Survival Strategies of Mangrove (Ceriops tagal (perr.) C. B. Rob) and the Inspired Corrosion Inhibitor
- 1Department of Mechanical Engineering, City University of Hong Kong, Hong Kong, Hong Kong SAR, China
- 2Shenzhen Institute of Advanced Technology, Chinese Academy of Sciences, Shenzhen, China
Corrosion is ubiquitous, inevitable, and very common in our daily lives; it has been one of the most significant challenges and needs to be addressed for the development of modern society. Corrosion-resisting strategies found in nature have been promising for many halophytes surviving in marine tidal zones facing high salinity and humidity. Among the halophytes, mangroves have developed a lot of functions to deal with such extreme conditions that could provide novel inspirations for the fabrication of engineering materials, especially for materials resisting corrosion. In this study, we first investigated two survival strategies of mangroves, namely, functional salt-secreting and mangrove tannin composite. Salt-secreting realized by mangrove salt glands could make mangroves excrete excessive salt and avoid harmful effects. On the other hand, mangrove tannins existing in large quantities in mangrove bark could help the mangroves survive some marine bacteria. Inspired by the functional mangrove tannins, we developed a corrosion inhibitor to protect Q235 carbon steel in acid 3.5 wt% NaCl solution. Furthermore, we found that the mangrove-inspired corrosion inhibitor could be adsorbed at the metal–solution interface, functioning as a cathodic inhibitor with 60.51% anti-corrosion efficiency.
1 Introduction
Corrosion is an inevitable process that transforms materials (mainly metals) from an unfavorable high-energy state to a stable low energy state and is one of the long-standing challenges impeding the rapid and sustainable deployment of materials. To some extent, the progress of industrial processes is also marked by the search for new solutions to fight against material corrosion (Cui et al., 2019a). Corrosion-resistant methods, including passive coatings, cathodic protection, and corrosion inhibitor, have been developed to solve this crisis. Among those approaches, corrosion inhibitors, including inorganic and organic inhibitors, have been applied to achieve significant anti-corrosion efficiency, especially in aggressive environments. Corrosion inhibitors are substances that could be added to the corrosive medium in small concentrations to reduce or delay the corrosion reactions of metals (Kusmierek and Chrzescijanska, 2015). The underlying reason for the protective ability is that inhibitors could form a protective layer on the substrate surface to isolate the contact between the metal substrate and corrosive substances. Previously, inorganic inhibitors such as chromate-based compounds were first used to achieve corrosion protection in various systems, but they are seldom used nowadays due to environmental toxicity. Therefore, the aggressive inorganic inhibitors are gradually displaced by organic inhibitors to balance effective corrosion inhibition efficiency with ecological concerns. Significantly, the harmful effects of synthetic inhibitors have set the trend to find safer and environmentally benign natural products (Flores Merino et al., 2017) functioning as efficient inhibitors. The natural organics are biodegradable, eco-friendly, available, and cost-effective, and some have been successfully applied as inhibitors or composited to synergetic inhibitors (Majd et al., 2020). For instance, the organic extracts of Chrysophyllum albidum leaves (Akalezi and Oguzie, 2015) mainly constitute carbohydrates, proteins, flavonoids, glycosides, and terpenoids that inhibit mild steel corrosion in 1 M HCl solution. In addition, organic extractions of Cannabis sativa leaves comprised heteroatoms that could delay the corrosion process of low carbon steel (LCS) in the acidic medium (0.5 M sulfuric acid) (Haldhar et al., 2021). Moreover, the organic substances from Mangifera indica L. were found to provide corrosion prevention for commercial steel in a 3.5 wt% NaCl environment. Generally, those natural organic products could adsorb on the metal surface and structure coordination bonds with free d-orbital of iron or other metals, forming a defensive covering with high inhibition efficiency (Cui et al., 2021).
Organic mangrove compounds containing heteroatoms such as oxygen, nitrogen, or sulfur tend to be suitable inhibitors with excellent corrosion inhibition efficiency in aggressive acidic conditions. The mangrove extractions contain functional tannins, a complex mixture of non-toxic, biodegradable polyphenolic compounds (Tan and Kassim, 2011) that could inhibit corrosion processes by adsorbing on the metal surface (Shah et al., 2011). This significant corrosion-resistant performance lies in the vicinity of hydroxyl groups in the ortho-position in the polyphenolic moieties, causing mangrove tannins to be adsorbed on the protected metal surface following the Langmuir adsorption isotherm model. Therefore, organic mangrove products could form chelates with iron, other metallic cations and rust-phase components for the functional hydroxyl groups (Rahim et al., 2007; Jaén et al., 2011; Shah et al., 2013; Li et al., 2014). In addition, mangrove tannins can be applied in synergy with other substances, such as halide ions (Ridhwan et al., 2012; Adam et al., 2014), to enhance the inhibition performance for the co-adsorption effect synergistically. Although some satisfying corrosion-resistant results have been revealed in acid surroundings, organic mangrove compounds were not applied to protect the metals under marine surroundings, a corrosive situation for any engineering material. Moreover, organic mangrove compounds are only one innovative approach that the mangrove has evolved to protect against environmental extremes from the challenges of living surroundings. It is significant to explore the survival approaches of mangroves from the aspect of structure and functional compounds that could provide remarkable inspiration for designing novel engineering corrosion-resistant materials. In particular, the advancement in biomimetics has provided outstanding inspiration to develop new artificial, functional corrosion-resistant materials which are environmentally benign and functionally versatile. In our previous research, one of the survival strategies, salt-secreting ability, has been studied and mimicked to block the transportation of corrosive ions in the corresponding bioinspired coatings (Cui et al., 2019b). However, we did not comprehensively reveal the underlying mechanism of salt-secretion and the contributions of some active compounds toward mangrove survival, and there is no mangrove-based bionic corrosion inhibitor that could be applied under marine surroundings.
In this work, we explored the approaches for mangrove survival in the marine surroundings from the two aspects of functional structure and active–active ingredients; based on this, we developed new environmentally benign anti-corrosion materials. By exploring the available designs of the mangrove leaves, we found that the mangrove regulates excessive internal inorganic ions by salt glands, mainly having three morphologies: hump, flat, and sunken on the leaf surface. Furthermore, we revealed that salt glands of different morphologies exhibit salt-secreting activity under the immersion of salt solution by observing the secreted salt solution circle covering the isolated mangrove leaf tissue. Under the protection of salt-regulating function, mangrove exhibits excellent survival advantages compared to ornamental plants. In addition, we demonstrated the protective ability of the active–active ingredients composited in mangrove bark. By culturing the mangrove bark blocks and aggressive bacteria (Escherichia coli), we found that mangrove bark could inhibit the growth of bacteria with the presence of mangrove tannins that helped the mangrove survive in the ocean. Inspired by the protective function of mangrove tannins, we applied the mangrove tannins as a corrosion inhibitor to protect the metals from corrosion under marine surroundings. By performing electrochemical measurements, we found that mangrove tannins could be adsorbed in the metal–solution interface, replacing the water film and acting as cathodic inhibitors with an anti-corrosion efficiency of 60.51%. Excitingly, mangrove tannins are environmentally benign and play much better defensive performance than commercial ones.
