Targeting the GRP78 Pathway for Cancer Therapy
- 1Guangdong Key Laboratory for Research and Development of Natural Drugs, The Marine Biomedical Research Institute, Guangdong Medical University, Zhanjiang, China
- 2The Marine Biomedical Research Institute of Guangdong Zhanjiang, Zhanjiang, China
- 3Southern Marine Science and Engineering Guangdong Laboratory, Zhanjiang, China
- 4The Key Lab of Zhanjiang for R&D Marine Microbial Resources in the Beibu Gulf Rim, Guangdong Medical University, Zhanjiang, China
The 78-kDa glucose-regulated protein (GRP78) plays an important part in maintaining protein stability, regulating protein folding, and inducing apoptosis autophagy, which is considered as a powerful protein. Meanwhile, it also plays a role in ensuring the normal function of organs. In recent years, more and more researches have been carried out on the targeted therapy of GRP78, mainly focusing on its relevant role in tumor and its role as a major modulator and modulator of subordinate pathways. The ability of GRP78 to respond to endoplasmic reticulum stress (ERS) determines whether tumor cells survive and whether the changes in expression level of GRP78 regulated by endoplasmic reticulum (ER) caused by various factors will directly or indirectly affect cell proliferation, apoptosis, and injury, or reduce the body's defense ability, or have protective effects on various organs.
Introduction
Glucose regulated protein (GRP78) is a mature endoplasmic reticulum (ER)-resident chaperone, belonging to the large chaperone family of heat-shock protein 70 (HSP70) molecules (1). Physiologically, GRP78 binds to the polypeptide chain in a non-covalent bond on the ER and then disassociates, facilitating proper protein folding and assembly, helping protein transport across the ER (2). It has 60% homology with the HSP70 family, and its substrate binding region contains a lysine-rich structural domain (3), which has the characteristic of binding to abnormal proteins under stress conditions, but it has differences in the regulation of protein expression (4). In ER, GRP78 assists the ER protein to stabilize and induces protein folding reaction (unfolded protein response, UPR). The surface-related GRP78 plays a role in cell protection and mediated cytoskeletal remodeling (5), while the secreted GRP78 plays an immunomodulatory role (6). GRP78 can move in under the condition of ER outside the region, even secrete to the outside of the cell with the corresponding ligand binding, playing its function by affecting various signaling pathways, influencing the cell signal transduction and the development (Figure 1). Under certain pathological conditions, cytokines produced by the body, due to exposure to inflammatory environment, promote the surface translocation of GRP78, which further explains the prominent position of GRP78 in many diseases (7). Recent studies have demonstrated that GRP78 has far more functions than this (4, 8, 9). A series of researches have shown that GRP78 plays a valid role in tumor activity and treatment.
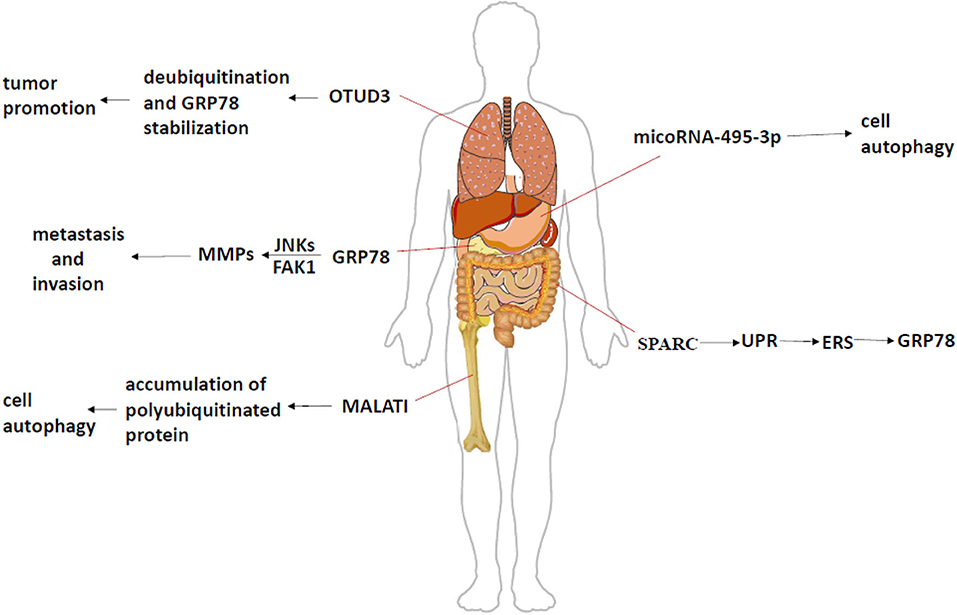
Figure 1. The mechanism of GRP78 in different tumors. In lung adenocarcinoma, GRP78, as a specific substrate of OTUD3, mediates deubiquitination under the state of high expression of OTUD3, and enables the stable proliferation of tumor cells. GRP78 activates JNKs and FAK1 pathways in pancreatic cancer, and then increases the expression of downstream products and promotes tumor invasion and metastasis. In the process of multiple myeloma, the expression of MALATI will lead to the accumulation of polyubiquitinated proteins, which will activate ERS, promote the expression of GRP78, and induce cell apoptosis. MicroRNA in gastric cancer leads to different malignant phenotypes through different effects on GRP78. SPARC can interfere ERS by affecting UPR in patients with colorectal cancer, thereby changing the expression of GRP78 and controlling the apoptosis of different cells.
