Effect of Aging on Motor Inhibition during Action Preparation under Sensory Conflict
- 1Institute of Neuroscience, Université catholique de Louvain, Brussels, Belgium
- 2Movement Control and Neuroplasticity Research Group, Group Biomedical Sciences, KU Leuven, Leuven, Belgium
Motor behaviors often require refraining from selecting options that may be part of the repertoire of natural response tendencies but that are in conflict with ongoing goals. The presence of sensory conflict has a behavioral cost but the latter can be attenuated in contexts where control processes are recruited because conflict is expected in advance, producing a behavioral gain compared to contexts where conflict occurs in a less predictable way. In the present study, we investigated the corticospinal correlates of these behavioral effects (both conflict-driven cost and context-related gain). To do so, we measured motor-evoked potentials (MEPs) elicited by transcranial magnetic stimulation (TMS) over the primary motor cortex (M1) of young and healthy older adults performing the Eriksen Flanker Task. Subjects performed button-presses according to a central arrow, flanked by irrelevant arrows pointing in the same (congruent trial) or opposite direction (incongruent trial). Conflict expectation was manipulated by changing the probability of congruent and incongruent trials in a given block. It was either high (mostly incongruent blocks, MIB, 80% incongruent trials) or low (mostly congruent blocks, MCB, 80% congruent). The MEP data indicate that the conflict-driven behavioral cost is associated with a strong increase in inappropriate motor activity regardless of the age of individuals, as revealed by larger MEPs in the non-responding muscle in incongruent than in congruent trials. However, this aberrant facilitation disappeared in both groups of subjects when conflict could be anticipated (i.e., in the MIBs) compared to when it occurred in a less predictably way (MCBs), probably allowing the behavioral gain observed in both the young and the older individuals. Hence, the ability to overcome and anticipate conflict was surprisingly preserved in the older adults. Nevertheless, some control processes are likely to evolve with age because the behavioral gain observed in the MIB context was associated with an attenuated suppression of MEPs at the time of the imperative signal (i.e., before conflict is actually detected) in older individuals, suggesting altered motor inhibition, compared to young individuals. In addition, the behavioral analysis suggests that young and older adults rely on different strategies to cope with conflict, including a change in speed-accuracy tradeoff.
Introduction
Human beings are often faced with a multitude of stimuli in front of which they need to decide how to behave (Oliveira et al., 2010; Doya and Shadlen, 2012; Klein et al., 2012; Thura and Cisek, 2014; Zénon et al., 2015; Derosiere et al., 2016). In this context, irrelevant stimuli can occasionally induce a powerful activation of action representations that are not consistent with the ongoing goals. This can happen when the irrelevant stimuli are particularly salient or because the inappropriate actions are part of the intrinsic response repertoire, sometimes even more than the relevant options (Praamstra et al., 1998; Mattler, 2003; Taylor et al., 2007; Chen et al., 2009; Mars et al., 2009; Michelet et al., 2010). Under these circumstances, the goal-orientated and inappropriate actions are in “conflict”, producing a behavioral cost as evidenced by the prolonged time usually needed to deliver the correct response and the reduced accuracy (Takezawa and Miyatani, 2005; Hughes and Yeung, 2011; Duprez et al., 2016). Conflict resolution is thought to rely on the recruitment of a set of areas in the frontal cortex; the engagement of this cognitive control network would depend on the degree to which conflict is expected in advance (Botvinick et al., 1999; Siegel et al., 2011; Young and Shapiro, 2011; Grandjean et al., 2012; King et al., 2012; Cohen and Ridderinkhof, 2013; Zmigrod et al., 2016).
In a recent study (Klein et al., 2014), rather than focusing on cognitive control networks, we investigated the impact of their recruitment on the activity of the motor output system. To do so, we considered motor-evoked potentials (MEPs) elicited by transcranial magnetic stimulation (TMS) over the primary motor cortex (M1) of young adults performing an arrow-based version of the Eriksen Flanker Task (Eriksen and Eriksen, 1974). Several observations were made. Behaviorally speaking, we observed that subjects were more proficient in resolving conflict when it could be anticipated compared to when it occurred unpredictably, consistent with many previous reports and with the view that additional control processes are recruited to assist competition resolution in the former situation (Zmigrod et al., 2016). Interestingly, the MEP data revealed that this behavioral gain was associated with a strengthened suppression of motor activity at the onset time of the imperative signal. That is, although MEPs were systematically suppressed at this time, as frequently reported in the past (Klein et al., 2012, 2016; Greenhouse et al., 2015b; Bestmann and Duque, 2016; Quoilin et al., 2016; Wilhelm et al., 2016), this effect was much stronger when conflict was expected than when it was not. Besides, we also found that, during actions selection, the motor representations were less affected by the presence of irrelevant distractors. That is, MEPs elicited after the imperative signal, in a muscle controlling an inappropriate action, typically show some temporary facilitatory changes in the presence of information calling erroneously for that movement (Michelet et al., 2010; van Campen et al., 2014), yet this effect was reduced when conflict could be predicted in advance. Hence, in young subjects, control processes recruited to deal more proficiently with conflict seem to involve, on the one hand, a strengthening of inhibitory influences directed at motor representations before action selection begins (i.e., before conflict is actually detected in the imperative signal) and, on the other hand, a reduced influence of conflicting irrelevant information on corticospinal activity, possibly through an enhanced motor inhibition, during action selection.
Aging is often associated with a decline in various executive functions (Hedden and Gabrieli, 2004; Gazzaley and D’Esposito, 2007; Levin and Netz, 2015) including memory, attention, reasoning abilities and inhibitory control (Fujiyama et al., 2012; Cuypers et al., 2013; Levin et al., 2014; Stewart et al., 2014; Bönstrup et al., 2015; McNab et al., 2015; Smittenaar et al., 2015; Cid-Fernández et al., 2016; Kleerekooper et al., 2016). Several hypotheses have been proposed emphasizing the possible relationship between these deficits and a progressive degeneration of the prefrontal cortex (Hedden and Gabrieli, 2004; Gazzaley et al., 2008). In addition and especially relevant to the current issue, previous studies have reported that the aging population displays a reduced ability to make appropriate decisions and to choose between conflicting alternatives (Vallesi and Stuss, 2010; Korsch et al., 2014; Marshall et al., 2016), a function that requires a tight interaction between cognition and action and thus also relies on prefrontal functioning (Hedden and Gabrieli, 2004, 2005). Interestingly, it has been proposed that the aging brain progressively loses its capacity to suppress irrelevant information (Eriksen and Eriksen, 1974; Radvansky et al., 2005; Lucci et al., 2013; Korsch et al., 2016), resulting in an overflow of inappropriate neural activity which in turn, interferes with the processing of pertinent information (Gazzaley et al., 2008; Zhu et al., 2010). Such a deficit is likely to also strongly impact on decisional abilities, especially in highly demanding tasks.
The goal of the present study was to investigate how older adults cope with conflict in the context of visuomotor decisions. We used the arrow-based version of the Eriksen Flanker Task to characterize: (1) the behavioral cost associated with the presence of sensory distractors; and (2) the behavioral gain occurring in contexts when conflict can be anticipated, as observed in young individuals (Klein et al., 2014). We investigated the corticospinal aspects of these behavioral effects by measuring MEPs at specific time epochs during action preparation, with an emphasis on: (1) the MEP correlates of the conflict-driven behavioral cost; and (2) the MEP correlates of the context-related behavioral gain, focusing on motor inhibitory changes occurring before and during action selection (i.e., before and after detection of the sensory conflict), in young and older adults.
Materials and Methods
Participants
Data were collected on 12 young (7 women, mean age = 24 ± 2.1 years old) and 19 older (12 women, mean age = 71 ± 1.5 years old) healthy adults. Some findings obtained from the young adults were reported in a previous article (Klein et al., 2014). None of the participants had any neurological disorder, history of psychiatric illness, drug or alcohol abuse. The older adults were all fairly active in their everyday life and had no major physical disability. Their cognitive abilities were assessed using the Mini-Mental State Examination (MMSE; Folstein et al., 1975); the presence of depression or anxiety was evaluated by means of the Hospital Anxiety and Depression scale (HAD; Hamilton, 1960). All subjects were right-handed, except for one older adult, according to the condensed version of the Edinburgh Handedness Inventory (Oldfield, 1971) and were financially compensated for their participation. A description of the older participants is provided in Table 1. Participants were all naive to the purpose of the study. The protocol was approved by the Ethics Committee of the Université catholique de Louvain (Belgium) and all subjects gave written informed consent for their participation.
Task and Blocks
We used a modified version of the Eriksen flanker Task (Eriksen and Eriksen, 1974; Klein et al., 2014) implemented by means of Matlab 6.5 (The MathWorks, Natick, MA, USA) and the Cogent 2000 toolbox (Functional Imaging Laboratory, Laboratory of Neurobiology and Institute of Cognitive Neuroscience at the Wellcome Department of Imaging Neuroscience, London, UK). Participants were positioned about 60 cm in front of a computer screen. On each trial, they were required to perform a button-press with the left or right index finger according to the orientation of a left- or right-wards arrow (i.e., < or >, respectively) which was presented at the center of the screen. Importantly, this central arrow (called the “target”) was always flanked by a set of two irrelevant arrows on each side (called the “flankers”); the target and flankers either pointed in the same direction (congruent signal, “< < < < <” or “ > > > > >”) or in opposite directions (incongruent signal, “ > > > > >”or “< < >< <”). Hence, subjects performed left or right button-presses in congruent or incongruent trials (see Figure 1A, left side). By adjusting the spatial mapping between the stimuli in our environment and the associated responses (i.e., the stimulus response compatibility), the Eriksen Flanker task represents an interesting vehicle to manipulate response conflict during action selection.
