Electroencephalographic Measurement on Post-stroke Sensory Deficiency in Response to Non-painful Cold Stimulation
- 1Department of Biomedical Engineering, The Hong Kong Polytechnic University, Hong Kong, Hong Kong SAR, China
- 2Institute of Textiles and Clothing, The Hong Kong Polytechnic University, Hong Kong, Hong Kong SAR, China
- 3Beijing Key Laboratory of Rehabilitation Technical Aids for Old-Age Disability, National Research Centre for Rehabilitation Technical Aids Beijing, Beijing, China
- 4University Research Facility in Behavioral and Systems Neuroscience (UBSN), The Hong Kong Polytechnic University, Hong Kong, Hong Kong SAR, China
- 5The Hong Kong Polytechnic University Shenzhen Research Institute, Shenzhen, China
- 6Research Institute for Smart Ageing (RISA), The Hong Kong Polytechnic University, Hong Kong, Hong Kong SAR, China
Background: Reduced elementary somatosensation is common after stroke. However, the measurement of elementary sensation is frequently overlooked in traditional clinical assessments, and has not been evaluated objectively at the cortical level. This study designed a new configuration for the measurement of post-stroke elementary thermal sensation by non-painful cold stimulation (NPCS). The post-stroke cortical responses were then investigated during elementary NPCS on sensory deficiency via electroencephalography (EEG) when compared with unimpaired persons.
Method: Twelve individuals with chronic stroke and fifteen unimpaired controls were recruited. A 64-channel EEG system was used to investigate the post-stroke cortical responses objectively during the NPCS. A subjective questionnaire of cold sensory intensity was also administered via a numeric visual analog scale (VAS). Three water samples with different temperatures (i.e., 25, 10, and 0°C) were applied to the skin surface of the ventral forearm for 3 s via glass beaker, with a randomized sequence on either the left or right forearm of a participant. EEG relative spectral power (RSP) and topography were used to evaluate the neural responses toward NPCS with respect to the independent factors of stimulation side and temperature.
Results: For unimpaired controls, NPCS initiated significant RSP variations, mainly located in the theta band with the highest discriminative resolution on the different temperatures (P < 0.001). For stroke participants, the distribution of significant RSP spread across all EEG frequency bands and the temperature discrimination was lower than that observed in unimpaired participants (P < 0.05). EEG topography showed that the NPCS could activate extensive and bilateral sensory cortical areas after stroke. Significant group differences on RSP intensities were obtained in each EEG band (P < 0.05). Meanwhile, significant asymmetry cortical responses in RSP toward different upper limbs were observed during the NPCS in both unimpaired controls and participants with stroke (P < 0.05). No difference was found between the groups in the VAS ratings of the different temperatures (P > 0.05).
Conclusion: The post-stroke cortical responses during NPCS on sensory deficiency were characterized by the wide distribution of representative RSP bands, lowered resolution toward different temperatures, and extensive activated sensory cortical areas.
Introduction
Reduced somatosensation is common after stroke, with one in two stroke survivors experiencing this sensory deficit (Carey et al., 2018). It mainly impairs their discrimination in sensations of thermal, touch, and pain and further affects their ability of functional independence and overall quality of life (Carey, 1995; Kessner et al., 2016). Due to the learned non-use of the sensory affected limb, post-stroke motor restoration was reported to be hindered by the impaired somatosensation, which is typically represented by slower recovery, reduced movement control, and even lesser rehabilitation outcomes (Wu et al., 2006; De Diego et al., 2013; Serrada et al., 2019). Despite the high prevalence and apparent importance of somatosensation in post-stroke motor restoration, sensory deficiency and its functional evaluation received little attention, compared to those of the motor function (De Diego et al., 2013; Carey et al., 2016; Serrada et al., 2019).
To evaluate the sensory impairment, quantitative and precise measurement of somatosensory functions is crucial. It is known that the somatosensory functions could be divided into two subtypes, the elementary and the intermediate (Takeda et al., 2000; Satoh et al., 2002; Dijkerman and De Haan, 2007; Matsuda et al., 2020). The elementary somatosensory functions mainly consist of light touch, pain, thermal sensation, joint position sense, and vibration sense (Matsuda et al., 2020). The intermediate somatosensory functions are usually the integration of the multiple tactile related functions, including 2-point discrimination, tactile localization, weight, texture, and shape perceptions (Matsuda et al., 2020). The traditional clinical practices on post-stroke sensory evaluation were mainly focused on the intermediate somatosensation because it is generally believed that brain lesions, e.g., stroke, or brain trauma, mainly leads to the impairment of intermediate somatosensation (Head and Holmes, 1911; Takeda et al., 2000) rather than the elementary ones. For instance, the two-point discrimination (Dellon et al., 1987) and the Semmes-Weinstein monofilament tests (Bell-Krotoski and Tomancik, 1987) are two typical assessments widely used in clinical practice for multiple integrated sensory functions. Recently, evidence was found that brain lesions also led to disturbances of the elementary somatosensation. For example, infarction at the postcentral gyrus would cause disturbance of thermal sensation (Satoh et al., 2002). However, the measurement of elementary sensation is underdeveloped in traditional clinical sensory assessments. A challenge is the configuration of the measurement with minimum introduction of other unrelated sensory inputs, when performing an elementary somatosensory evaluation. In the current clinical measurements for stroke rehabilitation, both elementary and intermediate somatosensory functions, as well as the motor functions, are usually evaluated in a mixture related to daily tasks, e.g., the Fugl-Meyer Assessment (FMA) (Fugl-Meyer et al., 1974). Among them, somatosensory measurements are oversimplified in these mixed scales with limited resolution by subjective ordinal scales (Dellon et al., 1987). For example, although the Rivermead assessment of somatosensory performance contains the item on thermal sensation, it only has two grades of “warm” and “cold,” and requires the intact cognition of a subject to give subjective verbal response in the measurement as in most of the clinical somatosensory assessments (Steimann et al., 2012). Therefore, objective measurements with more precise control on the stimulation to evoke elementary somatosensory responses are preferred in the understanding of the related impairment after stroke.
The cortical responses to external sensory inputs could be objectively captured by brain imaging and electrophysiological technologies, e.g., functional magnetic resonance imaging (fMRI) and electroencephalography (EEG). fMRI has been applied in exploration of tactile, thermal, and pain stimulations to unimpaired persons with the advantage of its high spatial resolution (Chen et al., 2002; Mazzola et al., 2012). Relatively static spatial segregations in relation to these sensory inputs were observed (Chen et al., 2002; Mazzola et al., 2012). However, fMRI is hard to detect transient responses because of its lower temporal resolution compared to other modalities, e.g., EEG (Lystad and Pollard, 2009; Caliandro et al., 2017). EEG features represented by somatosensory evoked potential (SSEP) in time domain (Misra et al., 2008) and selected frequency components in EEG power spectra (Ahn et al., 2016) have been applied in objective measurement on cortical responses to sensory stimulations. SSEP parameters, e.g., onset value, peak intensity, duration, etc., have been widely used to determine the afferent neural integrity (Hwang et al., 2016; Yoon et al., 2018), based on the detection of the whole SSEP waveform with high repetitions to achieve a high signal-to-noise ratio (SNR) (Society, 2015). On the other hand, frequency features in the EEG power spectra, e.g., alpha and beta powers, could be less affected by the background noises to demonstrate the cortical responses to the sensory inputs, because of the less overlapped distribution between the selected power bands and those of the noises in the frequency domain (Thut and Miniussi, 2009). Therefore, EEG frequency features could be obtained with less repetitions, compared to those SSEP detections in the time domain (Thut and Miniussi, 2009). EEG spectral patterns also have been introduced to investigate the neural activities during the touch (Singh et al., 2014; Ahn et al., 2016) and thermal (Chang et al., 2005; Shao et al., 2012) stimulations in both unimpaired and stroke persons. Based on the study conducted by Singh et al. (2014), beta oscillation was found to be highly related to touch sensations, and its power changes could differentiate pleasant stimuli from unpleasant stimuli with different textile fabrics on a single trial basis. However, similar to the clinical manual assessments, the configurations in cortical measurements of elementary sensations were mixed with intermediate somatosensation in the reported EEG and fMRI studies (Kwan et al., 2000; Tseng et al., 2010; Mazzola et al., 2012; Ansari et al., 2018), e.g., the application of contact thermode with fastening belts for thermal stimulation to the skin would introduce multiple tactile and pressure stimuli, besides the thermal stimulation in the experiment (Kwan et al., 2000; Tseng et al., 2010).
In this study, we designed a new configuration for the measurement of post-stroke elementary thermal sensation by non-painful cold stimulation (NPCS), with minimized introduction of other sensations, such as tactile sensations with different textures and shapes. During thermal stimulation, pain sensation could be triggered and mixed with the thermal sensation according to an individual’s temperature threshold and the stimulus duration (Chang et al., 2005). Additionally, heat stimulation without pain (warm) is prone to make one drowsy, disturbing the cortical responses to the sensory inputs and lowering the efficiency of cortical network processing (Slater, 2008). Therefore, NPCS was selected to investigate the elementary thermal sensation in this study, with the purpose of minimizing the involvements of elementary pain sensation and potential disturbance from sleepiness. Then, we used quantitative EEG power spectra to investigate the cortical responses during the elementary NPCS on post-stroke sensory deficiency, when compared with unimpaired individuals.
Methods
In this study, the post-stroke neural responses during the NPCS were investigated using EEG. A visual analog scale (VAS) questionnaire was administered to collect the subjective perceptions of cold stimulation. Three water samples, each at a different temperature, were applied to the forearm via glass beakers to induce the NPCSs in both stroke survivors and unimpaired individuals, with minimized disturbance of other sensory inputs. EEG power spectra and topography were used to evaluate the neural responses toward NPCSs.
Participants
Before conducting the experiment, an approval was obtained from the Human Subjects Ethics Sub-Committee of the Hong Kong Polytechnic University. Twelve participants from local districts who entered the chronic stage after stroke were recruited to the “stroke group.” The following inclusion criteria were applied: (1) at least 6 months post-onset of a singular and unilateral brain lesion due to stroke; (2) the stroke-induced lesions occurred in the subcortical area; (3) an absence of visual, cognitive or attention deficits that would prevent participants from following instructions or performing the experimental procedures (assessed using the Mini-Mental State Examination (MMSE) score > 21) (Roselli et al., 2009); (4) presence of moderate-to-severe motor disability in the paretic limb [13 < Fugl-Meyer Assessment (FMA) < 47 (Fugl-Meyer et al., 1974; Woytowicz et al., 2016)] and muscle spasticity at the wrist and elbow [Modified Ashworth Score (MAS) ≤ 2 (Bohannon and Smith, 1987)]; (5) moderate-to-severe sensory impairment of their affected forearm, with a score of 1 as measured by the sensation part of light touch in FMA (Fugl-Meyer et al., 1974); (6) without symptom of central post-stroke pain (CPSP). The rationale for recruiting persons with chronic stroke was that their sensorimotor functions were relatively stable and were representative of a large population of stroke survivors. Meanwhile, to ensure the accessibility of cortical EEG responses, particularly those of the sensorimotor cortex, subjects with stroke and lesions in subcortical areas were recruited, since their cerebral cortex were not directly impaired due to stroke. Stroke patients with CPSP (overall prevalence around 6–8%; Krause et al., 2016) were excluded from this study to minimize bias, because patients with CPSP frequently show impairments of thermal and pain sensations that are different from patients without CPSP (Krause et al., 2016). The stroke group was subdivided into the “stroke affected group” and the “stroke unaffected group” according to the hemiplegic sides of the participants with stroke.