Correlation With Tumor Occurrence and Development
Advanced breast cancer patients have a high mortality rate, mainly because of tumor metastasis. GRP78 has a low expression in benign breast lesions, but it greatly increases in breast cancer (10–12). Stathmin1 (STMN1), also known as oncoprotein 18, is a phosphorylated protein associated with multiple tumor metastases, which can bind with GRP78 to form STMN1-GRP78 stable complex, mediating tumor metastasis (13) (Table 1). High expression of GRP78 can promote the expression of MMPs (matrix metalloproteinases) and metastasis and invasion of pancreatic cancer by activating some pathways like JNK (c-Jun N-terminal kinase) and FAK (focal adhesion kinase) (24) (Figure 1). However, the deletion of GRP78 not only reduced the expression of MMPs but also inhibited the RhoA (Ras homolog gene family, member A) signaling pathway and prevented tumor invasion (25). CRIPTO (Teratocarcinoma-derived growth factor 1), one of the major regulators, participate in the development of prostate cancer. It has been found that its expression is elevated to many cancers, especially in metastatic tumors, and GRP78 also shows high expression (Figure 1) (11, 26, 27). As we all know, ERS in tumor cells is an important tumor response to help tumor survival. The knockout of CRIPTO or GRP78 can reduce the invasion of cancer cells, thereby reducing cell proliferation, migration, colony formation, and other processes (28). KRAS (Kirsten rat sarcoma viral oncogene) mutations are present in 90% of pancreatic cancer patients, but an expression of only half of GRP78 prevents early pancreatic cancer development (29). Therefore, these results approve the importance of GRP78 in the metastasis and survival of most tumors whatever directly or indirectly. In colorectal cancer, SPARC (Secreted Protein, Acidic and Rich in Cysteine) interference ERS to further enhance apoptosis by regulating the UPR (Figure 2); the low expression of SPARC and GRP78 prompts settlement colorectal cancer patients with a lower survival rate (Figure 1; Table 1) (14). In addition, hepatocellular carcinoma cells under ER stress transfer specific miRNA through exosomes to infiltrating macrophages in the tumor micro-environment to promote the immune escape of hepatocellular carcinoma cells (30–33). As we all know, the diversity of various binding proteins and functional results of GRP78 reflects the function of GRP78 in regulating cell activities and making different responses to ER, so as to make the body change, aggravate damage, or play a protective role.
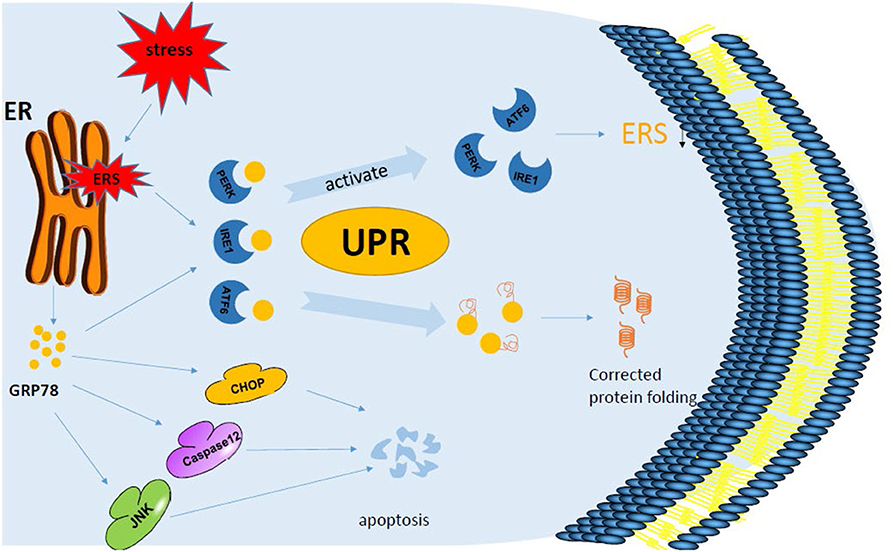
Figure 2. GRP78 and endoplasmic reticulum stress. When a cell is under the influence of foreign factors, the endoplasmic reticulum is stressed, leading to the appearance of unfolded proteins or misfolded proteins inside the cell, which accumulates in the cell. At this time, three specific signaling pathways, PERK, IRE1, and ATF6, trigger the unfolded protein response. PERK, IRE1, ATF6, and GRP78 are activated from the binding state to the detached state. GRP78 binds to unfolded proteins or misfolded proteins to correct protein folding, while PERK and other physiological reactions reduce protein synthesis after a series of phosphorylation. In addition, the expression of GRP78 is up-regulated when ERS occurred, and the pro-apoptotic pathways caspase 12, JNK, and CHOP are activated. When UPR exceeds a specified limit, it also induces apoptosis through the caspase 12 pathway.
