Lung function benefits of traditional Chinese medicine Qiju granules against fine particulate air pollution exposure: a randomized controlled trial
- 1School of Public Health, Zhejiang Chinese Medical University, Hangzhou, China
- 2Zhejiang International Science and Technology Cooperation Base of Air Pollution and Health, Hangzhou, China
- 3First Affiliated Hospital of Zhejiang Chinese Medical University, Hangzhou, China
Introduction: Multiple targets are considered as the causes of ambient fine particulate matter [aerodynamic diameters of < 2.5 μm (PM2.5)] induced lung function injury. Qiju granules are derived from the traditional Chinese medicine (TCM) formula known as Qi-Ju-Di-Huang-Wan (Lycium, Chrysanthemum, and Rehmannia Formula, QJDHW), which has been traditionally used to treat symptoms such as cough with phlegm, dry mouth and throat, and liver heat. This treatment approach involves attenuating inflammation, oxidative stress, and fibrosis response. This study investigated the effects of Qiju granules on protecting lung function against PM2.5 exposure in a clinical trial.
Methods: A randomized, double-blinded, and placebo-controlled trial was performed among 47 healthy college students in Hangzhou, Zhejiang Province in China. The participants were randomly assigned to the Qiju granules group or the control group based on gender. Clinical follow-ups were conducted once every 2 weeks during a total of 4 weeks of intervention. Real-time monitoring of PM2.5 concentrations in the individually exposed participants was carried out. Data on individual characteristics, heart rate (HR), blood pressure (BP), and lung function at baseline and during the follow-ups were collected. The effects of PM2.5 exposure on lung function were assessed within each group using linear mixed-effect models.
Results: In total, 40 eligible participants completed the scheduled follow-ups. The average PM2.5 level was found to be 64.72 μg/m3 during the study period. A significant negative correlation of lung function with PM2.5 exposure concentrations was observed, and a 1-week lag effect was observed. Forced expiratory volume in one second (FEV1), peak expiratory flow (PEF), maximal mid-expiratory flow (MMEF), forced expiratory flow at 75% of forced vital capacity (FVC) (FEF75), forced expiratory flow at 50% of FVC (FEF50), and forced expiratory flow at 25% of FVC (FEF25) were significantly decreased due to PM2.5 exposure in the control group. Small airway function was impaired more seriously than large airway function when PM2.5 exposure concentrations were increased. In the Qiju granules group, the associations between lung function and PM2.5 exposure were much weaker, and no statistical significance was observed.
Conclusion: The results of the study showed that PM2.5 exposure was associated with reduced lung function. Qiju granules could potentially be effective in protecting lung functions from the adverse effects of PM2.5 exposure.
Clinical Trial Registration: identifier: ChiCTR1900021235.
1 Introduction
Ambient air pollution is an important global public health challenge. Epidemiological studies have demonstrated that exposure to particulate matter (PM2.5) increases mortality and morbidity in respiratory diseases (1, 2). The global burden of disease study estimated that 1.4 million deaths were due to respiratory diseases caused by ambient particulate matter alone (3), with PM2.5 identified as the most common detrimental factor among ambient particulate matter pollutants (3). The most effective way to minimize health hazards is by improving air quality by reducing pollutant release. Although more countries have been taking increased actions, over 90% of the people worldwide continue to breathe polluted air (4). Thus, personalized behavioral and preventive interventions may offer an alternative way to mitigate health hazards due to air pollution.
Previous studies have proved that both personal protective equipment and dietary supplementation could mitigate the adverse effects of PM2.5 air pollution (5–7). Health benefits have been demonstrated by using air purifiers and particulate-filtering respirators (5, 7). However, on many occasions, these interventions are not portable or readily available (e.g., outdoors and social interactions). Omega-3 fatty acid supplementation has been shown to alleviate some adverse effects in cardiovascular, metabolic, and neurological systems from air pollution (6). Given the role of the lung as the entrance of PM2.5 inhalational exposure, attenuating respiratory damage from air pollution should help prevent subsequential systemic injuries in our bodies. The exact pathophysiological mechanisms of lung injury are yet to be fully understood. Multiple targets including airway inflammation, oxidative stress, and pulmonary fibrosis are regarded as potential mechanisms that are involved in PM2.5-induced lung dysfunction (8). Therefore, it is reasonable to speculate that multi-target intervention of these biological pathways may offer more effective and preventive benefits against pulmonary injury from PM2.5 air pollution.
The traditional Chinese medicine (TCM) formula known as Qi-Ju-Di-Huang-Wan (Lycium, Chrysanthemum, and Rehmannia Formula, QJDHW) originates from “Yi Ji Juan Ba” of Dong Xiyuan of the Qing Dynasty (9). Qiju granules are derived from QJDHW, which has been traditionally used to treat symptoms such as cough with phlegm, dry mouth and throat, and liver heat (10, 11). In TCM's Five Phases Theory, Qiju granules are considered to have the properties of nourishing Yin and clearing heat with wood and fire attributes. Qiju granules contain five edible herbs, including Rhodiola rosea L. (Crassulaceae), Portulaca oleracea L. (Portulacaceae), Lycium barbarum L. (Solanaceae), Chrysanthemum × morifolium (Ramat.) Hemsl. (Asteraceae), and Siraitia grosvenorii (Swingle) C.Jeffrey ex A.M.Lu & Zhi Y.Zhang (Cucurbitaceae). These herbs are often used as a part of the regimen to treat lung disorders, which show attenuation in inflammation, oxidative stress, and fibrosis response (12–16). Our preliminary study has demonstrated the potential lung benefits of using Qiju granules against exposure to PM2.5 air pollution in an animal model (17). An oral administration of Qiju decoction decreases the concentration of tumor necrosis factor-α (TNF-α) and malondialdehyde (MDA), improving the expression of glutathione-peroxidase (GSH-PX) in serum (17). Qiju granules may act as a better alternative to attenuate lung injury from PM2.5. However, it remains unknown whether Qiju granules have protective effects against PM2.5-induced lung damage in humans.
In order to verify the effects of Qiju granules on humans, we conducted a randomized, double-blinded, and placebo-controlled trial to assess whether Qiju granules offered lung benefits against the adverse effects of PM2.5 exposure among healthy young adults.
2 Methods
2.1 Study participants and design
This trial was conducted from December 2018 to April 2019. We initially recruited 60 healthy college students at Zhejiang Chinese Medical University, Hangzhou, China, with the exclusion of students who are primary tobacco smokers or whose roommates are smokers, who are clinically diagnosed with cardiovascular or lung diseases, who had recent infection, who took antibiotics within the past month, or who had a history of allergies or family allergies. A total of 47 eligible participants were randomly assigned to two different treatment groups: the Qiju group and the placebo group. The randomization process was conducted using computer-generated random numbers. Gender was considered during randomization to ensure baseline comparability between the two groups. The random assignment procedure was carried out by independent researchers who were not involved in participant recruitment or diagnostic assessments, thus minimizing potential biases. All participants were intervened and monitored in a double-blinded manner throughout the study period. Two rounds of follow-up, with an interval of 2 weeks, were scheduled during a total intervention of 4 weeks. Data on individual characteristics (i.e., age, sex, height, and weight) and vital signs (i.e., heart rate (HR), blood pressure (BP), and respiration rate) were recorded, and lung function at the baseline and during the follow-ups was measured. Peripheral blood and the first-morning urine (midstream) samples were collected at the baseline and the end of the trial. Real-time PM2.5 exposure concentrations of those participants were also measured daily during the study time periods.
