Optimal Synthesis and Evaluation of Tri-Amine Modified Ordered Mesoporous Carbon (TriFeOMC) and Its Application for the Adsorption of Arsenic and Lead From Aqueous Solution
- 1Key Laboratory for Integrated Regulation and Resources Exploitation on Shallow Lakes, Ministry of Education, Hohai University, Nanjing, China
- 2College of Environment, Hohai University, Nanjing, China
- 3State Key Laboratory of Lake Science and Environment, Nanjing Institute of Geography and Limnology, Chinese Academy of Science, Nanjing, China
- 4Civil Technology Department, The University of Lahore, Lahore, Pakistan
- 5College of Agricultural Engineering, Hohai University, Nanjing, China
Heavy metal pollution has become a serious problem due to increasing industrial waste, causing damage to water ecological environment, economic development and human health through their toxic effects. A unique Triamine-Iron-ordered mesoporous carbon (TriFeOMC) material was produced via a soft template approach. The ensuing sample of TriFeOMC was tested for the adsorption of arsenic (As) and Lead (Pb) along with its comparison with other two materials, which are Ordered mesoporous carbon (OMC) and Iron-ordered mesoporous carbon (FeOMC). The adsorption efficiency of TriFeOMC was excellent (92%) for the adsorption of As whereas, OMC has shown higher efficiency (88%) for the adsorption of Pb. The interaction between amino group and acidic groups on the surface of carbon formed a strong layer during Pb ions adsorption process, which resulted decreased adsorption of Pb on TriFeOMC. The Langmuir model fit for OMC, while Freundlich model fit for FeOMC. Both models fit for TriFeOMC for As whereas, Langmuir model showed comparatively better fit for Pb. The adsorption process followed an almost similar way of both pseudo models for Arsenic and Lead adsorption for OMC, FeOMC, and TriFeOMC and the adsorption process has been followed by pseudo-second-order for the adsorption of Pb. The novel material TriFeOMC with large pore volume and large surface area showed excellent results for the adsorption of arsenic from the aqueous solution. The results show the highest efficiency of TriFeOMC for adsorption of arsenic from arsenic-polluted water due to higher adsorption capacity with total pore volume 1.70 to 300.00 nm along with highly suitable pore width of 0.0680 cm3/g for adsorption, and therefore, it is highly recommended to use TriFeOMC for the adsorption of arsenic from polluted water.
Introduction
Over the past few decades, industrialization and urbanization posed severe threat to the surface and ground waters through polluting them with toxic heavy metals. Arsenic (As) and Lead (Pb) contamination is a global environmental issue (Hu et al., 2017; Mohan et al., 2017). Arsenic has carcinogenic and it has toxic effects on humans and other organisms. The global emissions and cycling of As is expected to increase due to increased industrial applications of As as pesticides and so on (Mandal and Suzuki, 2002; Sun et al., 2009; Hu et al., 2017). Lead has the ability to imitate zinc, iron and calcium due to which it has been considered as disaster for living organisms. Lead effects on human beings are related to certain types of disorders which includes carcinogenic, genotoxic, reproductive, and neurological defects (Kumar et al., 2014; Mohan et al., 2017). Therefore, removal of As and Pb from water is one of the major environmental problems.
Adsorption is being considered as a favorable technological adaptation for the treatment of the heavy metals. Adsorption is a comparatively simple, cost-effective, eco-friendly (Alqadami et al., 2016; Li et al., 2018; Xiang et al., 2019) and versatile method as compared to other methods such as reverse osmosis, biological treatment, or coagulation (Ning, 2002; Song et al., 2006; Ma and Zhang, 2008). The mesocarbon materials have been considered as most sophisticated materials having large pore volumes, regular pore structure, high specific surface areas, and are highly stable mechanically (Lee et al., 2002; Hamad et al., 2016). The adsorption mechanisms of heavy metals are complex due to a variety of structural complexions, electrostatic attractions, and adsorption. The studies showed the extensive use of ordered mesoporous carbon (OMC) materials for heavy metals and organic materials removal from drinking waters (Zhang et al., 2009; Wang et al., 2012; Ding et al., 2014).
The OMC materials has been recognized as the promising nanomaterials for water treatment applications due to high surface area, large pore size and volume, and adjustable meso-structure with excellent thermal stability (Liu et al., 2006; Wan et al., 2008; Zhu et al., 2014; Qiu et al., 2015). The advanced recent techniques for their synthesis and manipulations with higher availability and low cost, offers new opportunities for such materials to be used for environmental applications (Zhang et al., 2005; Xue et al., 2009). The OMCs and modified OMCs has been proven very efficient for adsorption of bio-chemical pollutants (Szabó et al., 2006; Yang et al., 2010), and heavy metals (Zhang et al., 2009; Wang et al., 2012; Yadaei et al., 2018) from drinking and wastewater (Zhao et al., 1998; Vinu et al., 2007; Zhu et al., 2009; Wu et al., 2010; Hamad et al., 2016). The amine functionalized OMC possesses the high surface area, ordered structure, and interconnected large mesopores (Teng et al., 2015). There are two types of templates which include the hard (Lee et al., 2002) and soft templates (Liang et al., 2008; Mayes et al., 2010) being used for the production of mesocarbons. The structure of mesoporous materials can be adjusted using the optimization process. There are two types of soft templates to adjust the pore size of mesoporous carbons known as Triblock copolymers Pluronic F-127 and P-123 (Lee et al., 2002; Liu et al., 2006; Zhang et al., 2006). As discussed above, previous studies on adsorption of heavy metals on mesoporous carbons have suggested that mesoporous carbon has good efficiency as an adsorbent for the adsorption of heavy metals from the aqueous solution. However, the modified mesocarbons application and their efficiency for heavy metals removal are still needed to investigate. Especially, the modification of mesocarbon materials with organic compounds is a challenge itself.
The ordered mesoporous silica modifications with amine group has been well documented (Zeleňák et al., 2008; Chew et al., 2010; Wang et al., 2015) but there has not been a strong study supporting the modified OMC materials modification with amine group. Titirici et al. (2007) performed the one step modification of mesoporous carbon with primary amine group using SBA-15 silica template and results showed the excellent structural properties of the amine modified carbon materials. Teng et al. (2015) developed amino-functionalized ordered mesoporous material, which had also been found with excellent material structural properties.
The objective of the current study was to prepare highly sophisticated modified mesocarbon materials for the adsorption of heavy metals (Arsenic and Lead) from the aqueous solution. Also, to evaluate the performance of modified mesocarbon materials for adsorption of As and Pb as compared to mesocarbons from water, including the adsorption isotherms and adsorption kinetics. The experiments were conducted to estimate the adsorption behavior, mechanisms, and efficiencies of all materials. The results of synthetically optimized adsorption were compared to conclude.
Materials and Methods
Materials Used for the Experiment
The material used as a soft template (triblock copolymer Pluronic F127) and formaldehyde solution with % of weight 36.5–38 were bought from Sigma-Aldrich. Other materials including phenol, HF, AR grade ethanol, HCl, and NaOH were obtained from Nanjing the preferred chemical Co., Ltd., Nanjing, China. The above-mentioned materials were used directly without any further sanitization.
Preparation of Resol Precursors
Using the base catalytic process, phenol soluble resin resol was produced by phenol and formaldehyde solution. A NaOH aqueous solution (1.3 g with a weight of 20%) was added to the molten phenol (6.10 g at the melting temperature of 40°C). The resulting solution was mixed with formaldehyde solution (10 g) and stirred at 70°C temperature for 1 h. The materials obtained were normalized by temperature reduction at 25°C, and the pH value of 7 was maintained by HCl (0.6 mol). Vacuum furnace technology had been used to remove the water from the material. To obtain the ethanol solution synthesized from phenolic resin RESOL (weight 20%), the synthesized material was dissolved in ethanol.
