Longitudinal evaluation of the impact of immunosuppressive regimen on immune responses to COVID-19 vaccination in kidney transplant recipients
- 1Vaccine Research Institute, Université Paris-Est Créteil, Faculté de Médecine, INSERM U955, Créteil, France
- 2Service of Immunology and Allergy, Department of Medicine, Lausanne University Hospital, University of Lausanne, Lausanne, Switzerland
- 3Department of Nephrology, Assistance Publique Hopitaux de Paris (APHP), Creteil, France
- 4Paris-Saclay University, Gustave Roussy Institut, Institut National de la Santé et de la Recherche Médicale (INSERM) - Unité Mixte de Recherche (UMR) 1186, Integrative Tumor Immunology and Immunotherapy, Villejuif, France
- 5Université Paris-Est Créteil, Faculté de Médecine, INSERM U955, Créteil, France
- 6Swiss Vaccine Research Institute, Lausanne University Hospital, University of Lausanne, Lausanne, Switzerland
- 7Groupe Henri-Mondor Albert-Chenevier, Service Immunologie Clinique, Assistance Publique-Hôpitaux de Paris, Créteil, France
Immunocompromised patients have a high risk of death from SARS-CoV-2 infection. Vaccination with an mRNA vaccine may protect these patients against severe COVID-19. Several studies have evaluated the impact of immune-suppressive drug regimens on cellular and humoral responses to SARS-CoV-2 variants of concern in this context. We performed a prospective longitudinal study assessing specific humoral (binding and neutralizing antibodies against spike (S) and T-lymphocyte (cytokine secretion and polyfunctionality) immune responses to anti-COVID-19 vaccination with at least two doses of BNT162b2 mRNA vaccine in stable kidney transplant recipients (KTR) on calcineurin inhibitor (CNI)- or belatacept-based treatment regimens. Fifty-two KTR−31 receiving CNI and 21 receiving belatacept—were enrolled in this study. After two doses of vaccine, 46.9% of patients developed anti-S IgG. Anti-spike IgG antibodies were produced in only 21.4% of the patients in the belatacept group, vs. 83.3% of those in the CNI group. The Beta and Delta variants and, more importantly, the Omicron variant, were less well neutralized than the Wuhan strain. T-cell functions were also much weaker in the belatacept group than in the CNI group. Renal transplant patients have an impaired humoral response to BNT162b2 vaccination. Belatacept-based regimens severely weaken both humoral and cellular vaccine responses. Clinically, careful evaluations of at least binding IgG responses, and prophylactic or post-exposure strategies are strongly recommended for transplant recipients on belatacept-based regimens.
Introduction
Patients with a compromised immune system are highly susceptible to SARS-CoV-2 infection and are among those most at risk of developing severe COVID-19, long-term complications, or fatal disease (1, 2). These individuals are also less likely to respond to COVID-19 vaccines (3–5) because of their immunodeficiency, immunosuppressive treatment or clinical condition (6, 7).
Seroconversion rates following SARS-CoV-2 mRNA vaccination remain lower in kidney transplant recipients (KTR) than in healthy immunocompetent individuals after one, two, or even three vaccine injections, as recommended by the French health authorities since April 2021 (4, 5). This third (booster) injection increased seroconversion rates, but the frequency and magnitude of anti-Spike IgG responses remains lower in immunocompromised individuals than in the general population (7). This is unsurprisingly, as several previous studies in patients with kidney failure reported weak responses to vaccination against flu or hepatitis B, leading to the adaptation of vaccination protocols and recommendations to increase vaccine dose or the number of booster doses (8–10). The efficacy of such strategies for SARS-CoV-2 mRNA vaccines is unclear, but the weaker response to these vaccines in immunocompromised individuals is a general observation across all COVID-19 vaccine platforms (7).
Decreasing vaccine-induced protective immunity against SARS-CoV-2 variants of concern (VOCs) is another issue, with higher transmissibility and hospitalized breakthrough case rates among immunocompromised individuals (11). Moreover, vaccine-elicited anti-Spike (anti-S) IgG levels gradually decline over about 6 months in the general population, but much more rapidly immunocompromised individuals with solid cancers, inflammatory diseases, or after organ transplantation (6, 12–16).
The impact of immunosuppressive regimens on these cellular and humoral responses also warrants further investigation. Rates of seroconversion after COVID-19 vaccination are generally low in KTR treated with calcineurin inhibitors (CNI) or belatacept (CTLA-4 Ig), but with significant differences between these two regimen types (16–19). It remains unclear whether post-vaccination cellular immunity can compensate for waning immunity and/or immune escape by VOC, and few exploratory studies have evaluated cellular responses to vaccination in transplant patients (16). The different modes of action of CNI and belatacept may result in different impacts on cellular and humoral responses to vaccines. Belatacept is associated with higher rates of graft and patient survival after kidney transplantation, but can affect the balance between cellular (increasing the risk of T-cell rejection) and humoral (decreasing the risk of anti-HLA antibody production) rejection (20–24). It is therefore important to investigate the impact of these drugs on both cellular and humoral responses to vaccines. We characterized humoral responses by measuring levels of both binding and neutralizing IgG antibodies against the original, SARS-CoV-2 (2019-nCoV) and the Alpha, Beta, Gamma, Delta and Omicron variants, and functional T-cell responses to S peptides over a 6-month period in a prospective cohort of KTR treated with either CNI or belatacept.
