Experimental study on deterioration characteristics of foamed concrete under dry-wet cycles in acidic environment
- 1The Seventh Engineering Co., Ltd of CFHEC, Zhengzhou, China
- 2School of Civil Engineering, Chang’ an University, Xi’an, China
Foamed concrete, which has excellent performance, has been gradually used to enhance the thermal insulation performance of buildings in recent years. However, there are few studies on the durability of foamed concrete under environmental action. In order to understand the law of damage and deterioration of foamed concrete under dry-wet cycles in acidic environment, the standard sample of foamed concrete was first pre-treated with different times of dry-wet cycles under neutral and two acidic environments, then quality inspection, uniaxial compression test and scanning electron microscope were carried out on the treated foamed concrete by electronic scale, universal testing machine and electron microscope. Accordingly, the deterioration effect and deterioration mechanism of foamed concrete under dry-wet cycles in acidic environment is analyzed. The results show that the quality and uniaxial compressive strength of foamed concrete decrease gradually with the increase of the number of dry-wet cycles in both neutral and two acidic environments; the stronger the acidity of dry-wet cycles, the more the quality of foamed concrete decreases and the more obvious the deterioration effect of uniaxial compressive strength of foamed concrete; the acidic dry-wet cycles will corrode the foamed concrete, resulting in the expansion and consolidation of pores and the appearance of cracks, and the more the number of dry-wet cycles, the more obvious the corrosion effect of the foamed concrete. The research results can provide scientific basis for the analysis of the safety and stability of foamed concrete members under the dry-wet cycle in acidic environment.
1 Introduction
Foamed concrete is a new type of porous building material made by mixing foam and cement slurry uniformly (Amran et al., 2015). Compared with traditional concrete, foamed concrete has many characteristics, such as low density, light weight, large pores, strong water absorption, and many advantages, such as good seismic performance and energy absorption, sound absorption and noise reduction, thermal insulation and fire resistance (Raj et al., 2019). In recent years, foamed concrete has been successively applied to various practical projects in various countries (Weigler and Karl, 1980). However, due to the short development time, the current research on the performance of foamed concrete mostly focuses on the selection and ratio of materials (Park et al., 1999; Jones and McCarthy, 2005; Pasupathy et al., 2021; Chandni and Anand, 2018; Nambiar and Ramamurthy, 2006), ignoring various damages in practical applications. This limitation limits the application of foamed concrete in engineering to a certain extent. As an emergent building material, further research on the mechanical properties of foamed concrete is urgently needed.
Foamed concrete has special pore structure, which is often used to improve the thermal insulation, fire prevention and sound insulation of roof or wall in practical engineering (Mydin and Wang, 2011; Oginni, 2015). Therefore, foamed concrete components in practical engineering may be exposed to air for a long time, and the factors affecting their mechanical properties are complex and changeable. For example, in rainy seasons, the high porosity of foamed concrete easily leads to internal infiltration and storage of water, significantly reducing its functionality and service life. In addition, the water in nature is often not completely neutral, but presents a certain acidic or alkaline. Especially in recent years, the increase in industrial emissions of pollutants, resulting in a wide range of acid rain areas around the world, which will seriously threaten the safety and stability of buildings. When foamed concrete is subjected to acidic conditions, its mechanical properties will be significantly affected by the corrosive action of acid. In addition, in rainy seasons, foamed concrete is often in an alternating working environment of dry and wet. Numerous studies have shown that, compared with continuous soaking, the dry-wet cycle has a greater degree of corrosion on concrete (Cody et al., 2001; Polder and Peelen, 2002; Sahmaran et al., 2007; Wang et al., 2011).
For ordinary Portland concrete, researchers have systematically studied its performance under dry-wet cycle, acidic action and alkaline action. Through different experiments, some significant results are obtained from the aspects of strength deterioration, environmental damage mechanism, damage constitutive model and durability. For example, Okochi et al. (2000) used a spray device to test the mechanical properties of concrete under acidic conditions and obtained the effect of acid deposition on the deterioration of concrete structure. In previous studies, weight loss, reduction in compressive strength, and change in dynamic modulus of elasticity were used to evaluate the extent of concrete deterioration due to sulfuric acid attack (Meyer and Ledbetter, 1970; Fattuni and Hughes, 1983). The deterioration of concrete under the action of acid dry-wet cycle was studied by Emmanuel and Sami (1988). The results indicated that the degree of concrete deterioration is increased by alternate wet-dry cycles of exposure to sulfuric acid. Generally speaking, the weight loss of the test specimens increases with a decreasing pH level of the acid solutions (Raju and Dayaratnam, 1984). However, Kong and Orbison (1987) found that a solution with a pH of 3 causes a greater weight loss of concrete than a solution with a pH of 2. Tixier et al. (2000) pointed out that under acidic conditions, the concentration of acid ions entering concrete surface is proportional to the porosity of concrete. Hua et al. (2019) presented an experimental study on the flexural durability of beams strengthened with TRC subjected to dry-wet cycles, the results indicated that increasing the number of dry-wet cycles can significantly exacerbate the diffusion process of chloride ions in concrete and aggravate the deterioration effect of concrete. These tests provide a basic method for scientific research on the damage and deterioration of concrete in acidic environment. However, considering the particularity of the internal pore structure of foamed concrete, whether the existing research results can guide the application of foamed concrete in complex environments is still to be discussed, and the mechanical properties of foamed concrete under the action of dry-wet cycle are still to be studied.