Preparation of Mesoporous Carbon Material
As a carbon resource, phenolic resin RESOL solution is used to synthesize mesoporous carbon and was added in ethanol solution with Triblock F-127 copolymer as a template. The mass ratio of 1:1 of synthetic phenol and F127 resin has been used (Meng et al., 2006). When preparing the solution, ethanol (19.0 g) and F127 (1.0 g) are added to the phenolic Resin RESOL solution (5.0 g weighing 20%) and stirred to obtain a standardized solution (Liu et al., 2006; Ding et al., 2014). Ethanol evaporated from the synthetic material by using the combustion furnace at temperatures from 90 to 110°C (Ding et al., 2015). After the ethanol evaporates, a transparent layer was obtained for calcination. Quartz containers with a nitrogen atmosphere and with a flow rate of 70–100 cm3/min are applied to synthetic materials. Triblock copolymer template has been removed by using a tubular boiler with the temperature maintained at 400°C for 6 h. The materials were carbonized at temperature up to 900°C for 3 h with up to 3°C/min heating rate after calcination. After the completion of calcination and removal of Triblock copolymer template, the resultant product was cleaned by washing and drying (Yuan et al., 2013). The resulting carbon material obtained after removal of the template was powdered through crushing before use with 0.2 and 0.5 mm controlled particle diameter.
For the preparation of FeOMC, initially, the mixture was prepared by using 1.0 g as-synthesized OMC. The solution was prepared in alcohol (10 mL) by adding oxalic acid (0.05 g), and Fe(NO3)3.9H2O (0.721 g) and 1.0 g of OMC was then added into it along with drop-wise addition of furfuryl alcohol (2 mL). The resultant solution was dried by heating for 10 h at 90°C and calcinated at 900°C for 2 h. The powdered FeOMC was dissolved in NaOH solution (2.0 mol/L) with the temperature set at 90°C and processed for washing and drying.
For the preparation of TriFeOMC, firstly, 30 mL of toluene was dispersed on 1.0 g dried (at 100°C for an hour) FeOMC. The mixture was placed in an oil bath with 85°C fixed temperature by using a flask. Secondly, triamine (3 mL) was mixed using reflux in the flask of mixture up to 20 h. The toluene was used to wash the filtered mixture of TriFeOMC. The washed TriFeOMC solid was vacuum dried at 50°C for 2 h.
Characterization of the Materials
The nitrogen sorption method was used to determine the characteristics of OMC, FeOMC, and TriFeOMC which includes porosity and surface area, using surface area analyzer (ASAP2020). The pore width distribution and pore volume, and pore size distribution were determined by the Brunauer–Emmett–Teller (BET) method and Kelvin Eq. (1), respectively:
Whereas, Vm, α, G, and T are molar volumes, surface tension, the universal gas constant, and the absolute temperature of the adsorbate, respectively (Kruk et al., 1997; Ding et al., 2015).
The following equation has been used to determine the pore volume:
Whereas, A(BET, r), and Vr (p/p0) are the BET specific surface area and the adsorption isotherm, respectively (Kruk et al., 1997; Ding et al., 2015). The images of OMC, FeOMC, and TriFeOMC for mesoporous structures were obtained through SEM, S-3400N and TEM, H-800.
The microscopic structure of OMC, FeOMC, and TriFeOMC with large (SEM) and small (TEM) magnifications (20 nm) provided the clearer perspective of the materials. The properties of mesoporous materials changed which can be seen as a well-ordered and enhanced structure of TriFeOMC as compared to OMC and FeOMC. The SEM images show that OMC structures are more like hexagonally shaped monoliths, while FeOMC structure looks like irregular structured monolith shapes (Figure 1). The TriFeOMC structure is more ordered, as-synthesized microporous with cylindrical shapes. The TEM images showed cylindrical shapes of OMC, a high surface area with small microparticles in FeOMC, and string shaped well-ordered shapes of TriFeOMC. The TEM images of TriFeOMC has shown a higher-order periodic degree; which confirms the two-dimensional string shaped distribution of the pores with connected pore channels, and uniform sizes as compared to OMC and FeOMC. The current paper focuses on the adsorption of heavy metals (Arsenic and Lead) by using the three above mentioned mesoporous materials.
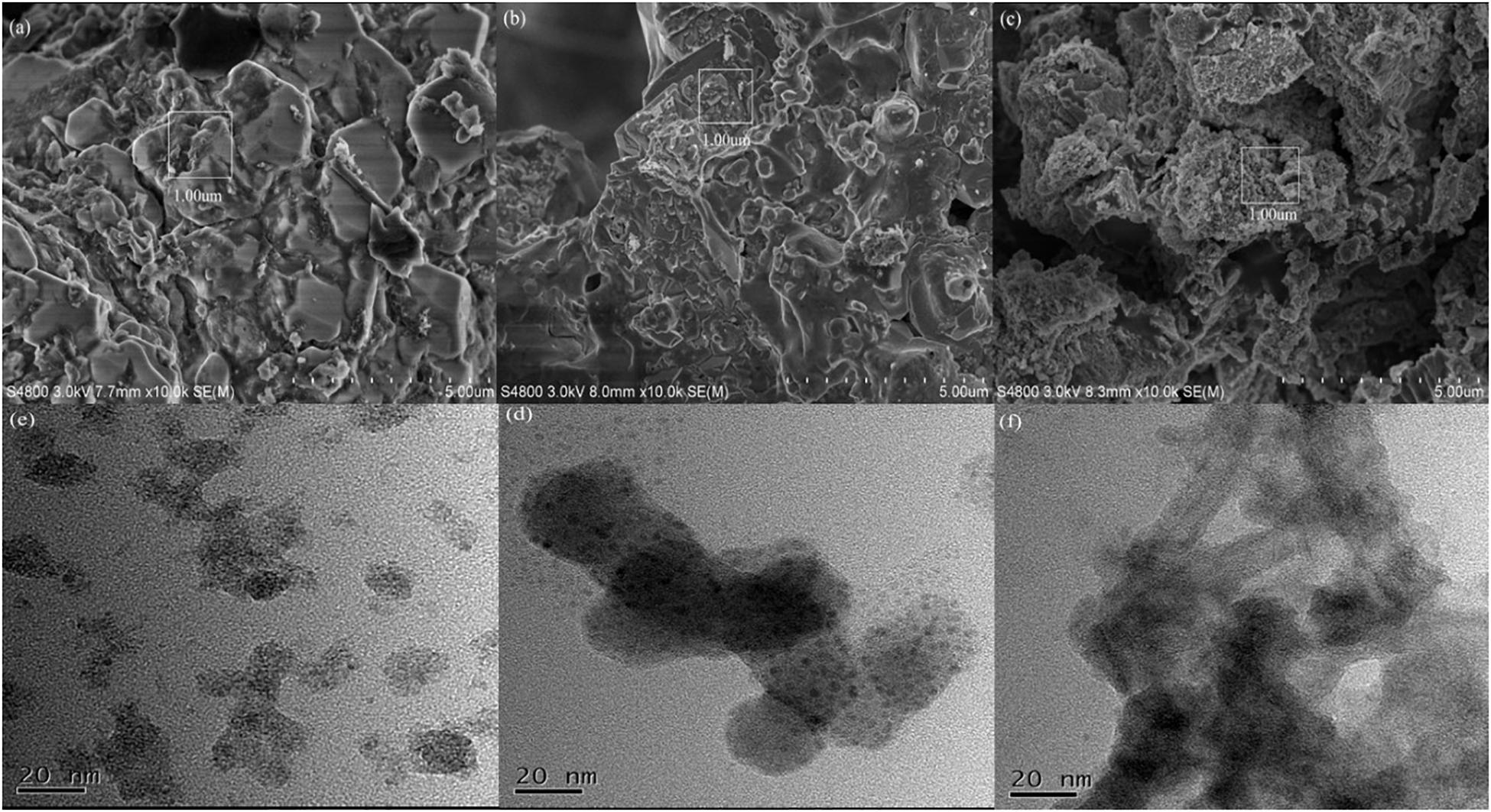
Figure 1. Scanning electron microscopy (a–c) with 5 times magnification of the specified area (1 μm) and transmission electron microscopy (d–f) images of OMC, FeOMC, and TriFeOMC, respectively.
The amine content of TriFeOMC has been determined by the Q500 (TGA Instruments) apparatus. The amine loading was calculated by weight loss during sample calcination at 1000°C (Harlick and Sayari, 2006). The magnetic field of 4.7 T has been used at room temperature on the instrument Bruker ASX200 to collect the Cross polarization (13°C) and CP-MAS NMR (magic angle spinning nuclear magnetic resonance) spectra for OMC, FeOMC, and TriFeOMC. The spinning frequency was 4.5 kHz with 50.32 MHz of the resonance frequency. There were 2 min contact time with 2 s recycle delay (Yangui et al., 2017). The FTIR spectroscopy analysis with wave number from 400 to 4000 cm–1 was performed by using Thermo Scientific Nicolet 6700 instrument.
