Genetic correlation between circulating metabolites and chalazion: a two-sample Mendelian randomization study
- 1Department of Ophthalmology, Yangpu Hospital, School of Medicine, Tongji University, Shanghai, China
- 2Department of Oncology, Shanghai Ninth People’s Hospital, Shanghai Jiao Tong University School of Medicine, Shanghai, China
- 3Department of General Practice, Clinical Research Center for General Practice, Yangpu Hospital, School of Medicine, Tongji University, Shanghai, China
Background: Lipid metabolism disorders were observationally associated with chalazion, but the causality of the related circulating metabolites on chalazion remained unknown. Here, we investigated the potential causal relationship between circulating metabolites and chalazion using two-sample Mendelian randomization (MR) analysis.
Methods: For the primary analysis, 249 metabolic biomarkers were obtained from the UK Biobank, and 123 circulating metabolites were obtained from the publication by Kuttunen et al. for the secondary analysis. Chalazion summary data were obtained from the FinnGen database. Inverse variance weighted (IVW) is the main MR analysis method, and the MR assumptions were evaluated in sensitivity and colocalization analyses.
Results: Two MR analyses results showed that the common metabolite, alanine, exhibited a genetic protective effect against chalazion (primary analysis: odds ratio [OR] = 0.680; 95% confidence interval [CI], 0.507–0.912; p = 0.010; secondary analysis: OR = 0.578; 95% CI, 0.439–0.759; p = 0.00008). The robustness of the findings was supported by heterogeneity and horizontal pleiotropy analysis. Two colocalization analyses showed that alanine did not share a region of genetic variation with chalazion (primary analysis: PPH4 = 1.95%; secondary analysis: PPH4 = 25.3%). Moreover, previous studies have suggested that an increase in the degree of unsaturation is associated with an elevated risk of chalazion (OR = 1.216; 95% CI, 1.055–1.401; p = 0.007), with omega-3 fatty acids (OR = 1.204; 95% CI, 1.054–1.377; p = 0.006) appearing to be the major contributing factor, as opposed to omega-6 fatty acids (OR = 0.850; 95% CI, 0.735–0.982; p = 0.027).
Conclusion: This study suggests that alanine and several unsaturated fatty acids are candidate molecules for mechanistic exploration and drug target selection in chalazion.
Introduction
Chalazion is a benign eyelid lesion and manifests as a chronic lipogranulomatous inflammatory response secondary to the degeneration of endogenous lipid secretion from the meibomian or Zeis glands (Knop and Knop, 2009). Infectious factors, such as blepharitis, viral infection, and ocular demodex infestation (Liang et al., 2014; Kim et al., 2023), along with non-infectious factors [such as rosacea, anxiety, smoking, nutritional deficiencies, and irritable bowel syndrome (IBS)], may increase chalazion risk (Nemet et al., 2011; Patel et al., 2022).
Studies have shown that the ratio of cholesterol to cholesteryl esters is higher in chalazion lipids than in normal meibum, probably because elevated cholesterol levels influence the chemotaxis of inflammatory cells into the meibomian gland, obstructing the gland and leading to excess lipid infiltration of the surrounding tissue (Melo et al., 2011; Wojtowicz et al., 2014). Notably, a study showed that oral statins reduced chalazion risk, indicating its linkage to inadequate lipid metabolism (Feng et al., 2022). Apart from disorders of lipid metabolism, deficiencies in circulating nutrients such as amino acids, vitamins, and thyroxine can also contribute to chalazion risk (Malekahmadi et al., 2017; Cheng et al., 2022; Ilhan, 2022).
However, most studies on circulating metabolites for chalazion have been observational and susceptible to potential confounders and reverse causality (Lawlor et al., 2008). Mendelian randomization (MR) is a method that can evaluate the causal relationship between exposures and outcomes by employing a genetic variant as an instrumental variable for exposure. This approach helps to mitigate the confounding effects inherent in observational studies (Davey Smith and Ebrahim, 2005; Yuan et al., 2021). We used a two-sample MR to investigate the causal relationship between circulating metabolites and chalazion risk, and other factors for chalazion, including inflammation of the eyelid, conjunctivitis, anxiety/panic attacks, alcohol consumption, body mass index (BMI), current tobacco smoking, and IBS were analyzed to assess the effect of confounders.
Materials and methods
Study population and design
We conducted a two-sample MR analysis to determine the causal effect of circulating metabolites on chalazion. Genome-wide association study (GWAS) summary level data for circulating metabolites were obtained from two different datasets, and chalazion summary data were obtained from the FinnGen database. The robustness of these causal relationships was assessed by sensitivity and colocalization analyses. Only individuals of European origin were included in the analysis. All included studies received informed consent and local ethics committee approval. An overview of the study design is shown in Figure 1.