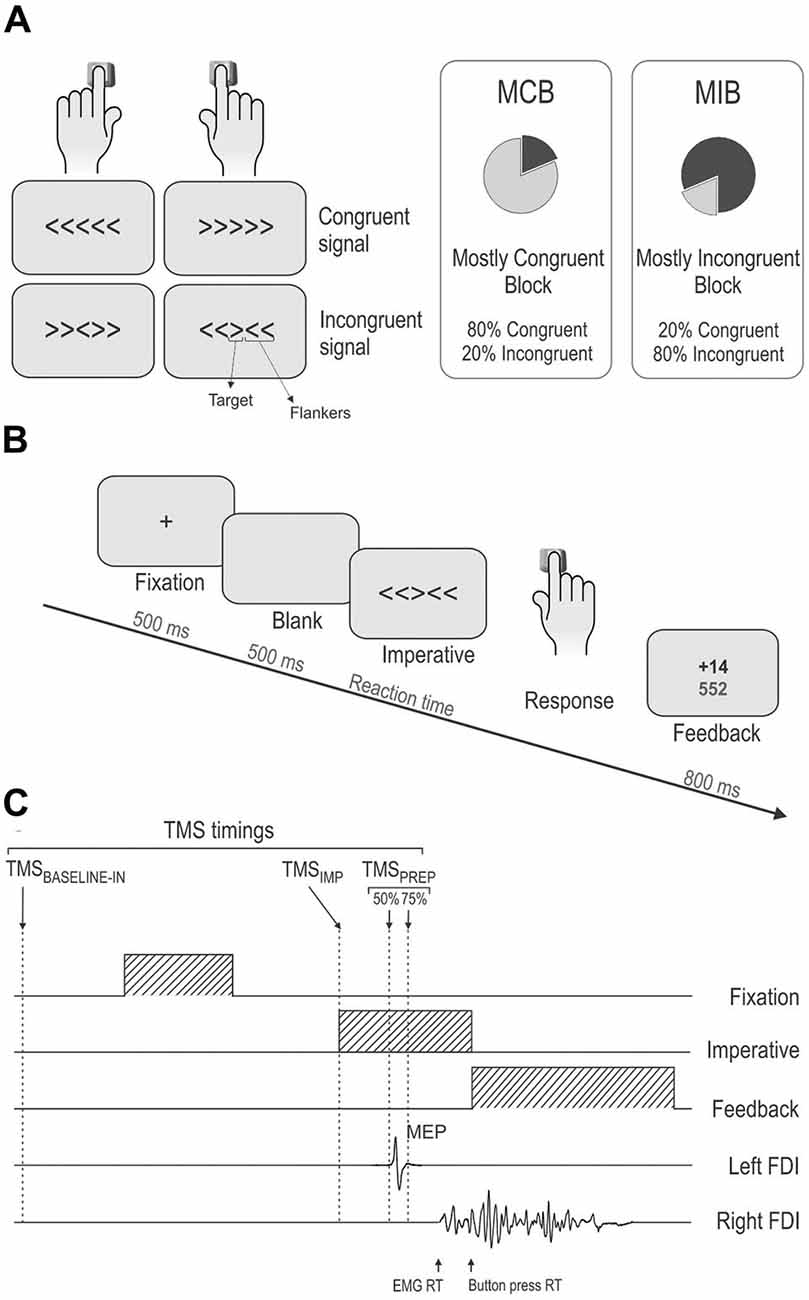
Figure 1. (A) Experimental conditions. Subjects were asked to respond with a left or right button press according to the orientation of a left or right-pointing arrow displayed in the center of the screen. This target arrow was always flanked by a set of two distractor arrows on each side; the target and flankers either pointed in the same direction (congruent signal) or in the opposite direction (incongruent signal). The proportion of congruent and incongruent trials was manipulated within a block to produce the mostly congruent block (MCB) and Mostly Incongruent Block (MIB) contexts. (B) Time course of a trial. Each trial started with a fixation cross. Then, after a blank screen, the imperative signal appeared indicating the required response (right button press in the current example). A visual feedback in the form of a numerical score was displayed after each response. (C) Sequence and TMS timings. A single TMS pulse was applied over the right primary motor cortex (M1) at four possible timings (TMSBASELINE-IN, TMSIMP, TMSPREP50%–75%). FDI, First Dorsal Interosseous; TMS, transcranial magnetic stimulation; MEP, motor evoked potential; PREP, Movement preparation (see “Materials and Methods” Section for the meaning of 50% and 75%); IMP, imperative signal onset.
Top down control was manipulated by adjusting the proportion of congruent and incongruent trials in two types of blocks (see Figure 1A, right side). In the “Mostly Congruent Blocks” (MCB), 80% of trials required subjects to choose a button-press on the basis of a congruent signal; only a few signals were incongruent (20%). The reversed ratio was used in the “Mostly Incongruent Blocks” (MIB) which contained a majority of incongruent signals (80%). Hence, subjects had to overcome conflict on most trials in the latter blocks. Participants were always told about the type of block they would start performing next. The degree to which subjects anticipated conflict was thus clearly different in the MCB and MIB contexts.
Experimental Procedure
The participants sat on a chair with both forearms resting on a pillow in a semi-flexed position and the hands placed palms down on a keyboard. The keyboard was turned upside-down so that subjects could press on the required buttons with the left or right index fingers (keys “F12” and “F5”, respectively).
Each trial started with a central fixation cross (500 ms, Figure 1B) followed, 500 ms later (blank screen), by the imperative signal specifying the required response. The imperative signal consisted of one of the four possible arrangements of target and flankers (“< < < < < ”, “ > > > > >”, “ > > < > >”, “< < > < < ”) and remained on the screen until a button-press was detected or for a maximum duration of 600 ms (400 ms for the young individuals). Reaction times (RTs) were recorded by means of a homemade microcontroller (μC; MSP430F249—Texas Instrument) based system receiving VGA and keyboard events: a timer started on specific VGA events (imperative signal) and stopped on keyboard events (button-press). The μC sent the pressed key code and the timer value (128 μs resolution) to the main computer through a USB interface, providing RT measurements with very high temporal resolution. At the end of each trial, participants received a feedback of their performance (displayed for 800 ms). It consisted of a green-colored positive score following a correct response or a red-colored negative score following an incorrect response. Positive scores were always inversely proportional to the RTs (score = k/RT with k = 5000) whereas the negative scores corresponded to a fixed value (score = −10). The feedback screen also provided the subjects with the total amount of points collected since the beginning of the block; this value was displayed just below the current trial score (see Figure 1B, right side). Participants were told that they would receive a financial bonus according to their final score. The feedback screen was followed by an inter-trial interval ranging from 2400 ms to 2800 ms.
The experiment involved two sessions (one for each block type) performed on different days; the order of the sessions was counterbalanced between participants. Each session always began with two neutral blocks (50% of each trial type) without TMS. The first block served to familiarize the participants with the task whereas the second one was used to compute the individual RTs. This value was used to determine the pre-movement TMS timings within that session (see “Stimulation Procedure” Section below). Then, in the main phase of the experiment, participants performed six blocks (about 6 min each). There was a 5-min break every other block or whenever the subjects felt they needed to rest.
Stimulation Procedure
TMS Location and Intensity
TMS was delivered with a small figure-of-eight coil (wing external diameter 70 mm) connected to a Magstim 200 magnetic stimulator (Magstim, Whitland, Dyfed, UK) and placed tangentially over M1 with the handle pointing backward and laterally at a 45° angle away from the midline, approximately perpendicular to the central sulcus. The optimal scalp position for eliciting an MEP in the left first dorsal interosseous muscle (FDI), so-called the hotspot, was identified and marked on a head cap fitted on the participant’s head to provide a reference landmark throughout the experimental session (Vandermeeren et al., 2009). We focused on a left hand muscle because the motor correlates of inhibitory control processes are thought to be more evident in the non-dominant compared to the dominant hand (Leocani et al., 2000; Duque et al., 2007; Quoilin et al., 2016; Wilhelm et al., 2016), but see also Klein et al. (2016). The resting motor threshold (rMT) was determined at the hotspot as the minimal TMS intensity required to evoke MEPs of about 50 μV peak-to-peak in the relaxed FDI muscle in 5 out of 10 consecutive trials. It was measured at the beginning of each session. Across participants and sessions, the rMT equalled 37 ± 1.9% of the maximum stimulator output (MSO) in young adults (n = 12) and 40 ± 1.6% of the MSO in older individuals (n = 19); these values did not differ significantly between young and older adults. The intensity of TMS was then always set at 120% of the individual rMT.
TMS Timings
In order to evaluate CS excitability changes associated with conflict resolution in young and older adults, we applied TMS at different time epochs during response preparation; only one single TMS pulse was delivered in each trial (see dotted vertical lines in Figure 1C). First, to obtain a baseline of CS excitability, some MEPs were elicited by a TMS pulse applied during the inter-trial interval. This timing of stimulation, referred to as TMSBASELINE-IN, occurred at a random time between 500 ms and 900 ms before the onset of the fixation cross. In older adults, we included a large number of trials with TMS at this timing (20 MEPs/block, 120 MEPs total for each context) in order to obtain a reliable baseline; fewer trials were obtained in young participants (5 MEPs/block, 30 MEPs in total for each context). Second, TMS pulses were also applied between the onset of the imperative signal and the motor response, referred to as the TMSPREP timings. In young adults, there were four different TMSPREP time epochs (see Klein et al., 2014). However, only two time epochs were kept in older adults to reduce the overall duration of the experiment for this group of participants (see rightward vertical dotted lines in Figure 1C). These two time epochs were selected because they were associated with the strongest MEP inhibitory effects in young adults. Hence, in this article, we focused on the comparison of motor inhibitory effects at these two time epochs in young and older adults (less frequent trials [20%] = 2 MEPs/block for each time epoch, 12 MEPs total for each condition in each context; more frequent trials [80%] = 8 MEPs/block for each time epoch, 48 MEPs total for each condition in each context). These time epochs were determined on an individual basis and corresponded respectively to 50% and 75% of the premotor part (66%) of the individual median RT (referred to as TMSPREP−50% and TMSPREP−75%). This RT was measured at the beginning of each experiment in the second no-TMS block (see “Blocks and Sessions” Section above) and correspond to the time elapsed between the onset of the imperative signal and the detection of the button press. The value of 66% of the RT was chosen because it corresponds roughly to the onset of the FDI muscle EMG activity preceding the key press (Klein et al., 2012), also denoted as premotor time. Hence at 50% and 75% of this value, only very few MEPs were located after initiation of EMG activity. The few trials in which the TMS pulse fell after EMG onset were removed from the data set. Finally, TMS pulses could also occur at the onset of the imperative signal (TMSIMP; 5 MEPs/block, 30 MEPs in total for each context). This timing was used to check for a possible effect of conflict expectation on CS excitability, which would occur even before the subjects perceive the information provided by the imperative signal and hence even before they can detect the presence of conflict. Note that all young participants but only 9 out of the 19 older adults received TMS at this time point. This is because in the older participants, the TMSIMP timing was added a posteriori, based on the analysis of the TMSPREP data in the 10 first subjects suggesting that aging may impact on the way conflict expectation influences CS excitability, even before conflict detection. Given the preceding paragraph, in the subjects who received TMS at all timings, each block included 65 trials; in the 10 older adults who did not receive TMSIMP, each block lasted 60 trials. Note that the preceding numbers are provided for the older individuals. Although most numbers are comparable between the two groups, please see Klein et al. (2014) for a full description of blocks and trials in the young adults.