The study was registered in the Chinese Clinical Trial Registry (ChiCTR1900021235) on 13 February 2019 and was approved by the Ethics Committee of the First Affiliated Hospital of Zhejiang Chinese Medical University. All participants gave written informed consent at enrollment.
2.2 Ultra Performance Liquid Chromatography-Quadrupole-Time of Flight/Mass Spectrometry analysis of Qiju granules
The Ultra Performance Liquid Chromatography-Quadrupole-Time of Flight/Mass Spectrometry (UPLC-Q-TOF/MS) instrument was set to a capillary voltage of 2.5 kV (negative ion mode), sample cone voltage of 40 V, source temperature of 120°C, desolvation temperature of 500°C, and desolvation gas flow of 1000 L/h. Collision energy was set at 6 eV, and the full scan spectra were recorded from 50 to 1200 Da. Qiju granules were dissolved in purified water at a concentration of 100 mg/ml, and the solution was centrifuged to obtain the supernatant. The supernatant was analyzed by UPLC using reversed-phase C18 analytical columns (2.1 × 100 mm) packed with silica beads of 1.6 μm diameter (Cortecs® UPLC® T3, Waters, SYNAPT G2-Si, USA). The mobile phase consisted of 10% of solvent A (0.1% of formic acid) and 90% of solvent B (acetonitrile). The elution of Qiju granules was achieved over a period of 35 min with an injection volume of 2 μl and flow rates of 0.3 ml/min. The gradient elution program was set as follows: 0–2 min, 5% B; 2–32 min, 5–100% B; 32–33 min, 100% B; and 33–35 min, 5% B. The MS spectra of Qiju granules were acquired in negative-ion mode (Supplementary Figure S1).
2.3 Interventions
Qiju granules and placebo granules were provided by Huadong Medicine Co., LTD (Hangzhou, Zhejiang Province, China). Qiju granules were extracted and purified from Rhodiola rosea L. [Crassulaceae], Portulaca oleracea L. [Portulacaceae], Lycium barbarum L. [Solanaceae], Chrysanthemum × morifolium (Ramat.) Hemsl. [Asteraceae], and Siraitia grosvenorii (Swingle) C.Jeffrey ex A.M.Lu & Zhi Y.Zhang [Cucurbitaceae]. Placebo granules were purified from dextrin and caramel, with their appearance being identical and taste being similar to Qiju granules. The granules were stored at room temperature. Participants in the Qiju and control groups were instructed to dissolve 1 package of granules (15 g) in warm drinking water and take them orally every morning throughout the study period. The participants and researchers were blinded to either intervention.
2.4 Exposure assessment
To obtain real-time measurements of ambient PM2.5 concentrations, a portable environmental dust monitor (AirCasting Air Monitor Casing, New York Hall of Science, NY, USA) was provided to every two or three participants with similar activities who lived in the same dorm room. Enrolled participants were asked to wear the portable monitors from 8 am to 8 pm. The portable monitor was able to record ambient PM2.5 concentrations every second, and the data were collected to calculate daily average PM2.5 concentrations. To ensure the accuracy of the monitor, each portable monitor was calibrated through an aerosol monitoring meter (pDR-1500, Thermo Scientific, China) beforehand, and PM2.5 concentrations were calculated using the following formula:
Y represents the concentrations of the portable monitor; X represents ambient PM2.5 concentrations; Bmax represents the maximum specific binding in the same units as Y; and Kd represents the equilibrium dissociation constant, in the same units as X. The fitting degree of R2 was found to be between 0.90 and 0.98 in this formula (Supplementary Table S1, Supplementary Figure S2).
2.5 Health examination
At each round of health examinations, we measured the key characteristics of the participants, including height, body weight, blood pressure (BP), heart rate (HR), and lung function between 8:00 and 9:00 AM to minimize potential influences from circadian rhythms. Height and weight were measured by electronic scale without shoes and heavy coats, and then body mass index (BMI) was calculated. After the participant sat for at least 5 min, BP and HR were measured by an electronic sphygmomanometer (Omron J710, Japan). BP was measured using the left upper arm for at least three times at 1-min intervals. The mean values of the second and third HR, systolic BP (SBP), and diastolic BP (DBP) measurements were obtained. If the differences between the second and third readings of BP were >5 mmHg, additional measurements were obtained until satisfaction was achieved. Fasting peripheral blood and the first-morning midstream urine samples were collected between 8:00 and 9:00 AM. Routine blood and urine tests and hepatic and renal function examinations were conducted at the beginning and the end of the trial. A lung function detector (HI-101, Chestgraph, Tokyo, Japan) was utilized to measure the major parameters of lung function, including forced vital capacity (FVC), forced expiratory volume in one second (FEV1), peak expiratory flow (PEF), maximal mid-expiratory flow (MMEF), forced expiratory flow at 75% of FVC (FEF75), forced expiratory flow at 50% of FVC (FEF50), and forced expiratory flow at 25% of FVC (FEF25). Then, the ratio of the measured value to the predicted value was calculated as the lung function parameter.
2.6 Statistical analyses
PM2.5 concentrations and health data were associated with the time of lung function measured. Health data including lung function, BP, and HR were directly used as those were normally distributed. Linear mixed-effects models were applied to evaluate PM2.5 exposure concentrations in relation to each of the lung function parameters within each group. In the basic model, PM2.5 was included as a fixed-effect independent term, and a random-effect intercept was added for each subject to account for correlations among repeated measurements per person, assuming a compound symmetry covariance structure, which was further adjusted for sex and BMI. In order to explore the lag patterns regarding the impact of PM2.5 exposure on individual lung function, multiple averaging periods preceding the measurement of lung function were applied, i.e., lag 0 week (7 days before the examination day), lag 1 week (from 8 to 14 days before the examination day), and lag 0–1 week (14 days before the examination day). All linear mixed-effects models were conducted using the “lme4” package of R software version 3.3.1. In different group analyses, the difference in the lung function parameters and 95% CIs between the two groups were presented.
3 Results
3.1 Demographic characteristics
A total of 60 participants were screened, and 47 were enrolled and underwent randomization (24 were assigned to the Qiju group and 23 were assigned to the placebo group). Among those, 40 participants completed the scheduled follow-ups; of which 20 were in the Qiju group and 20 were in the control group (Figure 1). The participants in this study are aged between 19 and 22 years old, with 47.50% being male participants. There were no statistically significant differences in age or gender between the two groups. The other characteristics of these two groups were no significant differences at enrollment in the distributions of BMI, HR, SBP, DBP, respiratory rate (RPR), FVC, FEV1, PEF, MMEF, FEF75, FEF25, and FEF50 (Table 1). There was no statistically significant difference in biomarkers of the routine blood test, routine urine test, hepatic function, or renal function between these two groups at either baseline or at the end of the trial (Supplementary Table S2).
