People having hematological disorders and hypercoagulability state need extra precautions because of the increased risk of thrombosis after COVID-19 vaccination
- 1School of Medicine, Koc University, Sariyer, Istanbul, Türkiye
- 2Koc University Research Center for Translational Medicine (KUTTAM), Sariyer, Istanbul, Türkiye
Introduction
Millions of people have been infected and died because of the COVID-19 pandemic since December 2019, which is not the first pandemic the world has faced (1). Older people and individuals with comorbidities such as cancer, diabetes mellitus, cardiovascular diseases, hematological disorders, immune disease, enzyme deficiencies, and chronic respiratory diseases are reported to be more vulnerable to COVID-19, making them major risk groups (2–5). According to the literature, people with hematological disorders and hypercoagulability states are more vulnerable to COVID-19 infection because of the increased risk of adverse health effects and mortality. However, the impact of the COVID-19 vaccination has not been widely investigated in patients having hematological disorders such as glucose 6-phosphate dehydrogenase (G6PD) deficiency, thalassemia, sickle cell disease (SCD), pyruvate kinase enzyme deficiency (PKD), thrombophilia, hypereosinophilic syndromes, Glanzmann syndrome, sticky platelet syndrome, immune thrombocytopenia, and antithrombin deficiency (6, 7).
COVID-19 infection and COVID-19 vaccines can trigger endothelial damage, inflammation, platelet activation, cytokine storm, oxidative stress, and altered coagulation, contributing to thrombosis in patients (8). Platelets play a major role in thrombosis and inflammatory and immune processes; thus, they have a frontline effect on COVID-19 pathogenesis. Platelet hyperactivity, platelet aggregation, increased platelet–platelet interaction, increased gene expression, adhesion, and spreading in the platelets have been reported in patients infected with COVID-19. On the other hand, platelet hyperactivation has been found in both severe and non-severe forms of COVID-19 disease (9–11). Endothelial injury, coagulation, and thrombosis are directly associated with the disease severity and increased mortality risk in patients infected with COVID-19 and people with hypercoagulability (12, 13). Despite COVID-19 vaccines, people are still infected and die since the virus mutates and spreads quickly. Although vaccination reduces the mortality rate, the severity of the disease, and hospitalization, several studies reported that some COVID-19 vaccines might cause adverse health effects in people having hematological disorders or hypercoagulability state because of increased risk of thrombosis and hemolysis (14).
Therefore, extra caution and supportive care can be crucial for those patients after COVID-19 vaccination (12–15). The severity of COVID-19 infection and possible post-vaccination complications can be monitored via coagulation and thrombosis-inducing factors (15) such as increased levels of factor VIII, fibrinogen, plasmin activator inhibitor-1 (PAI-1), Willebrand factor (VWF), tissue factor expression, thrombin generation, and platelet activation and decreased concentrations of antithrombin, protein C, and thrombomodulin (16). In this context, we discussed the possible impact of the COVID-19 vaccination on people with hematological and hypercoagulability disorders correlated with the increased risk of thrombosis.
COVID-19 vaccine-induced thrombotic events
People are vaccinated against COVID-19 infection via several types of vaccines, including inactivated, viral vector, and mRNA; however, there is an increasing concern about COVID-19 vaccines about their safety. Thrombosis, platelet aggregation, platelet activity, embolism, and thrombocytopenia have been investigated in healthy individuals vaccinated with adenoviral or mRNA vaccines. Several studies have reported that no vaccine-induced side effects such as persistent platelet aggregation, activation, or plasma thrombin generation have been observed in healthy individuals after COVID-19 vaccination in the long or short term (17–19). On the contrary, viral vector and mRNA COVID-19 vaccines might cause mild-to-severe adverse health effects in people with blood disorders, according to the literature. Thrombosis, portal vein thrombosis (PVT), immune thrombotic thrombocytopenia (ITT), deep vein thrombosis (DVT), vaccine-induced immune thrombocytopenia (VITT), and heparin-induced thrombocytopenia (HIT) are reported as vaccine-induced adverse effects in people with blood disorders; thus, anticoagulation treatment following vaccination (non-heparin anticoagulant and intravenous immunoglobulin) has been recommended by the Expert Hematology Panel in March 2021 and the National Institute for Health Care Excellence (Figure 1) (20). NETosis is reported as the major contributor to VITT and HIT in patients with COVID-19, and NETs formed by neutrophils are involved in the innate immunological response as a first-line defense against pathogens. Increased levels of NETs' formation result in the overactivated immune cells and platelets associated with increased coagulation and endothelial damage, according to the literature (Figure 1) (21). Further studies should be conducted to reveal the mechanisms behind COVID-19 vaccine-induced thrombosis in patients having hematological disorders to prevent vaccine-related complications.