For the analysis of CS excitability during movement preparation, MEPs evoked at the two TMSPREP timings were pooled together (on an individual basis) and sorted according to the actual time between the TMS pulse and the “EMG onset” (estimated at 66% of the time of key-press). We then included all MEPs that resided within a 300 ms to 20 ms time epoch before EMG onset to obtain a single measure of CS excitability preceding movement onset. On average, the MEPs included in this window (MEPsPREP) were located at a comparable time with respect to movement onset in the two block types (177 ms [MCB] and 172 ms [MIB], all F < 1.57, all p > 0.23; see Table 1). MEPsPREP were elicited a little further away from movement onset in incongruent (180 ms) than congruent trials (168 ms; F = 8.1, all p = 0.01) but again this effect was present in both blocks (TRIAL × BLOCK F = 1.48, p = 0.24).
In all the older participants (n = 19) and 8 out of the 12 young adults, we also measured CS excitability outside the blocks by applying 20 TMS pulses (TMSBASELINE-OUT) at three different phases during the experiment (before the first block, after the third block and after the last block). MEPs at TMSBASELINE-OUT were not part of the original paradigm and were thus not acquired in the first 4 young subjects tested in the experiment. MEPs elicited at TMSBASELINE-IN (elicited during the blocks) were compared with those elicited at TMSBASELINE-OUT in order to check for the presence of a global context effect (MIB vs. MCB) on CS excitability.
EMG Recordings
EMG activity was recorded from surface electrodes (Neuroline, Medicotest, Oelstykke, Denmark) placed over the left FDI muscle. EMG data were collected for 2600 ms on each trial, starting 200 ms before the TMS pulse. The EMG signals were amplified and bandpass filtered on-line (10–500 Hz [Neurolog; Digitimer, Hertfordshire, UK]) and digitized at 2000 Hz for off-line analysis. These signals were used to measure the peak-to-peak amplitude of MEPs elicited in the left FDI. Note that at the TMSPREP timings, left FDI MEPs were either elicited preceding a left or right hand response, reflecting thus CS excitability changes associated with a selected or non-selected condition, respectively. In order to prevent contamination of the MEP measurements by significant fluctuations in background EMG Trials, we excluded all trials in which the TMS pulse fell after EMG onset or with any background EMG activity exceeding 100 μV in the 200 ms window preceding the TMS pulse (Duque et al., 2005, 2007; Sartori et al., 2011; Cavallo et al., 2012; Klein et al., 2016; Wilhelm et al., 2016). Finally, trials in which subjects pressed the wrong button were also removed for the MEP analysis. After trimming the data for errors, background EMG activity and outliers, a minimum of 10 MEPs remained to assess CS excitability in each condition.
Statistical Analyses
To characterize behavior, we focused on trials in which TMS was applied during the inter-trial interval (TMSBASELINE-IN). We did so to obtain a “clean” measure of the participants’ performance. As such, in young adults, the TMS pulse has been shown to affect behavior when applied close to movement onset (TMSPREP−50% and TMSPREP−75%) but not at TMSBASELINE-IN. The RTs and %Errors were analyzed using two separate ANOVAs with CONTEXT (MCB, MIB), TRIAL (congruent, incongruent) and HAND (left, right) as within-subject factors and GROUP (young, older) as between-subject factor. We also computed a ratio to capture the effect of having incongruent flankers on the RTs and on the %Errors (Incongruency effect = incongruent trials/congruent trials). For this analysis, we used an ANOVA with CONTEXT (MCB, MIB) and HAND (left, right) as within-subject factors and GROUP (young, older) as between-subject factor.
For the analysis of the MEP data, we first evaluated the impact of the context on baseline CS excitability. To do so, we compared MEPs elicited at TMSBASELINE-IN with those evoked at TMSBASELINE-OUT within the same session (either MCB or MIB). As these MEPs were not normally distributed (Kolmogorov-Smirnov (K-S) test; failed), a logarithmic transformation was applied prior to the statistical tests. The log-transformed MEPs were analyzed using an ANOVA with CONTEXT (MCB, MIB), TMS-TIME (baseline-in, baseline-out) as within-subject factors and GROUP (young, older) as between-subject factor. Note that, as already mentioned above, all older adults (n = 19) but only a subgroup of the young adults (n = 8) received TMSBASELINE-OUT and were thus considered in this analysis. Second, we assessed control processes that are recruited proactively in anticipation of conflict. To do so, we compared the left hand MEPs elicited at TMSIMP (log-transformed) with respect to those elicited at TMSBASELINE-IN in the corresponding context. Because the baseline MEPs were significantly different in the two groups (see “Results” Section), we performed separate ANOVAs for the young (n = 12) and older adults (n = 9) with CONTEXT (MCB, MIB) and TMS-TIME (baseline-in, baseline-out) as within-subject factors. Third, we analyzed CS excitability changes during movement preparation. To do so, left FDI MEPs elicited at TMSPREP were expressed with respect to MEPs (%) elicited at TMSBASELINE-IN within the same session (either MCB or MIB). Hence, MEPs at TMSBASELINE-IN were used as reference for the TMSPREP timings within each session, canceling out any possible context effect on the amplitude of MEPs measured during movement preparation. Again, a logarithmic transformation was applied on these MEPs (%TMSBASELINE-IN) as they were not normally distributed (K-S failed). The log-transformed MEPs were analyzed using a four-way ANOVA with the factors CONTEXT (MCB, MIB), TRIAL (congruent, incongruent) and CONDITION (selected, non-selected) as within-subject factors and GROUP (young, older) as between-subject factor. Finally, similar to the approach used for the analysis of behavior, we computed a ratio reflecting the effect of having incongruent flankers on MEPsPREP (incongruency effect = incongruent/congruent MEPsPREP). These data were analyzed using an ANOVA with CONTEXT (MCB, MIB) and CONDITION (selected, non-selected) as within-subject factors and GROUP (young, older) as between-subject factor. All post hoc comparisons were conducted using the Fisher’s LSD procedure. All of the data are expressed as mean ± SE.
Results
Behavioral Data
Reaction Times
In the young adults (n = 12), the mean RT in congruent trials was 379 ± 12.7 ms and 388 ± 13.8 ms in the MCB and MIB contexts, respectively; the mean RT values for the older adults in these trials were 481 ± 10.1 ms and 488 ± 11.0 ms respectively (n = 19). In incongruent trials, the mean RT was 439 ± 23.3 ms and 418 ± 17.2 ms in the young subjects and 586 ± 18.5 ms and 523 ± 13.7 ms for the older adults, in the MC and MI contexts respectively (Figure 2A, left side).
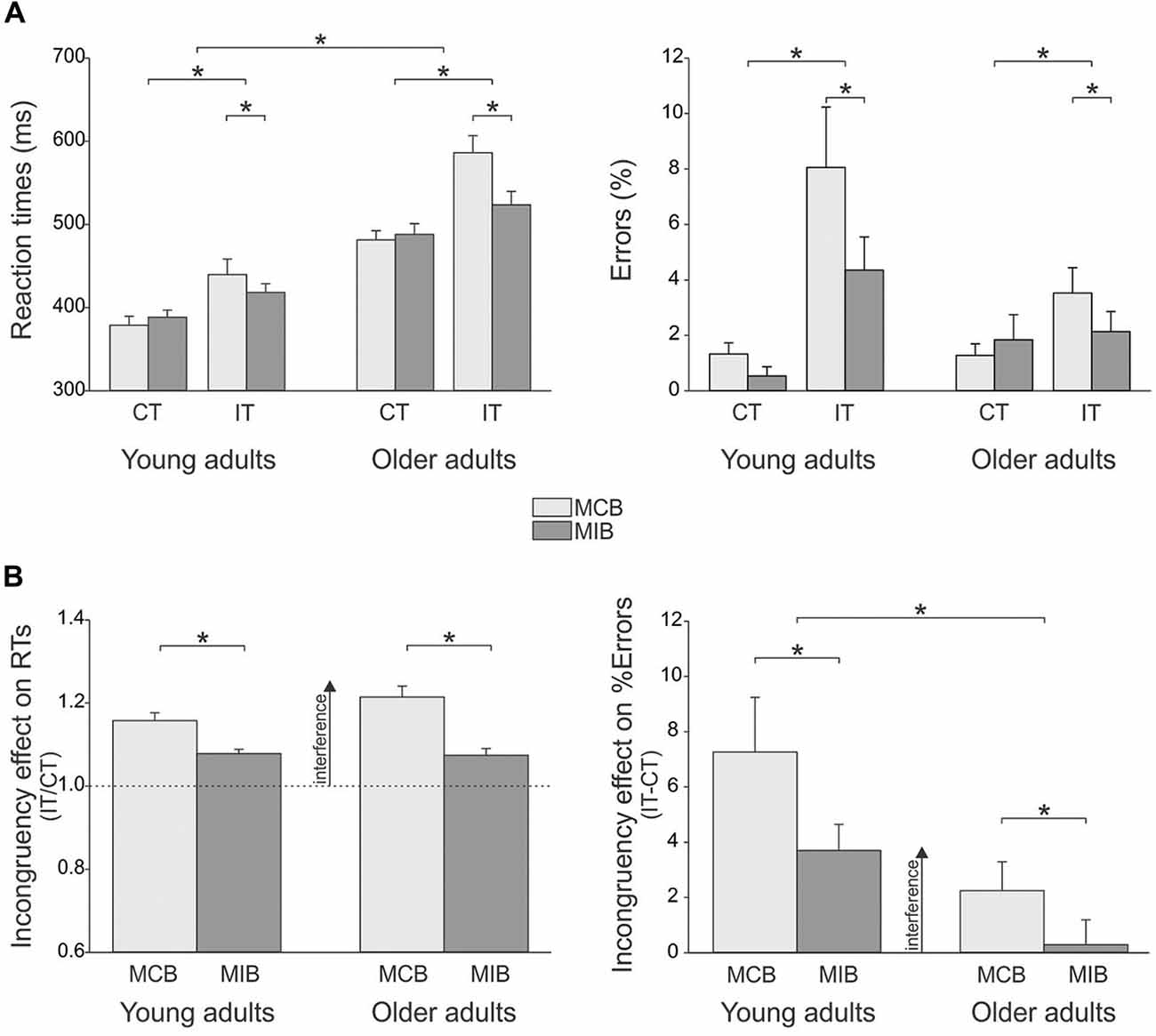
Figure 2. (A) Illustration of the mean (± SE) reaction times (RTs, left side) and error rate (%Errors, right side) for the two types of trials (congruent [CT], incongruent [IT]) in the two contexts (MCB, MIB) in young and older adults. RTs were globally longer in older individuals compared to the young subjects. Yet the older group made less errors in incongruent trials. Both groups displayed shorter RTs and lower %Errors for incongruent trials in MIBs than in MCBs. (B) Incongruency effect (incongruent trials/congruent trials) on RTs (left side) and %Errors (right side). The incongruency effect decreased in both groups in the MIB compared to the MCB context. Note the stronger incongruency effect on the %Errors in the young individuals compared to the older group. *p-value < 0.05.