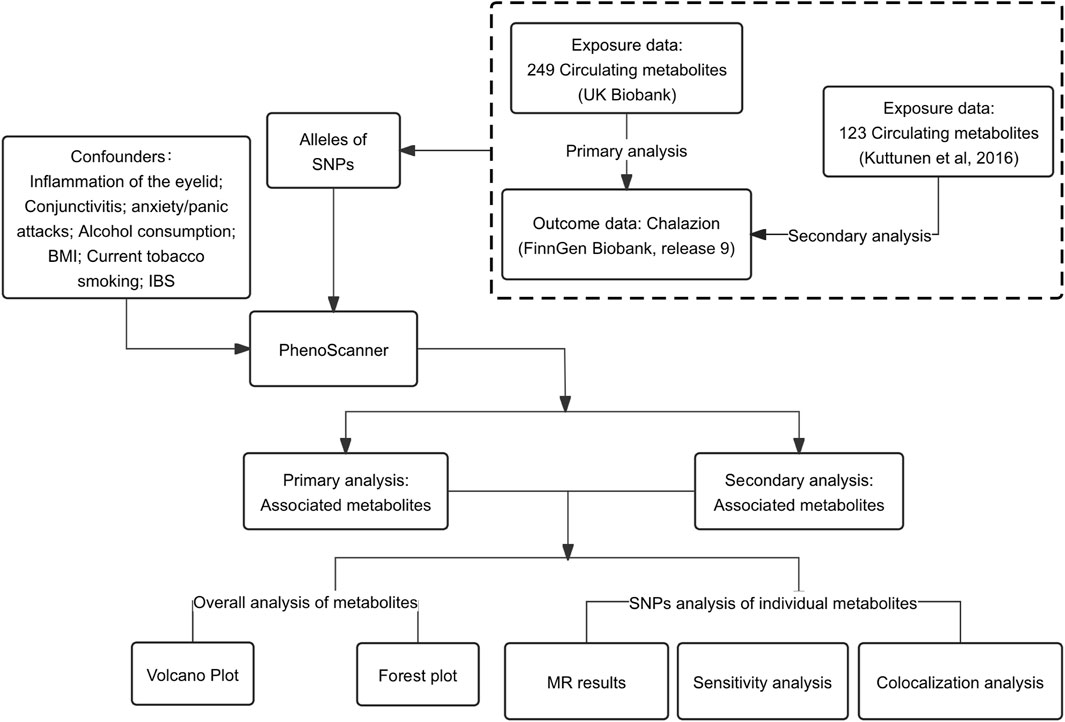
Figure 1. Flowchart of the present Mendelian randomization analysis. Abbreviations: SNPs, single nucleotide polymorphisms; MR, Mendelian randomization; IBS, irritable bowel syndrome; BMI, body mass index.
Sources of data included in the analysis
Summary-level GWAS datasets of 249 metabolic biomarkers for the primary analysis were derived from the UK Biobank and measured using the Nightingale Health Metabolic Biomarkers Phase 1 release study. This study included 115,078 randomly selected participants from the UK Biobank cohort, and analysed a total of 249 metabolites, with 168 metabolites measured in absolute concentrations (mmol/L) and 81 measurements presented in ratios (https://biobank.ndph.ox.ac.uk/ukb/label.cgi?id=220) (Mi et al., 2022). Summary-level datasets of 123 circulating metabolites used for secondary analyses were obtained from a previous publication by Kettunen et al. (2016), including 24,925 individuals of European ancestry. Summary-level statistics for chalazion were obtained from the FinnGen database (Release 9), which included 348,073 individuals of European ancestry (case: 3,389; control: 344,684). Confounders included eyelid inflammation, conjunctivitis, anxiety/panic attacks, alcohol consumption, BMI, smoking, and IBS, which were obtained from the IEU OpenGWAS project (https://gwas.mrcieu.ac.uk/datasets/) (Supplementary Table S1).
Selection of genetic instrumental variables
SNPs associated with circulating metabolites were selected according to a significance threshold (p < 5 × 10−8) and a reference panel (with a window size of 10,000 kb and r2 < 0.001) to estimate the linkage disequilibrium between SNPs and select independent genetic variants (Levin et al., 2020). The F-statistic was calculated to test for weak instruments (F-statistics < 10) as previously described (Burgess et al., 2011; Burgess and Thompson, 2011). Instrumental variables (IVs) for MR studies need to fulfil three hypotheses: 1) IVs should be strongly correlated with exposure; 2) IVs should not be associated with any confounders; and 3) IVs affect outcomes only through exposure and not through any other alternative pathways (Palmer et al., 2012).
Colocalization analysis
The presence of shared causal variants between exposure and outcome was analysed using GWAS-GWAS colocalization, with a reference panel (GWAS gene window default of 50 kb, p < 5 × 10−8) (Venkateswaran et al., 2018). Colocalization analyses were performed using the “coloc” package, and a Bayesian framework was used to generate posterior probabilities for five mutually exclusive hypotheses about causal variance sharing between two traits: (H0–H4): H0: the locus is not associated with circulating metabolites and chalazion; H1: the locus is associated with circulating metabolites, but not with chalazion; H2: the locus is associated with chalazion, but not with circulating metabolites; H3: the locus is associated with circulating metabolites and chalazion (two independent SNPs) and H4: the locus is associated with circulating metabolites and chalazion (one shared SNP) (Giambartolomei et al., 2014; Venkateswaran et al., 2018). A posterior probability (PP) of H4 (PPH4) > 0.8 was identified as evidence in favour of colocalization. The “locuscomparer” package was used for visualization of colocalization (Liu et al., 2019).
Statistical analysis
Causal associations between each metabolite and chalazion were determined using IVW methods. The IVW estimate is the mean of ratio estimates from two or more instruments, based on the assumption that all SNPs are valid instruments or that the overall bias is zero (Burgess et al., 2013). MR Egger regression, weighted median, and weighted mode methods were used to relax the IVW assumptions (Burgess et al., 2017a; Burgess et al., 2019). For single genetic instruments, causal effects were estimated by the wald ratio test (Burgess et al., 2017b). Phenoscanner (http://www.phenoscanner.medschl.cam.ac.uk/) was used to exclude the SNPs associated with confounders (Staley et al., 2016). Sensitivity analyses were performed to assess the validity and robustness of the MR assumptions, including Cochran’s Q to test for heterogeneity among IVs of metabolites (Burgess et al., 2013), MR-Egger regression for horizontal pleiotropy assessment (Bowden et al., 2015), and leave-one-out test. If heterogeneity was present, the multiplicative random effects model was chosen (Burgess et al., 2019). MR-Steiger was used to filter out SNPs suggesting opposite causal directions (Hemani et al., 2017).
We chose the Bonferroni method for multiple testing; associations with p-values below 0.0002 (0.05/249) in the primary analysis and 0.0004 (0.05/121) in the secondary analysis were considered strong evidence of an association, and associations with p-values between 0.0002 and 0.05 in the primary analysis, and between 0.0004 and 0.05 in the secondary analysis were considered suggestive. All analyses were performed on the R platform (version 4.1.0) using the “TwoSampleMR,” “Coloc,” and “forestploter” packages.