Materials and methods
Patients
We enrolled 52 KTR in the VACOTRARE longitudinal single-center cohort, to evaluate the durability of immunity after COVID-19 mRNA vaccination. Patients received two injections of the BNT162b2 mRNA vaccine 4 weeks apart. At vaccination, 31 KTR were treated with CNI, and 21 were treated with belatacept (Table 1). During follow-up, 40 patients received a third injection (booster) of BNT162b2 mRNA vaccine in accordance with French national recommendations. Blood samples were collected before vaccination (pre-vacc), 15 days after the first dose (post-dose 1), 15 days after the second dose (post-dose 2), and then at months 3 (M3) and 6 (M6), for peripheral blood mononuclear cell (PBMC) and serum isolation. All adverse effects of vaccination and cases of viral infection were recorded. All patients gave written informed consent for the collection, storage, and use of biological samples. The study protocol was approved by the appropriate Ethics Committee (No. IDRCB: 2018-A01610-55).
Determinations of anti-spike IgG levels and VOC neutralization
The levels of binding and neutralizing IgG antibodies against Spike were determined in two Luminex-based assays (Luminex Corporation), as previously described (6, 25, 26). For binding assays, two different types of MagPlex beads (Luminex Corporation) were covalently coupled with the SARS-CoV-2 Spike protein trimer and nucleocapsid (NuC) protein. Beads were then incubated 1 h with a 1/300 serum dilution or a negative control of pre-pandemic pool of human AB serum (BioWest, VWR). After washing, we added anti-human IgG-PE (phycoerythrin) secondary antibody (One Lambda, Thermo Fisher Scientific) to each well and incubated the plate for 45 min. The plate was read with a Luminex 200 analyzer (Luminex Corporation, BioRad). The mean fluorescence intensity (MFI) signal of each serum was divided by the mean negative control MFI, to obtain a ratio. A conversion factor was applied to the ratio to obtain equivalent anti-S U/ml. For the Spike protein ACE2 surrogate neutralization assay, different types of MagPlex beads were covalently coupled with different spike variants (2019nCoV, Alpha, Beta, Gamma, Delta, Omicron) were incubated 1 h with serum samples at different dilutions (1:10; 1:30; 1:90; 1:270; 1:810; 1:2,430) in PBS. The negative control was as previously described. The positive control was a recombinant anti-Spike neutralizing antibody (CHUV, Lausanne). We added ACE2 mouse Fc fusion protein (EPFL, Lausanne) to the wells at a final concentration of 1 μg/ml. The plates were washed twice with PBS-Tween and anti-mouse IgG-PE secondary antibody (Invitrogen, Thermo Fisher Scientific) was added and the plates were read with a Luminex 200 analyzer (Luminex Corporation, BioRad). The negative control MFI was considered to correspond to 100% binding of the ACE2 receptor to the bead-coupled spike trimer. The MFI of the well containing the highest concentration (>1 μg/ml) of commercial anti-spike blocking antibody was considered to represent maximum inhibition. The percent inhibition of the spike protein trimer–ACE2 interaction was calculated as follows: % inhibition = (1 – ([MFI test dilution – MFI max inhibition]/[MFI max binding – MFI max inhibition]) × 100).
Characterization of spike-specific T-cell responses
Briefly, PBMCs were stimulated by overnight incubation with two pools of 15-mer peptides, overlapping by 11 amino acids, covering the whole spike protein of the SARS-CoV-2 reference strain Human 2019-nCoV HKU-SZ-005b (JPT Peptide Technologies, Berlin, Germany): S1 (168 peptides), S2 (144 peptides). Total S-specific responses were determined by summing S1 and S2 responses. Unstimulated cells were used as a negative control. The flow cytometry panel included a viability marker, CD3, CD4, and CD8 for T-cell lineage determination, and antibodies against IFN-γ, IL-2, and TNF. Data were acquired on a LSRII Fortessa 4-laser (488, 640, 561, and 405 nm) cytometer (BD Biosciences), and analyzed with FlowJo software version 9.9.6 (Tree Star Inc.).
Statistical analyses
Graphpad Prism software version 8 was used for non-linear four-parameter curve-fitting analyses, and for non-parametric statistics and plots, as described in the figure legends.
Results
Fifty-two patients were enrolled in the VACOTRARE cohort (Table 1). Mean ± SD follow-up since kidney transplantation was 6.4 ± 5.2 years. Mean age ± SD was 49.7 ± 14.2 years, and 40.7% of the patients were female. Thirty-one patients were treated with CNI, and 21 with belatacept. In the CNI group, 8/31 patients were treated with a CNI-mTOR inhibitor (mTORi) combination and 23/31 were treated with a CNI-anti-metabolite (MMF or azathioprine) combination. Belatacept was associated with MMF or azathioprine in 15 patients, and with mTORi in five patients. All but two patients received low doses of steroids. The trough levels of CNI and mTORi at the time of vaccination are shown in Table 1. Renal function, age and lymphocyte counts were similar in the CNI and belatacept groups (Table 1). All patients received at least two doses of BTN162b2 mRNA vaccine, and 40 received a booster. This third dose was not administered to patients who died (n = 2), or experienced symptomatic COVID-19 during follow-up (n = 7) or to patients refusing the booster (n = 3).