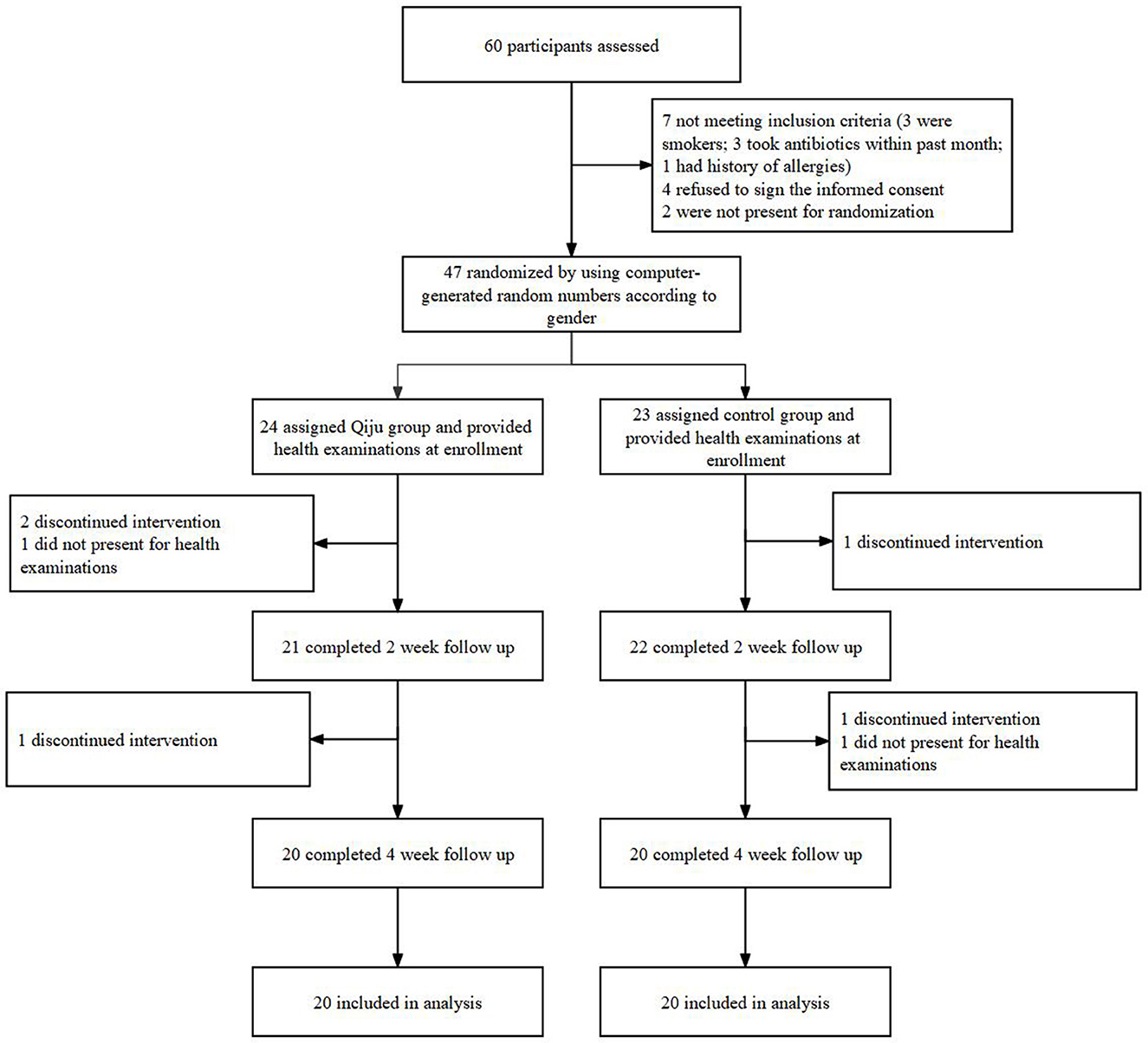
Figure 1. Flow diagram of enrollment and randomization. The intervention lasted for consecutive 4 weeks for both randomized arms. Of the 20 participants in the Qiju group who were included in the primary analysis, 3 participants discontinued intervention and 1 participant refused clinical follow-up. Of the 20 participants in the control group who were included in the primary analysis, 2 discontinued intervention and 1 refused clinical follow-up.
3.2 Monitoring of PM2.5 concentration
The average concentrations of individual PM2.5 exposure among participants were 64.72 ± 34.98 μg/m3 during the study period, which is slightly higher than that from the average level of Hangzhou city. At the individual level, daily and weekly averages of PM2.5 concentrations aligned well between the two groups during the study period (Figures 2A, B). The exposure of PM2.5 concentrations during different time periods before the conduction of the health examination was also calculated. The average concentrations at lag 0 week (7 days before the examination day), lag 1 week (from 8 to 14 days before the examination day), and lag 0–1 week (14 days before the examination day) were 57.58 ± 6.26 μg/m3, 80.12 ± 6.53 μg/m3, and 68.85 ± 4.63 μg/m3, respectively (Supplementary Table S3).
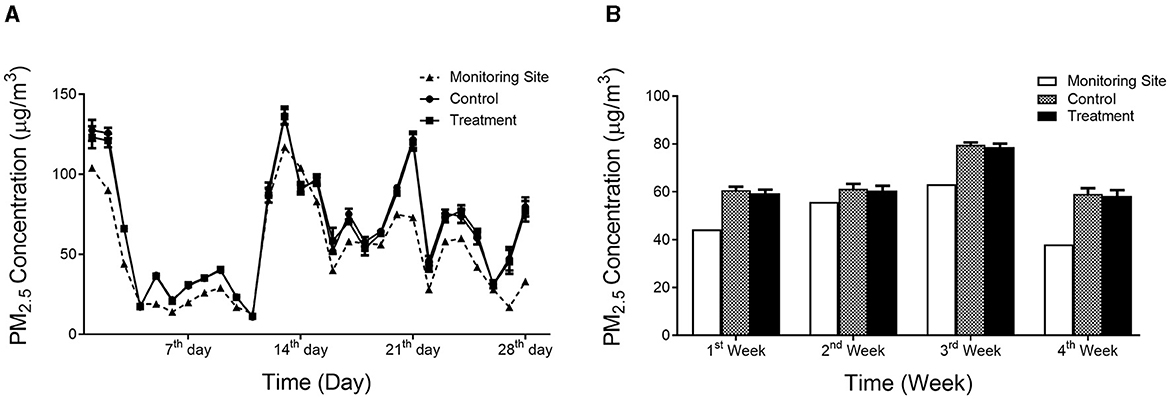
Figure 2. PM2.5 concentrations during the study period. (A) average daily PM2.5 concentrations; (B) average weekly PM2.5 concentrations. Values are expressed as mean ± SEM.