Results
Association of relevant confounders with chalazion
We first estimated the causal relationship of confounders, including inflammation of the eyelid, conjunctivitis, anxiety/panic attacks, alcohol consumption, BMI, current tobacco smoking, and IBS, with chalazion risk, using a two-sample MR method. The results showed that BMI [OR = 0.970; 95% CI, 0.826–1.138; P(IVW) = 0.709], Current tobacco smoking [OR = 0.724; 95% CI, 0.214–2.454; P(IVW) = 0.604], Alcohol consumption [OR = 1.334; 95% CI, 0.099–17.99; P(IVW) = 0.828], IBD [OR = 2.49E-06; 95% CI, 9.06E-24-6.81704E+11; P(Wald ratio) = 0.529], and conjunctivitis [OR = 0.945; 95% CI, 0.495–1.805; P(Wald ratio) = 0.863] were not association with chalazion risk, and the remaining confounders failed to establish associations with chalazion due to the absence of SNPs (Supplementary Table S2).
Association of metabolites on chalazion
In the primary analysis, we screened 249 circulating metabolites with a total of 13,275 SNPs when the screening threshold was (r2 < 0.001, kb = 10,000, p < 5 × 10−8). In the secondary analysis, we screened 123 circulating metabolites with a total of 1,375 SNPs when the screening threshold was (r2 < 0.001, kb = 10,000, p < 5 × 10−8), among them, two metabolites glycerol (met-c-861) and urea (met-c-939), had no SNP extracted. All IVs in the current analysis were robust instruments, with the range of F-statistics was 23-16414 (Supplementary Tables S3, S4). We used a two-sample MR to estimate the causal effect of 249 metabolites on chalazion in the primary analysis and 121 circulating metabolic biomarkers risk in the secondary analysis (Supplementary Tables S5, S6). Based on the IVW method, seven of the 249 metabolites were found to be suggestively associated with chalazion, with five unsaturation-associated biomarkers showing an association with a high risk of chalazion and two unsaturation-associated biomarkers showing a causal effect with a low risk of a chalazion (Figure 2A). There were as follows: alanine (OR = 0.680; 95% CI, 0.507–0.912; p = 0.010), acetone (OR = 1.726; 95% CI, 1.064–2.798; p = 0.027), degree of unsaturation (OR = 1.216; 95% CI, 1.055–1.401; p = 0.007), ratio of omega-3 fatty acids to total fatty acids (OR = 1.204; 95% CI, 1.054–1.377; p = 0.006), ratio of omega-6 to omega-3 fatty acids (OR = 0.850; 95% CI, 0.735–0.982; p = 0.027), ratio of polyunsaturated fatty acids to total fatty acids (OR = 1.214; 95% CI, 1.008–1.462; p = 0.041), and ratio of docosahexaenoic acid to total fatty acids (OR = 1.267; 95% CI, 1.092–1.471; p = 0.002) (Figure 2B; Table 1). In the secondary analysis, four of the 121 metabolites were found to be significantly associated with chalazion and three were suggestively associated with chalazion. Of these, three unsaturation-associated biomarkers significantly increased the risk of chalazion, and four unsaturation-associated biomarkers showed a negative correlation with chalazion susceptibility (Figure 3A). They were alanine (OR = 0.578; 95% CI, 0.439–0.759; p = 0.00008), the average number of methylene groups per double bond (OR = 0.768; 95% CI, 0.615–0.958; p = 0.020), the average number of double bonds in a fatty acid chain (OR = 1.380; 95% CI, 1.135–1.678; p = 0.001), ratio of bisallylic groups to double bonds (1.298 [95% CI, 1.145–1.471], p = 0.00005), ratio of bisallylic groups to total fatty acids (OR = 1.364; 95% CI, 1.163–1.600; p = 0.00013), 18:2, linoleic acid (LA) (OR = 0.827; 95% CI, 0.720–0.950; p = 0.007), and pyruvate (OR = 0.422; 95% CI, 0.265–0.674; p = 0.00029) (Figure 3B; Table 2). MR results of individual SNPs for all metabolites were shown in Supplementary Tables S7, S8.
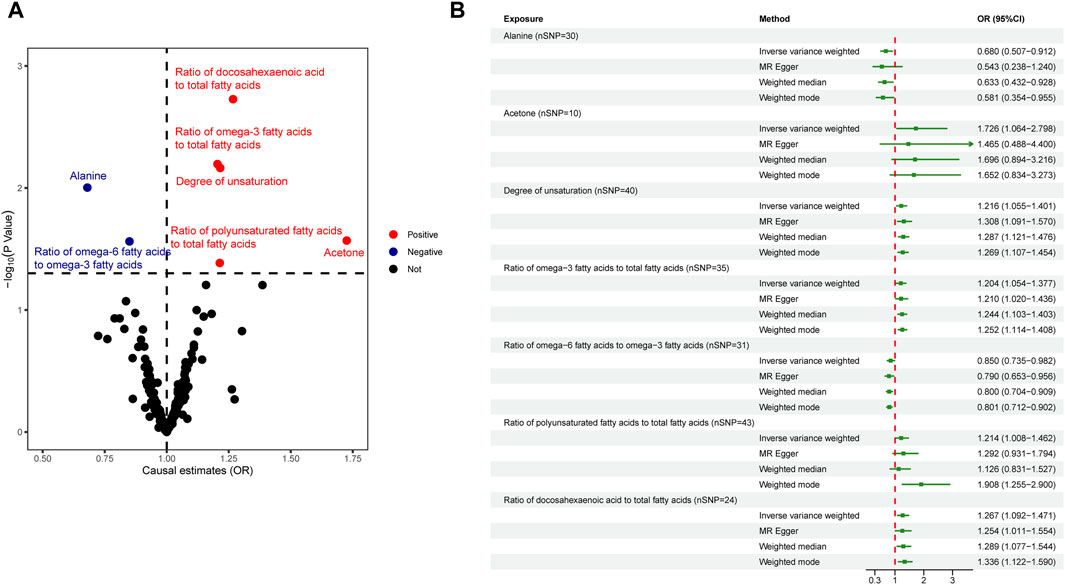
Figure 2. Causal relationship between 249 metabolites and chalazion. (A) Volcano plot analysis of Mendelian randomization results. Red plots indicate OR > 1, which is positively associated with the risk of developing chalazion, and blue plots indicate OR < 1, which is negatively associated with the risk of developing chalazion. (B) Forest plot analysis of Mendelian randomization results. Abbreviations: OR, odds ratio.
