthin improves feed utilization, growth performance and survival of Asian seabass, Lates calcarifer (Bloch 1790). Aquac. Nutr. 25, 1410–1421. doi: 10.1111/anu.12961
Lin X. J., Tan A. P., Deng Y. T., Liu W. W., Zhao F., Huang Z. B. (2023). High occurrence of antibiotic resistance genes in intensive aquaculture of hybrid snakehead fish. Front. Mar. Sci. 9. doi: 10.3389/fmars.2022.1088176
Lin S. M., Zhou X. M., Zhou Y. L., Kuang W. M., Chen Y. J., Luo L., et al. (2020). Intestinal morphology, immunity and microbiota response to dietary fibers in largemouth bass, Micropterus salmoide. Fish Shellfish Immunol. 103, 135–142. doi: 10.1016/j.fsi.2020.04.070
Liu F., Shi H. Z., Guo Q. S., Yu Y. B., Wang A. M., Lv F., et al. (2016). Effects of astaxanthin and emodin on the growth, stress resistance and disease resistance of yellow catfish (Pelteobagrus fulvidraco). Fish Shellfish Immunol. 51, 125–135. doi: 10.1016/j.fsi.2016.02.020
Liu B., Xie J., Ge X. P., Xu P., Wang A. M., He Y. J., et al. (2010). Effects of anthraquinone extract from Rheum officinale Bail on the growth performance and physiological responses of Macrobrachium rosenbergii under high temperature stress. Fish Shellfish Immunol. 29, 49–57. doi: 10.1016/j.fsi.2010.02.018
Liu T., Xu H. Y., Han T., Wang J. T., Wang C. L. (2021). Effect of dietary egg yolk lecithin levels on survival, growth, lipid metabolism, and antioxidant capacity of early juvenile green mud crab Scylla paramamosain. Aquaculture 540, 736706. doi: 10.1016/j.aquaculture.2021.736706
Livak K. J., Schmittgen T. D. (2001). Analysis of relative gene expression data using real- time quantitative PCR and the 2-ΔΔCT method. Methods 25, 402–408. doi: 10.1006/meth.2001.1262
Martin H. D., JaGer C., Christoph R., Marcus S., Walsh R., Paust J. (1999). Anti- and prooxidant properties of carotenoids. Advanced Synthesis & Catalysis 341, 3, 302–308. doi: 10.1002/(sici)1521–3897(199904)341:3<302::aid-prac302>3.0.co;2–6
Naguib, Yousry M. A. (2000). Antioxidant activities of astaxanthin and related carotenoids. J. Agric. Food Chem. 48, 1150–1154. doi: 10.1021/jf991106k
Nematipour G. R., Gatlin D. M. (1993). Requirement of hybrid striped bass for dietary (n-3) highly unsaturated fatty acids. J. Nutr. 123, 744–753. doi: 10.1093/jn/123.4.744
Pedro A., Marit E., Eva L., Yang Y., Elisabeth H. (2021). Differential production of prostaglandins and prostacyclins by liver and head kidney cells from Atlantic salmon challenged with arachidonic and eicosapentaenoic acids. Fish Shellfish Immunol. Rep. 2, 100015. doi: 10.1016/j.fsirep.2021.100015
Qi H. Q., Liu Y., Jian F. J., Xing X., Wang J. H., Li C. (2022). Effects of dietary arachidonic acid (ARA) on immunity, growth and fatty acids of Apostichopus japonicus. Fish Shellfish Immunol. 127, 901–909. doi: 10.1016/j.fsi.2022.07.037
Rashidian G., Rainis S., Prokić M. D., Faggio C. (2020). Effects of different levels of carotenoids and light sources on swordtail fish (Xiphophorus helleri) growth, survival rate and reproductive parameters. Natural Prod. Res. 35, 3675–3686. doi: 10.1080/14786419.2020.1723091
Sargent J. R., Tocher D. R., Bell J. G. (2002). “The lipids,” in Fish Nutrition. Eds. Halver J. E., Hardy R. W.(San Diego, California: ACADEMIC PRESS), 182–246.