Blood samples were collected before the first vaccination (pre-vacc), 21 days after the first dose (post-dose 1), 14 days after the second dose (post-dose 2), and at M3 and M6 post-vaccination in patients treated with CNI (n = 18) or belatacept (n = 14). Two weeks after the second dose, 15/32 patients (46.9%) were tested positive for binding IgG antibodies against S. Anti-S IgG levels (median [IQR]) were significantly lower in the belatacept (1 U/ml [0.01–6]) than in the CNI group (101 U/ml [2.5–1466]); (P = 0.01), with 21.4% (3/14 patients) and 83.3% (15/18 patients) responders, respectively (Figure 1). KTR in the CNI group received a booster between the post-dose 2 and M3 visits (n = 5) or between the M3 and M6 visits (n = 9). The rates of responders with detectable anti-S IgG antibodies at the M3 and M6 time points in this group were 11/18 (61.1%) and 15/18 (83.3%), respectively, and all but three responders had received boosters by the M6 visit. In the belatacept group, 6/14 patients had received their boosters by the M3 visit, and 10/14 had received their booster by the M6 visit. Most KTR remained non-responders, with anti-S IgG antibody levels below the limit of detection in all but 2/14 patients (14.2%) at M3 and 3/14 patients (21.4%) at M6. All seropositive patients had received the booster by the M6 visit. Response rates differed significantly between the groups at M3 and M6 post-vaccination, with 61% and 83.3% responders at M3 and M6, respectively, in the CNI group vs. only 14.2 and 21.4%, respectively, in the belatacept group (P = 0.007 and P = 0.002 at M3 and M6, respectively; chi-squared test). Binding IgG anti-S antibody levels remained high in the CNI group at M3 and M6 (median [IQR]: 421.5 [4.7–1,812] U/ml and 1,481 [41.7–2,180] U/ml, respectively, P = 0.04, Wilcoxon t-test) (Figure 1).
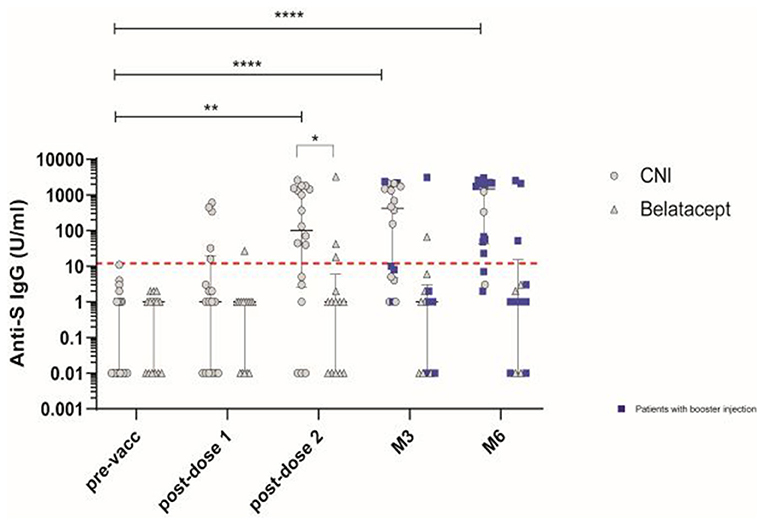
Figure 1. Antibody responses induced by vaccination with the BNT162b2 mRNA vaccine in patients treated with calcineurin inhibitor (CNI) or belatacept. SARS-CoV-2-specific IgG binding antibody responses directed against the native trimeric S protein at baseline (pre-vacc), 21 days after the first dose (post-dose 1), 14 days after the second dose (post-dose 2), and at M3 and M6 post-vaccination in patients treated with CNI (n = 18) (circles)or belatacept (n = 14) (triangles). Blue squares indicate patients who received the 3rd (booster) dose of vaccine at least 10 days before the follow-up visit. The dashed red line indicates the positivity threshold (12 U/ml). Median values ± IQR are shown. Mann-Whitney tests and, Friedman and Dunn's multiple comparison tests were used for statistical analysis. Statistical p value are indicated as followed (*P < 0.05, **P < 0.01, ***P < 0.001, ****P < 0.0001).
We determined nAb responses to vaccination against 2019-nCoV Wuhan and the Alpha, Beta, Gamma, Delta and Omicron variants, in a cell- and virus-free assay (6, 25, 26), in patients with detectable levels of binding IgG from the CNI group. The frequency of responders with nAb against 2019-nCoV Wuhan was 60% of KTR post-dose 2, remaining stable at M3 and M6 (Figure 2A). At M6, the response rate was 60% against Alpha, 55% against Beta, 55% against Gamma, 50% against Delta, and 30% against Omicron (Figure 2A). We evaluated the magnitude of the nAb response, using IC50 dilutions > 50 as the cutoff for positivity. For example, at M3, the IC50 titers against 2019-nCoV Wuhan, and the Delta, Alpha, Beta, Gamma and Omicron variants were (median [IQR]): 188 [125–467], 58 [41–143], 236.5 [81–336], 69.5 [33–116], 195.5 [72–233] and 28.5 [12–61], respectively. The corresponding values at M6 were 227 [38–87], 57 [18–180], 183 [14–399], 67 [20–147], 159 [18–343], and 33 [14–83]. At each time point, the IC50 titers against Beta, Delta and Omicron were three- to seven-fold lower than those against the Wuhan strain. By contrast, IC50 titers against variants remained stable throughout follow-up (Figure 2B).