Compressive strength is one of the most important parameters to evaluate the performance of foamed concrete. There are many factors affecting the compressive strength of foamed concrete, such as density, aggregate grading and water-cement ratio. Many studies have found that density is an important factor affecting the compressive strength of foamed concrete (Chung et al., 2019; Samson and Cyr, 2018; Kilincarslan et al., 2018). Generally speaking, the higher the density, the higher the compressive strength of foamed concrete. In addition, the change of the density of foamed concrete will affect its internal pore structure. Previous studies have shown that the porosity of foamed concrete is an internal factor affecting its compressive strength (Kearsley and Wainwright, 2002), and within a certain density range, the porosity has a significant influence on the compressive strength of foamed concrete (Kearsley and Visagie, 2002). Narayanan and Ramamurthy (2000) conducted SEM analysis on foamed concrete, the results showed that uniformly distributed spherical pores is conducive to the improvement of compressive strength of foamed concrete. To sum up, the study of the internal structure change of foamed concrete is the key to understand the mechanism of its strength change. However, there are still few researches on the pore structure change of foamed concrete under environmental degradation, especially under the action of dry-wet cycle.
Based on the above considerations, the standard sample of foamed concrete was adopted as the research object in this study, and the foamed concrete was pre-treated with different times of dry-wet cycle under neutral environment and two kinds of acidic environment. On this basis, the quality of the foamed concrete sample was measured and the uniaxial compression test and electron microscope scanning test were carried out. Then, the variation of the quality and uniaxial compressive strength of foamed concrete under the action of acid dry-wet cycle and the deterioration mechanism of the internal structure of foamed concrete are analyzed.
2 Sample pre-treatment and test process
The foamed concrete used in this study is taken from the first section of the complex of Henan Houjiang Enterprise Headquarters, which is mainly used for roof waterproofing. The pouring thickness of foamed concrete is 180 mm, the strength design grade is 1MPa, the dry density is 500 kg/m3, and the size of the cube samples is 100 × 100 × 100mm, the specific mix design is shown in Table 1. In Table 1, the water is Zhengzhou tap water, the cement is 42.5 grade ordinary Portland cement, and the blowing agent is YK type blowing agent produced by Anyang Chuangke Building Materials Co., LTD. The aggregate includes mineral powder and stone powder.
2.1 Preparation of dry-wet cycle samples
The pre-treatment of dry-wet cycle included two stages: soaking and air drying. The soaking treatment was carried out in a self-made soaking box. The volume of solution in each soaking box was 6L and the number of soaking samples in each soaking box is 6. It should be noted that due to the low bulk weight of foamed concretes, they will float on the surface of the water during the soaking process, affecting the soaking effect. In order to ensure that the acidic solution can better act on the foamed concrete and improve the accuracy of the test results, the cover plate is placed on the samples during the soaking process to ensure that the samples are fully immersed in the solution. The foamed concrete was subjected to 0 (full drying), 5, 10, and 15 dry-wet cycles respectively, of which one dry-wet cycle was defined as: the foamed concrete was soaked in the soaking box for 3 h and then air-dried for 3 h, as shown in Figure 1. The soaking solution is prepared by mixing distilled water with 37% concentrated hydrochloric acid, and configured as a neutral solution with pH = 7 (without concentrated hydrochloric acid) and two acidic solutions with pH = 5 and pH = 3 according to certain proportions. Three samples were prepared for each group of dry-wet cycles treatment under different conditions to ensure the accuracy of the test results. Before each dry-wet cycle, the pH value of three solutions should be tested and adjusted to ensure that the foamed concrete is subject to the same neutral or acidic action.
2.2 Test process
The specific test flow is shown in Figure 2. The deterioration effect tests of foamed concrete under the action of dry-wet cycle mainly include uniaxial compression test and microscopic test.
Figure 3 shows the physical diagram of the foamed concrete sample after 0 dry-wet cycle (initial sample) and 15 dry-wet cycles under three pH values. As can be seen from the Figure 3, the surface of the foamed concrete after dry-wet cycle in the three environments changed from gray to yellow brown, and the stronger the acidity of the dry-wet cycle solution, the more obvious the color change, which is caused by various chemical reactions in the acidic environment. Specifically, the foamed concrete contains iron powder, which reacts with the acid solution to form yellow trivalent iron ions (Fe3+). And the stronger the acidity, the more intense the chemical reaction. In addition, when the pH of the solution is 3, the surface of the foamed concrete is obviously shed after 15 acidic dry-wet cycles, which indicates that the dry-wet cycles under strong acid environment will cause serious corrosion to the foamed concrete. During the experiment, the pH value of the soaking solution will increase after each dry-wet cycle, which also proves that part of the acidic ions in the solution are consumed by the foamed concrete, resulting in a decrease in the acidity of the solution. Therefore, the pH of the solution should be reformulated before each dry-wet cycle.
After the pre-treatment of the acidic dry-wet cycle, the foamed concrete was subjected to uniaxial compression test by YJS-3-465 microcomputer controlled electronic universal testing machine, and the samples were dried by 101-1EBS electric blast drying oven before the uniaxial compression test. The loading rate of uniaxial compression test is 5 mm/min. During each compression test, the foamed concrete sample is placed in the center of the pressure plate of the testing machine to ensure its vertical compression. Zeiss gemini 360 scanning electron microscope (SEM) was used to test the internal structure of foamed concrete after acid dry-wet cycle. The specific operation is as follows: split the foamed concrete sample after different dry-wet cycling and drying, take small fragments with an area of about 10 cm3 and a thickness of about 2 mm, then observe the internal microscopic morphology of the cross section by scanning electron microscope. The experimental equipment is shown in Figure 4.