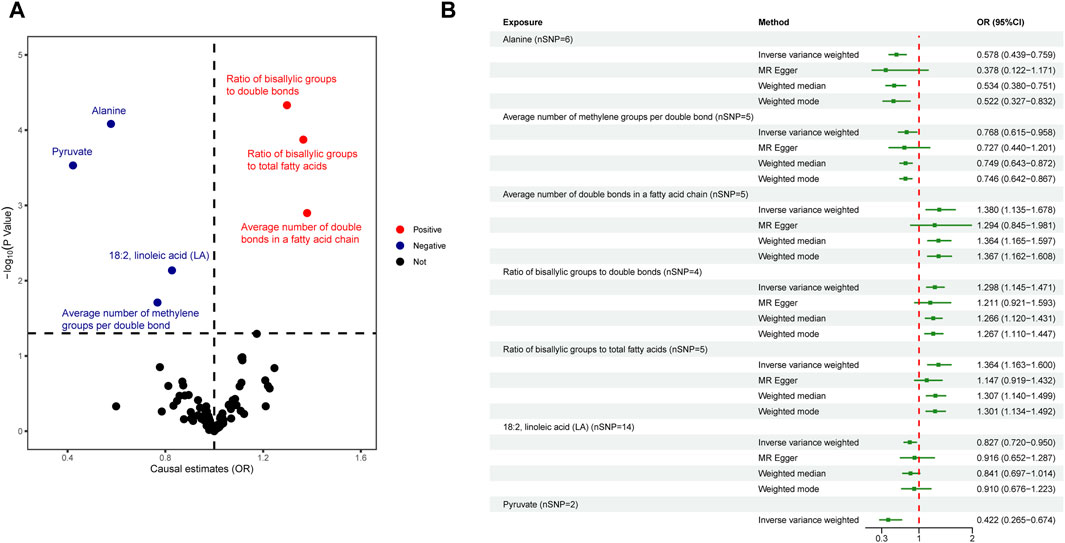
Figure 3. Causal relationship between 123 metabolites and chalazion. (A) Volcano plot analysis of Mendelian randomization results. Red plots indicate OR > 1, which is positively associated with the risk of developing chalazion, and blue plots indicate OR < 1, which is negatively associated with the risk of developing chalazion. (B) Forest plot analysis of Mendelian randomization results. Abbreviations: OR, odds ratio.
Sensitivity analysis
In the sensitivity analysis of all the differential metabolites, heterogeneity (IVW method) was present only for unsaturation (p = 0.045), ratio of omega-6 to omega-3 fatty acids (p = 0.008), ratio of omega-3 fatty acids to total fatty acids (p = 0.018) in 249 metabolites (Supplementary Table S9), and average number of methylene groups per double bond (p = 0.016) in 123 metabolites (Supplementary Table S10), and we adopted a MR analysis with multiplicative random effects model. The horizontal pleiotropy analysis showed that there was no direct association between IVs and chalazion, and MR Steiger test results showed overall SNPs affected outcomes only by exposure and not by other pathways (Supplementary Tables S11, S12), suggesting that the results were relatively robust. Plots of the leave-one-out test showed that regardless of which SNP was excluded, it did not have any effect on the MR results for alanine, ratio of bisallylic groups to double bonds, and ratio of bisallylic groups to total fatty acids (All lines were on one side of 0). However, for other unsaturated fatty acids, there is a potentially influential SNP driving the causal link between these metabolites and chalazion. Therefore, we need to interpret this result with caution (Supplementary Figure S1).
Colocalization analysis
Two colocalization analyses showed that there was no shared genetic variation region between alanine and chalazion (1.95% for PPH4 in the primary analysis and 25.3% in the secondary analysis), suggesting that the genetic variant SNPs were reducing chalazion risk through their impact on alanine. Shared regions of genetic variation were not observed for the differential metabolites in the primary analysis. In the secondary analysis, we identified two metabolites: the ratio of bisallylic groups to double bonds (PPH4 = 0.817) and 18:2, linoleic acid (LA) (PPH4 = 0.870), which shared one SNP with chalazion (rs174555), corresponding to the nearest genes FADS1 and FADS2 (Table 3; Supplementary Figure S2).
Discussion
In this study, we conducted an MR analysis to explore the causal relationship between circulating metabolites and chalazion. The primary analysis results showed a negative causal association between the metabolite alanine and chalazion risk, which was similarly verified in the secondary analysis. Sensitivity analyses enhanced the reliability of our results in eliminating pleiotropy. The colocalization analyses showed that genetic variant SNPs could exclusively mitigate the risk of chalazion through their influence on alanine. Alanine is the link between glucose, the tricarboxylic acid cycle, and amino acid metabolism and can form pyruvate and glutamate via an alanine aminotransferase-catalysed reaction with α-ketoglutarate, which plays a key role in the glucose-alanine cycle between tissues and the liver (Peng et al., 1991). Alanine can shuttle between neurones and astrocytes, transport nitrogen from the glutamine amide group, exchange lactate, and facilitate metabolite exchange between neurones and astrocytes (Schousboe et al., 2003). Previous studies have found that concentrations of lactate and alanine are low in patients with type 2 diabetes mellitus, breast cancer, and cervical cancer, suggesting altered energy metabolism in these diseases (Morvan and Demidem, 2007; Mamtimin et al., 2014).
In addition to alanine, pyruvate was effective in reducing the risk of chalazion development. Pyruvate is produced from alanine in the liver by the reaction of alanine aminotransferase to produce pyruvate and regenerate glucose in the body through gluconeogenesis to provide energy (Prochownik and Wang, 2021). Pyruvate is an important product of glycolysis and can be converted to acetyl-CoA before entering the tricarboxylic acid (TCA) cycle (Han et al., 2020), and acetyl-CoA is an important intermediate metabolite in the metabolism of energy substances. Alanine and pyruvate are closely related to the metabolic pathways of alanine, aspartate and glutamate metabolism, the TCA cycle, glycolysis or gluconeogenesis, and pyruvate metabolism, which are important for the maintenance of normal physiological states of the retina (Rueda et al., 2016), optic nerve cells (Agudo-Barriuso et al., 2013), lens (Saravanan et al., 2023), and cornea (Hamuro et al., 2020). And these studies give us confidence that alanine and pyruvate may be biomarkers in the metabolic process of eye diseases.