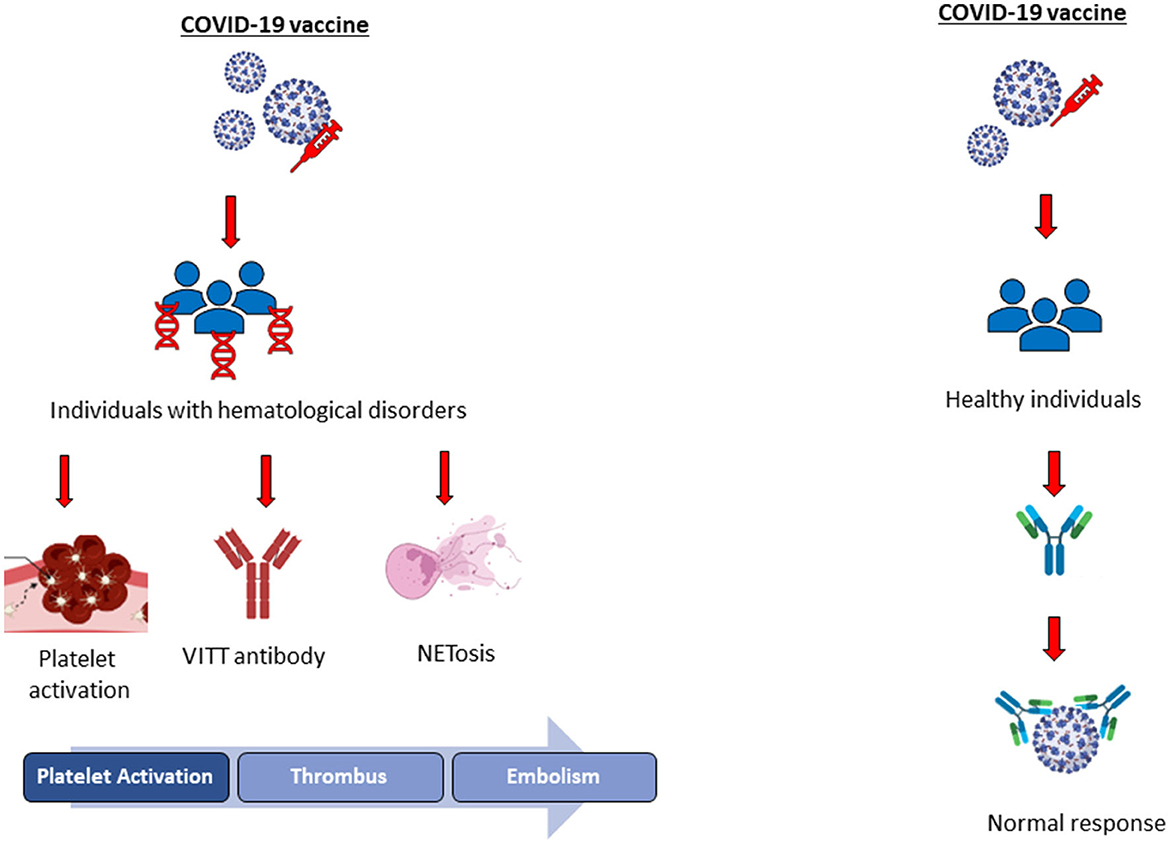
Figure 1. Illustration of COVID-19 vaccine-induced adverse health effects in healthy individuals and people with hematological disorders.
