Probing on growth and characterizations of SnFe2O4 epitaxial thin films on MgAl2O4 substrate
- 1Department of Chemistry, Pittsburg State University, Pittsburg, KS, USA
- 2Department of Mechanical Engineering, North Carolina A&T State University, Greensboro, NC, USA
- 3National Physical Laboratory (CSIR), New Delhi, India
- 4Department of Physics, Pittsburg State University, Pittsburg, KS, USA
Epitaxial tin ferrite (SnFe2O4) thin films were grown using KrF excimer (248 nm) pulsed laser deposition technique under different growth conditions. Highly epitaxial thin films were obtained at growth temperature of 650°C. The quality and epitaxial nature of the films were examined by X-ray diffraction technique. Furthermore, the phi-scans of the film and substrate exhibit fourfold symmetry, which indicates a cube-on-cube epitaxial growth of the film on MgAl2O4 substrate. Moreover, the magnetic force microscopy measurement shows domains with cluster-like structure, which is associated with ferromagnetic phase at room temperature. The coercive field and remnant magnetization of the films decrease with increase in temperature. These high quality ingenious magnetic films could be potentially used in data storage devices.
Introduction
Recently, spinel ferrites with the general formula MFe2O4 (where M = Co, Mn, Mg, Sn, etc.) attract considerable research interest because of their wide applications in heterogeneous catalyst, sensors, transformers, magnetic recording, biomedical, etc. (Abdeen, 1998; Sedlár et al., 2000; Bao et al., 2007; Barcena et al., 2008; Xiang et al., 2010). The ferrites can be classified into different categories depending upon their cation distributions. Based on the cations distribution among the tetrahedral and octahedral sites of the coordinated oxygen, they can be either normal spinel , or inverse spinel (Anantharaman et al., 1998).
Till date, most of the works on spinels have been reported on bulk in order to understand their magnetic behavior and correlate magnetic properties to their structural properties to improve their applications (Lüders et al., 2005). Epitaxial thin films of spinels have not drawn such a wide research attention despite of the fact that epitaxial films could modify the physical properties compared to the bulk material (Lüders et al., 2005). Epitaxial thin films of various ferrites have been grown using different techniques (Zimnol et al., 1997; Reisinger et al., 2003; Huang et al., 2007; Leung et al., 2008; Su et al., 2010). Among these techniques, pulsed laser deposition (PLD) is a very versatile and cost effective method which allows the stoichiometry transfer of multi-component materials from target to substrate (Green et al., 1995).
Pulsed laser deposition technique has been used for deposition of epitaxial thin films of magnesium ferrite on strontium titanate (Kim et al., 2010). The effect of post-annealing on the magnetic properties of epitaxial thin films of cobalt ferrite was studied (Axelsson et al., 2009). Nanostructured tin ferrites have been synthesized using different techniques (Liu et al., 2004; Liu and Li, 2005). It was also observed that the coercivity of the tin ferrite particles decreases with increase in the particle size (Liu et al., 2004). Superparamagnetic behavior was observed for nanostructured tin ferrite (Liu and Li, 2005). PLD has been used to deposit (111) oriented epitaxial tin ferrite films on (0001) sapphire substrate (Gupta et al., 2011). In this communication, we report the epitaxial growth of tin ferrite films on (001) MgAl2O4 substrate using PLD technique. The quality and epitaxial nature of the films were evaluated by X-ray diffraction (XRD) diffraction technique. Magnetic domains with cluster-like structure were observed in the magnetic force microscopy (MFM) image of the film.
Experiments
SnFe2O4 target for PLD was made using solid state reaction method. SnO2 (99.9%, Alfa Aesar, USA) and Fe2O3 (99.5%, Alfa Aesar, USA) were used as received. The well-ground mixture was heated at 1200°C for 10 h. The powder mixture was cold pressed at 6 × 106 N/m2 load and sintered at 1400°C for 10 h. The films were deposited using KrF excimer PLD technique (Lambda Physik COMPex, λ = 248 nm and pulsed duration of 20 ns) at different substrate temperatures (550, 600, 650, and 690°C) under oxygen pressure of 0.1 mbar. The laser was operated at a pulse rate of 10 Hz, with energy of 300 mJ/pulse. The laser beam was focused onto a rotating target at a 45° angle of incidence. The target to substrate distance was 5 cm. Single crystal of (001) oriented MgAl2O4 was used as substrate. The substrate was ultrasonically cleaned in acetone and isopropanol for 10 min in each solvent.