2 Materials and Experiment
Observation of salt gland: A digital camera captured the optical images of living mangroves. Mature mangrove (Ceriops tagal (Perr.) C. B. Rob) leaves and some mangrove branches were collected from Shenzhen Bay. For scanning electron microscopy, the mangrove leaf samples were sputter-coated by a Leica EMACE200 automatic low-vacuum coating apparatus (platinum, the 30s) (Leica, Wetzlar, Germany) and then observed by an electron microscope (ZEISS SUPRA55, Carl Zeiss, Jena, Germany). The surface covering salt glands was stripped by a focused ion beam (Scios, FEI), and the images of the inner structure were captured by scanning electron microscopy. According to the procedure of previous studies, mangrove leaves were stained with safranin and fast green (Xiao et al., 2009; Yuanyue et al., 2009; Yin et al., 2012) and observed under a light microscope. The samples were first fixed with FAA solution (formalin (38% formaldehyde), glacial acetic acid, and 70% ethanol, volume ratio of 1:1:18) for 24 h and then dehydrated with a series of gradient ethanol solutions (50 vol%, 70 vol%, 85 vol%, 95 vol%, and 100 vol% twice), each spending 1 hour. Then, they were dealt with transparent treatment (2/3 vol ethanol: 1/3 vol xylene, 1/2 vol ethanol: 1/2 vol xylene, 1/3 vol ethanol: 2/3 vol xylene, xylene, xylene, each for 30 min). The samples were further dipped into wax at 58°C (1/2 vol xylene: 1/2 vol paraffin, 30 min; paraffin, 3 h; paraffin, 24 h), embedded into solid-state wax, sectioned by a microtome (15 µm in thickness), and fixed on slides. Then, these samples were dewaxed by immersing in xylene twice (each lasted for 10–15 min), rehydrated through gradient ethanol solutions (100 vol% twice, 95 vol%, 85 vol%, 70 vol%, and 50 vol%, each spent 1 h), and stained with safranin (1%, 1 h), fast green (0.1%, 2–5 s), or basic fuchsin (2%, 30 min). They were further dehydrated through gradient ethanol solutions (50 vol%, 70 vol%, 85 vol%, 95 vol%, and 100 vol% twice, 1 h each step) and dealt with the same transparent treatment. Finally, the specimens were mounted with Canada balsam and observed under an optical microscope (Olympus BX53). The collected mangrove leaf was sliced to 1 cm2 and was separated from the middle. The upper part of the mangrove leaf was incubated in the culture equipment as shown in Supplementary Figure S1 and was observed under a 3D microscope system.
Culturing ornamental plants: ornamental plants (Bracketplant, Sedum makinoi ogon, Begonia, and Lobelia) from a flower shop were cultured in 3.5 wt% NaCl for 30 days, and a digital camera captured the daily living state.
Preparation of the mangrove bark: mangrove bark was collected from Shenzhen bay and was washed and dried in the oven at 60°C for 24 h. After that, the mangrove bark was sliced into 1 cm × 0.5 cm. Moreover, the sliced mangrove bark was mixed with 70% acetone and stirred for 72 h to remove the mangrove tannins. Moreover, the antifouling agents in mangrove barks were extracted by the same method. Briefly, finely ground mangrove (Ceriops tagal (Perr.)) bark powder was mixed with 70% acetone and stirred for 72 h to obtain the mangrove tannins solution. Then, the obtained solution was concentrated at 40°C under reduced pressure in a rotary evaporator to remove the acetone. Finally, the concentrated extraction was frozen for 24 h before being freeze-dried for 48 h.
Test of the bacteria resistant ability of mangrove bark: The antibacterial activity of mangrove bark was evaluated by the spread plate method. The counted number of the bacterial colony reflects the strength of the antibacterial activity. We assumed the initial concentration of the pure culture suspension of E. coli (atcc25922) pathogens to be about 105–106 CFU/ml. Briefly, the bacterial suspensions with different mangrove bark samples or mangrove tannins were incubated in a shaking incubator overnight at 200 rpm and 37°C. About 100 ml of the overnight E. coli suspension described above was first pipetted into an agar plate and then spread throughout the surface. These agar plates were then placed in an incubator at 37°C for 24 h. After that, the agar plates were visually observed for bacteria colonies. This experiment was repeated three times to ensure the accuracy of the results.
Electrochemical experiments: The electrochemical measurements were performed by using a CHI760E workstation with CHI software. Three tested Q235 steel coupons were cut into specimens of dimensions 10 mm × 10 mm and soldered to a copper wire. Moreover, the samples were covered with epoxy resin, leaving only 1 cm2 uncovered. The uncovered surface area was abraded to smoothness, degreased in ethanol, rinsed with distilled water, and dried in warm air. Electrochemical experiments were carried out in a conventional three-electrode cell with a platinum (2 cm × 2 cm) counter electrode and a saturated calomel electrode coupled to a fine Luggin capillary as the reference electrode. All measurements were performed at 303 K and run in triplicate to confirm the reproducibility. All electrochemical tests were performed after 3,600 s to allow stabilization of the systems. Electrochemical impedance spectroscopy (EIS) measurements were conducted over a frequency range of 100 kHz–10 mHz, with a signal amplitude perturbation of 5 mV. Potentiodynamic polarization experiments were carried out at the scan rate of 1 mV/s over the potential scope of −300 mV to 1.2 V against the OCP. The solutions 0.5 M H2SO4 in 3.5 wt% NaCl (SS) containing 0 gL−1, 3 gL−1 mangrove extract, and 3 gL−1 commercial tannins were prepared as the electrolytes. All the electrochemical tests were performed three times to ensure the accuracy of the results.