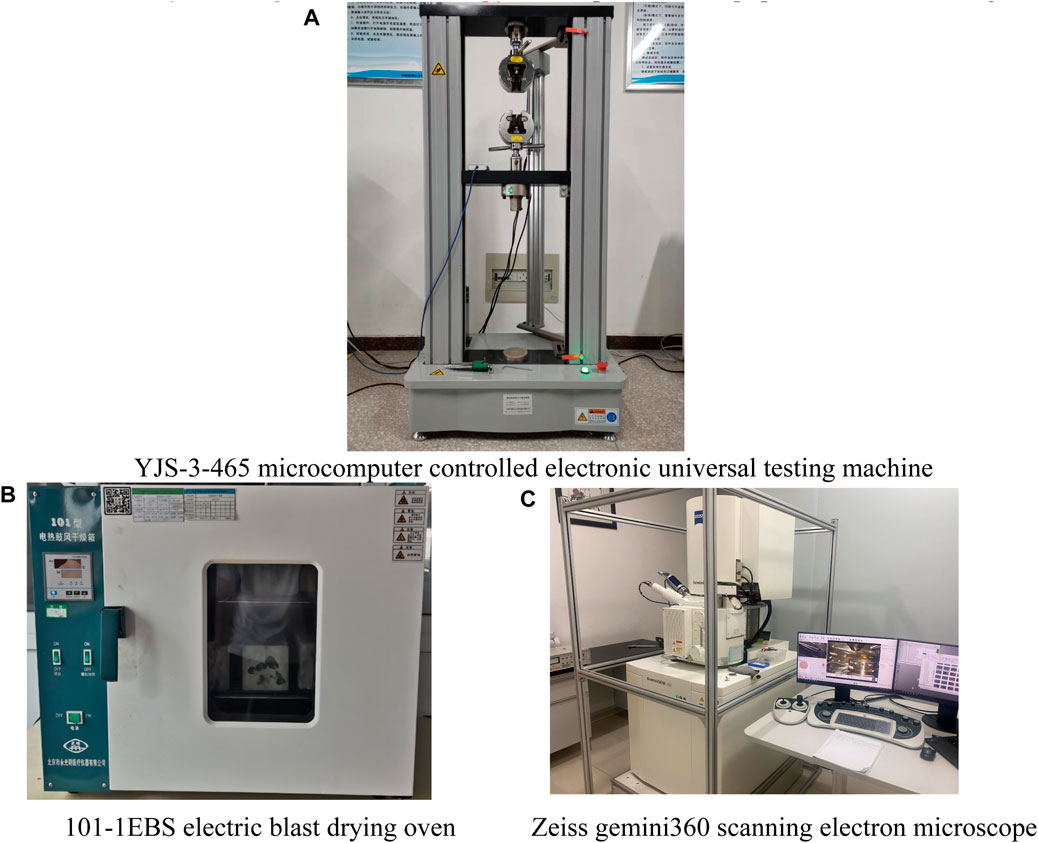
FIGURE 4. Experimental equipment. (A) YJS-3-465 microcomputer controlled electronic universal testing machine; (B) 101-1EBS electric blast drying oven; (C) Zeiss gemini360 scanning electron microscope.
3 Analysis of experimental results
3.1 Quality change
The quality of the foamed concrete samples after drying was measured respectively. The change curve of the quality of foamed concrete samples with the number of dry-wet cycles under three pH conditions is shown in Figure 5.
As can be seen from Figure 5, with the increase of the number of dry-wet cycles, the quality of the foamed concrete sample gradually decreases. This is because the stone powder of foamed concrete contains calcium carbonate, which can react with acidic solution to produce carbon dioxide gas, and the escape of carbon dioxide gas resulting in the quality of foamed concrete reduced. The smaller the pH value, the more obvious the quality change. Meanwhile, it can be observed that the soaking solution gradually becomes cloudy, that is, part of the foamed concrete falls off and dissolves in water. Among them, the quality change at pH = 7 is mainly caused by the falling off of dust particles on the surface of the sample, so the quality reduction is the smallest. When the solution is acidic, in addition to the dust falling off the surface of the foamed concrete sample, the acidic solution will also chemically react with the mineral powder or stone powder in the sample to generate gas, and cause corrosion to the internal pores, which jointly leads to the reduction of the sample quality.
3.2 Failure characteristics analysis of uniaxial compression
The failure process of foamed concrete in uniaxial compression test can be roughly divided into four stages: compaction stage, elastic deformation stage, crack propagation stage and failure stage. As shown in Figure 6, taking the initial sample (0 dry-wet cycle) as an example, during the compression process, the internal pores of the foamed concrete were first compressed and the sample became more compact, without significant changes in the surface of the sample; with the further application of the load, the sample reached the state of compaction, the edge of the concrete raised slightly, and small cracks appeared at the surface of the edge; with continued loading, the surface cracks of the sample increased and became larger, and the crack growth of the edge part was more significant, accompanied by debris shedding; as the loading continues, the cracks of the sample gradually expanded and penetrated with the increase of the load, the cracks were formed and the edge parts were bulged; finally, a large area of the sample edge uplift fell off, cracks penetrated through the whole sample of foamed concrete, then the foamed concrete was damaged.