Sies H. (1997). Oxidative stress: oxidants and antioxidants. Exp. Physiol. 82, 291–295. doi: 10.1113/expphysiol.1997.sp004024
Song X. L., Wang L., Li X. Q., Chen Z. Z., Liang G. Y., Leng X. G. (2017). Dietary astaxanthin improved the body pigmentation and antioxidant function, but not the growth of discus fish (Symphysodon spp.). Aquac. Res. 48, 1359–1367. doi: 10.1111/are.13200
Tal B. H., Burge C. A., Bushek D., Groner M. L., Carnegie R. B. (2018). Intensive oyster aquaculture can reduce disease impacts on sympatric wild oysters. Aquac. Environ. Interact. 10, 557–567. doi: 10.3354/aei00290
Tan X., Sun Z., Huang Z., Zhou C., Lin H., Tan L., et al. (2017). Effects of dietary hawthorn extract on growth performance, immune responses, growth and immune-related genes expression of juvenile golden pompano (Trachinotus ovatus) and its susceptibility to Vibrio harveyi infection. Fish Shellfish Immunol. 70, 656. doi: 10.1016/j.fsi.2017.09.041
Tocher D. R. (2010). Metabolism and functions of lipids and fatty acids in teleost fish. Rev. Fish. Sci. 11, 107–184. doi: 10.1080/713610925
Todorčević M., Škugor S., Ruyter B. (2010). Alterations in oxidative stress status modulate terminal differentiation in Atlantic salmon adipocytes cultivated in media rich in n-3 fatty acids. Comp. Biochem. Physiol. Part B Biochem. Mol. Biol. 156, 309–318. doi: 10.1016/j.cbpb.2010.04.010
Trine Y. S. A., Bente R., Bjarne H., Tone K., Aleksei K. (2021). Transcriptome and functional responses to absence of astaxanthin in Atlantic salmon fed low marine diets. Comp. Biochem. Physiol. Part D Genomics Proteomics 39, 100841. doi: 10.1016/j.cbd.2021.100841
Valle G. D., Taniguchi N., Tsujimura A. (1995). Effects of stress on some hematological traits of chromosome manipulated Ayu, Plecoglossus altivelis. Suisanzoshoku 43, 89–95. doi: 10.11233/aquaculturesci1953.43.89
Wu P., Xu X. H., Yu T. (2021). Dietary watermelon residue influencing the nonspecific immunity of juvenile Pseudorasbora parva. Fish Shellfish Immunol. 118, 421–425. doi: 10.1016/j.fsi.2021.08.007
Xie J., Fang H., He X., Liao S., Liu Y., Tian L., et al. (2019). Study on mechanism of synthetic astaxanthin and Haematococcus pluvialis improving the growth performance and antioxidant capacity under acute hypoxia stress of golden pompano (Trachinotus ovatus) and enhancing anti-inflammatory by activating Nrf2-ARE pathway to antagonize the NF-κB pathway. Aquaculture. 518, 734657. doi: 10.1016/j.aquaculture.2019.734657
Xie J., Liu B., Zhou Q., Su Y. T., He Y. J., Pan L. K., et al. (2008). Effects of anthraquinone extract from rhubarb Rheum officinale Bail on the crowding stress response and growth of common carp Cyprinus carpio var. Jian. Aquac. 281, 5–11. doi: 10.1016/j.aquaculture.2008.03.038
Xie S. W., Yin P., Tian L. X., Yu Y. Y., Liu Y. J., Niu J. (2020). Dietary supplementation of astaxanthin improved the growth performance, antioxidant ability and immune response of juvenile largemouth bass (Micropterus salmoides) fed high-fat diet. Mar. Drugs 18 (12), 642. doi: 10.3390/md18120642
Yu L., Wen H., Jiang M., Wu F., Liu W. (2020). Effects of ferulic acid on intestinal enzyme activities, morphology, microbiome composition of genetically improved farmed tilapia (Oreochromis niloticus) fed oxidized fish oil. Aquaculture 528, 735543. doi: 10.1016/j.aquaculture.2020.735543
Yu L. L., Yu H. H., Liang X. F., Li N., Wang X., Li F. H., et al. (2018). Dietary butylated hydroxytoluene improves lipid metabolism, antioxidant and anti-apoptotic response of largemouth bass (Micropterus salmoides). Fish Shellfish Immunol. 72, 220–229. doi: 10.1016/j.fsi.2017.10.054