Fifteen unimpaired adults from local districts were recruited to the “control group.” They had no history of neurological, psychiatric, cognitive and/or cardiovascular diseases. All recruited participants gave written consent before the start of the experiment. The demographic characteristics of the participants are presented in Table 1. In this study, the recruited participants were mainly middle aged [45–65 years-old (Dictionary, 2016)], with a mean age of 55.13 years for the stroke group and 46.40 years for the control group. The difference in age was not statistically significant (p = 0.192, t = 1.341, independent t-test). However, significant difference in gender was found between the stroke participants and unimpaired controls (p = 0.005, Fisher’s exact test). In addition, all unimpaired participants and stroke survivors before their stroke onset were right-handed.
Electroencephalography Measurement of Cortical Response During Non-painful Cold Stimulation
Experimental Setup
The experiment was conducted in a lab which was controlled on temperature and humidity. The room temperature was maintained at 25 ± 1°C and the relative humidity was fixed at 60 ± 5%. Figure 1A shows the experimental setup. Each participant was comfortably seated in front of a table with both forearms placed on the table. Each participant wore an eye mask and ear plugs to minimize the disturbances from visual and audio stimuli from the surroundings. A 64-channel EEG system (BP-01830, Brain Products Inc.) was mounted on the scalp of each participant based on the standard 10–20 system, which was used to record the whole brain EEG with the skin impedance of each channel under 5 KΩ (Nuwer et al., 1999). The whole brain EEG recording was adopted because cold stimulation could activate multiple brain cortices in addition to the primary somatosensory cortex (Singh et al., 2014). The recording sampling frequency was set at 1,000 Hz.
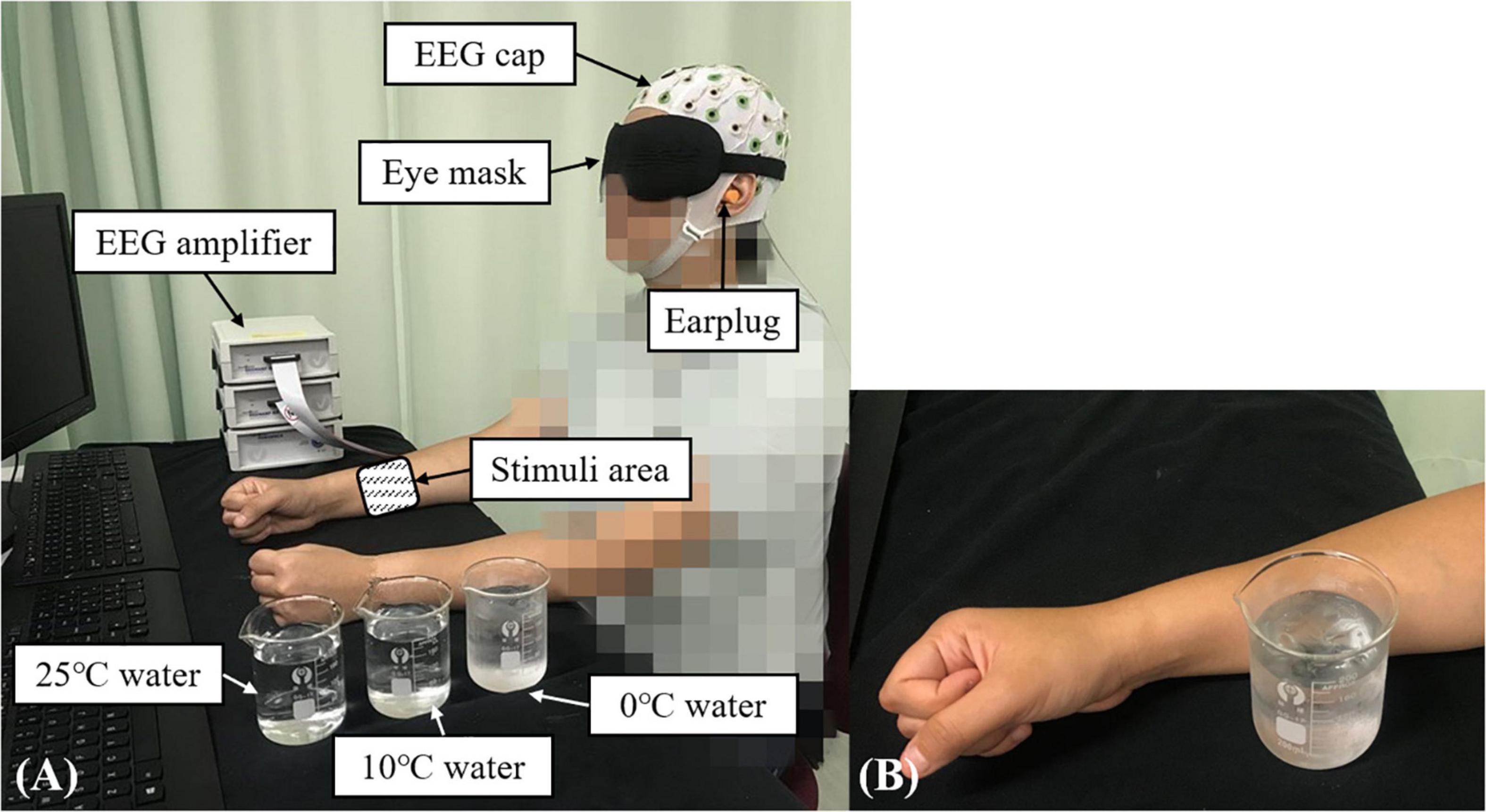
Figure 1. The experimental setup for the EEG evaluation during the non-painful cold stimulation (NPCS): (A) participant in the recording position; (B) demonstration of the NPCS.
Non-painful Cold Stimulation
In this study, the configuration for the measurement of post-stroke elementary thermal sensation using NPCS is shown in Figure 1B via glass beaker with different temperatures of water. During the stimulation, the glass beaker was designed to statically attach to the skin surface without friction, or load of weight. The selection of glass beaker was mainly due to its smooth surface and the monocomponent in the material, and these features could minimize the unnecessary sensory inputs of tactile sensations with different textures and shapes, when compared with the contact thermode fastened by belts used in the literature (Kwan et al., 2000; Tseng et al., 2010). The static attachment without friction and weight loading during the stimulation could further limit the introduction of friction sensation and weight perception. The NPCSs were delivered by three water samples with different temperatures of 25, 10, and 0°C, and the three temperatures could provoke weak to strong non-painful cold sensations, respectively. In the clinical application, 25°C usually acts as the adaptation temperature for control. The 10°C is frequently used for cool stimulation (Shao et al., 2012; Ansari et al., 2018), where the 0°C is a typical temperature for cold-induced pain. As mentioned above, pain sensation is related with the intensity and duration of the thermal stimulation (Chang et al., 2005). In unimpaired adults, exposing the skin to 0°C will evoke cold pain in 5 s (Rebbeck et al., 2015). To avoid the cold pain during the 0°C water stimulus, a 3-s short stimulation duration was used in this study. The target temperature was achieved by mixing room temperature water and different quantities of ice. The 0°C water was maintained as a mixture of ice and water during the whole experiment. Before applying each stimulus, a thermometer was used to ensure that the water temperature was within ±0.1°C of the target temperature. The NPCS with different temperatures was delivered to the skin surface of ventral forearm around the muscle belly of the flexor carpi radialis (FCR) and flexor digitorum (FD) muscles by the same experimenter. The FCR and FD muscles were selected because they were not only a common area to use in studies investigating the cold sensation for unimpaired participants (Chang et al., 2005), but also related to the wrist-hand flexion, which could promote post-stroke upper limb sensorimotor restoration for wrist-hand joints with appropriate stimulation (Lin et al., 2017). The estimated size of the contact area was around 3 cm × 3 cm.
Electroencephalography Measurement Protocol
The timeline of EEG measurement during the NPCS is summarized in Figure 2. Each single trial of EEG measurement consisted of a 30-s baseline test, and three 3-s cold stimuli, which were separated by two 90-s resting times. Each participant was asked to keep their eyes closed, place both forearms on the table while remaining relaxed and still (Figure 1A). During the baseline test, no stimulation was applied to the participants, and they were required to remain awake without mental activity. During each NPCS, the glass beaker with a target temperature was statically attached to the skin surface around the FCR and FD muscles (Figure 1B), i.e., without friction, for 3 s. There was a computer program with timer to instruct the operator to deliver each NPCS. The operator would receive beep sounds though a headphone for instructions to start and stop. A countdown of the instruction would also be displayed on the computer screen as a reminder. Whether the stimulus was placed on the participant’s right or left forearm was determined by a randomized sequence. Participants were asked not to perform active cognition toward the cold stimuli during the EEG recording. The 90-s duration of rest was selected based upon the average clearance of cortical responses to cold stimulation in unimpaired persons (Garkavenko et al., 2008; Shao et al., 2012; De Pascalis et al., 2019). The cycles of EEG measurement for each participant were repeated three times on each forearm. The neural responses of both forearms were evaluated because the post-stroke cortical responses between different sides were varied during both sensory and motor tasks. This could be due to the different handedness before the stroke (Özcan et al., 2005; Dirnberger et al., 2011), and this asymmetric cortical response was also observed in our previous work in post-stroke fine touch to textile fabrics (Huang et al., 2020).
Electroencephalography Data Processing
After acquiring the targeted EEG data, the signals were processed off-line with a band-pass filter from 0.1 to 100 Hz, and a notch filter from 49 to 51 Hz to eliminate the 50 Hz noise from the environment. Following this, the EEG signals were divided into individual segments containing the baseline and cold stimulation periods. Then, the relative powers of each EEG frequency band, were calculated [i.e., Delta (δ, 0.1–4 Hz), Theta (θ, 4–8 Hz), Alpha (α, 8–13 Hz), Beta (β, 13–30 Hz), and Gamma (γ, 30–100 Hz) (Lebeau, 2010; Ahmed et al., 2012)]. The following equation was used:
where, the PRelative/band is the relative spectral power (RSP) of a frequency band; p(f) is the power spectral density of an EEG segment for a cold stimulation event, which is estimated by Fast Fourier Transform. F1 and F2 are the cutoff frequencies of a EEG frequency band, as stated above; and pBaseline(f) is the power spectral density of the EEG segments in the baseline tests of each trial. Similar analysis methods were adopted in our previous study (Huang et al., 2020).
Non-painful Cold Sensation Evaluated by Questionnaire
The subjective evaluation of sensory intensities to the three cold stimuli were assessed using a numeric visual analog scale (VAS, range from 0 to 10), and it was conducted half an hour after the EEG measurement. Scores of 0–5 indicate no sensation to maximum non-painful cold sensation, while 6–10 score denotes minimum to maximum cold pain. During the VAS questionnaire, a non-painful cold stimulus was applied to the participants’ skin surface around the FCR and FD muscles, i.e., without friction, for 3 s. After removing the stimulus, participants were asked to rate the intensity of the cold sensation caused by the applied stimulus. The sequence for each cold stimulus was randomized. The subjective evaluation of cold sensations was also repeated three times on each forearm. Both forearms were assessed for the stroke participants, while only the dominant forearm (the right forearm in this study) was assessed for those unimpaired participants.