The adsorption rate was quantified by using the following equation:
Whereas, Ci and Ce are the initial and the equilibrium concentrations (mg/L), respectively. The number of heavy metals (As, Pb) adsorbed per unit mass of adsorbent qe (mg/g) can be calculated as:
Whereas, m (g) is the adsorbent mass and V (L) is the solution volume used in the present study.
Results and Discussion
Properties of Materials
Nitrogen adsorption/desorption analysis explained the order of OMC and modified materials. BET surface area was 46.5697, 56.3290, and 54.3382 m2/g for OMC, FeOMC, and TriFeOMC, respectively (Figure 2). The results revealed that the isotherms of OMC and TriFeOMC were strongly constant channelized mesopores. The synthesized TriFeOMC had very well defined uniform mesopores, which shows similarity with the results of TEM images.
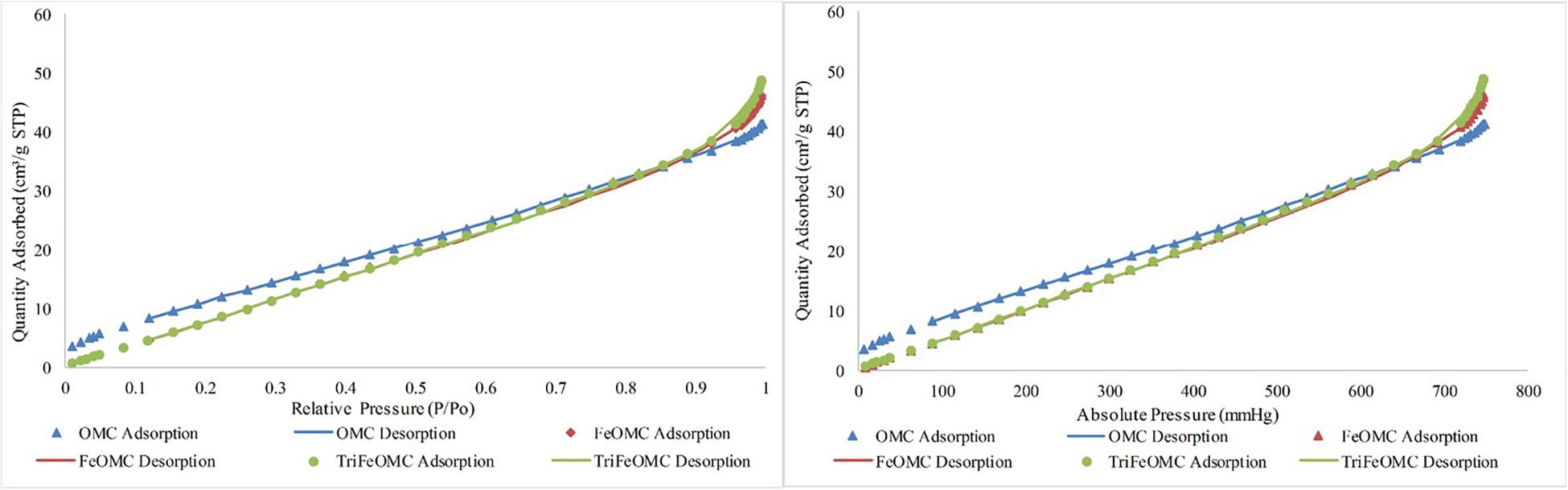
Figure 2. Nitrogen adsorption/desorption Isotherm absolute and linear plots of OMC, FeOMC, and TriFeOMC.
The Langmuir and BET linear plots further confirmed the higher order of amine-modified mesoporous materials. The Langmuir fits were highly fitted with the higher surface area while the BET surface area graph showed the comparatively better surface area of TriFeOMC than other two materials (Figure 3).
The OMC, FeOMC and TriFeOMC adsorption at a single point has been found nearly 187.2160 nm with the pore width at P/Po = 0.9896: 0.0629, 0.9896: 0.0687, and 0.9896: 0.0727 cm3/g, respectively whereas, BJH Adsorption capacity of OMC, FeOMC and TriFeOMC from the total pore volume has been found from 1.70 to 300.00 nm with the pore width of 0.0562, 0.0638, and 0.0680 cm3/g respectively. The BJH Desorption capacity of OMC, FeOMC, and TriFeOMC from the total pore volume has been found from 1.70 to 300.00 nm with the pore width of 0.0587, 0.0746, and 0.0788 cm3/g respectively. The adsorption average pore diameter (4V/A by BET) of OMC, FeOMC, and TriFeOMC were 5.4059, 4.8709, and 5.34833 nm (Figure 4).
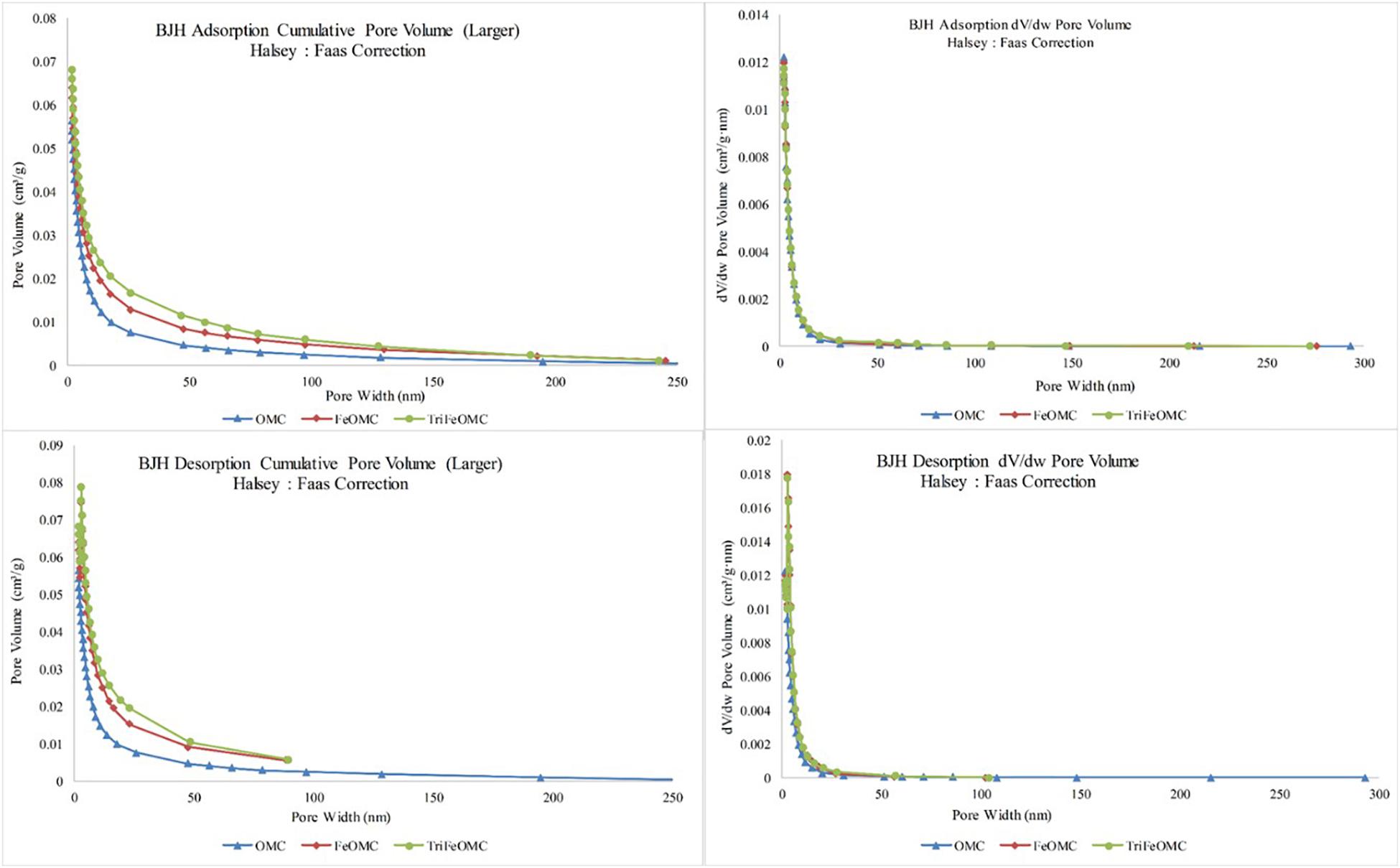
Figure 4. The pore volume and pore width comparisons for adsorption and desorption of OMC, FeOMC, and TriFeOMC.
