The effect of different sources of mesenchymal stem cells on microglia states
- Department of Neurotrauma Surgery, The First Hospital of Jilin University, Changchun, China
Microglial reaction plays a key role in the prognosis of traumatic CNS injuries (TBI and SCI). A growing number of studies have shown that mesenchymal stem cells (MSCs) play an important role in regulating microglial states. This review summarizes the effects and mechanisms of different sources of MSCs on microglial states in the last 5 years. In general, bone marrow-derived mesenchymal stem cells are the most accessible and widely used, and can produce immunosuppressive effects on a variety of brain injuries including TBI through tissue engineering in situ implantation; MSCs mainly regulate inflammatory pathways and promote the states of microglia in the anti-inflammatory direction, which also secrete certain cytokines or extracellular vesicles to affect apoptotic pathways, such as the extracellular vesicles miR-21-5p, acting as a neuronal protector.
Introduction
Traumatic CNS injuries include traumatic brain injury (TBI), spinal cord injury (SCI), and so on. TBI, according to the Centers for Disease Control and Prevention, is a disruption of normal brain function caused by a blow, strike, bump, or penetrating injury to the head (Capizzi et al., 2020). Globally, approximately 70 million people suffer from TBI each year, resulting in significant physical and psychological harm and economic burden to patients (Wiles, 2022). Neurological impairment after brain injury includes cognitive impairment, coma, sensory and motor impairment, visual impairment, and language impairment (McKee and Lukens, 2016). SCI refers to structural or functional spinal cord damage that occurs following damage to the spinal cord from a variety of causes, resulting in permanent loss of movement and sensation below the plane of injury. TBI and SCI are divided into primary and secondary injuries, with transient mechanical external forces acting on the skull causing primary injury while secondary injury results in a series of pathophysiological responses of the body in response to primary injury (Xiong et al., 2013). In the absence of exogenous infection, primary injury is followed by a sterile neuroimmune and neuroinflammatory response (Corps et al., 2015). In contrast, secondary injuries are characterized by glial cell activation, leucocyte recruitment, and upregulation of inflammatory mediators (Xiong et al., 2018). In addition to traumatic CNS injuries, traumatic subarachnoid hemorrhage combined with cerebral contusion, occlusion of major cerebral blood vessels, and stroke can also cause severe microglial reactions. Traditional treatments for severe craniocerebral injuries include debridement decompression, prophylactic sub-hypothermia, hyperosmotic therapy, cerebrospinal fluid drainage, and ventilation therapy (Galgano et al., 2017). Traditional treatments for SCI include surgical decompression, therapeutic hypothermia, and drugs targeting inflammation or excitotoxicity (Ramer et al., 2014). Despite significant advances in these treatments for traumatic CNS injuries, mortality rates for patients following traumatic CNS injuries remain high and their neurologic damage is not effectively repaired. In contrast, tissue engineering approaches using MSCs seem to have broader prospects. The results from some preclinical studies have shown that transplantation of bone marrow-derived mesenchymal stem cells (BMSCs) reduces secondary neurodegeneration and neuroinflammation, promotes neural and vascular neogenesis, and improves functional prognosis in experimental animals (Li J. R. et al., 2021).
Mesenchymal stem cells (MSCs) are pluripotent stem cells that have all the common properties of stem cells, namely, self-renewal and multidirectional differentiation capabilities. Now found in almost all tissues of the human body, the pluripotency of MSCs allows them to differentiate into osteoblasts, chondrocytes, adipocytes, or other lineages (Wang et al., 2021). MSCs exhibit immune tolerance by influencing innate and adaptive immune responses. This is reflected in the ability of MSCs to suppress immune cell responses by secreting immunomodulatory factors as well as to promote an immunosuppressive environment (Fernandes et al., 2020). MSCs slow down inflammatory injury in the central nervous system by interacting with macrophages/microglia (Harrell et al., 2019). Microglia, as resident immune cells of the CNS, are involved in the secondary inflammatory response after TBI together with macrophages of peripheral origin (Woodcock and Morganti-Kossmann, 2013).
The two states of macrophages include classically activated M1 and alternatively activated M2 phenotypes (Yunna et al., 2020). Microglia have a similar state process during CNS injuries (Hu et al., 2012). The traditional classification of the states of microglia responding to inflammation is M1 and M2 (Table 1). M1 macrophages/microglia are mainly involved in proinflammatory responses, and the M2 phenotype are mainly involved in anti-inflammatory responses. M1 macrophages/microglia usually release proinflammatory factors, including TNF-α, CCL2, and IL-6, which induce neuroinflammatory and toxic effects; in contrast, M2 macrophages/microglia secrete anti-inflammatory factors, such as IL-4, and neurotrophic factors, such as insulin growth factor (IGF), to exert anti-inflammatory repair effects (Li M. et al., 2021). Another method of categorization is to classify M2-type microglia into M2a, M2b, and M2c. They think the normal wound repair process is the sequential activation of classically activated M1-type and alternatively, activated M2a, M2b, and M2c-type macrophages to achieve structural and functional recovery of the injured tissue. However, nerve repair after TBI is difficult not only because neurons are permanent cells, but the excessive inflammatory response present at the site of injury is also an important factor that hinders nerve repair. The process of wound repair consists of three phases: inflammation, proliferation, and remodeling. The pro-inflammatory markers IL-12, IL-1β, TNF-α, and IL-6 increased dramatically in the early post-TBI period, and microglia in this phase also expressed high levels of arginase (Arg) and Ym-1, suggesting that the inflammation phase consisted of microglia of M1 and M2a phenotypes. The M2 microglia in the proliferation phase have different phenotypic characteristics from the M2 microglia in the inflammation phase. The expression of IL-10 is a hallmark of the M2b phenotype, which is secreted by the M2b microglia to promote tissue remodeling and thus plays a crucial role in the proliferation phase. It has been shown that the M2b microglia phenotype is improperly activated after SCI. The remodeling phase of normal wound healing is mainly dominated by M2c macrophages, which exhibit high expression of TGF-β and CD206, along with a decrease in Arg-1 (Gensel and Zhang, 2015). MSCs achieve nerve repair by promoting sequential activation of microglia after TBI. Extracellular vesicles were extracted from the supernatant of BMSCs cell culture, and the TBI mouse model was induced after tail vein injection. It was demonstrated that BMSCs promoted the expression of Arg-1, IL-10, and TGF-β after TBI by secreting the extracellular vesicles miR-181b (Wen et al., 2022), suggesting that MSCs can promote the expression of all the subtypes of M2-type microglia (Li et al., 2017), and in particular, promote the effective states of M2b and M2c.3 d and 7 d after TBI, we measured the expression of TNFα, CD86, Ym-1, Arg-1, and CD206 mRNA expression, and the results showed that BMSCs implanted at the injury site were effective in promoting microglia states to M2a and M2c (Zanier et al., 2014). Subtype differentiation of MSCs was found in vitro under exposure to stimuli, however, the complex environment in vivo may not apply to these states of differentiation. Paolicelli et al. (2022) put forward describe microglia using as many layers of complexity as possible and do not use M1 versus M2 classification. The following description of MSCs utilizes recommendations made by Paolicelli et al. (2022).