Overall, young adults were faster than older adults (main effect of GROUP F(1,29) = 36.4, p ≤ 0.0001). The ANOVA also revealed a significant main effect of the factor TRIAL (F(1,29) = 77.7, p ≤ 0.0001): as expected, RTs were generally shorter in congruent (on average 434 ± 7.6 ms) than in incongruent trials (on average 492 ± 11.8 ms). More relevant to the current issue, there was a significant CONTEXT × TRIAL × GROUP interaction (F(1,29) = 6.1, p ≤ 0.02; Figure 2A, left side): RTs were influenced by the context within which the trials were performed but in a way that depended on the compatibility of the target and flanker arrows. Indeed, RTs in incongruent trials were shorter in the MIB context than in the MCB context, both in young (p ≤ 0.02) and older adults (p ≤ 0.0001) whereas RTs in congruent trials were comparable in the two contexts (p > 0.27 in both groups). Hence, both groups showed a specific gain on RTs when incongruent trials were expected, in the MIB context, compared to when they were unlikely, in the MCB context, and this effect was more pronounced in the older than young adults group. The factor HAND was also significant (F(1,29) = 5.3, p ≤ 0.03) and this effect did not depend on the group (HAND × GROUP F(1,29) = 0.3, p ≥ 0.60): both young and older adults were faster when responding with their right (dominant) hand than when providing responses with the left hand (not shown in the Figure).
In order to further compare the effect of conflict on RTs in the two groups, we computed a ratio expressing RTs in incongruent trials with respect to those obtained in congruent trials (incongruency effect, Figure 2B, left side). The ANOVA performed on these data confirmed the occurrence of a main CONTEXT effect (F(1,29) = 48.9, p ≤ 0.0001). That is, the incongruency effect was attenuated in the MCI compared to the MCB context, consistent with the analyses performed on raw RTs. Of note is that the CONTEXT × GROUP interaction was nearly significant (F(1,29) = 3.7, p ≤ 0.064). This trend is due to the fact that in the MCB context, the incongruency effect tended to be larger in the older than in the young adults (p ≤ 0.065), suggesting that the older adults slowed down to a larger degree than young individuals when they were presented with a less expected incongruent signal.
Hence, the analyses performed on the RT data indicate that the older adults were globally slower than the young adults. Besides that, there were no major differences between the two groups of subjects. If anything, the older adults tended to slow down to a larger degree than young subjects when they had to respond to a less expected incongruent signal (in MCB blocks). However, this difference disappeared when incongruent signals were expected (in MIB blocks), revealing a comparable ability to recruit top-down control processes to overcome conflict in the two groups of subjects.
%Errors
In the young adults (n = 12), the mean %Errors in congruent trials was 1.3 ± 0.5% and 0.5 ± 0.9% in the MCB and MIC contexts, respectively; the mean %Error values for the older adults in these trials were 1.3 ± 0.4% and 1.8 ± 0.7%, respectively (n = 19). In incongruent trials, the mean %Errors was 8.1 ± 1.6% and 4.4 ± 1.0% in the young subjects and 3.5 ± 1.3% and 2.1 ± 0.8% for the older adults, in the MCB and MIB contexts respectively. The ANOVA revealed a main effect of the factor TRIAL on these data (F(1,29) = 21.4, p ≤ 0.0001, see Figure 2A, right side). On average, subjects made more errors (higher %Error scores) in incongruent trials (4.5 ± 0.7%) than in congruent trials (1.2 ± 0.3%). In addition, the TRIAL × GROUP interaction was significant (F(1,29) = 5.1, p ≤ 0.03): although the %Error values were comparable between the two groups for congruent trials (p ≥ 0.58), they were higher in young than older adults in incongruent trials (p ≤ 0.004). Finally, as evident in the figure, there was a significant CONTEXT × TRIAL interaction (F(1,29) = 5.1, p ≤ 0.03): the %Error scores were lower in the MIB than in the MCB context for incongruent trials only (p ≤ 0.005; congruent trial p ≥ 0.959). Importantly, this effect was present in both groups (CONTEXT × TRIAL × GROUP F = 0.19, p ≥ 0.662), suggesting that older adults were quite good in attenuating the impact of interfering information when it could be predicted.
Finally, similar to the RT analysis, we considered the incongruency effect (incongruent/congruent %Error score) in the two contexts for the two groups of subjects. We found a significant effect of CONTEXT (F(1,29) = 5.1, p ≤ 0.03) and GROUP (F(1,29) = 8.0, p ≤ 0.008). As expected, the incongruency effect was smaller in the MIB than MCB context. More surprisingly, the incongruency effect was globally smaller in the older than younger individuals, indicating a more pronounced accuracy cost when having to deal with an incongruent signal in the younger group.
Hence, our behavioral data suggest that a main difference between the two groups is a change in the speed-accuracy tradeoff. Young individuals were faster than the older adults but made in general more errors when they had to cope with conflict. Both groups showed a similar ability to enhance conflict resolution when the latter could be predicted.
MEP Data
In the young adults, the mean amplitude of left FDI MEPs elicited during the inter-trial interval (at TMSBASELINE-IN) was 2.34 ± 0.5 mV and 2.26 ± 0.4 mV in the MCB and MIB contexts, respectively; in the older adults, the MEPs at TMSBASELINE-IN equaled 1.41 ± 0.3 mV and 1.18 ± 0.3 mV in the MCB and MIB contexts, respectively. Because the two block types were tested on separate days, the direct comparison of MEPs elicited at TMSBASELINE-IN is not appropriate because it is influenced by several factors that vary between the sessions, including the position of electrodes, the location of TMS coil and the degree of vigilance of the subjects. In contrast, one can compare MEPs obtained at TMSBASELINE-IN to those elicited at TMSBASELINE-OUT (outside the block) within the same session. The ANOVA revealed a significant main effect of the factor GROUP (F(1,25) = 7.4, p ≤ 0.01). That is, baseline MEPs were larger in young than in older adults, although they were elicited at 120% of the individual rMT in both groups. In addition, the MEPs elicited during the block (TMSBASELINE-IN) were generally larger than MEPs elicited outside the block (166.1 ± 29% of MEPs at TMSBASELINE-OUT; F(1,25) = 20.1, p ≤ 0.0001), as already reported in the past (Labruna et al., 2011). However, this facilitation of MEPs was similar for the two contexts and the two groups (CONTEXT × TMS-TIME × GROUP interaction: F(1,7) < 1.12, p ≥ 0.300, see Figure 3A). These results indicate that the level of conflict expectation (higher in MIBs than MCBs) did not impact on baseline CS excitability, neither in the young adults nor in the older individuals.