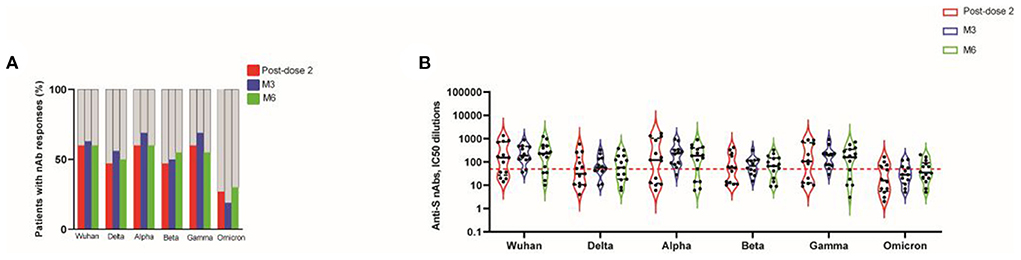
Figure 2. Neutralizing activity against spike protein mutations associated with VOCs, 14 days after the second dose (post-dose 2), and at M3 and M6 post-vaccination, in transplanted patients. Frequency of patients with nAb responses directed against the original strain and the various VOCs. A negative result (gray bars) indicates an IC50 titers < 50 dilutions; a positive result (colored bars) indicates an IC50 titers > 50 dilutions (A). Neutralizing antibody responses were assessed by determining the half maximal inhibitory concentration (IC50) dilutions. The dotted red line indicates the threshold for assay positivity (i.e., IC50 > 50 dilutions). Data are expressed as IC50 (half maximal inhibitory concentration) dilutions (B).
We then evaluated vaccine-induced T-cell responses against the Spike (S) protein, using overlapping peptide pools (OLP) spanning the S1 and S2 regions from S present in the mRNA vaccine, in KTR from the CNI (n = 24) and belatacept (n = 17) groups. After two or three doses of vaccine, the median frequencies [IQR] of S-specific CD4 T cells (IFN-γ ± IL-2 ± TNF) in CNI-treated patients were 0.15% [0.04–0.3]; 0.13% [0.04–0.21] and 0.16% [0.07–0.8], at 15 days post-dose 2, M3 and M6, respectively (P = 0.009; P = 0.04, and P = 0.0007 vs. the pre-vaccination visit). A longitudinal analysis of long-term follow-up visits (M3 and M6) showed no decrease in the frequency of S-specific T-cell responses relative to the post-dose 2 time point. Several patients received boosters (third dose) during this period, which may account for the maintenance of the cellular response. No increase in S-specific CD8 T cells was detected after vaccination with the BNT162b2 mRNA vaccine (Figure 3A). By contrast, no S-specific CD4 or CD8 T cells were detected in patients treated with belatacept, as shown by comparison with the pre-vaccination visit (Figure 3B). S-specific CD4 T-cell responses in the CNI group were polyfunctional, with the simultaneous production of up to three cytokines, and no major differences from post-dose 2 to M6 (Figure 3C). Thus, KTR treated with belatacept are less likely to be capable of mounting humoral and T-cell responses after the two initial injections or booster vaccination.
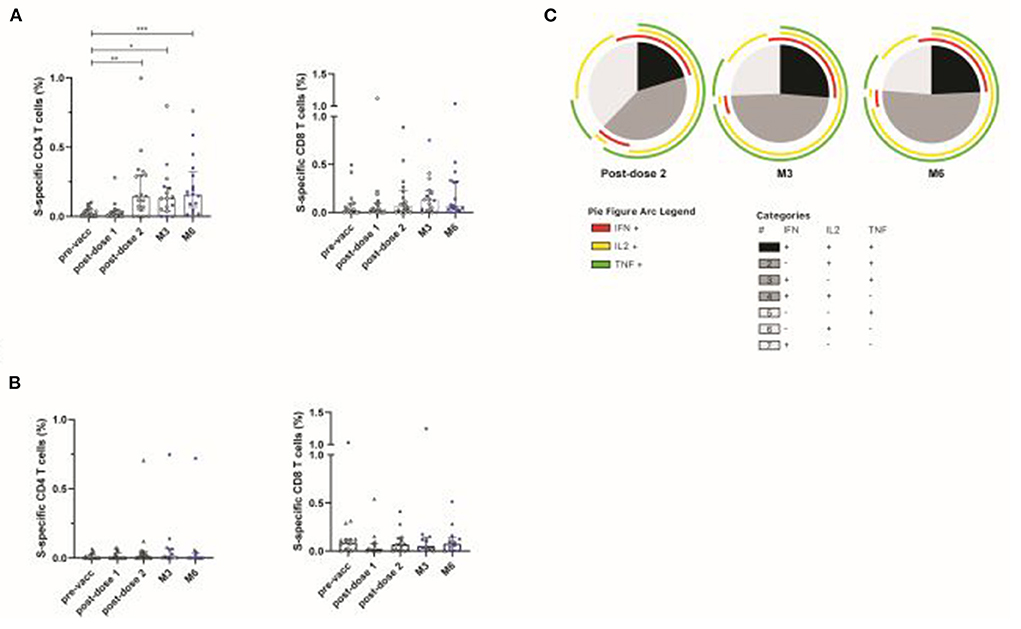
Figure 3. Spike-specific T-cell responses induced by vaccination with BNT162b2 in patients treated with calcineurin inhibitor (CNI) or belatacept. S-specific CD4 and CD8 T-cell responses in patients treated with CNI (n = 24) (A) or belatacept (n = 17) (B), after overnight stimulation with a pool of overlapping peptides covering the wild-type Spike protein at baseline (pre-vacc), 21 days after the first dose (post-dose 1), 14 days after the second dose (post-dose 2), and at M3 and M6 post-vaccination. Blue squares indicate patients who received a 3rd (booster) dose of vaccine at least 10 days before the follow-up visit. Functional composition of S-specific CD4 T-cell responses in vaccinated patients treated with CNI. Responses are color-coded according to the combination of cytokines produced. The arcs identify cytokine-producing subsets (IFN-γ, IL-2, and TNF) within the CD4 T-cell population (C). Kruskal-Wallis and Dunn's multiple comparison tests were used for statistical analysis (*P < 0.05, **P < 0.01, ***P < 0.001).