Statistical Analysis
The normality tests for the EEG data and VAS scores were first evaluated using the Lilliefors method (Razali and Wah, 2011); the probabilities were statistically insignificant (P > 0.05), and the data obeyed normal distribution. During the intragroup comparisons, two-way repeated ANOVA was used to evaluate the RSP differences with respect to the independent factors of the stimulation side (i.e., left side and right side) and temperature (i.e., 25, 10, and 0°C) for each EEG frequency band. Then an independent t-test was used to evaluate the RSP differences with respect to the independent factor of stimulation side. One-way repeated measure ANOVA was conducted to investigate the RSP differences with respect to the independent factor of temperature with Bonferroni post-hoc test. During the intergroup comparisons, RSP differences of each EEG frequency band for each stimulation side with respect to the independent factors of the group and temperature were evaluated via mixed-model repeated measure ANOVA. Following this, one-way ANOVA was conducted to compare the group differences of RSPs on each stimulation side, with Bonferroni post-hoc test or Dunnett’s T3 post-hoc test. The selection of which post-hoc test to use was based on the homogeneity of the variance test. Specifically, for equal variance, the Bonferroni post-hoc test was adopted, otherwise, Dunnett’s T3 post-hoc test was used (Shingala and Rajyaguru, 2015). In addition, the intragroup VAS differences with respect to the independent factor of temperature were evaluated by one-way repeated measure ANOVA, while the intergroup differences of the VAS scores were calculated by one-way ANOVA, and both of them used Bonferroni post-hoc test. In this work, the level of statistical significance was set at 0.05, and the significance at levels 0.01 and 0.001 were also indicated.
Results
Figure 3 shows the EEG RSPs in response to the cold stimuli for each group at different EEG frequency bands. Table 2 presents the two-way repeated ANOVA probabilities and predicted effect sizes (EFs) with respect to the independent factors of the stimulation side and temperature. Table 3 provides a summary of the detailed values, means, and 95% CIs for each RSP, in addition to the one-way repeated ANOVA and independent t-test probabilities and EFs. In the control group (Figure 3A), significant RSP differences with respect to the temperatures were detected at all EEG frequency bands (P < 0.001, two-way repeated ANOVA, Table 2). In terms of the stimulation sides, significant differences were observed in the delta (P = 0.047, two-way repeated ANOVA, Table 2) and alpha (P = 0.001, two-way repeated ANOVA, Table 2) bands. Significant interactions between the temperature and the stimulation side were detected in the alpha (P = 0.042, two-way repeated ANOVA, Table 2) and gamma (P < 0.001, two-way repeated ANOVA, Table 2) bands. The theta RSPs of both sides in response to the 0°C water were significantly higher than those for 25 and 10°C stimuli (P < 0.001, one-way repeated ANOVA, Table 3), and the theta RSPs of both sides to 10°C water were significantly higher than those for 25°C water (P < 0.001, one-way repeated ANOVA, Table 3). The alpha and beta RSPs in response to 25°C were significantly lower than those in response to the 10 and 0°C water when the right side was stimulated (P < 0.001, one-way repeated ANOVA, Table 3). When the left side was stimulated, the beta and gamma RSPs in response to 0°C were found to be significantly higher than those at the 10 and 25°C water (P < 0.001, one-way repeated ANOVA, Table 3). Meanwhile, independent t-test established significant RSP differences in the delta, alpha, beta, and gamma bands for the different stimulation sides (P < 0.05, Table 3). The RSP of the right side was significantly lower than the left side in the delta band, while significantly higher in the alpha (P < 0.01, independent t-test, Table 3) and beta (P < 0.05, independent t-test, Table 3) bands. The gamma RSP for the right side was significantly higher than that for the left side when stimulated by the 10°C water (P = 0.012, independent t-test, Table 3), and significantly lower when stimulated by the 0°C water (P = 0.004, independent t-test, Table 3).
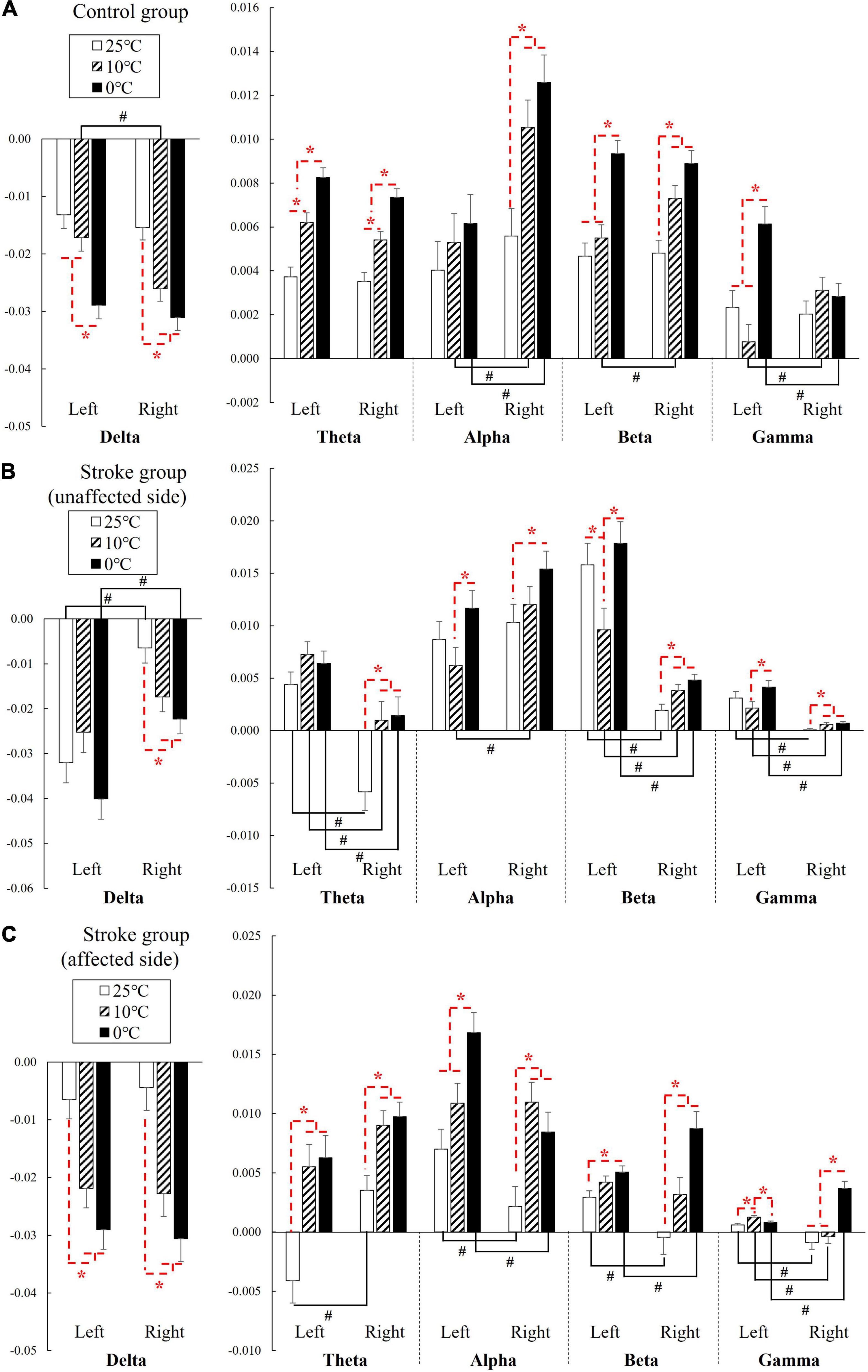
Figure 3. The EEG relative spectral power in response to the NPCSs on the left and right forearms for (A) control group, (B) stroke group-unaffected side, and (C) stroke group-affected side on the whole brain at the delta, theta, alpha, beta, and gamma band presented as mean value with SE (error bar). The significant intragroup differences are indicated by “*” (p < 0.05, one-way repeated ANOVA with Bonferroni post-hoc tests), and the significant intergroup differences are indicated by “#” (p < 0.05, independent t-test).
In the stroke unaffected group (Figure 3B), two-way repeated ANOVA identified significant RSP differences with respect to the temperatures observed at all EEG frequency bands (P < 0.01, Table 2). Meanwhile, significant differences with respect to the stimulation sides were detected at the delta, beta, and gamma bands (P < 0.01, two-way repeated ANOVA, Table 2). In terms of the interaction between the temperature and the stimulation side, significances were found at the delta, alpha, beta, and gamma bands (P < 0.05, two-way repeated ANOVA, Table 2). One-way repeated ANOVA showed the RSPs of 25°C in the theta, beta, and gamma bands were significantly lower than that of 10 and 0°C for right-side stimulation (P < 0.01, Table 3). When the left side was stimulated, the significant variations between the RSPs of 10 and 0°C were observed in the alpha, beta, and gamma bands (P < 0.05, one-way repeated ANOVA, Table 3). Furthermore, significant differences were detected in all EEG frequency bands for the stroke unaffected group in respect of stimulation sides (P < 0.05, independent t-test, Table 3). Furthermore, the RSPs of the right side were significantly higher in the delta and alpha bands (P < 0.05, independent t-test, Table 3), and lower in theta, beta, and gamma bands (P < 0.05, independent t-test, Table 3), when compared with the left side.
In the stroke affected group (Figure 3C), significant differences with respect to the stimulation sides were detected at the theta (P = 0.019, two-way repeated ANOVA, Table 2) and gamma (P = 0.002, two-way repeated ANOVA, Table 2) bands. In terms of the temperature factor, significant differences were observed in all EEG frequency bands (P < 0.001, two-way repeated ANOVA, Table 2). No significant interactions between the temperature and the stimulation side was captured (P > 0.05, two-way repeated ANOVA, Table 2). One-way repeated ANOVA showed significant RSP variations in the delta (P < 0.001, one-way repeated ANOVA, Table 3), theta (P < 0.001, one-way repeated ANOVA, Table 3) and gamma (P = 0.001, one-way repeated ANOVA, Table 3) bands when the left side was stimulated with 25 and 10°C water. Additionally, significant RSP variations between the 25 and 0°C were found at the delta (P < 0.001, one-way repeated ANOVA, Table 3), theta (P < 0.001, one-way repeated ANOVA, Table 3), alpha (P < 0.001, one-way repeated ANOVA, Table 3), and beta (P = 0.006, one-way repeated ANOVA, Table 3) bands. When the right side was stimulated, the 25°C RSPs were significantly lower than those RSPs of 10 and 0°C in the theta, alpha, and beta bands (P < 0.001, one-way repeated ANOVA, Table 3). The gamma RSP for 0°C was significantly higher than that of both 25 and 10°C (P < 0.001, one-way repeated ANOVA, Table 3). Furthermore, there were significant RSP variations between the right side and the left side at theta, alpha, beta, and gamma bands, as determined by the independent t-test (P < 0.05, Table 3).
