Neuropsychiatric Symptoms Mediated the Relationship Between Odor Identification and Cognition in Alzheimer's Disease Spectrum: A Structural Equation Model Analysis
- 1Department of Geriatric Psychiatry, Memory Clinic, The Affiliated Brain Hospital of Guangzhou Medical University, Guangzhou Huiai Hospital, Guangzhou, China
- 2Department of Geriatric Psychiatry, The Second People's Hospital of Dali Bai Autonomous Prefecture, Dali, China
- 3Department of Neurology, The Affiliated Brain Hospital of Guangzhou Medical University, Guangzhou Huiai Hospital, Guangzhou, China
- 4Department of Otorhinolaryngology, Smell and Taste Clinic, Technische Universität Dresden, Dresden, Germany
- 5The First School of Clinical Medicine, Southern Medical University, Guangzhou, China
- 6Guangdong Engineering Technology Research Center for Translational Medicine of Mental Disorders, Guangzhou, China
Background: Odor identification dysfunction is an early predictor of the development of Alzheimer's disease (AD), but neuropsychiatric symptoms (NPS), which are common in AD and mild cognitive impairment (MCI), are also associated with odor identification dysfunction. Whether NPS affect the specificity of using odor identification dysfunction to predict cognitive decline in AD and MCI remains unclear.
Methods: Patients (233 with MCI and 45 with AD) and 45 healthy controls (HCs) underwent assessments of odor identification (Sniffin' Sticks), NPS (Neuropsychiatric Inventory-12), and cognitive function (global cognition, memory, language, executive function, visual-spatial skill, and attention). Structural equation modeling (SEM) with bootstrapping estimation was conducted to explore the relationships between odor identification, NPS, and cognition.
Results: Patients with NPS showed significantly worse performance in odor identification and cognition than patients without NPS and HCs. The SEM showed odor identification to be positively associated with cognition, and cognition had special indirect effects on odor identification through affective and psychosis symptoms (two factors extracted from Neuropsychiatric Inventory-12). Additionally, affective and psychosis symptoms partially mediated the effect of cognition on odor identification.
Conclusion: Neuropsychiatric symptoms are associated with odor identification dysfunction in patients with AD and MCI. Studies exploring the relationship between odor identification dysfunction and cognitive decline in patients with AD and MCI should include an assessment of affective and psychosis symptoms, and adjust their confounding effects.
Background
Odor identification dysfunction is commonly observed in Alzheimer's disease (AD) (85–90%) (Woodward et al., 2017) and mild cognitive impairment (MCI) (47–65%) (Velayudhan, 2015; Wang et al., 2021). It precedes cognitive decline and clinical manifestations of AD and MCI and may be paralleled with tau-mediated neuronal damage during disease progression (Murphy, 2019). Poor odor identification has been repeatedly shown to be associated with worse general cognitive performance (Wang et al., 2021), increased cortical amyloid burden (Bahar-Fuchs et al., 2010), and lower ratios of CSF t-tau and P181-tau to Aβ1−42 (Lafaille-Magnan et al., 2017). Additionally, longitudinal studies suggested that poor odor identification predicts cognitive decline and conversion to major neurocognitive disorder in amnestic MCI subjects (Devanand et al., 2015; Roberts et al., 2016) and in older individuals with normal cognitive function (Djordjevic et al., 2008). A recent study also showed a combination of Aβ1−42 and odor identification scores to improve the predictive accuracy of conversion from MCI to AD (Zhao et al., 2020). These observations suggest that odor identification dysfunction serves as a non-invasive and cost-effective marker for predicting cognitive decline in AD spectrum disease.
Numerous studies have found a close relationship between odor identification dysfunction and neuropsychiatric symptoms (NPS) such as depression, anxiety, and psychosis symptoms (Ropacki and Jeste, 2005; Moberg et al., 2014; Croy and Hummel, 2017; Kamath et al., 2018). With regard to affective symptoms, patients with olfactory loss are more likely to exhibit symptoms of depression and anxiety (Croy et al., 2010), and patients with major depression exhibit impaired odor identification (Chen et al., 2019). In addition, olfactory dysfunction recovers with the remission of depressive symptoms (Zucco and Bollini, 2011). In patients with late-life depression, those with odor identification dysfunction exhibit poorer cognitive performance and more structural and functional brain abnormalities (Chen et al., 2018, 2021). Likewise, high-trait anxiety individuals detect odors faster than low-trait anxiety participants, and trait anxiety levels are negatively correlated with the speed of reactions to odors (La Buissonnière-Ariza et al., 2013). With respect to psychosis symptoms, a meta-analysis found moderate to high olfactory dysfunction in schizophrenia patients (Moberg et al., 2014), and poorer odor identification scores were found to be associated with longer disease duration (Moberg et al., 2006). Moreover, typical characteristics of schizophrenia, such as negative symptoms and lower intelligence, were found to be related to odor identification dysfunction (Corcoran et al., 2005), and odor identification dysfunction is also reported in first-degree relatives and monozygotic twins of schizophrenia patients (Ugur et al., 2005; Turetsky et al., 2008). Overall, these findings show that odor identification dysfunction is strongly associated with NPS, especially for affective and psychosis symptoms.
Notably, various kinds of NPS are common manifestations in patients with AD and MCI, including depression, anxiety, apathy, delusions, hallucinations, episodes of verbal, and physical aggression, etc. (Ropacki and Jeste, 2005; Hollingworth et al., 2006). The prevalence of NPS in patients with AD is 56–98% in the community and up to 91–96% in hospitals and long-term care facilities (Gerlach and Kales, 2018). For MCI, NPS have been reported in 35–85% of patients and may occur prior to cognitive decline (Gallagher et al., 2017). Additionally, NPS are associated with greater functional impairment, poorer quality of life, accelerated cognitive decline and a more significant degree of AD neurodegeneration (Kales et al., 2015).
All of these studies illustrate that odor identification dysfunction, cognitive decline, and NPS are closely intertwined, raising the question of whether the prediction of cognitive decline by odor identification dysfunction may be affected by NPS in patients with AD and MCI. Considering what is mentioned above, we hypothesized that the relationship between odor identification dysfunction and cognitive decline is mediated by NPS (especially affective and psychosis symptoms) in patients with AD and MCI. The present study aimed to explore the relationship between NPS, odor identification dysfunction, and cognitive decline in patients with AD and MCI via structural equation modeling (SEM) analysis. The results provide a deeper understanding of how odor identification dysfunction, cognitive decline, and NPS interact with each other and contribute to the rational use of odor identification in clinical practice.
Materials and Methods
Participants
In total, 233 subjects with MCI and 45 with AD were continuously recruited from the Affiliated Brain Hospital of Guangzhou Medical University (Guangzhou Huiai Hospital), and 45 age-matched healthy controls (HCs) were recruited from communities in Guangzhou. All subjects or their legal guardians provided signed informed consent to participate in the study. The present study was approved by the Ethics Committees of the Affiliated Brain Hospital of Guangzhou Medical University (Guangzhou Huiai Hospital). All procedures performed in this study were done in accordance with the 1964 Helsinki declaration and its later amendments or comparable ethical standards.