Our study also identified some associations between unsaturated fatty acids and chalazion risk. In the primary analysis, the higher the percentage of unsaturated fatty acids, the higher the risk of chalazion. We found that the higher the ratio of omega-6 to omega-3 fatty acids, the lower the risk of chalazion, suggesting that omega-6 fatty acids protect against chalazion among unsaturated fatty acids, whereas omega-3 fatty acids and other unsaturated fatty acids may increase the risk of chalazion. In the results of the secondary analysis, the ability of 18:2, LA [an omega-6 fatty acid (Balić et al., 2020)] to reduce the risk of chalazion also supports the results of the primary analysis of the protective effect of omega-6 fatty acids on chalazion. This has been somewhat controversial in previous studies on ocular unsaturated fatty acids. Studies have shown that omega-3 fatty acids are effective in reducing inflammation associated with dry eye, improving the lipid layer of the tear film, and normalising the function of the levator palpebral and lacrimal glands (Cortina and Bazan, 2011; Georgakopoulos et al., 2017). In recent years, several studies have questioned the efficacy of omega-3 fatty acids in the treatment of dry eye and found that low concentrations of omega-6 fatty acids (not omega-3 fatty acids) are beneficial in improving tear stability in patients with dry eye (Mudgil, 2020). Similarly, in the fatty acid category of the secondary analyses, we observed that more double bonds in the fatty acids increased the risk of chalazion. A higher number and proportion of double bonds and bisallylic groups in the fatty acid chain increase the risk of chalazion formation. Unsaturated fatty acids contain more double bonds than saturated fatty acids, which is consistent with the results of our primary analysis. In summary, we found an increased risk of chalazion when fatty acids had a higher number and ratio of double bonds and bisallylic groups because omega-6 fatty acids have fewer double bonds than omega-3 fatty acids (2–4 vs. 3-6 double bonds). An increase in the number of bisallylic groups can cause oxidative stress reactions (Yamaguchi et al., 2012; Chistyakov et al., 2018). A higher number and proportion of dienophiles have been reported to increase cellular susceptibility to free radical-mediated peroxidative events, which increases the risk of chalazion (Wagner et al., 1994). The gene colocalization results highlighted the significance of targeting rs174555, which is associated with FADS1 and FADS2. FADS1 and FADS1 encode fatty acid δ-5 and δ-56 desaturases (D5D and D6D), respectively, crucial for the conversion of PUFAs (polyunsaturated fatty acids) into eicosapentaenoic acid (EPA), docosahexaenoic acid, (DHA) arachidonic acid (ARA) (Santana et al., 2022). Studies have shown that SNPs in the FADS1 and FADS2 genes [such as rs174545, rs174546, rs174548, and rs174553 (FADS1); rs1535 and rs174583 (FADS2)] can affect the bioavailability of omega-3 and omega-6 PUFAs in various tissues. They can also increase plasma levels of LA and ALA, and decrease levels of ARA, EPA, and DHA (Steer et al., 2013; de la Garza Puentes et al., 2017). Our study revealed rs174555 (FADS1, FADS2) had a two-sided effect on chalazion risk, being associated with the ratio of bisallylic groups to double bonds (1.298 [95% CI, 1.145–1.471], PPH4 = 0.817) and 18:2, linoleic acid (LA, 0.827 [95% CI, 0.720–0.950], PPH4 = 0.870) metabolism. This suggests that rs174555 (FADS1, FADS2) as a drug target related to fatty acid metabolism needs to be considered carefully for the presence of bidirectional effects.
Acetone was associated with a higher chalazion risk. It is a metabolite of fatty acids (Møller, 2020), and we hypothesised that increased fatty acid levels would increase the risk of chalazion and may be partly related to the fatty acid metabolite acetone. However, further research is required to verify these hypotheses. A higher average number of methylene groups per double bond reduces the risk of chalazion formation. Studies have shown that methylene groups can increase the lipophilicity of compounds (Møller, 2020), and in addition, methylene groups can act as methylating agents, playing a key role in DNA methylation (Botezatu et al., 2014). Additionally, methylene groups are involved in the synthesis of many amino acids and biologically active molecules as well as in metabolic processes (Stocke and Lamont, 2023).
However, it is essential to acknowledge the primary limitation of this MR analysis: First, the study was based exclusively on patients with chalazion of European origin, which limits its generalisability to other ethnic populations. Further testing of GWAS for metabolites and chalazions in other populations would facilitate larger MR analyses. Second, although we did not observe multidirectional evidence of causal associations across the MR methods, the variants used in MR may confer a risk for chalazion development through multidirectional pathways. Further MR analyses using individual-level data should be performed to assess the potential dose-response causality between individual metabolites and chalazion risk. Meanwhile, further studies are required to elucidate the potential mechanisms underlying these findings.
This study offers evidence supporting the role of alanine in providing protection against chalazion, suggesting its significance as a potential metabolite in chalazion treatment. We also suggest that the degree of unsaturation in circulating fatty acids is a prominent factor in the development of chalazion, highlighting the need for further investigation into the mechanisms of omega-3 and omega-6 fatty acids in this context.
Data availability statement
The original contributions presented in the study are included in the article/Supplementary Material, further inquiries can be directed to the corresponding authors.
Author contributions
XZ: Data curation, Methodology, Writing–original draft. YuC: Data curation, Methodology, Writing–original draft. YJ: Methodology, Writing–original draft. WD: Writing–original draft, Data curation. WA: Writing–original draft. QF: Supervision, Validation, Visualization, Writing–review and editing. YiC: Conceptualization, Funding acquisition, Supervision, Writing–review and editing.
Funding
The authors declare financial support was received for the research, authorship, and/or publication of this article. This research was supported by the National Natural Science Foundation of China (82271050).