Figure 4 compares the group differences of RSPs in response to the cold stimuli at each EEG frequency band with respect to the stimulation sides. Table 4 displays the values of statistical results including probabilities and EFs of the mixed-model repeated measure ANOVA and one-way ANOVA. Significant differences between both factors of groups and temperatures were found in the mixed-model repeated measure ANOVA for all the EEG frequency bands (P < 0.05, Table 4) on both stimulation sides. Significant interactions between the temperature factor and the group were recorded at delta and alpha band on the left side (P < 0.05, mixed-model repeated measure ANOVA, Table 4), as well as the theta, beta, and gamma bands on both sides (P < 0.05, mixed-model repeated measure ANOVA, Table 4). In comparison to the control group, when the left side was stimulated, the RSPs of the stroke affected group were significantly lower at the theta, beta, and gamma bands (P ≤ 0.001, one-way ANOVA, Table 4), and higher in the alpha band (P = 0.001, one-way ANOVA, Table 4). Meanwhile, when the right side was stimulated, the RSPs of the stroke affected group were significantly lower at the beta (P < 0.001, one-way ANOVA, Table 4) and gamma (P = 0.002, one-way ANOVA, Table 4) bands, and higher in the delta and theta bands (P < 0.001, one-way ANOVA, Table 4). Furthermore, significant RSP differences between the stroke affected group and the stroke unaffected group were noted in response to different temperatures across all frequency bands (P < 0.05, one-way ANOVA, Table 4). During the right-side stimulation, the alpha RSP of the stroke affected group was significantly lower than that of the unaffected group (P < 0.001, one-way ANOVA, Table 4), while the theta and gamma RSPs of the stroke affected group were significantly higher than those of the unaffected group (P < 0.05, one-way ANOVA, Table 4). During the left-side stimulation, the RSPs of the stroke affected group at theta, beta, and gamma bands were significantly lower than those of the unaffected group (P < 0.001, one-way ANOVA, Table 4), while the alpha RSPs of the stroke affected group were significantly higher than that of the unaffected group (P < 0.001, one-way ANOVA, Table 4). A comparison of the RSP values of the control group and the stroke unaffected group revealed significant differences in the theta, alpha, beta, and gamma bands (P ≤ 0.001, one-way ANOVA, Table 4) during the right-side stimulation. Similarly, significant differences were found in the delta, beta, and gamma bands (P < 0.05, one-way ANOVA, Table 4) during the left-side stimulation.
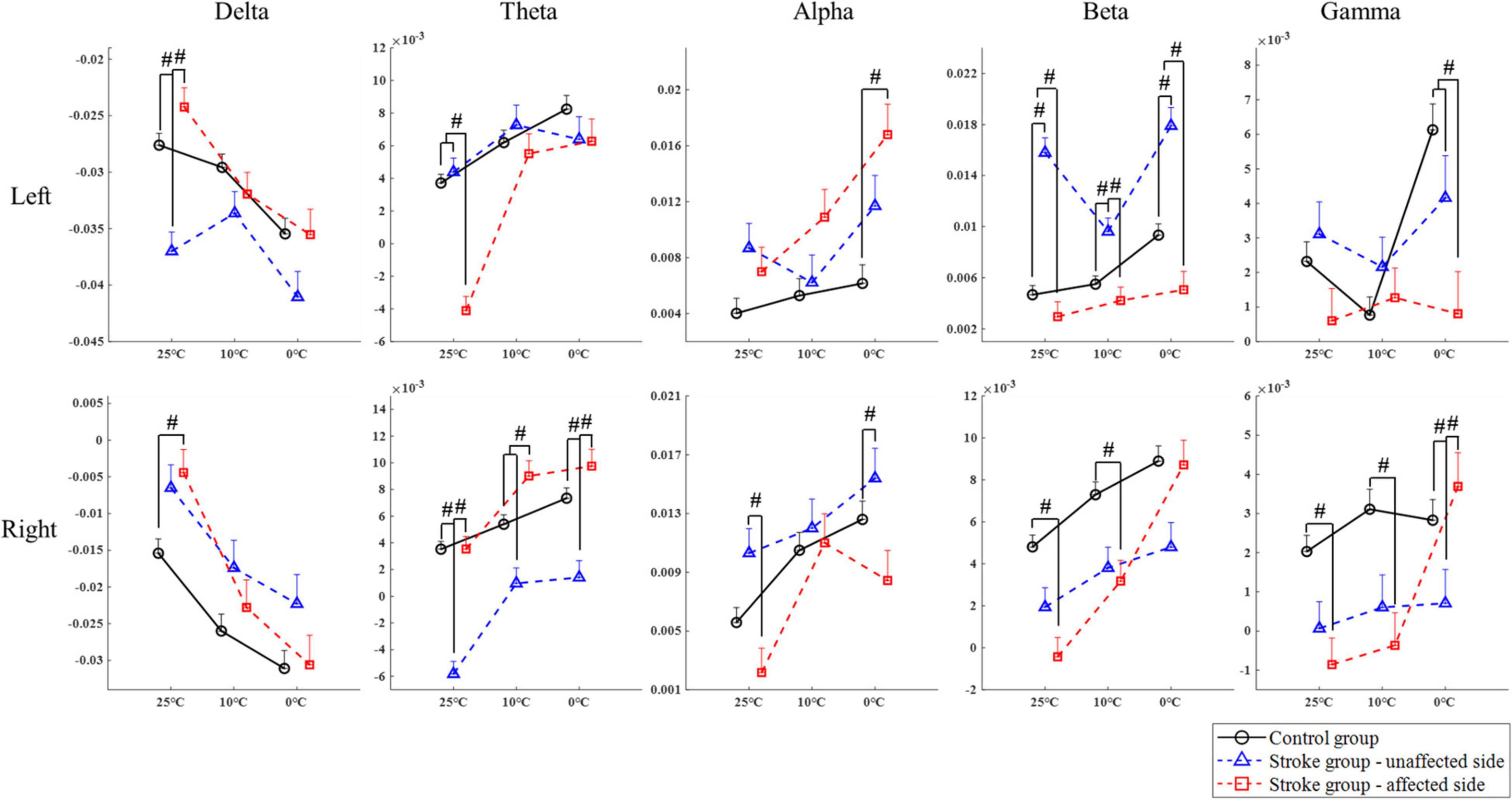
Figure 4. The EEG overall relative spectral power in response to the non-painful cold stimuli on the left and right forearms for each group at the delta, theta, alpha, beta, and gamma band, respectively, presented as mean value with SE (error bar). The significant intergroup differences are indicated by “#” (P < 0.05, one-way ANOVA with Bonferroni post-hoc tests or Dunnett’s T3 post-hoc tests).
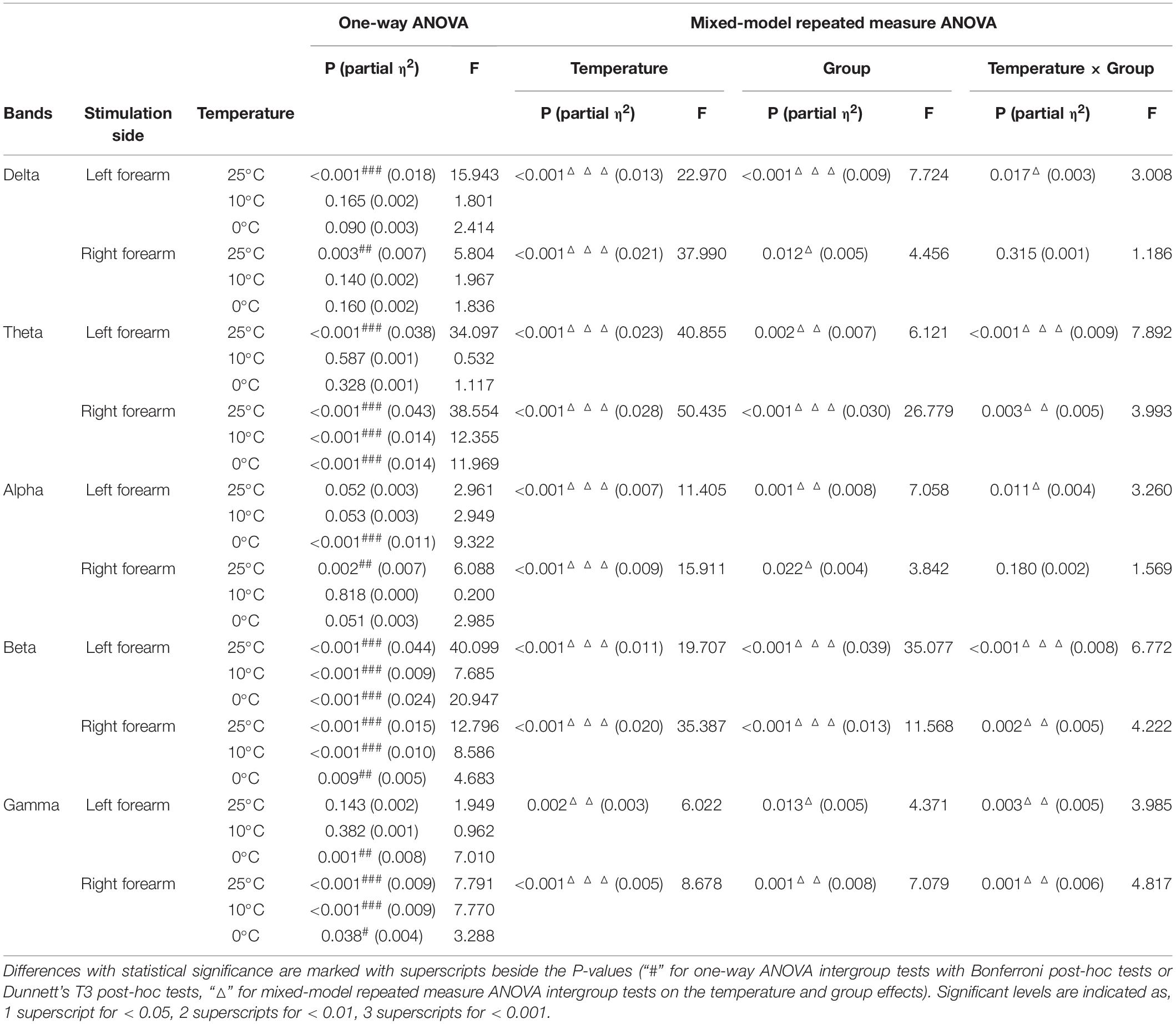
Table 4. Intergroup comparisons on the whole brain relative spectral power of each EEG frequency band.
Figure 5 demonstrates the whole brain EEG topography of the mean RSPs in all EEG frequency bands for each group with respect to the independent factors of temperature and stimulation side. Hotspots of significant RSPs in all EEG frequency bands were captured bilaterally, mainly in the parietal, frontal, and occipital regions of both stroke and unimpaired participants. In the control group, there were increased theta power (Figure 5B) in the parietal and occipital regions bilaterally. The powers of high frequency bands (i.e., beta and gamma bands, Figures 5D,E) were increased not only in the parietal and occipital regions, but also in the occipital lobe. For stroke participants who had experienced right-side brain lesions (left hemiplegia), theta activity was increased in the bilateral parietal areas and decreased in the paramedian central area for both stimulation sides. Brain activity in the beta band (Figure 5D) only slightly increased in the bilateral parietal regions for both stimulation sides. In contrast, for participants with stroke who had experienced left-side brain lesions (right hemiplegia), theta activity (Figure 5B) increased in the left hemisphere of the brain when the unaffected left forearms were stimulated, while a remarkable increase in theta activity could be observed over the frontal, parietal, and occipital lobes when their affected right forearms were stimulated. In terms of the high frequency bands (i.e., beta and gamma bands, Figures 5D,E), brain activity in the central areas were significantly increased for the affected right forearms, where the 0°C stimulus initiated greater brain activity than the 25 and 10°C stimuli. Meanwhile, high frequency brain activities increased significantly almost over the whole brain when the unaffected left forearms were stimulated.