The impact of COVID-19 vaccines on people with hematological and hypercoagulability state disorders
People with hematological or hypercoagulability disorders such as G6PD deficiency, thalassemia, SCD, pyruvate dehydrogenase deficiency, thrombophilia, and sticky platelet syndrome have a higher risk of developing thrombosis, anemia, hemolysis, and embolism than healthy individuals. G6PD enzyme is the rate-limiting enzyme in the pentose phosphate pathway responsible for producing NADPH involved in maintaining the redox balance in the cell (22–25). Therefore, G6PD enzyme deficiency causes enhanced oxidative stress directly associated with hemoglobin denaturation and intravascular hemolysis (23, 25–27). According to the literature, people with G6PD deficiency can develop life-threatening hemolytic anemia and thrombosis after COVID-19 vaccination or during COVID-19 infection (2–4, 28). On the contrary, PKD is the second most common enzyme deficiency associated with hemolytic anemia, following G6PD deficiency. Since PKD shows clinical heterogeneity due to the type of the mutation, either autosomal recessive or dominant, the disease can be asymptomatic, and hemolysis occurs under stress conditions. Hemolysis, thrombosis, and decreased glycolysis have been found in people with PKD; however, no publication correlates PKD with COVID-19 infection or vaccination. Further studies should be conducted to evaluate the impact of the COVID-19 disease and vaccines on those patients (29, 30).
Thalassemia and SCD are the most common hemoglobin disorders or hemoglobinopathies, and people with hemoglobinopathies require lifelong follow-up and therapy (31). Thrombosis, hypercoagulability state, hemolysis, anemia, and embolism are clinical symptoms of hemoglobinopathies that increase those patients' mortality risk (32). Thalassemia is characterized by the defects of one or more hemoglobin chains and phenotypes of the disease ranging from severe anemia to asymptomatic individuals classified as thalassemia major, intermedia, and minor (33, 34). SCD is another type of hemoglobinopathy characterized by chronic anemia, hemolysis, and vasculopathy (35). Since people with thalassemia or SCD are more vulnerable to COVID-19 infection, they have a higher risk of hospitalization, mortality, and complications (36). Although vaccination enables immunity in both thalassemia and patients with SCD, some people with thalassemia showed vaccine-related adverse effects, including decreased hemoglobin levels and hemolysis (37). Those patients should be followed up closely following the vaccination because of vaso-occlusive crisis (VOC) along with increased levels of white blood cells (WBC) and liver enzyme; in contrast, a significant decrease in hemoglobin and platelet levels has been observed after COVID-19 vaccination (38).
Thrombophilia is characterized by abnormal blood coagulation in almost 50% of people with past thrombotic events. It can be congenital or acquired with clinical symptoms including deep vein thrombosis (DVT) and pulmonary embolism (PE). Protein S deficiency, protein C deficiency, antithrombin deficiency, antiphospholipid syndrome, factor V Leiden (FVL), and hereditary thrombophilia are the types of thrombophilia. Patients with thrombophilia have an increased tendency to clot, venous thrombosis, and thromboembolism because of the hypercoagulability observed in some patients with COVID-19 (39, 40). Antiphospholipid syndrome, also known as Hughes syndrome, is characterized by arterial, venous, or small vessel thrombosis associated with an increased risk of mortality in the patients. It has been reported that antiphospholipid antibodies are found in healthy individuals at approximately 1–5%, which is associated with antiphospholipid syndrome. On the contrary, these antibodies are also determined in patients infected with COVID-19; also Seeley et al. (41) reported that a woman with antiphospholipid syndrome has catastrophic episodes following COVID-19 vaccination (41).