3 Results and Discussions
3.1 Survival Approaches of Mangrove
3.1.1 Functional Structure and Salt-Secreting Ability
Mangrove forests are located along the marine coastline, enduring high humidity, severe salinity, and periodic erosion of seawater in the marine environment all day long. However, mangrove exhibits strong vitality for their survival advantages (Figure 1) (Mimura et al., 2003; Parida et al., 2004; Parida and Jha, 2010) compared to the cultivated ornamental plants (Bracketplant, Sedum makinoi ogon, Begonia, and Lobelia) that gradually withered after being cultured in salt solution (3.5 wt% NaCl). For lacking self-protection function under salt solution, the ornamental plants did not adapt to high salinity and weakened because of losing cellular water. Distinctively, all the ornamental plants faded 10 days later; interestingly, some of the ornamental plants, such as Lobelia, began to wilt only after 2 days of cultivation. In contrast, the mangrove has a tenacious vitality in marine surroundings and exhibits an excellent ability to resist frequent washes and high salinity of the ocean.
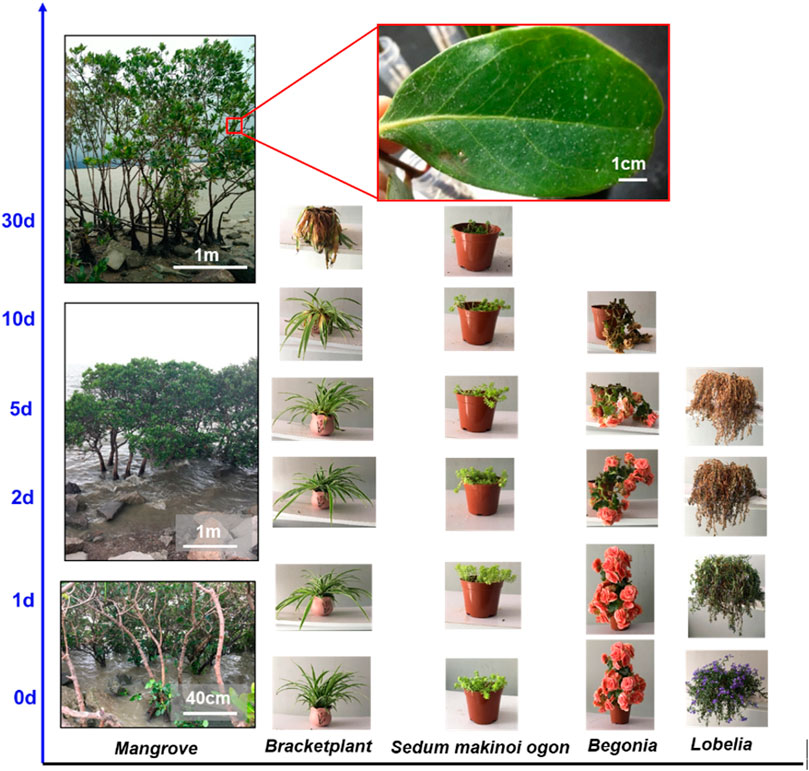
FIGURE 1. Salt-resistant mangrove in comparison with other four types of common plants: their growing conditions under salted water supply with increasing culture time. The mangrove lives well and the leaves show deposited salt particles, while the four common plants (Bracketplant, Sedum makinoi ogon, Begonia, and Lobelia) gradually withered/died from the 5th day (d) up to the 30th day.
We collected some mangrove samples, including leaves, branches, and roots, from Shenzhen Bay to reveal the underlying salt-secreting reason. After cleaning, washing, and drying the mangrove leaves, we found that many salt particles were deposited, as in our previous reports (Cui et al., 2019b). The deposited salt particles are the excess salt substances that were secreted by a plethora of salt glands to help mangroves survive in the marine surroundings (Sobrado, 2007; Liang et al., 2008). To explore the salt gland functional structure, we fixed, dehydrated, and stained some mangrove leaf slices to observe the microstructure. As shown in the optical staining photos in Figure 2, the salt glands exist on both the top and lower mangrove leaf (Figure 2A), which is different from the situation of the stoma, a breathing tissue on the lower surface. Moreover, mangrove salt glands are multicellular tissues, typically including cell types differentiated into basal collecting cells and distal secretory cells (Figure 2B), consisting of some reports. In these cells, the collecting cells are presumed to create a salt efflux gradient to collect salt from neighboring mesophyll cells and transport it to the secretory cells. The secretory cells are surrounded by a cuticle, except where they contact the subtending basal collecting cells, which is a feature that appears to channel the flow of salt through the secretory cells and prevent leakage back into the neighboring tissue via the apoplast (Parida and Jha, 2010). The observed cuticular cavities in the mangrove salt gland represent a temporary collecting compartment where the secreted salts accumulate before elimination from the leaf. Furthermore, observing the structure of the salt gland, we found that salt glands are approximately 30–40 μm in diameter. In particular, mangrove salt glands have three types of morphologies, namely, hump, flat, and sunken salt glands on mangrove leaves (Figure 2C). This is not to be confused with the well-known three types of glands of plants in a general sense (Thomson, 1975; Thomson et al., 1988), including the two-celled glands of the grasses (I), the bladder cells of the Chenopodiaceae (II), and the multicellular glands in other dicotyledonous families (III). We here report firstly three featured morphologies of salt glands belonging to the type III of the above classical categorization. Moreover, the same results can be observed from the SEM. The underlying reason is that the cuticle layer, which entirely encloses the upper portion of the glands, gradually expands with mangrove leaves and finally makes the salt gland sink in the mangrove leaf (Naidoo and Naidoo, 1998; Parida et al., 2004; Tan et al., 2015). Moreover, the cuticular cavity becomes smaller and ultimately could not be observed in the sunken salt gland.