New Targets for Tumor Therapy
GRP78 can promote the survival of head and neck cancer cells by maintaining lysosomal activity (Figure 1), meanwhile, through inhibiting the development of head and neck cancer by inducing a series of ubiquitination of mitochondrial ubiquitin ligase activator (such as MUL1, one of E3 ubiquitin-protein ligases) to down-regulate GRP78 (Table 1) (15). Tumor growth and GRP78 expression were inhibited after MUL1 cancer cell was knocked out, suggesting that the MUL1–GRP78 axis will become a new strategy for the treatment of head and neck cancer. In multiple myeloma, the antagonistic effect of a form of RNA called MALAT1 (metastasis-associated lung adenocarcinoma transcript1) will lead to the accumulation of ubiquitin proteins, induce ERS, up-regulate the expression of ER sensor proteins such as GRP78, activate autophagy, and induce apoptosis (Figure 1; Table 1) (16, 34). This suggests that the key link in the treatment of myeloma may be to induce ERS and promote the up-regulation of GRP78 expression to control the tumor. A domain-containing protein 3 (OTUD3) showed high expression in lung adenocarcinoma, and GRP78 could be used as a specific substrate. OTUD3 was first identified as the deubiquitination enzyme of GRP78, mediating deubiquitination in tumor cells and maintaining the function of GRP78, so as to stabilize the proliferation of tumor cells (Figure 1; Table 1) (17). In patients with gastric cancer, microRNA mir-495-3p regulates autophagy by targeting GRP78. The expression level of GRP78 can antagonize the autophagy induced by mir-495-3p. Clinically, both the up-regulation of GRP78 and the down-regulation of mir-495-3p are linked to the malignant phenotype of gastric cancer (Figure 1; Table 1) (18). Therefore, GRP78 can be combined with the specific factor as an indicator of treatment response and prognostic indicator (35, 36).
Recently, researchers have designed a new ferritin probe that can specifically identify and kill liver cancer cells. The targeted peptide SP94 on its surface can specifically bind GRP78 on the surface of liver cancer cells to identify liver cancer (19, 20). GRP78 further mediates endocytosis into lysosomes of liver cancer cells, and ferritin lysis releases adriamycin under acidic environment, so as to kill liver cancer cells (Table 1) (19, 20). In addition, GRP78 level on the surface of cancer cells has been found in prostate cancer studies to be related to tumor stage, and the level of anti-GRP78 antibody is parallel to the concentration of tumor-specific antigen in serum (27, 37, 38), which further suggests the effect of GRP78 on driving tumor progression. Therefore, the expression of GRP78 can be considered as a promoter of many cancer characteristics, and it has been proved that it is up-regulated not only in a variety of tumor cells, but also in tumor-related macrophages. Under the action of ERS, multiple factors combined with GRP78 work together to regulate the autophagy apoptosis of cells and make the cells progress in different directions (Figure 2).