The diagnosis of probable AD was defined according to the clinical criteria of the National Institute of Neurological and Communicative Disorders and Stroke-Alzheimer's Disease and Related Disorders Association (Mckhann et al., 1984), and diagnostic criteria for MCI were based on the Peterson criteria (Petersen, 2004). All recruited subjects with a Hachinski score of higher than four were also excluded (Hachinski et al., 1975). The other exclusion criteria were as follows. (1) Major systemic, past, or concomitant diagnoses of psychiatric disorders (such as major depression, schizophrenia, bipolar disorder, posttraumatic stress disorders, panic disorder, etc.); (2) patients with a history of concomitant diagnosis of any neurodegenerative disease aside from AD; (3) other causes (infectious, toxic, and metabolic) of cognitive impairment were excluded; (4) Other causes that significantly influence olfaction, including active upper respiratory/sinus infection or respiratory distress at the time of testing, congenital or traumatic anosmia, known nasal polyps or tumors, current or recent (past 6 months) smoking, and alcohol or substance dependence were also excluded. In addition, a trained psychologist, via dedicated clinical interviews, carefully screened all HCs to exclude any evidence of psychopathological symptoms. All subjects completed structured interviews, standardized olfactory tests, and clinical symptom and comprehensive cognitive assessments on the same day.
Assessments
Assessments of Odor Identification
Odor identification function was assessed using the standardized Sniffin' Sticks Screen 16 test (Hummel et al., 1997) which involves the presentation of odorants through felt-tip pens. For odor performance, the cap of a pen was removed, and the pen's tip was placed approximately 2 cm in front of the participant's nostrils for 3 s. Subjects were presented with 16 common odorants. Odors were identified from flash cards listing four verbal odor descriptors each (forced choice, score range of 0–16). Olfactory testing was performed in a quiet, odorless, and well-ventilated room at the Affiliated Brain Hospital of Guangzhou Medical University.
Assessments of Cognitive Function
Cognitive function in different domains was evaluated by the following neuropsychological tests: the mini mental state examination (MMSE) (Folstein et al., 1975), auditory verbal learning task (AVLT) (Zhao et al., 2012), trail-making test (TMT) (Lu et al., 2006), symbol-digit modality test (SDMT) (Sheridan et al., 2006), boston naming test (BNT) (Guo et al., 2006), and ReyO-sterrieth complex figure (ROCF) test (Guo et al., 2000). The scores of the MMSE represent global cognition. The time take to complete TMT Part B was used to represent executive function. The AVLT N1-3, BNT, ROCF, and SMDT scores represent memory, language, visual-spatial skill, and attention, respectively.
Assessments of Neuropsychiatric Symptoms
Neuropsychiatric symptoms were measured using the Chinese Neuropsychiatric Inventory (NPI) originally proposed by Cummings et al. (1994) and Kaufer et al. (1998). Neuropsychiatric symptoms were scored by monitoring caregivers' responses obtained from a self-reporting questionnaire wherein they selected the frequency (four-point scale) and severity (three-point scale) of symptoms. The frequency and severity scores for each symptom were multiplied as symptom scores with a higher symptom score indicating higher severity (including delusions, hallucinations, agitation, irritability, depression, anxiety, apathy, euphoria, disinhibition, aberrant motor behavior, and sleep and eating disorders). The sum of 12 kinds of symptom scores was defined as the NPI total score.
Patients with NPI total scores of ≥1 were defined as the NPS group, and those with total scores of = 0 were defined as other patients classified as the No-NPS group.
Statistical Analysis
The data were analyzed using the Statistical Package for the Social Sciences version 26.0 (SPSS 26.0) and Amos 24.0 programs (https://www.ibm.com/products/spss-statistics). Neuropsychiatric symptoms were classified by using factor analysis. Before performing factor analysis, the suitability of the data was tested using the Kaiser–Meyer–Olkin (KMO) and Bartlett tests. The internal reliability of the classified NPS symptoms was measured using Cronbach's α. Exploratory factor analysis (EFA) was performed using maximum likelihood analysis followed by varimax factor rotation. Based on the EFA results, models of factorial grouping for the NPI-12 were established using confirmatory factor analysis (CFA). The correlation between the measured variables were determined using the partial correlation coefficient, and age, sex, and years of education were set as control variables.
Structural equation modeling was used to test the hypothesized model. According to Jöreskog and Sörbom (1982), SEM provides a maximum-likelihood estimation of the entire system of a hypothesized model and enables the assessment of variables with data. The error variance of a single variable is determined by the following formula: error variance of X1 = (1 − reliability coefficient) * (S2) (Randall and Schumacker, 2010). In the present analysis, we adopted Anderson and Gerbing (1988) two-step strategy to test the hypothesized model. First, the measurement model was confirmed using CFA, and then we performed a SEM analysis to measure the fit and path coefficients of the hypothesized model. The chi-square (χ2) value, degrees of freedom (df ), the value of χ2/df , the goodness of fit (GFI), the comparative fix index (CFI), Bollen's incremental fit index (IFI), the normed fit index (NFI), the standardized root mean square residual (SRMR), and the root mean square error of approximation (RMSEA) were adopted to estimate model fit. The significance of the effects of the study model was tested using the bootstrapping method (10,000) (Hayes, 2009).
Results
Demographic, Olfactory, and Cognitive Information
Demographic, olfactory, and cognitive information is shown in Table 1. In total, 168 patients were grouped into the NPS group, and 110 were grouped into the No-NPS group.
Exploratory Factor Analysis of Neuropsychiatric Symptom Clusters
The scores for each symptom as shown in Figure 1. Based on the symptom scores for NPS, three factors were extracted via the EFA. The variances for Factor 1, Factor 2, and Factor 3 were 2.27, 2.23, and 1.85, respectively. The explanatory power values of Factor 1, Factor 2, and Factor 3 were 18.9, 18.5, and 17.9%, respectively. The total explanatory power of the three factors represented 55.4% of the total variance.
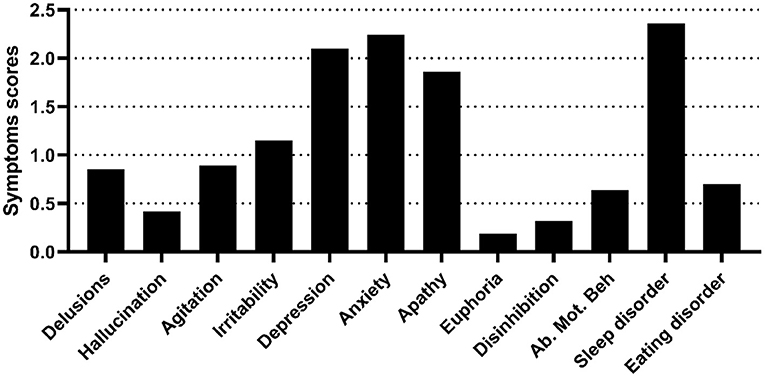
Figure 1. Neuropsychiatric symptoms of the NPS group. The figure shows the symptom scores of the NPS group, as assessed by the NPI-12. Ab. Mot. Beh, aberrant motor behavior.
Symptom Cluster 1 (Factor 1) described affective symptoms, including depression, anxiety and apathy. Symptom Cluster 2 (Factor 2) described psychosis symptoms, including delusions, hallucinations, agitation, and irritability. Symptom Cluster 3 (Factor 3) described behavioral symptoms, including disinhibition, euphoria, aberrant motor behavior, and eating and sleep disorders (Table 2).