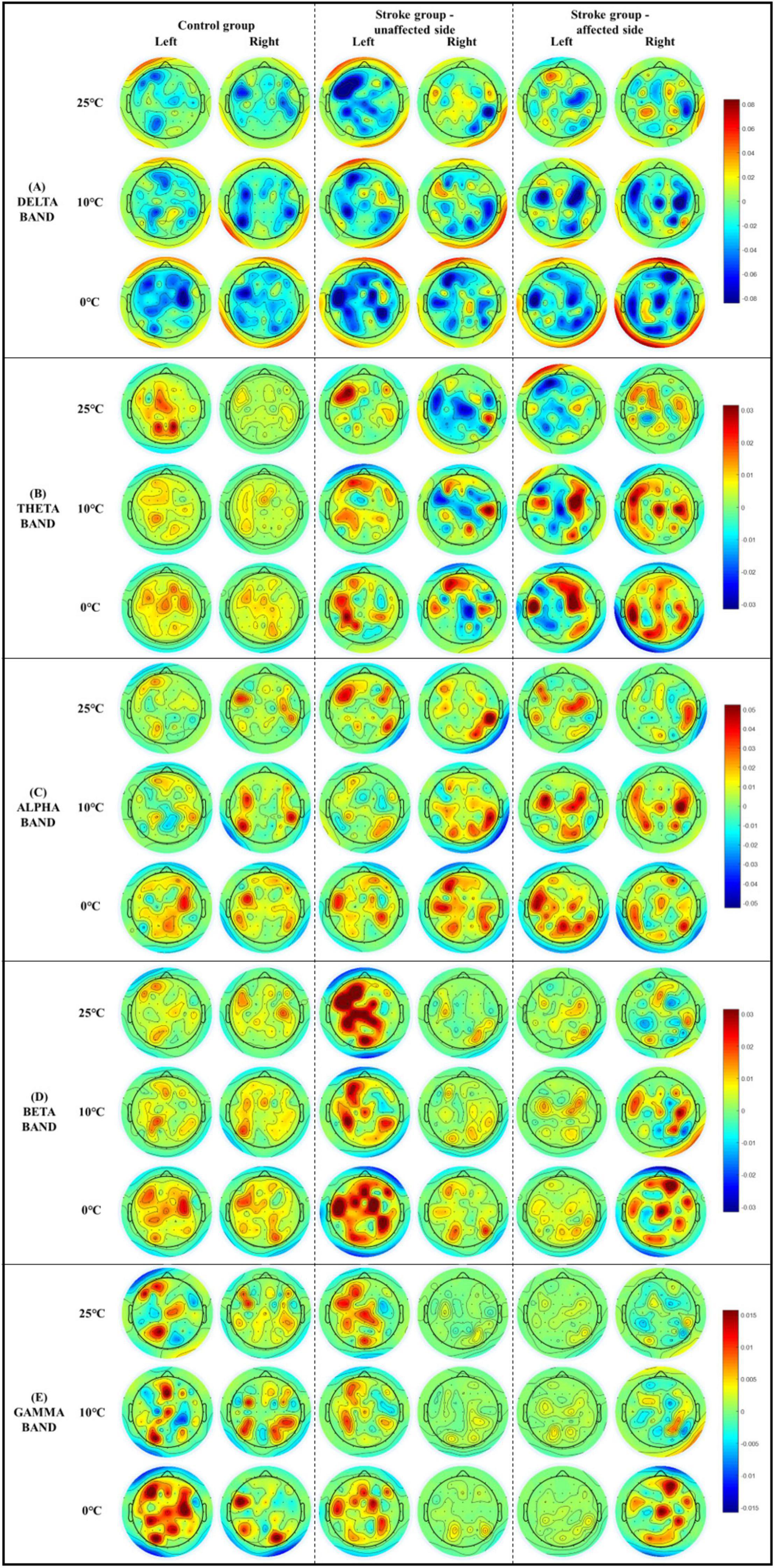
Figure 5. The whole brain EEG topography on the mean relative spectral power of (A) delta, (B) theta, (C) alpha, (D) beta, (E) gamma in response to the non-painful cold stimuli with respect to the stimulation side.
Table 5 presents the means and 95% CIs of each NPCS evaluated by VAS, in addition to the statistical probabilities and the EFs. The results demonstrated that the three temperatures of cold water could provoke weak to strong non-painful cold sensations in both stroke and unimpaired participants. Significant VAS variations with respect to the different temperatures were observed for each group via one-way repeated ANOVA (P < 0.001, Table 5). No statistical difference was found in the intergroup comparison for each cold stimulus (P > 0.05, one-way ANOVA, Table 5).
Discussion
This study designed a novel configuration for the measurement of post-stroke elementary thermal sensation with NPCS. We utilized glass beaker with different temperatures of water, which statically attached to the skin surface without friction and load, to deliver the NPCSs with different intensities. In the literature, cold stimuli were mainly delivered by thermal pack (Mazzola et al., 2012; Ansari et al., 2018), thermode (Kwan et al., 2000; Tseng et al., 2010), or direct contact with the water (Maihöfner et al., 2002; Fardo et al., 2017), and these approaches unavoidably introduced mixed sensory inputs other than the thermal sensation. For example, direct contact with the water would mix the thermal sensation and the wetness sensation, while thermal pack and thermode with fasteners would introduce the intermediate texture, shape, or even weight perceptions. In comparison with those configurations in the literature, the involvements of other unrelated sensory inputs like texture, shape, wetness, and weight perceptions were effectively minimized with the configuration of this study. Meanwhile, the pain sensation was also avoided in the configuration with the precise 3 s stimulus duration. The NPCSs of this study were further verified by the VAS questionnaire (Table 5), where the VAS scores of all stroke and unimpaired participants were lower than 6 indicating without cold pain.
We measured the post-stroke sensory deficiency on the elementary non-painful cold sensation using both EEG RSP and VAS questionnaire. The VAS scores indicated that both stroke and unimpaired participants could distinguish the different NPCSs with statistical significance (Table 5). However, there was no significant intergroup difference in VAS scores between the stroke and unimpaired participants (Table 5), which could not further assess the post-stroke sensory alteration on thermal sensation. The insignificant intergroup VAS result could be due to the compensation of voluntary cognition toward sensory inputs for those stroke participants, leading to high thermal discrimination (Barulli and Stern, 2013). During the VAS questionnaire, participants were asked to rate the cold sensation provoked by each NPCS. This cognitive process could be related to the individual experiences, and mainly consisted of four primary elements including comprehension, retrieval, judgment, and response (Sudman et al., 1997; Tourangeau et al., 2000). On the contrary, significant group differences in cortical responses were found between the stroke and unimpaired groups toward different NPCSs. That is because the cortical responses captured by EEG RSPs were associated with involuntary attention drawn by the NPCS. During the EEG recording, to minimize the bias caused by the participants’ active cognitive process, the participants were requested to remain awake yet mentally inactive. Therefore, the EEG RSP could reveal much more preliminary cortical response on the post-stroke sensory deficiency than the compensated cognitive process during the VAS questionnaire rating.
The EEG results of the unimpaired participants (Figure 3A) highlighted that different NPCSs could induce significant RSP variations across the target frequency bands (i.e., theta, alpha, and beta bands). These three bands were activated with increased power during the NPCS. In line with our observations of enhanced EEG beta RSP in both fronto-temporal and parietal brain regions (Figure 5D), it has been reported in the literature that beta activity increased bilaterally in the fronto-temporal cortex in unimpaired people during the cold stimulation, especially during the cold pain (Chang et al., 2002; De Pascalis et al., 2019). Chang et al. (2002) suggested that the increased beta power was related to the frontal and temporal muscle activities in response to cold stimulation, caused by situational tension factors like stress or pain. The increased beta activities were then suspected to associate with residual muscle artifact, as EEG artifact. However, the increased beta power of this study was mainly located in sensorimotor cortex (Figure 5D), not closely to the frontal or temporal muscles, which could not be accounted as muscle artifact. In addition, the present results also found that beta RSP intensities were increased with the increase of stimulus intensities. De Pascalis et al. (2019) reported a similar observation during the cold pain, and they found that the higher EEG beta power in the left frontal, midline central, posterior temporal cortices were correlated with the higher cold pain scores (De Pascalis et al., 2019). Together with the increased beta power during the NPCS in this work, it implied that the beta power could be proportional to the intensities of cold stimulations whether it was painful or non-painful. It was also observed that both theta and alpha RSPs were increased during the NPCS. It could be the involuntary attention aroused during the non-painful thermal stimulation, i.e., outside events attract spontaneous attentional processes (Eimer et al., 1996; Iannetti et al., 2008; Wang et al., 2010), for example, to process the different NPCSs in this work. Chen et al. (2017) reported that the intensity of attentive oscillation (i.e., theta and alpha bands) increased with the difficulty of sensory tasks. The attentive oscillation would be relatively low when processing an easy task, while its intensity would be higher when a difficult task lasts for a longer duration to obtain more information (Chen et al., 2017). These are consistent with our findings (Figure 3A), among the three stimuli, the 0°C stimulus obtained the highest RSP intensity, and the RSP of 10°C was higher than that of the 25°C stimulus. Of the three representative frequency bands, the theta band showed the highest sensitivity in distinguishing different cold stimuli in unimpaired participants, because theta RSP obtained statistical differences between three cold stimuli for both stimulation sides. The limited sensitivity toward cold stimuli in the alpha and beta RSPs were possibly due to the dominance of the theta band during involuntary attention evoked by the sensory stimulation, as designed in this work.
Post-stroke neural responses of the elementary cold sensation induced by NPCS were also investigated for both affected and unaffected sides in the stroke participants. In the stroke unaffected group, the sensitive EEG RSPs of distinguishing different cold stimuli were concentrated in the beta and gamma bands (Figure 3B). Varied with the control group, the theta band was no longer the dominant frequency band toward cold stimuli in the stroke unaffected group, and it only remained limited resolution to different temperatures during the right-side stimulation. Instead, significant variations were observed in beta and gamma RSPs during the NPCS on both stimulation sides, whereas its thermal resolution was also limited. For example, both beta and gamma RSPs of the right side could not distinguish the difference between the 10°C and the 0°C water. The sensory deficiency in the unaffected upper limb of stroke survivors was frequently overlooked in routine stroke clinical practice. Recently, it has been pointed out that sensorimotor deficits frequently occurring in the unaffected upper limb were mainly due to the transcallosal interactions between the hemispheres (Grefkes and Fink, 2011), and decreased sensory discrimination is one of the representative symptoms for sensory deficiency in the unaffected upper limb. In this study, the reduced sensory discrimination toward elementary cold sensation for the unaffected side of the stroke participants was captured.