Tumor Effect of Drugs and Improvement of Drug Resistance
As the treatment progresses, cancer cells will develop resistance to therapeutic drugs. There are studies that have shown that high levels of GRP78 in tumor cells lead to multidrug resistance (MDR) in treatment (39); thus, GRP78 can be a good target. Controlling its expression can restore the sensitivity of tumor cells to therapeutic drugs (40–43). In addition, OSU-03012 and phosphodiesterase 5 inhibitor were used in some experiments to target GRP78 and its related proteins, which can prevent some viruses and bacteria from replicating and killing most tumor cells without harming other normal cells (Table 1) (21). This mechanism is mainly achieved by reducing the expression of virus receptor and oncogene receptor. Thiazolaminobenzene sulfamides can bind to GRP78 in the ER of melanoma cells and enhance ERS, exert cytotoxic effects, and induce autophagy and apoptosis and other anti-tumor effects (Figure 2); in addition, it can reverse the sensitivity of drug-resistant mice to anti-tumor drugs (Table 1) (22). In traditional Chinese medicine, triptolide, an extract of Tripterygium wilfordii, can induce chronic ERS and down-regulate the expression of GRP78, leading to cell death (Figure 2; Table 1) (23). Therefore, the treatment of a tumor can be started from the proliferation and growth of tumor cells and the use of the drug inhibition or targeting GRP78 to control its expression and improve the sensitivity to treatment.
Participation in Immune Activation and Immunosuppression
All members of the HSP70 family have the ability to bind to tumor-specific antigens and mediate immune responses. As a member with a certain correlation with antigen epitopes, GRP78 mainly stimulates anti-tumor immune responses through the cross-expression of histocompatibility complex molecules and initiates CD8+ cytotoxic T cell responses (44). At present, radiation therapy has become one of the main methods to treat tumors. Cells irradiated by radiation will release damage-associated molecular patterns (DAMPs) by activating GRP78, and tumor cells will be devoured by antigen-presenting cells. At the same time, cytotoxic T cells will get information and promote the destruction of tumor cells (45–49). However, its overexpression on proliferating and quiescent cancer cells and tumor-related endothelial cells is conducive to escape from chemotherapy and other therapeutic methods. Meanwhile, it inhibits apoptosis and stimulates autophagy through a series of signaling pathways, thus reducing the sensitivity of tumor cells to radiotherapy and chemotherapy (50, 51). In addition, the anti-GRP78 antibody has been found to have anti-tumor activity, which can not only reduce the tumor growth rate but also enhance the efficacy of radiotherapy for non-small cell lung cancer (52, 53). This provides a more effective and feasible method for the limitation of internal medicine treatment of non-small cell lung cancer (54). Meanwhile, GRP78 can be used as a medium to induce the transformation of cell activities, so that the tumor cells are restricted or even die, which also provides a new path for physical-immune combination therapy to deal with some of the tumors that cannot be treated by non-surgical treatment.
Perspectives
With the development of research and technology, it is believed that GRP78 may have obvious therapeutic potential as a target (55, 56). Both GRP78 itself and other forms of GRP78 have a unique role in controlling cell survival and signal transduction, which is developing into an excellent treatment tool for malignant tumors in the targeted treatment of some cancers, and is expected to be applied to other diseases. According to the biological characteristics of GRP78 in different tumors and the effects of its regulated downstream pathways, we can predict the positive or negative effects. Although the effect of GRP78 in the treatment of tumor is still not completely clear, it can be further studied according to its biological effects and characteristics, or combined with specific markers of diseases, which can provide certain theoretical guidance for precise treatment and achieve the goal of implementing treatment at the molecular level. Therefore, in view of the significance of GRP78 in the occurrence, development, and treatment of different diseases, targeted GRP78 has important value, and its mechanisms of action should be comprehensively considered, and further attention and in-depth research should be made on it.
Author Contributions
GL and XZ performed the literature search and wrote the first draft of the manuscript. GL, HL, and XZ revised and edited the final version of the manuscript. All authors contributed to the article and approved the submitted version.
Funding
This work was supported partly by the National Natural Science Foundation of China (81541153); Guangdong Science and Technology Department (2016A050503046, 2015A050502048 and 2019B090905011); The Public Service Platform of South China Sea for R&D Marine Biomedicine Resources (GDMUK201808); Southern Marine Science and Engineering Guangdong Laboratory Zhanjiang (ZJW-2019-07). The funders had no role in the design of the study; the collection, analysis, and interpretation of the data; the writing of the manuscript; and the decision to submit the manuscript for publication.
Conflict of Interest
The authors declare that the research was conducted in the absence of any commercial or financial relationships that could be construed as a potential conflict of interest.