Figure 7 shows the uniaxial compression failure pattern of foamed concrete samples after 15 dry-wet cycles under different acidic conditions. It can be seen from the figure that the stronger the acidity of the dry-wet cycle solution, the more serious the damage of the foamed concrete sample. When the pH of the dry-wet cycle solution is 7, the cracks of the sample after compression failure are less and smaller in scale, and a small amount of the edge part falls off; when the pH of the dry-wet cycle solution is 5, multiple through cracks appear on the surface of the sample after compression failure, and multiple fragments are separated at the same time; when the pH of the dry-wet cycle solution is 3, the surface of the sample after compression failure is covered with cracks, the edge part falls off in a large area, and the surface of the sample is almost completely separated. This is because with the decrease of pH value, the more serious the corrosion of the acidic solution to the foamed concrete, the more cracks and pores are generated inside the foamed concrete, making the damage degree of the foamed concrete sample greater.
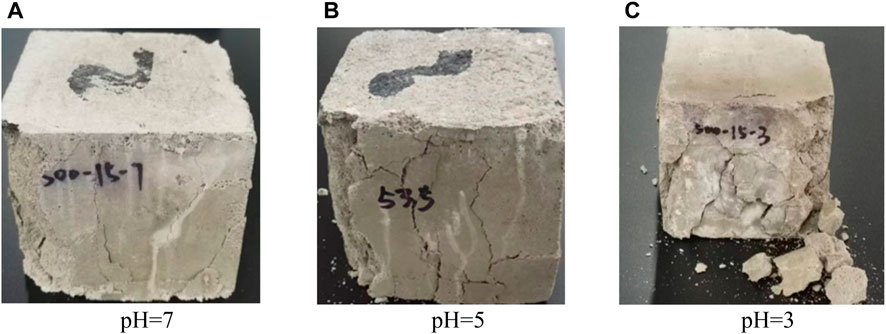
FIGURE 7. Failure modes of foamed concrete under uniaxial compression with different acidic conditions. (A) pH=7; (B) pH=5; (C) pH=3.
It can be observed in Figure 8 that when pH values are both 3, with the increase of the number of dry-wet cycles, the shedding phenomenon of foamed concrete is intensified, the cracks increase, the width of cracks increase, and surface fragments of samples gradually increase. The cracks of foamed concrete after 5 and 10 dry-wet cycles are mainly concentrated on the edge of the sample, while the cracks of foamed concrete after 15 dry-wet cycles are distributed on the whole surface of the sample. This is because the more the number of dry-wet cycles, the longer the acid corrosion time experienced by the foamed concrete, the more and larger pores and cracks appear in the sample after long-term corrosion, and the cracks on the surface and inside expand at the same time during compression, resulting in a more significant degree of damage to the sample.
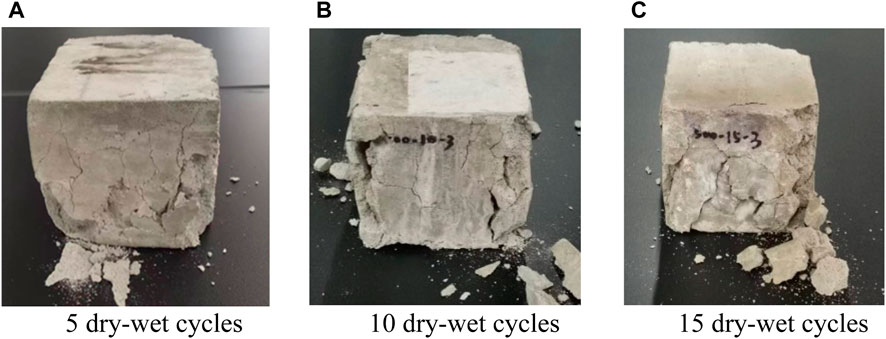
FIGURE 8. Failure modes of foamed concrete under uniaxial compression with different number of dry-wet cycles. (A) 5 dry-wet cycles; (B) 10 dry-wet cycles; (C) 15 dry-wet cycles.
3.3 Analysis of uniaxial compression strength degradation
Uniaxial compression tests were carried out on foamed concrete under different dry-wet cycles at neutral and two acidic environments, the stress-strain curves are shown in Figure 9.
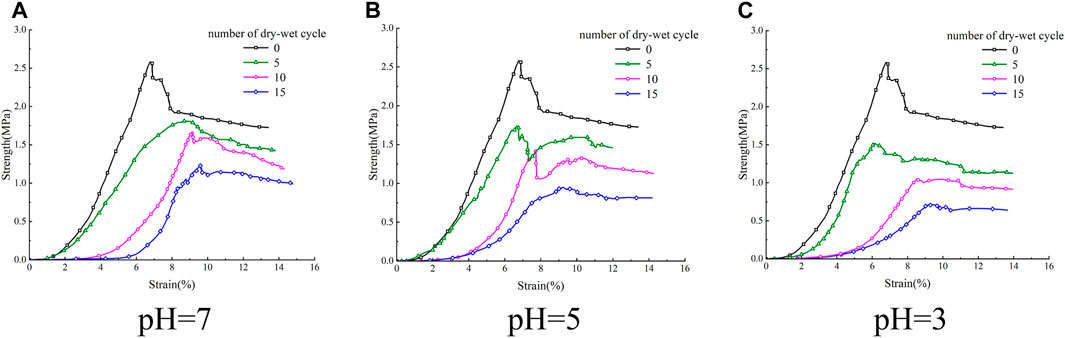
FIGURE 9. Uniaxial compressive stress-strain curve of foamed concrete under dry-wet cycles. (A) pH=7; (B) pH=5; (C) pH=3.