For the stroke affected group, in comparison with both unimpaired participants and the unaffected side of stroke survivors, a wider distribution of representative RSP across the whole spectrum toward NPCSs was observed, and all EEG frequency bands showed significant RSP differences toward the different cold stimuli (Figure 3C). These broadly activated EEG frequency responses could be related to the excessive cortical effort after stroke. It indicated that the desired sensory responses were challenging for chronic stroke survivors, even after the recovery period of pathological reorganization (Thibaut et al., 2017). It was widely reported that excessive cortical effort was required in post-stroke motor recovery when a task became more complex (Jones and Adkins, 2015; Thibaut et al., 2017). In this work, we observed the similar additional cortical effort in the sensory experiences during the NPCS. Meanwhile, although significant RSP variations toward different cold stimuli could be obtained in EEG frequency bands for the affected sides, their thermal resolutions on different temperatures represented by EEG RSP were low. For example, in unimpaired participants, the theta RSP demonstrated the highest sensitivity in distinguishing the three cold stimuli on both sides, while the theta RSP did not show a significant difference between the 10°C and the 0°C water on both sides for the stroke affected group. Another finding related to the power spectrum was the involvement of high frequency gamma band during the NPCS to the affected limbs of the stroke participants (Figure 3C). It could be due to the compensation of the impaired afferent proprioception and efferent control in sensorimotor system (Chen et al., 2018). Increased unconscious employments of higher-level attention and behavioral processes were usually observed in stroke survivors, when compared with the unimpaired individuals (Bannister et al., 2015; Chen et al., 2018). Previous studies also highlighted the contributions of post-stroke gamma activation, i.e., contributed to cognitive motor tasks (Gross and Gotman, 1999; Meador et al., 2002), and acted as a coding feature for functional prevalence in hand sensory areas (Tecchio et al., 2003, 2004). In our previous studies on post-stroke sensory deficiency during mechanical tactile stimulation (i.e., textile fabric stimulations), similar engagements of high frequency bands (i.e., beta and gamma bands) were observed in persons with chronic stroke (Huang et al., 2020). It further indicated the importance of gamma oscillation for somatosensory functions, including thermal and tactile sensations, following a stroke.
We also investigated the variations of neural responses during the NPCS between the left and right sides for each group. These variations were mainly investigated from two aspects, which included the RSP intensity and its resolution. From the resolution aspect, the RSP resolutions of the control and stroke affected group toward different cold stimuli between the left and right sides were comparable (Figures 3A,C). From the intensity aspect, the control group obtained significantly higher RSP intensities in the relative high frequency bands (i.e., alpha, beta, and gamma bands) during the right-side stimulation than left-side stimulation (Figure 3A). This could be related to the higher amplitude of cortical potentials during the dominant hand movement, in comparison with the non-dominant hand (Tarkka and Hallett, 1990). In this study, all the unimpaired participants were right-handed. In the stroke affected group, the right-side stimulation achieved significant higher RSP intensities in the relative high frequency bands (i.e., alpha, beta, and gamma bands) during the strong NPCS (i.e., 0°C) than the left-side stimulation, while the right-side stimulation obtained significant lower RSP intensities during the weak NPCS (i.e., 25°C) (Figure 3C). It might imply that the affected left forearm is more sensitive to a weak stimulus, where the affected right forearm is more sensitive to a high stimulus. This particular finding could be related to the lateralization of brain function, which result in the differences of behavior and recovery outcome between the left hemiplegia and right hemiplegia (Chen et al., 2000; Park and Lee, 2011; Frenkel-Toledo et al., 2019). For example, patients with left-hemisphere stroke often develop a slow and cautious behavioral style; on the contrary, those patients with right-hemisphere stroke usually present a quick and overly curious behavior (Hayn and Fisher, 1997). Owing to the superiority of controlling complex motor movements in the left hemisphere (Serrien and Spapé, 2009), it was also reported that patients with right hemiplegia had slower motor restoration in gait functions (Laufer et al., 2003; Gama et al., 2017). However, few works have been done on the differences in sensory impairment after the left- and right-hemisphere lesions, which necessitate further investigations (Goodin et al., 2018).
When it comes to the stroke unaffected group, asymmetric neural responses from both aspects were observed in all EEG frequency bands, especially in the high frequency bands (i.e., beta and gamma bands, Figures 3B, 5). Firstly, the thermal resolution of different temperatures for right unaffected forearms was higher than those of the left. In the theta band, significant RSP variation toward different cold stimuli was only observed during the right-side stimulation. In the gamma band, the RSP of the right forearm could significantly distinguish the 25°C stimulus from the other two stimuli, where the RSP of the left forearm could not. Secondly, the RSP intensities (theta, beta, and gamma bands) were significantly higher for the left unaffected forearms than the right ones (Figures 3B, 5). This excessive neural response suggested that additional cortical efforts were needed during the neural processing of thermal inputs for the unaffected left forearms. The imbalance of neural responses toward thermal stimulation for the stroke unaffected group could be related to the handedness alteration after stroke. Typically, dominant hands have better sensorimotor performance during single-handed tasks, like better manual dexterity during motor movements, and higher sensation thresholds for touch and pain than non-dominant hands (Özcan et al., 2005; Dirnberger et al., 2011). Most stroke survivors will use their unaffected forearms to perform sensorimotor tasks in their daily lives after stroke. In some stroke survivors with affected right dominant forearms, the unaffected left forearms will gradually become their new dominant upper limbs (Harris and Eng, 2006). However, it can be difficult for stroke survivors during the handedness alteration, with the unaffected left forearms presenting low sensation resolution and poor control over voluntary motor tasks, compared to their original dominant right arms. In this study, all stroke survivors were right-handed before their stroke onset. Among them, half were right hemiplegics, who had experienced handedness alteration after stroke. The RSP results of this study further verified the thermal sensitivity during the NPCS for the unaffected left forearm was lower than the unaffected right ones. In line with our previous study (Huang et al., 2020), the unaffected right forearms also showed better touch discrimination to the different fabrics during the tactile stimulation when compared with the unaffected left forearms.
Significant intergroup differences of RSP intensities were also observed (Figure 4). Significant variation on RSP intensity between the stroke unaffected group and control group was mainly captured in the beta band (Figure 4). When the left sides were stimulated, the intensity of beta RSP in the unaffected group was significantly higher than that in the control group. This might also be related to the alteration in handedness after stroke. When compared with the control group, the stroke affected group displayed sensory deficiency related to attenuated cortical responses during the weak cold stimuli (i.e., 10 and 25°C). When the right side was stimulated with the 10 and 25°C water, the RSP intensities of high frequency bands (i.e., beta and gamma bands, Figure 4) in the stroke affected group were significantly lower than the control group; however, the RSP intensities between the two groups were comparable when stimulated by 0°C. The cortical activation in the stroke affected group was relatively low during the processing of weak to moderate NPCSs (i.e., 10 and 25°C), while strong NPCS (i.e., 0°C stimulus) could initiate high cortical activation. It implied that the reduced somatosensation after stroke was mainly characterized as the insensitivity to those weak sensory inputs and their discriminations.
The sensory cortical centers in response to elementary thermal stimulation for both stroke and unimpaired participants were revealed by the whole-brain RSP topography (Figure 5). For those unimpaired participants, multiple brain regions were activated bilaterally during the NPCS, which included the primary sensorimotor cortex, insula cortex, occipital cortex, prefrontal, and frontal cortices. These findings were in line with the literature (Chang et al., 2002, 2005; De Pascalis et al., 2019), and further verified the cortical processing of thermal stimulation was a distributed system, rather than a strict somatotopic arrangement (Berman et al., 1998; Chang et al., 2005). In several fMRI studies, innocuous thermal-related activations in the anterior cingulate cortex and lateral-thalamus pathway were also reported (Davis et al., 1998; Kwan et al., 2000; Mazzola et al., 2012). For stroke survivors, the brain cortices that process non-painful cold sensation were also activated bilaterally in multiple brain regions as seen in unimpaired individuals, where the activated regions were more extensive with higher intensity. Similar distribution of post-stroke cortical activation after thermal stimulation was reported by one fMRI study (Chen et al., 2020). They observed that brain activations promoted by thermal stimuli were in the inferior-middle frontal cortex, superior-middle temporal cortex, insula, and primary sensorimotor cortex (Chen et al., 2020).
One of the concerns in the EEG measurements is the high signal to noise ratio (SNR) to ensure the signal quality, and it could be achieved by ensemble mean via repetitions (Väisänen and Malmivuo, 2009). In this study, we preferred EEG power spectra to investigate the cortical responses to NPCS, since it requires less repetition or signal length than the measurement of intact waveform of SSEP in the time domain. It is because the frequency components are less affected by the background noises, e.g., environmental noise (50 Hz power line) and spontaneous EEG unrelated to the stimulation, as the target EEG frequency features may not be overlapped with the noise features in the spectrum (Thut and Miniussi, 2009). For instance, the SSEP waveform detection usually requires averaging over 250–1,000 repetitions to obtain a reasonable SNR (Society, 2015), while Singh et al. (2014) only used a single trial to achieve the significant power changes between the pleasant and unpleasant touch stimuli.
The small sample size was one of the limitations of this study. However, under the current small sample size, significant differences in EEG RSP between the unimpaired population and the chronic stroke population had been obtained. Another limitation of this study was the significant gender differences between the two groups (p = 0.005, Fisher exact test, Table 1). In this study, we focused on the investigation of the impairment in elementary thermal sensation introduced by the neurological lesion after stroke, rather than the difference of gender. Gender differences in post-stroke sensory impairment, e.g., thermal sensation, has not been investigated yet. In future work, more stroke participants in different recovery stages, gender, age groups, and lesion sites will be investigated for a more comprehensive understanding.
Conclusion
The contribution of this study was the objective and quantitative evaluation of post-stroke elementary thermal impairment toward non-painful cold stimulation via EEG RSP analysis, with a new configuration minimizing the unnecessary sensory inputs. The characteristics of cortical responses during the NPCS following a stroke were (1) wide distribution of representative RSP bands, (2) limited sensitivity toward different thermal stimuli, (3) more extensive sensory cortical areas, when compared with the EEG RSP features of the unimpaired control. Meanwhile, the post-stroke somatosensation of unaffected side was also impaired, which was represented by lower resolution toward different NPCSs and asymmetric RSP intensities toward different stimulation sides. EEG RSP pattern could quantify the neural responses of elementary thermal sensation after stroke, and enhance the understanding of post-stroke sensory deficiency on elementary thermal sensation.
Data Availability Statement
The datasets presented in this article are not readily available because it has been stated in the consent approved by the Human Subjects Ethics Sub-Committee of the Hong Kong Polytechnic University that the results of the experiment may be published, but the individual results should be kept confidentially for each subject. Requests to access the datasets should be directed to XLH, xiaoling.hu@polyu.edu.hk.
Ethics Statement
The studies involving human participants were reviewed and approved by the Human Subjects Ethics Sub-Committee of the Hong Kong Polytechnic University. The patients/participants provided their written informed consent to participate in this study.
Author Contributions
YHH contributed to the experimental design, data analysis, and manuscript drafting. JYH and ZYL contributed to the experimental design. JJ, CCH, ZQL, and YY contributed to the subject management and experimental process. XLH conceived of the study and coordinated the whole project, including the study design, human subject experiments, and manuscript revising. All authors read and approved the final manuscript.
Funding
This project was funded by the National Natural Science Foundation of China (NSFC 81771959), University Grants Committee Research Grants Council, Hong Kong (GRF 15207120), and Science and Technology Innovation Committee of Shenzhen, China (2021Szvup142).
Conflict of Interest
The authors declare that the research was conducted in the absence of any commercial or financial relationships that could be construed as a potential conflict of interest.
Publisher’s Note
All claims expressed in this article are solely those of the authors and do not necessarily represent those of their affiliated organizations, or those of the publisher, the editors and the reviewers. Any product that may be evaluated in this article, or claim that may be made by its manufacturer, is not guaranteed or endorsed by the publisher.
Acknowledgments
We would like to thank the participants of this study.