Abbreviations
ANF, atrial sodium factor; ATF6, Activating transcription factor 6; CHOP, CCAAT/enhancer-binding protein homologous protein; CRIPTO, Teratocarcinoma-derived growth factor 1; DAMP, damage associated molecular patterns; ERS, endoplasmic reticulum stress; FAK, Focal adhesion kinase; GRP78, Glucose regulated protein; GATA-4, gata binding protein 4; HSP70, ; heat shock protein 70, ; IRE1, Inositol requiring enzyme; JNK, c-Jun N-terminal kinase; KRAS, Kirsten rat sarcoma viral oncogene; LXR, liver X receptor; MALATI, metastasis associated lung adenocarcinoma transcript1; MMPs, matrix metalloproteinases; OTUD3, Domain-containing protein 3; PERK, Protein kinase-like ER kinase; PI3K, Phosphoinositide 3-kinase; RhoA, Ras homolog gene family, member A; SPARC, Secreted Protein, Acidic and Rich in Cysteine; STMN1, Stathmin1; UPR, unfolded protein response.
References
1. Rose MD, Misra LM, Vogel JP. KAR2, a karyogamy gene, is the yeast homolog of the mammalian BiP/GRP78 gene. Cell. (1989) 57:1211–21. doi: 10.1016/0092-8674(89)90058-5
2. Qian Y, Tiffany-Castiglioni E. Lead-induced endoplasmic reticulum (ER) stress responses in the nervous system. Neurochem Res. (2003) 28:153–62. doi: 10.1023/A:1021664632393
3. Morgner N, Schmidt C, Beilsten-Edmands V, Ebong IO, Patel NA, Clerico EM, et al. Hsp70 forms antiparallel dimers stabilized by post-translational modifications to position clients for transfer to Hsp90. Cell Rep. (2015) 11:759–69. doi: 10.1016/j.celrep.2015.03.063
4. Ibrahim IM, Abdelmalek DH, Elfiky AA. GRP78: A cell's response to stress. Life Sci. (2019) 226:156–63. doi: 10.1016/j.lfs.2019.04.022
5. Birukova AA, Singleton PA, Gawlak G, Tian X, Mirzapoiazova T, Mambetsariev B, et al. GRP78 is a novel receptor initiating a vascular barrier protective response to oxidized phospholipids. Mol Biol Cell. (2014) 25:2006–16. doi: 10.1091/mbc.e13-12-0743
6. Corrigall VM, Bodman-Smith MD, Brunst M, Cornell H, Panayi GS. Inhibition of antigen-presenting cell function and stimulation of human peripheral blood mononuclear cells to express an antiinflammatory cytokine profile by the stress protein BiP: relevance to the treatment of inflammatory arthritis. Arthritis Rheum. (2004) 50:1164–71. doi: 10.1002/art.20134
7. Vig S, Buitinga M, Rondas D, Crevecoeur I, van Zandvoort M, Waelkens E, et al. Cytokine-induced translocation of GRP78 to the plasma membrane triggers a pro-apoptotic feedback loop in pancreatic beta cells. Cell Death Dis. (2019) 10:309. doi: 10.1038/s41419-019-1518-0
8. Tseng CC, Zhang P, Lee AS. The COOH-terminal proline-rich region of GRP78 is a key regulator of its cell surface expression and viability of tamoxifen-resistant breast cancer cells. Neoplasia. (2019) 21:837–48. doi: 10.1016/j.neo.2019.05.008
9. Cultrara CN, Kozuch SD, Ramasundaram P, Heller CJ, Shah S, Beck AE, et al. GRP78 modulates cell adhesion markers in prostate cancer and multiple myeloma cell lines. BMC Cancer. (2018) 18:1263. doi: 10.1186/s12885-018-5178-8
10. Fernandez PM, Tabbara SO, Jacobs LK, Manning FC, Tsangaris TN, Schwartz AM, et al. Overexpression of the glucose-regulated stress gene GRP78 in malignant but not benign human breast lesions. Breast Cancer Res Treat. (2000) 59:15–26. doi: 10.1023/A:1006332011207
11. Sokolowska I, Woods AG, Gawinowicz MA, Roy U, Darie CC. Identification of potential tumor differentiation factor (TDF) receptor from steroid-responsive and steroid-resistant breast cancer cells. J Biol Chem. (2012) 287:1719–33. doi: 10.1074/jbc.M111.284091