Factor V Leiden is the most common hereditary thrombophilia characterized by a decreased anticoagulant response, increased risk of deep venous thrombosis, and pulmonary embolism (42). People with FVL are more vulnerable to COVID-19 infection since the increased risk of pulmonary embolism and thrombosis; moreover, deep vein thrombosis has been reported after COVID-19 vaccination in a patient with FVL (43). Protein C deficiency is a rare hereditary or acquired life-threatening risk factor that can cause various health problems, such as thrombophilia to venous thromboembolism; it can also be asymptomatic (44). However, there is no publication about protein C deficiency and COVID-19 infection and/or the COVID-19 vaccine in the literature. Protein S deficiency is one of the hypercoagulability syndromes, and the synthesis of this plasma protein depends on vitamin K. Protein S has a central role in protein coagulation, and deficiency of protein S causes thrombotic complications in COVID-19-infected individuals (45, 46). On the contrary, cerebral venous sinus thrombosis has been reported in a patient with protein S deficiency following COVID-19 vaccination; thus, people with thrombophilia should be followed closely after vaccination or during COVID-19 (47).
Immune thrombocytopenia (ITP) is a rare autoimmune disorder with an incidence rate between 1.6 and 3.9 per 100 000 and is commonly found in women. In this disorder, the immune system destroys platelets; therefore, blood cannot clot accurately, leading to the increased risk of venous and arterial thromboembolism. People with ITP are reported to be more vulnerable to COVID-19 vaccination (48–50). Thrombocytopenia and purpuric lesions were observed after several mRNA COVID-19 vaccines, and adverse health effects were reported in patients with ITP (51, 52). Moreover, hyperviscosity syndrome (HVS) is a rare syndrome characterized by high immunoglobins and proteins, which cause an increase in blood thickness. Plasma hyperviscosity has been found in both COVID-19 infection and after vaccination, correlated with disease severity; therefore, people with HVS syndrome can be more vulnerable to COVID-19 infection and vaccination (53, 54).
Sticky platelet syndrome is an autosomal dominant disease characterized by platelet aggregation leading to the increased risk of thrombosis and embolism. According to the literature, people with this syndrome have a higher risk of developing thrombosis during COVID-19 infection (55, 56). Glanzmann thrombasthenia (GT) is a rare autoimmune disorder that can be inherited in an autosomal recessive manner or acquired. Platelet aggregation and decreased coagulation lead to bleeding in patients because of the defects in integrin αIIbβ3. Since thrombosis and embolism are observed in people with GT, they can have severe illness and increased mortality risk during COVID-19 infection, according to the literature (56). However, no detailed study or information about the impact of the COVID-19 infection or vaccination on those patients should be further investigated.
Conclusion
People are still infected and die because of the COVID-19 virus despite vaccination since the virus mutates and spreads very quickly. Therefore, as major risk groups, older people and individuals with comorbidities should be followed up carefully. People with hematological disorders and hypercoagulability are more vulnerable to COVID-19 infection and vaccination because of the increased risk of thrombosis according to the literature. This is a knife-edge situation because viral vector and mRNA COVID-19 vaccines might cause mild-to-severe adverse health effects but also provide immunity in patients. Therefore, people with hematological disorders are required close follow-up during COVID-19 infection and after COVID-19 vaccines.
Author contributions
All authors listed have made a substantial, direct, and intellectual contribution to the work and approved it for publication.
Acknowledgments
The authors gratefully acknowledge the use of the services and facilities of the Koc University Research Center for Translational Medicine (KUTTAM), funded by the Presidency of Turkey, Presidency of Strategy and Budget. The content is solely the responsibility of the authors and does not necessarily represent the official views of the Presidency of Strategy and Budget.
Conflict of interest
The authors declare that the research was conducted in the absence of any commercial or financial relationships that could be construed as a potential conflict of interest.