References
Ahmed, S. A., Rani, D. E., and Sattar, S. A. (2012). Alpha activity in EEG and intelligence. Int. J. Adv. Inf. Technol. 2:27. doi: 10.5121/ijait.2012.2103
Ahn, S.-N., Lee, J.-W., and Hwang, S. (2016). Tactile perception for stroke induce changes in electroencephalography. Hong Kong J. Occupat. Therapy 28, 1–6. doi: 10.1016/j.hkjot.2016.10.001
Ansari, Y., Remaud, A., and Tremblay, F. (2018). Variations in corticomotor excitability in response to distal focal thermal stimulation. Somatosens Mot. Res. 35, 69–79. doi: 10.1080/08990220.2018.1460263
Bannister, L. C., Crewther, S. G., Gavrilescu, M., and Carey, L. M. (2015). Improvement in touch sensation after stroke is associated with resting functional connectivity changes. Front. Neurol. 6:165–165. doi: 10.3389/fneur.2015.00165
Barulli, D., and Stern, Y. (2013). Efficiency, capacity, compensation, maintenance, plasticity: emerging concepts in cognitive reserve. Trends Cogn. Sci. 17, 502–509. doi: 10.1016/j.tics.2013.08.012
Bell-Krotoski, J., and Tomancik, E. (1987). The repeatability of testing with semmes-weinstein monofilaments. J. Hand Surgery 12, 155–161. doi: 10.1016/S0363-5023(87)80189-2
Berman, H. H., Kim, K. H., Talati, A., and Hirsch, J. (1998). Representation of nociceptive stimuli in primary sensory cortex. Neuroreport 9, 4179–4187. doi: 10.1097/00001756-199812210-00033
Bohannon, R. W., and Smith, M. B. (1987). Interrater reliability of a modified Ashworth scale of muscle spasticity. Physical Therapy 67, 206–207. doi: 10.1093/ptj/67.2.206
Caliandro, P., Vecchio, F., Miraglia, F., Reale, G., Della Marca, G., La Torre, G., et al. (2017). Small-world characteristics of cortical connectivity changes in acute stroke. Neurorehabilitation Neural Repair 31, 81–94. doi: 10.1177/1545968316662525
Carey, L. M. (1995). Somatosensory Loss after Stroke. Crit. Rev. Phys. Rehabil. Med 7, 51–91. doi: 10.1615/CritRevPhysRehabilMed.v7.i1.40
Carey, L. M., Lamp, G., and Turville, M. (2016). The state-of-the-science on somatosensory function and its impact on daily life in adults and older adults, and following stroke: a scoping review. OTJR (Thorofare N J) 36, 27s–41s. doi: 10.1177/1539449216643941
Carey, L. M., Matyas, T. A., and Baum, C. (2018). Effects of somatosensory impairment on participation after stroke. Am. J. Occup. Ther. 72, 7203205100p7203205101-7203205100p7203205110. doi: 10.5014/ajot.2018.025114
Chang, P. F., Arendt-Nielsen, L., and Chen, A. C. (2002). Dynamic changes and spatial correlation of EEG activities during cold pressor test in man. Brain Res. Bull. 57, 667–675. doi: 10.1016/s0361-9230(01)00763-8
Chang, P. F., Arendt-Nielsen, L., and Chen, A. C. (2005). Comparative cerebral responses to non-painful warm vs. cold stimuli in man: EEG power spectra and coherence. Int J Psychophysiol 55, 73–83. doi: 10.1016/j.ijpsycho.2004.06.006
Chen, A., Wang, A., Wang, T., Tang, X., and Zhang, M. (2017). Behavioral oscillations in visual attention modulated by task difficulty. Front. Psychol. 8:1630. doi: 10.3389/fpsyg.2017.01630
Chen, C.-L., Tang, F.-T., Chen, H.-C., Chung, C.-Y., and Wong, M.-K. (2000). Brain lesion size and location: Effects on motor recovery and functional outcome in stroke patients. Archiv. Physical Med. Rehabil. 81, 447–452. doi: 10.1053/mr.2000.3837
Chen, J.-I., Ha, B., Bushnell, M. C., Pike, B., and Duncan, G. H. (2002). Differentiating noxious-and innocuous-related activation of human somatosensory cortices using temporal analysis of fMRI. J. Neurophysiology 88, 464–474. doi: 10.1152/jn.2002.88.1.464
Chen, S. C.-J., Hsu, M.-J., Kuo, Y.-T., Lin, R.-T., Lo, S.-K., and Lin, J.-H. (2020). Immediate effects of noxious and innocuous thermal stimulation on brain activation in patients with stroke. Medicine 99:e19386. doi: 10.1097/MD.0000000000019386
Chen, X., Xie, P., Zhang, Y., Chen, Y., Cheng, S., and Zhang, L. (2018). Abnormal functional corticomuscular coupling after stroke. NeuroImage Clin. 19, 147–159. doi: 10.1016/j.nicl.2018.04.004
Davis, K. D., Kwan, C. L., Crawley, A. P., and Mikulis, D. J. (1998). Functional MRI study of thalamic and cortical activations evoked by cutaneous heat, cold, and tactile stimuli. J. Neurophysiology 80, 1533–1546. doi: 10.1152/jn.1998.80.3.1533
De Diego, C., Puig, S., and Navarro, X. (2013). A sensorimotor stimulation program for rehabilitation of chronic stroke patients. Restor. Neurol. Neurosci. 31, 361–371. doi: 10.3233/rnn-120250
De Pascalis, V., Scacchia, P., Papi, B., and Corr, P. J. (2019). Changes of EEG band oscillations to tonic cold pain and the behavioral inhibition and fight-flight-freeze systems. Personal. Neurosci. 2:e12. doi: 10.1017/pen.2019.9
Dellon, A. L., Mackinnon, S. E., and Crosby, P. M. (1987). Reliability of two-point discrimination measurements. J. Hand Surgery 12, 693–696. doi: 10.1016/S0363-5023(87)80049-7
Dictionary, O. (2016). Middle Age: Definition Of Middle Age In Oxford Dictionary (American English) (Us) [Online]. Available online at: https://www.lexico.com/en/definition/middle_age (accessed April 26, 2022).
Dijkerman, H. C., and De Haan, E. H. F. (2007). Somatosensory processes subserving perception and action. Behav. Brain Sci. 30, 189–201. doi: 10.1017/S0140525X07001392
Dirnberger, G., Duregger, C., Lindinger, G., and Lang, W. (2011). On the regularity of preparatory activity preceding movements with the dominant and non-dominant hand: a readiness potential study. Int. J. Psychophysiol. 81, 127–131. doi: 10.1016/j.ijpsycho.2011.04.008
Eimer, M., Nattkemper, D., Schröger, E., and Prinz, W. (1996). “Chapter 5 Involuntary attention,” in Handbook of Perception and Action, eds O. Neumann and A. F. Sanders (Cambridge: Academic Press), 155–184. doi: 10.1016/s1874-5822(96)80022-3
Fardo, F., Vinding, M. C., Allen, M., Jensen, T. S., and Finnerup, N. B. (2017). Delta and gamma oscillations in operculo-insular cortex underlie innocuous cold thermosensation. J Neurophysiol. 117, 1959–1968. doi: 10.1152/jn.00843.2016
Frenkel-Toledo, S., Fridberg, G., Ofir, S., Bartur, G., Lowenthal-Raz, J., Granot, O., et al. (2019). Lesion location impact on functional recovery of the hemiparetic upper limb. PLoS One 14:e0219738. doi: 10.1371/journal.pone.0219738
Fugl-Meyer, A. R., Jääskö, L., Leyman, I., Olsson, S., and Steglind, S. (1974). The post-stroke hemiplegic patient. 1. a method for evaluation of physical performance. Scand. J. rehabil. Med. 7, 13–31.
Gama, G. L., Larissa, C. D. L., Brasileiro, A.C.D.a.L, Silva, E. M. G. D. S., Galvão, ÉR. V. P., Maciel, ÁC., et al. (2017). Post-stroke hemiparesis: does chronicity, etiology, and lesion side are associated with gait pattern? Top Stroke Rehabil 24, 388–393. doi: 10.1080/10749357.2017.1304865
Garkavenko, V. V., Man’kovskaya, O. P., Omel’chenko, T. G., Gorkovenko, A. V., and Shevko, A. N. (2008). Effect of cold stimulation of the arm fingers on the spectral/coherent EEG characteristics in humans. Neurophysiology 40, 228–230. doi: 10.1007/s11062-008-9041-4
Goodin, P., Lamp, G., Vidyasagar, R., McArdle, D., Seitz, R. J., and Carey, L. M. (2018). Altered functional connectivity differs in stroke survivors with impaired touch sensation following left and right hemisphere lesions. NeuroImage Clin. 18, 342–355. doi: 10.1016/j.nicl.2018.02.012
Grefkes, C., and Fink, G. R. (2011). Reorganization of cerebral networks after stroke: new insights from neuroimaging with connectivity approaches. Brain 134, 1264–1276. doi: 10.1093/brain/awr033
Gross, D. W., and Gotman, J. (1999). Correlation of high-frequency oscillations with the sleep–wake cycle and cognitive activity in humans. Neuroscience 94, 1005–1018. doi: 10.1016/s0306-4522(99)00343-7
Harris, J. E., and Eng, J. J. (2006). Individuals with the dominant hand affected following stroke demonstrate less impairment than those with the nondominant hand affected. Neurorehabil. Neural Rep. 20, 380–389. doi: 10.1177/1545968305284528
Hayn, M. A., and Fisher, T. R. (1997). Stroke rehabilitation: salvaging ability after the storm. Nursing 27:40.