During the post-processing of OMC, the iron and Triamine have been transferred toward the pores of the template due to the condensation in the capillaries. Similarly, the surface of the BET surface area decreased sharply and pores volume decreased slightly. The obtained TriFeOMC has a low surface area as compared to OMC and FeOMC, as mentioned above. The existence of small surface area and a decrease in the pore volume of TriFeOMC have been found the reason for highest Arsenic adsorption capacity. The results of Hamad et al. (2016) also showed that amino modification caused a decrease in the pore volume and pore size of the surface area of the modified material. The results by Yangui et al. (2017) also showed that the surface of P-10 and SBA-15 decreased abruptly during the process of amine grafting, it also decreased the pore diameter and pore volume which indicated the grafting of organo-silane groups on the internal surface of the amine modified materials.
The effects of thickness on the nitrogen adsorption/desorption were investigated and found that the increase in thickness is in direct proportion to the adsorption capacity of the materials (Figure 3). More precisely, at the low thickness, the adsorption capacity of OMC was higher than the other materials while at the higher thickness, the adsorption of modified materials was slightly higher than OMC as determined by t-plot drawn in Figure 5 through Harkins and Jura analytical approach.
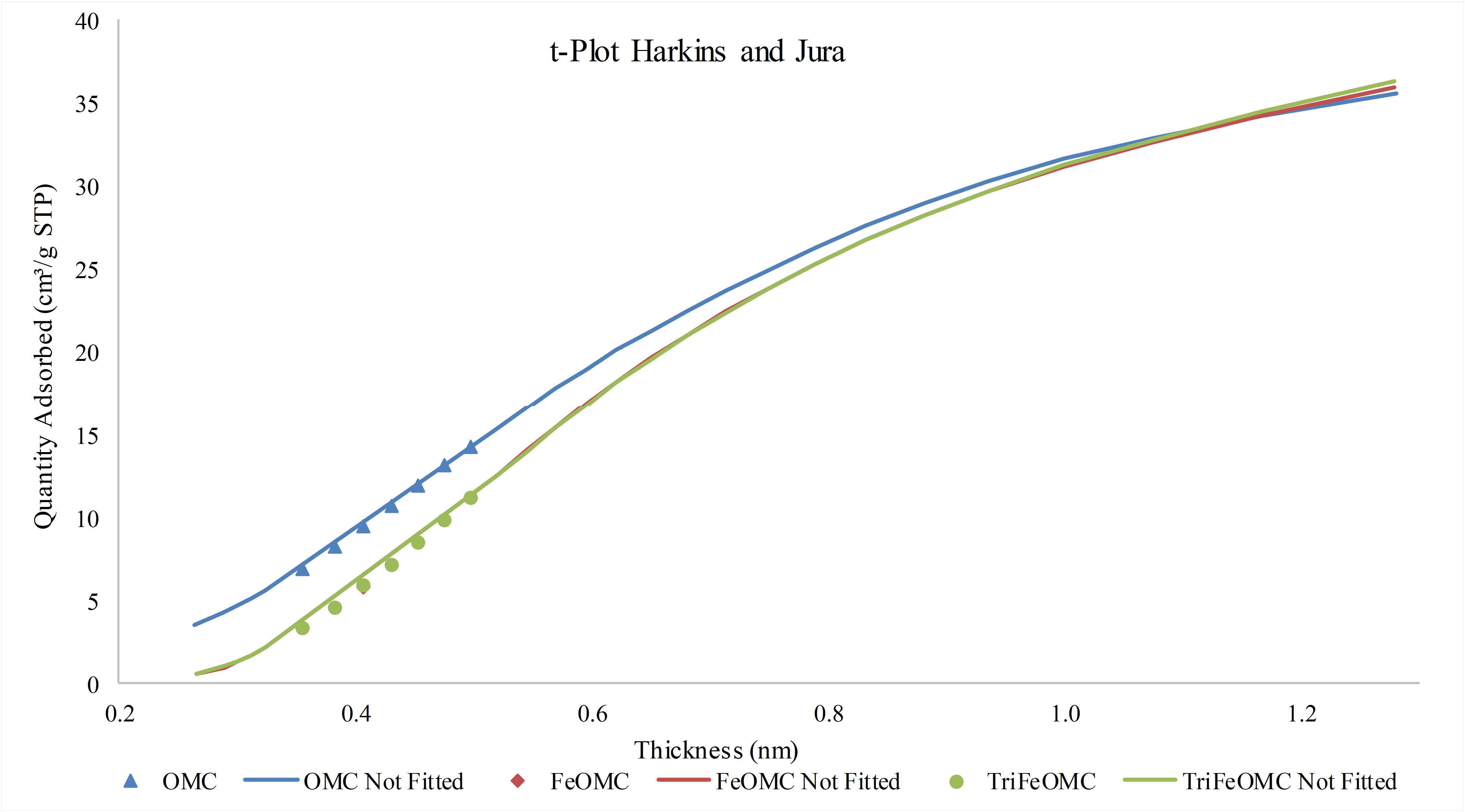
Figure 5. The t-plot (Harkins and Jura) between nitrogen adsorption quantity vs thickness of OMC, FeOMC, and TriFeOMC.
The amine loading up to 1000°C of TriFeOMC was calculated and found that modified weight loss decreased to 40% at 1000°C. There were higher fluctuations found in derived weight (%/°C) during the calcination of samples and reached at 0.059 (%/°C) from a total of 0.20 (%/C°) as shown in Figure 6. The total size of the sample was 14.96 mg for amine content measurement in the present study. The amine content ranged from 3.29 to 7.18 mmol N/g in the present study.
Fourier transforms infrared (FTIR) spectra for OMC, FeOMC, and TriFeOMC has been recorded. Figure 7 shows comparative spectra of OMC, FeOMC, and TriFeOMC with two band ranges from 1633 to 1666 cm–1 and 3413 to 3444 cm–1 for all materials. The elongation at 935 and 3689 cm–1 could be associated with the CH2 deformation, symmetric CH stretching, and aliphatic CH stretching vibrations (Silverstein et al., 2014; Yangui et al., 2017). These results showed that the functionalization of triamine group materials was effective. Alqadami et al. (2016) used the FTIR technique to describe the behavior of materials after the adsorption of toxic dye from wastewater; concluding that material nanoparticle size increased after adsorption.
The experiment was conducted to identify the efficient dosage which could be used for further experiments on heavy metals adsorption by OMC, FeOMC, and TriFeOMC. 0.04 (g) dosage has been found the best for estimating the adsorption capacities of OMC, FeOMC, and TriFeOMC, as shown in Figure 8. More precisely, TriFeOMC was the most efficient material found for the adsorption of As and OMC showed the highest adsorption efficiency for Pb in the current study.