It can be seen from Figure 9 that the stress-strain curve of foamed concrete under the action of different times of wet-dry cycles is generally consistent. The uniaxial compression process of foamed concrete can be roughly divided into several stages according to the change rule of the curves during the compression process: the internal pore compaction stage (curve rising slowly), elastic deformation stage (curve rising straight), crack expansion and through stage (curve from upward trend to downward trend) and failure stage (curve declining slowly).
Figure 10 can more intuitively show several stages of uniaxial compression failure of foamed concrete. During the compaction stage, the internal pores of foamed concrete gradually close, and the stress increases slowly but the strain increases rapidly; then the elastic deformation occurs and the stress-strain curve rises linearly; in the late stage of elastic deformation, when the crack extends to a certain extent, the foamed concrete sample reaches the peak stress, then loses the bearing capacity, and enters the failure stage, that is, the stress no longer increases and the strain further increases.
In addition, it can be seen from Figure 9 that when the number of dry-wet cycles is low, the pore compaction stage is short, and with the increase of the number of dry-wet cycles, the compaction stage gradually becomes longer, and an obvious plastic yield period appears. This is because the dry-wet cycle will dissolve the foamed concrete, resulting in the expansion and consolidation of pores inside the sample, reducing the bonding strength between mineral particles, making the internal structure of the foamed concrete loose, and then affecting its deformation characteristics. According to the stress-strain curve in Figure 9, compressive strength and peak strain of foamed concrete under different dry-wet cycles can be obtained, and the data are listed in Table 2.
As can be seen from Table 2, the compressive strength of foamed concrete in both neutral environment and two acidic environments gradually decreases with the increase of the number of dry-wet cycles, that is, the dry-wet cycle will cause obvious deterioration of the uniaxial compressive strength of foamed concrete samples, and the more cycles, the more obvious the deterioration effect. In addition, with the increase of dry-wet cycles, the peak strain of foamed concrete samples under different solution conditions gradually increases, and the change is more obvious under acidic conditions. This indicates that the dry-wet cycle will erode the foamed concrete, expand the internal pores of the sample and reduce the degree of cementation between aggregates, resulting in the sample more prone to deformation, and the acidity of the solution will further aggravate the erosion of foamed concrete.
With the increase of the number of dry-wet cycles, the total deterioration degree of the foamed concrete strength increases, indicating that the deterioration damage of the dry-wet cycles on the compressive strength of foamed concrete is constantly accumulated. For this purpose, uniaxial compressive strength data were fitted, as shown in Figure 11.
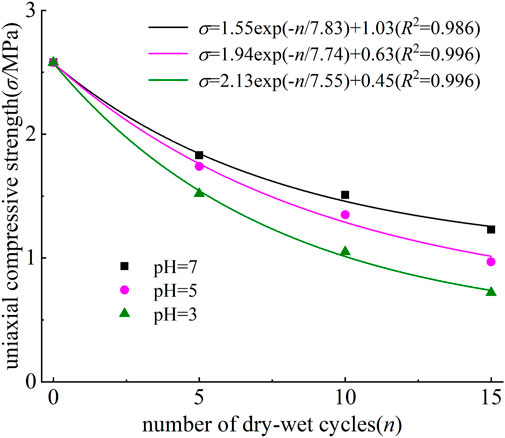
FIGURE 11. Relationship between uniaxial compressive strength of foamed concrete and the number of dry-wet cycles.
It can be seen from Figure 11 that the fitting curve is very consistent with the test data. The general expression of the fitting curve for three kinds of foamed concrete with different strengths is as follows
where, σ is uniaxial compressive strength; n is the number of dry-wet cycles; α, β and γ are the fitting parameters. This exponential fitting equation is basically consistent with the existing research results on the damage characteristics of concrete materials or concrete-like materials (Chai et al., 2022; Chai et al., 2023).
As can be seen from Figure 11, the correlation coefficients of the fitting curves of uniaxial compressive strength of foamed concrete under three pH values are all greater than 0.98. In this experiment, α ranges from 1.55 to 2.13, and is negatively correlated with pH value. The range of β is 7.83–7.55, which is positively correlated with pH value. Both α and β vary in a small range, indicating that the three curves in Figure 11 are very similar in shape, with the biggest difference being the parameter γ. It can be seen from the fitting data in the figure that the values of γ are 1.03, 0.63, and 0.45 respectively, which are very close to but slightly smaller than the strength values under the action of 15 dry-wet cycles of three different pH values, indicating that the γ values reflect the strength attenuation threshold under the action of dry-wet cycles of foamed concrete. The fitting formula shows that the strength of foamed concrete samples decreases exponentially under the action of dry-wet cycle. The strength deterioration degree of foamed concrete under the action of dry-wet cycle is defined as:
where, σn is the uniaxial compressive strength of foamed concrete subjected to n dry-wet cycles. σ0 is the initial uniaxial compressive strength of foamed concrete. Taking pH = 5 as an example, according to the fitting formula, the deterioration compressive strength after 15 dry-wet cycles is 0.63 MPa, and the total strength deterioration degree reaches 75.6%. However, the actual degree of deterioration calculated from Table 2 is 61.6%. It can be concluded that the damage and deterioration of the uniaxial compressive strength of the foamed concrete caused by the dry-wet cycle is not infinite accumulation, but there is a certain threshold. When the dry-wet cycle reaches the threshold, the mechanical parameters of the foamed concrete will no longer decrease, unless the overall structure of the foamed concrete is damaged by the dry-wet cycle.