Mesenchymal stem cells can effectively suppress microglial reaction after TBI and SCI by regulating CNS microglial states and have a wide range of applications. However, it is not clear how the implanted MSCs regulate microglial states, so further studies on the regulatory mechanisms of MSCs are demanded to provide new ideas for neural repair after traumatic CNS injuries.
Mechanisms by which mesenchymal stem cells regulate microglial states
In the early stages after traumatic CNS injuries, microglia create a favorable microenvironment for tissue regeneration by removing tissue debris (Devanney et al., 2020; An et al., 2021). In contrast, few reparative microglia are induced after TBI (Kigerl et al., 2009). Therefore, using a tissue engineering approach after the beneficial effects of the microglia would facilitate neural repair in TBI.
MSCs regulate microglial states through a paracrine mechanism of cytokines
Studies have shown that MSCs can promote recovery from neurologically related diseases by secreting a variety of soluble factors, including trophic factors and cytokines (Teixeira et al., 2017). It has been suggested that the prolonged presence of microglial reaction after TBI increases the chance of Alzheimer’s disease (Ramos-Cejudo et al., 2018). In studies of Alzheimer’s disease models, transplanted MSCs were found to promote the conversion of microglia from the CD86+ to the Arg-1+ state through paracrine effects (Campos et al., 2022; Zhang R. et al., 2022). MSCs regulate microglial states by secreting the paracrine factors Galectin-1 (Gal-1) (Seo et al., 2022), tumor necrosis factor-alpha-induced gene/protein 6 (TSG-6) (Liu et al., 2019; Nam et al., 2020), and IL-13 (Taj et al., 2018), etc. Promoting microglial states toward the anti-inflammatory phenotype facilitates the relief of deafferentation pain. MSCs may regulate the functional state of microglia by activating the STAT6 signaling pathway, decreasing the expression of proinflammatory cytokine and promoting the expression of anti-inflammatory cytokine (Liu et al., 2022). MSCs, stimulated with TNF-α, regulate microglial activity by secreting TNF-α stimulated gene/protein 6 (TSG-6), which may interact with CD44 on the surface of microglia and inhibit LPS-induced activation of the TLR4 signaling pathway in microglial cells. Inhibition of the TLR4 signaling pathway impeded activation of the nuclear factor (NF)-κB and mitogen-activated protein kinase (MAPK) signaling pathways, thereby reducing the expression level of neuroinflammatory cytokines in BV2 microglia (Liu et al., 2014).
Homeostatic-state microglia do not express iNOS and Arg-1, but the stimulation of LPS upregulates iNOS expression and decreases Arg-1 expression. In a preclinical study, BMSCs were found to affect the miR-30a-3p/XBP1/MANF pathway in microglia by secreting platelet-derived growth factor-AA (PDGF-AA). Specifically, the PDGF-AA, secreted by BMSCs, downregulates miR-30a-3p in microglia. The decline of miR-30a-3p can enhance X-box binding protein (XBP) 1 expression, leading to increased expression of mesencephalic astrocyte-derived neurotrophic factor (MANF). This leads to the states of microglia from an iNOS-expressing to an Arg-1-expressing state (Yang et al., 2020). MANF is an inducible protein of endoplasmic reticulum stress and prevents cellular damage caused by such stress (Xu et al., 2019). The application of MANF treatment improved behavioral recovery in a rat model of stroke (Mätlik et al., 2018). In an in vitro model study using oxyhemoglobin-stimulated BV2 cells to simulate subarachnoid hemorrhage, it was found that microglia were cocultured with BMSCs, and BMSCs caused significant activation of the FoxO, TNF, and PI3K-Akt signaling pathways in microglia through cytokine secretion. Another study found BMSCs may inhibit the NF-κB and promote the PI3K/AKT signaling pathway by secreting glial cell-derived neurotrophic factor (GDNF) to inhibit LPS-induced expression of IL-1β, TNF-α, and CD86 and up-regulate the expression of IL-10, CD206 in microglia (Zhang et al., 2020; Zhong et al., 2020a,b). Yin et al. (2023) isolated multilineage-differentiating stress-enduring (Muse) cells from bone marrow mesenchymal stem cells. By co-culturing Muse cells with lipopolysaccharide-stimulated microglia, the results showed that Muse cells inhibited the expression of p38 mitogen-activated protein kinase (MyD88) and toll-like receptor 4 (TLR4) in microglia through paracrine effects. It also inhibited the phosphorylation of p38 MAPK, NF-κB inhibitor alpha, and transcription factor p65 in microglia, increasing the ratio of microglia expressing CD206 and Arg-1. That is, Muse cells produce anti-neuroinflammatory effects by inhibiting the TLR4/MyD88/NF-κB and p38 MAPK signaling pathways in microglia.
In a rat model of middle cerebral artery occlusion-induced brain injury, human umbilical cord-derived mesenchymal stem cells (hUC-MSCs) were found to migrate into the region of cerebral infarct and secrete various soluble cytokines, including prostaglandin E2 (PGE2). The ratio of expressing CD206 microglia in the brain area was significantly increased after hUC-MSCs (Teng et al., 2021). It has been shown that abnormalities in the immune-inflammatory pathway contribute to the development of major depression (Fenn et al., 2014), and inhibiting the overreactive state of microglia in response to inflammation facilitates the reduction of depressive-like behaviors. hUC-MSCs alter microglial states by inhibiting the C3a-C3aR signaling pathway through the secretion of C3a receptor antagonist-like substances, resulting in a decrease in hippocampal regions expressing CD16 + /Iba1 + microglia and an increase in the number of CD206 + /Iba1 + cells (Li et al., 2020).
In addition to BMSCs, hUC-MSCs and adipose-derived stem cells (ADSCs) have also been shown to regulate microglial states (Table 2). Nrf2-ADSC and BV2 cells were cocultured in the LPS environment. The results showed that ADSCs could increase Nrf2/HO-1 signaling pathway and decrease TLR4 and NF-κB signaling pathway activation in BV2 cells. As a result, BV2 cells expressed reduced levels of inflammatory cytokines such as MCP-1, TNF-α, IL-1β, and IL-6. Meanwhile, Nrf2-ADSC treatment also promoted the states of microglia toward the state of expressing Ym-1 and Arg-1 (Huang et al., 2020).
MSCs regulate microglial states through their secreted extracellular vesicles
Extracellular vesicles are produced within the Golgi body and then released outside the cell through the merger of the Golgi body with the plasma membrane. Extracellular vesicles mediate intercellular communication by transferring proteins, miRNAs, and mRNAs (Kalluri and LeBleu, 2020).