12. Baptista MZ, Sarian LO, Vassallo J, Pinto GA, Soares FA, de Souza GA. Prognostic significance of GRP78 expression patterns in breast cancer patients receiving adjuvant chemotherapy. Int J Biol Markers. (2011) 26:188–96. doi: 10.5301/JBM.2011.8624
13. Kuang XY, Jiang HS, Li K, Zheng YZ, Liu YR, Qiao F, et al. The phosphorylation-specific association of STMN1 with GRP78 promotes breast cancer metastasis. Cancer Lett. (2016) 377:87–96. doi: 10.1016/j.canlet.2016.04.035
14. Chern YJ, Wong JCT, Cheng GSW, Yu A, Yin Y, Schaeffer DF, et al. The interaction between SPARC and GRP78 interferes with ER stress signaling and potentiates apoptosis via PERK/eIF2alpha and IRE1alpha/XBP-1 in colorectal cancer. Cell Death Dis. (2019) 10:504. doi: 10.1038/s41419-019-1687-x
15. Kim SY, Kim HJ, Kim HJ, Kim DH, Han JH, Byeon HK, et al. HSPA5 negatively regulates lysosomal activity through ubiquitination of MUL1 in head and neck cancer. Autophagy. (2018) 14:385–403. doi: 10.1080/15548627.2017.1414126
16. Amodio N, Stamato MA, Juli G, Morelli E, Fulciniti M, Manzoni M, et al. Drugging the lncRNA MALAT1 via LNA gapmeR ASO inhibits gene expression of proteasome subunits and triggers anti-multiple myeloma activity. Leukemia. (2018) 32:1948–57. doi: 10.1038/s41375-018-0067-3
17. Du T, Li H, Fan Y, Yuan L, Guo X, Zhu Q, et al. The deubiquitylase OTUD3 stabilizes GRP78 and promotes lung tumorigenesis. Nat Commun. (2019) 10:2914. doi: 10.1038/s41467-019-10824-7
18. Chen S, Wu J, Jiao K, Wu Q, Ma J, Chen D, et al. MicroRNA-495-3p inhibits multidrug resistance by modulating autophagy through GRP78/mTOR axis in gastric cancer. Cell Death Dis. (2018) 9:1070. doi: 10.1038/s41419-018-0950-x
19. Jiang B, Yan L, Zhang J, Zhou M, Shi G, Tian X, et al. Biomineralization synthesis of the cobalt nanozyme in SP94-ferritin nanocages for prognostic diagnosis of hepatocellular carcinoma. ACS Appl Mater Interfaces. (2019) 11:9747–55. doi: 10.1021/acsami.8b20942
20. Jiang B, Zhang R, Zhang J, Hou Y, Chen X, Zhou M, et al. GRP78-targeted ferritin nanocaged ultra-high dose of doxorubicin for hepatocellular carcinoma therapy. Theranostics. (2019) 9:2167–82. doi: 10.7150/thno.30867
21. Booth L, Roberts JL, Cash DR, Tavallai S, Jean S, Fidanza A, et al. GRP78/BiP/HSPA5/Dna K is a universal therapeutic target for human disease. J Cell Physiol. (2015) 230:1661–76. doi: 10.1002/jcp.24919
22. Cerezo M, Lehraiki A, Millet A, Rouaud F, Plaisant M, Jaune E, et al. Compounds triggering ER stress exert anti-melanoma effects and overcome BRAF inhibitor resistance. Cancer Cell. (2016) 30:183. doi: 10.1016/j.ccell.2016.06.007
23. Mujumdar N, Banerjee S, Chen Z, Sangwan V, Chugh R, Dudeja V, et al. Triptolide activates unfolded protein response leading to chronic ER stress in pancreatic cancer cells. Am J Physiol Gastrointest Liver Physiol. (2014) 306:G1011–20. doi: 10.1152/ajpgi.00466.2013
24. Malhi H, Kaufman RJ. Endoplasmic reticulum stress in liver disease. J Hepatol. (2011) 54:795–809. doi: 10.1016/j.jhep.2010.11.005
25. Yuan XP, Dong M, Li X, Zhou JP. GRP78 promotes the invasion of pancreatic cancer cells by FAK and JNK. Mol Cell Biochem. (2015) 398:55–62. doi: 10.1007/s11010-014-2204-2
26. Chang YJ, Chiu CC, Wu CH, An J, Wu CC, Liu TZ, et al. Glucose-regulated protein 78 (GRP78) silencing enhances cell migration but does not influence cell proliferation in hepatocellular carcinoma. Ann Surg Oncol. (2010) 17:1703–9. doi: 10.1245/s10434-010-0912-8