Publisher's note
All claims expressed in this article are solely those of the authors and do not necessarily represent those of their affiliated organizations, or those of the publisher, the editors and the reviewers. Any product that may be evaluated in this article, or claim that may be made by its manufacturer, is not guaranteed or endorsed by the publisher.
References
1. Patterson GE, McIntyre KM, Clough HE, Rushton J. Societal impacts of pandemics: comparing COVID-19 with history to focus our response. Front Public Health. (2021) 9:630449. doi: 10.3389/fpubh.2021.630449
2. Aydemir D, Ulusu NN. Influence of lifestyle parameters – dietary habit, chronic stress and environmental factors, jobs – on the human health in relation to the COVID-19 pandemic. Disaster Med Public Health Prep. (2020) 14:e36–7. doi: 10.1017/dmp.2020.222
3. Aydemir D, Ulusu NN. People with blood disorders can be more vulnerable during COVID-19 pandemic: A hypothesis paper. Transfusion and Apheresis Sci. (2021) 60:103080. doi: 10.1016/j.transci.2021.103080
4. Aydemir D, Ulusu NN. Is glucose-6-phosphate dehydrogenase enzyme deficiency a factor in Coronavirus-19 (COVID-19) infections and deaths? Pathog Glob Health. (2020) 114:109–10. doi: 10.1080/20477724.2020.1751388
5. Motamedi H, Ari MM, Dashtbin S, Fathollahi M, Hossainpour H, Alvandi A, et al. An update review of globally reported SARS-CoV-2 vaccines in preclinical and clinical stages. Int Immunopharmacol. (2021) 96:107763. doi: 10.1016/j.intimp.2021.107763
6. Chan MY, Andreotti F, Becker RC. Hypercoagulable states in cardiovascular disease. Circulation. (2008) 118:2286–97. doi: 10.1161/CIRCULATIONAHA.108.778837
7. Emmerich J. Congenital and Acquired Hypercoagulable States Trauma Induced Coagulopathy. Cham: Springer International Publishing (2016). p. 435–52.
8. Gorog DA, Storey RF, Gurbel PA, Tantry US, Berger JS, Chan MY, et al. Current and novel biomarkers of thrombotic risk in COVID-19: a consensus statement from the international COVID-19 thrombosis biomarkers colloquium. Nat Rev Cardiol. (2022) 19:475–95. doi: 10.1038/s41569-021-00665-7
9. Shen S, Zhang J, Fang Y, Lu S, Wu J, Zheng X, et al. SARS-CoV-2 interacts with platelets and megakaryocytes via ACE2-independent mechanism. J Hematol Oncol. (2021) 14:72. doi: 10.1186/s13045-021-01082-6
10. Zaid Y, Puhm F, Allaeys I, Naya A, Oudghiri M, Khalki L, et al. Platelets Can Associate With SARS-CoV-2 RNA and Are Hyperactivated in COVID-19. Circ Res. (2020) 127:1404–18. doi: 10.1161/CIRCRESAHA.120.317703
11. Manne BK, Denorme F, Middleton EA, Portier I, Rowley JW, Stubben C, et al. Platelet gene expression and function in patients with COVID-19. Blood. (2020) 136:1317–29. doi: 10.1182/blood.2020007214
12. Savla SR, Prabhavalkar KS, Bhatt LK. Cytokine storm associated coagulation complications in COVID-19 patients: pathogenesis and management. Expert Rev Anti Infect Ther. (2021) 19:1397–413. doi: 10.1080/14787210.2021.1915129
13. Avila J, Long B, Holladay D, Gottlieb M. Thrombotic complications of COVID-19. Am J Emerg Med. (2021) 39:213–8. doi: 10.1016/j.ajem.2020.09.065