The structural characterizations were performed using XRD. The XRD pattern of the films were recorded with Bruker AXS X-ray diffractometer using the 2θ– θ scan, rocking curve, and phi-scan with CuKα1(λ = 1.5406 Å) radiation which operated at 40 kV and 40 mA. The XRD measurements were performed using 0.1 mm aperture of the slits. The instrument broadening was corrected using LaB6 as an instrumental broadening standard. MFM imaging was performed under ambient conditions using a Digital Instruments (Veeco) Dimension-3100 unit with Nanoscope® III controller, operated in tapping mode. Magnetic measurements were performed on Quantum Design vibrating sample magnetometer (VSM). The optical transmittance measurements were made using UV–visible spectrophotometer (Ocean Optics HR4000).
Results and Discussion
The epitaxial nature of the films was investigated by XRD technique. The different scans such as θ–2θ, rocking (ω) curve, and phi (ϕ)-scans were used to study the quality and epitaxy of the films on (001) oriented MgAl2O4 substrate. Gupta and Yakuphanoglu (2011) and Gupta et al. (2011) have used sapphire and SrTiO3 as substrate for epitaxial growth of SnFe2O4. In the present study, MgAl2O4 was chosen as substrate since both film and substrate have cubic crystal structure with small lattice mismatch (~3.8%). All the films grown at different temperatures showed preferred orientation along (002) direction. Figure 1 shows the θ–2θ and rocking curve for (002) peak for the film grown at 650°C. It is seen in the XRD pattern that only one peak oriented along (002) direction is observed, indicating the epitaxial nature of the film along (002) direction. The epitaxial nature of the film is due to the close lattice parameters of film and substrate as both SnFe2O4 (face-centered cubic, a = 0.842 nm) and MgAl2O4 (cubic, a = 0.808 nm) exhibit cubic symmetry (space-group Fd3m). The full width at half maximum (FWHM) of (002) peak was estimated using the rocking curve. The FWHM was calculated to be 0.42°, 0.42°, 0.39°, and 0.44° for the films grown at 550, 600, 650, and 690°C, respectively. The lowest FWHM was observed for film grown at 650°C, indicating highly quality of the film. The FWHM was for SnFe2O4 film grown on SrTiO3 substrate was reported to be 0.96°, 0.94°, 0.56°, and 0.96° for the films grown at 550, 600, 650, and 690°C, respectively (Gupta and Yakuphanoglu, 2011). As observed, the FWHM for the SnFe2O4 films grown on MgAl2O4 are better than that on SrTiO3, which is due to the close lattice match of SnFe2O4 and MgAl2O4. Although the lattice mismatch between the substrate and films is about 3.8%, the film shows strain of about 1.4%. We consider this high quality film for further characterizations. The phi (ϕ)-scan of the film and substrate was recorded using (311) reflection plane (2θ = 34.28 and ψ = 25.24) and is shown in Figure 2. The phi-scan of the film and substrate revealed fourfold symmetry for both. The phi-scan shows a cube-on-cube epitaxial growth of SnFe2O4 on MgAl2O4 substrate.
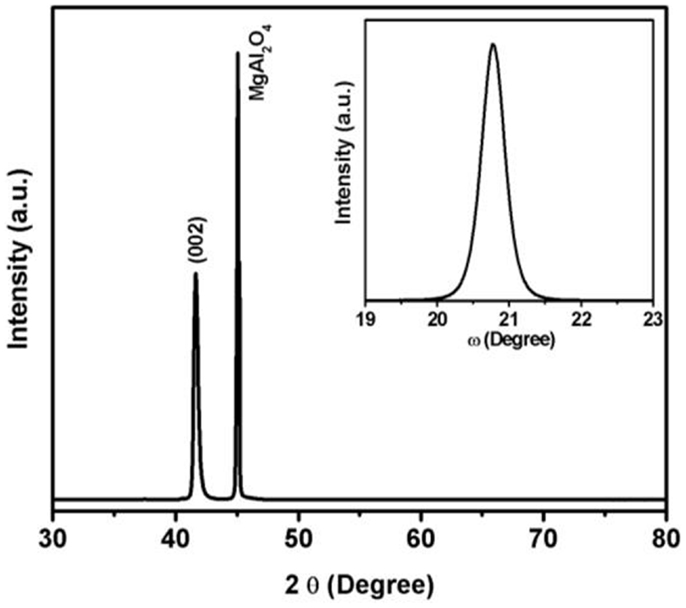
Figure 1. X-ray diffraction patterns of SnFe2O4 film grown at 650°C (inset figure shows the rocking curve of the film).