Head, H., and Holmes, G. (1911). Sensory disturbances from cerebral lesions 1. Brain 34, 102–254. doi: 10.1093/brain/34.2-3.102
Huang, Y., Jiao, J., Hu, J., Hsing, C., Lai, Z., Yang, Y., et al. (2020). Measurement of sensory deficiency in fine touch after stroke during textile fabric stimulation by electroencephalography (EEG). J. Neural Eng. 17:045007. doi: 10.1088/1741-2552/aba160
Hwang, P., Sohn, M. K., Kim, C. S., and Jee, S. (2016). Tibial somatosensory evoked potential can prognosticate for ambulatory function in subacute hemiplegic stroke. J. Clin Neurosci. 26, 122–125. doi: 10.1016/j.jocn.2015.05.070
Iannetti, G. D., Hughes, N. P., Lee, M. C., and Mouraux, A. (2008). Determinants of laser-evoked EEG responses: pain perception or stimulus saliency? J. Neurophysiol. 100, 815–828. doi: 10.1152/jn.00097.2008
Jones, T. A., and Adkins, D. L. (2015). Motor system reorganization after stroke: stimulating and training toward perfection. Physiology (Bethesda, Md.) 30, 358–370. doi: 10.1152/physiol.00014.2015
Kessner, S. S., Bingel, U., and Thomalla, G. (2016). Somatosensory deficits after stroke: a scoping review. Topics Stroke Rehabil. 23, 136–146. doi: 10.1080/10749357.2015.1116822
Krause, T., Asseyer, S., Geisler, F., Fiebach, J. B., Oeltjenbruns, J., Kopf, A., et al. (2016). Chronic sensory stroke with and without central pain is associated with bilaterally distributed sensory abnormalities as detected by quantitative sensory testing. Pain 157, 194–202. doi: 10.1097/j.pain.0000000000000354
Kwan, C. L., Crawley, A. P., Mikulis, D. J., and Davis, K. D. (2000). An fMRI study of the anterior cingulate cortex and surrounding medial wall activations evoked by noxious cutaneous heat and cold stimuli. Pain 85, 359–374. doi: 10.1016/s0304-3959(99)00287-0
Laufer, Y., Sivan, D., Schwarzmann, R., and Sprecher, E. (2003). Standing balance and functional recovery of patients with right and left hemiparesis in the early stages of rehabilitation. Neurorehabil. Neural Rep. 17, 207–213. doi: 10.1177/0888439003259169
Lebeau, F. (2010). Gamma oscillations and the cellular components of consciousness? New Horiz. Neurosci. Consci. 79, 29–37. doi: 10.1075/aicr.79.07leb
Lin, R., Hsu, M. J., Lin, R. T., Huang, M. H., Koh, C. L., Hsieh, C. L., et al. (2017). No difference between noxious and innocuous thermal stimulation on motor recovery of upper extremity in patients with acute stroke: a randomized controlled trial with 6-month follow-up. PMR 9, 1191–1199. doi: 10.1016/j.pmrj.2017.05.012
Lystad, R. P., and Pollard, H. (2009). Functional neuroimaging: a brief overview and feasibility for use in chiropractic research. J. Can. Chiropr. Assoc. 53, 59–72. doi: 10.4324/9780203938904-12
Maihöfner, C., Kaltenhäuser, M., Neundörfer, B., and Lang, E. (2002). Temporo-spatial analysis of cortical activation by phasic innocuous and noxious cold stimuli–a magnetoencephalographic study. Pain 100, 281–290. doi: 10.1016/s0304-3959(02)00276-2
Matsuda, K., Satoh, M., Tabei, K.-I., Ueda, Y., Taniguchi, A., Matsuura, K., et al. (2020). Impairment of intermediate somatosensory function in corticobasal syndrome. Sci. Rep. 10:11155. doi: 10.1038/s41598-020-67991-7
Mazzola, L., Faillenot, I., Barral, F.-G., Mauguière, F., and Peyron, R. (2012). Spatial segregation of somato-sensory and pain activations in the human operculo-insular cortex. NeuroImage 60, 409–418. doi: 10.1016/j.neuroimage.2011.12.072
Meador, K. J., Ray, P. G., Echauz, J. R., Loring, D. W., and Vachtsevanos, G. J. (2002). Gamma coherence and conscious perception. Neurology 59, 847–854. doi: 10.1212/WNL.59.6.847
Misra, U. K., Kalita, J., and Kumar, B. (2008). A study of clinical, magnetic resonance imaging, and somatosensory-evoked potential in central post-stroke pain. J. pain 9, 1116–1122. doi: 10.1016/j.jpain.2008.06.013
Nuwer, M. R., Comi, G., Emerson, R., Fuglsang-Frederiksen, A., Guerit, J.-M., Hinrichs, H., et al. (1999). Recommendations For The Practice Of Clinical Neurophysiology: Guidelines Of The International Federation Of Clinical Physiology, Electroencephalography and Clinical Neurophysiology Supplement. Amsterdam: Elsevier.
Özcan, A., Ozdirenç, M. M., and Akln, F. (2005). Light touch and pain sensation differences between dominant and non-dominant hands of healthy subjects. British J. Hand Ther. 10, 76–79. doi: 10.1177/1758998305010003-401
Park, S. Y., and Lee, I. H. (2011). The influence of age, lesion side and location on rehabilitation outcome after stroke. J. Physic. Ther. Sci. 23, 817–819. doi: 10.1080/09297040701792383
Razali, N. M., and Wah, Y. B. (2011). Power comparisons of shapiro-wilk, kolmogorov-smirnov, lilliefors and anderson-darling tests. J. Stat. Model. Anal. 2, 21–33.
Rebbeck, T., Moloney, N., Azoory, R., Hübscher, M., Waller, R., Gibbons, R., et al. (2015). Clinical ratings of pain sensitivity correlate with quantitative measures in people with chronic neck pain and healthy controls: cross-sectional study. Phys. Ther. 95, 1536–1546. doi: 10.2522/ptj.20140352
Roselli, F., Tartaglione, B., Federico, F., Lepore, V., Defazio, G., and Livrea, P. (2009). Rate of MMSE score change in alzheimer’s disease: influence of education and vascular risk factors. Clin. Neurol. Neurosurg. 111, 327–330. doi: 10.1016/j.clineuro.2008.10.006
Satoh, M., Terada, S., Onouchi, K., Takeda, K., and Kuzuhara, S. (2002). Somatosensory and skin temperature disturbances caused by infarction of the postcentral gyrus: a case report. J. Neurol. 249, 1404–1408. doi: 10.1007/s00415-002-0853-7
Serrada, I., Hordacre, B., and Hillier, S. L. (2019). Does sensory retraining improve sensation and sensorimotor function following stroke: a systematic review and meta-analysis. Front. Neurosci. 13:402–402. doi: 10.3389/fnins.2019.00402
Serrien, D. J., and Spapé, M. M. (2009). The role of hand dominance and sensorimotor congruence in voluntary movement. Exp. Brain Res. 199, 195–200. doi: 10.1007/s00221-009-1998-8
Shao, S., Shen, K., Yu, K., Wilder-Smith, E. P., and Li, X. (2012). Frequency-domain EEG source analysis for acute tonic cold pain perception. Clin. Neurophysiol. 123, 2042–2049. doi: 10.1016/j.clinph.2012.02.084
Shingala, M. C., and Rajyaguru, A. (2015). Comparison of post hoc tests for unequal variance. Int. J. New Technol. Sci. Eng. 2, 22–33.
Singh, H., Bauer, M., Chowanski, W., Sui, Y., Atkinson, D., Baurley, S., et al. (2014). The brain’s response to pleasant touch: an EEG investigation of tactile caressing. Front. Human Neurosci. 8:893. doi: 10.3389/fnhum.2014.00893
Slater, J. D. (2008). A definition of drowsiness: One purpose for sleep? Med. Hypotheses 71, 641–644. doi: 10.1016/j.mehy.2008.05.035
Society, A. C. N. (2015). Guideline 11b: Recommended Standards For Intraoperative Monitoring Of Somatosensory Evoked Potentials. 2009. Retrieved from Acns. Org. Hauppauge: Nova Science.
Steimann, L., Missala, I., Van Kaick, S., Walston, J., Malzahn, U., Heuschmann, P. U., et al. (2012). Rivermead assessment of somatosensory performance. Der. Nervenarzt 83, 1632–1637. doi: 10.1007/s00115-012-3614-6
Sudman, S., Bradburn, N., and Schwarz, N. (1997). Thinking about answers: the application of cognitive processes to survey methodology. Psyccritiques 42, 652–652. doi: 10.1023/A:1025127424627
Takeda, K., Shozawa, Y., Sonoo, M., Shimizu, T., and Kaminaga, T. (2000). The rostrocaudal gradient for somatosensory perception in the human postcentral gyrus. J. Neurol. Neurosurg. Psychiatry 69, 692–693. doi: 10.1136/jnnp.69.5.692
Tarkka, I. M., and Hallett, M. (1990). Cortical topography of premotor and motor potentials preceding self-paced, voluntary movement of dominant and non-dominant hands. Electroencephalogr Clin Neurophysiol. 75, 36–43. doi: 10.1016/0013-4694(90)90150-i
Tecchio, F., Babiloni, C., Zappasodi, F., Vecchio, F., Pizzella, V., Romani, G. L., et al. (2003). Gamma synchronization in human primary somatosensory cortex as revealed by somatosensory evoked neuromagnetic fields. Brain Res. 986, 63–70. doi: 10.1016/s0006-8993(03)03171-8
Tecchio, F., De Lucia, M., Salustri, C., Montuori, M., Bottaccio, M., Babiloni, C., et al. (2004). District-related frequency specificity in hand cortical representation: dynamics of regional activation and intra-regional synchronization. Brain Res. 1014, 80–86. doi: 10.1016/j.brainres.2004.04.028
Thibaut, A., Simis, M., Battistella, L. R., Fanciullacci, C., Bertolucci, F., Huerta-Gutierrez, R., et al. (2017). Using brain oscillations and corticospinal excitability to understand and predict post-stroke motor function. Front. Neurol. 8:187–187. doi: 10.3389/fneur.2017.00187
Thut, G., and Miniussi, C. (2009). New insights into rhythmic brain activity from TMS–EEG studies. Trends Cogn. Sci. 13, 182–189. doi: 10.1016/j.tics.2009.01.004
Tourangeau, R., Rips, L. J., and Rasinski, K. (2000). The Psychology Of Survey Response. Cambridge: Cambridge University Press.
Tseng, M. T., Tseng, W. Y., Chao, C. C., Lin, H. E., and Hsieh, S. T. (2010). Distinct and shared cerebral activations in processing innocuous versus noxious contact heat revealed by functional magnetic resonance imaging. Hum. Brain Mapp. 31, 743–757. doi: 10.1002/hbm.20902
Väisänen, O., and Malmivuo, J. (2009). Improving the SNR of EEG generated by deep sources with weighted multielectrode leads. J. Physiol. Paris 103, 306–314. doi: 10.1016/j.jphysparis.2009.07.003
Wang, A.-L., Mouraux, A., Liang, M., and Iannetti, G. D. (2010). Stimulus novelty, and not neural refractoriness, explains the repetition suppression of laser-evoked potentials. J. Neurophysiol. 104, 2116–2124. doi: 10.1152/jn.01088.2009
Woytowicz, E., Rietschel, J., Goodman, R., Conroy, S., Sorkin, J., Whitall, J., et al. (2016). Determining levels of upper extremity movement impairment by applying cluster analysis to upper extremity fugl-meyer assessment in chronic stroke. Arch. Phys. Med. Rehabil. 98, 456–462. doi: 10.1016/j.apmr.2016.06.023
Wu, C. W., Seo, H. J., and Cohen, L. G. (2006). Influence of electric somatosensory stimulation on paretic-hand function in chronic stroke. Arch. Phys. Med. Rehabil. 87, 351–357. doi: 10.1016/j.apmr.2005.11.019
Keywords: stroke, sensory deficiency, electroencephalography, elementary somatosensation, thermal sensation, non-painful cold stimulation
Citation: Huang YH, Jiao J, Hu JY, Hsing CC, Lai ZQ, Yang Y, Li ZY and Hu XL (2022) Electroencephalographic Measurement on Post-stroke Sensory Deficiency in Response to Non-painful Cold Stimulation. Front. Aging Neurosci. 14:866272. doi: 10.3389/fnagi.2022.866272
Received: 31 January 2022; Accepted: 08 April 2022;
Published: 11 May 2022.
Edited by:
Le Li, Northwestern Polytechnical University, ChinaReviewed by:
Qiuhua Yu, Sun Yat-sen University, ChinaSio Hang Pun, University of Macau, Macao SAR, China
Copyright © 2022 Huang, Jiao, Hu, Hsing, Lai, Yang, Li and Hu. This is an open-access article distributed under the terms of the Creative Commons Attribution License (CC BY). The use, distribution or reproduction in other forums is permitted, provided the original author(s) and the copyright owner(s) are credited and that the original publication in this journal is cited, in accordance with accepted academic practice. No use, distribution or reproduction is permitted which does not comply with these terms.
*Correspondence: Xiaoling Hu, xiaoling.hu@polyu.edu.hk