Globally, KTR remained highly susceptible to breakthrough infection. Fifteen (8 in the CNI group, corresponding to 24.2%; and 7 in the belatacept group, corresponding to 33.3%) of the 52 patients developed symptomatic COVID-19 during follow-up. One fatal case occurred a few days after vaccination in the CNI group.
Discussion
Since the beginning of the SARS-CoV-2 pandemic, immunocompromised individuals have remained a fragile population highly susceptible to severe disease and with a weak response to current vaccines or following natural infection (27). The humoral immune response (either nAb or non-nAbs) following natural infection or vaccination has been shown to be protective (28–31). In immunocompetent individuals, vaccine-elicited anti-S antibodies are detected in up to 95% of those vaccinated with mRNA vaccines (32). Antibody detection rates after vaccination are much lower in solid organ transplant (SOT) patients on long-term maintenance immunosuppressive therapy (3, 6). This study significantly extends these previous findings, by showing that both humoral and cellular responses are weaker in KTR, even after booster vaccination. Humoral responses in our group of kidney transplant patients were weaker than those measured with the same assays in a historical cohort of healthy volunteers (median: 1,900.4 U/ml; 95% CI, 1,816.1–2,119.8) (6). We also show that belatacept, a checkpoint inhibitor of immune cell activation, almost completely abolishes the ability of patients to mount a vaccine response. This observation has significant clinical implications, because these patients should undergo a careful evaluation of their vaccine responses and should benefit from prophylactic measures. Overall, our results are consistent with previous studies showing a weak response to SARS-CoV-2 vaccination in solid organ transplant patients (3, 16). However, we also demonstrate that belatacept-treated patients have much lower response rates than patients treated with CNI, indicating a strong dependence of the vaccine responses of these patients on the mechanism of action of their immunosuppressive regimens (18, 19). These results are consistent with those of the BENEFIT and BENEFIT-Ext studies, showing that patients treated with belatacept are significantly less likely to develop de novo anti-donor anti-HLA antibodies than patients on a CNI-based regimen (20). Thus, belatacept is more effective at preventing de novo B-cell activation than CNI, even though CNI strongly inhibit T-cell activation and the cytokine production (IL-2) required for B-cell activation (33–35).
T cells are also crucial for viral clearance (36). SARS-CoV-2 virus clearance has been reported in patients with Bruton agammaglobulinemia (37). Specific T-cell functionality increased after booster vaccination in CNI-treated patients but not in belatacept-treated patients, suggesting that belatacept-treated patients are at risk of severe COVID or long-term infection due to a lack of both T- and B-cell responses. This is in agreement with the observations of Zhang et al. who found an impaired CD4 T cell response in tacrolimus or belatacept treated patients (38). In addition, the development of a specific high-affinity immune response requires the coordination of T and B cells, CD4 follicular T cells (Tfhs) being essential for the maturation and differentiation of B cells for the synthesis of high-affinity antibodies (36). We show here that T-cell responses are not homogeneous and can be severely impaired in SOT patients, as reported for immunocompromised patients (6, 16). On the contrary to Zhang's study, this effect was more marked for belatacept-treated patients, consistent with previous studies showing that belatacept impairs the naïve T-cell response and B-cell help, with a lesser effect on memory T cells (21, 38–41). Thus, belatacept, which inhibits the CD28 pathway in addition to its direct effect on B-cell maturation, inducing the accumulation of transitional B cells (42), also impairs T-cell activation after vaccination. As a result, anti-S IgG responses were almost undetectable in most patients treated with belatacept. We also show, despite their ability to inhibit T-cell activation and IL-2 production, CNI have a much milder effect on humoral responses. In CNI-treated patients, the humoral response may be preserved to some extent through extragerminal center cross-reacting B lymphocytes, as recently reported (43). These cross-reacting B cells, and the impairment of CD4+ T-cell help may contribute to the steeper slope of the antibody level curve in these patients (6, 43).
Clinically, these results highlight the need to check the biological immune response to vaccination in immunocompromised patients, particularly those treated with belatacept, which almost completely abolishes humoral responses in most patients. No benefit of an additional booster injection would be expected in patients on belatacept, whereas an improvement of humoral responses was observed in CNI-treated patients. However, all these patients remain at high risk of infection, because vaccine responders display significantly lower levels of neutralizing antibody activity against VOC than the general population, with lower levels of activity against the Omicron variant (6) than for other variants, as reported in the general population. Thus, for immunocompromised patients with low titers of anti-S antibodies, additional prophylactic or post-exposure strategies may be required in cases of infection.
In accordance with national recommendations, the patients from our belatacept group were offered treatment with anti-SARS-CoV-2 monoclonal antibodies (n = 9) for the prevention (n = 8) or treatment (n = 1) of infection. One patient also received high-titer convalescent plasma. Of note, these treatments were administered after our study. However, unsurprisingly, given the epidemiology of the emergence of more infectious variants, a number of breakthrough infections (BI) were observed in our cohort. Mortality rates were high during the first and second waves of the pandemic, but only two deaths occurred in this cohort. One death, related to BI, occurred shortly after the first dose of vaccine was administered, suggesting that the patient did not develop an effective immune response.
One of the limitations of this study is the small number of patients that we were able to include, due to the need to vaccinate as many patients as possible, as rapidly as possible, and frequently close to their site of residence, outside of hospital structures. This also affected the availability of samples for exhaustive T- and B-cell assessments.