Figure 3 shows the MFM image of the film recorded in the demagnetized state. The presence of magnetic domain due to grains of SnFe2O4 is quite evident in the MFM image. The grain size of the SnFe2O4 films was estimated to be 22 nm using (002) peak of XRD pattern (Gupta et al., 2011). The size of the magnetic domain was observed to be about 200 nm, indicating that about 10 grains make a domain. As seen in Figure 3, the magnetic image consists of domains with cluster-like structure where the magnetization is confined up and down with light and dark color, respectively.
The optical properties such as transparency and optical bandgap of the epitaxially grown tin ferrite were studied. Figure 4 shows the optical transmittance spectra of the film. The optical bandgap of the film was calculated from absorption coefficient and photon energy. The absorption coefficient (α) of the film was calculated using the following expression (Gupta et al., 2009)
where T is transmittance and d is film thickness. The optical bandgap of the films was calculated using the following equation (Dolia et al., 2006)
where A and Eg are constant and optical bandgap, respectively. The Eg can be determined by extrapolations of the linear regions of the plots to zero absorption. Inset of Figure 3 shows (αhν)2 vs. hν plot for the film. The bandgap of the film was calculated to be 2.8 eV. A bandgap of 2.7 eV is observed for tin ferrite film grown on sapphire substrate (Gupta et al., 2011). Dolia et al. (2006) have observed a bandgap of 2.5 eV for nickel ferrite film, whereas the bandgap of 2.7 eV was reported for zinc ferrite film (Wu et al., 2001).
The magnetic properties of the film were studied under different conditions. Figure 5 shows the variation of magnetization with temperature (M vs. T). As seen in Figure 5, the effect of temperature on magnetization was studied in zero-field-cooled (ZFC) and field-cooled (FC) process under different applied magnetic fields. For the ZFC measurements, the film was cooled from high temperature to 10 K without applying any external magnetic field. After cooling to 10 K, an external magnetic field was applied and the magnetization of the film was recorded during the heating. For FC measurements, the magnetization is recorded while cooling the sample under an applied external magnetic field. As seen in Figure 5, during ZFC measurements the magnetization of the film increases with temperature up to ~275 K and then decreases with further increase in temperature. The nature of ZFC and FC curves is very similar but the magnitude of magnetization for FC curves is high. Similar results were observed for M vs. T process under high magnetic field. Furthermore, it should be noted that there is a distinct irreversibility between the ZFC and FC magnetization curves. This irreversibility persists up to high temperature of 375 K. Similar nature in M vs. T has been observed for the SnFe2O4 films grown on SrTiO3 and sapphire substrate (Gupta and Yakuphanoglu, 2011; Gupta et al., 2011). The magnetization at 10 K in FC measurement was observed to be 12.9, 14.2 and 67.6 emu/cm3 for the SnFe2O4 film grown on MgAl2O4, SrTiO3, and sapphire substrate, respectively. Although the maximum magnetization was observed on sapphire substrate, the difference in the values of magnetization measured during ZFC and FC at 10 K was almost constant (~10 emu/cm3) for the SnFe2O4 films on MgAl2O4, SrTiO3, and sapphire substrate. The different values of magnetization for SnFe2O4 films on different substrates could be due to strain introduced by lattice mismatch of film and MgAl2O4, SrTiO3, and sapphire substrates (Belenky et al., 2005). The lattice mismatch between SnFe2O4 and MgAl2O4, SrTiO3, and sapphire was estimated to be 3.8, 7.3, and 8.4%, respectively. The strain introduced by lattice mismatch is an important parameter contributing to magnetic properties such as Curie temperature, coercivity, saturation magnetization, and anisotropy (Rao et al., 1998).