The KMO measure for this study was measured as 0.681, indicating an appropriate sample size. In addition, Bartlett's test of sphericity showed that statistical significance was <0.001, thereby confirming the goodness-of-fit of the model.
Confirmatory Factor Analysis of Neuropsychiatric Symptom Clusters
The results of testing the goodness of fit of the CFA show that GFI = 0.786, CFI =0.809, IFI = 0.789, χ2/df = 5.096, and RMSEA = 0.157, indicating a poor fit. To improve reliability, the model was modified by removing variables with factor loadings of <0.6 (hallucinations and sleep and eating disorders) (Bagozzi and Yi, 1989) while simultaneously verifying the model fit (Figure 2). Successively, the goodness-of-fit indices of the modified model were χ2/df = 2.06, GFI = 0.96, CFI = 0.98, NFI = 0.96, IFI = 0.98, and RMSEA = 0.06, indicating a good fit.
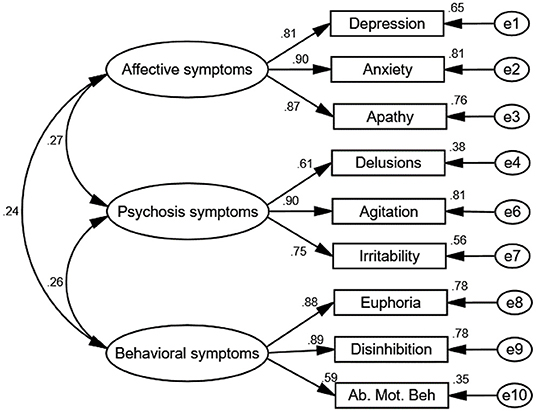
Figure 2. Path diagram of the confirmatory factor analysis. The goodness-of-fit indices of the modified model were as follows: χ2/df = 2.06, GFI = 0.96, CFI = 0.98, NFI = 0.96, IFI = 0.98, and RMSEA = 0.06, which indicate a good fit. Three factors were verified from the CFA: Factor 1 described affective symptoms, including depression, anxiety, and apathy. Factor 2 described psychosis symptoms, including delusions, agitation, and irritability. Factor 3 described behavioral symptoms, including disinhibition, euphoria, snf aberrant motor behavior. Rotation converged in nine iterations. Ab. Mot. Beh, aberrant motor behavior. e1–10 represent residuals of the respective variables.
Correlation Between Clinical Characteristics and Odor Identification in Subjects With NPS
The odor identification score was positively correlated with cognitive scores, and negatively correlated with scores of psychosis and affective symptoms in the NPS group (P < 0.05). There was no significant correlation between odor identification and behavioral symptoms (Table 3).
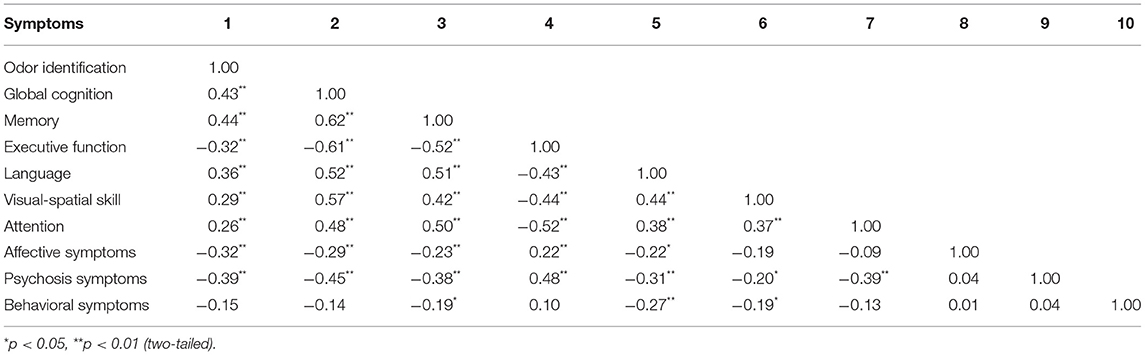
Table 3. Correlation between cognition, NPS, and odor identification in patients with NPS (N = 168).
Testing the Mediator Models
Preliminary Analyses
To measure the internal consistency reliability, convergent validity, and discriminant validity of the constructs in our proposed model, we performed a CFA analysis on the four constructs of cognitive decline, affective symptoms, psychosis symptoms, and odor identification dysfunction (Figures 2, 3). The results reveal that the composite reliability (CR) of each construct ranged from 0.64 to 0.89, exceeding the 0.60 CR threshold value and giving evidence of internal consistency reliability (Fornell and Larcker, 1981; Bagozzi and Yi, 1989). In addition, the factor loadings of the individual items in the model were significant (all p < 0.01) (Table 4), showing preliminary evidence for the convergent validity of the measurement model. Meanwhile, the average variance extracted (AVE) values of all constructs ranged from 0.51 to 0.73, exceeding the 0.50 AVE threshold value (Fornell and Larcker, 1981; Bagozzi and Yi, 1989), denoting acceptable convergent validity.
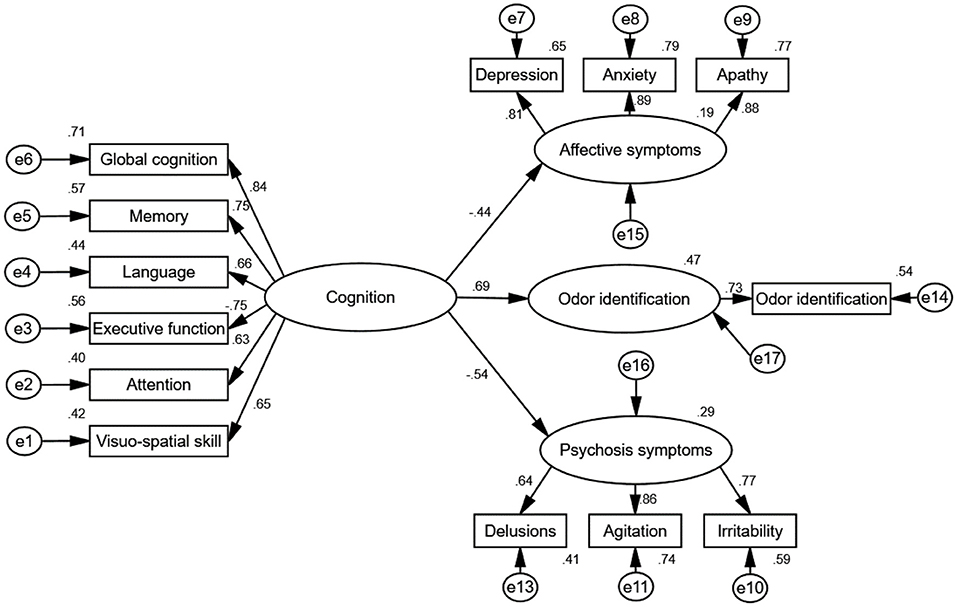
Figure 3. Standardized direct effects of cognition on odor identification, affective symptoms and psychosis symptoms. The goodness-of-fit indices of the model were χ2/df = 1.73, GFI = 0.91, CFI = 0.96, NFI = 0.90, IFI = 0.96, and RMSEA = 0.07, indicating a good fit. The direct effect of cognition on odor identification (standardized direct effect 0.69, p < 0.01), direct effect of cognition on affective symptoms (standardized direct effect 0.44, p < 0.01), and direct effect of cognition on psychosis symptoms (standardized direct effect −0.54, p < 0.01). All results indicate that cognition is associated with odor identification, affective symptoms and psychosis symptoms. e1–17 represent residuals of the respective variables.