3.3 Correlation of lung function with PM2.5 concentrations
Lung function was primarily measured by FVC, FEV1, MMEF, PEF, FEF25, FEF50, and FEF75. The majority of these parameters were significantly negatively correlated with PM2.5 exposure concentrations at lag 1 week except FVC. None of these parameters was significantly correlated with PM2.5 exposure concentrations at lag 0 week. The majority of these parameters of lung function was not significantly correlated with PM2.5, and only PEF and PEF25 were significantly correlated with PM2.5 exposure concentrations at lag 0–1 week (Table 2).
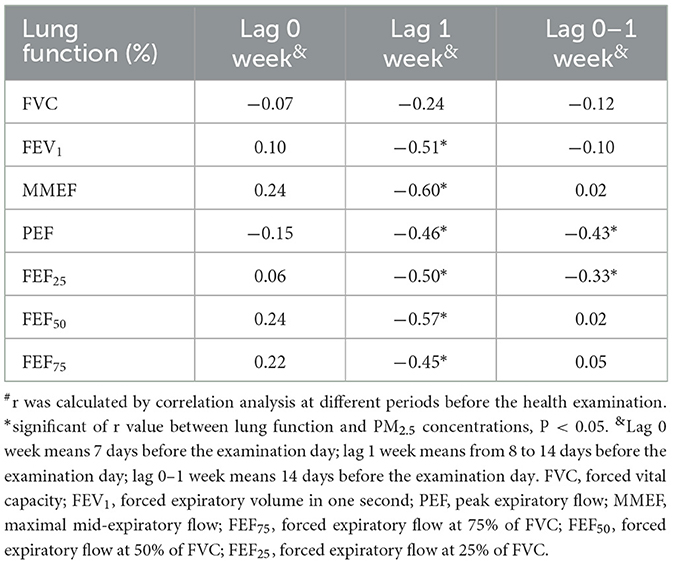
Table 2. Correlation between lung function and PM2.5 exposure concentrations at different periods before health examination#.
3.4 Percentage changes in lung function
Overall, the majority of the parameters related to lung function was significantly decreased in response to PM2.5 exposure concentration at lag 1 week in the control group. However, weaker associations with no statistical significance were observed in the Qiju group. Lung function did not vary significantly at lag 0 week and 0–1 week in response to PM2.5 exposures in either group (Figures 3, 4).
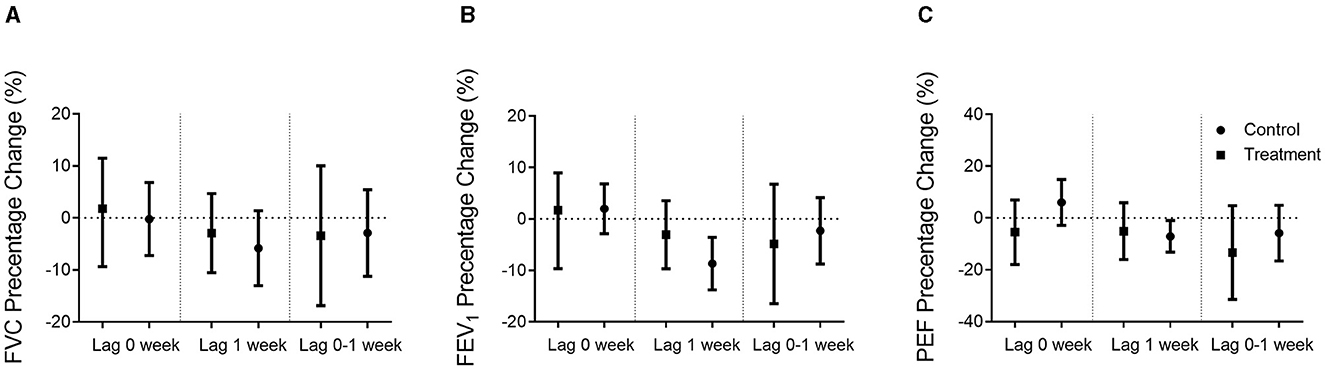
Figure 3. Percentage changes in airway function when adjusted sex and BMI. Percentage changes (mean and 95% confidence intervals) in airway function per 10 μg/m3 increase in PM2.5 exposure concentrations in the Qiju group and the control group at different lag times were shown. (A) FVC percentage of the predicted value change. (B) FEV1 percentage of the predicted value change. (C) PEF percentage of the predicted value change. Values are expressed as mean ± SEM. FVC, forced vital capacity; FEV1, forced expiratory volume in 1 s; PEF, peak expiratory flow.
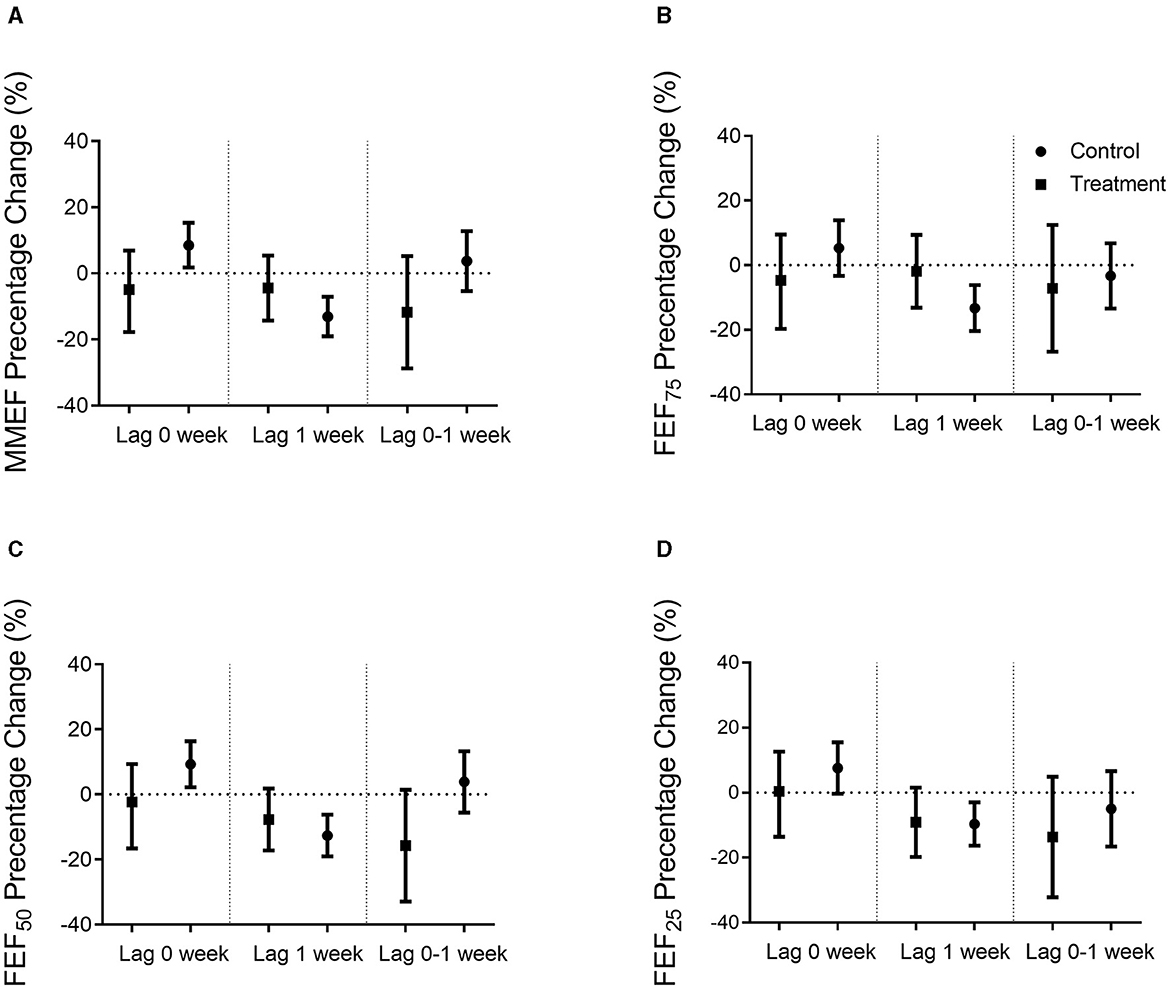
Figure 4. Percentage changes in small airway function when adjusted sex and BMI. Percentage changes (mean and 95% confidence intervals) in small airway function per 10 μg/m3 increase in PM2.5 exposure concentrations in the Qiju and the control groups at different lag times were shown. (A) MMEF percentage of the predicted value change. (B) FEF75 percentage of the predicted value change. (C) FEF50 percentage of the predicted value change. (D) FEF25 percentage of the predicted value change. Values are expressed as mean ± SEM. MMEF, maximal mid-expiratory flow; FEF75, forced expiratory flow at 75% of FVC; FEF50, forced expiratory flow at 50% of FVC; FEF25, forced expiratory flow at 25% of FVC.