Overall, our results confirm the weak antibody and cellular responses of transplant recipients vaccinated with BNT162b2, particularly those treated with belatacept. New vaccination strategies are required for these patients, possibly including immunosuppression conversion under close monitoring for a limited period around vaccination, given the risk of allogeneic immunization, to facilitate the development of a vaccine response.
Data availability statement
The original contributions presented in the study are included in the article/supplementary material, further inquiries can be directed to the corresponding author.
Ethics statement
The studies involving human participants were reviewed and approved by Ethics Committee (No. IDRCB: 2018-A01610-55). The patients/participants provided their written informed consent to participate in this study.
Author contributions
YL, AD, and AW conceived and designed the study. AW, CP, CL, GP, AD, and YL analyzed and interpreted the data. LG, MDé, CK, and CP performed the experiments. AD, MDe, and PG participated in sample and clinical data collection. AW, CP, AD, and YL drafted the first version and wrote the final version of the manuscript. All authors approved the final version.
Funding
This work was supported by INSERM and the Investissements d'Avenir program, and the Vaccine Research Institute (VRI), managed by the ANR under reference ANR-10-LABX-77-01 and by the European Union's Horizon Europe Research and Innovation program under Grant Agreement No. 101046041.
Acknowledgments
We thank the patients for the donation of blood samples and the nurses and physicians of Henri Mondor Hospital for managing patients during the SARS-CoV-2 pandemic.
Conflict of interest
The authors declare that the research was conducted in the absence of any commercial or financial relationships that could be construed as a potential conflict of interest.
Publisher's note
All claims expressed in this article are solely those of the authors and do not necessarily represent those of their affiliated organizations, or those of the publisher, the editors and the reviewers. Any product that may be evaluated in this article, or claim that may be made by its manufacturer, is not guaranteed or endorsed by the publisher.
Author disclaimer
The views and opinions expressed are those of the authors only, and do not necessarily reflect those of the European Union or the European Commission.
References
1. Thaunat O, Legeai C, Anglicheau D, Couzi L, Blancho G, Hazzan M, et al. IMPact of the COVID-19 epidemic on the moRTAlity of kidney transplant recipients and candidates in a French Nationwide registry sTudy (IMPORTANT). Kidney Int. (2020) 98:1568–77. doi: 10.1016/j.kint.2020.10.008
2. Caillard S, Chavarot N, Francois H, Matignon M, Greze C, Kamar N, et al. Is Covid-19 infection more severe in kidney transplant recipients? Am J Transplant. (2020) 21:1295–303. doi: 10.1111/ajt.16424
3. Benotmane I, Gautier-Vargas G, Cognard N, Olagne J, Heibel F, Braun-Parvez L, et al. Low immunization rates among kidney transplant recipients who received 2 doses of the mRNA-1273 SARS-CoV-2 vaccine. Kidney Int. (2021) 99:1498–500. doi: 10.1016/j.kint.2021.04.005
4. Kamar N, Abravanel F, Marion O, Esposito L, Hebral AL, Médrano C, et al. Anti-SARS-CoV-2 spike protein and neutralizing antibodies at one and 3 months after 3 doses of SARS-CoV-2 vaccine in a large cohort of solid-organ-transplant patients. Am J Transplant. (2022) 22:1467–74. doi: 10.1111/ajt.16950
5. Kamar N, Abravanel F, Marion O, Couat C, Izopet J, Del Bello A. Three doses of an mRNA Covid-19 vaccine in solid-organ transplant recipients. N Engl J Med. (2021) 385:661–2. doi: 10.1056/NEJMc2108861
6. Obeid M, Suffiotti M, Pellaton C, Bouchaab H, Cairoli A, Salvadé V, et al. Humoral responses against variants of concern by COVID-19 mRNA vaccines in immunocompromised patients. JAMA Oncol. (2022) 8:e220446. doi: 10.1001/jamaoncol.2022.0446
7. Lee ARYB, Wong SY, Chai LYA, Lee SC, Lee MX, Muthiah MD, et al. Efficacy of covid-19 vaccines in immunocompromised patients: systematic review and meta-analysis. BMJ. (2022) 376:e068632. doi: 10.1136/bmj-2021-068632
8. Le Corre N, Thibault F, Noble CP, Meiffrédy V, Daoud S, Cahen R, et al. Effect of two injections of non-adjuvanted influenza A H1N1pdm2009 vaccine in renal transplant recipients: INSERM C09-32 TRANSFLUVAC trial. Vaccine. (2012) 30:7522–8. doi: 10.1016/j.vaccine.2012.10.047
9. Beaudreuil S, Krivine A, Hebibi H, Ducot B, Mazet AA, Taoufik Y, et al. Efficacy and safety of the H1N1 monovalent vaccine in renal-transplant recipients and dialysis patients. Human Vaccines. (2011) 7:868–73. doi: 10.4161/hv.7.8.16083