Zhang J., Liu Y. J., Tian L. X., Yang H. J., Liang G. Y., Yue Y. R., et al. (2013). Effects of dietary astaxanthin on growth, antioxidant capacity and gene expression in Pacific white shrimp Litopenaeus vannamei. Aquac. Nutr. 19, 917–927. doi: 10.1111/anu.12037
Zhao W., Cui X., Wang Z. Q., Yao R., Xie S. H., Gao B. Y., et al. (2022a). Beneficial Changes in Growth Performance, Antioxidant Capacity, Immune Response, Hepatic Health, and Flesh Quality of Trachinotus ovatus Fed With Oedocladium carolinianum. Front. Immunol. 13. doi: 10.3389/fimmu.2022.940929
Zhao W., Guo Y. C., Huai M. Y., Li L., Man C., Pelletier W., et al. (2022b). Comparison of the retention rates of synthetic and natural astaxanthin in feeds and their effects on pigmentation, growth, and health in rainbow trout (Oncorhynchus mykiss). Antioxidants 11 (12), 2473. doi: 10.3390/antiox11122473
Zhao X., Li L., Li C., Liu E., Zhu H., Ling Q. (2022c). Heat stress-induced endoplasmic reticulum stress promotes liver apoptosis in largemouth bass (Micropterus salmoides). Aquaculture 546, 737401. doi: 10.1016/j.aquaculture.2021.737401
Zhao J., Lin Y., Zhao Y. B., Wang Y. H., Ning C., Ma Y., et al. (2018). Polyphenol-rich blue honeysuckle extract alleviates silica-induced lung fibrosis by modulating Th immune response and NRF2/HO-1 MAPK signaling. J. Funct. Foods 46, 463–474. doi: 10.1016/j.jff.2018.05.024
Zhao W., Xie J. J., Fang H. H., Liu Y. J., Niu J. (2020). Effects of corn starch level on growth performance, antioxidant capacity, gut morphology and intestinal microflora of juvenile golden pompano, Trachinotus ovatus. Aquaculture 524, 735197. doi: 10.1016/j.aquaculture.2020.735197
Zhao W., Yao R., Wei H. L., Guo Y. C., Chen A. Q., Chen B. Y., et al. (2023). Astaxanthin, bile acid and chlorogenic acid attenuated the negative effects of high-fat diet on the growth, lipid deposition, and liver health of Oncorhynchus mykiss. Aquaculture 567, 739255. doi: 10.1016/j.aquaculture.2023.739255
Zhu X., Hao R., Zhang J., Tian C., Hong Y., Zhu C., et al. (2022). Dietary astaxanthin improves the antioxidant capacity, immunity and disease resistance of coral trout (Plectropomus leopardus). Fish Shellfish Immunol. 122, 38–47. doi: 10.1016/j.fsi.2022.01.037
Keywords: Micropterus salmoides, astaxanthin, fatty acid composition, antioxidant, immunity
Citation: Zhang J, Yang Y, Xu H, Li X, Dong F, Chen Q, Han T, Wang J and Wu C (2024) Effects of dietary astaxanthin on growth performance, immunity, and tissue composition in largemouth bass, Micropterus salmoides. Front. Mar. Sci. 11:1404661. doi: 10.3389/fmars.2024.1404661
Received: 21 March 2024; Accepted: 30 April 2024;
Published: 15 May 2024.
Edited by:
Lei Wang, Anhui Normal University, ChinaReviewed by:
Samad Rahimnejad, University of Murcia, SpainMichael Habte-Tsion, University of Maine, United States
Copyright © 2024 Zhang, Yang, Xu, Li, Dong, Chen, Han, Wang and Wu. This is an open-access article distributed under the terms of the Creative Commons Attribution License (CC BY). The use, distribution or reproduction in other forums is permitted, provided the original author(s) and the copyright owner(s) are credited and that the original publication in this journal is cited, in accordance with accepted academic practice. No use, distribution or reproduction is permitted which does not comply with these terms.
*Correspondence: Jiteng Wang, wangjiteng1971@gmail.com