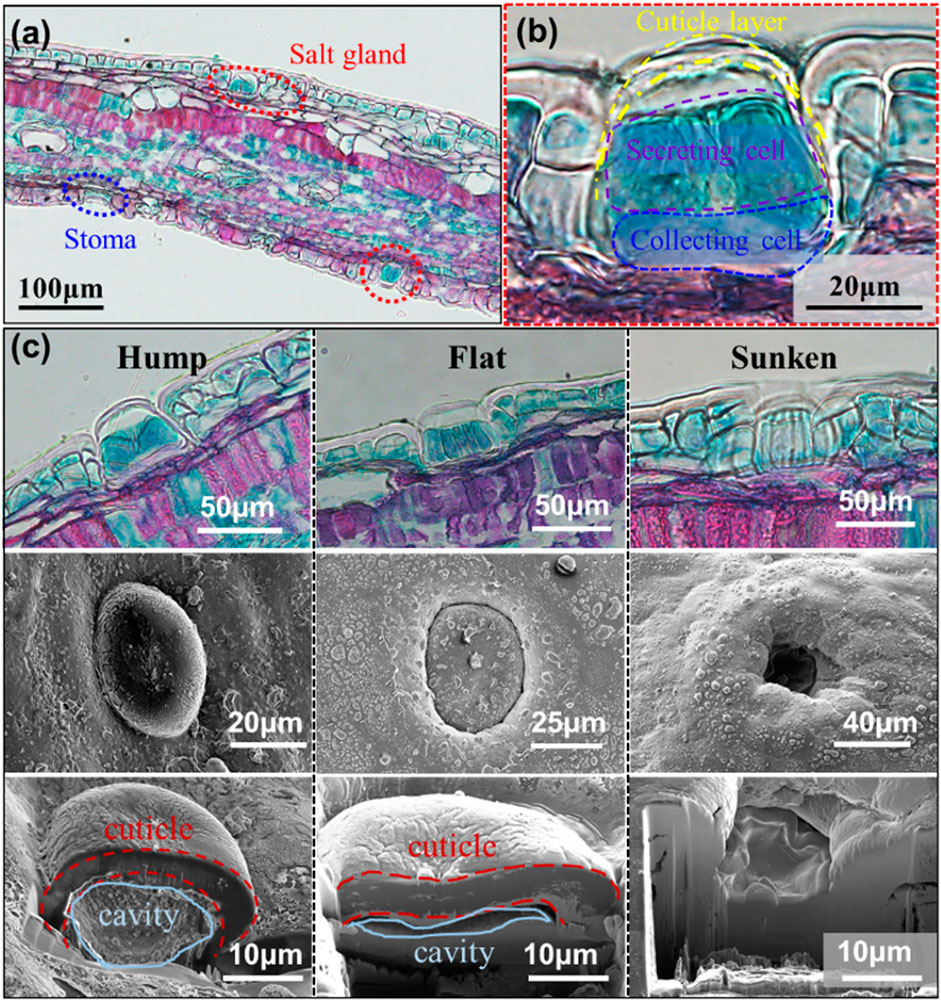
FIGURE 2. The morphologies of the mangrove salt glands which are within the leaf surfaces. Optical images of (A) a sectional view of the stained leaf and (B) a magnified view of the salt gland showing the cellular structure. (C) Optical and SEM images of the hump salt gland, the flat salt gland, and the sunken salt gland.
Furthermore, we isolated adaxial mangrove leaf peels to identify the salt-secreting ability of mangrove salt glands by studying the dynamic secreting process. The salt glands with three different morphologies, including hump, flat, and sunken, were also observed under a 3D microscope, as shown in Figure 3A. For the protruding of the cuticle, the hump salt gland is entirely green without a black circle surrounding it. By contrast, the flat salt gland was surrounded by a black circle for the sinking of the cuticle, and the sunken salt gland is a black hole in mangrove leaves. To explore the dynamic salt-secreting process, the isolated mangrove leaves tissues were made to float on 3.5 wt% NaCl solution and covered with a layer of paraffin oil that is separated from the water underneath and floated above the observed tissue. In the salt-secreting process, the salt glands could generate a water circle by secreting excessive salt solution for the incompatibility of oil–water phases, as shown in Figure 3B. Real-time imaging of several salt glands showed secretions appearing above salt glands, including hump and flat salt glands, forming a transparent circle above each of them. Moreover, the salt gland secreted more and more salt solution, reflected by the increase in diameter of the fluid over time (Figure 3C). Although we did not observe the dynamic salt-secreting process of the sunken salt gland, we captured the sunken salt gland covered by secretions (Figure 3D), which implies that the sunken gland also has salt-secreting ability. Therefore, mangrove salt glands, including hump, flat, and sunken, could adjust the excessive salt solution by processing salt-secretion.
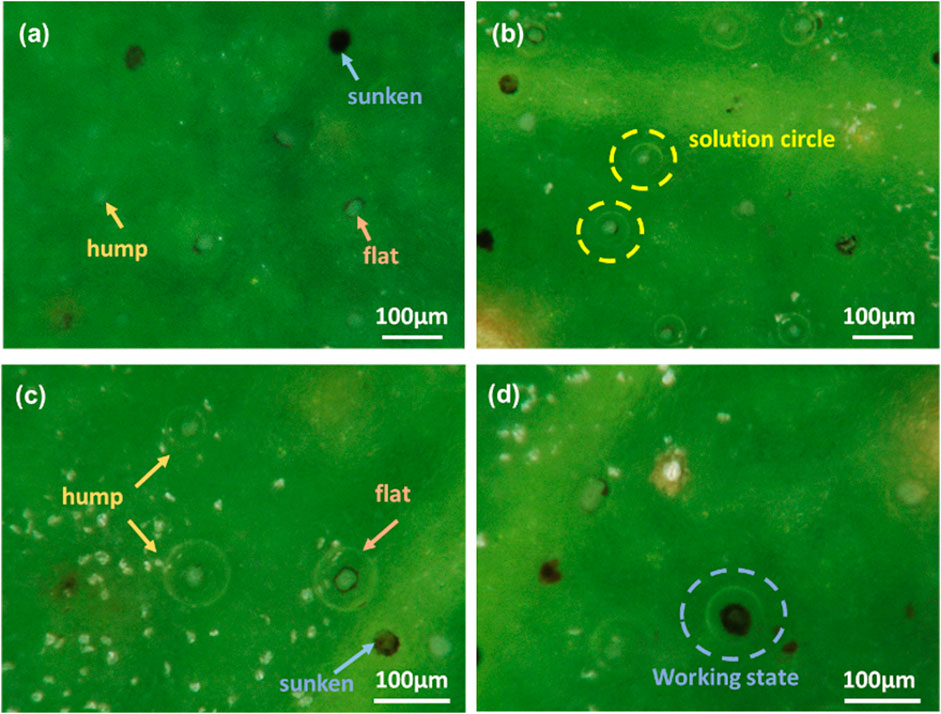
FIGURE 3. The optical images of mangrove leaves with the three types of salt glands (a) at the beginning of salt secretion and (b–d) after salt secretion.