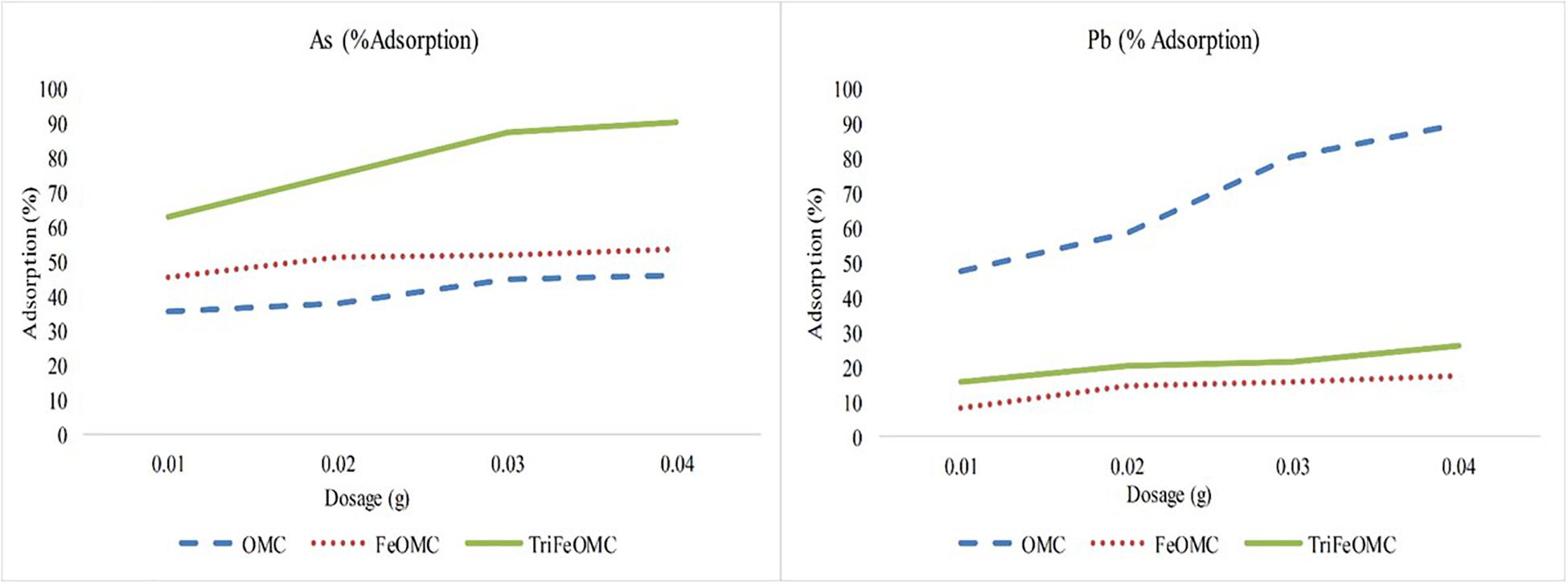
Figure 8. The percent adsorption of Arsenic (As) and Lead (Pb) by OMC, FeOMC, and TriFeOMC at different dosages with constant pH = 7 and T = 25°C.
Most of the heavy metals including As and Pb are pH dependent contaminants but use of pH 7 is well documented to understand the initial effects of new materials on the adsorption of heavy metals at this neutral pH. The surface of the mesoporous carbon materials is negative, which interacts with Pb ions at higher pH. At lower pH, the adsorption of Pb is very low due to strong competition between protons and available Pb ions for adsorption. Whereas, pH from 5.0 to 6.5 increases the negatively charged carbon materials interaction with Pb ions (Mohammadi et al., 2010; Mouni et al., 2011; Alghamdi et al., 2019) until it goes pH > 7. The Lead hydroxide starts to precipitate above pH 7 in the aqueous solution (Boujelben et al., 2009; Shen et al., 2018). Moreover, the interaction between amino group and acidic groups on the surface of carbon formed a strong layer during Pb ions adsorption process, which also resulted in the decreased adsorption of Pb on TriFeOMC. Alqadami et al. (2017) used the surface charge potential to describe the agglomeration of materials and showed that nanomaterials containing metallic ions showed negative charge at pH ≤ 7 and positive surface charge at pH > 7.
Adsorption Isotherms
To compare the adsorption capacity of OMC, FeOMC and TriFeOMC, the As and Pb adsorption isotherms data were fitted by Langmuir non-linear model Eq. (5) and Freundlich non-linear model Eq (6);
Where Ce: heavy metals concentration at equilibrium (mg/L); qe: adsorption capacity at equilibrium (mg/g); qm: maximum adsorption capacity (mg/g); and KL: adsorption constant.
Ce and qe have been shown above, Kf and 1/n are constants of Freundlich isotherm.
The Langmuir and Freundlich isotherms of As and Pb for OMC, FeOMC, and TriFeOMC are shown in Figure 9. The results for both model fit were complex. Langmuir model fit for OMC, while Freundlich model fit for FeOMC. Both models fit for TriFeOMC for As whereas, Langmuir model showed comparatively better fit for Pb. The maximum adsorption capacities for Arsenic were 21.44, 27.41, and 37.78 mg/g for the materials (OMC, FeOMC, and TriFeOMC, respectively), which indicates that TriFeOMC was most efficient and effective for the adsorption of Arsenic. The results of Wang et al. (2018) showed that the iron-containing mesocarbon materials (FeOMC) had a higher affinity for As adsorption than OMC, which is in agreement with our results. Showkat et al. (2007) prepared amine modified materials using ordered mesoporous silica and found that new materials had a comparatively better adsorption efficiency for As and Pb than the other previously prepared ordered mesoporous silica materials.
Similarly, the maximum adsorption capacities of OMC, FeOMC and TriFeOMC for Lead (Pb) adsorption were 28.53, 4.95, and 9.39 mg/g, respectively, which shows the higher capacity of OMC material as compared to FeOMC and TriFeOMC. The results of Lee et al. (2016) also agrees with our results in terms of Pb adsorption, showing that amine modified mesoporous silica showed less affinity for Pb adsorption than Thiol-functionalized mesoporous silica.
More precisely, TriFeOMC was comparatively better adsorbent than FeOMC. The reason for TriFeOMC reduced adsorption capacity for Pb might be due to the Pb response to the amines, which created a layer on the upper surface of the adsorbent resulting in the reduced adsorption. Therefore, it can be concluded that the newly developed amine modified ordered mesoporous carbon (TriFeOMC) is the best adsorbent for adsorption of Arsenic whereas, it has less capacity for Lead (Pb) adsorption.
There is a long history of preparation of materials for the adsorption of heavy metals from wastewater. Li et al. (2017) used nanoscale zero-valent iron to remove heavy metals from waste water using batch experiment and removed As(v) with comparatively high efficiency. Graphene oxide-MnFe2O4 nanohybrids have been used by Kumar et al. (2014) and successfully removed Pb, As(III) and As(V) from aqueous solution. The use of cation exchangers also showed the higher efficiency for the removal of Pb from aqueous solutions (Naushad, 2014; Naushad and ALOthman, 2015; Naushad et al., 2015). The use of amine surface functionalized magnetite nanoparticles prepared by Nata et al. (2011) resulted in the enhanced thermal and mechanical properties of cellulose nanofibrils and demonstrated the higher efficiency of As(V) removal due to the presence of iron-oxide and amine contents. Taleb et al. (2019) also removed As from magnetite-loaded amino modified nano-cellulose adsorbents with good removal efficiency. Önnby et al. (2014) used Aluminum nanoparticles incorporated amine rich cryogels nano-composites for the removal of As showing successful removal of As(V) from aqueous solution.
Adsorption Kinetics
The adsorption kinetics of Arsenic and Lead were also investigated for adsorption behaviors of OMC, FeOMC, and TriFeOMC. The dosage of 0.03 g of adsorbents (OMC, FeOMC, and TriFeOMC) has been used in the solution with the initial concentrations of 9.45, 10.28, and 9.97 mg/L of Arsenic and solution was monitored at different time, namely 20, 40, 60, 80, and 120 min at optimum pH (7) and at constant temperature of 25°C. The initial concentrations of Lead (Pb) for the same experiment were 11.15, 11.46, and 11.68 mg/L with 0.03 g of OMC, FeOMC, and TriFeOMC with the same temperature, time, and pH. The study of adsorption kinetics at the solid-phase interface is of major importance for determining the uptake rate of the prepared material (adsorbate). The pseudo-first-order kinetic model in non-linear (Eq. 7) equation form is given below;
K1 is adsorption constent, adsorption at equilibrium (qe) and R2 by using linear fit statistics for both heavy metals. Similarly, the non-linear pseudo-second-order equations is;
K2 is adsorption rate constant, equilibrium adsorption (qe), R2, and initial adsorption rate (H).
The results of both non-linear kinetic models are presented in Table 1. For OMC, FeOMC, and TriFeOMC, the adsorption process followed an almost similar way of both pseudo models for Arsenic and Lead adsorption and the adsorption process has been followed by pseudo-second-order for the adsorption of Pb (Table 1). The fact that equilibrium adsorption capacities (qe) which have been calculated through the pseudo-second-order model overall have been found comparatively more closely and therefore suggests the usage of this model to analyze the data for such experiments.