27. Al-Hashimi AA, Lebeau P, Majeed F, Polena E, Lhotak S, Collins CAF, et al. Autoantibodies against the cell surface-associated chaperone GRP78 stimulate tumor growth via tissue factor. J Biol Chem. (2017) 292:21180–92. doi: 10.1074/jbc.M117.799908
28. Zoni E, Chen L, Karkampouna S, Granchi Z, Verhoef EI, La Manna F, et al. CRIPTO and its signaling partner GRP78 drive the metastatic phenotype in human osteotropic prostate cancer. Oncogene. (2017) 36:4739–49. doi: 10.1038/onc.2017.87
29. Shen J, Ha DP, Zhu G, Rangel DF, Kobielak A, Gill PS, et al. GRP78 haploinsufficiency suppresses acinar-to-ductal metaplasia, signaling, and mutant Kras-driven pancreatic tumorigenesis in mice. Proc Natl Acad Sci USA. (2017) 114:E40209. doi: 10.1073/pnas.1616060114
30. Liu J, Fan L, Yu H, Zhang J, He Y, Feng D, et al. Endoplasmic reticulum stress causes liver cancer cells to release exosomal miR-23a-3p and up-regulate programmed death ligand 1 expression in macrophages. Hepatology. (2019) 70:241–58. doi: 10.1002/hep.30607
31. La X, Zhang L, Yang Y, Li H, Song G, Li Z. Tumor-secreted GRP78 facilitates the migration of macrophages into tumors by promoting cytoskeleton remodeling. Cell Signal. (2019) 60:1–16. doi: 10.1016/j.cellsig.2019.04.004
32. Tang Z, Li D, Hou S, Zhu X. The cancer exosomes: clinical implications, applications and challenges. Int J Cancer. (2020) 146:2946–59. doi: 10.1002/ijc.32762
33. Liu J, Li D, Luo H, Zhu X. Circular RNAs: the star molecules in cancer. Mol Aspects Med. (2019) 70:141–52. doi: 10.1016/j.mam.2019.10.006
34. Abdel Malek MA, Jagannathan S, Malek E, Sayed DM, Elgammal SA, Abd El-Azeem HG, et al. Molecular chaperone GRP78 enhances aggresome delivery to autophagosomes to promote drug resistance in multiple myeloma. Oncotarget. (2015) 6:3098–110. doi: 10.18632/oncotarget.3075
35. Aran G, Sanjurjo L, Barcena C, Simon-Coma M, Tellez E, Vazquez-Vitali M, et al. CD5L is upregulated in hepatocellular carcinoma and promotes liver cancer cell proliferation and antiapoptotic responses by binding to HSPA5 (GRP78). FASEB J. (2018) 32:3878–91. doi: 10.1096/fj.201700941RR
36. Zhu X, Chen MS, Tian LW, Li DP, Xu PL, Lin MC, et al. Single nucleotide polymorphism of rs430397 in the fifth intron of GRP78 gene and clinical relevance of primary hepatocellular carcinoma in Han Chinese: risk and prognosis. Int J Cancer. (2009) 125:1352–7. doi: 10.1002/ijc.24487
37. Kurowska P, Mlyczynska E, Dawid M, Opydo-Chanek M, Dupont J, Rak A. In vitro effects of vaspin on porcine granulosa cell proliferation, cell cycle progression, and apoptosis by activation of GRP78 receptor and several kinase signaling pathways including MAP3/1, AKT. and STAT3. Int J Mol Sci. (2019) 20:5816. doi: 10.3390/ijms20225816
38. Hebert-Schuster M, Rotta BE, Kirkpatrick B, Guibourdenche J, Cohen M. The interplay between glucose-regulated protein 78 (GRP78) and steroids in the reproductive system. Int J Mol Sci. (2018) 19:1842. doi: 10.3390/ijms19071842
39. Bian T, Tagmount A, Vulpe C, Vijendra KC, Xing C. CXL146, a novel 4H-chromene derivative, targets GRP78 to selectively eliminate multidrug-resistant cancer cells. Mol Pharmacol. (2020) 97:402–408. doi: 10.1124/mol.119.118745
40. Cook KL, Soto-Pantoja DR, Clarke PA, Cruz MI, Zwart A, Warri A, et al. Endoplasmic reticulum stress protein GRP78 modulates lipid metabolism to control drug sensitivity and antitumor immunity in breast cancer. Cancer Res. (2016) 76:5657–70. doi: 10.1158/0008-5472.CAN-15-2616
41. Ahmad M, Hahn IF, Chatterjee S. GRP78 up-regulation leads to hypersensitization to cisplatin in A549 lung cancer cells. Anticancer Res. (2014) 34:3493–500.