Brain contusion with traumatic subarachnoid hemorrhage is a common clinical presentation in traumatic brain injury. The inflammatory response has been shown to be present throughout subarachnoid hemorrhage. It has been shown that MSCs significantly suppress the inflammatory response after subarachnoid hemorrhage by transferring extracellular vesicles (Han et al., 2021). Further studies revealed that MSCs-derived extracellular vesicles (MSCs-EV) inhibit NF-κB activation via activation of AMPK via its downstream proteins sirtuin-1 and Forkhead Box O3, thereby reducing the mRNA levels of IL-1β, CD11b, CD16, and iNOS and increasing the mRNA levels of TGF-β, Arg-1 and CD20 in microglia (Han et al., 2021). MSCs transfer miR-140-5p into microglia via extracellular vesicles. MiR-140-5p targets the activin-like kinase 5 gene and inhibits its expression, thereby downregulating NADPH oxidase 2 and thereby inhibiting CD86+ microglia activation in a mouse model of subarachnoid hemorrhage (Qian et al., 2022). Furthermore, extracellular vesicles miR-223-3p from MSCs attenuated cerebral ischemia/reperfusion injury by inhibiting the proinflammatory response mediated by microglial. MSCs promoted the conversion of microglia from the CD16/CD32+ to the CD206+ state, which may be related to the inhibitory effect of extracellular vesicles miR-223-3p on CysLT2R/ERK1/2 signaling pathway (Zhao Y. et al., 2020; Zhao Y. M. et al., 2020). Cysteinyl leukotrienes (CysLTs) are potent inflammatory mediators expressed after cell necrosis, and their inflammatory effects are mediated by the CysLTs receptor (Sasaki and Yokomizo, 2019). ERK1/2 are important mitogenic kinases in the ERK signaling pathway involved in the inflammatory response (Zhao Y. et al., 2020). MSCs also transfer miR-21a-5p via extracellular vesicles and repress the STAT3 gene, inducing microglia to shift from a proinflammatory to an anti-inflammatory state (Xin et al., 2022). Microglia play an important role in mediating CNS demyelinating diseases and their repair, while the generation of microglial reaction secondary to TBI is also dominated by microglial activation, and the treatment of both involves the regulation of microglial states. MSCs reduce TLR2/IRAK1/NF-kB signaling pathway activation in microglia by transferring extracellular vesicles, which in turn promote microglial conversion from the iNOS+ to the Ym-1+ (Zhang J. et al., 2022). Let-7a, miR-23a, miR-125b, and miR-199 in extracellular vesicles may play an important role in this process (Ferguson et al., 2018; Fan et al., 2020). In the treatment of a mouse model of spinal cord injury, it was found that MSCs pretreated with melatonin could promote the conversion of mouse microglia by secreting extracellular vesicles. The number of microglia expressing iNOS, TNF-α, and IL-1β was reduced, and the number of microglia expressing Arg-1, CD206, and Ym-1 was increased (Liu et al., 2021). Ubiquitin-specific protease 29 (USP29) in extracellular vesicles interacts with nuclear factor-like 2 in microglia, thereby regulating microglial states (Liu et al., 2021). In a model applying MSCs to treat spinal cord injury, it was found that extracellular vesicles miR-216a-5p secreted by hypoxia-pretreated MSCs are involved in the regulation of microglial states from the Iba-1+/iNOS+ to the Iba-1+/Arg-1+ state by regulating the TLR4/NF-kB/PI3K/AKT signaling pathway, namely, by inhibiting TLR4/NF-κB and activating the PI3K/AKT signaling pathway (Liu et al., 2020). More interestingly, the surface receptor type of extracellular vesicles also affects microglial states. MSCs influenced the states of microglia in vivo by secreting extracellular vesicles expressing the C-C chemokine receptor type 2 surface receptor, which may be a result of the binding of CCR2-expressing extracellular vesicles to endogenous C-C chemokine ligand 2 ligands that inhibit exogenous macrophage hypertropia and therefore suppress subsequent inflammatory cell states (Yang et al., 2020).
Extracellular vesicles secreted by human BMSCs (BMSCs-EV) reduce the number of CD86+ microglia by inhibiting the P38MAPK/P65NF-κB signaling pathway (Shu et al., 2022). A controlled cortical impact method was used to induce a mouse model of TBI, and the BMSCs-EV were injected through retro-orbital, which showed a decrease in the expression of pro-inflammatory cytokines (IL-1β, TNF-α) and an increase in the expression of anti-inflammatory proteins (Arg-1). The BMSCs-EV promotes the transformation of microglial cells of the Iba-1+/iNOS+ to the Iba-1+/CD206+ state (Ni et al., 2019). In a therapeutic model of cerebral ischemia-reperfusion, conditioned medium from hypoxia-pretreated BMSCs was found to promote anti-inflammatory states of microglia, and the exocytosis of these effects was mainly attributed to the extracellular vesicles secreted by BMSCs (Yu et al., 2021). In a rat model of middle cerebral artery occlusion, BMSCs were found to transfer miR-23a-3p via extracellular vesicles to downregulate the number of iNOS+ cells and upregulate the number of Arg-1+ cells (Dong et al., 2022). Lipopolysaccharide-stimulated BV2 microglia are widely accepted as a common in vitro model of microglial reaction. The secretion of extracellular vesicles H19 by BMSCs alleviated LPS-induced expression of IL-1β, IL-6, and TNF-a and increased the levels of IL-10 by targeting miR-29b-3p expression in microglia (Zong et al., 2021). Duan et al. (2020) found that BMSCs, by secreting extracellular vesicles miR-146a-5p, interact with interleukin-1 receptor-associated kinase 1 and nuclear factor of activated T cells 5 in microglia, thereby inhibiting the proinflammatory states of microglia after cerebral hemorrhage in rats. The development of multiple sclerosis also involves the states of inflammatory microglia in the CNS. In a study of a rat model of experimental autoimmune encephalomyelitis, Zi found that extracellular vesicles secreted by BMSCs inhibited the expression of the M1 phenotypic markers CD68, iNOS, and TNF-α in microglia and promoted the expression of the M2 phenotypic markers CD206, Arg-1, IL-10, and TGF-β (Li et al., 2019).
In a study of a rat model of TBI, it was found that the expression of pro-inflammatory factors was reduced by intracerebroventricular injection of human adipose mesenchymal stem cells (hADSCs)-derived extracellular vesicles (hADSCs-EV). Further studies found that hADSCs-EV inhibited the morphological transformation from the resting to the amoebic appearance of microglia by inhibiting the activation of NF-κB and P38/MAPK signaling pathways (Chen Y. F. et al., 2020). In a study of a mouse model of middle cerebral artery occlusion, ADSCs pretreated by hypoxia were found to transfer circ-Rps5 via extracellular vesicles. MiR-124-3p interacts with the Sirtuin 7 (SIRT7) 3′UTR. Overexpression of circ-Rps5 downregulates miR-124-3, which in turn promotes the expression of SIRT7 and converts microglia from the iNOS+ to the CD206+ state (Yang et al., 2022). SIRT7 and miR-124-3p are downstream targets of circ-Rps5 (Yang et al., 2022). MiR-124-3p inhibits the activation of nucleotide-binding oligomerization domain-like receptor pyrin domain-containing 3 inflammatory vesicles by targeting tumor necrosis factor receptor-associated factor 6, thereby suppressing microglial activation after brain basal ganglia hemorrhage cells secondary to microglial reaction (Fang and Hong, 2021). SIRT7 is an NAD + -dependent deacetylase that is involved in a variety of biological processes in organisms. In addition to mediating ribosomal RNA synthesis and protein synthesis, tumorigenesis, DNA damage response, and metabolism, SIRT7 can also inhibit LPS-induced inflammatory responses through the NF-κB signaling pathway (Chen et al., 2019). The extracellular vesicles secreted by ADSCs (ADSCs-EV) pretreated with hypoxia are enriched with LncGm37494, which promotes the states of microglial from the Iba-1+/iNOS+ to the Iba-1+/Arg-1+ state by inhibiting miR-130b-3p expression in microglia. ADSCs-EV also promote the expression of peroxisome proliferator-activated receptor γ (PPARγ), which in turn repairs spinal cord injury (Shao et al., 2020). PPARγ belongs to a family of nuclear receptors that was shown to polarize macrophages to the M2 phenotype (Yao et al., 2018). In addition, ADSCs inhibit ischemia-induced autophagy by secreting extracellular vesicles miR-30d-5p which targets the Beclin-1 and Atg5 genes. Consequently, ADSCs can inhibit the expression of inflammatory cytokines by microglia, promoting the states of microglia into a state that expresses anti-inflammatory cytokines such as IL-4 and IL-10 and alleviating neurological damage caused by cerebral infarction (Jiang et al., 2018).