3.1.2 Active–Active Ingredients and Bacteria-Resisting Ability
Mangrove has another self-protection measure from the root that contains some active–active ingredients alleviating the detrimental damage from the reductive and acidic soil conditions (Liang et al., 2008). Unlike mangrove leaves being exposed to the marine atmosphere, mangrove roots are immersed in seawater and rooted into the deep ocean soil, facing many microorganisms and the pressure washed away by seawater. Therefore, mangrove roots must have a solid ability to resist the adhesion or aggression of harmful bacteria to help mangroves survive in such harsh surroundings. The mangrove roots grow with abundant polyphenols (mangrove tannins) that could combine with ferric ions in the soil solution to alleviate excess iron damage and is an essential factor regulating intertidal meiobenthic communities (Alongi, 1987; Kimura and Wada, 1989). Moreover, mangrove tannins especially had potential antibacterial ability on marine bacteria (Noor Idora et al., 2015; Prakash et al., 2015). We verified the potential antibacterial power of natural mangrove tannins using the spread plate method in this research. Figure 4 shows that the number of growing bacteria colonies of solution co-cultured with natural bark is less than that of the solution co-cultured with extracted bark samples. There are 212 E. coli colonies (Figure 4A) on the medium plate, which increases to 400 colonies in the absence of mangrove tannins (Figure 4B). Due to being rich in tannins, mangrove barks can resist the harmful effects imposed by marine bacteria (E. coli). Therefore, the antibacterial function also contributes to the survival of mangroves. Furthermore, mangrove tannins were extracted to function as a natural antibacterial agent. We found that no E. coli colonies grew on agar plates after the bacteria solution was co-cultured with extracted tannins (Figure 4D ). By contrast, numerous bacteria colonies grew on agar plates without extracted tannins (Figure 4C), and those results manifested the advanced antibacterial property of extracted mangrove tannins.
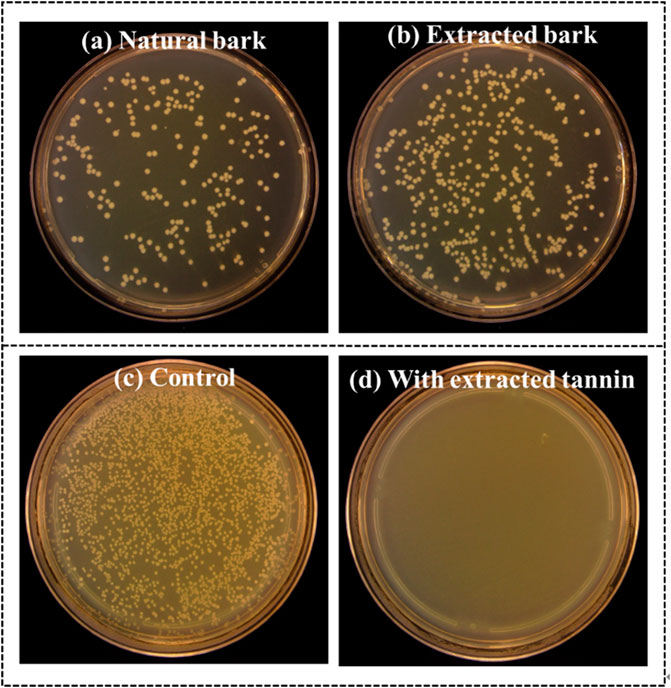
FIGURE 4. Bacteria colonies on the medium plates. (a) E. coli co-cultured with natural mangrove bark. (b) E. coli co-cultured with extracted mangrove bark. (c) Cultured E. coli. (d) E. coli co-cultured with extracted mangrove tannin.
3.2 Mangrove-Inspired Corrosion Inhibitor
In this work, we further explored the roles of mangrove tannins and developed the mangrove-inspired corrosion inhibitor to protect metals from corrosion (Ridhwan et al., 2012). Although the inhibitive function of mangrove tannins has been manifested, there is an urgent need to verify the application advantages of mangrove tannins over the current commercial tannins. We tested the protective property of mangrove tannins and commercial tannins in acidic 3.5 wt% NaCl solution to demonstrate the potential practical application value of mangrove tannins. Figure 5A presents the potentiodynamic polarization curves in 3.5 wt% NaCl solution containing 0.5 M sulfuric acid, from which the fitting kinetic parameters (Icorr and Ecorr) were obtained. Moreover, the current density can be applied to calculate the anti-corrosion efficiency(ƞ) according to Eq. 1, and the computed results and the fitting Ecorr are shown in Figure 5B. It is noted in Table 1 that with the addition of commercial tannins (CT) and mangrove tannins (MT), the corrosion current density was significantly reduced, and the corresponding calculated anti-corrosion efficiency could reach 43.76 and 60.51%, respectively. Our preliminary mangrove tannins-containing epoxy coatings could sustain immersion in 3 wt% NaCl solution for three months without blistering or falling off. The abovementioned results revealed that mangrove tannins has a more efficient protective ability than commercial tannins for Q235 steel in acidic 3.5 wt% NaCl solution (SS) and has some practical application advantages over commercial tannins.
where icorr0 and icorr are the corrosion current densities in the absence and presence of the inhibitor, respectively.
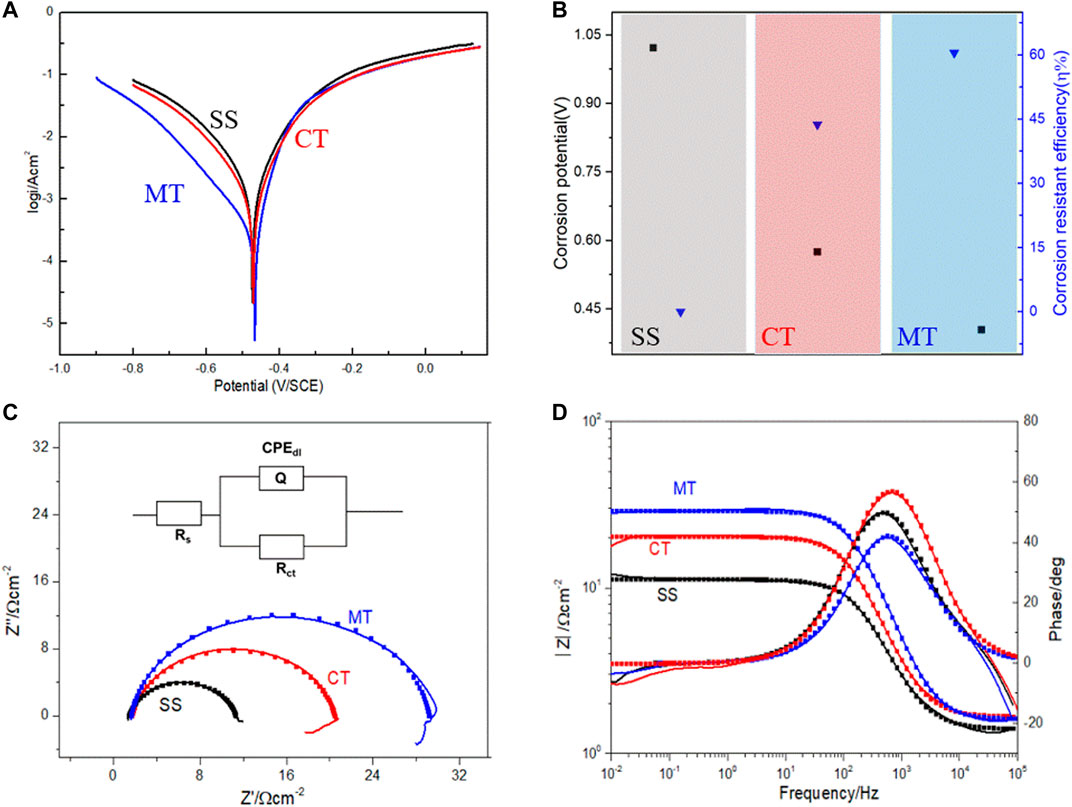
FIGURE 5. Electrochemical corrosion performance results. (A) Polarization curves, (B) fitting parameters of polarization curves, (C) Nyquist and (D) Bode plots of Q235 steel exposed in 3.5% NaCl solution with 0.5 M sulfuric acid and with 3gL−1 mangrove tannins or 3gL−1 commercial tannins.