3.5 Percentage changes in large airway function
As shown in Figure 3, FEV1 and PEF, which are the major parameters of large airway function, were negatively correlated with PM2.5 exposure concentrations in the control group at lag 1 week, but not statistically significant in the Qiju group. In the control group, there was an 8.80% (95% CI: 3.54% to 13.74%) decrease in the percentage of estimate FEV1 and a 7.07% (95% CI: 1.00% to 13.21%) decrease in the percentage of estimate PEF per 10 μg/m3 increase in PM2.5 at lag 1 week. However, the changes in FEV1 and FEF were not significant in the Qiju group with only 2.86% (95% CI: −3.45% to 9.77%) and 4.98% (95% CI: −5.82% to 13.21%) decrease in the percentage of estimate FEV1 and FEF, respectively, at lag 1 week. The association between FVC and PM2.5 exposure concentrations was not significant at each time point in both groups (Figure 3).
3.6 Percentage changes in small airway function
As shown in Figure 4, MMEF, FEF75, FEF50, and FEF25, which are the major parameters of small airway function, were negatively correlated with PM2.5 exposure concentrations in the control group at lag 1 week, but not statistically significant in the Qiju group. In the control group, there was 13.07% (95% CI: 7.12% to 19.03%) decrease in the percentage of estimate MMEF, 13.38% (95% CI: 6.12% to 20.30%) decrease in the percentage of estimate FEF75, 12.53% (95% CI: 6.31% to 19.08%) decrease in the percentage of estimate FEF50, and 9.67% (95% CI: 2.95% to 16.26%) decrease in the percentage of estimate FEF25 per 10 μg/m3 increase in PM2.5 at lag 1 week. However, the changes in these parameters were not significant in the Qiju group with only 3.71% (95% CI: −4.98% to 14.63%), 4.98% (95% CI: −9.06% to 13.47%), 6.81% (95% CI: −1.36% to 17.68%), and 8.84% (95% CI: −1.46% to 19.92%) decrease in the percentage of estimate MMEF, FEF75, FEF50, and FEF25, respectively, at lag 1 week (Figure 4).
3.7 The difference in airway function between groups when PM2.5 was adjusted
As shown in Figure 5, no parameters of lung function were statistically significant between the Qiju group and the control group at baseline. The difference in lung function parameters between these two groups became greater, and the trend to provide lung health benefits tended to increase in the Qiju group in the 2nd and 4th weeks. However, there were no statistical differences in all time periods (Figure 5).
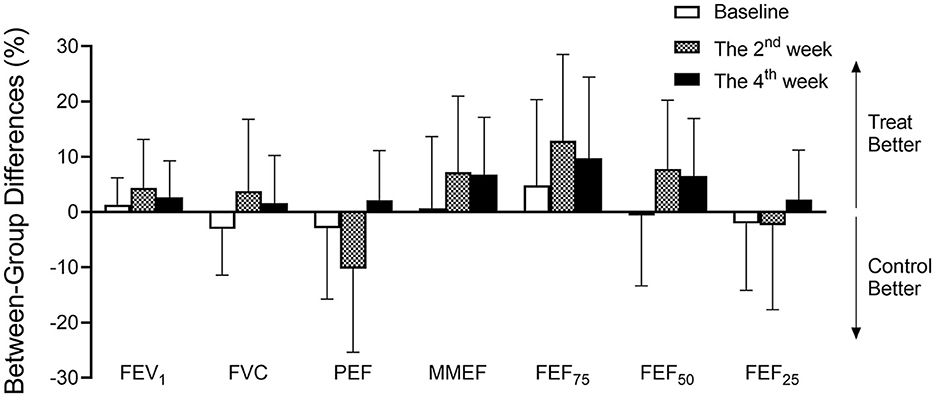
Figure 5. The difference in airway function between groups when adjusted PM2.5 concentrations. Different percentages (mean and 95% confidence intervals) in airway function between the Qiju group and the control group in different intervention times (baseline, the 2nd week, and the 4th week). PM2.5 exposure concentrations were adjusted in the 2nd and 4th weeks. Values are expressed as mean ± SEM. FVC, forced vital capacity; FEV1, forced expiratory volume in 1 s; PEF, peak expiratory flow; MMEF, maximal mid-expiratory flow; FEF75, forced expiratory flow at 75% of FVC; FEF50, forced expiratory flow at 50% of FVC; FEF25, forced expiratory flow at 25% of FVC.
4 Discussion
In this randomized trial among healthy young adults in Hangzhou, China, we examine lung function benefits of Qiju granules against PM2.5 exposure at different time periods occurring at lag intervals of 0, 1, or 0–1 week before health examination, which include large airways function (FVC, FEV1, and PEF) and small airways function (MMEF, FEF75, FEF50, and FEF25). Our major findings include the following: (1) PM2.5 exposure concentration is associated with reduced lung function parameters, including FEV1, PEF, MMEF, FEF75, FEF50, and FEF25 in healthy adults. High-level PM2.5 exposure has a stronger correlation with a decrease in lung function. PM2.5 may impair small airway function more seriously than large airway function (2). The impact of PM2.5 on lung function depends on the time lag (3). Qiju granules may alleviate the decrease in lung function after PM2.5 exposure.