In a model of retinal ischemia/reperfusion injury, TNF-α stimulated extracellular vesicles miR-21-5p secreted by gingival mesenchymal stem cells (GMSCs) was found to convert microglia from the Iba-1+/CD86+ state to the Iba-1+/Arg-1+ state by combining with programmed cell death 4 (PDCD4) (Yu et al., 2022).
In summary, MSCs mainly modulate inflammatory pathways after traumatic CNS injuries and promote the states of activated microglia in an anti-inflammatory direction (Table 3). Among them, BMSCs are easily isolated and expanded in vitro, in relatively abundant supply, and without ethical issues, making them ideal candidates for cell transplantation for inflammation suppression and cellular repair after various brain injuries, including TBI and spinal cord injury (Ning et al., 2019). For example, Bansal et al. (2016) successfully extracted bone marrow mesenchymal stem cells in patients and treated them with injections at the L1/L2 level. In a clinical trial of 10 patients with spinal cord injury, improvements in ASIA grading were observed in 6 patients, bladder control in 3 patients, sexual function in 5 patients, and relief of spasticity in 8 patients. Jarocha et al. (2015) conducted a 2-year trial of intensive treatment with bone marrow stem cell transplantation in a patient with a T2-3 spinal cord injury. After treatment, the patient’s ASIA classification increased from A to C/D, the sensory level increased from T1 to L3-4 and control of the body and urine was restored.
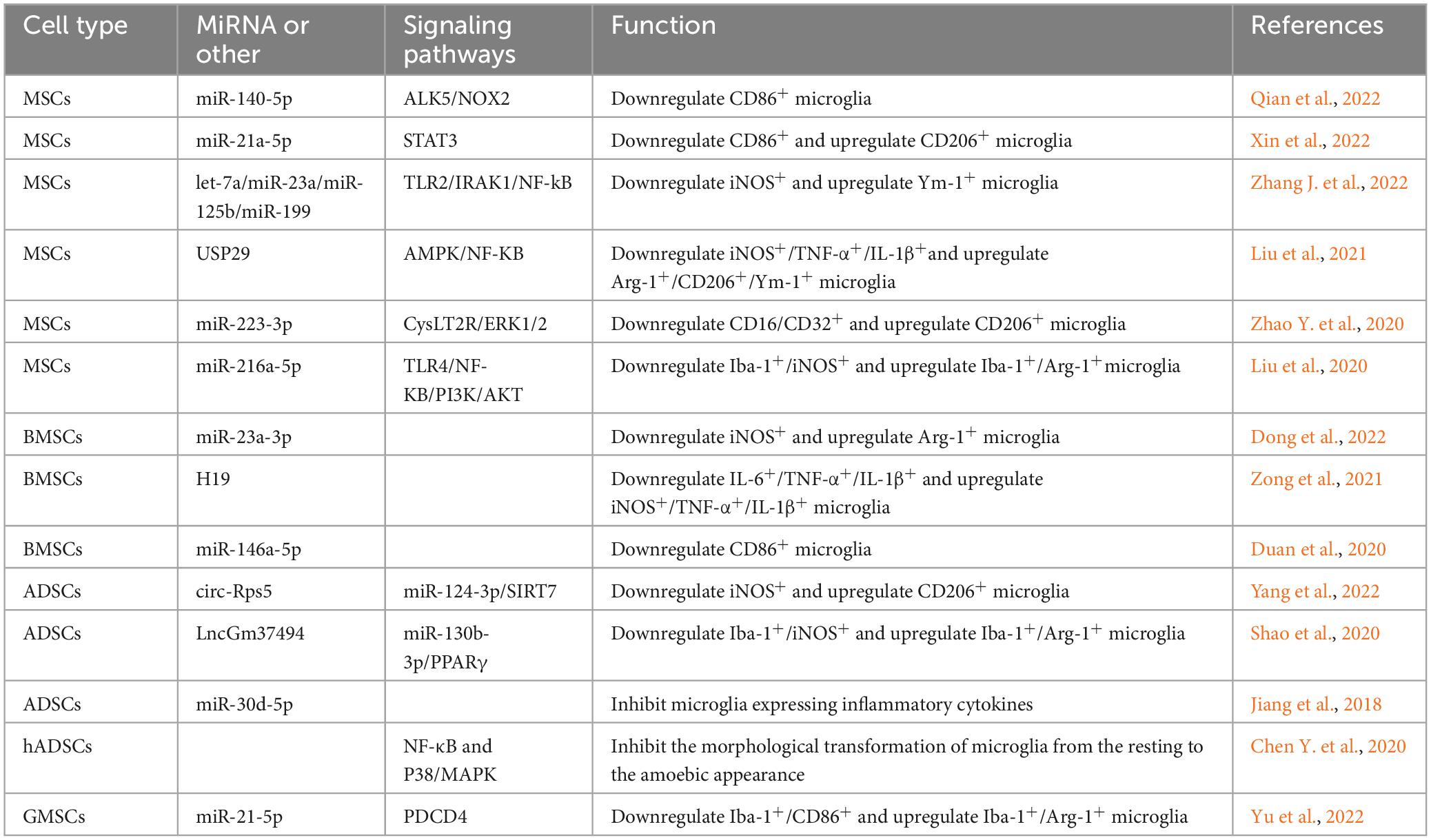
Table 3. The signaling pathway of extracellular vesicular miRNA secreted by MSCs to regulate microglia state.
Conclusion and perspectives nomenclature
All in all, BMSCs are the most accessible and widely used, while AMSCs can also be used in a range of brain injury diseases, all of which have a role in suppressing secondary inflammation response. MSCs implantation mainly regulates inflammatory pathways but also secretes certain cytokines or extracellular vesicles that affect apoptotic pathways, such as extracellular vesicles miR-21-5p, thus playing a role in neuronal protection.