Structural Model
We followed Baron and Kenny (1986) suggestion and used a strategy to examine the first condition of mediation. As shown in Table 3, the correlation coefficients indicate that cognition was positively associated with odor identification. In addition, the result for the direct effect of cognition on odor identification is statistically significant (standardized direct effect 0.69, p < 0.01, see Figure 3).
From test of the second condition of mediation, the results for the direct effects of cognition on affective symptoms (standardized direct effect −0.42, p < 0.01), the direct effect of affective symptoms on odor identification (standardized direct effect −0.33, p < 0.01), the direct effect of cognition on psychosis symptoms (standardized direct effect −0.53, p < 0.01), and the direct effect of psychosis symptoms on odor identification (standardized direct effect −0.27, p < 0.01) are statistically significant (Table 5; Figure 4). Therefore, the second conditions of mediation in our proposed model are supported. To investigate the special indirect effects of the dependent variable through the mediators, we performed percentile bootstrapping and bias-corrected percentile bootstrapping at a 95% confidence interval with 10,000 bootstrap samples (Taylor et al., 2008). We followed the suggestions of Hayes (2009) and calculated the confidence interval of the lower and upper bounds to test whether the special indirect effects were significant. As shown in Table 5, the results of the bootstrap test confirm the existence of positive and significant special indirect effects for affective and psychosis symptoms between cognition and odor identification. However, we found no difference in special indirect effects between affective and psychosis symptoms. Thus, affective and psychosis symptoms partially mediated the effect of cognition on odor identification.
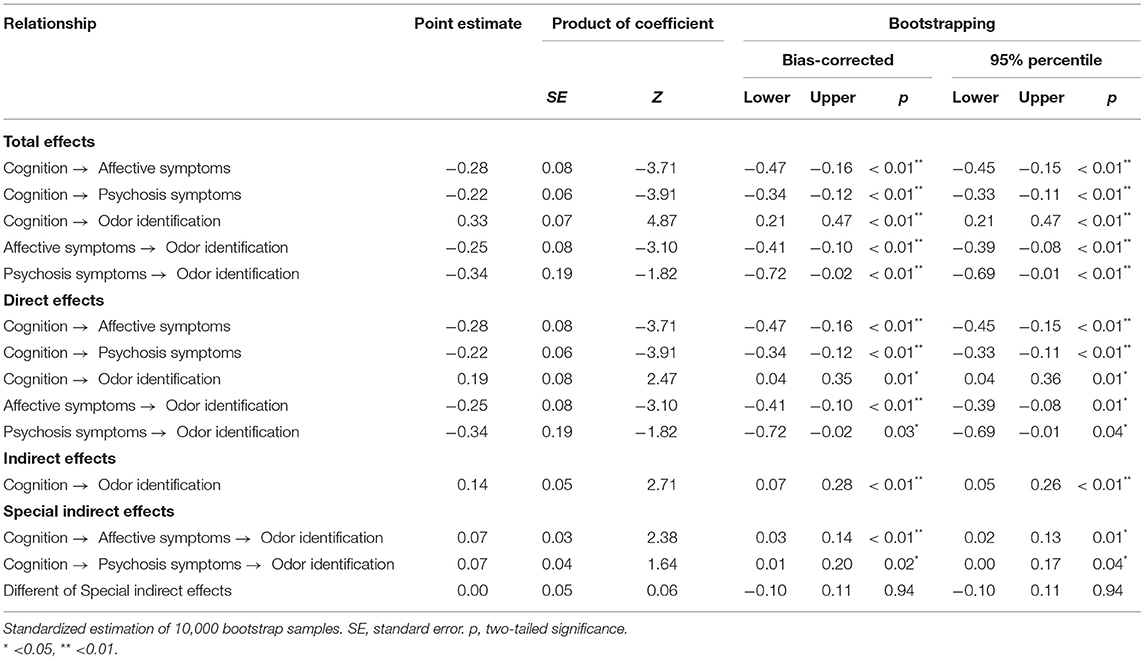
Table 5. Unstandardized total, direct, indirect, and specific indirect effects of the mediation model (N = 168).
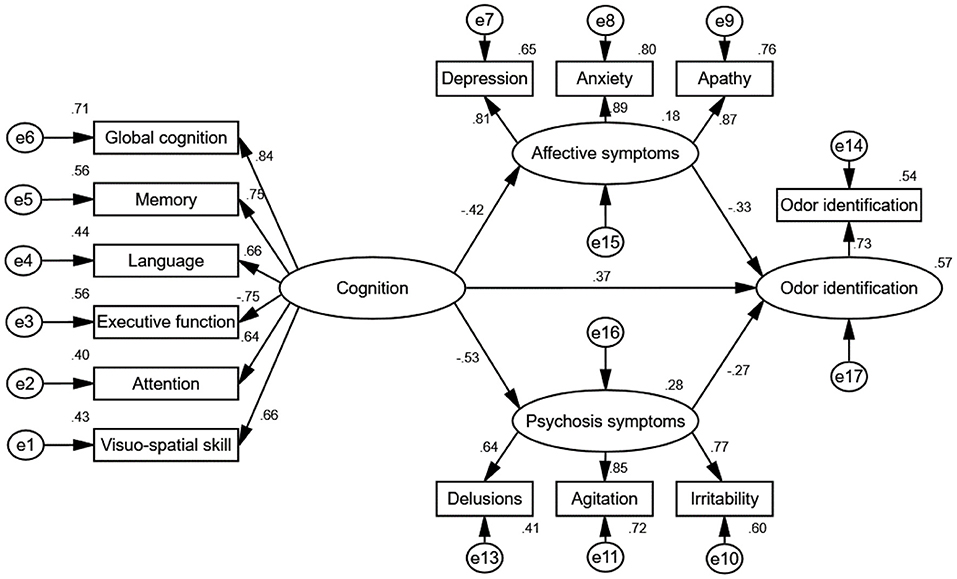
Figure 4. Path diagram of the standardized estimate mediation model. The goodness-of-fit indices of the mediation model were χ2/df = 1.14, GFI = 0.99, CFI = 0.99, NFI = 0.94, IFI = 0.99, and RMSEA = 0.03, indicating a good fit. The mediation model shows that cognition had special indirect effects on odor identification through affective and psychosis symptoms, indicating that affective and psychosis symptoms exhibited partial mediated effects. Rotation converged in 9 iterations. e1–17 represent residuals of the respective variables.
Discussion
The present study explored the relationship between odor identification, NPS and cognition in patients with AD and MCI via SEM. The main findings of this study are as follows: (1) patients with NPS exhibited significantly poorer odor identification and cognition scores than those without NPS and HCs. (2) In patients with NPS, odor identification scores were negatively correlated with affective and psychosis symptoms (factors extracted from the NPI-12) but not with behavioral symptoms. (3) Affective and psychosis symptoms exhibited specific indirect effects between odor identification and cognition. Moreover, there were positively mediated effects of affective and psychosis symptoms on the relationship between odor identification and cognition.