10. Crespo M, Collado S, Mir M, Cao H, Barbosa F, Serra C, et al. Efficacy of influenza A H1N1/2009 vaccine in hemodialysis and kidney transplant patients. Clin J Am Soc Nephrol. (2011) 6:2208–14. doi: 10.2215/CJN.02160311
11. Tenforde MW, Self WH, Gaglani M, Ginde AA, Douin DJ, Talbot HK, et al. Effectiveness of mRNA vaccination in preventing COVID-19-associated invasive mechanical ventilation and death - United States, March 2021-January 2022. MMWR Morb Mortal Wkly Rep. (2022) 71:459–65. doi: 10.15585/mmwr.mm7112e1
12. Levin EG, Lustig Y, Cohen C, Fluss R, Indenbaum V, Amit S, et al. Waning immune humoral response to BNT162b2 Covid-19 vaccine over 6 months. N Engl J Med. (2021) 385:e84. doi: 10.1056/NEJMoa2114583
13. Chemaitelly H, Tang P, Hasan MR, AlMukdad S, Yassine HM, Benslimane FM, et al. Waning of BNT162b2 vaccine protection against SARS-CoV-2 infection in Qatar. N Engl J Med. (2021) 385:e83. doi: 10.1056/NEJMoa2114114
14. Eliakim-Raz N, Massarweh A, Stemmer A, Stemmer SM. Durability of response to SARS-CoV-2 BNT162b2 vaccination in patients on active anticancer treatment. JAMA Oncol. (2021) 7:1716–8. doi: 10.1001/jamaoncol.2021.4390
15. Ashokkumar C, Rohan V, Kroemer AH, Rao S, Mazariegos G, Higgs BW, et al. Impaired T-cell and antibody immunity after COVID-19 infection in chronically immunosuppressed transplant recipients. bioRxiv. [Preprint]. (2021). doi: 10.1101/2021.05.03.442371
16. Chavarot N, Ouedrani AP, Marion O, Leruez-Ville M, Villain EB, Baaziz M, et al. Poor anti-SARS-CoV-2 humoral and T-cell responses after 2 injections of mRNA vaccine in kidney transplant recipients treated with belatacept. Transplantation. 105:e94–5. doi: 10.1097/TP.0000000000003784
17. Dolff S, Korth J, Jahn M, Kribben A, Witzke O, Wilde B. Anti-SARS-CoV-2 T-cell responses after mRNA vaccination in belatacept-treated renal transplant patients. Transplantation. (2021) 105:e99. doi: 10.1097/TP.0000000000003812
18. Chavarot N, Morel A, Leruez-Ville M, Vilain E, Divard G, Burger C, et al. Weak antibody response to three doses of mRNA vaccine in kidney transplant recipients treated with belatacept. Am J Transplant. (2021) 21:4043–51. doi: 10.1111/ajt.16814
19. Ou MT, Boyarsky BJ, Chiang TPY, Bae S, Werbel WA, Avery RK, et al. Immunogenicity and reactogenicity after SARS-CoV-2 mRNA vaccination in kidney transplant recipients taking belatacept. Transplantation. (2021) 105:2119–23. doi: 10.1097/TP.0000000000003824
20. Everly MJ, Roberts M, Townsend R, Bray RA, Gebel HM. Comparison of de novo IgM and IgG anti-HLA DSAs between belatacept- and calcineurin-treated patients: an analysis of the BENEFIT and BENEFIT-EXT trial cohorts. Am J Transplant. (2018) 18:2305–13. doi: 10.1111/ajt.14939
21. Espinosa J, Herr F, Tharp G, Bosinger S, Song M, Farris AB, et al. CD57(+) CD4 T Cells underlie belatacept-resistant allograft rejection. Am J Transplant. (2016) 16:1102–12. doi: 10.1111/ajt.13613
22. Bray RA, Gebel HM, Townsend R, Roberts ME, Polinsky M, Yang L, et al. De novo donor-specific antibodies in belatacept-treated vs cyclosporine-treated kidney-transplant recipients: post hoc analyses of the randomized phase III BENEFIT and BENEFIT-EXT studies. Am J Transplant. (2018) 18:1783–9. doi: 10.1111/ajt.14721
23. Durrbach A, Pestana JM, Florman S, Del Carmen Rial M, Rostaing L, Kuypers D, et al. Long-term outcomes in belatacept- versus cyclosporine-treated recipients of extended criteria donor kidneys: final results from BENEFIT-EXT, a phase III randomized study. Am J Transplant. (2016) 16:3192–201. doi: 10.1111/ajt.13830
24. Vincenti F, Rostaing L, Grinyo J, Rice K, Steinberg S, Gaite L, et al. Belatacept and long-term outcomes in kidney transplantation. N Engl J Med. (2016) 374:333–43. doi: 10.1056/NEJMoa1506027
25. Fenwick C, Croxatto A, Coste AT, Pojer F, André C, Pellaton C, et al. Changes in SARS-CoV-2 spike versus nucleoprotein antibody responses impact the estimates of infections in population-based seroprevalence studies. J Virol. (2021) 95:e01828–20. doi: 10.1128/JVI.01828-20
26. Fenwick C, Turelli P, Pellaton C, Farina A, Campos J, Raclot C, et al. A high-throughput cell- and virus-free assay shows reduced neutralization of SARS-CoV-2 variants by COVID-19 convalescent plasma. Sci Trans Med. (2021) 13:eabi8452. doi: 10.1126/scitranslmed.abi8452
27. Zervou FN, Ali NM, Neumann HJ, Madan RP, Mehta SA. SARS-CoV-2 antibody responses in solid organ transplant recipients. Transpl Infect Dis. (2021) 23:e13728. doi: 10.1111/tid.13728
28. Du L, He Y, Zhou Y, Liu S, Zheng BJ, Jiang S. The spike protein of SARS-CoV–a target for vaccine and therapeutic development. Nat Rev Microbiol. (2009) 7:226–36. doi: 10.1038/nrmicro2090