How MSCs regulate macrophage states has been reported in the literature. In addition to the secretion of soluble cytokines and extracellular vesicles, MSCs can also regulate macrophage states through metabolic reprogramming, mitochondrial transfer, apoptosis, and phagocytosis. As intrinsic immune cells of the central nervous system, microglia may also be regulated in several of the above ways. In addition, MSCs from different sources have different roles. It has been reported that injection of allogeneic BMSCs improves neonatal hypoxic-ischemic brain injury, whereas ADSCs induce severe pulmonary hemorrhage and increased mortality (Sugiyama et al., 2018), which was not found in the abovementioned study. Whether these effects are related to the source of MSCs, the implantation method, or the implantation time, whether there are interactions between the pathways of action of MSCs secretions and how to improve the survival of implanted MSCs need to be demonstrated by further studies to better exploit the immunomodulatory function of MSCs.
Author contributions
QX drafted the manuscript. WZ, CH, TL, and HW critically revised the manuscript. All authors contributed to the manuscript revision, read, and approved the submitted version.
Funding
This work was supported by grants from the National Natural Science Foundation of China (No. 81871555), the Department of Finance of Jilin Province (JLSCZD2019-006, 2018SCZWSZX-006, and JLSWSRCZX2021-028), and the Jilin Province Development and Reform Commission (ZKJCFGW2023038).
Conflict of interest
The authors declare that the research was conducted in the absence of any commercial or financial relationships that could be construed as a potential conflict of interest.
Publisher’s note
All claims expressed in this article are solely those of the authors and do not necessarily represent those of their affiliated organizations, or those of the publisher, the editors and the reviewers. Any product that may be evaluated in this article, or claim that may be made by its manufacturer, is not guaranteed or endorsed by the publisher.
References
An, N., Yang, J. X., Wang, H. Q., Sun, S. F., Wu, H., Li, L. S., et al. (2021). Mechanism of mesenchymal stem cells in spinal cord injury repair through macrophage polarization. Cell Biosci. 11:14. doi: 10.1186/s13578-021-00554-z
Bansal, H., Verma, P., Agrawal, A., Leon, J., Sundell, I. B., and Koka, P. S. (2016). Autologous bone marrow-derived stem cells in spinal cord injury. J. Stem Cells 11, 51–61.
Campos, H. C., Ribeiro, D. E., Hashiguchi, D., Hukuda, D. Y., Gimenes, C., Romariz, S. A. A., et al. (2022). Distinct effects of the hippocampal transplantation of neural and mesenchymal stem cells in a transgenic model of Alzheimer’s disease. Stem Cell Rev. Rep. 18, 781–791. doi: 10.1007/s12015-021-10321-9
Capizzi, A., Woo, J., and Verduzco-Gutierrez, M. (2020). Traumatic brain injury an overview of epidemiology, pathophysiology, and medical management. Med. Clin. North Am. 104, 213–238. doi: 10.1016/j.mcna.2019.11.001
Chen, K. L., Li, L., Li, C. M., Wang, Y. R., Yang, F. X., Kuang, M. Q., et al. (2019). SIRT7 regulates lipopolysaccharide-induced inflammatory injury by suppressing the NF-κB signaling pathway. Oxid. Med. Cell. Longev. 2019:3187972.
Chen, Y., Li, J., Ma, B., Li, N., Wang, S., Sun, Z., et al. (2020). MSC-derived exosomes promote recovery from traumatic brain injury via microglia/macrophages in rat. Aging 12, 18274–18296. doi: 10.18632/aging.103692
Chen, Y. F., Li, J., Ma, B. T., Li, N., Wang, S. H., Sun, Z., et al. (2020). MSC-derived exosomes promote recovery from traumatic brain injury via microglia/macrophages in rat. Aging 12, 18274–18296.
Cherry, J. D., Olschowka, J. A., and O’Banion, M. K. (2014). Neuroinflammation and M2 microglia: The good, the bad, and the inflamed. J. Neuroinflammation 11:98. doi: 10.1186/1742-2094-11-98
Corps, K. N., Roth, T. L., and McGavern, D. B. (2015). Inflammation and neuroprotection in traumatic brain injury. JAMA Neurol. 72, 355–362. doi: 10.1001/jamaneurol.2014.3558
Devanney, N. A., Stewart, A. N., and Gensel, J. C. (2020). Microglia and macrophage metabolism in CNS injury and disease: The role of immunometabolism in neurodegeneration and neurotrauma. Exp. Neurol. 329:113310. doi: 10.1016/j.expneurol.2020.113310
Dong, C., Chen, M., Cai, B., Zhang, C., Xiao, G., and Luo, W. (2022). Mesenchymal stem cell-derived exosomes improved cerebral infarction via transferring miR-23a-3p to activate microglia. Neuromol. Med. 24, 290–298. doi: 10.1007/s12017-021-08686-8
Duan, S., Wang, F., Cao, J., and Wang, C. (2020). Exosomes derived from microrna-146a-5p-enriched bone marrow mesenchymal stem cells alleviate intracerebral hemorrhage by inhibiting neuronal apoptosis and microglial M1 polarization. Drug Des. Dev. Ther. 14, 3143–3158. doi: 10.2147/dddt.S255628
Ellwanger, D. C., Wang, S., Brioschi, S., Shao, Z., Green, L., Case, R., et al. (2021). Prior activation state shapes the microglia response to antihuman TREM2 in a mouse model of Alzheimer’s disease. Proc. Natl. Acad. Sci. U.S.A. 118:e2017742118. doi: 10.1073/pnas.2017742118
Fan, B., Li, C., Szalad, A., Wang, L., Pan, W., Zhang, R., et al. (2020). Mesenchymal stromal cell-derived exosomes ameliorate peripheral neuropathy in a mouse model of diabetes. Diabetologia 63, 431–443. doi: 10.1007/s00125-019-05043-0
Fang, Y., and Hong, X. (2021). miR-124-3p inhibits microglial secondary inflammation after basal ganglia hemorrhage by targeting TRAF6 and repressing the activation of NLRP3 inflammasome. Front. Neurol. 12:653321. doi: 10.3389/fneur.2021.653321
Fenn, A. M., Gensel, J. C., Huang, Y., Popovich, P. G., Lifshitz, J., and Godbout, J. P. (2014). Immune activation promotes depression 1 month after diffuse brain injury: A role for primed microglia. Biol. Psychiatry 76, 575–584. doi: 10.1016/j.biopsych.2013.10.014
Ferguson, S. W., Wang, J., Lee, C. J., Liu, M., Neelamegham, S., Canty, J. M., et al. (2018). The microRNA regulatory landscape of MSC-derived exosomes: A systems view. Sci. Rep. 8:1419. doi: 10.1038/s41598-018-19581-x
Fernandes, T. L., Gomoll, A. H., Lattermann, C., Hernandez, A. J., Bueno, D. F., and Amano, M. T. (2020). Macrophage: A potential target on cartilage regeneration. Front. Immunol. 11:111. doi: 10.3389/fimmu.2020.00111
Galgano, M., Toshkezi, G., Qiu, X. C., Russell, T., Chin, L., and Zhao, L. R. (2017). Traumatic brain injury: Current treatment strategies and future endeavors. Cell Transplant. 26, 1118–1130. doi: 10.1177/0963689717714102
Gensel, J. C., and Zhang, B. (2015). Macrophage activation and its role in repair and pathology after spinal cord injury. Brain Res. 1619, 1–11. doi: 10.1016/j.brainres.2014.12.045