The present SEM analyses suggest additive effects of NPS and odor identification dysfunction on cognitive decline where affective and psychosis symptoms partially mediate the relationship between odor identification dysfunction and cognitive decline. Previous studies demonstrate that both odor identification dysfunction and NPS are common in patients with AD and MCI and that odor identification dysfunction could serve as a marker of indicating early AD pathology and cognitive decline (Devanand et al., 2015; Lafaille-Magnan et al., 2017; Murphy, 2019; Zhao et al., 2020; Wang et al., 2021), but few consider the potentially confounding effect of NPS.
The current study shows that among the three factors of NPS, affective symptoms are most related to odor identification and cognitive decline, and they show a partial mediation of the relationship between cognitive decline and odor identification. It is well acknowledged that numerous brain areas that are altered with affective symptoms and cognitive impairment are also involved in olfaction processing, such as that occurring in the olfactory bulb, hippocampus, amygdala, insula, orbitofrontal cortex, and habenula nucleus (Li et al., 2015; Croy and Hummel, 2017). For depression, Croy et al. identified potential mechanisms linking depression and olfactory dysfunction. First, decreased olfactory function in depression may result from decreased attention to olfaction and a consecutively decreased turnover rate of olfactory receptor neurons in the olfactory epithelium where these effects are temporary and diminish after remission (Li et al., 2015). Second, a smaller olfactory bulb volume may cause decreased signaling from the olfactory bulb to the amygdala, hippocampus, striatum and orbitofrontal cortex (Croy and Hummel, 2017). Similar to what is observed in depression, there are direct connections between olfactory relay neurons and the amygdala, a key node in the regulation of anxiety (Ballanger et al., 2019). Moreover, depression and anxiety can also lead to decreased neurogenesis in the hippocampus and olfactory bulb (Mineur et al., 2007), and olfactory training seems to increase olfactory bulb volume (Negoias et al., 2016), cognitive function (Birte-Antina et al., 2018), and depressive and anxious symptoms (Ballanger et al., 2019). For apathy, a previous study reported a specific association between odor identification dysfunction and the severity of apathy, suggesting that olfactory dysfunction and apathy might result from the progression of disease pathology in shared neural substrates (Seligman et al., 2013). Previous studies have already shown that depression, apathy, and anxiety are precursor symptoms and can predict cognitive decline in the AD spectrum (Craig et al., 2005; Seligman et al., 2013; Ma, 2020). Given the close relationships between odor identification dysfunction, affective symptoms, and cognitive decline, the confounding effect of affective symptoms should be adjusted when using odor identification dysfunction to predict cognitive decline in patients with AD and MCI.
Similar to affective symptoms, psychosis symptoms also had a partial mediated effect on the relationship between cognitive function and odor identification. Previous research indicates that odor identification dysfunction is present in schizophrenia patients (Moberg et al., 2006), first-degree relatives of schizophrenia patients (Turetsky et al., 2008), and subjects at risk for psychosis symptoms (Takahashi et al., 2018; Tang et al., 2018) and is believed to be highly associated with disease duration (Moberg et al., 2006), negative symptoms, and social-cognitive function (Corcoran et al., 2005). The anatomic proximity of the olfactory network to limbic structures provides a potential explanation for the relation between olfaction and affective symptoms, and may account for their shared dysfunction in various psychiatric disorders. Many of these regions, including the amygdala, hippocampus, insula, anterior cingulate cortex, and orbitofrontal cortex, have been described as common neural substrates for emotional symptoms, psychosis symptoms, and olfactory processing. Similarly, psychosis symptoms were also found to be strongly associated with cognitive decline in AD and MCI (Mallo et al., 2020). Overall, because psychosis symptoms heavily interact with abnormalities of olfactory and cognitive processing, their confounding effect should be considered when exploring the relationship between odor identification dysfunction and cognitive decline in patients with AD and MCI.
No significant association was observed between behavioral symptoms and odor identification, indicating that odor identification dysfunction may be independent of the presence of behavioral symptoms. Previous studies have suggested that affective symptoms are commonly observed from early stages of the disease (Craig et al., 2005) and that psychosis symptoms are more obvious at a more advanced AD stage (Piccininni et al., 2005), while behavioral symptoms are more often considered to be a transient symptom that fluctuates throughout disease progression (Garre-Olmo et al., 2010). On the other hand, behavioral symptoms are not linked to specific brain regions, and studies have suggested that they may be linked to dysfunctions in the posterior cingulate cortex, frontal cortex, and bilateral parietal lobes (Liu et al., 2004; Ng et al., 2021) which are less associated with olfactory processing. Thus, the effect of behavioral symptoms on cognitive decline and odor identification dysfunction is less significant or even undetectable compared to affective and psychosis symptoms.
The present study has limitations. (1) The results are based on cross-sectional analyses, and the causal relationships between olfactory dysfunction, NPS, and cognitive decline must be further explored in longitudinal studies. (2) The present study demonstrates that NPS mediated the relationship between cognitive decline and odor identification dysfunction, but whether they mediated the relationship between odor identification dysfunction and neurodegeneration needs to be confirmed by future studies involving, for example, the assessment of cerebrospinal fluid biomarkers and PET-CT. (3) The current study only involved an assessment of odor identification, because it is the strongest predictor of AD. However, significant associations between NPS and other aspects of olfaction (such as odor thresholds and odor discrimination) have also been reported in previous studies (Moberg et al., 2006; Pollatos et al., 2007; Chen et al., 2019). Future studies including odor thresholds and discrimination could provide a deeper understanding of how olfaction and NPS interact with each other in patients with AD/MCI. (4) Reduced self-awareness is associated with some NPS (disinhibition, apathy, anxiety, executive dysfunctions) in AD spectrum and a more aggressive progression of MCI and AD (Amanzio et al., 2011, 2020). Therefore, to minimize the effect of awareness, the present study used objective assessments rather than self-report scales for measuring odor identification, cognitive function, and NPS. However, we are not sure whether the awareness may mildly affect the result.
Conclusion
In summary, the current study demonstrates that NPS mediate the relationship between odor identification dysfunction and cognitive decline in patients with MCI and AD. When odor identification is used to predict cognitive decline in patients with AD and MCI, the confounding effect of affective symptoms and psychosis symptoms should be taken into account. Longitudinal studies must explore the causal relationships between olfactory dysfunction, NPS, and cognitive decline, and neuroimaging and CSF markers could better clarify the underlying mechanisms through which olfactory dysfunction, NPS, and cognitive decline interact with each other.
Data Availability Statement
The raw data supporting the conclusions of this article will be made available by the authors, without undue reservation, to any qualified researcher.
Ethics Statement
The studies involving human participants were reviewed and approved by the Ethics Committees of the Affiliated Brain Hospital of Guangzhou Medical University. The patients/participants provided their written informed consent to participate in this study. Written informed consent was obtained from the individual(s) for the publication of any potentially identifiable images or data included in this article.
Author Contributions
All authors contributed to the writing and revision of the manuscript, read, and approved the final manuscript.