Figure 6 shows the variation of magnetization with applied magnetic field (M vs. H) at different temperatures. The M vs. H plots were measured at 10 and 300 K. The open hysteresis loop near origin at room temperature confirms the ferromagnetic nature of the film. It is observed that the coercive field and remnant magnetization of the film decrease with increase in the temperature. The coercive field of 4575 and 431 Oe is observed at 10 and 300 K, respectively. On the other hand, the value of remnant magnetization of 25.2 emu/cm3 and 8.3 emu/cm3 is observed at 10 and 300 K, respectively. The coercive field of 4861 and 1323 Oe was reported for SnFe2O4 film on sapphire substrate at 10 and 300 K, respectively (Gupta et al., 2011). On the other hand, the coercive field of 1853 and 801 Oe was observed for SnFe2O4 film on SrTiO3 substrate at 10 and 300 K, respectively (Gupta and Yakuphanoglu, 2011). Again the difference in the remnant magnetization and coercive field for SnFe2O4 films on MgAl2O4, SrTiO3, and sapphire substrates could be due to lattice mismatch between the film and substrates. The structural and magnetic characterizations of SnFe2O4 film on different substrates indicate that the properties of the film can be modified by using different substrates.
Conclusion
We have successfully demonstrated the deposition of epitaxial tin ferrite thin films on MgAl2O4 substrate using PLD technique. XRD measurements confirm the epitaxial nature of the tin ferrite film. The phi-scan of the film and substrate shows fourfold symmetry, which evidenced the cube-on-cube epitaxial growth of tin ferrite on MgAl2O4 substrate. The optical bandgap of the film was observed to be 2.8 eV. Furthermore, the magnetic measurements exhibit the ferromagnetic nature of the film at room temperature. These epitaxial, transparent, and ferromagnetic films could be potentially used in the next generation data storage devices.
Conflict of Interest Statement
The authors declare that the research was conducted in the absence of any commercial or financial relationships that could be construed as a potential conflict of interest.
References
Abdeen, A. M. (1998). Electric conduction in Ni–Zn ferrites. J. Magn. Magn. Mater. 185, 199–206. doi: 10.1016/S0304-8853(97)01144-X
Anantharaman, M. R., Jagatheesan, S., Malini, K. A., Sindhu, S., Narayanasamy, A., Chinnasamy, C. N., et al. (1998). On the magnetic properties of ultra-fine zinc ferrites. J. Magn. Magn. Mater. 189, 83–88. doi:10.1016/S0304-8853(98)00171-1
Axelsson, A.-K., Valant, M., Fenner, L., Wills, A. S., and Alford, N. M. (2009). Chemistry of post-annealing of epitaxial CoFe2O4 thin films. Thin Solid Films 517, 3742–3747. doi:10.1016/j.tsf.2009.01.142
Bao, N., Shen, L., Wang, Y., Padhan, P., and Gupta, A. (2007). A facile thermolysis route to monodisperse ferrite nanocrystals. J. Am. Chem. Soc. 129, 12374–12375. doi:10.1021/ja074458d
Barcena, C., Sra, A. K., Chaubey, G. S., Khemtong, C., Liu, J. P., and Gao, J. (2008). Zinc ferrite nanoparticles as MRI contrast agents. Chem. Commun. 2224–2226. doi:10.1039/B801041B
Belenky, L. J., Ke, X., Rzchowski, M., and Eom, C. B. (2005). Epitaxial La0.67Sr0.33MnO3/La0.67Ba0.33MnO3 superlattices. J. Appl. Phys. 97, 10J107. doi:10.1063/1.1850384
Dolia, S. N., Sharma, R., Sharma, M. P., and Saxena, N. S. (2006). Synthesis, X-ray diffraction and optical band gap study of nanoparticles of NiFe2O4. Indian J. Pure Appl. Phys. 44, 774–776.
Green, S. M., Pique, A., and Harshavardhan, K. S. (1995). Pulsed Laser Deposition of Thin Films. New York: Wiley.