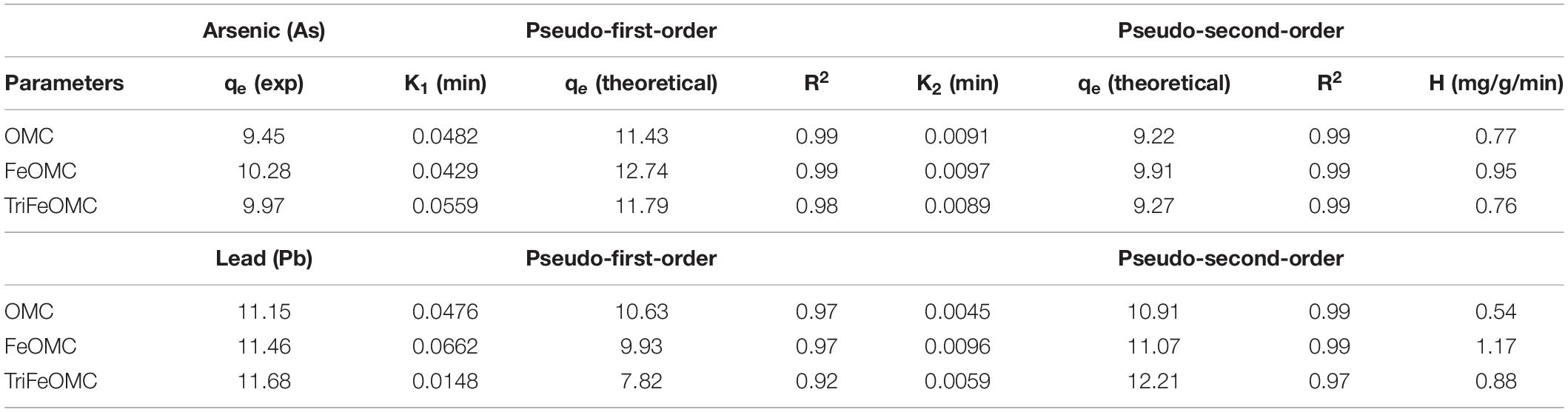
Table 1. The parameters of adsorption kinetics of arsenic and lead on OMC, FeOMC, and TriFeOMC at pH 7 (R2 is the correlation coefficient of the model fits) using non-linear models.
The mechanisms for the heavy metals adsorption on to the mesoporous carbons and modified mesoporous carbons are important to understand. Adsorption is the most favorable technique adopted by researchers around the globe for heavy metals removal. Naushad et al. (2016) used the adsorption techniques for the removal of mercury from aqueous solution and successfully removed it using an alizarin red-S-loaded amberlite IRA-400 resin. Mironyuk et al. (2019) used mesoporous carbonated TiO2 for the adsorption of strontium and successfully removed the strontium ions from aqueous solution. A nano-adsorbent prepared by using an organic ligant with mesoporous silica for the adsorption of Pb(II) by Shahat et al. (2015), and successfully removed Pb(II) with excellent adsorption efficiency.
There are several interactions between surface functional groups and ions of the target metals, including electrostatic interactions, chemical interactions, and surface precipitation (Hamad et al., 2016). In the case of the present study, Tri-amine modified mesocarbon materials have shown major chemical interactions with Pb. The interaction strength between the surface functional groups and metal ions mainly depends on the properties of material surface ions. Phenol resin resol template consists of the acidic groups and in case of amine groups; the adsorption in the amine group is mainly dependent on nitrogen atoms which are mainly responsible for the formations of the ligand with the metal ions. In Figure 7 it has been shown that symmetrical bending of NH2 have not found at the bands of 400 and 3689 cm–1 for Triamine modified mesoporous material for both heavy metals. This result verifies the interaction between amino group and acidic groups on the surface of carbon during heavy metal ions adsorption process. The discussed mechanism has more effect on the efficiency of Pb adsorption onto TriFeOMC due to the creation of a strong layer at the surface after interaction with Pb. The amine group –NH2 interaction with Arsenic easily formed coordination complexes which have been found highly responsible for efficient adsorption of As by TriFeOMC.
Conclusion
The synthesis of OMC materials with iron and later with Tri-amine has been done successfully using a soft template containing phenol resin resol and triblock copolymer F-127. The physiochemical properties of materials were characterized using N2 adsorption/desorption isotherms, scanning electron microscopy (SEM), transmission electron microscopy (TEM), and surface area analysis determined by Brunauer–Emmett–Teller (BET). The spectra through Fourier-transform infrared spectroscopy (FTIR) were recorded for OMC, FeOMC, and TriFeOMC. The finally obtained TriFeOMC material has been found with higher porosity and a large surface area which make this material an excellent adsorbent of As. The comparison of OMC, FeOMC, and TriFeOMC has been made to check their efficiency for adsorption of As and Pb. The adsorption percentage of TriFeOMC was excellent for As whereas, Pb adsorption was highest on OMC. The less efficiency of TriFeOMC for Pb was due to the formation of a thin layer due to the chemical reaction between amine and Pb. The Langmuir model fit for OMC, while Freundlich model fit for FeOMC. Both models fit for TriFeOMC for As whereas, Langmuir model showed comparatively better fit for Pb. The adsorption process followed an almost similar way of both pseudo models for Arsenic and Lead adsorption for OMC, FeOMC, and TriFeOMC and the adsorption process has been followed by pseudo-second-order for the adsorption of Pb. The present study provided strong evidence of As adsorption from aqueous solution using the novel material TriFeOMC as an excellent adsorbent with excellent performance.
Data Availability Statement
All datasets generated for this study are included in the article/supplementary material.
Author Contributions
HK and JZ contributed to the conceptualization of the study. HK devised the methodology. HK, AJ, and FX contributed to model analysis. FH and HK contributed to formal analysis. HK, AJ, and RS prepared the original draft. FH reviewed and edited the manuscript. JZ supervised.
Funding
This research was done in support of the National Natural Science Foundation of China (Grant Nos. 51979077, 51438006, and 2014YQ060773), the Fundamental Research Funds for the Central Universities (Grant No. 2019B42414), and Jiangsu Provincial Science and technology Program Project (Grant No. SBA2018030430).
Conflict of Interest
The authors declare that the research was conducted in the absence of any commercial or financial relationships that could be construed as a potential conflict of interest.
References
Alghamdi, A. A., Al-Odayni, A.-B., Saeed, W. S., Al-Kahtani, A., Alharthi, F. A., and Aouak, T. (2019). Efficient adsorption of lead (II) from aqueous phase solutions using polypyrrole-based activated carbon. Materials 12:2020. doi: 10.3390/ma12122020
Alqadami, A. A., Naushad, M., Abdalla, M. A., Khan, M. R., and Alothman, Z. A. (2016). Adsorptive removal of toxic dye using Fe3O4–TSC nanocomposite: equilibrium, kinetic, and thermodynamic studies. J. Chem. Eng. Data 61, 3806–3813.
Alqadami, A. A., Naushad, M., Alothman, Z. A., and Ghfar, A. A. (2017). Novel metal–organic framework (MOF) based composite material for the sequestration of U (VI) and Th (IV) metal ions from aqueous environment. ACS Appl. Mater. Interf. 9, 36026–36037. doi: 10.1021/acsami.7b10768
Boujelben, N., Bouzid, J., and Elouear, Z. (2009). Removal of lead (II) ions from aqueous solutions using manganese oxide-coated adsorbents: characterization and kinetic study. Adsorpt. Sci. Technol. 27, 177–191.
Chew, T.-L., Ahmad, A. L., and Bhatia, S. (2010). Ordered mesoporous silica (OMS) as an adsorbent and membrane for separation of carbon dioxide (CO2). Adv. Coll. Interf. Sci. 153, 43–57. doi: 10.1016/j.cis.2009.12.001
Ding, Y., Zhu, J., Cao, Y., and Chen, S. (2014). Adsorption equilibrium, kinetics and thermodynamics of dichloroacetic acid from aqueous solution using mesoporous carbon. Environ. Technol. 35, 1962–1970. doi: 10.1080/09593330.2014.889216
Ding, Y., Zhu, J., Ji, D., Cao, Y., Ling, X., and Chen, W. (2015). Enhancing adsorption efficiency of dichloroacetic acid onto mesoporous carbons: procedure optimization, mechanism and characterization. J. Coll. Interf. Sci. 452, 134–140. doi: 10.1016/j.jcis.2015.04.040
Hamad, H., Ezzeddine, Z., Lakis, F., Rammal, H., Srour, M., and Hijazi, A. (2016). An insight into the removal of Cu (II) and Pb (II) by aminopropyl-modified mesoporous carbon CMK-3: adsorption capacity and mechanism. Mater. Chem. Phys. 178, 57–64.