Acknowledgments
We thank all the researchers and participants for making the GWAS summary data publicly available.
Conflict of interest
The authors declare that the research was conducted in the absence of any commercial or financial relationships that could be construed as a potential conflict of interest.
Publisher’s note
All claims expressed in this article are solely those of the authors and do not necessarily represent those of their affiliated organizations, or those of the publisher, the editors and the reviewers. Any product that may be evaluated in this article, or claim that may be made by its manufacturer, is not guaranteed or endorsed by the publisher.
Supplementary material
The Supplementary Material for this article can be found online at: https://www.frontiersin.org/articles/10.3389/fmolb.2024.1368669/full#supplementary-material
SUPPLEMENTARY FIGURE S1 | Leave-one-out tests for 13 metabolites on chalazion in two MR analysis (except for Pyruvate). Primary analysis (A–G): (A) alanine; (B) acetone; (C) degree of unsaturation; (D) ratio of omega-3 fatty acids to total fatty acids; (E) ratio of omega-6 fatty acids to omega-3 fatty acids; (F) ratio of polyunsaturated fatty acids to total fatty acids; (G) ratio of docosahexaenoic acid to total fatty acids. Secondary analysis (H–M): (H) alanine; (I) average number of methylene groups per double bond; (J) average number of double bonds in a fatty acid chain; (K) ratio of bisallylic groups to double bonds; (L) ratio of bisallylic groups to total fatty acids; (M) 18:2, linoleic acid (LA).
SUPPLEMENTARY FIGURE S2 | Colocalization analyses for 14 metabolites on chalazion in two MR analysis. Primary analysis (A–G): (A) alanine; (B) acetone; (C) degree of unsaturation; (D) ratio of omega-3 fatty acids to total fatty acids; (E) ratio of omega-6 fatty acids to omega-3 fatty acids; (F) ratio of polyunsaturated fatty acids to total fatty acids; (G) ratio of docosahexaenoic acid to total fatty acids. Secondary analysis (H–N): (H) alanine; (I) average number of methylene groups per double bond; (J) average number of double bonds in a fatty acid chain; (K) ratio of bisallylic groups to double bonds; (L) ratio of bisallylic groups to total fatty acids; (M) 18:2, linoleic acid (LA); (N) pyruvate.
References
Agudo-Barriuso, M., Lahoz, A., Nadal-Nicolás, F. M., Sobrado-Calvo, P., Piquer-Gil, M., Díaz-Llopis, M., et al. (2013). Metabolomic changes in the rat retina after optic nerve crush. Invest. Ophthalmol. Vis. Sci. 54 (6), 4249–4259. doi:10.1167/iovs.12-11451
Balić, A., Vlašić, D., Žužul, K., Marinović, B., and Bukvić Mokos, Z. (2020). Omega-3 versus omega-6 polyunsaturated fatty acids in the prevention and treatment of inflammatory skin diseases. Int. J. Mol. Sci. 21 (3), 741. doi:10.3390/ijms21030741
Botezatu, A., Socolov, R., Socolov, D., Iancu, I. V., and Anton, G. (2014). Methylation pattern of methylene tetrahydrofolate reductase and small nuclear ribonucleoprotein polypeptide N promoters in oligoasthenospermia: a case-control study. Reprod. Biomed. Online 28 (2), 225–231. doi:10.1016/j.rbmo.2013.10.010
Bowden, J., Davey Smith, G., and Burgess, S. (2015). Mendelian randomization with invalid instruments: effect estimation and bias detection through Egger regression. Int. J. Epidemiol. 44 (2), 512–525. doi:10.1093/ije/dyv080
Burgess, S., Bowden, J., Fall, T., Ingelsson, E., and Thompson, S. G. (2017). Sensitivity analyses for robust causal inference from mendelian randomization analyses with multiple genetic variants. Epidemiology 28 (1), 30–42. doi:10.1097/EDE.0000000000000559
Burgess, S., Butterworth, A., and Thompson, S. G. (2013). Mendelian randomization analysis with multiple genetic variants using summarized data. Genet. Epidemiol. 37 (7), 658–665. doi:10.1002/gepi.21758
Burgess, S., Davey Smith, G., Davies, N. M., Dudbridge, F., Gill, D., Glymour, M. M., et al. (2019). Guidelines for performing Mendelian randomization investigations: update for summer 2023. Wellcome Open Res. 4, 186. doi:10.12688/wellcomeopenres.15555.2
Burgess, S., Small, D. S., and Thompson, S. G. (2017). A review of instrumental variable estimators for Mendelian randomization. Stat. Methods Med. Res. 26 (5), 2333–2355. doi:10.1177/0962280215597579
Burgess, S., and Thompson, S. G. (2011). Bias in causal estimates from Mendelian randomization studies with weak instruments. Stat. Med. 30 (11), 1312–1323. doi:10.1002/sim.4197
Burgess, S., and Thompson, S. G.CRP CHD Genetics Collaboration (2011). Avoiding bias from weak instruments in Mendelian randomization studies. Int. J. Epidemiol. 40 (3), 755–764. doi:10.1093/ije/dyr036