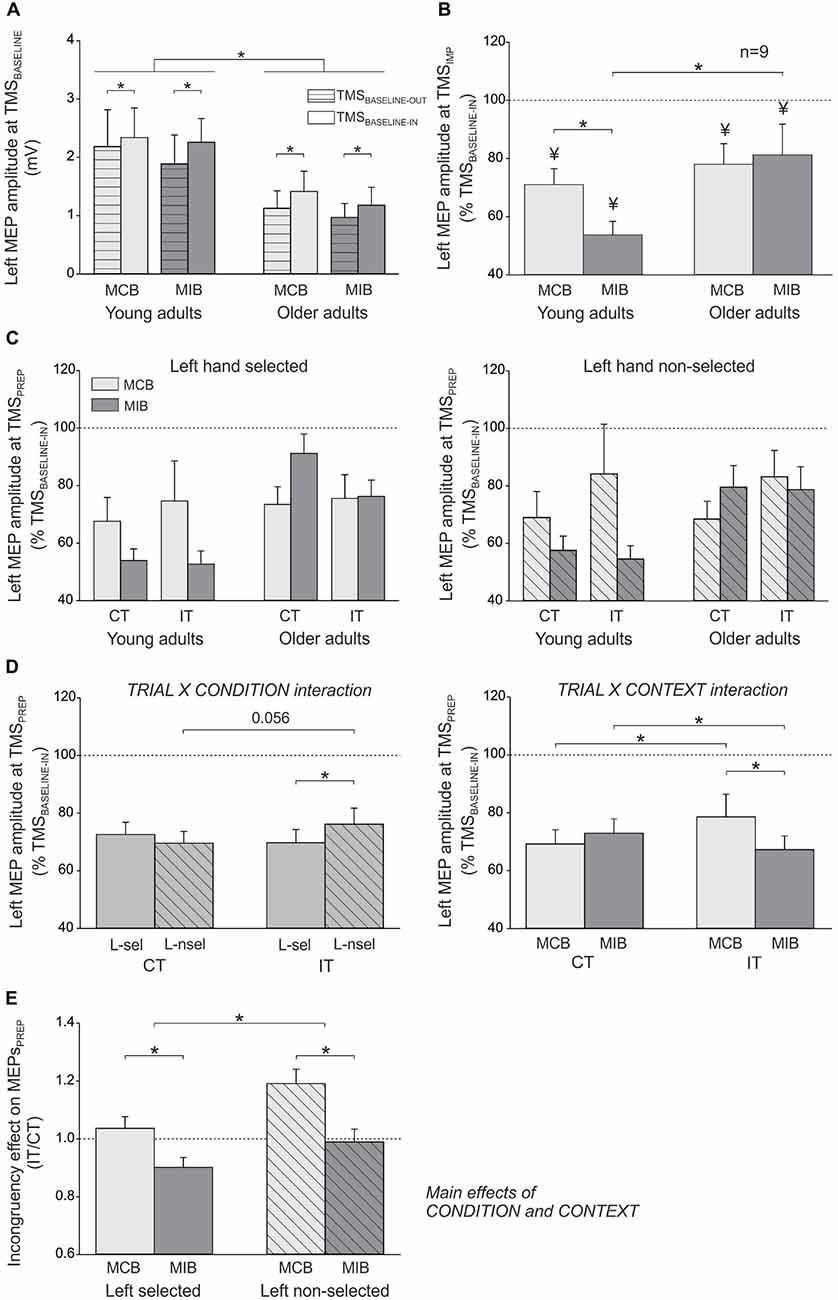
Figure 3. (A) Illustration of the mean (± SE) left FDI MEP amplitude (millivolts, mV) elicited by TMS between the blocks (TMSBASELINE-OUT) or during the inter-trial interval within the blocks (TMSBASELINE-IN) in the two sessions (MCB and MIB contexts) and for both groups of subjects. (B) Left FDI MEPs elicited by TMS at the onset of the imperative signal (TMSIMP, expressed in percentage of TMSBASELINE-IN). (C) Left FDI MEPs elicited by TMS during movement preparation (TMSPREP, expressed in percentage of TMSBASELINE-IN) in congruent trials (CT) and incongruent trials (IT) when the left hand was either selected (left side, L-sel) or non-selected (right side, L-nsel) for the forthcoming response. (D) illustration of the significant TRIAL × CONDITION (left side) and TRIAL × CONTEXT (right side) interactions. (E) Incongruency effect on MEPsPREP (incongruent trials/congruent trials). The incongruency effect was largest in the non-selected condition of the MCB blocks, both in young and older adults. *p-value ≤ 0.05 on all figures. ¥ = significantly different from TMSBASELINE-IN.
In order to assess control processes that were proactively recruited in anticipation of conflict, we compared the left hand MEPs elicited at TMSIMP with respect to those elicited at TMSBASELINE-IN in the corresponding context. In the young participants (n = 12), there was a significant effect of TMS-TIME (F(1,11) = 54.98, p ≤ 0.0001). MEPs were smaller when elicited at TMSIMP compared to baseline, reaching on average 62.4 ± 5.05% of MEPs elicited at TMSBASELINE-IN (see Figure 3B). However, the strength of this MEP suppression depended on the context within which the subjects performed the task (CONTEXT × TMS-TIME interaction F(1,11) = 4.61, p ≤ 0.05). That is, although MEPs were suppressed in both contexts (both p ≤ 0.002), they became smaller in the MIB than the MCB context (p ≤ 0.04). Hence in young adults, at the onset of the imperative signal, MEPs were suppressed to a greater extent when conflict was more expected (MIB context; 53.8 ± 4.61% of MEPs elicited at TMSBASELINE-IN) compared to when conflict was less likely (MCB context; 71 ± 5.48%). In the older adults who received TMSIMP (n = 9), MEPs were also suppressed at the onset of the imperative signal, reaching 79.6 ± 8.84% of the baseline value (F(1,8) = 7.21, p ≤ 0.03). However, here the effect did not depend on the context (CONTEXT × TMS-TIME interaction F(1,8)≤0.001, p ≥ 0.98, see Figure 3B). This finding shows that, contrary to the young adults, the degree of MEP suppression at TMSIMP in older subjects was not modulated by the degree to which they expected conflict to occur. Consistently, the amount of MEP suppression at TMSIMP was comparable between the two groups in the MCB (t19 = 0.71, p ≥ 0.48), but more pronounced in the young than the older individuals for the MIB (t19 = 2.26, p ≤ 0.04). Hence, we did not find any proactive inhibitory effect on MEPs at TMSIMP in the older individuals that could explain their enhanced ability to overcome conflict in the MIB relative to MCB context.
Figure 3C displays the amplitude of MEPs elicited during movement preparation, in each experimental condition (expressed in percentage of TMSBASELINE-IN MEPs), when the left hand had to be selected (left side) or non-selected (right side) for the forthcoming response. These MEPsPREP were elicited relatively far from movement onset (~175 ms before EMG onset, see “Materials and Methods” Section) and were strongly inhibited (below 100%) whether they were elicited in a selected or non-selected condition. This is consistent with our choice to focus on a time epoch at which inhibition was prominent and to compare this inhibition in young and older adults. In the following paragraphs, we report the analyses performed on these data (see Figure 3D).
The ANOVA revealed a significant TRIAL × CONDITION interaction on MEPsPREP (F(1,27) = 6.13, p ≤ 0.02; see Figure 3D, left side). In congruent trials, the left hand MEPs were comparable whether the imperative signal had indicated a left hand response (“< < < < < ”: left hand selected) or a right hand response (“ > > > > >”: left hand non-selected; p ≥ 0.192). Hence, MEPPREP amplitudes do not reflect yet the specific preparation of the required motor response. Interestingly, in incongruent trials, the left hand MEPs were in fact larger when the imperative signal had indicated a right (“< < > < < ”: right hand should be selected but flankers point to the left) than a left hand response (“ > > < > >”: left hand should be selected but flankers point to the right, p ≤ 0.014). This effect did not depend on the group (TRIAL × CONDITION × GROUP interaction F(1,27) = 2.66, p ≥ 0.115) and suggests that the presentation of incongruent flankers substantially modified CS excitability during movement preparation, despite the fact that the task required ignoring these stimuli. Consistently, a comparison between the two trial types revealed that MEPs elicited in a non-selected condition (preceding right hand responses) tended to be larger in incongruent compared with congruent trials (p ≤ 0.056).
The TRIAL × CONTEXT interaction was also significant (F(1,27) = 8.36, p ≤ 0.007; see Figure 3D, right side). When elicited during congruent trials, MEPs were comparable in the MCB and MIB contexts (p ≥ 0.216). However, in incongruent trials, the MEPs were significantly smaller in the MIB compared to the MCB context (p ≤ 0.005). As a consequence, whereas MEPs were found larger in incongruent trials compared to congruent trials in the MCB context (p ≤ 0.042), they were in fact smaller in incongruent trials than congruent trials in the MIB context p≤0.04). Hence, anticipation of conflict seems to allow for the recruitment of a control mechanism that ensures a specific suppression of MEPs when an incongruency between the target and flankers is effectively detected. This effect was observed in both groups (TRIAL × CONTEXT × GROUP interaction F(1,27) = 0.29, p ≥ 0.597) and did not depend on whether the left hand was selected or non-selected (TRIAL × CONTEXT × CONDITION × GROUP interaction F(1,27) = 0.51, p ≥ 0.480).
In order to further investigate the extent to which detection of a conflict influenced CS excitability during movement preparation, we computed ratios of MEPsPREP (incongruent/congruent trials) in the selected and non-selected conditions for the two contexts and in the two groups of subjects (see “Incongruency effect” in Figure 3E). The ANOVA performed on these data revealed a significant effect of the CONDITION (F(1,27) = 6.13, p ≤ 0.02), regardless of the CONTEXT (CONDITION × CONTEXT F(1,27) = 0.65, p ≥ 0.427) and the GROUP (CONDITION × GROUP F(1,27) = 2.66, p ≥ 0.115). This means that in both groups, the incongruency effect was generally larger when MEPsPREP were probed in a non-selected compared to a selected condition. Hence, incongruent flankers increased CS excitability of the (inappropriate) response towards which they pointed. Besides, the ANOVA revealed a main effect of the CONTEXT (F(1,27) = 8.36, p ≤ 0.007), regardless of the GROUP (CONTEXT × GROUP F(1,27) = 0.29, p ≥ 0.597); the triple CONTEXT × CONDITION × GROUP interaction was also not significant (F(1,27) = 0.51, p ≥ 0.480). These results indicate that in both groups, MEPsPREP ratios were smaller in the MIB than in the MCB context, whether they were computed in a selected or non-selected condition. Hence, the incongruency effect was attenuated in both groups when conflict could be foreseen.
In summary, the MEP analyses indicate that visual distractors can lead to a strong increase in inappropriate motor activity regardless of the age of individuals, probably at the origin of the substantial behavioral cost observed in incongruent trials. However, when the presence of sensory distractors could be anticipated (i.e., in MIBs), both groups of subjects were able to attenuate their interfering impact on motor activity, resulting in faster RTs and lower error rates. Nevertheless, the control processes underlying this behavioral gain is likeley to differ between the two groups because the anticipation of conflict was associated with a larger suppression of MEPs at the time of the imperative signal (TMSIMP) in young than in older individuals. In addition, the behavioral data suggests that young and older adults relied on different strategies to cope with conflict, including a change in speed-accuracy tradeoff.
Discussion
Daily life presents us with abundant opportunities for action in a broad variety of contexts. Given the incessant flow of external stimuli, behaving in a goal-oriented manner often requires to refrain from selecting inappropriate stimulus-driven options that may be part of the repertoire of natural response tendencies but that are in conflict with the ongoing goals (Heuninckx et al., 2005; Montague et al., 2006; Gold and Shadlen, 2007). In young adults, the requirement to overcome sensory conflict has a behavioral cost but the latter can be attenuated when the presence of conflict is anticipated in a given context. Our results indicate that the ability to overcome and anticipate conflict is generally preserved in older adults, though the behavioral and MEP data suggest a change in strategy, probably a shift in speed-accuracy tradeoff.