Guo, S. R., Wang, H., and Yin, Y. F. (2022). Microglia polarization from M1 to M2 in neurodegenerative diseases. Front. Aging Neurosci. 14:815347. doi: 10.3389/fnagi.2022.815347
Han, M., Cao, Y., Guo, X., Chu, X., Li, T., Xue, H., et al. (2021). Mesenchymal stem cell-derived extracellular vesicles promote microglial M2 polarization after subarachnoid hemorrhage in rats and involve the AMPK/NF-kappa B signaling pathway. Biomed. Pharmacother. 133:111048. doi: 10.1016/j.biopha.2020.111048
Harrell, C. R., Markovic, B. S., Fellabaum, C., Arsenijevic, A., and Volarevic, V. (2019). Mesenchymal stem cell-based therapy of osteoarthritis: Current knowledge and future perspectives. Biomed. Pharmacother. 109, 2318–2326. doi: 10.1016/j.biopha.2018.11.099
Hu, X., Li, P., Guo, Y., Wang, H., Leak, R. K., Chen, S., et al. (2012). Microglia/macrophage polarization dynamics reveal novel mechanism of injury expansion after focal cerebral ischemia. Stroke 43, 3063–3070. doi: 10.1161/strokeaha.112.659656
Huang, X., Fei, G.-Q., Liu, W.-J., Ding, J., Wang, Y., Wang, H., et al. (2020). Adipose-derived mesenchymal stem cells protect against CMS-induced depression-like behaviors in mice via regulating the Nrf2/HO-1 and TLR4/NF-kappa B signaling pathways. Acta Pharmacol. Sin. 41, 612–619. doi: 10.1038/s41401-019-0317-6
Jarocha, D., Milczarek, O., Wedrychowicz, A., Kwiatkowski, S., and Majka, M. (2015). Continuous improvement after multiple mesenchymal stem cell transplantations in a patient with complete spinal cord injury. Cell Transplant. 24, 661–672. doi: 10.3727/096368915x687796
Jiang, M., Wang, H., Zin, M., Yang, X., Ji, H., Jiang, Y., et al. (2018). Exosomes from MiR-30d-5p-ADSCs reverse acute ischemic stroke-induced, autophagy-mediated brain injury by promoting M2 microglial/macrophage polarization. Cell. Physiol. Biochem. 47, 864–878. doi: 10.1159/000490078and
Jurga, A. M., Paleczna, M., and Kuter, K. Z. (2020). Overview of general and discriminating markers of differential microglia phenotypes. Front. Cell. Neurosci. 14:198. doi: 10.3389/fncel.2020.00198
Kalluri, R., and LeBleu, V. S. (2020). The biology, function, and biomedical applications of exosomes. Science 367:eaau6977. doi: 10.1126/science.aau6977
Kigerl, K. A., Gensel, J. C., Ankeny, D. P., Alexander, J. K., Donnelly, D. J., and Popovich, P. G. (2009). Identification of two distinct macrophage subsets with divergent effects causing either neurotoxicity or regeneration in the injured mouse spinal cord. J. Neurosci. 29, 13435–13444. doi: 10.1523/jneurosci.3257-09.2009
Li, J., Wang, H., Du, C., Jin, X., Geng, Y., Han, B., et al. (2020). hUC-MSCs ameliorated CUMS-induced depression by modulating complement C3 signaling-mediated microglial polarization during astrocyte-microglia crosstalk. Brain Res. Bull. 163, 109–119. doi: 10.1016/j.brainresbull.2020.07.004
Li, J. R., Zhang, D., Guo, S., Zhao, C. B., Wang, L. Y., Ma, S. S., et al. (2021). Dual-enzymatically cross-linked gelatin hydrogel promotes neural differentiation and neurotrophin secretion of bone marrow-derived mesenchymal stem cells for treatment of moderate traumatic brain injury. Int. J. Biol. Macromol. 187, 200–213. doi: 10.1016/j.ijbiomac.2021.07.111
Li, M., Hou, Q., Zhong, L., Zhao, Y., and Fu, X. (2021). Macrophage related chronic inflammation in non-healing wounds. Front. Immunol. 12:681710. doi: 10.3389/fimmu.2021.681710
Li, Y., Yang, Y. Y., Ren, J. L., Xu, F., Chen, F. M., and Li, A. (2017). Exosomes secreted by stem cells from human exfoliated deciduous teeth contribute to functional recovery after traumatic brain injury by shifting microglia M1/M2 polarization in rats. Stem Cell Res. Ther. 8:198. doi: 10.1186/s13287-017-0648-5
Li, Z., Liu, F., He, X., Yang, X., Shan, F., and Feng, J. (2019). Exosomes derived from mesenchymal stem cells attenuate inflammation and demyelination of the central nervous system in EAE rats by regulating the polarization of microglia. Int. Immunopharmacol. 67, 268–280. doi: 10.1016/j.intimp.2018.12.001
Liu, W., Rong, Y., Wang, J., Zhou, Z., Ge, X., Ji, C., et al. (2020). Exosome-shuttled miR-216a-5p from hypoxic preconditioned mesenchymal stem cells repair traumatic spinal cord injury by shifting microglial M1/M2 polarization. J. Neuroinflammation 17:47. doi: 10.1186/s12974-020-1726-7
Liu, W., Tang, P., Wang, J., Ye, W., Ge, X., Rong, Y., et al. (2021). Extracellular vesicles derived from melatonin-preconditioned mesenchymal stem cells containing USP29 repair traumatic spinal cord injury by stabilizing NRF2. J. Pineal Res. 71:e12769. doi: 10.1111/jpi.12769
Liu, X. L., Ouyang, F. B., Hu, L. T., Sun, P., Yang, J., Sun, Y. J., et al. (2022). Mesenchymal stem cells improve cognitive impairment and reduce a beta deposition via promoting AQP4 polarity and relieving neuroinflammation in rats with chronic hypertension-induced cerebral small-vessel disease. Front. Aging Neurosci. 14:883503. doi: 10.3389/fnagi.2022.883503
Liu, Y., Zeng, R., Wang, Y., Huang, W., Hu, B., Zhu, G., et al. (2019). Mesenchymal stem cells enhance microglia M2 polarization and attenuate neuroinflammation through TSG-6. Brain Res. 1724:146422. doi: 10.1016/j.brainres.2019.146422
Liu, Y., Zhang, R., Yan, K., Chen, F. F., Huang, W. Y., Lv, B. K., et al. (2014). Mesenchymal stem cells inhibit lipopolysaccharide-induced inflammatory responses of BV2 microglial cells through TSG-6. J. Neuroinflammation 11:135. doi: 10.1186/1742-2094-11-135
Mätlik, K., Anttila, J. E., Kuan-Yin, T., Smolander, O. P., Pakarinen, E., Lehtonen, L., et al. (2018). Poststroke delivery of MANF promotes functional recovery in rats. Sci. Adv. 4:eaa8957. doi: 10.1126/sciadv.aap8957
McKee, C. A., and Lukens, J. R. (2016). Emerging roles for the immune system in traumatic brain injury. Front. Immunol. 7:556. doi: 10.3389/fimmu.2016.00556
Nam, Y., Yoon, D., Hong, J., Kim, M. S., Lee, T. Y., Kim, K. S., et al. (2020). Therapeutic effects of human mesenchymal stem cells in a mouse model of cerebellar ataxia with neuroinflammation. J. Clin. Med. 9:3654. doi: 10.3390/jcm9113654
Ni, H. Q., Yang, S., Siaw-Debrah, F., Hu, J. N., Wu, K., He, Z. B., et al. (2019). Exosomes derived from bone mesenchymal stem cells ameliorate early inflammatory responses following traumatic brain injury. Front. Neurosci. 13:14. doi: 10.3389/fnins.2019.00014
Ning, G. Z., Song, W. Y., Xu, H., Zhu, R. S., Wu, Q. L., Wu, Y., et al. (2019). Bone marrow mesenchymal stem cells stimulated with low-intensity pulsed ultrasound: Better choice of transplantation treatment for spinal cord injury: Treatment for SCI by LIPUS-BMSCs transplantation. CNS Neurosci. Ther. 25, 496–508. doi: 10.1111/cns.13071
Paolicelli, R. C., Sierra, A., Stevens, B., Tremblay, M. E., Aguzzi, A., Ajami, B., et al. (2022). Microglia states and nomenclature: A field at its crossroads. Neuron 110, 3458–3483.