TABLE 1. Fitting results of obtained Nyquist and Bode plots by exposing Q235 steel to acidic 3.5 wt% NaCl solution without or with mangrove tannins.
We also verified the prohibitive ability of mangrove tannins by electrochemical impedance spectroscopy (EIS), which is a versatile and standard characterization method in exploring electrochemical corrosion and is described in Nyquist and Bode plots (Huang et al., 2016). The Nyquist actions in Figure 5C show that charge transfer occurs at the electrode/solution interface and signifies the charge transfer process as the main controlling factor in the corrosion process. Therefore, we applied the one-time constant model shown in Figure 5C to describe this three-electrode system. Moreover, ZSimDemo software was used to fit the impedance spectra to obtain the circuit parameters, and the fitting errors were controlled by 10%. Among the fitted circuit parameters, a constant phase angle component CPE is used to replace the electrode/solution interface capacitance to analyze the interfacial double-layer impedance effect (Deyab et al., 2016) accurately. In particular, “n” obtained by fitting was applied to demonstrate the impact of the diffusing phenomenon, whose range is −1–1. The value of n has specific effect on the capacity of CPEdl obtained by calculating according to Eq. 2 (Okafor et al., 2009; Torres et al., 2014).
As shown in Table 1, the calculated capacity of CPEdl decreases with the addition of CT and MT. The underlying reason is the adsorption of tannin, which replaced water at the metal–solution interface. Formation of the tannin film leads to a decrease in the local dielectric constant and an increase in the thickness of the electrical double layer (Oguzie et al., 2007; Deyab et al., 2016). Hence, the capacitance of the double electric layer decreases after adding tannins into the solution (Mallaiya et al., 2011). In addition, the charge transfer resistance (Rct) could directly reveal the inhibition ability of the applied inhibitor, and the larger Rct, the more extraordinary anti-corrosion ability (El-Haddad and Fouda, 2015). The value of Rct increases with CT and MT, indicating that existing CT and MT could prohibit the corrosion of Q235. Moreover, the general shape of Nyquist plots is the same, showing that the corrosion mechanisms were almost the same in the presence of various inhibitors (Amin, 2010). Generally, the addition of mangrove tannins causes a more significant increase in the Rct value (Figure 5D) while diminishing the value of CPEdl. The decrease in CPEdl, which can result from a decrease in local dielectric constant and an increase in the thickness of the electrical double layer, suggested that the inhibitor molecules function by adsorption at the metal/solution interface. The Rct values were used to calculate the anti-corrosion efficiency that agrees with those calculated from potentiodynamic polarization curves.
where Y0 is a constant obtained by impedance spectrum fitting and fmax is the frequency at which the imaginary component of the impedance is a maximum.
where d is the thickness of the film, S is the surface area of the electrode, ε0 is the dielectric constant of the air, and ε is the dielectric constant of the solution medium.
where Rct0 and Rct are the charge transfer resistance in the absence and presence of the inhibitor, respectively.
4 Conclusion
In summary, we investigated the survival strategies of mangroves, namely, salt-secretion and mangrove tannins. Onthe one hand, salt-secretion realized by mangrove salt glands could make the mangrove excrete excessive salt and avoid harmful effects. Mangrove salt glands are distributed on both surfaces of the leaves (Ceriops tagal (perr.) C. B. Rob) and have three types of morphologies, namely, hump, flat, and sunken states. More significantly, dynamic salt-secretion was observed by a simple method, in which there is no need to separate a single salt gland. Moreover, we verified the salt-secretion ability of hump, flat, and sunken salt glands, and all three types of salt glands could be in inactive working states in any kind of morphology.
On the other hand, mangrove tannins existing in large quantities in mangrove bark could help the mangrove survive the damage of some marine bacteria. Moreover, extracted mangrove tannins have an excellent ability to resist the growth of E. coli. It is hard to find E. coli growing after the co-culture with extracted mangrove tannins. Finally, we explored the performance of mangrove tannins and commercial tannins in corrosion inhibition. We discovered that mangrove tannins could be adsorbed at the metal–solution interface by replacing water and could function as a cathodic inhibitor with the anti-corrosion efficiency of 60.51% in acid 3.5 wt% NaCl solution. Compared with commercial tannins, mangrove tannins provide a much higher anti-corrosion efficiency.
Data Availability Statement
The original contributions presented in the study are included in the article/Supplementary Material, further inquiries can be directed to the corresponding authors.
Author Contributions
Conceptualization: MC and ZW; data curation: MC and BW; investigation and methodology: MC, ZW, and BW; writing—original draft: MC, writing—review and editing.
Funding
BW appreciated the financial supports from the Shenzhen-Hong Kong Joint Research Project (No. SGDX2019091716460172) and the Guangdong Basic and Applied Basic Research Foundation (No. 2019A1515012093). ZW is grateful for financial support from the Innovation Technology Fund (9440260).
Conflict of Interest
The authors declare that the research was conducted in the absence of any commercial or financial relationships that could be construed as a potential conflict of interest.
The handling editor declared a past co-authorship with one of the authors CM
Publisher’s Note
All claims expressed in this article are solely those of the authors and do not necessarily represent those of their affiliated organizations, or those of the publisher, the editors, and the reviewers. Any product that may be evaluated in this article, or claim that may be made by its manufacturer, is not guaranteed or endorsed by the publisher.