14. Altmann DM, Boyton RJ. COVID-19 vaccination: the road ahead. Science. (2022) 375:1127–32. doi: 10.1126/science.abn1755
15. Khan WH, Hashmi Z, Goel A, Ahmad R, Gupta K, Khan N, Alam I, Ahmed F, Ansari MA. COVID-19 pandemic and vaccines update on challenges and resolutions. Front Cell Infect Microbiol. (2021) 11: 690621. doi: 10.3389/fcimb.2021.690621
16. Mazzeffi MA, Chow JH, Tanaka K. COVID-19 Associated hypercoagulability: manifestations, mechanisms, and management. Shock. (2021) 55:465–71. doi: 10.1097/SHK.0000000000001660
17. Zhou Y, Nishikawa M, Kanno H, Yang R, Ibayashi Y, Xiao T, et al. Long-term effects of Pfizer-BioNTech COVID-19 vaccinations on platelets. Cytometry Part A. (2022) doi: 10.1002/cyto.a.24677
18. Campello E, Simion C, Bulato C, Radu CM, Gavasso S, Sartorello F, et al. Absence of hypercoagulability after nCoV-19 vaccination: an observational pilot study. Thromb Res. (2021) 205:24–8. doi: 10.1016/j.thromres.2021.06.016
19. Limami Y, Khalki L, Zaid N, Khyatti M, Turk J. el, Ammara M, Mtairag EM, Oudghiri M, Naya A, Taberkant M, et al. Oxford-astrazeneca ChAdOx1 COVID-19 vaccine does not alter platelet aggregation. Semin Thromb Hemost. (2022) 48:109–11. doi: 10.1055/s-0041-1728831
20. Misbah SA, Pavord S, Foster M. Dosing of intravenous immunoglobulin in COVID-19 vaccine-induced immune thrombocytopenia and thrombosis. J Clin Pathol. (2022) J Clin Path. 2022:208377. doi: 10.1136/jcp-2022-208377
21. Leung HHL, Perdomo J, Ahmadi Z, Zheng SS, Rashid FN, Enjeti A, et al. NETosis and thrombosis in vaccine-induced immune thrombotic thrombocytopenia. Nat Commun. (2022) 13:5206. doi: 10.1038/s41467-022-32946-1
22. Akbay E, Nuray Ulusu N, Töröner F, Ayvaz G, Taneri F, Aktürk M, et al. Effects of rosiglitazone treatment on the pentose phosphate pathway and glutathione-dependent enzymes in liver and kidney of rats fed a high-fat diet. Cur Ther Res. (2004) 65:79–89. doi: 10.1016/S0011-393X(04)90007-0
23. Tandogan B, Kuruüzüm-Uz A, Sengezer C, Güvenalp Z, Demirezer LÖ, Nuray Ulusu N. In vitro effects of rosmarinic acid on glutathione reductase and glucose 6-phosphate dehydrogenase. Pharm Biol. (2011) 49:587–94. doi: 10.3109/13880209.2010.533187
24. Ulusu NN, Gök M, Sayin Sakul AA, Ari N, Stefek M, Karasu Ç. Antioxidant SMe1EC2 modulates pentose phosphate pathway and glutathione-dependent enzyme activities in tissues of aged diabetic rats. Interdiscip Toxicol. (2017) 10:148–54. doi: 10.1515/intox-2017-0021
25. Aydemir D, Ulusu NN. The possible role of the glucose-6-phosphate dehydrogenase enzyme deficiency in the polyneuropathies. J Basic Clin Health Sci. (2020) 4:212–7. doi: 10.30621/jbachs.2020.1151
26. Evlice A, Ulusu NN. Glucose-6-phosphate dehydrogenase a novel hope on a blood-based diagnosis of Alzheimer's disease. Acta Neurol Belg. (2017) 117:229–34. doi: 10.1007/s13760-016-0666-6
27. Ulusu NN. Glucose-6-phosphate dehydrogenase deficiency and Alzheimer's disease: Partners in crime? The hypothesis. Med Hypotheses. (2015) 85:219–23. doi: 10.1016/j.mehy.2015.05.006