Qian, Y., Li, Q., Chen, L., Sun, J., Cao, K., Mei, Z., et al. (2022). Mesenchymal stem cell-derived extracellular vesicles alleviate M1 microglial activation in brain injury of mice with subarachnoid hemorrhage via microRNA-140-5p delivery. Int. J. Neuropsychopharmacol. 25, 328–338. doi: 10.1093/ijnp/pyab096
Ramer, L. M., Ramer, M. S., and Bradbury, E. J. (2014). Restoring function after spinal cord injury: Towards clinical translation of experimental strategies. Lancet Neurol. 13, 1241–1256. doi: 10.1016/s1474-4422(14)70144-9
Ramos-Cejudo, J., Wisniewski, T., Marmar, C., Zetterberg, H., Blennow, K., de Leon, M. J., et al. (2018). Traumatic BRAIN INJURY and Alzheimer’s DISEase: The CEREBROVASCULAR LINK. EBioMedicine 28, 21–30. doi: 10.1016/j.ebiom.2018.01.021
Sasaki, F., and Yokomizo, T. (2019). The leukotriene receptors as therapeutic targets of inflammatory diseases. Int. Immunol. 31, 607–615. doi: 10.1093/intimm/dxz044
Seo, Y., Ahn, J.-S., Shin, Y. Y., Oh, S.-J., Song, M.-H., Kang, M.-J., et al. (2022). Mesenchymal stem cells target microglia via galectin-1 production to rescue aged mice from olfactory dysfunction. Biomed. Pharmacother. 153:113347. doi: 10.1016/j.biopha.2022.113347
Shao, M., Jin, M., Xu, S., Zheng, C., Zhu, W., Ma, X., et al. (2020). Exosomes from long noncoding RNA-Gm37494-ADSCs repair spinal cord injury via shifting microglial M1/M2 polarization. Inflammation 43, 1536–1547. doi: 10.1007/s10753-020-01230-z
Shu, J., Jiang, L., Wang, M., Wang, R., Wang, X., Gao, C., et al. (2022). Human bone marrow mesenchymal stem cells-derived exosomes protect against nerve injury via regulating immune microenvironment in neonatal hypoxic-ischemic brain damage model. Immunobiology 227:152178. doi: 10.1016/j.imbio.2022.152178
Sugiyama, Y., Sato, Y., Kitase, Y., Suzuki, T., Kondo, T., Mikrogeorgiou, A., et al. (2018). Intravenous administration of bone marrow-derived mesenchymal stem cell, but not adipose tissue-derived stem cell, ameliorated the neonatal hypoxic-ischemic brain injury by changing cerebral inflammatory state in rat. Front. Neurol. 9:757. doi: 10.3389/fneur.2018.00757
Taj, S. H., Le Blon, D., Hoornaert, C., Daans, J., Quarta, A., Praet, J., et al. (2018). Targeted intracerebral delivery of the anti-inflammatory cytokine IL13 promotes alternative activation of both microglia and macrophages after stroke. J. Neuroinflammation 15:174. doi: 10.1186/s12974-018-1212-7
Teixeira, F. G., Carvalho, M. M., Panchalingam, K. M., Rodrigues, A. J., Mendes-Pinheiro, B., Anjo, S., et al. (2017). Impact of the secretome of human mesenchymal stem cells on brain structure and animal behavior in a rat model of Parkinson’s disease. Stem Cells Transl. Med. 6, 634–646. doi: 10.5966/sctm.2016-0071
Teng, S.-W., Sung, H.-Y., Wen, Y.-C., Chen, S.-Y., Lovel, R., Chang, W.-Y., et al. (2021). Potential surrogate quantitative immunomodulatory potency assay for monitoring human umbilical cord-derived mesenchymal stem cells production. Cell Biol. Int. 45, 1072–1081. doi: 10.1002/cbin.11553
Wang, J., He, W. B., and Zhang, J. L. (2023). A richer and more diverse future for microglia phenotypes. Heliyon 9:e14713. doi: 10.1016/j.heliyon.2023.e14713
Wang, L. T., Liu, K. J., Sytwu, H. K., Yen, M. L., and Yen, B. L. (2021). Advances in mesenchymal stem cell therapy for immune and inflammatory diseases: Use of cell-free products and human pluripotent stem cell-derived mesenchymal stem cells. Stem Cells Transl. Med. 10, 1288–1303. doi: 10.1002/sctm.21-0021
Wen, L., Wang, Y. D., Shen, D. F., Zheng, P. D., Tu, M. D., You, W. D., et al. (2022). Exosomes derived from bone marrow mesenchymal stem cells inhibit neuroinflammation after traumatic brain injury. Neural Regen. Res. 17, 2717–2724. doi: 10.4103/1673-5374.339489
Wiles, M. D. (2022). Management of traumatic brain injury: A narrative review of current evidence. Anaesthesia 77, 102–112. doi: 10.1111/anae.15608
Woodcock, T., and Morganti-Kossmann, M. C. (2013). The role of markers of inflammation in traumatic brain injury. Front. Neurol. 4:18. doi: 10.3389/fneur.2013.00018
Xin, D.-Q., Zhao, Y.-J., Li, T.-T., Ke, H.-F., Gai, C.-C., Guo, X.-F., et al. (2022). The delivery of miR-21a-5p by extracellular vesicles induces microglial polarization via the STAT3 pathway following hypoxia-ischemia in neonatal mice. Neural Regen. Res. 17, 2238–2246. doi: 10.4103/1673-5374.336871
Xiong, Y., Mahmood, A., and Chopp, M. (2013). Animal models of traumatic brain injury. Nat. Rev. Neurosci. 14, 128–142. doi: 10.1038/nrn3407
Xiong, Y., Mahmood, A., and Chopp, M. (2018). Current understanding of neuroinflammation after traumatic brain injury and cell-based therapeutic opportunities. Chin. J. Traumatol. 21, 137–151. doi: 10.1016/j.cjtee.2018.02.003
Xu, S., Di, Z., He, Y., Wang, R., Ma, Y., Sun, R., et al. (2019). Mesencephalic astrocyte-derived neurotrophic factor (MANF) protects against Aβ toxicity via attenuating Aβ-induced endoplasmic reticulum stress. J. Neuroinflammation 16:35. doi: 10.1186/s12974-019-1429-0