Cheng, H., Lv, X., Yao, J., and Chen, Z. (2022). Clinical report: correlation of serum vitamins and chalazion. Optom. Vis. Sci. 99 (6), 540–543. doi:10.1097/OPX.0000000000001887
Chistyakov, D. V., Filimonov, I. S., Azbukina, N. V., Goriainov, S. V., Chistyakov, V. V., Fomich, M. A., et al. (2018). Deuterated arachidonic acids library for regulation of inflammation and controlled synthesis of eicosanoids: an in vitro study. Molecules 23 (12), 3331. doi:10.3390/molecules23123331
Cortina, M. S., and Bazan, H. E. (2011). Docosahexaenoic acid, protectins and dry eye. Curr. Opin. Clin. Nutr. Metab. Care 14 (2), 132–137. doi:10.1097/MCO.0b013e328342bb1a
Davey Smith, G., and Ebrahim, S. (2005). What can mendelian randomisation tell us about modifiable behavioural and environmental exposures? BMJ 330 (7499), 1076–1079. doi:10.1136/bmj.330.7499.1076
de la Garza Puentes, A., Montes Goyanes, R., Chisaguano Tonato, A. M., Torres-Espínola, F. J., Arias García, M., de Almeida, L., et al. (2017). Association of maternal weight with FADS and ELOVL genetic variants and fatty acid levels- the PREOBE follow-up. PLoS One 12 (6), e0179135. doi:10.1371/journal.pone.0179135
Feng, K. M., Chung, C. H., Chen, Y. H., Chien, W. C., and Chien, K. H. (2022). Statin use is associated with a lower risk of blepharitis: a population-based study. Front. Med. (Lausanne) 9, 820119. doi:10.3389/fmed.2022.820119
Georgakopoulos, C. D., Makri, O. E., Pagoulatos, D., Vasilakis, P., Peristeropoulou, P., Kouli, V., et al. (2017). Effect of omega-3 fatty acids dietary supplementation on ocular surface and tear film in diabetic patients with dry eye. J. Am. Coll. Nutr. 36 (1), 38–43. doi:10.1080/07315724.2016.1170643
Giambartolomei, C., Vukcevic, D., Schadt, E. E., Franke, L., Hingorani, A. D., Wallace, C., et al. (2014). Bayesian test for colocalisation between pairs of genetic association studies using summary statistics. PLoS Genet. 10 (5), e1004383. doi:10.1371/journal.pgen.1004383
Hamuro, J., Numa, K., Fujita, T., Toda, M., Ueda, K., Tokuda, Y., et al. (2020). Metabolites interrogation in cell fate decision of cultured human corneal endothelial cells. Invest. Ophthalmol. Vis. Sci. 61 (2), 10. doi:10.1167/iovs.61.2.10
Han, Y., Jia, Y., Tian, J., Zhou, S., Chen, A., and Luo, X. (2020). Urine metabolomic responses to aerobic and resistance training in rats under chronic unpredictable mild stress. PLoS One 15 (8), e0237377. doi:10.1371/journal.pone.0237377
Hemani, G., Tilling, K., and Davey Smith, G. (2017). Orienting the causal relationship between imprecisely measured traits using GWAS summary data. PLoS Genet. 13 (11), e1007081. doi:10.1371/journal.pgen.1007081
Ilhan, C. (2022). Serum levels of thyroid hormone, vitamin B12, vitamin D3, folic acid, and ferritin in chalazion. Ocul. Immunol. Inflamm. 30 (4), 776–780. doi:10.1080/09273948.2020.1828490
Kettunen, J., Demirkan, A., Würtz, P., Draisma, H. H. M., Haller, T., Rawal, R., et al. (2016). Genome-wide study for circulating metabolites identifies 62 loci and reveals novel systemic effects of LPA. Nat. Commun. 7, 11122. doi:10.1038/ncomms11122
Kim, D. H., Briceño, C. A., McGeehan, B., and VanderBeek, B. L. (2023). Risk factors for chalazion diagnosis and subsequent surgical excision. Ophthalmic Epidemiol., 31 1–7. doi:10.1080/09286586.2023.2199838
Knop, N., and Knop, E. (2009). Meibomian glands. Part I: anatomy, embryology and histology of the Meibomian glands. Ophthalmologe 106 (10), 872–883. doi:10.1007/s00347-009-2006-1
Lawlor, D. A., Harbord, R. M., Sterne, J. A., Timpson, N., and Davey Smith, G. (2008). Mendelian randomization: using genes as instruments for making causal inferences in epidemiology. Stat. Med. 27 (8), 1133–1163. doi:10.1002/sim.3034
Levin, M. G., Judy, R., Gill, D., Vujkovic, M., Verma, S. S., Bradford, Y., et al. (2020). Genetics of height and risk of atrial fibrillation: a Mendelian randomization study. PLoS Med. 17 (10), e1003288. doi:10.1371/journal.pmed.1003288
Liang, L., Ding, X., and Tseng, S. C. (2014). High prevalence of demodex brevis infestation in chalazia. Am. J. Ophthalmol. 157 (2), 342–348. doi:10.1016/j.ajo.2013.09.031
Liu, B., Gloudemans, M. J., Rao, A. S., Ingelsson, E., and Montgomery, S. B. (2019). Abundant associations with gene expression complicate GWAS follow-up. Nat. Genet. 51 (5), 768–769. doi:10.1038/s41588-019-0404-0
Malekahmadi, M., Farrahi, F., and Tajdini, A. (2017). Serum vitamin A levels in patients with chalazion. Med. Hypothesis Discov. Innov. Ophthalmol. 6 (3), 63–66.