28. Gómez-Manzo S, Marcial-Quino J, Vanoye-Carlo A, Serrano-Posada H, Ortega-Cuellar D, González-Valdez A, et al. Glucose-6-phosphate dehydrogenase: update and analysis of new mutations around the world. Int J Mol Sci. (2016) 17:2069. doi: 10.3390/ijms17122069
29. Roy NBA, Telfer P, Eleftheriou P, de la Fuente J, Drasar E, Shah F, et al. Protecting vulnerable patients with inherited anaemias from unnecessary death during the COVID-19 pandemic. Br J Haematol. (2020) 189:635–9. doi: 10.1111/bjh.16687
30. Chonat S, Eber SW, Holzhauer S, Kollmar N, Morton DH, et al. Pyruvate kinase deficiency in children. Pediatr Blood Cancer. (2021) 68:29148. doi: 10.1002/pbc.29148
31. Farmakis D, Giakoumis A, Cannon L, Angastiniotis M, Eleftheriou A. COVID-19 and thalassaemia: a position statement of the thalassaemia international federation. Eur J Haematol. (2020) 105:378–86. doi: 10.1111/ejh.13476
32. Ataga KI. Hypercoagulability and thrombotic complications in hemolytic anemias. Haematologica. (2009) 94:1481–4. doi: 10.3324/haematol.2009.013672
33. Zheng L, Huang H, Wu X, Shen Q, Chen M, Wang M, et al. Erythrocyte indices and hemoglobin analysis for α-thalassemia screening in an area with high carrying rate. Indian J Hematol Blood Trans. (2022) 38:352–8. doi: 10.1007/s12288-021-01449-2
34. Galanello R, Origa R. Beta-thalassemia. Orphanet J Rare Dis. (2010) 5:11. doi: 10.1186/1750-1172-5-11
35. Rees DC, Williams TN, Gladwin MT. Sickle-cell disease. Lancet. (2010) 376:2018–31. doi: 10.1016/S0140-6736(10)61029-X
36. Radhwi OO, Jan H, Waheeb A, Alamri SS, Alahwal HM, Denetiu I, et al. Immunogenicity of the BNT162b2 COVID-19 mRNA and ChAdOx1 nCoV-19 vaccines in patients with hemoglobinopathies. Vaccines. (2022) 10:151. doi: 10.3390/vaccines10020151
37. Delaporta P, Kyriakopoulou D, Ioakeimidou N, Solomou E, Binenbaum I, Toutoudaki K, et al. S124: adverse events following covid-19 vaccination in transfusion-dependent -thalassemia patients. Hemasphere. (2022) 6:13–13. doi: 10.1097/01.HS9.0000821464.52797.65
38. Alkindi S, Elsadek RA, Pathare A. Safety warning for ChAdOx1 nCov-19 vaccine in patients with sickle cell disease. Mediterr J Hematol Infect Dis. (2021) 13:e2021059. doi: 10.4084/MJHID.2021.059
39. Astrit D. Hereditary thrombophilia. Acta Biomed. (2019) 90:44–6. doi: 10.23750/abm.v90i10-S.8758
40. Ali E, Ibrahim I. Multi-factorial mechanism behind COVID-19 related thrombosis. Med Archives. (2022) 76:62. doi: 10.5455/medarh.2022.76.62-65
41. Seeley EA, Zimmer M, Berghea R. Suspected COVID-19 immunization-induced probable catastrophic antiphospholipid syndrome. Cureus. (2022) 14: 27313. doi: 10.7759/cureus.27313
42. Kujovich JL. Factor V Leiden thrombophilia. Genetics Med. (2011) 13:1–16. doi: 10.1097/GIM.0b013e3181faa0f2
43. Carli G, Nichele I, Ruggeri M, Barra S, Tosetto A. Deep vein thrombosis (DVT) occurring shortly after the second dose of mRNA SARS-CoV-2 vaccine. Intern Emerg Med. (2021) 16:803–4. doi: 10.1007/s11739-021-02685-0
44. Dinarvand P, Moser KA. Protein C Deficiency. Arch Pathol Lab Med. (2019) 143:1281–5. doi: 10.5858/arpa.2017-0403-RS