3.4 Analysis of internal microstructure
In order to analyze the damage mechanism of foamed concrete under the action of acid dry-wet cycles, electron microscope scanning tests were carried out on the samples after 0, 10, and 15 dry-wet cycles (pH = 3), respectively, and the changes of the internal microstructure of the sample were observed. The changes of foamed concrete internal microstructure morphology under the action of acid dry-wet cycle are shown in Figures 12, 13. The magnification in Figure 12 is 100 times and the magnification in Figure 13 is 10000 times.
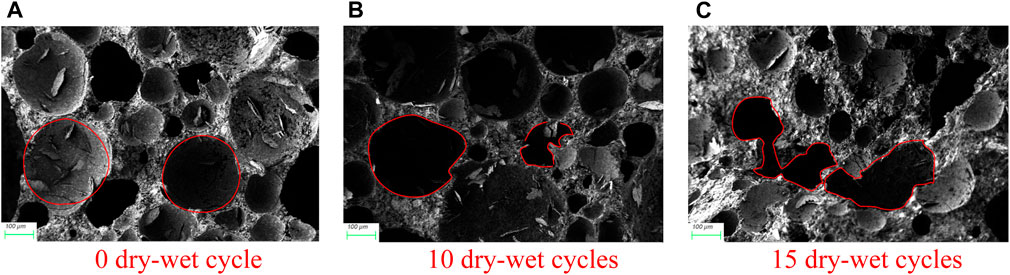
FIGURE 12. Scanning electron microscope (SEM) of foamed concrete pores under different acidic dry-wet cycles: (A) 0 dry-wet cycle; (B) 10 dry-wet cycles; (C) 15 drywet cycles.
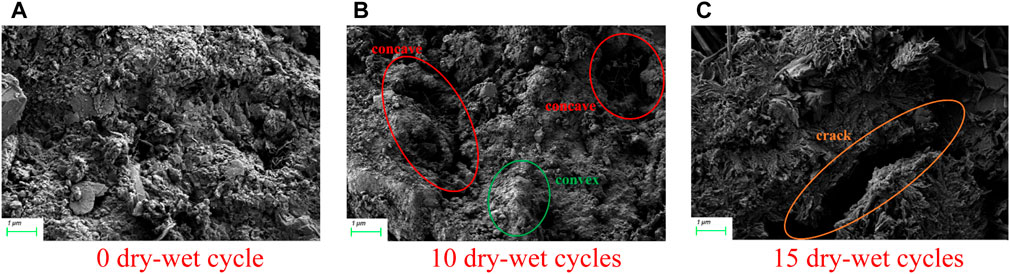
FIGURE 13. Scanning electron microscope (SEM) of foamed concrete aggregate under different acidic dry-wet cycles: (A) 0 dry-wet cycle; (B) 10 dry-wet cycles; (C) 15 dry-wet cycles.
As can be seen from Figure 12, the internal pores of the initial foamed concrete sample are circular, and the structure is relatively complete and dense. Due to the erosion of acidic solution, the pores inside the foamed concrete changed from round to irregular shape, and there were obvious expansion and merger. In addition, by comparing the SEM images under different dry-wet cycle conditions, it can be seen that the more cycles, the more serious the erosion of pores by acidic solution, which also indicates that the acidic dry-wet cycle can affect the mechanical properties of foamed concrete by changing its internal pore structure.
As can be seen from Figure 13, in the part without pores, the aggregate inside the initial foamed concrete sample is complete; after 10 dry-wet cycles, the foamed concrete sample has concave and convex, but no obvious cracks; after 15 dry-wet cycles, the foamed concrete has obvious cracks. This indicates that the acid dry-wet cycle will gradually produce cracks in the foamed concrete, reduce the adhesion between aggregate and mortar, and then affect its mechanical properties. In addition, the more acid dry-wet cycles, the more obvious the cracks inside the foamed concrete.
In order to further analyze the damage to foamed concrete caused by acidic dry-wet cycling, the microstructure evolution model of foamed concrete under different times of acidic dry-wet cycle was established, as shown in Figure 14. It can be seen that the pores in foamed concrete expand and micro-cracks appear under the action of acidic dry-wet cycle in the early stage. With the increase of the number of acid dry-wet cycles, the erosion inside the foamed concrete is more serious, the pores begin to merge and the cracks gradually expand, and the structure damage is gradually serious.
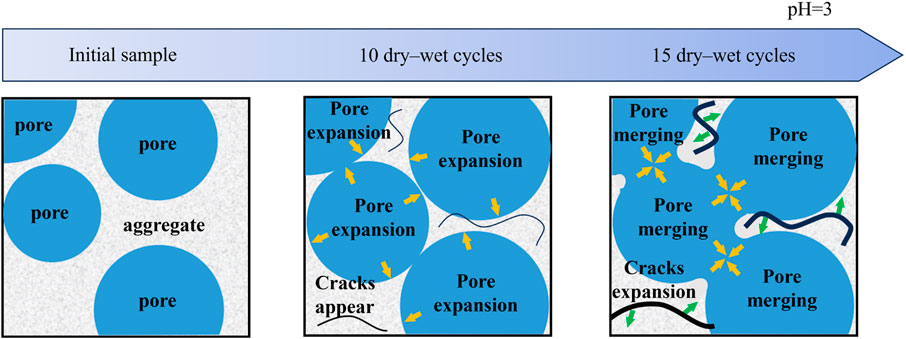
FIGURE 14. Evolution diagram of internal structure of foamed concrete under the action of acid dry-wet cycle.