Funding
This study was supported by a grant from the National Natural Science Foundation of China (No. 81701341); Guangzhou Municipal Psychiatric Diseases Clinical Transformation Laboratory (No. 201805010009); Key Laboratory for Innovation Platform Plan, the Science and Technology Program of Guangzhou, China, Science and Technology Plan Project of Guangdong Province (No. 2019B030316001); and National Key Research and Development Program of China (No. 2016YFC0906300). The funders had no role in the study design, data collection and analysis, decision to publish or preparation of the manuscript.
Conflict of Interest
The authors declare that the research was conducted in the absence of any commercial or financial relationships that could be construed as a potential conflict of interest.
Publisher's Note
All claims expressed in this article are solely those of the authors and do not necessarily represent those of their affiliated organizations, or those of the publisher, the editors and the reviewers. Any product that may be evaluated in this article, or claim that may be made by its manufacturer, is not guaranteed or endorsed by the publisher.
Acknowledgments
We thank Cong Ouyang, Weiru Zhang, Yinling Liu, and Chunying Dai for assisting in collecting the data. We are grateful for assistance from the Department of Neurology and the Department of Geriatric Psychiatry of the Affiliated Brain Hospital of Guangzhou Medical University (Guangzhou Huiai Hospital).
Abbreviations
Ab. Mot. Beh, aberrant motor behavior; AD, Alzheimer's disease; AVE, average variance extracted; AVLT, auditory verbal learning test; BNT, Boston naming test; CFA, confirmatory factor analysis; CFI, comparative fix index; CR, composite reliability; EFA, exploratory factor analysis; HCs, healthy controls; IFI, Bollen's incremental fit index; KMO, Kaiser–Meyer–Olkin; MCI, mild cognitive impairment; MMSE, mini-mental state examination; NFI, normed fit index; No-NPS, no neuropsychiatric symptoms; NPI, neuropsychiatric Inventory; NPS, neuropsychiatric symptoms; RMSEA, root mean square error of approximation; ROCF, ReyO-Sterrieth complex figure test; SDMT, symbol-digit modality test; SE, standard error; SEM, structural equation modeling; SRMR, standardized root mean square residual; TMT, trail-making test.
References
Amanzio, M., Bartoli, M., Cipriani, G. E., and Palermo, S. (2020). Executive dysfunction and reduced self-awareness in patients with neurological disorders. A mini-review. Front. Psychol. 11:1697. doi: 10.3389/fpsyg.2020.01697
Amanzio, M., Torta, D. M., Sacco, K., Cauda, F., D'agata, F., Duca, S., et al. (2011). Unawareness of deficits in Alzheimer's disease: role of the cingulate cortex. Brain 134, 1061–1076. doi: 10.1093/brain/awr020
Anderson, J. C., and Gerbing, D. W. (1988). Structural equation modeling in practice: a review and recommended two-step approach. Psychol. Bull. 103, 411–423. doi: 10.1037/0033-2909.103.3.411
Bagozzi, R. P., and Yi, Y. (1989). On the use of structural equation models in experimental designs. J. Market. Res. 26, 271–284. doi: 10.2307/3172900
Bahar-Fuchs, A., Chételat, G., Villemagne, V. L., Moss, S., Pike, K., Masters, C. L., et al. (2010). Olfactory deficits and amyloid-β burden in Alzheimer's disease, mild cognitive impairment, and healthy aging: a PiB PET study. J. Alzheimers. Dis. 22, 1081–1087. doi: 10.3233/jad-2010-100696
Ballanger, B., Bath, K. G., and Mandairon, N. (2019). Odorants: a tool to provide nonpharmacological intervention to reduce anxiety during normal and pathological aging. Neurobiol. Aging 82, 18–29. doi: 10.1016/j.neurobiolaging.2019.06.007
Baron, R. M., and Kenny, D. A. (1986). The moderator-mediator variable distinction in social psychological research: conceptual, strategic, and statistical considerations. J. Pers. Soc. Psychol. 51, 1173–1182. doi: 10.1037//0022-3514.51.6.1173
Birte-Antina, W., Ilona, C., Antje, H., and Thomas, H. (2018). Olfactory training with older people. Int. J. Geriatr. Psychiatry 33, 212–220. doi: 10.1002/gps.4725
Chen, B., Klarmann, R., Israel, M., Ning, Y., Colle, R., and Hummel, T. (2019). Difference of olfactory deficit in patients with acute episode of schizophrenia and major depressive episode. Schizophr. Res. 212, 99–106. doi: 10.1016/j.schres.2019.08.004
Chen, B., Zhong, X., Mai, N., Peng, Q., Wu, Z., Ouyang, C., et al. (2018). Cognitive impairment and structural abnormalities in late life depression with olfactory identification impairment: an Alzheimer's disease-like pattern. Int. J. Neuropsychopharmacol. 21, 640–648. doi: 10.1093/ijnp/pyy016
Chen, B., Zhong, X., Zhang, M., Mai, N., Wu, Z., Chen, X., et al. (2021). The additive effect of late-life depression and olfactory dysfunction on the risk of dementia was mediated by hypersynchronization of the hippocampus/fusiform gyrus. Transl. Psychiatry 11:172. doi: 10.1038/s41398-021-01291-0
Corcoran, C., Whitaker, A., Coleman, E., Fried, J., Feldman, J., Goudsmit, N., et al. (2005). Olfactory deficits, cognition and negative symptoms in early onset psychosis. Schizophr. Res. 80, 283–293. doi: 10.1016/j.schres.2005.07.028
Craig, D., Mirakhur, A., Hart, D. J., Mcilroy, S. P., and Passmore, A. P. (2005). A cross-sectional study of neuropsychiatric symptoms in 435 patients with Alzheimer's disease. Am. J. Geriatr. Psychiatry 13, 460–468. doi: 10.1176/appi.ajgp.13.6.460
Croy, I., and Hummel, T. (2017). Olfaction as a marker for depression. J. Neurol. 264, 631–638. doi: 10.1007/s00415-016-8227-8
Croy, I., Hummel, T., Pade, A., and Pade, J. (2010). Quality of life following nasal surgery. Laryngoscope 120, 826–831. doi: 10.1002/lary.20824