Mamtimin, B., Hizbulla, M., Kurbantay, N., You, L., Yan, X., and Upur, H. (2014). An magnetic resonance-based plasma metabonomic investigation on abnormal Savda in different complicated diseases. J. Tradit. Chin. Med. 34 (2), 166–172. doi:10.1016/s0254-6272(14)60073-x
Melo, R. C., D'Avila, H., Wan, H. C., Bozza, P. T., Dvorak, A. M., and Weller, P. F. (2011). Lipid bodies in inflammatory cells: structure, function, and current imaging techniques. J. Histochem Cytochem 59 (5), 540–556. doi:10.1369/0022155411404073
Mi, J., Jiang, L., Liu, Z., Wu, X., Zhao, N., Wang, Y., et al. (2022). Identification of blood metabolites linked to the risk of cholelithiasis: a comprehensive Mendelian randomization study. Hepatol. Int. 16 (6), 1484–1493. doi:10.1007/s12072-022-10360-5
Møller, N. (2020). Ketone body, 3-hydroxybutyrate: minor metabolite - major medical manifestations. J. Clin. Endocrinol. Metab. 105 (9), dgaa370. doi:10.1210/clinem/dgaa370
Morvan, D., and Demidem, A. (2007). Metabolomics by proton nuclear magnetic resonance spectroscopy of the response to chloroethylnitrosourea reveals drug efficacy and tumor adaptive metabolic pathways. Cancer Res. 67 (5), 2150–2159. doi:10.1158/0008-5472.CAN-06-2346
Mudgil, P. (2020). Evaluation of use of essential fatty acids in topical ophthalmic preparations for dry eye. Ocul. Surf. 18 (1), 74–79. doi:10.1016/j.jtos.2019.10.001
Nemet, A. Y., Vinker, S., and Kaiserman, I. (2011). Associated morbidity of chalazia. Cornea 30 (12), 1376–1381. doi:10.1097/ICO.0b013e31821de36f
Palmer, T. M., Lawlor, D. A., Harbord, R. M., Sheehan, N. A., Tobias, J. H., Timpson, N. J., et al. (2012). Using multiple genetic variants as instrumental variables for modifiable risk factors. Stat. Methods Med. Res. 21 (3), 223–242. doi:10.1177/0962280210394459
Patel, S., Tohme, N., Gorrin, E., Kumar, N., Goldhagen, B., and Galor, A. (2022). Prevalence and risk factors for chalazion in an older veteran population. Br. J. Ophthalmol. 106 (9), 1200–1205. doi:10.1136/bjophthalmol-2020-318420
Peng, L. A., Schousboe, A., and Hertz, L. (1991). Utilization of alpha-ketoglutarate as a precursor for transmitter glutamate in cultured cerebellar granule cells. Neurochem. Res. 16 (1), 29–34. doi:10.1007/BF00965824
Prochownik, E. V., and Wang, H. (2021). The metabolic fates of pyruvate in normal and neoplastic cells. Cells 10 (4), 762. doi:10.3390/cells10040762
Rueda, E. M., Johnson, J. E., Giddabasappa, A., Swaroop, A., Brooks, M. J., Sigel, I., et al. (2016). The cellular and compartmental profile of mouse retinal glycolysis, tricarboxylic acid cycle, oxidative phosphorylation, and ∼P transferring kinases. Mol. Vis. 22, 847–885.
Santana, J. D. M., Pereira, M., Carvalho, G. Q., Gouveia Peluzio, M. d. C., Drumond Louro, I., Santos, D. B. D., et al. (2022). FADS1 and FADS2 gene polymorphisms modulate the relationship of omega-3 and omega-6 fatty acid plasma concentrations in gestational weight gain: a nisami cohort study. Nutrients 14 (5), 1056. doi:10.3390/nu14051056
Saravanan, M., Xu, R., Roby, O., Wang, Y., Zhu, S., Lu, A., et al. (2023). Tissue-specific sex difference in mouse eye and brain metabolome under fed and fasted states. Invest. Ophthalmol. Vis. Sci. 64 (3), 18. doi:10.1167/iovs.64.3.18
Schousboe, A., Sonnewald, U., and Waagepetersen, H. S. (2003). Differential roles of alanine in GABAergic and glutamatergic neurons. Neurochem. Int. 43 (4-5), 311–315. doi:10.1016/s0197-0186(03)00017-2
Staley, J. R., Blackshaw, J., Kamat, M. A., Ellis, S., Surendran, P., Sun, B. B., et al. (2016). PhenoScanner: a database of human genotype-phenotype associations. Bioinformatics 32 (20), 3207–3209. doi:10.1093/bioinformatics/btw373
Steer, C. D., Lattka, E., Koletzko, B., Golding, J., and Hibbeln, J. R. (2013). Maternal fatty acids in pregnancy, FADS polymorphisms, and child intelligence quotient at 8 y of age. Am. J. Clin. Nutr. 98 (6), 1575–1582. doi:10.3945/ajcn.112.051524
Stocke, K. S., and Lamont, R. J. (2023). One-carbon metabolism and microbial pathogenicity. Mol. Oral Microbiol. doi:10.1111/omi.12417
Venkateswaran, S., Prince, J., Cutler, D. J., Marigorta, U. M., Okou, D. T., Prahalad, S., et al. (2018). Enhanced contribution of HLA in pediatric onset ulcerative colitis. Inflamm. Bowel Dis. 24 (4), 829–838. doi:10.1093/ibd/izx084
Wagner, B. A., Buettner, G. R., and Burns, C. P. (1994). Free radical-mediated lipid peroxidation in cells: oxidizability is a function of cell lipid bis-allylic hydrogen content. Biochemistry 33 (15), 4449–4453. doi:10.1021/bi00181a003
Wojtowicz, J. C., Butovich, I. A., McMahon, A., Hogan, R. N., Itani, K. M., Mancini, R., et al. (2014). Time-dependent degenerative transformations in the lipidome of chalazia. Exp. Eye Res. 127, 261–269. doi:10.1016/j.exer.2014.08.008
Yamaguchi, T., Sugimura, R., Shimajiri, J., Suda, M., Abe, M., Hosokawa, M., et al. (2012). Oxidative stability of glyceroglycolipids containing polyunsaturated fatty acids. J. Oleo Sci. 61 (9), 505–513. doi:10.5650/jos.61.505
Keywords: eyelid lesion, chalazion, circulating metabolites, alanine, fatty acids, Mendelian randomization
Citation: Zhang X, Cai Y, Jiang Y, Du W, An W, Fu Q and Chen Y (2024) Genetic correlation between circulating metabolites and chalazion: a two-sample Mendelian randomization study. Front. Mol. Biosci. 11:1368669. doi: 10.3389/fmolb.2024.1368669
Received: 19 January 2024; Accepted: 06 March 2024;
Published: 21 March 2024.
Edited by:
Xavier Domingo-Almenara, Eurecat, SpainReviewed by:
Jack Pattee, University of Colorado Anschutz Medical Campus, United StatesEvangelia Sarandi, University of Crete, Greece
Copyright © 2024 Zhang, Cai, Jiang, Du, An, Fu and Chen. This is an open-access article distributed under the terms of the Creative Commons Attribution License (CC BY). The use, distribution or reproduction in other forums is permitted, provided the original author(s) and the copyright owner(s) are credited and that the original publication in this journal is cited, in accordance with accepted academic practice. No use, distribution or reproduction is permitted which does not comply with these terms.
*Correspondence: Qiangqiang Fu, qiangqiang.fu@tongji.edu.cn; Yihui Chen, 1300089@tongji.edu.cn
†These authors have contributed equally to this work and share first authorship