MEP Correlates of Motor Inhibition in Young and Older Adults
Overall, we observed substantial motor inhibition while subjects were involved in the Eriksen Flanker Task. Compared to baseline, left hand MEPs were already suppressed at the onset of the imperative signal, both in young and older adults. This indicates that MEPs were suppressed even before the participants had detected the presence (or absence) of incongruent flankers. Such a suppression of MEPs at TMSIMP has been reported in many other RT tasks; it has usually been related to impulse control as it is thought to help prevent responses from being released prematurely (Klein et al., 2012, 2016; Duque et al., 2013, 2014; Labruna et al., 2014; Bestmann and Duque, 2016).
When the left hand MEPs were elicited by TMS pulses applied at a later time point, between the imperative signal and the subjects’ responses (MEPsPREP), they remained strongly inhibited irrespective of whether they occurred in a selected condition (preceding left hand responses) or a non-selected condition (preceding right hand responses), both in young and older individuals. Note that MEPsPREP were purposely elicited relatively far from movement onset, at a time when inhibitory influences are strongest during action preparation (see “Materials and Method” Section). This time epoch occurs before corticospinal excitability of the selected effector begins to ramp up due to excitatory processes (Cos et al., 2014; Duque et al., 2014; Klein et al., 2016).
MEP Correlates of the Conflict-Driven Behavioral Cost in Young and Older Adults
As expected, selecting responses in the presence of sensory conflict had a behavioral cost. Participants responded more slowly following incongruent compared to congruent imperative signals. They also made more errors in the former condition, consistent with the detrimental impact of incongruent sensory information on motor performance. Besides, older individuals were overall slower than young adults, regardless of the type of trial they were involved in. That is, their RTs were longer, both in congruent and incongruent trials, suggesting that they behaved generally more cautiously. Consistent with this view, they made less errors in incongruent trials compared to young subjects, although the error rate was comparable for the two groups of subjects in congruent trials. This means that the flankers had a less deteriorating effect on accuracy in the older than in the young participants. Consistently, when computed on the rate of errors, the incongruency effect was generally smaller in older than in young adults. The effect of incongruency on the RTs was comparable in the two groups. Hence, older individuals used a strategy which permitted them to behave more accurately than young subjects in incongruent trials. Similar observations have been reported in several previous studies (Wild-Wall et al., 2008; Verhaeghen, 2011; Hsieh and Fang, 2012; Hsieh et al., 2012).
Despite this behavioral discrepancy, the pattern of motor excitability changes was comparable during movement preparation in the young and older individuals. That is, left MEPsPREP were similarly suppressed in both groups, both when they were elicited in a selected (preceding left hand responses) or non-selected (preceding right hand responses) condition. In addition, incongruent sensory information impacted on motor activity in a comparable way in both groups. When MEPsPREP were elicited in a non-selected condition, they were larger following incongruent signals (flankers indicating a left hand response) than following congruent signals (flankers pointing to the right). Accordingly, when elicited in incongruent trials, the amplitude of MEPsPREP was even higher in the non-selected compared to the selected condition; the amplitude of MEPsPREP was comparable in the two conditions during congruent trials.
In view of the lack of significant difference between the two groups, the MEPsPREP results indicate that the lower rate of errors found in older than young participants cannot be accounted for by a smaller impact of incongruent sensory information on motor activity in the former group of subjects. Rather, the greater accuracy of older individuals could be due to a shift in speed-accuracy tradeoff (Lamb et al., 2016): older subjects might have favored accuracy over speed. That is, they might have responded more carefully, slowing down their responses to cope better with conflict, as already proposed in the past (Wild-Wall et al., 2008; Verhaeghen, 2011; Hsieh and Fang, 2012; Hsieh et al., 2012). Alternatively, the smaller speed-accuracy ratio in older participants may result from a lower information processing speed (Cid-Fernández et al., 2016; Correa-Jaraba et al., 2016). Such a deterioration would lead to longer RTs and the enhanced accuracy might emerge as a positive side effect of this alteration. That is, because sensory information takes more time to be transmitted from visual to motor areas, incongruent cues may have less impact on motor excitability. Although appealing, this hypothesis is not supported by our data as the MEPsPREP displayed a comparable influence of the incongruent flankers in the two groups of subjects. Future studies are nevertheless required to test whether the increased accuracy of older individuals is due to a voluntary change in strategy and/or is caused by a reduction in processing speed.
MEP Correlates of the Context-Related Behavioral Gain in Young and Older Adults
The impact of incongruent sensory information on MEPsPREP was most evident in the MCB context that is, when incongruent signals were unlikely. Interestingly, when incongruent signals were strongly expected because they occurred in a majority of trials (MIB context), performance was significantly enhanced and this was true both in young and older adults. In both groups, responses were provided faster following conflicting signals in the MIB than MCB context and this was associated with a higher level of accuracy.
The behavioral gain described above was associated with an enhanced motor inhibition during movement preparation, both in young and older adults. As such, MEPsPREP were more strongly suppressed during incongruent trials performed in the MIB than MCB context. Interestingly, such a context-related strengthening of motor inhibition was not observed in the absence of conflict. That is, we did not observe any increased suppression of MEPsPREP in congruent trials of the MIB context. This suggests that anticipating conflict allowed the recruitment of additional inhibitory influences but the latter were only released when incongruent flankers were detected.
Importantly, the boost in motor inhibition occurred in a generic manner, altering MEPsPREP whether they were probed in a selected or a non-selected condition. This observation is not consistent with the view that inhibitory influences are specifically directed at (unwanted) non-selected motor representations during action preparation. Rather, inhibitory influences seem to be released in a broad manner, affecting motor representations regardless of their function in the forthcoming response. Accordingly, several recent studies have reported widespread motor inhibition during action preparation, both during regular (Duque et al., 2014) and instructed-delay choice RT tasks (Greenhouse et al., 2015b; Quoilin and Derosiere, 2015; Wilhelm et al., 2016). Our current results provide support for the view that the strength of this motor inhibition is calibrated as a function of the task demand (Greenhouse et al., 2015a).
Interestingly, in young subjects, anticipating conflict was also associated with a stronger MEP suppression at the onset of the imperative signal. That is, MEPs at TMSIMP were smaller in the MIB than in the MCB context. Hence, in young subjects, a first strengthening of motor inhibition occurred even before incongruent flankers were detected. The older participants also displayed MEP suppression at TMSIMP. However, the latter effect was not modulated by the context. In fact, although the strength of MEP suppression was comparable between young and older subjects in the MCB context, it was found much less pronounced in older than in young individuals in the MIB context. Hence, anticipating conflict was not associated with a strengthening of motor inhibition in the older participants. In these subjects, motor inhibitory influences were only enhanced during movement preparation, if incongruent signals were detected. One possible explanation for this age-related change may be found in the fact that older participants behaved more slowly. As such, older participants might have been at a less advanced stage of motor preparation at TMSIMP, reducing the need to adjust inhibition at such an early time. Alternatively, the slower performance of older adults may result, at least in part, from an altered ability to generate inhibition anticipatively. Future experiments are required to test whether the absence of anticipatory modulation of motor inhibition in older adults reflects a deterioration of control processes or the consequence of a change in strategy. One possibility would be to investigate control processes while forcing the older adults to respond as fast as the young individuals.
Baseline Motor Excitability in Young and Older Adults
In line with previous observations (Peinemann et al., 2001; Pitcher et al., 2003; Oliviero et al., 2006; Rossini et al., 2007; Cuypers et al., 2013), our data indicate that the motor output system becomes less excitable by TMS along with aging. The MEPs elicited at 120% of the rMT were of smaller amplitude in the older individuals compared to the young adults. This change is likely due to a combination of age-related alterations to the motor output system, including cortical atrophy, less synchronous activation of motor neurons, loss of motor peripheral fibers and a decline in the neuromuscular system. Notably, beside this difference in motor excitability, resting MEPs displayed comparable changes in both groups of participants: baseline MEPs were globally larger when probed within the blocks (TMSBASELINE-IN) compared to between the blocks (TMSBASELINE-OUT) and this effect at TMSBASELINE-IN occurred regardless of the context within which the MEPs were probed (MCB or MIB).
Conclusion
Older subjects were surprisingly proficient in the Eriksen Flanker task. Although generally slower, they made less errors than young subjects in incongruent trials. In addition, all subjects performed better when they anticipated conflict compared to when they did not. In both groups, this behavioral gain was associated with an increased motor inhibition once conflict was detected during action preparation; such a strengthening of inhibitory influences was not observed in the absence of conflict, following congruent signals. In the young subjects, the behavioral gain was also associated with a stronger MEP suppression at the onset of the imperative signal, before conflict was actually detected. Such a modulation was not found in the older participants. This may be due to the fact that the use of a different strategy, favoring accuracy over speed, did not require them to recruit such a process. An alternative explanation is that the ability to recruit inhibition in anticipation of conflict is altered in older adults, forcing them to change their strategy (i.e., to respond slower), an issue for future investigation.
Author Contributions
JD and SPS contributed to the conception and design of the study. JD and CP performed the experiments, analyzed the data and contributed to their interpretation. JD, CP and SPS wrote the manuscript.
Funding
This work was supported by grants from the Fonds Spéciaux de Recherche (FSR) of the Université catholique de Louvain, from the Fondation Médicale Reine Elisabeth (FMRE), from the Fonds de la Recherche Scientifique (FRS-FNRS: MIS F.4512.14), from the Research Foundation-Flanders (FWO) (G0708.14) and from the KU Leuven Research Fund (C16/15/070).
Conflict of Interest Statement
The authors declare that the research was conducted in the absence of any commercial or financial relationships that could be construed as a potential conflict of interest.
Acknowledgments
We are thankful to Pierre-Alexandre Klein and Sophie Verset for their help on the data acquisition and analysis.
Abbreviations
EMG, electromyography; FDI, First dorsal interosseous muscle; M1, primary motor cortex; MCB, Mostly Congruent Block; MEP, Motor-evoked potential; MIB, Mostly Incongruent Block; RT, Reaction time; rMT, resting motor threshold; TMS, Transcranial Magnetic Stimulation.