Some studies have reported significantly different sensitivity to air pollution between male and female subjects (18–20), and others have found significant differences in the association between lung function and air pollution according to BMI (6, 21). Guo et al. (6) reported that the association between FEV1 and air pollution was stronger for the participants with low and normal BMI (BMI < 23 kg/m2). Siddique et al. (21) reported that the association between lung function and air pollution was stronger for both low normal BMI and obese participants compared with normal weight participants. Those studies suggest that sex and BMI may have an impact on the association between lung function and air pollution exposure. Therefore, we used linear mixed-effects models, adjusted sex and BMI to evaluate the association between PM2.5 exposure concentrations and lung function outcomes within each group at different time periods.
The association between PM2.5 exposure concentrations and lung health has been investigated in detail. Numerous epidemiology studies provided evidence that PM2.5 exposure damages lung function (6, 22, 23). A longitudinal cohort study indicates that each 10 μg/m3 increase in PM2.5 exposure concentrations is associated with decreases in FVC and FEV1 (6). A cross-sectional study of adults from Shanghai, China has shown that higher PM2.5 exposure concentrations are significantly associated with lower FVC, IC, and VC (12). PM2.5 is a main pollutant of ambient air pollution. The adverse effect on lung health is mostly attributed to its smaller particle size, larger surface area, physical characteristics of the particles, and stronger activity. In addition, PM2.5 may be composed of sulfur dioxide, sulfates, metals, nitrates, acids, and carbon particles with various chemicals adsorbed onto their surfaces (24). A recent study in an animal model demonstrated that PM2.5 led to easier deposition into lung tissues compared with other pollutants (25). The deposition of PM2.5 increases the expression of inflammatory cytokine by activating inflammatory-associated cells and triggers oxida-tive stress by impairing the immune balance (25). Some studies indicate that PM2.5 is a critical factor for overall lung function impairment progression by the regulation of oxidative stress in the lungs and inflammation in mice (26, 27). Hence, anti-oxidation and anti-inflammatory supplementation may protect people from the adverse effects of PM2.5 on lung function.
Qiju granules are extracted from traditional Chinese herbs, including Rhodiola rosea L. [Crassulaceae], Portulaca oleracea L. [Portulacaceae], Lycium barbarum L. [Solanaceae], Chrysanthemum × morifolium (Ramat.) Hemsl. [Asteraceae] and Siraitia grosvenorii (Swingle) C.Jeffrey ex A.M.Lu & Zhi Y.Zhang [Cucurbitaceae]. Rhodiola rosea L. [Crassulaceae] is often used as a part of the regimen to treat lung disorders. The main constituent of Rhodiola rosea L. [Crassulaceae] is salidroside, which shows attenuation in inflammation and oxidative stress response (12, 13). Portulaca oleracea L. [Portulacaceae] is a nutritious vegetable, which is useful for the prevention of diseases caused by oxidative stress. Lycium barbarum L. [Solanaceae] exhibits anti-oxidative and anti-inflammatory effects by modulating mRNA expression in many diseases (14). Chrysanthemum × morifolium (Ramat.) Hemsl. [Asteraceae] has been widely consumed as tea for centuries. The combinations of Chrysanthemum × morifolium (Ramat.) Hemsl. [Asteraceae] and Lycium barbarum L. [Solanaceae] also possess anti-oxidation and anti-inflammation properties by inhibiting MAPKs and NF-kB pathways (15). Siraitia grosvenorii (Swingle) C.Jeffrey ex A.M.Lu & Zhi Y.Zhang [Cucurbitaceae] has been used as a natural sweetener and as a traditional medicine for the treatment of pharyngitis and tussis. Its active constituents also show anti-oxidative, hypoglycemic, immunologic, anti-tussive, and sputum-reducing efficacy (16). These traditional Chinese herbs are widely used to treat and prevent lung disorders.
Our study demonstrates that exposure to PM2.5 concentration induced lung function impairment. However, the results indicate that only some lung function parameters are consistent with previous studies. In our study, we find an 8.80% decrease in the percentage of estimate of FEV1, and a 7.07% decrease in the percentage of estimate of PEF per 10 μg/m3 increase in PM2.5 exposure concentrations. These results are stronger than those reported in other studies (6, 28). This discrepancy may be attributable to the higher PM2.5 concentrations in our study time periods. We also measured small airway function parameters, including MMEF, FEF75, FEF50, and FEF25. Only a few previous studies have examined MMEF, FEF75, FEF50, and FEF25. We suggest that those are important parameters of small airway function that are critical in small airway disease development, such as asthma and chronic obstructive pulmonary disease (COPD) (29). In our study, we demonstrated that PM2.5 exposure concentrations generally had a stronger association with MMEF, FEF75, FEF50, and FEF25 than FEV1 and PEF. There is a 13.07% decrease in the percentage of the estimate of MMEF, a 13.38% decrease in FEF75, a 12.53% decrease in FEF50, a 9.67% decrease in FEF25 per 10 μg/m3 of increase in PM2.5 exposure concentration. However, there is only an 8.80% decrease in FEV1, a 7.07% decrease in PEF, and no significant decrease in FVC. It seems that our findings are consistent with several previous studies (6, 20, 22), which suggest that PM2.5 may have more serious impacts on small airway function than on large airway function.
Previous studies indicated that PM2.5 induced a significant delayed effect on population health (6, 23, 30, 31). In our study, we show that most lung function parameters are significantly correlated with weekly average PM2.5 exposure concentrations of lag 1 week, although other periods of average PM2.5 concentrations (lag 0 and 0–1 week) are not significantly correlated with most parameters of lung function, which seems different when compared with some previous studies. We assume it may be primarily due to the fact that weekly average PM2.5 concentrations were applied instead of daily average concentrations in those analyses while lag 1 week average concentrations were much higher than those in other time periods. It suggests that high-level PM2.5 exposure may have a stronger correlation with lung function, and the impact of PM2.5 on lung health does have time lags, which could be acute or even lifetime.
Our study also demonstrates that Qiju granules protect against PM2.5 induced lung function impairment, especially in small airway functions. In the control group, FEV1, PEF, MMEF, FEF75, FEF50, and FEF25 were significantly decreased when PM2.5 exposure concentrations were increased. However, this did not happen in the Qiju group when PM2.5 exposure concentrations were increased, which suggests that Qiju may have a preventive effect on small airway functions against PM2.5 exposure-related injury. This reason may be due to the active constituents of Qiju granules being able to attenuate inflammation, oxidative stress, and fibrosis response, protecting people from the adverse effects of PM2.5 on lung function. We also compare the change in parameters of lung function between the Qiju group and the control group when adjusting the PM2.5 exposure concentrations. Our data demonstrate that the majority of lung function parameters are slightly higher in the Qiju group than in the control group in the 2nd week and 4th week. Unfortunately, there is no significant difference between the two groups in all lung function parameters during these time periods. This could be attributed to our limited enrollment size (40) along with the brief duration of the study (4 weeks), which might not provide sufficient power to demonstrate any further potential lung function benefits.