Yang, F., Li, W.-B., Qu, Y.-W., Gao, J.-X., Tang, Y.-S., Wang, D.-J., et al. (2020). Bone marrow mesenchymal stem cells induce M2 microglia polarization through PDGF-AA/MANF signaling. World J. Stem Cells 12, 633–658. doi: 10.4252/wjsc.v12.i7.633
Yang, H., Tu, Z., Yang, D., Hu, M., Zhou, L., Li, Q., et al. (2022). Exosomes from hypoxic pre-treated ADSCs attenuate acute ischemic stroke-induced brain injury via delivery of circ-Rps5 and promote M2 microglia/macrophage polarization. Neurosci. Lett. 769:136389. doi: 10.1016/j.neulet.2021.136389
Yao, Q., Liu, J., Zhang, Z., Li, F., Zhang, C., Lai, B., et al. (2018). Peroxisome proliferator-activated receptor γ (PPARγ) induces the gene expression of integrin α(V)β(5) to promote macrophage M2 polarization. J. Biol. Chem. 293, 16572–16582. doi: 10.1074/jbc.RA118.003161
Yin, X. Y., Wang, C. C., Du, P., Wang, X. S., Lu, Y. C., Sun, Y. W., et al. (2023). Muse cells decrease the neuroinflammatory response by modulating the proportion of M1 and M2 microglia in vitro. Neural Regen. Res. 18, 213–218. doi: 10.4103/1673-5374.343885
Yu, H., Xu, Z., Qu, G., Wang, H., Lin, L., Li, X., et al. (2021). Hypoxic preconditioning enhances the efficacy of mesenchymal stem cells-derived conditioned medium in switching microglia toward anti-inflammatory polarization in ischemia/reperfusion. Cell. Mol. Neurobiol. 41, 505–524. doi: 10.1007/s10571-020-00868-5
Yu, Z., Wen, Y., Jiang, N., Li, Z., Guan, J., Zhang, Y., et al. (2022). TNF-alpha stimulation enhances the neuroprotective effects of gingival MSCs derived exosomes in retinal ischemia-reperfusion injury via the MEG3/miR-21a-5p axis. Biomaterials 284:121484. doi: 10.1016/j.biomaterials.2022.121484
Yunna, C., Mengru, H., Lei, W., and Weidong, C. (2020). Macrophage M1/M2 polarization. Eur. J. Pharmacol. 877:173090. doi: 10.1016/j.ejphar.2020.173090
Zanier, E. R., Pischiutta, F., Riganti, L., Marchesi, F., Turola, E., Fumagalli, S., et al. (2014). Bone marrow mesenchymal stromal cells drive protective M2 microglia polarization after brain trauma. Neurotherapeutics 11, 679–695. doi: 10.1007/s13311-014-0277-y
Zhang, J., Buller, B. A., Zhang, Z. G., Zhang, Y., Lu, M., Rosene, D. L., et al. (2022). Exosomes derived from bone marrow mesenchymal stromal cells promote remyelination and reduce neuroinflammation in the demyelinating central nervous system. Exp. Neurol. 347:113895. doi: 10.1016/j.expneurol.2021.113895
Zhang, L., Guo, K., Yin, S., Peng, J., Pang, J., Ma, N., et al. (2020). RNA-seq reveals underlying transcriptomic mechanisms of bone marrow-derived mesenchymal stem cells in the regulation of microglia-mediated neuroinflammation after subarachnoid hemorrhage. Stem Cells Dev. 29, 562–573. doi: 10.1089/scd.2019.0216
Zhang, R., Li, C., Du, R., Yuan, Y., Zhao, B., Zhang, Y., et al. (2022). Mesenchymal stem cells: As a multi-target cell therapy for clearing beta-amyloid deposition in Alzheimer’s disease. Biocell 46, 583–594. doi: 10.32604/biocell.2022.017248
Zhao, Y., Gan, Y., Xu, G., Hua, K., and Liu, D. (2020). Exosomes from MSCs overexpressing microRNA-223-3p attenuate cerebral ischemia through inhibiting microglial M1 polarization mediated inflammation. Life Sci. 260:118403. doi: 10.1016/j.lfs.2020.118403
Zhao, Y. M., Gan, Y. X., Xu, G. W., Yin, G. L., and Liu, D. D. (2020). MSCs-derived exosomes attenuate acute brain injury and inhibit microglial inflammation by reversing CysLT2R-ERK1/2 mediated microglia M1 polarization. Neurochem. Res. 45, 1180–1190. doi: 10.1007/s11064-020-02998-0
Zhong, Z., Chen, A., Fa, Z., Ding, Z., Xiao, L., Wu, G., et al. (2020a). Bone marrow mesenchymal stem cells upregulate PI3K/AKT pathway and down-regulate NF-kappa B pathway by secreting glial cell-derived neurotrophic factors to regulate microglial polarization and alleviate deafferentation pain in rats. Neurobiol. Dis. 143:104945. doi: 10.1016/j.nbd.2020.104945
Zhong, Z., Chen, A., Fa, Z., Ding, Z., Xie, J., Sun, Y., et al. (2020b). Adipose-derived stem cells modulate BV2 microglial M1/M2 polarization by producing glial cell-derived neurotrophic factor. Stem Cells Dev. 29, 714–727. doi: 10.1089/scd.2019.0235
Keywords: mesenchymal stem cells, microglia, states, different sources, effect
Citation: Xin Q, Zhu W, He C, Liu T and Wang H (2023) The effect of different sources of mesenchymal stem cells on microglia states. Front. Aging Neurosci. 15:1237532. doi: 10.3389/fnagi.2023.1237532
Received: 09 June 2023; Accepted: 09 August 2023;
Published: 24 August 2023.
Edited by:
Jagdeep K. Sandhu, National Research Council Canada (NRC), CanadaReviewed by:
Xiuli Yang, Johns Hopkins Medicine, United StatesCopyright © 2023 Xin, Zhu, He, Liu and Wang. This is an open-access article distributed under the terms of the Creative Commons Attribution License (CC BY). The use, distribution or reproduction in other forums is permitted, provided the original author(s) and the copyright owner(s) are credited and that the original publication in this journal is cited, in accordance with accepted academic practice. No use, distribution or reproduction is permitted which does not comply with these terms.
*Correspondence: Haifeng Wang, hfwang@jlu.edu.cn