29. Xie Y, Karki CB, Du D, Li H, Wang J, Sobitan A, et al. Spike proteins of SARS-CoV and SARS-CoV-2 utilize different mechanisms to bind with human ACE2. Front Mol Biosci. (2020) 7:591873. doi: 10.3389/fmolb.2020.591873
30. Cao Y, Su B, Guo X, Sun W, Deng Y, Bao L, et al. Potent neutralizing antibodies against SARS-CoV-2 identified by high-throughput single-cell sequencing of convalescent patients' B cells. Cell. (2020) 182:73–84.e16. doi: 10.1016/j.cell.2020.05.025
31. Ni L, Ye F, Cheng ML, Feng Y, Deng YQ, Zhao H, et al. Detection of SARS-CoV-2-specific humoral and cellular immunity in COVID-19 convalescent individuals. Immunity. (2020) 52:971–7.e3. doi: 10.1016/j.immuni.2020.04.023
32. Mulligan MJ, Lyke KE, Kitchin N, Absalon J, Gurtman A, Lockhart S, et al. Phase I/II study of COVID-19 RNA vaccine BNT162b1 in adults. Nature. (2020) 586:589–93. doi: 10.1038/s41586-020-2639-4
33. Larsen CP, Elwood ET, Alexander DZ, Ritchie SC, Hendrix R, Tucker-Burden C, et al. Long-term acceptance of skin and cardiac allografts after blocking CD40 and CD28 pathways. Nature. (1996) 381:434–8. doi: 10.1038/381434a0
34. Larsen CP, Pearson TC, Adams AB, Tso P, Shirasugi N, Strobertm E, et al. Rational development of LEA29Y (belatacept), a high-affinity variant of CTLA4-Ig with potent immunosuppressive properties. Am J Transplant. (2005) 5:443–53. doi: 10.1111/j.1600-6143.2005.00749.x
35. Larsen CP, Ritchie SC, Hendrix R, Linsley PS, Hathcock KS, Hodes RJ, et al. Regulation of immunostimulatory function and costimulatory molecule (B7-1 and B7-2) expression on murine dendritic cells. J Immunol. (1994) 152:5208–19.
36. de Goer de Herve MG, Abdoh M, Jaafoura S, Durali D, Taoufik Y. Follicular CD4 T Cells tutor CD8 early memory precursors: an initiatory journey to the frontier of B cell territory. iScience. (2019) 20:100–9. doi: 10.1016/j.isci.2019.09.012
37. Soresina A, Moratto D, Chiarini M, Paolillo C, Baresi G, Focà E, et al. Two X-linked agammaglobulinemia patients develop pneumonia as COVID-19 manifestation but recover. Pediatr Allergy Immunol. (2020) 31:565–9. doi: 10.1111/pai.13263
38. Zhang R, Shin B, Gadsden TM, Petrosyan A, Vo A, Ammerman N, et al. Assessment of humoral and cellular immune responses to SARS CoV-2 vaccination (BNT162b2) in immunocompromised renal allograft recipients. Transpl Infect Dis. (2022) 24:e13813. doi: 10.1111/tid.13813
39. Herr F, Desterke C, Bargiel K, Vernochet A, Vanhove B, Vadanici R, et al. The proliferation of belatacept-resistant T cells requires early IFNα pathway activation. Am J Transplant. (2022) 22:489–503. doi: 10.1111/ajt.16811
40. Mathews DV, Dong Y, Higginbotham LB, Kim SC, Breeden CP, Stobert EA, et al. CD122 signaling in CD8+ memory T cells drives costimulation-independent rejection. J Clin Invest. (2018) 128:4557–72. doi: 10.1172/JCI95914
41. Adams AB, Shirasugi N, Durham MM, Strobert E, Anderson D, Rees P, et al. Calcineurin inhibitor-free CD28 blockade-based protocol protects allogeneic islets in nonhuman primates. Diabetes. (2002) 51:265–70. doi: 10.2337/diabetes.51.2.265
42. Leibler C, Thiolat A, Hénique C, Samson C, Pilon C, Tamagne M, et al. Control of humoral response in renal transplantation by belatacept depends on a direct effect on B cells and impaired T follicular helper-B cell crosstalk. J Am Soc Nephrol. (2018) 29:1049–62. doi: 10.1681/ASN.2017060679
Keywords: COVID-19, immunocompromised, immunosuppressive regimen, mRNA vaccine, immune responses
Citation: Wiedemann A, Pellaton C, Dekeyser M, Guillaumat L, Déchenaud M, Krief C, Lacabaratz C, Grimbert P, Pantaleo G, Lévy Y and Durrbach A (2022) Longitudinal evaluation of the impact of immunosuppressive regimen on immune responses to COVID-19 vaccination in kidney transplant recipients. Front. Med. 9:978764. doi: 10.3389/fmed.2022.978764
Received: 04 July 2022; Accepted: 03 August 2022;
Published: 22 August 2022.
Edited by:
Nianqiao Gong, Huazhong University of Science and Technology, ChinaReviewed by:
Gaurav Gupta, Virginia Commonwealth University, United StatesPetra Hruba, Institute for Clinical and Experimental Medicine (IKEM), Czechia
Copyright © 2022 Wiedemann, Pellaton, Dekeyser, Guillaumat, Déchenaud, Krief, Lacabaratz, Grimbert, Pantaleo, Lévy and Durrbach. This is an open-access article distributed under the terms of the Creative Commons Attribution License (CC BY). The use, distribution or reproduction in other forums is permitted, provided the original author(s) and the copyright owner(s) are credited and that the original publication in this journal is cited, in accordance with accepted academic practice. No use, distribution or reproduction is permitted which does not comply with these terms.
*Correspondence: Antoine Durrbach, a.durrbach@gmail.com
†These authors have contributed equally to this work