5 Strengths
This study has several strengths. The first strength of the study is reducing the effects of psychological and other confounding factors on outcomes by double-blinded and placebo control. The second strength of the study is monitoring PM2.5 exposure concentrations with personal and portable devices, rather than estimating exposures based on outdoor monitors, are precise real-time personal exposures and variation range of exposures for regression analysis. The third strength of the study is that it is conducted among healthy college students on campus, rather than in an exposure-controlled environment, which is closer to real-world situations where local residents live. The fourth strength of the study is that the participants are healthy college students, who are a vulnerable population to PM2.5 exposure (32). In addition, the season of the study period has relatively high PM2.5 concentrations, which occur frequently in major cities in China and in other developing countries. It has enabled us to obtain stable and precise estimates. Finally, we analyze numerous lung function parameters, including large and small airway function parameters, and use mixed-effects models, adjusting sex and BMI to evaluate PM2.5 exposure in relation to lung functions. It provides more stable and reliable results.
6 Limitations
This study also has several limitations. The first limitation is the study sample, comprising only 20 participants per group, which consisted of healthy individuals within a relatively ideal experimental setting. Thus, caution should be exercised when generalizing these findings to the broader population and real-world contexts. The second limitation is that healthy adults may be less susceptible to the adverse effects of PM2.5 exposure on lung dysfunction and respiratory disorders compared with vulnerable populations, such as children and seniors. The lung function benefits from Qiju granules await further verification with more susceptible and diverse populational studies. The third limitation is that other air pollutants, such as SO2, NO2, and Ozone, and meteorological parameters, including temperature and humidity, are not collected and analyzed. Therefore, we cannot distinguish the association between PM2.5 and lung dysfunction due to PM2.5 specifically or in conjunction with other air pollutants and meteorological factors. Finally, although we adjust sex and BMI for the association between PM2.5 and lung function, more confounding factors, such as behavior, diet, and living environment, should be considered in future studies.
7 Conclusion
In summary, PM2.5 exposure is associated with reduced lung function parameters. Qiju granules significantly alleviate the decrease in lung function after PM2.5 exposure, which may be effective in protecting lung health against adverse effects of PM2.5 exposure.
Data availability statement
The original contributions presented in the study are included in the article/Supplementary material, further inquiries can be directed to the corresponding author.
Ethics statement
The studies involving humans were registered in Chinese Clinical Trial Registry (ChiCTR1900021235) on 13 February 2019 and was approved by the Ethics Committee of the First Affiliated Hospital of Zhejiang Chinese Medical University. The studies were conducted in accordance with the local legislation and institutional requirements. The participants provided their written informed consent to participate in this study.
Author contributions
RC: Writing – original draft. LuZ: Writing – original draft. WG: Writing – original draft. RL: Writing – original draft. HH: Writing – original draft. LiZ: Writing – original draft. JZ: Writing – original draft. YW: Writing – original draft. PN: Writing – original draft. SX: Writing – original draft. ZW: Writing – review & editing. QS: Writing – review & editing. CL: Writing – review & editing. JY: Writing – review & editing.
Funding
The author(s) declare financial support was received for the research, authorship, and/or publication of this article. This work was supported by the National Natural Science Foundation of China (grant number 82004143), Education Project of Zhejiang Province (grant number Y202045128), Key R&D International Cooperation Projects (grant number 2019YFE0114500), Medical Health Science and Technology Project of Zhejiang Province (grant numbers 2019KY471 and 2018KY545), and Foundation of Zhejiang Chinese Medical University (grant number 2019ZZ04).
Acknowledgments
The authors gratefully acknowledge all volunteers who participated in this study.
Conflict of interest
The authors declare that the research was conducted in the absence of any commercial or financial relationships that could be construed as a potential conflict of interest.
Publisher's note
All claims expressed in this article are solely those of the authors and do not necessarily represent those of their affiliated organizations, or those of the publisher, the editors and the reviewers. Any product that may be evaluated in this article, or claim that may be made by its manufacturer, is not guaranteed or endorsed by the publisher.
Supplementary material
The Supplementary Material for this article can be found online at: https://www.frontiersin.org/articles/10.3389/fmed.2024.1370657/full#supplementary-material
References
1. Liu C, Chen R, Sera F, Vicedo-Cabrera AM, Guo Y, Tong S, et al. Ambient particulate air pollution and daily mortality in 652 cities. N Engl J Med. (2019) 381:705–15. doi: 10.1056/NEJMc1913285
2. Pun VC, Kazemiparkouhi F, Manjourides J, Suh HH. Long-Term PM2. 5 exposure and respiratory, cancer, and cardiovascular mortality in older US adults. Am J Epidemiol. (2017) 186:961–9. doi: 10.1093/aje/kwx166
3. Collaborators GBDRF. Global, regional, and national comparative risk assessment of 84 behavioural, environmental and occupational, and metabolic risks or clusters of risks for 195 countries and territories, 1990-2017: a systematic analysis for the Global Burden of Disease Study 2017. Lancet. (2018) 392:1923–94. doi: 10.1016/S0140-6736(18)32225-6
4. Organization WH. Out of 10 People Worldwide Breathe Polluted Air, But More Countries are Taking Action. (2018). Available online at: http://wwwwhoint/news-room/detail/02-05-2018-9-out-of-10-people-worldwide-breathe-polluted-air-but-more-countries-are-taking-action (accessed May 2, 2018).
5. Chen R, Zhao A, Chen H, Zhao Z, Cai J, Wang C, et al. Cardiopulmonary benefits of reducing indoor particles of outdoor origin: a randomized, double-blind crossover trial of air purifiers. J Am Coll Cardiol. (2015) 65:2279–87. doi: 10.1016/j.jacc.2015.03.553
6. Guo C, Hoek G, Chang LY, Bo Y, Lin C, Huang B, et al. Long-term exposure to ambient fine particulate matter (PM2. 5) and lung function in children, adolescents, and young adults: a longitudinal cohort study. Environ Health Perspect. (2019) 127:127008. doi: 10.1289/EHP5220
7. Pacitto A, Amato F, Moreno T, Pandolfi M, Fonseca A, Mazaheri M, et al. Effect of ventilation strategies and air purifiers on the children's exposure to airborne particles and gaseous pollutants in school gyms. Sci Total Environ. (2020) 712:135673. doi: 10.1016/j.scitotenv.2019.135673
8. Ni L, Chuang CC, Zuo L. Fine particulate matter in acute exacerbation of COPD. Front Physiol. (2015) 6:294. doi: 10.3389/fphys.2015.00294
9. Wang J, Xiong X, Yang G, Zhang Y, Liu Y, Zhang Y, et al. Chinese herbal medicine qi ju di huang wan for the treatment of essential hypertension: a systematic review of randomized controlled trials. Evid Based Complement Alternat Med. (2013) 2013:262685. doi: 10.1155/2013/262685