Cummings, J. L., Mega, M., Gray, K., Rosenberg-Thompson, S., Carusi, D. A., and Gornbein, J. (1994). The Neuropsychiatric Inventory: comprehensive assessment of psychopathology in dementia. Neurology 44, 2308–2314. doi: 10.1212/wnl.44.12.2308
Devanand, D. P., Lee, S., Manly, J., Andrews, H., Schupf, N., Doty, R. L., et al. (2015). Olfactory deficits predict cognitive decline and Alzheimer dementia in an urban community. Neurology 84, 182–189. doi: 10.1212/WNL.0000000000001132
Djordjevic, J., Jones-Gotman, M., De Sousa, K., and Chertkow, H. (2008). Olfaction in patients with mild cognitive impairment and Alzheimer's disease. Neurobiol. Aging 29, 693–706. doi: 10.1016/j.neurobiolaging.2006.11.014
Folstein, M. F., Folstein, S. E., and Mchugh, P. R. (1975). “Mini-mental state”. A practical method for grading the cognitive state of patients for the clinician. J Psychiatr. Res. 12, 189–198. doi: 10.1016/0022-3956(75)90026-6
Fornell, C., and Larcker, D. F. (1981). Evaluating structural equation models with unobservable variables and measurement error. J. Market. Res. 18, 39–50. doi: 10.2307/3151312
Gallagher, D., Fischer, C. E., and Iaboni, A. (2017). Neuropsychiatric symptoms in mild cognitive impairment. Can. J. Psychiatry 62, 161–169. doi: 10.1177/0706743716648296
Garre-Olmo, J., López-Pousa, S., Vilalta-Franch, J., De Gracia Blanco, M., and Vilarrasa, A. B. (2010). Grouping and trajectories of the neuropsychiatric symptoms in patients with Alzheimer's disease, part I: symptom clusters. J. Alzheimers. Dis. 22, 1157–1167. doi: 10.3233/jad-2010-101212
Gerlach, L. B., and Kales, H. C. (2018). Managing behavioral and psychological symptoms of dementia. Psychiatr. Clin. North Am. 41, 127–139. doi: 10.1016/j.psc.2017.10.010
Guo, Q., Chuanzhen, L., and Hong, Z. (2000). Application of Rey-Osterrieth complex figure test in Chinese normal old people. Chin. J. Clin. Psychol. 8, 205–207. doi: 10.16128/j.cnki.1005-3611.2000.04.003
Guo, Q.-H., Hong, Z., and Shi, W.-X. (2006). Boston naming test in Chinese elderly, patient with mild cognitive impairment and Alzheimer's dementia. Chin. Ment. Health J. 20, 81–84. doi: 10.3321/j.issn:1000-6729.2006.02.003
Hachinski, V. C., Iliff, L. D., Zilhka, E., Du Boulay, G. H., Mcallister, V. L., Marshall, J., et al. (1975). Cerebral blood flow in dementia. Arch. Neurol. 32, 632–637. doi: 10.1001/archneur.1975.00490510088009
Hayes, A. F.. (2009). Beyond Baron and Kenny: statistical mediation analysis in the new millennium. Commun. Monogr. 76, 408–420. doi: 10.1080/03637750903310360
Hollingworth, P., Hamshere, M. L., Moskvina, V., Dowzell, K., Moore, P. J., Foy, C., et al. (2006). Four components describe behavioral symptoms in 1,120 individuals with late-onset Alzheimer's disease. J. Am. Geriatr. Soc. 54, 1348–1354. doi: 10.1111/j.1532-5415.2006.00854.x
Hummel, T., Sekinger, B., Wolf, S. R., Pauli, E., and Kobal, G. (1997). ‘Sniffin' Sticks': olfactory performance assessed by the combined testing of odor identification, odor discrimination and olfactory threshold. Chem. Senses 22, 39–52. doi: 10.1093/chemse/22.1.39
Jöreskog, K. G., and Sörbom, D. (1982). Recent developments in structural equation modeling. J. Market. Res. 19, 404–416. doi: 10.1177/002224378201900402
Kales, H. C., Gitlin, L. N., and Lyketsos, C. G. (2015). Assessment and management of behavioral and psychological symptoms of dementia. BMJ 350:h369. doi: 10.1136/bmj.h369
Kamath, V., Lasutschinkow, P., Ishizuka, K., and Sawa, A. (2018). Olfactory functioning in first-episode psychosis. Schizophr. Bull. 44, 672–680. doi: 10.1093/schbul/sbx107
Kaufer, D. I., Cummings, J. L., Christine, D., Bray, T., Castellon, S., Masterman, D., et al. (1998). Assessing the impact of neuropsychiatric symptoms in Alzheimer's disease: the Neuropsychiatric Inventory Caregiver Distress Scale. J. Am. Geriatr. Soc. 46, 210–215. doi: 10.1111/j.1532-5415.1998.tb02542.x
La Buissonnière-Ariza, V., Lepore, F., Kojok, K. M., and Frasnelli, J. (2013). Increased odor detection speed in highly anxious healthy adults. Chem. Senses 38, 577–584. doi: 10.1093/chemse/bjt028
Lafaille-Magnan, M. E., Poirier, J., Etienne, P., Tremblay-Mercier, J., Frenette, J., Rosa-Neto, P., et al. (2017). Odor identification as a biomarker of preclinical AD in older adults at risk. Neurology 89, 327–335. doi: 10.1212/wnl.0000000000004159
Li, Q., Yang, D., Wang, J., Liu, L., Feng, G., Li, J., et al. (2015). Reduced amount of olfactory receptor neurons in the rat model of depression. Neurosci. Lett. 603, 48–54. doi: 10.1016/j.neulet.2015.07.007
Liu, W., Miller, B. L., Kramer, J. H., Rankin, K., Wyss-Coray, C., Gearhart, R., et al. (2004). Behavioral disorders in the frontal and temporal variants of frontotemporal dementia. Neurology 62, 742–748. doi: 10.1212/01.wnl.0000113729.77161.c9
Lu, J., Guo, Q., Hong, Z., Shi, W.-X., and Lv, C.-Z. (2006). Trail making test used by Chinese elderly patients with mild cognitive impairment and mild Alzheimer's dementia. Chin. J. Clin. Psychol. 14, 118–120. doi: 10.3969/j.issn.1005-3611.2006.02.003
Ma, L.. (2020). Depression, anxiety, and apathy in mild cognitive impairment: current perspectives. Front. Aging Neurosci. 12, 9. doi: 10.3389/fnagi.2020.00009
Mallo, S. C., Patten, S. B., Ismail, Z., Pereiro, A. X., Facal, D., Otero, C., et al. (2020). Does the neuropsychiatric inventory predict progression from mild cognitive impairment to dementia? A systematic review and meta-analysis. Ageing Res. Rev. 58:101004. doi: 10.1016/j.arr.2019.101004
Mckhann, G., Drachman, D., Folstein, M., Katzman, R., Price, D., and Stadlan, E. M. (1984). Clinical diagnosis of Alzheimer's disease: report of the NINCDS-ADRDA Work Group under the auspices of Department of Health and Human Services Task Force on Alzheimer's Disease. Neurology 34, 939–944.