Harlick, P. J. E., and Sayari, A. (2006). Applications of pore-expanded mesoporous silicas. 3. triamine silane grafting for enhanced CO2 adsorption. Indust. Eng. Chem. Res. 45, 3248–3255. doi: 10.1021/ie051286p
Hu, Q., Liu, Y., Gu, X., and Zhao, Y. (2017). Adsorption behavior and mechanism of different arsenic species on mesoporous MnFe2O4 magnetic nanoparticles. Chemosphere 181, 328–336. doi: 10.1016/j.chemosphere.2017.04.049
Kruk, M., Jaroniec, M., and Sayari, A. (1997). Application of large pore MCM-41 molecular sieves to improve pore size analysis using nitrogen adsorption measurements. Langmuir 13, 6267–6273. doi: 10.1021/la970776m
Kumar, S., Nair, R. R., Pillai, P. B., Gupta, S. N., Iyengar, M., and Sood, A. (2014). Graphene oxide–MnFe2O4 magnetic nanohybrids for efficient removal of lead and arsenic from water. ACS Appl. Mater. Interf. 6, 17426–17436. doi: 10.1021/am504826q
Lee, J., Chen, C., Cheng, S., and Li, H.-Y. (2016). Adsorption of Pb(II) and Cu(II) metal ions on functionalized large-pore mesoporous silica. Intern. J. Environ. Sci. Technol. 13, 65–76. doi: 10.1007/s13762-015-0841-y
Lee, J.-S., Joo, S. H., and Ryoo, R. (2002). Synthesis of mesoporous silicas of controlled pore wall thickness and their replication to ordered nanoporous carbons with various pore diameters. J. Am. Chem. Soc. 124, 1156–1157. doi: 10.1021/ja012333h
Li, S., Wang, W., Liang, F., and Zhang, W.-X. (2017). Heavy metal removal using nanoscale zero-valent iron (nZVI): theory and application. J. Hazard. Mater. 322, 163–171. doi: 10.1016/j.jhazmat.2016.01.032
Li, Y., Zhang, J., and Liu, H. (2018). Removal of chloramphenicol from aqueous solution using low-cost activated carbon prepared from Typha orientalis. Water 10:351.
Liang, C., Li, Z., and Dai, S. (2008). Mesoporous carbon materials: synthesis and modification. Angewandte Chem. Intern. Edn. 47, 3696–3717. doi: 10.1002/anie.200702046
Liu, R., Shi, Y., Wan, Y., Meng, Y., Zhang, F., Gu, D., et al. (2006). Triconstituent Co-assembly to Ordered mesostructured polymer-silica and carbon-silica nanocomposites and large-pore mesoporous carbons with high surface areas. J. Am. Chem. Soc. 128, 11652–11662. doi: 10.1021/ja0633518
Ma, L., and Zhang, W.-X. (2008). Enhanced Biological Treatment Of Industrial Wastewater With Bimetallic Zero-Valent Iron. Washington, DC: ACS Publications.
Mayes, R. T., Tsouris, C., Kiggans, J. O. Jr., Mahurin, S. M., DePaoli, D. W., and Dai, S. (2010). Hierarchical ordered mesoporous carbon from phloroglucinol-glyoxal and its application in capacitive deionization of brackish water. J. Mater. Chem. 20, 8674–8678. doi: 10.1039/C0JM01911A
Meng, Y., Gu, D., Zhang, F., Shi, Y., Cheng, L., Feng, D., et al. (2006). A family of highly ordered mesoporous polymer resin and carbon structures from organic-organic self-assembly. Chem. Mater. 18, 4447–4464. doi: 10.1021/cm060921u
Mironyuk, I., Tatarchuk, T., Naushad, M., Vasylyeva, H., and Mykytyn, I. (2019). Highly efficient adsorption of strontium ions by carbonated mesoporous TiO2. J. Mol. Liquids 285, 742–753.
Mohammadi, S. Z., Karimi, M. A., Afzali, D., and Mansouri, F. (2010). Removal of Pb (II) from aqueous solutions using activated carbon from Sea-buckthorn stones by chemical activation. Desalination 262, 86–93.
Mohan, S., Kumar, V., Singh, D. K., and Hasan, S. H. (2017). Effective removal of lead ions using graphene oxide-MgO nanohybrid from aqueous solution: isotherm, kinetic and thermodynamic modeling of adsorption. J. Environ. Chem. Eng. 5, 2259–2273.
Mouni, L., Merabet, D., Bouzaza, A., and Belkhiri, L. (2011). Adsorption of Pb (II) from aqueous solutions using activated carbon developed from Apricot stone. Desalination 276, 148–153.
Nata, I. F., Sureshkumar, M., and Lee, C.-K. (2011). One-pot preparation of amine-rich magnetite/bacterial cellulose nanocomposite and its application for arsenate removal. RSC Adv. 1, 625–631.
Naushad, M. (2014). Surfactant assisted nano-composite cation exchanger: development, characterization and applications for the removal of toxic Pb2+ from aqueous medium. Chem. Eng. J. 235, 100–108.
Naushad, M., ALOthman, Z., Awual, M. R., Alam, M. M., and Eldesoky, G. (2015). Adsorption kinetics, isotherms, and thermodynamic studies for the adsorption of Pb 2+ and Hg 2+ metal ions from aqueous medium using Ti (IV) iodovanadate cation exchanger. Ionics 21, 2237–2245.
Naushad, M., and ALOthman, Z. A. (2015). Separation of toxic Pb2+ metal from aqueous solution using strongly acidic cation-exchange resin: analytical applications for the removal of metal ions from pharmaceutical formulation. Desalinat. Water Treat. 53, 2158–2166.
Naushad, M., Vasudevan, S., Sharma, G., Kumar, A., and Alothman, Z. (2016). Adsorption kinetics, isotherms, and thermodynamic studies for Hg2+ adsorption from aqueous medium using alizarin red-S-loaded amberlite IRA-400 resin. Desalinat. Water Treat. 57, 18551–18559.
Ning, R. Y. (2002). Arsenic removal by reverse osmosis. Desalination 143, 237–241. doi: 10.1016/S0011-9164(02)00262-X
Önnby, L., Kumar, P. S., Sigfridsson, K. G., Wendt, O. F., Carlson, S., and Kirsebom, H. (2014). Improved arsenic (III) adsorption by Al2O3 nanoparticles and H2O2: Evidence of oxidation to arsenic (V) from X-ray absorption spectroscopy. Chemosphere 113, 151–157. doi: 10.1016/j.chemosphere.2014.04.097
Qiu, B., Wang, Y., Sun, D., Wang, Q., Zhang, X., Weeks, B. L., et al. (2015). Cr (VI) removal by magnetic carbon nanocomposites derived from cellulose at different carbonization temperatures. J. Mater. Chem. A 3, 9817–9825.
Shahat, A., Awual, M. R., Khaleque, M. A., Alam, M. Z., Naushad, M., and Chowdhury, A. S. (2015). Large-pore diameter nano-adsorbent and its application for rapid lead (II) detection and removal from aqueous media. Chem. Eng. J. 273, 286–295.
Shen, J., Wang, N., Wang, Y., Yu, D., and Ouyang, X. K. (2018). Efficient adsorption of Pb (II) from aqueous solutions by metal organic framework (Zn-BDC) coated magnetic montmorillonite. Polymers 10:1383. doi: 10.3390/polym10121383
Showkat, A., Zhang, Y. P., Min, S. K., Iyengar Gopalan, A., Reddy, R., and Lee, K.-S. (2007). Analysis of heavy metal toxic ions by adsorption onto amino-functionalized ordered mesoporous silica. Bull. Korea. Chem. Soc. 28, 1985–1992.