45. Alshaikh N. Protein S: a central regulator of blood coagulation. Clin Lab. (2022) 68: 21101. doi: 10.7754/Clin.Lab.2021.211010
46. Dahlbäck B. Vitamin K–Dependent protein s: beyond the protein c pathway. Semin Thromb Hemost. (2018) 44:176–84. doi: 10.1055/s-0037-1604092
47. Mele F, Tafuri S, Stefanizzi PD, Amati A, Calvano M, Leonardelli M, et al. Cerebral venous sinus thrombosis after COVID-19 vaccination and congenital deficiency of coagulation factors: is there a correlation? Hum Vaccin Immunother. (2022) 18: 2095166. doi: 10.1080/21645515.2022.2095166
48. Bomhof G, Mutsaers PGNJ, Leebeek FWG, Boekhorst PAW, Hofland J, Croles FN, Jansen AJG. COVID-19-associated immune thrombocytopenia. Br J Haematol. (2020) 190:16850. doi: 10.1111/bjh.16850
49. Tarawneh O, Tarawneh H. Immune thrombocytopenia in a 22 -year-old post COVID-19 vaccine. Am J Hematol. (2021) 96:26106. doi: 10.1002/ajh.26106
50. Alam W. COVID-19 vaccine-induced immune thrombotic thrombocytopenia: A review of the potential mechanisms and proposed management. Sci Prog. (2021) 104:003685042110259. doi: 10.1177/00368504211025927
51. Pishko AM, Bussel JB, Cines DB. COVID-19 vaccination and immune thrombocytopenia. Nat Med. (2021) 27:1145–6. doi: 10.1038/s41591-021-01419-1
52. Visser C, Swinkels M, van Werkhoven ED, Croles FN, Noordzij-Nooteboom HS, Eefting M, et al. COVID-19 vaccination in patients with immune thrombocytopenia. Blood Adv. (2022) 6:1637–44. doi: 10.1182/bloodadvances.2021006379
53. Mungmunpuntipantip R, Wiwanitkit V. Margem de Segurança para Hiperviscosidade Plasmática para Prevenção de Trombose em Pacientes com Doença Cardiovascular após Vacinação contra COVID-19. Arq Bras Cardiol. (2021) 117:1217–8. doi: 10.36660/abc.20210555
54. Truong AD, Auld SC, Barker NA, Friend S, Wynn AT, Cobb J, et al. Therapeutic plasma exchange for COVID-19-associated hyperviscosity. Transfusion. (2021) 61:1029–34. doi: 10.1111/trf.16218
55. Kubisz P, Stasko J, Holly P. Sticky platelet syndrome. Semin Thromb Hemost. (2013) 39:674–83. doi: 10.1055/s-0033-1353394
Keywords: hematological disorders, thrombosis, hypercoagulability, COVID-19, vaccination
Citation: Aydemir D and Ulusu NN (2023) People having hematological disorders and hypercoagulability state need extra precautions because of the increased risk of thrombosis after COVID-19 vaccination. Front. Med. 9:1082611. doi: 10.3389/fmed.2022.1082611
Received: 28 October 2022; Accepted: 29 December 2022;
Published: 14 February 2023.
Edited by:
Oral Alpan, Amerimmune, United StatesReviewed by:
Younes Zaid, Mohammed V University, MoroccoCopyright © 2023 Aydemir and Ulusu. This is an open-access article distributed under the terms of the Creative Commons Attribution License (CC BY). The use, distribution or reproduction in other forums is permitted, provided the original author(s) and the copyright owner(s) are credited and that the original publication in this journal is cited, in accordance with accepted academic practice. No use, distribution or reproduction is permitted which does not comply with these terms.
*Correspondence: Duygu Aydemir, daydemir16@ku.edu.tr; Nuriye Nuray Ulusu,
nulusu@ku.edu.tr