Gupta, R. K., Ghosh, K., and Kahol, P. K. (2011). Structural and magnetic properties of epitaxial SnFe2O4 thin films. Mater. Letters 65, 2149–2151. doi:10.1016/j.matlet.2011.04.059
Gupta, R. K., Ghosh, K., Patel, R., and Kahol, P. K. (2009). Highly conducting and transparent Ti-doped CdO films by pulsed laser deposition. Appl. Surf. Sci. 255, 6252–6255. doi:10.1016/j.apsusc.2009.01.091
Gupta, R. K., and Yakuphanoglu, F. (2011). Epitaxial growth of tin ferrite thin films using pulsed laser deposition technique. J. Alloys Comp. 509, 9523–9527. doi:10.1016/j.jallcom.2011.07.058
Huang, W., Zhou, L. X., Zeng, H. Z., Wei, X. H., Zhu, J., Zhang, Y., et al. (2007). Epitaxial growth of the CoFe2O4 film on SrTiO3 and its characterization. J. Cryst. Growth 300, 426–430. doi:10.1016/j.jcrysgro.2007.01.004
Kim, K. S., Muralidharan, P., Han, S. H., Kim, J. S., Kim, H. G., and Cheon, C. I. (2010). Influence of oxygen partial pressure on the epitaxial MgFe2O4 thin films deposited on SrTiO3 substrate. J. Alloys Comp. 503, 460–463. doi:10.1016/j.jallcom.2010.05.033
Leung, G. W., Vickers, M. E., Yu, R., and Blamire, M. G. (2008). Epitaxial growth of Fe3O4 on SrTiO3 substrates. J. Cryst. Growth 310, 5282–5286. doi:10.1016/j.jcrysgro.2008.07.126
Liu, F., Li, T., and Zheng, H. (2004). Structure and magnetic properties of SnFe2O4 nanoparticles. Phys. Letters A 323, 305–309. doi:10.1016/j.physleta.2004.01.077
Liu, F. X., and Li, T. Z. (2005). Synthesis and magnetic properties of SnFe2O4 nanoparticles. Mater. Letters 59, 194–196. doi:10.1016/j.matlet.2004.09.028
Lüders, U., Bibes, M., Bobo, J.-F., Cantoni, M., Bertacco, R., and Fontcuberta, J. (2005). Enhanced magnetic moment and conductive behavior in NiFe2O4 spinel ultrathin films. Phys. Rev. B 71, 134419. doi:10.1103/PhysRevB.71.134419
Rao, R. A., Lavric, D., Nath, T. K., Eom, C. B., Wu, L., and Tsui, F. (1998). Three-dimensional strain states and crystallographic domain structures of epitaxial colossal magnetoresistive La0.8Ca0.2MnO3 thin films. Appl. Phys. Lett. 73, 3294–3296. doi:10.1063/1.122749
Reisinger, D., Schonecke, M., Brenninger, T., Opel, M., Erb, A., Alff, L., et al. (2003). Epitaxy of Fe3O4 on Si(001) by pulsed laser deposition using a TiN/MgO buffer layer. J. Appl. Phys. 94, 1857–1863. doi:10.1063/1.1587885
Sedlár, M., Matejec, V., Grygar, T., and Kadlecová, J. (2000). Sol–gel processing and magnetic properties of nickel zinc ferrite thick films. Ceramics Int. 26, 507–512. doi:10.1016/S0272-8842(99)00086-3
Su, H.-C., Dai, J.-Y., Liao, Y.-F., Wu, Y.-H., Huang, J. C. A., and Lee, C.-H. (2010). The preparation of Zn-ferrite epitaxial thin film from epitaxial Fe3O4:ZnO multilayers by ion beam sputtering deposition. Thin Solid Films 518, 7275–7278. doi:10.1016/j.tsf.2010.04.089
Wu, Z., Okuya, M., and Kaneko, S. (2001). Spray pyrolysis deposition of zinc ferrite films from metal nitrates solutions. Thin Solid Films 385, 109–114. doi:10.1016/S0040-6090(00)01906-4
Xiang, X., Fan, G., Fan, J., and Li, F. (2010). Porous and superparamagnetic magnesium ferrite film fabricated via a precursor route. J. Alloys Comp. 499, 30–34. doi:10.1016/j.jallcom.2010.03.125
Keywords: epitaxial thin films, SnFe2O4, pulse laser deposition, ferromagnetic, bandgap
Citation: Gupta RK, Candler J, Kumar D, Gupta BK and Kahol PK (2014) Probing on growth and characterizations of SnFe2O4 epitaxial thin films on MgAl2O4 substrate. Front. Mater. 1:10. doi: 10.3389/fmats.2014.00010
Received: 20 June 2014; Paper pending published: 07 July 2014;
Accepted: 16 July 2014; Published online: 06 August 2014.
Edited by:
Ibrahim Sayed Hussein, Ain Shams University, EgyptCopyright: © 2014 Gupta, Candler, Kumar, Gupta and Kahol. This is an open-access article distributed under the terms of the Creative Commons Attribution License (CC BY). The use, distribution or reproduction in other forums is permitted, provided the original author(s) or licensor are credited and that the original publication in this journal is cited, in accordance with accepted academic practice. No use, distribution or reproduction is permitted which does not comply with these terms.
*Correspondence: Ram K. Gupta, Department of Chemistry, Pittsburg State University, 1701 South Broadway, Pittsburg, KS-66762, USA e-mail: ramguptamsu@gmail.com