Silverstein, R. M., Webster, F. X., Kiemle, D. J., and Bryce, D. L. (2014). Spectrometric Identification Of Organic Compounds. Hoboken, NJ: John wiley & sons.
Song, S., Lopez-Valdivieso, A., Hernandez-Campos, D. J., Peng, C., Monroy-Fernandez, M. G., and Razo-Soto, I. (2006). Arsenic removal from high-arsenic water by enhanced coagulation with ferric ions and coarse calcite. Water Res. 40, 364–372. doi: 10.1016/j.watres.2005.09.046
Sun, X., Hu, C., and Qu, J. (2009). Adsorption and removal of arsenite on ordered mesoporous Fe-modified ZrO2. Desalinat. Water Treat. 8, 139–145.
Szabó, T., Tombácz, E., Illés, E., and Dékány, I. (2006). Enhanced acidity and pH-dependent surface charge characterization of successively oxidized graphite oxides. Carbon 44, 537–545. doi: 10.1016/j.carbon.2005.08.005
Taleb, K., Markovski, J., Veličković, Z., Rusmirović, J., Rančić, M., Pavlović, V., et al. (2019). Arsenic removal by magnetite-loaded amino modified nano/microcellulose adsorbents: Effect of functionalization and media size. Arab. J. Chem. 12, 4675–4693.
Teng, W., Wu, Z., Fan, J., Zhang, W.-X., and Zhao, D. (2015). Amino-functionalized ordered mesoporous carbon for the separation of toxic microcystin-LR. J. Mater. Chem. A 3, 19168–19176. doi: 10.1039/c5ta05320j
Titirici, M.-M., Thomas, A., and Antonietti, M. (2007). Aminated hydrophilic ordered mesoporous carbons. J. Mater. Chem. 17, 3412–3418. doi: 10.1039/b703569a
Vinu, A., Srinivasu, P., Takahashi, M., Mori, T., Balasubramanian, V. V., and Ariga, K. (2007). Controlling the textural parameters of mesoporous carbon materials. Micropor. Mesopor. Mater. 100, 20–26. doi: 10.1016/j.micromeso.2006.10.008
Wan, Y., Shi, Y., and Zhao, D. (2008). Supramolecular aggregates as templates: ordered mesoporous polymers and carbons. Chem. Mater. 20, 932–945.
Wang, K., Huang, B., Liu, D., and Ye, D. (2012). Ordered mesoporous carbons with various pore sizes: preparation and naphthalene adsorption performance. J. Appl. Polymer Sci. 125, 3368–3375.
Wang, L., Yao, M., Hu, X., Hu, G., Lu, J., Luo, M., et al. (2015). Amine-modified ordered mesoporous silica: The effect of pore size on CO2 capture performance. Appl. Surf. Sci. 324, 286–292. doi: 10.1016/j.apsusc.2014.10.135
Wang, Z., Hu, W., Kang, Z., He, X., Cai, Z., and Deng, B. (2018). Arsenate adsorption on iron-impregnated ordered mesoporous carbon: fast kinetics and mass transfer evaluation. Chem. Eng. J. 357, 463–472.
Wu, Z., Webley, P. A., and Zhao, D. (2010). Comprehensive study of pore evolution, mesostructural stability, and simultaneous surface functionalization of ordered mesoporous carbon (FDU-15) by wet oxidation as a promising adsorbent. Langmuir 26, 10277–10286. doi: 10.1021/la100455w
Xiang, Y., Xu, Z., Wei, Y., Zhou, Y., Yang, X., Yang, Y., et al. (2019). Carbon-based materials as adsorbent for antibiotics removal: Mechanisms and influencing factors. J. Environ. Manag. 237, 128–138. doi: 10.1016/j.jenvman.2019.02.068
Xue, C., Tu, B., and Zhao, D. (2009). Facile fabrication of hierarchically porous carbonaceous monoliths with ordered mesostructure via an organic organic self-assembly. Nano Res. 2, 242–253.
Yadaei, H., Beyki, M. H., Shemirani, F., and Nouroozi, S. (2018). Ferrofluid mediated chitosan@mesoporous carbon nanohybrid for green adsorption/preconcentration of toxic Cd(II): modeling, kinetic and isotherm study. React. Funct. Polym. 122, 85–97. doi: 10.1016/j.reactfunctpolym.2017.10.011
Yang, J., Zhai, Y., Deng, Y., Gu, D., Li, Q., Wu, Q., et al. (2010). Direct triblock-copolymer-templating synthesis of ordered nitrogen-containing mesoporous polymers. J. Coll. Interf. Sci. 342, 579–585. doi: 10.1016/j.jcis.2009.10.037
Yangui, A., Abderrabba, M., and Sayari, A. (2017). Amine-modified mesoporous silica for quantitative adsorption and release of hydroxytyrosol and other phenolic compounds from olive mill wastewater. J. Taiwan Instit. Chem. Eng. 70, 111–118.
Yuan, B., Wu, X., Chen, Y., Huang, J., Luo, H., and Deng, S. (2013). Adsorption of CO2, CH4, and N2 on ordered mesoporous carbon: approach for greenhouse gases capture and biogas upgrading. Environ. Sci. Technol. 47, 5474–5480. doi: 10.1021/es4000643
Zeleňák, V., Badaničová, M., Halamová, D., Čejka, J., Zukal, A., Murafa, N., et al. (2008). Amine-modified ordered mesoporous silica: effect of pore size on carbon dioxide capture. Chem. Eng. J. 144, 336–342. doi: 10.1016/j.cej.2008.07.025
Zhang, F., Meng, Y., Gu, D., Yan, Y., Chen, Z., Tu, B., et al. (2006). An aqueous cooperative assembly route to synthesize ordered mesoporous carbons with controlled structures and morphology. Chem. Mater. 18, 5279–5288.
Zhang, F., Meng, Y., Gu, D., Yan, Y., Yu, C., Tu, B., et al. (2005). A facile aqueous route to synthesize highly ordered mesoporous polymers and carbon frameworks with Ia 3 d bicontinuous cubic structure. J. Am. Chem. Soc. 127, 13508–13509. doi: 10.1021/ja0545721
Zhang, Y., Wei, S., Liu, F., Du, Y., Liu, S., Ji, Y., et al. (2009). Superhydrophobic nanoporous polymers as efficient adsorbents for organic compounds. Nano Today 4, 135–142.
Zhao, D., Huo, Q., Feng, J., Chmelka, B. F., and Stucky, G. D. (1998). Nonionic triblock and star diblock copolymer and oligomeric surfactant syntheses of highly ordered, hydrothermally stable, mesoporous silica structures. J. Am. Chem. Soc. 120, 6024–6036. doi: 10.1021/ja974025i
Zhu, J., Gu, H., Guo, J., Chen, M., Wei, H., Luo, Z., et al. (2014). Mesoporous magnetic carbon nanocomposite fabrics for highly efficient Cr (VI) removal. J. Mater. Chem. A 2, 2256–2265.
Keywords: adsorption, triamine, ordered mesoporous carbon, arsenic, lead
Citation: Khan HOS, Zhu J, Jalil A, Sarwar RT, Hameed F and Xu F (2020) Optimal Synthesis and Evaluation of Tri-Amine Modified Ordered Mesoporous Carbon (TriFeOMC) and Its Application for the Adsorption of Arsenic and Lead From Aqueous Solution. Front. Mater. 7:112. doi: 10.3389/fmats.2020.00112
Received: 19 September 2019; Accepted: 14 April 2020;
Published: 19 May 2020.
Edited by:
Federico Cesano, University of Turin, ItalyReviewed by:
Che Azurahanim Che Abdullah, Universiti Putra Malaysia, MalaysiaZeid ALOthman, King Saud University, Saudi Arabia
Copyright © 2020 Khan, Zhu, Jalil, Sarwar, Hameed and Xu. This is an open-access article distributed under the terms of the Creative Commons Attribution License (CC BY). The use, distribution or reproduction in other forums is permitted, provided the original author(s) and the copyright owner(s) are credited and that the original publication in this journal is cited, in accordance with accepted academic practice. No use, distribution or reproduction is permitted which does not comply with these terms.
*Correspondence: Jianzhong Zhu, zhuhhai2010@hhu.edu.cn