Mineur, Y. S., Belzung, C., and Crusio, W. E. (2007). Functional implications of decreases in neurogenesis following chronic mild stress in mice. Neuroscience 150, 251–259. doi: 10.1016/j.neuroscience.2007.09.045
Moberg, P. J., Arnold, S. E., Doty, R. L., Gur, R. E., Balderston, C. C., Roalf, D. R., et al. (2006). Olfactory functioning in schizophrenia: relationship to clinical, neuropsychological, and volumetric MRI measures. J. Clin. Exp. Neuropsychol. 28, 1444–1461. doi: 10.1080/13803390500434409
Moberg, P. J., Kamath, V., Marchetto, D. M., Calkins, M. E., Doty, R. L., Hahn, C. G., et al. (2014). Meta-analysis of olfactory function in schizophrenia, first-degree family members, and youths at-risk for psychosis. Schizophr. Bull. 40, 50–59. doi: 10.1093/schbul/sbt049
Murphy, C.. (2019). Olfactory and other sensory impairments in Alzheimer disease. Nat. Rev. Neurol. 15, 11–24. doi: 10.1038/s41582-018-0097-5
Negoias, S., Hummel, T., Symmank, A., Schellong, J., Joraschky, P., and Croy, I. (2016). Olfactory bulb volume predicts therapeutic outcome in major depression disorder. Brain Imaging Behav. 10, 367–372. doi: 10.1007/s11682-015-9400-x
Ng, K. P., Pascoal, T. A., Mathotaarachchi, S., Chan, Y. H., Jiang, L., Therriault, J., et al. (2021). Neuropsychiatric symptoms are early indicators of an upcoming metabolic decline in Alzheimer's disease. Transl. Neurodegener. 10:1. doi: 10.1186/s40035-020-00225-y
Petersen, R. C.. (2004). Mild cognitive impairment as a diagnostic entity. J. Intern. Med. 256, 183–194. doi: 10.1111/j.1365-2796.2004.01388.x
Piccininni, M., Di Carlo, A., Baldereschi, M., Zaccara, G., and Inzitari, D. (2005). Behavioral and psychological symptoms in Alzheimer's disease: frequency and relationship with duration and severity of the disease. Dement. Geriatr. Cogn. Disord. 19, 276–281. doi: 10.1159/000084552
Pollatos, O., Albrecht, J., Kopietz, R., Linn, J., Schoepf, V., Kleemann, A. M., et al. (2007). Reduced olfactory sensitivity in subjects with depressive symptoms. J. Affect. Disord. 102, 101–108. doi: 10.1016/j.jad.2006.12.012
Randall, E., and Schumacker, R. G. L. (2010). A Beginner's Guide to Structural Equation Modeling. New York, NY: Routledge.
Roberts, R. O., Christianson, T. J. H., Kremers, W. K., Mielke, M. M., Machulda, M. M., Vassilaki, M., et al. (2016). Association between olfactory dysfunction and amnestic mild cognitive impairment and Alzheimer disease dementia. JAMA Neurol. 73, 93–101. doi: 10.1001/jamaneurol.2015.2952
Ropacki, S. A., and Jeste, D. V. (2005). Epidemiology of and risk factors for psychosis of Alzheimer's disease: a review of 55 studies published from 1990 to 2003. Am. J. Psychiatry 162, 2022–2030. doi: 10.1176/appi.ajp.162.11.2022
Seligman, S. C., Kamath, V., Giovannetti, T., Arnold, S. E., and Moberg, P. J. (2013). Olfaction and apathy in Alzheimer's disease, mild cognitive impairment, and healthy older adults. Aging Ment. Health 17, 564–570. doi: 10.1080/13607863.2013.768208
Sheridan, L. K., Fitzgerald, H. E., Adams, K. M., Nigg, J. T., Martel, M. M., Puttler, L. I., et al. (2006). Normative symbol digit modalities test performance in a community-based sample. Arch. Clin. Neuropsychol. 21, 23–28. doi: 10.1016/j.acn.2005.07.003
Takahashi, T., Nakamura, M., Sasabayashi, D., Komori, Y., Higuchi, Y., Nishikawa, Y., et al. (2018). Olfactory deficits in individuals at risk for psychosis and patients with schizophrenia: relationship with socio-cognitive functions and symptom severity. Eur. Arch. Psychiatry Clin. Neurosci. 268, 689–698. doi: 10.1007/s00406-017-0845-3
Tang, S. X., Moberg, P. J., Yi, J. J., Wiemken, A. S., Dress, E. M., Moore, T. M., et al. (2018). Olfactory deficits and psychosis-spectrum symptoms in 22q11.2 deletion syndrome. Schizophr. Res. 202, 113–119. doi: 10.1016/j.schres.2018.07.012
Taylor, A. B., Mackinnon, D. P., and Tein, J.-Y. (2008). Tests of the three-path mediated effect. Organ. Res. Methods 11, 241–269. doi: 10.1177/1094428107300344
Turetsky, B. I., Kohler, C. G., Gur, R. E., and Moberg, P. J. (2008). Olfactory physiological impairment in first-degree relatives of schizophrenia patients. Schizophr. Res. 102, 220–229. doi: 10.1016/j.schres.2008.03.013
Ugur, T., Weisbrod, M., Franzek, E., Pfuller, U., and Sauer, H. (2005). Olfactory impairment in monozygotic twins discordant for schizophrenia. Eur. Arch. Psychiatry Clin. Neurosci. 255, 94–98. doi: 10.1007/s00406-004-0536-8
Velayudhan, L.. (2015). Smell identification function and Alzheimer's disease: a selective review. Curr. Opin. Psychiatry 28, 173–179. doi: 10.1097/yco.0000000000000146
Wang, Q., Chen, B., Zhong, X., Zhou, H., Zhang, M., Mai, N., et al. (2021). Olfactory dysfunction is already present with subjective cognitive decline and deepens with disease severity in the Alzheimer's disease spectrum. J. Alzheimers. Dis. 79, 585–595. doi: 10.3233/JAD-201168
Woodward, M. R., Amrutkar, C. V., Shah, H. C., Benedict, R. H., Rajakrishnan, S., Doody, R. S., et al. (2017). Validation of olfactory deficit as a biomarker of Alzheimer disease. Neurol. Clin. Pract. 7, 5–14. doi: 10.1212/cpj.0000000000000293
Zhao, A., Li, Y., Yan, Y., Qiu, Y., Li, B., Xu, W., et al. (2020). Increased prediction value of biomarker combinations for the conversion of mild cognitive impairment to Alzheimer's dementia. Transl. Neurodegener. 9:30. doi: 10.1186/s40035-020-00210-5
Zhao, Q., Lv, Y., Zhou, Y., Hong, Z., and Guo, Q. (2012). Short-term delayed recall of auditory verbal learning test is equivalent to long-term delayed recall for identifying amnestic mild cognitive impairment. PLoS ONE 7:e51157. doi: 10.1371/journal.pone.0051157
Keywords: neuropsychiatric symptoms, odor identification, cognition, Alzheimer's disease, mild cognitive impairment, structural equation modeling
Citation: Wang Q, Chen B, Zhong X, Zhou H, Zhang M, Mai N, Wu Z, Chen X, Yang M, Zhang S, lin G, Hummel T and Ning Y (2022) Neuropsychiatric Symptoms Mediated the Relationship Between Odor Identification and Cognition in Alzheimer's Disease Spectrum: A Structural Equation Model Analysis. Front. Aging Neurosci. 13:732840. doi: 10.3389/fnagi.2021.732840
Received: 29 June 2021; Accepted: 06 December 2021;
Published: 12 January 2022.
Edited by:
Rosalba Morese, University of Italian Switzerland, SwitzerlandReviewed by:
Paulo Giusti Rossi, University of São Paulo, BrazilSara Palermo, Fondazione IRCCS Istituto Neurologico Carlo Besta, Italy
Copyright © 2022 Wang, Chen, Zhong, Zhou, Zhang, Mai, Wu, Chen, Yang, Zhang, lin, Hummel and Ning. This is an open-access article distributed under the terms of the Creative Commons Attribution License (CC BY). The use, distribution or reproduction in other forums is permitted, provided the original author(s) and the copyright owner(s) are credited and that the original publication in this journal is cited, in accordance with accepted academic practice. No use, distribution or reproduction is permitted which does not comply with these terms.
*Correspondence: Yuping Ning, ningjeny@126.com
†These authors have contributed equally to this work