Supplementary Material
The Supplementary Material for this article can be found online at: https://www.frontiersin.org/articles/10.3389/fmats.2022.879525/full#supplementary-material
References
Adam, M. R., Rahim, A. A., and Shah, A. M. (2014). Synergy between Iodide Ions and Mangrove Tannins as Inhibitors of Mild Steel Corrosion. Ann. For. Sci. 72 (1), 9–15. doi:10.1007/s13595-014-0398-9
Akalezi, C. O., and Oguzie, E. E. (2015). Evaluation of Anticorrosion Properties of Chrysophyllum Albidum Leaves Extract for Mild Steel Protection in Acidic Media. Int. J. Ind. Chem. 7 (1), 81–92. doi:10.1007/s40090-015-0057-5
Alongi, D. M. (1987). The Influence of Mangrove-Derived Tannins on Intertidal Meiobenthos in Tropical Estuaries. Oecologia 71 (4), 537–540. doi:10.1007/bf00379293
Amin, M. A. (2010). A Newly Synthesized glycine Derivative to Control Uniform and Pitting Corrosion Processes of Al Induced by SCN− Anions - Chemical, Electrochemical and Morphological Studies. Corros. Sci. 52 (10), 3243–3257. doi:10.1016/j.corsci.2010.05.041
Cui, M., Njoku, D. I., Li, B., Yang, L., Wang, Z., Hou, B., et al. (2021). Corrosion Protection of Aluminium Alloy 2024 through an Epoxy Coating Embedded with Smart Microcapsules: The Responses of Smart Microcapsules to Corrosive Entities. Corros. Commun. 1, 1–9. doi:10.1016/j.corcom.2021.06.001
Cui, M., Wang, P.-Y., Wang, Z., and Wang, B. (2019b). Mangrove Inspired Anti-Corrosion Coatings. Coatings 9 (11), 725. doi:10.3390/coatings9110725
Cui, M., Wang., B., and Wang, Z. (2019a). Nature‐Inspired Strategy for Anticorrosion. Adv. Eng. Mat. 21 (7), 1801379. doi:10.1002/adem.201801379
Deyab, M. A., Ouarsal, R., Lachkar, M., El Bali, B., and Essehli, R. (2016). Phosphites Compound: Novel Corrosion Inhibitor for Radioactive Waste Container (Carbon Steel) in Simulated Callovo-Oxfordian (COx) Groundwater. J. Mol. Liq. 219, 994–999. doi:10.1016/j.molliq.2016.04.027
El-Haddad, M. N., and Fouda, A. S. (2015). Electroanalytical, Quantum and Surface Characterization Studies on Imidazole Derivatives as Corrosion Inhibitors for Aluminum in Acidic Media. J. Mol. Liq. 209, 480–486. doi:10.1016/j.molliq.2015.06.005
Flores Merino, S., Caprari, J. J., Vasquez Torres, L., Figueroa Ramos, L., and Hadzich Girola, A. (2017). Inhibitive Action of Tara Tannin in Rust Converter Formulation. Anti-Corrosion Methods Mater. 64 (2), 136–147. doi:10.1108/acmm-06-2015-1548
Haldhar, R., Prasad, D., Mandal, N., Benhiba, F., Bahadur, I., and Dagdag, O. (2021). Anticorrosive Properties of a Green and Sustainable Inhibitor from Leaves Extract of Cannabis Sativa Plant: Experimental and Theoretical Approach. Colloids Surfaces A Physicochem. Eng. Aspects 614, 126211. doi:10.1016/j.colsurfa.2021.126211
Huang, J., Li, Z., Liaw, B. Y., and Zhang, J. (2016). Graphical Analysis of Electrochemical Impedance Spectroscopy Data in Bode and Nyquist Representations. J. Power Sources 309, 82–98. doi:10.1016/j.jpowsour.2016.01.073
Jaén, J. A., De Obaldía, J., and Rodríguez, M. V. (2011). Application of Mössbauer Spectroscopy to the Study of Tannins Inhibition of Iron and Steel Corrosion. Hyperfine Interact. 202 (1-3), 25–38. doi:10.1007/s10751-011-0337-1
Kimura, M., and Wada, H. (1989). Tannins in Mangrove Tree Roots and Their Role in the Root Environment. Soil Sci. Plant Nutr. 35 (1), 101–108. doi:10.1080/00380768.1989.10434741
Kusmierek, E., and Chrzescijanska, E. (2015). Tannic Acid as Corrosion Inhibitor for Metals and Alloys. Mater. Corros. 66 (2), 169–174. doi:10.1002/maco.201307277
Li, Y., Ma, Y., Zhang, B., Lei, B., and Li, Y. (2014). Enhancement the Adhesion between Epoxy Coating and Rusted Structural Steel by Tannic Acid Treatment. Acta Metall. Sin. Engl. Lett.) 27 (6), 1105–1113. doi:10.1007/s40195-014-0132-5
Liang, S., Zhou, R., Dong, S., and Shi, S. (2008). Adaptation to Salinity in Mangroves: Implication on the Evolution of Salt-Tolerance. Sci. Bull. 53 (11), 1708–1715. doi:10.1007/s11434-008-0221-9
Majd, M. T., Ramezanzadeh, M., Ramezanzadeh, B., and Bahlakeh, G. (2020). Production of an Environmentally Stable Anti-Corrosion Film Based on Esfand Seed Extract Molecules-Metal Cations: Integrated Experimental and Computer Modeling Approaches. J. Hazard. Mater. 382, 121029. doi:10.1016/j.jhazmat.2019.121029
Mallaiya, K., Subramaniam, R., Srikandan, S. S., Gowri, S., Rajasekaran, N., and Selvaraj, A. (2011). Electrochemical Characterization of the Protective Film Formed by the Unsymmetrical Schiff's Base on the Mild Steel Surface in Acid Media. Electrochimica Acta 56 (11), 3857–3863. doi:10.1016/j.electacta.2011.02.036
Mimura, T., Kura-Hotta, M., Tsujimura, T., Ohnishi, M., Miura, M., Okazaki, Y., et al. (2003). Rapid Increase of Vacuolar Volume in Response to Salt Stress. Planta 216 (3), 397–402. doi:10.1007/s00425-002-0878-2
Naidoo, Y., and Naidoo, G. (1998). Sporobolus Virginicus Leaf Salt Glands: Morphology and Ultrastructure. South Afr. J. Bot. 64 (3), 198–204. doi:10.1016/s0254-6299(15)30867-x