4 Conclusion
In this paper, uniaxial compression test and scanning electron microscope test were carried out on foamed concrete under different dry-wet cycles in acidic and neutral environments, and the deterioration effect and deterioration mechanism of acidic and neutral dry-wet cycles on foamed concrete were analyzed and compared. The main conclusions are as follows:
(1) With the increase of the number of dry-wet cycles, the quality of foamed concrete decreases continuously in both neutral and acidic environments, and the more acidic the solution, the greater the quality change.
(2) The uniaxial compression process of foamed concrete can be divided into compaction stage, elastic deformation stage, crack propagation stage and failure stage. The more acidic the solution, the more times of dry-wet cycles, the more serious the damage form of foamed concrete under uniaxial compression.
(3) The uniaxial compressive strength of foamed concrete in both neutral and acidic environments decreases gradually with the increase of dry-wet cycles. In addition, for the same number of dry-wet cycles, the stronger the acidity of the solution, the smaller the uniaxial compressive strength. With the increase of the number of dry-wet cycles, the strength decreases exponentially.
(4) The acid dry-wet cycle will corrode the foamed concrete, resulting in the expansion and consolidation of the pores in the foamed concrete samples. With the increase of the number of dry-wet cycles and the enhancement of the acidity of the solution, the expansion of the pores inside the foamed concrete becomes more obvious, that is, the acidic dry-wet cycles can affect the mechanical properties of the foamed concrete by changing the internal pore structure.
Data availability statement
The original contributions presented in the study are included in the article/Supplementary material, further inquiries can be directed to the corresponding author.
Author contributions
YW: Data curation, Formal Analysis, Writing–original draft. SC: Data curation, Formal Analysis, Investigation, Project administration, Writing–original draft. YC: Investigation, Resources, Software, Supervision, Writing–review and editing. SZ: Resources, Validation, Visualization, Writing–review and editing. LC: Conceptualization, Funding acquisition, Methodology, Writing–review and editing.
Funding
The author(s) declare financial support was received for the research, authorship, and/or publication of this article. The work described in this paper was supported by the Natural Science Basic Research Plan in Shaanxi Province, (No. 2021-JQ243), the Science-Technology project of China First Highway Engineering Co., Ltd. (grant number KJYF-2023-7GS-CG08) and Fundamental Research Funds for the Central Universities, CHD (No. 300102282201). The funder was not involved in the study design, collection, analysis, interpretation of data, the writing of this article, or the decision to submit it for publication.
Conflict of interest
Authors YW, SC, YC, and SZ were employed by The Seventh Engineering Co., Ltd of CFHEC.
The remaining author declares that the research was conducted in the absence of any commercial or financial relationships that could be construed as a potential conflict of interest.
Publisher’s note
All claims expressed in this article are solely those of the authors and do not necessarily represent those of their affiliated organizations, or those of the publisher, the editors and the reviewers. Any product that may be evaluated in this article, or claim that may be made by its manufacturer, is not guaranteed or endorsed by the publisher.
References
Amran, Y. H. M., Farzadnia, N., and Ali, A. A. A. (2015). Properties and applications of foamed concrete; a review. Build. Mater. 101, 990–1005. doi:10.1016/j.conbuildmat.2015.10.112
Chai, S. B., Jia, Y. S., Du, Y. X., Hu, B., and Li, X. P. (2022). Experimental study on compression mechanical characteristics of filled rock joints after multiple pre-impacts. Sci. Rep. 12 (1), 13628. doi:10.1038/s41598-022-15849-5
Chai, S. B., Liu, H., Song, L., Li, X. P., Fu, X. D., and Zhou, Y. Q. (2023). Static pressure and dynamic impact characteristics of filled jointed rock after frozen-thaw cycle damage. Front. Ecol. Evol. 11. doi:10.3389/fevo.2023.1222676
Chandni, T. J., and Anand, K. B. (2018). Utilization of recycled waste as filler in foam concrete. J. Build. Eng. 19, 154–160. doi:10.1016/j.jobe.2018.04.032
Chung, S. Y., Elrahman, M. A., Kim, J. S., Han, T. S., Stephan, D., and Sikora, P. (2019). Comparison of lightweight aggregate and foamed concrete with the same density level using image-based characterizations. Constr. Build. Mater. 211, 988–999. doi:10.1016/j.conbuildmat.2019.03.270
Cody, R. D., Cody, A. M., Spry, P. G., and Lee, H. (2001). Reduction of concrete deterioration by ettringite using crystal growth inhibition techniques. Ames, Iowa: Department of Geological and Atmospheric Sciences, Iowa State University.
Emmanuel, K. A., and Sami, H. R. (1988). Response of concrete to sulfuric acid attack. Mater. J. 85 (6), 481–488.
Fattuni, N. I., and Hughes, B. P. (1983). Effect of acid attack on concrete with different admixtures or protective coatings. Cem. Concr. Res. 13 (5), 655–665. doi:10.1016/0008-8846(83)90055-8
Hua, Y. T., Yin, S. P., Yu, Y. L., and Li, S. (2019). Research on chloride diffusion and flexural behavior of beams strengthened with TRC subjected to dry-wet cycles. Constr. Build. Mater. 229, 116906. doi:10.1016/j.conbuildmat.2019.116906
Jones, M. R., and McCarthy, A. (2005). Preliminary views on the potential of foamed concrete as a structural material. Mag. Concr. Res. 57 (1), 21–31. doi:10.1680/macr.2005.57.1.21
Kearsley, E., and Visagie, M. (2002). Properties of foamed concrete as influenced by air-void parameters. Concr. Beton. 101, 8–14.