42. Chang YJ, Tai CJ, Kuo LJ, Wei PL, Liang HH, Liu TZ, et al. Glucose-regulated protein 78 (GRP78) mediated the efficacy to curcumin treatment on hepatocellular carcinoma. Ann Surg Oncol. (2011) 18:2395–403. doi: 10.1245/s10434-011-1597-3
43. Zhao L, Li H, Shi Y, Wang G, Liu L, Su C, et al. Nanoparticles inhibit cancer cell invasion and enhance antitumor efficiency by targeted drug delivery via cell surface-related GRP78. Int J Nanomed. (2015) 10:245–56. doi: 10.2147/IJN.S74868
44. Srivastava P. Interaction of heat shock proteins with peptides and antigen presenting cells: chaperoning of the innate and adaptive immune responses. Annu Rev Immunol. (2002) 20:395–425. doi: 10.1146/annurev.immunol.20.100301.064801
45. Ebner DK, Tinganelli W, Helm A, Bisio A, Yamada S, Kamada T, et al. The immunoregulatory potential of particle radiation in cancer therapy. Front Immunol. (2017) 8:99. doi: 10.3389/fimmu.2017.00099
46. Wei D, Li NL, Zeng Y, Liu B, Kumthip K, Wang TT, et al. The molecular chaperone GRP78 contributes to toll-like receptor 3-mediated innate immune response to hepatitis C virus in hepatocytes. J Biol Chem. (2016) 291:12294–309. doi: 10.1074/jbc.M115.711598
47. Schafer M, Granato DC, Krossa S, Bartels AK, Yokoo S, Dusterhoft S, et al. GRP78 protects a disintegrin and metalloprotease 17 against protein-disulfide isomerase A6 catalyzed inactivation. FEBS Lett. (2017) 591:3567–87. doi: 10.1002/1873-3468.12858
48. Zou Z, Tao T, Li H, Zhu X. mTOR signaling pathway and mTOR inhibitors in cancer: progress and challenges. Cell Biosci. (2020) 10:31. doi: 10.1186/s13578-020-00396-1
49. Liang X, Li D, Leng S, Zhu X. RNA-based pharmacotherapy for tumors: from bench to clinic and back. Biomed Pharmacother. (2020) 125:109997. doi: 10.1016/j.biopha.2020.109997
50. Lee AS. GRP78 induction in cancer: therapeutic and prognostic implications. Cancer Res. (2007) 67:3496–9. doi: 10.1158/0008-5472.CAN-07-0325
51. Zhu X, Lin MCM, Fan W, Tian L, Wang J, Ng SS, et al. An intronic polymorphism in GRP78 improves chemotherapeutic prediction in non-small cell lung cancer. Chest. (2012) 141:1466–72. doi: 10.1378/chest.11-0469
52. Dadey DYA, Kapoor V, Hoye K, Khudanyan A, Collins A, Thotala D, et al. Antibody targeting GRP78 enhances the efficacy of radiation therapy in human glioblastoma and non-small cell lung cancer cell lines and tumor models. Clin Cancer Res. (2017) 23:2556–64. doi: 10.1158/1078-0432.CCR-16-1935
53. Tan S, Li D, Zhu X. Cancer immunotherapy: Pros, cons and beyond. Biomed Pharmacother. (2020) 124:109821. doi: 10.1016/j.biopha.2020.109821
54. Zhu X, Luo H, Xu Y. Transcriptome analysis reveals an important candidate gene involved in both nodal metastasis and prognosis in lung adenocarcinoma. Cell Biosci. (2019) 9:92. doi: 10.1186/s13578-019-0356-1
55. Zhu X, Zhang J, Fan W, Wang F, Yao H, Wang Z, et al. The rs391957 variant cis-regulating oncogene GRP78 expression contributes to the risk of hepatocellular carcinoma. Carcinogenesis. (2013) 34:1273–80. doi: 10.1093/carcin/bgt061
Keywords: GRP78, endoplasmic reticulum stress, autophagy, apoptosis, tumor-targeted therapy
Citation: Lu G, Luo H and Zhu X (2020) Targeting the GRP78 Pathway for Cancer Therapy. Front. Med. 7:351. doi: 10.3389/fmed.2020.00351
Received: 06 March 2020; Accepted: 11 June 2020;
Published: 30 July 2020.
Edited by:
Antonio Recchiuti, University of Studies G. d'Annunzio Chieti and Pescara, ItalyReviewed by:
Nan Chiang, Brigham and Women's Hospital and Harvard Medical School, United StatesLei Yang, Jiujiang University, China
Copyright © 2020 Lu, Luo and Zhu. This is an open-access article distributed under the terms of the Creative Commons Attribution License (CC BY). The use, distribution or reproduction in other forums is permitted, provided the original author(s) and the copyright owner(s) are credited and that the original publication in this journal is cited, in accordance with accepted academic practice. No use, distribution or reproduction is permitted which does not comply with these terms.
*Correspondence: Hui Luo, luohui@gdmu.edu.cn; Xiao Zhu, xzhu@gdmu.edu.cn