References
Bestmann, S., and Duque, J. (2016). Transcranial magnetic stimulation: decomposing the processes underlying action preparation. Neuroscientist 22, 392–405. doi: 10.1177/1073858415592594
Bönstrup, M., Hagemann, J., Gerloff, C., Sauseng, P., and Hummel, F. C. (2015). Alpha oscillatory correlates of motor inhibition in the aged brain. Front. Aging Neurosci. 7:193. doi: 10.3389/fnagi.2015.00193
Botvinick, M., Nystrom, L. E., Fissell, K., Carter, C. S., and Cohen, J. D. (1999). Conflict monitoring versus selection-for-action in anterior cingulate cortex. Nature 402, 179–181. doi: 10.1038/46035
Cavallo, A., Becchio, C., Sartori, L., Bucchioni, G., and Castiello, U. (2012). Grasping with tools: corticospinal excitability reflects observed hand movements. Cereb. Cortex 22, 710–716. doi: 10.1093/cercor/bhr157
Chen, C. Y., Muggleton, N. G., Tzeng, O. J., Hung, D. L., and Juan, C. H. (2009). Control of prepotent responses by the superior medial frontal cortex. Neuroimage 44, 537–545. doi: 10.1016/j.neuroimage.2008.09.005
Cid-Fernández, S., Lindín, M., and Díaz, F. (2016). Information processing becomes slower and predominantly serial in aging: characterization of response-related brain potentials in an auditory-visual distraction-attention task. Biol. Psychol. 113, 12–23. doi: 10.1016/j.biopsycho.2015.11.002
Cohen, M. X., and Ridderinkhof, K. R. (2013). EEG source reconstruction reveals frontal-parietal dynamics of spatial conflict processing. PLoS One 8:e57293. doi: 10.1371/journal.pone.0057293
Correa-Jaraba, K. S., Cid-Fernández, S., Lindín, M., and Díaz, F. (2016). Involuntary capture and voluntary reorienting of attention decline in middle-aged and old participants. Front. Hum. Neurosci. 10:129. doi: 10.3389/fnhum.2016.00129
Cos, I., Duque, J., and Cisek, P. (2014). Rapid prediction of biomechanical costs during action decisions. J. Neurophysiol. 112, 1256–1266. doi: 10.1152/jn.00147.2014
Cuypers, K., Thijs, H., Duque, J., Swinnen, S. P., Levin, O., and Meesen, R. L. (2013). Age-related differences in corticospinal excitability during a choice reaction time task. Age (Dordr) 35, 1705–1719. doi: 10.1007/s11357-012-9471-1
Derosiere, G., Zénon, A., Alamia, A., and Duque, J. (2016). Primary motor cortex contributes to the implementation of implicit value-based rules during motor decisions. Neuroimage doi: 10.1016/j.neuroimage.2016.10.010 [Epub ahead of print].
Doya, K., and Shadlen, M. N. (2012). Decision making. Curr. Opin. Neurobiol. 22, 911–913. doi: 10.1016/j.conb.2012.10.003
Duprez, J., Houvenaghel, J. F., Naudet, F., Dondaine, T., Auffret, M., Robert, G., et al. (2016). Evaluating cognitive action control using eye-movement analysis: an oculomotor adaptation of the simon task. Front. Hum. Neurosci. 10:84. doi: 10.3389/fnhum.2016.00084
Duque, J., Labruna, L., Cazares, C., and Ivry, R. B. (2014). Dissociating the influence of response selection and task anticipation on corticospinal suppression during response preparation. Neuropsychologia 65, 287–296. doi: 10.1016/j.neuropsychologia.2014.08.006
Duque, J., Mazzocchio, R., Dambrosia, J., Murase, N., Olivier, E., and Cohen, L. G. (2005). Kinematically specific interhemispheric inhibition operating in the process of generation of a voluntary movement. Cereb. Cortex 15, 588–593. doi: 10.1093/cercor/bhh160
Duque, J., Murase, N., Celnik, P., Hummel, F., Harris-Love, M., Mazzocchio, R., et al. (2007). Intermanual differences in movement-related interhemispheric inhibition. J. Cogn. Neurosci. 19, 204–213. doi: 10.1162/jocn.2007.19.2.204
Duque, J., Olivier, E., and Rushworth, M. (2013). Top-down inhibitory control exerted by the medial frontal cortex during action selection under conflict. J. Cogn. Neurosci. 25, 1634–1648. doi: 10.1162/jocn_a_00421
Eriksen, B. A., and Eriksen, C. W. (1974). Effect of noise letters upon the identification of a target letter in a nonsearch task. Percept. Psychophys. 16, 143–149. doi: 10.3758/bf03203267
Folstein, M. F., Folstein, S. E., and McHugh, P. R. (1975). “Mini-mental state”. A practical method for grading the cognitive state of patients for the clinician. J. Psychiatr. Res. 12, 189–198. doi: 10.1016/0022-3956(75)90026-6
Fujiyama, H., Hinder, M. R., Schmidt, M. W., Tandonnet, C., Garry, M. I., and Summers, J. J. (2012). Age-related differences in corticomotor excitability and inhibitory processes during a visuomotor RT task. J. Cogn. Neurosci. 24, 1253–1263. doi: 10.1162/jocn_a_00201
Gazzaley, A., Clapp, W., Kelley, J., McEvoy, K., Knight, R. T., and D’Esposito, M. (2008). Age-related top-down suppression deficit in the early stages of cortical visual memory processing. Proc. Natl. Acad. Sci. U S A 105, 13122–13126. doi: 10.1073/pnas.0806074105
Gazzaley, A., and D’Esposito, M. (2007). Top-down modulation and normal aging. Ann. N Y Acad. Sci. 1097, 67–83. doi: 10.1196/annals.1379.010
Gold, J. I., and Shadlen, M. N. (2007). The neural basis of decision making. Annu. Rev. Neurosci. 30, 535–574. doi: 10.1146/annurev.neuro.29.051605.113038
Grandjean, J., D’Ostilio, K., Phillips, C., Balteau, E., Degueldre, C., Luxen, A., et al. (2012). Modulation of brain activity during a Stroop inhibitory task by the kind of cognitive control required. PLoS One 7:e41513. doi: 10.1371/journal.pone.0041513
Greenhouse, I., Saks, D., Hoang, T., and Ivry, R. B. (2015a). Inhibition during response preparation is sensitive to response complexity. J. Neurophysiol. 113, 2792–2800. doi: 10.1152/jn.00999.2014
Greenhouse, I., Sias, A., Labruna, L., and Ivry, R. B. (2015b). Nonspecific inhibition of the motor system during response preparation. J. Neurosci. 35, 10675–10684. doi: 10.1523/JNEUROSCI.1436-15.2015
Hamilton, M. (1960). A rating scale for depression. J. Neurol. Neurosurg. Psychiatry 23, 56–62. doi: 10.1136/jnnp.23.1.56
Hedden, T., and Gabrieli, J. D. (2004). Insights into the ageing mind: a view from cognitive neuroscience. Nat. Rev. Neurosci. 5, 87–96. doi: 10.1038/nrn1323
Hedden, T., and Gabrieli, J. D. (2005). Healthy and pathological processes in adult development: new evidence from neuroimaging of the aging brain. Curr. Opin. Neurol. 18, 740–747. doi: 10.1097/01.wco.0000189875.29852.48
Heuninckx, S., Wenderoth, N., Debaere, F., Peeters, R., and Swinnen, S. P. (2005). Neural basis of aging: the penetration of cognition into action control. J. Neurosci. 25, 6787–6796. doi: 10.1523/JNEUROSCI.1263-05.2005
Hsieh, S., and Fang, W. (2012). Elderly adults through compensatory responses can be just as capable as young adults in inhibiting the flanker influence. Biol. Psychol. 90, 113–126. doi: 10.1016/j.biopsycho.2012.03.006
Hsieh, S., Liang, Y. C., and Tsai, Y. C. (2012). Do age-related changes contribute to the flanker effect? Clin. Neurophysiol. 123, 960–972. doi: 10.1016/j.clinph.2011.09.013
Hughes, G., and Yeung, N. (2011). Dissociable correlates of response conflict and error awareness in error-related brain activity. Neuropsychologia 49, 405–415. doi: 10.1016/j.neuropsychologia.2010.11.036
King, J. A., Korb, F. M., and Egner, T. (2012). Priming of control: implicit contextual cuing of top-down attentional set. J. Neurosci. 32, 8192–8200. doi: 10.1523/JNEUROSCI.0934-12.2012
Kleerekooper, I., van Rooij, S. J., van den Wildenberg, W. P., de Leeuw, M., Kahn, R. S., and Vink, M. (2016). The effect of aging on fronto-striatal reactive and proactive inhibitory control. Neuroimage 132, 51–58. doi: 10.1016/j.neuroimage.2016.02.031
Klein, P. A., Duque, J., Labruna, L., and Ivry, R. B. (2016). Comparison of the two cerebral hemispheres in inhibitory processes operative during movement preparation. Neuroimage 125, 220–232. doi: 10.1016/j.neuroimage.2015.10.007
Klein, P. A., Olivier, E., and Duque, J. (2012). Influence of reward on corticospinal excitability during movement preparation. J. Neurosci. 32, 18124–18136. doi: 10.1523/JNEUROSCI.1701-12.2012
Klein, P. A., Petitjean, C., Olivier, E., and Duque, J. (2014). Top-down suppression of incompatible motor activations during response selection under conflict. Neuroimage 86, 138–149. doi: 10.1016/j.neuroimage.2013.08.005
Korsch, M., Frühholz, S., and Herrmann, M. (2014). Ageing differentially affects neural processing of different conflict types-an fMRI study. Front. Aging Neurosci. 6:57. doi: 10.3389/fnagi.2014.00057
Korsch, M., Frühholz, S., and Herrmann, M. (2016). Conflict-specific aging effects mainly manifest in early information processing stages-an ERP study with different conflict types. Front. Aging Neurosci. 8:53. doi: 10.3389/fnagi.2016.00053
Labruna, L., Fernández-del-Olmo, M., and Ivry, R. B. (2011). Comparison of different baseline conditions in evaluating factors that influence motor cortex excitability. Brain Stimul. 4, 152–155. doi: 10.1016/j.brs.2010.09.010
Labruna, L., Lebon, F., Duque, J., Klein, P. A., Cazares, C., and Ivry, R. B. (2014). Generic inhibition of the selected movement and constrained inhibition of nonselected movements during response preparation. J. Cogn. Neurosci. 26, 269–278. doi: 10.1162/jocn_a_00492