Kearsley, E. P., and Wainwright, P. J. (2002). The effect of porosity on the strength of foamed concrete. Cem. Concr. Res. 32 (2), 233–239. doi:10.1016/S0008-8846(01)00665-2
Kilincarslan, Ş., Davraz, M., and Akça, M. (2018). The effect of pumice as aggregate on the mechanical and thermal properties of foam concrete. Arab. J. Geosci. 11, 289–296. doi:10.1007/s12517-018-3627-y
Kong, H. L., and Orbison, J. G. (1987). Concrete deterioration due to acid precipitation. ACI Mater. J. 84 (2), 110–116. doi:10.14359/1825
Meyer, A. H., and Ledbetter, W. B. (1970). Sulfuric acid attack on concrete sewer pipe. J. Sanit. Eng. Div. 96 (5), 1167–1182. doi:10.1061/JSEDAI.0001176
Mydin, M. A. O., and Wang, Y. C. (2011). Structural performance of lightweight steel-foamed concrete–steel composite walling system under compression. Thin Wall Struct. 49 (1), 66–76. doi:10.1016/j.tws.2010.08.007
Nambiar, E. K. K., and Ramamurthy, K. (2006). Influence of filler type on the properties of foam concrete. Cem. Concr. Compos. 28 (5), 475–480. doi:10.1016/j.cemconcomp.2005.12.001
Narayanan, N., and Ramamurthy, K. (2000). Microstructural investigations on aerated concrete. Cem. Concr. Res. 30 (3), 457–464. doi:10.1016/S0008-8846(00)00199-X
Oginni, F. A. (2015). Continental application of foamed concrete Technology: lessons for infrastructural development in africa. Br. J. Appl. Sci. Technol. 5 (4), 417–424. doi:10.9734/BJAST/2015/13063
Okochi, H., Kameda, H., Hasegawa, S., Saito, N., Kubota, K., and Igawa, M. (2000). Deterioration of concrete structures by acid deposition—an assessment of the role of rainwater on deterioration by laboratory and field exposure experiments using mortar specimens. Atmos. Environ. 34 (18), 2937–2945. doi:10.1016/S1352-2310(99)00523-3
Park, S. B., Yoon, E. S., and Lee, B. I. (1999). Effects of processing and materials variations on mechanical properties of lightweight cement composites11This paper was originally submitted to Advanced Cement Based Materials on 16 February 1998. The paper was received at the Editorial Office of Cement and Concrete Research on 12 October 1998 and accepted in final form on 16 November 1998. Cem. Concr. Res. 29 (2), 193–200. doi:10.1016/S0008-8846(98)00221-X
Pasupathy, K., Ramakrishnan, S., and Sanjayan, J. (2021). Influence of recycled concrete aggregate on the foam stability of aerated geopolymer concrete. Constr. Build. Mater. 271, 121850. doi:10.1016/j.conbuildmat.2020.121850
Polder, R. B., and Peelen, W. H. A. (2002). Characterisation of chloride transport and reinforcement corrosion in concrete under cyclic wetting and drying by electrical resistivity. Cem. Concr. Compos. 24 (5), 427–435. doi:10.1016/S0958-9465(01)00074-9
Raj, A., Sathyan, D., and Mini, K. M. (2019). Physical and functional characteristics of foam concrete: a review. Build. Mater. 221, 787–799. doi:10.1016/j.conbuildmat.2019.06.052
Raju, P. S. N., and Dayaratnam, P. (1984). Durability of concrete exposed to dilute sulphuric acid. Build. En. 19 (2), 75–79. doi:10.1016/0360-1323(84)90032-5
Sahmaran, M., Erdem, T. K., and Yaman, I. O. (2007). Sulfate resistance of plain and blended cements exposed to wetting–drying and heating–cooling environments. Constr. Build. Mater. 21 (8), 1771–1778. doi:10.1016/j.conbuildmat.2006.05.012
Samson, G., and Cyr, M. (2018). Porous structure optimisation of flash-calcined metakaolin/fly ash geopolymer foam concrete. Eur. J. Environ. Civ. En. 22 (12), 1482–1498. doi:10.1080/19648189.2017.1304285
Tixier, R. (2000). Microstructural development and sulfate attack modeling in blended cement-based materials. Tempe, Arizona: Arizona State University.
Wang, H. L., Lu, C. H., Jin, W. L., and Bai, Y. (2011). Effect of external loads on chloride transport in concrete. J. Mater. Civ. Eng. 23 (7), 1043–1049. doi:10.1061/(ASCE)MT.1943-5533.0000265
Keywords: foamed concrete, acidic environment, dry-wet cycle, uniaxial compressive strength, microstructure
Citation: Wu Y, Chai S, Chen Y, Zou S and Chai L (2024) Experimental study on deterioration characteristics of foamed concrete under dry-wet cycles in acidic environment. Front. Mater. 10:1332243. doi: 10.3389/fmats.2023.1332243
Received: 11 November 2023; Accepted: 28 December 2023;
Published: 16 January 2024.
Edited by:
Yongqiang Zhou, Chinese Academy of Sciences (CAS), ChinaReviewed by:
Xiao Wang, Shandong University of Science and Technology, ChinaLv Longlong, Ningxia University, China
Copyright © 2024 Wu, Chai, Chen, Zou and Chai. This is an open-access article distributed under the terms of the Creative Commons Attribution License (CC BY). The use, distribution or reproduction in other forums is permitted, provided the original author(s) and the copyright owner(s) are credited and that the original publication in this journal is cited, in accordance with accepted academic practice. No use, distribution or reproduction is permitted which does not comply with these terms.
*Correspondence: Lianzeng Chai, 2021128033@chd.edu.cn