Noor Idora, M. S., Ferry, M., Wan Nik, W. B., and Jasnizat, S. (2015). Evaluation of Tannin from Rhizophora A as Natural Antifouling Agents in Epoxy Paint for Marine Application. Prog. Org. Coatings 81, 125–131. doi:10.1016/j.porgcoat.2014.12.012
Oguzie, E. E., Li, Y., and Wang, F. H. (2007). Effect of 2-Amino-3-Mercaptopropanoic Acid (Cysteine) on the Corrosion Behaviour of Low Carbon Steel in Sulphuric Acid. Electrochimica Acta 53 (2), 909–914. doi:10.1016/j.electacta.2007.07.076
Okafor, P. C., Liu, X., and Zheng, Y. G. (2009). Corrosion Inhibition of Mild Steel by Ethylamino Imidazoline Derivative in CO2-Saturated Solution. Corros. Sci. 51 (4), 761–768. doi:10.1016/j.corsci.2009.01.017
Parida, A. K., Das, A. B., and Mittra, B. (2004). Effects of Salt on Growth, Ion Accumulation, Photosynthesis and Leaf Anatomy of the Mangrove, Bruguiera Parviflora. Trees - Struct. Funct. 18 (2), 167–174. doi:10.1007/s00468-003-0293-8
Parida, A. K., and Jha, B. (2010). Salt Tolerance Mechanisms in Mangroves: A Review. Trees 24 (2), 199–217. doi:10.1007/s00468-010-0417-x
Prakash, S., Ramasubburayan, R., Iyapparaj, P., Ramaswamy Arthi, A. P., Ahila, N. K., Ramkumar, V. S., et al. (2015). Environmentally Benign Antifouling Potentials of Triterpene-Glycosides from Streptomyces Fradiae: A Mangrove Isolate. RSC Adv. 5 (37), 29524–29534. doi:10.1039/c4ra15335a
Rahim, A. A., Rocca, E., Steinmetz, J., Kassim, M. J., Adnan, R., and Sani Ibrahim, M. (2007). Mangrove Tannins and Their Flavanoid Monomers as Alternative Steel Corrosion Inhibitors in Acidic Medium. Corros. Sci. 49 (2), 402–417. doi:10.1016/j.corsci.2006.04.013
Ridhwan, A. M., Rahim, A. A., and Shah, A. M. (2015). Synergy Between Iodide Ions and Mangrove Tannins as Inhibitors of Mild Steel Corrosion. Ann. Forest Sci. 72 (1), 9–15. doi:10.1007/s13595-014-0398-9
Shah, A. M., Rahim, A. A., Hamid, S. A., and Yahya, S. (2013). Green Inhibitors for Copper Corrosion by Mangrove Tannin. Int. J. Electrochem. Sci. 8 (2), 2140.
Shah, A. M., Rahim, A. A., Yahya, S., Raja, P. B., and Hamid, S. A. (2011). Acid Corrosion Inhibition of Copper by Mangrove Tannin. Pigment Resin Technol. 40 (2), 118–122. doi:10.1108/03699421111113783
Sobrado, M. A. (2007). Relationship of Water Transport to Anatomical Features in the Mangrove Laguncularia Racemosa Grown under Contrasting Salinities. New Phytol. 173 (3), 584–591. doi:10.1111/j.1469-8137.2006.01927.x
Tan, K. W., and Kassim, M. J. (2011). A Correlation Study on the Phenolic Profiles and Corrosion Inhibition Properties of Mangrove Tannins (Rhizophora A) as Affected by Extraction Solvents. Corros. Sci. 53 (2), 569–574. doi:10.1016/j.corsci.2010.09.065
Tan, W.-K., Lim, T.-K., Loh, C.-S., Kumar, P., and Lin, Q. (2015). Proteomic Characterisation of the Salt Gland-Enriched Tissues of the Mangrove Tree Species Avicennia O. PLoS One 10 (7), e0133386. doi:10.1371/journal.pone.0133386
Thomson, W. W. (1975)“The Structure and Function of Salt Glands,”. Plants in Saline Environment. Editors A. Poljakof-Maybcr, and J. Gale (Berlin: Springer), 118–146.
Thomson, W. W., Faraday, C. D., and Oross, J. W. (1988). “Salt Glands,” in Solute Transport in Plant Cells and Tissues. Editors D. A. Baker, and J. L. Hall (Essex, United Kingdom: Longman Scientific & Technical), 498–537.
Torres, V. V., Rayol, V. A., Magalhães, M., Viana, G. M., Aguiar, L. C. S., Machado, S. P., et al. (2014). Study of Thioureas Derivatives Synthesized from a Green Route as Corrosion Inhibitors for Mild Steel in HCl Solution. Corros. Sci. 79, 108–118. doi:10.1016/j.corsci.2013.10.032
Xiao, Y., Jie, Z., Wang, M., Lin, G., and Wang, W. (2009). Leaf and Stem Anatomical Responses to Periodical Waterlogging in Simulated Tidal Floods in Mangrove Avicennia M Seedlings. Aquat. Bot. 91 (3), 231–237. doi:10.1016/j.aquabot.2009.07.001
Yin, D., Zhang, Z., and Luo, H. (2012). Anatomical Responses to Waterlogging in Chrysanthemum Zawadskii. Sci. Hortic. 146, 86–91. doi:10.1016/j.scienta.2012.08.019
Keywords: mangrove, bacteria fouling, corrosion-resistant, bioinspired, the green inhibitor
Citation: Cui M, Wang Z and Wang B (2022) Survival Strategies of Mangrove (Ceriops tagal (perr.) C. B. Rob) and the Inspired Corrosion Inhibitor. Front. Mater. 9:879525. doi: 10.3389/fmats.2022.879525
Received: 19 February 2022; Accepted: 19 April 2022;
Published: 03 June 2022.
Edited by:
Demian Ifeanyi Njoku, University of Science and Technology of China, ChinaReviewed by:
Ganesh Chandrakant Nikalje, R. K. Talreja College of Arts, Science and Commerce, IndiaAisha Al-Moubaraki, Jeddah University, Saudi Arabia
Copyright © 2022 Cui, Wang and Wang. This is an open-access article distributed under the terms of the Creative Commons Attribution License (CC BY). The use, distribution or reproduction in other forums is permitted, provided the original author(s) and the copyright owner(s) are credited and that the original publication in this journal is cited, in accordance with accepted academic practice. No use, distribution or reproduction is permitted which does not comply with these terms.
*Correspondence: Bin Wang, bwang55@cityu.edu.hk; Zuankai Wang, zk.wang@cityu.edu.hk