Lamb, D. G., Correa, L. N., Seider, T. R., Mosquera, D. M., Rodriguez, J. A. Jr., Salazar, L., et al. (2016). The aging brain: movement speed and spatial control. Brain Cogn. 109, 105–111. doi: 10.1016/j.bandc.2016.07.009
Leocani, L., Cohen, L. G., Wassermann, E. M., Ikoma, K., and Hallett, M. (2000). Human corticospinal excitability evaluated with transcranial magnetic stimulation during different reaction time paradigms. Brain 123, 1161–1173. doi: 10.1093/brain/123.6.1161
Levin, O., Fujiyama, H., Boisgontier, M. P., Swinnen, S. P., and Summers, J. J. (2014). Aging and motor inhibition: a converging perspective provided by brain stimulation and imaging approaches. Neurosci. Biobehav. Rev. 43, 100–117. doi: 10.1016/j.neubiorev.2014.04.001
Levin, O., and Netz, Y. (2015). Aerobic training as a means to enhance inhibition: what’s yet to be studied? Eur. Rev. Aging Phys. Act. 12:14. doi: 10.1186/s11556-015-0160-9
Lucci, G., Berchicci, M., Spinelli, D., Taddei, F., and Di Russo, F. (2013). The effects of aging on conflict detection. PLoS One 8:e56566. doi: 10.1371/journal.pone.0056566
Mars, R. B., Klein, M. C., Neubert, F. X., Olivier, E., Buch, E. R., Boorman, E. D., et al. (2009). Short-latency influence of medial frontal cortex on primary motor cortex during action selection under conflict. J. Neurosci. 29, 6926–6931. doi: 10.1523/JNEUROSCI.1396-09.2009
Marshall, A. C., Cooper, N. R., and Geeraert, N. (2016). Experienced stress produces inhibitory deficits in old adults’ Flanker task performance: first evidence for lifetime stress effects beyond memory. Biol. Psychol. 113, 1–11. doi: 10.1016/j.biopsycho.2015.10.008
Mattler, U. (2003). Delayed flanker effects on lateralized readiness potentials. Exp. Brain Res. 151, 272–288. doi: 10.1007/s00221-003-1486-5
McNab, F., Zeidman, P., Rutledge, R. B., Smittenaar, P., Brown, H. R., Adams, R. A., et al. (2015). Age-related changes in working memory and the ability to ignore distraction. Proc. Natl. Acad. Sci. U S A 112, 6515–6518. doi: 10.1073/pnas.1504162112
Michelet, T., Duncan, G. H., and Cisek, P. (2010). Response competition in the primary motor cortex: corticospinal excitability reflects response replacement during simple decisions. J. Neurophysiol. 104, 119–127. doi: 10.1152/jn.00819.2009
Montague, P. R., King-Casas, B., and Cohen, J. D. (2006). Imaging valuation models in human choice. Annu. Rev. Neurosci. 29, 417–448. doi: 10.1146/annurev.neuro.29.051605.112903
Oldfield, R. C. (1971). The assessment and analysis of handedness: the Edinburgh inventory. Neuropsychologia 9, 97–113. doi: 10.1016/0028-3932(71)90067-4
Oliveira, F. T., Diedrichsen, J., Verstynen, T., Duque, J., and Ivry, R. B. (2010). Transcranial magnetic stimulation of posterior parietal cortex affects decisions of hand choice. Proc. Natl. Acad. Sci. U S A 107, 17751–17756. doi: 10.1073/pnas.1006223107
Oliviero, A., Profice, P., Tonali, P. A., Pilato, F., Saturno, E., Dileone, M., et al. (2006). Effects of aging on motor cortex excitability. Neurosci. Res. 55, 74–77. doi: 10.1016/j.neures.2006.02.002
Peinemann, A., Lehner, C., Conrad, B., and Siebner, H. R. (2001). Age-related decrease in paired-pulse intracortical inhibition in the human primary motor cortex. Neurosci. Lett. 313, 33–36. doi: 10.1016/s0304-3940(01)02239-x
Pitcher, J. B., Ogston, K. M., and Miles, T. S. (2003). Age and sex differences in human motor cortex input-output characteristics. J. Physiol. 546, 605–613. doi: 10.1113/jphysiol.2002.029454
Praamstra, P., Stegeman, D. F., Cools, A. R., and Horstink, M. W. (1998). Reliance on external cues for movement initiation in Parkinson’s disease. Evidence from movement-related potentials. Brain 121, 167–177. doi: 10.1093/brain/121.1.167
Quoilin, C., and Derosiere, G. (2015). Global and specific motor inhibitory mechanisms during action preparation. J. Neurosci. 35, 16297–16299. doi: 10.1523/JNEUROSCI.3664-15.2015
Quoilin, C., Lambert, J., Jacob, B., Klein, P. A., and Duque, J. (2016). Comparison of motor inhibition in variants of the instructed-delay choice reaction time task. PLoS One 11:e0161964. doi: 10.1371/journal.pone.0161964
Radvansky, G. A., Zacks, R. T., and Hasher, L. (2005). Age and inhibition: the retrieval of situation models. J. Gerontol. B Psychol. Sci. Soc. Sci. 60, P276–P278. doi: 10.1093/geronb/60.5.p276
Rossini, P. M., Rossi, S., Babiloni, C., and Polich, J. (2007). Clinical neurophysiology of aging brain: from normal aging to neurodegeneration. Prog. Neurobiol. 83, 375–400. doi: 10.1016/j.pneurobio.2007.07.010
Sartori, L., Cavallo, A., Bucchioni, G., and Castiello, U. (2011). Corticospinal excitability is specifically modulated by the social dimension of observed actions. Exp. Brain Res. 211, 557–568. doi: 10.1007/s00221-011-2650-y
Siegel, M., Engel, A. K., and Donner, T. H. (2011). Cortical network dynamics of perceptual decision-making in the human brain. Front. Hum. Neurosci. 5:21. doi: 10.3389/fnhum.2011.00021
Smittenaar, P., Rutledge, R. B., Zeidman, P., Adams, R. A., Brown, H., Lewis, G., et al. (2015). Proactive and reactive response inhibition across the lifespan. PLoS One 10:e0140383. doi: 10.1371/journal.pone.0140383
Stewart, J. C., Tran, X., and Cramer, S. C. (2014). Age-related variability in performance of a motor action selection task is related to differences in brain function and structure among older adults. Neuroimage 86, 326–334. doi: 10.1016/j.neuroimage.2013.10.016
Takezawa, T., and Miyatani, M. (2005). Quantitative relation between conflict and response inhibition in the Flanker task. Psychol. Rep. 97, 515–526. doi: 10.2466/pr0.97.6.515-526
Taylor, P. C., Nobre, A. C., and Rushworth, M. F. (2007). Subsecond changes in top down control exerted by human medial frontal cortex during conflict and action selection: a combined transcranial magnetic stimulation electroencephalography study. J. Neurosci. 27, 11343–11353. doi: 10.1523/JNEUROSCI.2877-07.2007
Thura, D., and Cisek, P. (2014). Deliberation and commitment in the premotor and primary motor cortex during dynamic decision making. Neuron 81, 1401–1416. doi: 10.1016/j.neuron.2014.01.031
Vallesi, A., and Stuss, D. T. (2010). Excessive sub-threshold motor preparation for non-target stimuli in normal aging. Neuroimage 50, 1251–1257. doi: 10.1016/j.neuroimage.2010.01.022
van Campen, A. D., Keuken, M. C., van den Wildenberg, W. P., and Ridderinkhof, K. R. (2014). TMS over M1 reveals expression and selective suppression of conflicting action impulses. J. Cogn. Neurosci. 26, 1–15. doi: 10.1162/jocn_a_00482
Vandermeeren, Y., Davare, M., Duque, J., and Olivier, E. (2009). Reorganization of cortical hand representation in congenital hemiplegia. Eur. J. Neurosci. 29, 845–854. doi: 10.1111/j.1460-9568.2009.06619.x
Verhaeghen, P. (2011). Aging and executive control: reports of a demise greatly exaggerated. Curr. Dir. Psychol. Sci. 20, 174–180. doi: 10.1177/0963721411408772
Wild-Wall, N., Falkenstein, M., and Hohnsbein, J. (2008). Flanker interference in young and older participants as reflected in event-related potentials. Brain Res. 1211, 72–84. doi: 10.1016/j.brainres.2008.03.025
Wilhelm, E., Quoilin, C., Petitjean, C., and Duque, J. (2016). A double-coil TMS method to assess corticospinal excitability changes at a near-simultaneous time in the two hands during movement preparation. Front. Hum. Neurosci. 10:88. doi: 10.3389/fnhum.2016.00088
Young, J. J., and Shapiro, M. L. (2011). The orbitofrontal cortex and response selection. Ann. N Y Acad. Sci. 1239, 25–32. doi: 10.1111/j.1749-6632.2011.06279.x
Zénon, A., Klein, P. A., Alamia, A., Boursoit, F., Wilhelm, E., and Duque, J. (2015). Increased reliance on value-based decision processes following motor cortex disruption. Brain Stimul. 8, 957–964. doi: 10.1016/j.brs.2015.05.007
Zhu, D. C., Zacks, R. T., and Slade, J. M. (2010). Brain activation during interference resolution in young and older adults: an fMRI study. Neuroimage 50, 810–817. doi: 10.1016/j.neuroimage.2009.12.087
Keywords: cognitive control, aging, action selection, corticospinal excitability, transcranial magnetic stimulation, Eriksen Flanker task
Citation: Duque J, Petitjean C and Swinnen SP (2016) Effect of Aging on Motor Inhibition during Action Preparation under Sensory Conflict. Front. Aging Neurosci. 8:322. doi: 10.3389/fnagi.2016.00322
Received: 30 September 2016; Accepted: 12 December 2016;
Published: 27 December 2016.
Edited by:
Lutz Jäncke, University of Zurich, SwitzerlandCopyright © 2016 Duque, Petitjean and Swinnen. This is an open-access article distributed under the terms of the Creative Commons Attribution License (CC BY). The use, distribution and reproduction in other forums is permitted, provided the original author(s) or licensor are credited and that the original publication in this journal is cited, in accordance with accepted academic practice. No use, distribution or reproduction is permitted which does not comply with these terms.
*Correspondence: Julie Duque, julie.duque@uclouvain.be