10. Chau C. The Chinese traditional diet: a socioecological approach. J Austr Trad Med Soc. (2019) 25:222–4.
11. Shahrajabian M, Sun W, Zandi P, Cheng Q, A. review of chrysanthemum, the eastern queen in traditional Chinese medicine with healing power in modern pharmaceutical sciences. Appl Ecol Environ Res. (2019) 17:13355–69. doi: 10.15666/aeer/1706_1335513369
12. Hu R, Wang MQ Ni SH, Wang M, Liu LY, You HY, et al. Salidroside ameliorates endothelial inflammation and oxidative stress by regulating the AMPK/NF-kappaB/NLRP3 signaling pathway in AGEs-induced HUVECs. Eur J Pharmacol. (2020) 867:172797. doi: 10.1016/j.ejphar.2019.172797
13. Pu WL, Zhang MY, Bai RY, Sun LK Li WH, Yu YL, et al. Anti-inflammatory effects of Rhodiola rosea L.: A review. Biomed Pharmacother. (2020) 121:109552. doi: 10.1016/j.biopha.2019.109552
14. Lee YJ, Ahn Y, Kwon O, Lee MY, Lee CH, Lee S, et al. Dietary wolfberry extract modifies oxidative stress by controlling the expression of inflammatory mRNAs in overweight and hypercholesterolemic subjects: a randomized, double-blind, placebo-controlled trial. J Agric Food Chem. (2017) 65:309–16. doi: 10.1021/acs.jafc.6b04701
15. Zhang N, He Z, He S, Jing P. Insights into the importance of dietary chrysanthemum flower (Chrysanthemum morifolium cv. Hangju)-wolfberry (Lycium barbarum fruit) combination in antioxidant and anti-inflammatory properties. Food Res Int. (2019) 116:810–8. doi: 10.1016/j.foodres.2018.09.015
16. Gong X, Chen N, Ren K, Jia J, Wei K, Zhang L, et al. The fruits of Siraitia grosvenorii: a review of a Chinese food-medicine. Front Pharmacol. (2019) 10:1400. doi: 10.3389/fphar.2019.01400
17. Xu S, Yang J, Liu C, Chen R, Wang Z, Hong H. Preventive effect of Qiju decoction on acute lung injury caused by PM2. 5 in mice. Zhejiang Clin Med J. (2020) 22:617–9.
18. Gauderman WJ, Vora H, McConnell R, Berhane K, Gilliland F, Thomas D, et al. Effect of exposure to traffic on lung development from 10 to 18 years of age: a cohort study. Lancet. (2007) 369:571–7. doi: 10.1016/S0140-6736(07)60037-3
19. Gauderman WJ, Urman R, Avol E, Berhane K, McConnell R, Rappaport E, et al. Association of improved air quality with lung development in children. N Engl J Med. (2015) 372:905–13. doi: 10.1056/NEJMoa1414123
20. Oftedal B, Brunekreef B, Nystad W, Madsen C, Walker SE, Nafstad P. Residential outdoor air pollution and lung function in schoolchildren. Epidemiology. (2008) 19:129–37. doi: 10.1097/EDE.0b013e31815c0827
21. Siddique S, Banerjee M, Ray MR, Lahiri T. Air pollution and its impact on lung function of children in Delhi, the capital city of India. Water, Air, & Soil Pollution. (2010) 212:89–100. doi: 10.1007/s11270-010-0324-1
22. Hwang BF, Chen YH, Lin YT, Wu XT, Leo Lee Y. Relationship between exposure to fine particulates and ozone and reduced lung function in children. Environ Res. (2015) 137:382–90. doi: 10.1016/j.envres.2015.01.009
23. Xu D, Zhang Y, Zhou L, Li T. Acute effects of PM2. 5 on lung function parameters in schoolchildren in Nanjing, China: a panel study. Environ Sci Pollut Res Int. (2018) 25:14989–95. doi: 10.1007/s11356-018-1693-z
24. Hu Y, Zhou Q, Liu T, Liu Z. Coixol suppresses NF-kappaB, MAPK pathways and NLRP3 inflammasome activation in lipopolysaccharide-induced RAW 264.7 Cells. Molecules. (2020) 25:894. doi: 10.3390/molecules25040894
25. Li Q, Liu H, Alattar M, Jiang S, Han J, Ma Y, et al. The preferential accumulation of heavy metals in different tissues following frequent respiratory exposure to PM2. 5 in rats Sci Rep. (2015) 5:16936. doi: 10.1038/srep16936
26. Jiang S, Bo L, Du X, Liu J, Zeng X, He G, et al. CARD9-mediated ambient PM2. 5-induced pulmonary injury is associated with Th17 cell. Toxicol Lett. (2017) 273:36–43. doi: 10.1016/j.toxlet.2017.03.015
27. Gu LZ, Sun H, Chen JH. Histone deacetylases 3 deletion restrains PM2. 5-induced mice lung injury by regulating NF-kappaB and TGF-beta/Smad2/3 signaling pathways. Biomed Pharmacother. (2017) 85:756–62. doi: 10.1016/j.biopha.2016.11.094
28. Gehring U, Gruzieva O, Agius RM, Beelen R, Custovic A, Cyrys J, et al. Air pollution exposure and lung function in children: the ESCAPE project. Environ Health Perspect. (2013) 121:1357–64. doi: 10.1289/ehp.1306770
29. Gilliland FD, Berhane K, McConnell R, Gauderman WJ, Vora H, Rappaport EB, et al. Maternal smoking during pregnancy, environmental tobacco smoke exposure and childhood lung function. Thorax. (2000) 55:271–6. doi: 10.1136/thorax.55.4.271
30. Hsu SC, Chang JH, Lee CL, Huang WC, Hsu YP, Liu CT, et al. Differential time-lag effects of ambient PM2. 5 and PM25-bound PAHs on asthma emergency department visits. Environ Sci Pollut Res Int. (2020) 27:43117–24. doi: 10.1007/s11356-020-10243-y
31. Chu H, Xin J, Yuan Q, Zhang X, Pan W, Zeng X, et al. Evaluation of vulnerable PM2. 5-exposure individuals: a repeated-measure study in an elderly population. Environ Sci Pollut Res Int. (2018) 25:11833–40. doi: 10.1007/s11356-018-1412-9
Keywords: fine particulate matter, lung function, traditional Chinese medicine, clinical trial, Qiju granules
Citation: Chen R, Zhang L, Gu W, Li R, Hong H, Zhou L, Zhang J, Wang Y, Ni P, Xu S, Wang Z, Sun Q, Liu C and Yang J (2024) Lung function benefits of traditional Chinese medicine Qiju granules against fine particulate air pollution exposure: a randomized controlled trial. Front. Med. 11:1370657. doi: 10.3389/fmed.2024.1370657
Received: 02 February 2024; Accepted: 01 April 2024;
Published: 29 April 2024.
Edited by:
James David Adams, Independent Researcher, Benicia, United StatesReviewed by:
Wanzhou Wang, Peking University, ChinaXiaobei Deng, Shanghai Jiao Tong University, China
Copyright © 2024 Chen, Zhang, Gu, Li, Hong, Zhou, Zhang, Wang, Ni, Xu, Wang, Sun, Liu and Yang. This is an open-access article distributed under the terms of the Creative Commons Attribution License (CC BY). The use, distribution or reproduction in other forums is permitted, provided the original author(s) and the copyright owner(s) are credited and that the original publication in this journal is cited, in accordance with accepted academic practice. No use, distribution or reproduction is permitted which does not comply with these terms.
*Correspondence: Junchao Yang, yangjunchaozj@zcmu.edu.cn
†These authors have contributed equally to this work and share first authorship